- Division of Comparative Medicine, Massachusetts Institute of Technology, Cambridge, MA, United States
Non-human primates (NHPs) for biomedical research are commonly infected with Shigella spp. that can cause acute dysentery or chronic episodic diarrhea. These animals are often prophylactically and clinically treated with quinolone antibiotics to eradicate these possible infections. However, chromosomally- and plasmid-mediated antibiotic resistance has become an emerging concern for species in the family Enterobacteriaceae. In this study, five individual isolates of multi-drug resistant Shigella flexneri were isolated from the feces of three macaques. Antibiotic susceptibility testing confirmed resistance or decreased susceptibility to ampicillin, amoxicillin-clavulanic acid, cephalosporins, gentamicin, tetracycline, ciprofloxacin, enrofloxacin, levofloxacin, and nalidixic acid. S. flexneri isolates were susceptible to trimethoprim-sulfamethoxazole, and this drug was used to eradicate infection in two of the macaques. Plasmid DNA from all isolates was positive for the plasmid-encoded quinolone resistance gene qnrS, but not qnrA and qnrB. Conjugation and transformation of plasmid DNA from several S. flexneri isolates into antibiotic-susceptible Escherichia coli strains conferred the recipients with resistance or decreased susceptibility to quinolones and beta-lactams. Genome sequencing of two representative S. flexneri isolates identified the qnrS gene on a plasmid-like contig. These contigs showed >99% homology to plasmid sequences previously characterized from quinolone-resistant Shigella flexneri 2a and Salmonella enterica strains. Other antibiotic resistance genes and virulence factor genes were also identified in chromosome and plasmid sequences in these genomes. The findings from this study indicate macaques harbor pathogenic S. flexneri strains with chromosomally- and plasmid-encoded antibiotic resistance genes. To our knowledge, this is the first report of plasmid-mediated quinolone resistance in S. flexneri isolated from NHPs and warrants isolation and antibiotic testing of enteric pathogens before treating macaques with quinolones prophylactically or therapeutically.
Introduction
Shigella spp., particularly Shigella flexneri, are common enteric pathogens isolated from non-human primates (NHPs), including macaques, that can cause acute dysentery and are associated with chronic episodic diarrhea. In addition to the lower bowel, Shigella spp. can also colonize the inflamed gingival mucosa (McClure et al., 1976; Armitage et al., 1982). Clinically, animals may not appear ill, but Shigella spp. can be cultured from normally formed, but blood-streaked feces. These animals represent Shigella spp. reservoirs capable of transmitting the pathogen to other colony-maintained NHPs and pose important zoonotic risks to research, veterinary, and zoo personnel (Fox, 1975; Tribe and Fleming, 1983; Kennedy et al., 1993).
Because of the high incidence of diarrhea in newly imported NHPs, antibiotics are often given prophylactically to reduce its clinical severity. Enrofloxacin, a fluoroquinolone antibiotic, is commonly prescribed because of its efficacy in treating gram-negative pathogens and its convenience of a once-daily parenteral treatment. In addition, enrofloxacin has also been used to successfully eradicate Shigella spp. from a variety of NHP species housed in a laboratory and zoological setting (Line et al., 1992; Banish et al., 1993). However, widespread and prophylactic use of enrofloxacin and other antibiotics act as selective pressures for the emergence of antibiotic resistant organisms.
Quinolone resistance is typically chromosomally-mediated; however, an increasing finding among species in the family Enterobacteriaceae is plasmid-mediated quinolone resistance due to qnr genes. While several homologs of qnr genes have been discovered, the best characterized are the qnrA, qnrB, and qnrS genes (Strahilevitz et al., 2009; Redgrave et al., 2014). These genes encode a ~218 amino acid protein that protects bacterial DNA gyrase and topoisomerase from quinolone and fluoroquinolone inhibition, thus resulting in low-level quinolone resistance (Tran and Jacoby, 2002; Tran et al., 2005a,b; Redgrave et al., 2014). Since first being reported in 1998 from a clinical isolate of Klebsiella pneumoniae (Martinez-Martinez et al., 1998), plasmid-mediated quinolone resistance has been reported in other Enterobacteriaceae species throughout the United States (Wang et al., 2004) and elsewhere in the world (Hata et al., 2005; Mammeri et al., 2005; Strahilevitz et al., 2009; Karczmarczyk et al., 2012).
Plasmids encoding qnr often harbor other antibiotic resistance genes and can be transferred to other bacterial species by conjugation (Martinez-Martinez et al., 1998). For example, the ~47 kb, conjugative plasmid pAH0376 was discovered in S. flexneri 2b isolates that caused a food poisoning outbreak and harbors both a TEM-1 β-lactamase gene and qnrS gene (Hata et al., 2005). Thus, plasmid-mediated quinolone resistance represents a significant public health concern because they act as vectors that propagate multi-drug antibiotic resistant pathogens.
Given the frequent use of quinolones and potential for zoonotic transmission, the purpose of this report is to describe the isolation of plasmid-mediated multiple-antibiotic resistant S. flexneri strains recovered from three colony-maintained macaques, and the successful eradication of these drug-resistant S. flexneri isolates.
Materials and Methods
Case History
Macaques (Macaca mulatta), obtained from a United States-based vendor in 2005, underwent physical examination and routine diagnostic evaluations during quarantine and thereafter at quarterly intervals. Macaques were routinely pair-housed and maintained in an AAALAC International-accredited animal facility. They were fed specified amounts of primate chow (Purina Lab Diet® 5038, St. Louis, MO) twice daily and provided water ad libitum. Housing conditions were maintained between 20 and 22°C, 30–70% humidity, 10–15 non-recirculated air changes per hour, and a light cycle of 12 h light, 12 h of dark per day.
During a quarterly physical and diagnostic evaluation, macaque 05-18 presented with fresh blood in its feces. Fecal samples were collected directly from the rectum and submitted for culture and antibiotic sensitivity. Shortly after the initial fecal sample was collected, macaque 05-18 and its cagemate underwent antibiotic treatment with enrofloxacin (5 mg/kg IM every 24 h for 10 days) following research-related surgical procedures. S. flexneri variant Y (strain 06-2384) was cultured from the original submission and found sensitive to trimethoprim-sulfamethoxazole, but resistant to ampicillin, amoxicillin-clavulanic acid, cephalothin, gentamicin, and enrofloxacin using Kirby-Bauer antibiotic testing. The two clinically normal monkeys were placed in quarantine together and a set of rectal and gingival swabs were submitted for culture. The second culture sample, as well as fecal samples collected twice more at 2-week intervals, were negative for Shigella spp. Following treatment with enrofloxacin, multiple follow-up fecal cultures were negative for Shigella spp.
Fecal samples were collected from all macaques (N = 24) and submitted for culture and antibiotic susceptibility testing during the same time interval the shigella-positive macaque, 05-18, was found. Two additional macaques, 05-15 and 05-16, housed in the adjacent cage to macaque 05-18, had fecal cultures positive for S. flexneri type 5b, designated 06-2835 and 06-2836, respectively. This serotype also showed multiple-antibiotic resistance, with intermediate sensitivity to enrofloxacin and sensitivity to trimethoprim-sulfamethoxazole. The second pair was moved to quarantine and treated with enrofloxacin (5 mg/kg IM every 24 h for 10 days). However, follow-up fecal cultures from the cage pan were still positive for S. flexneri type 5b, designated strain 06-3102. Subsequently, the pair was treated with trimethoprim-sulfadiazine (30 mg/kg SQ every 24 h for 10 days); all subsequent cultures were negative for Shigella spp.
Shigella Isolation
Cage pan feces and rectal swabs collected from macaques were swabbed onto XLD (xylose, lysine, deoxycholate) and HE (hektoen-enteric) agar media, and the samples were then placed into selenite broth (Remel, Inc., Lenexa, KS.). All media were incubated at 37°C for 18 to 24 h, after which the selenite broth was sub-cultured onto the same media and re-incubated. Isolates that were transparent on the XLD and HE agars were sub-cultured on BAP (Trypticase Soy Agar with 5% Sheep Blood; Remel) and identified as Shigella spp. by API 20E (bioMerieux, Inc., Hazelwood, MO). Isolates were submitted to the Texas Department of State Health Services lab for serotyping.
Antimicrobial Agents
Antibiotics used for broth microdilution assays (ampicillin, azithromycin, cefazolin, cefoxitin, ceftazidime, ceftriaxone, cephalothin, ciprofloxacin, clarithromycin, enrofloxacin, gentamicin, imipenem, levofloxacin, nalidixic acid, and tetracycline powders) were purchased from the Sigma Chemical Company (St. Louis, MO). Amoxicillin-clavulanic acid and trimethoprim-sulfamethoxazole were obtained as E-test strips from bioMerieux (Durham, NC.). Disks of ampicillin (10 μg), cephalothin (30 μg), enrofloxacin (5 μg), nalidixic acid (30 μg), tetracycline (30 μg), amoxicillin-clavulanic acid (30 μg), and trimethoprim-sulfamethoxazole (25 μg) were obtained from Remel (Lenexa, KS.).
Antimicrobial Susceptibility Testing
Antimicrobial susceptibility tests were initially performed by disk diffusion in accordance with the Clinical Laboratory Standards Institute (2010). In addition, to determine the level of sensitivity, antibiotic mean inhibitory concentrations (MICs) were determined by the broth microdilution method using 2-fold serial dilutions, as recommended by CLSI (Clinical Laboratory Standards Institute, 2012, 2014). MIC is defined as the lowest concentration of an antimicrobial agent that inhibits visible growth. Escherichia coli (ATCC25922) was used as the standard reference strain. Experiments were performed in triplicate from two or more independent experiments.
DNA Extraction
Whole DNA (includes both chromosomal and plasmid DNA) from the five S. flexneri isolates was extracted using the High Pure PCR Template Preparation Kit (Roche Molecular Biochemicals, Indianapolis, IN). Plasmid DNA was extracted using the QIAprep Spin Miniprep Kit (QIAGEN Inc. Valencia, CA).
Screening of qnr Antimicrobial-Resistance Genes
Screening for the qnrA, qnrB, and qnrS genes was performed on whole and plasmid DNA using the primers and PCR conditions described previously (Robicsek et al., 2006). PCR products were concentrated and purified with the QIAquick PCR purification kit (Qiagen, Valencia, CA) for sequencing with an ABI Prism cycle sequencing kit (BigDye Terminator cycle sequencing kit) on an ABI 3100 genetic analyzer (Applied Biosystems, Foster City, CA). Sequences were analyzed by BLAST hosted by the National Center for Biotechnology Information (NCBI) to identify homologous genes and calculate percent identity.
Conjugation and Transformation Assays
Conjugation experiments were performed using the S. flexneri isolates as donors and E. coli J53 Azr as the recipients (Wang et al., 2004). Cultures of donor and recipient cells were grown in 3 mL of fresh Luria Bertani (LB) broth (BBL®) at 37°C with shaking for 3 h. The conjugation assay was then performed in 3 mL of fresh LB broth using the donor and recipient cells in logarithmic phase (0.5 mL each) and incubated at 37°C for 3 h without shaking. Transconjugants were selected on trypticase soy agar plates (TSA; BBL®) containing sodium azide (100 μg/mL: Sigma-Aldrich Corporation, St. Louis, Mo.) for counter-selection, and ampicillin (50 μg/mL; Sigma-Aldrich Corp.) for selection of plasmid-encoded resistance (Fox, 1975; Mammeri et al., 2005; Cattoir et al., 2007). Colonies were also plated on TSA plates containing sodium azide (100 μg/mL) and nalidixic acid (16 μg/mL). The transconjugants were plated onto MacConkey agar plates, and their identities were reconfirmed as E. coli using with API 20E.
For transformation experiments, 5 μL of plasmid DNA from four donor strains was heat shocked at 42°C for 30 s into chemically component E. coli Top10 cells. The cells were then incubated in 250 μL of S.O.C. (super optimal broth with catabolic repressor) for 1 h at 37°C, followed by overnight growth on LB plates supplemented with ampicillin (50 μg/mL; Sigma-Aldrich Corp.) for selection of antibiotic-resistant transformants. Colonies were screened by PCR for the qnrS gene. MICs for the donors, recipient, transconjugants, and transformants were measured as described above.
Whole Genome Sequencing of Representative S. flexneri Isolates
Whole DNA was extracted from two representative S. flexneri isolates, 06-2384 and 06-3102, using the High Pure PCR Template Preparation Kit (Roche Molecular Biochemicals, Indianapolis, IN). These isolates were chosen in order to represent all infected animals (06-2384 cultured from monkey 05-18; 06-3102 cultured from co-housed monkeys 05-15 and 05-16) as well as the potential genetic diversity of both serotypes (variant Y and 5b, corresponding to 06-2384 and 06-3102, respectively). DNA was then prepared into libraries using the SMRTbell Template Prep Kit 1.0 and the DNA/Polymerase Binding Kit P6 v2 (Pacific Biosciences, Menlo Park, CA). DNA libraries were size-selected for fragments ≥~5kb and then were sequenced on a single SMRT cell per genome using a Pacific Biosciences RSII sequencer at the University of Massachusetts Deep Sequencing Core Facility (Worchester, MA). Sequencing reads were quality filtered and trimmed for de novo assembly using the Hierarchical Genome Assembly Process (HGAP 3.0) workflow hosted on the SMRT Portal 2.3. Resulting contigs were annotated using RASTtk (Aziz et al., 2008) hosted by Pathosystems Resource Integration Center (PATRIC) (Wattam et al., 2014, 2017). VirulenceFinder 1.5 (Joensen et al., 2014), ResFinder 2.1 (Zankari et al., 2012), and PlasmidFinder 1.3 (Carattoli et al., 2014) were used to identify virulence factors, antibiotic resistance genes, and plasmids, respectively. In silico multi-locus sequencing type (MLST) was determined using MLST-1.6 (Larsen et al., 2012) against the E. coli #1 allelic profile database. Average nucleotide identity (ANI) was calculated with OrthoANIu (Lee et al., 2016), and digital DNA-DNA hybridization (dDDH) was calculated with Genome-to-Genome Distance Calculator (GGDC) 2.1 (Auch et al., 2010). Graphical circular maps of chromosome and plasmid sequences were generated using the CGView Server (Grant and Stothard, 2008).
Results
Shigella spp. Identification
S. flexneri isolates were identified using selective agar and API 20E strips and were also serotyped by the Texas Department of State Health Services. S. flexneri variant Y strain 06-2384 was isolated in the index case (i.e., monkey 05-18). S. flexneri type 5b strains 06-2835 R1 and 06-2835 R2, which had different colony morphologies and API codes, were isolated from monkey 05-15. Strain 06-2836 was cultured from monkey 05-16. Following unsuccessful enrofloxacin treatment of paired monkeys 05-15 and 05-16, feces were collected from the cage pan and strain 06-3102 was cultured. These S. flexneri isolates were then analyzed for antibiotic susceptibility, the presence of qnr genes by PCR, and the ability to transfer plasmid-encoded antibiotic resistance genes to E. coli recipients by conjugation and transformation.
qnrS1 Gene Detected in S. flexneri Strains Isolated From Macaques
PCR detected qnrS, but not qnrA or qnrB, in both whole and plasmid DNA from all five S. flexneri isolates (Supplementary Figure 1). BLAST analysis of three representative qnrS PCR product sequences against the nr/nt database determined they were 100% identical to the plasmid-encoded qnrS1 genes found in other Enterobacteriaceae species, including pAH0376 from Shigella flexneri 2b and pVQS1 from Salmonella enterica, indicating these novel S. flexneri strains harbor the qnrS1 variant.
Antimicrobial Resistance Transferrable by Plasmid Conjugation and Transformation
Antimicrobial susceptibility testing of four S. flexneri isolates by microdilution indicated multi-drug resistance to ampicillin, cephalothin, ceftazidime, ceftriaxone, cefazolin, gentamicin (except strain 06-2835), nalidixic acid, and tetracycline and decreased susceptibility to amoxicillin-clavulanic acid, ciprofloxacin, enrofloxacin, and levofloxacin compared to the E. coli control strain ATCC25922 (Table 1). The S. flexneri isolates were in general susceptible to azithromycin, imipenem, cefoxitin, trimethoprim-sulfamethoxazole (Table 1).
Plasmid DNA from these S. flexneri isolates were successfully conjugated and transformed into the E. coli recipient strains J53 Azr and Top10, respectively. Plasmid DNA isolated from all the transconjugants and transformants were PCR-positive for the qnrS gene (Supplementary Figure 2). Likewise, plasmid profiles were similar between the donor S. flexneri isolates and the E. coli transconjugants and transformants, indicating successful transfer of plasmid DNA to the recipients (Figure 1). The S. flexneri isolates have several bands in the plasmid profile, indicating they may harbor multiple different plasmids.
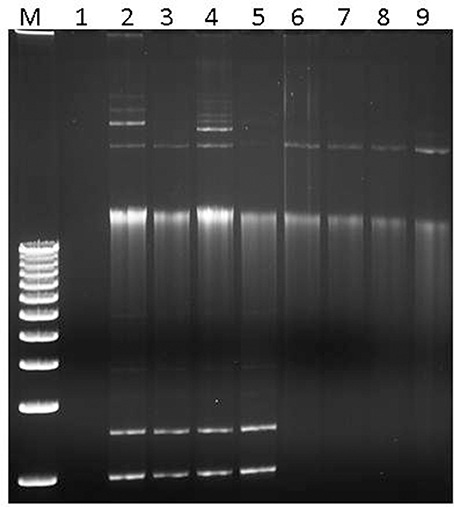
Figure 1. Plasmid separation profiles of the donor S. flexneri isolates, naïve E. coli recipient strain, and E. coli transformants. Lane 1: naïve E. coli Top10 recipient. Lanes 2–5: S. flexneri isolates 06-3102, 06-2384, 06-2835, 06-2836. Lanes 6–9: E. coli transformants t-06-3102, t-06-2384, t-06-2835, t-06-2836. Similar results were obtained for the transconjugants (not shown).
Compared to the naïve E. coli recipient strains J53 Azr and Top10, the transconjugants and transformants demonstrated increased resistance to all quinolone antibiotics tested (Table 1). The MIC of the fluoroquinolones was 8- to 32-fold higher in the transconjugants and transformants, while nalidixic acid showed a 2- to 8-fold increase versus their naïve E. coli recipient strains (Table 1). The transconjugants and transformants also had increased resistance to amoxicillin-clavulanic acid as compared to the naïve E. coli recipient strains. All transconjugants and transformants were highly resistant to ampicillin and several cephalosporins, characteristic of beta-lactamase activity. The transconjugants and transformants demonstrated similar susceptibility to azithromycin, clarithromycin, gentamicin, tetracycline, and trimethoprim-sulfamethoxazole compared to their naïve E. coli recipient strains. Interestingly, when compared to the S. flexneri donor isolates, the transconjugants and transformants in general had about 2- to 8-fold lower MIC levels for some of the quinolones tested. This result suggests the novel S. flexneri isolates may have additional mechanisms to resist quinolones not harbored on the plasmid(s) transferred to the transconjugants and transformants.
Whole Genome Analysis of S. flexneri Strains Isolated From Macaques
To corroborate antibiotic resistance phenotypes, whole genome sequences of two representative S. flexneri isolates, 06-2384 and 06-3102, were obtained (summarized in Table 2 and Supplementary Table 1). The genomes for S. flexneri 06-2384 and 06-3102 were assembled into 5 and 8 contigs, respectively. The largest contig for both isolates was ~4.7 Mb with a G+C content of ~50.9%, which is consistent with the chromosomal sequence of other S. flexneri genomes (Jin et al., 2002; Wei et al., 2003; Shen et al., 2017). Using PlasmidFinder-1.2, plasmid replicon sequences were detected in the remaining contigs, suggesting they are plasmids. Additionally, the top 5 BLAST hits for these contigs corresponded to plasmids sequences from Shigella spp., E. coli, S. enterica, K. pneumoniae, and Citrobacter freundii (Supplementary Table 2). Thus, S. flexneri 06-2384 and 06-3102 strains both appear to harbor several different plasmids in addition to their chromosome. This agrees with Figure 1 in that several bands were apparent after separating plasmid DNA. Despite representing the serotype variants Y and 5b, both 06-2384 and 06-3102 were identified as ST245 by in silico MLST and had an ANI of 99.95% and a dDDH of 99.80%, indicating substantial genetic similarity. However, the difference in serotypes as well as the number/size of plasmids (Figure 1 and Table 2) suggests more than one strain variant was present in the NHP colony.
Antibiotic Resistance Genes Detected by Whole Genome Analysis
ResFinder-2.1 identified several antibiotic resistance genes in the chromosome and plasmid sequences (Table 2). Aminoglycosides (aadA1, aac(3)-IId), beta-lactams (blaOXA-1), quinolones (oqxA, oqxB), chloramphenicol (catA), and tetracycline (tet(B)) resistance genes were detected in the chromosomes of both strains. In both genomes, oqxA, oqxB, and aac(3)-IId and aadA1, blaOXA-1, catA1, and tet(B) clustered together in separate regions. Both of these gene clusters were flanked by several mobile element protein, transposase, and integrase gene annotations. Mutations in the genes for DNA gyrase and topoisomerase subunits A and B were not identified in either of the genomes. Colistin resistance genes (mcr-1, mcr-2, and mcr-3) were also not identified.
The qnrS gene and beta-lactam resistance gene blaTEM-1B were detected on the 60 and 52 kb plasmid contigs for S. flexneri 06-2384 and 06-3102, respectively. BLAST analysis found these plasmid contigs were homologous to the completely-sequenced ~40 kb, conjugative plasmid pVQS1 from S. enterica (Karczmarczyk et al., 2012) and the partially-sequenced ~47 kb, conjugative plasmid pAH0376 from Shigella flexneri 2b (Hata et al., 2005), both of which harbor functional blaTEM-1B and qnrS1 resistance genes. The annotated qnrS genes from the novel S. flexneri isolates showed 100% sequence identity and coverage to the qnrS1 genes found in pAH0376 and pVQS1. In general, the resistance genes and flanking mobile element protein genes from S. flexneri 06-2384 and 06-3102 showed >98% identity and similar synteny to pVQS1 and pAH0376, except for the transposase genes tinR vs. tnpA, which had 31%, identify homology (Figure 2). Bidirectional BLASTP analysis using PATRIC's proteome comparison tool indicated >90% of the annotated genes in pVQS1 shared >97% identity to those of the S. flexneri 06-2384 and 06-3102 plasmid sequences, including homologs for replication and conjugation genes. This suggests these plasmid sequences from S. flexneri 06-2384 and 06-3102 have the potential for self-replication and ability to transfer to new hosts. The S. flexneri 06-2384 and 06-3102 plasmid sequences were identical to each other, except 06-3102 appears to contain a duplication of the region encoding qnrS and associated mobile element proteins. This second qnrS gene is truncated into a predicted 106 amino acid product and is likely non-functional as a result.
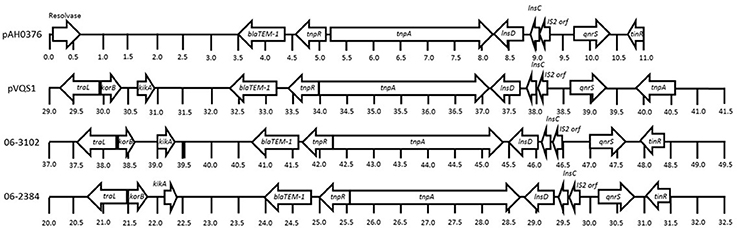
Figure 2. Comparative alignment showing the blaTEM-1B, qnrS, and flanking mobile element protein genes from the plasmid sequences of pAH0376 (Shigella flexneri 2b; GenBank: AB187515.1), pVQS1 (Salmonella enterica; GenBank: JQ609357.1), and Shigella flexneri 06-2384 and 06-3102 have nearly identical homology and synteny. Arrowheads represent annotated protein coding sequences and direction of their transcription. Numbers below each sequence represent distance expressed in kilobases.
S. flexneri 06-3102 also contained the beta-lactam resistance gene blaCTX-M-14 on a ~86 kb plasmid contig. BLAST analysis of this plasmid contig detected >99% identity to the ~74 kb IncFII2 plasmid pAC2901 from C. freundii strain AC290 that also encodes the blaCTX-M-14 resistance gene. The remaining plasmid contig sequences from S. flexneri 06-2384 and 06-3102 showed BLAST homology to plasmids from Enterboacteriaceae species (Supplemental Table 2). These plasmid sequences were typed as ColRNAI using PlasmidFinder1.2, primarily contained hypothetical proteins, and some encoded phage-related gene annotations. Interestingly, the 15 and 19 kb plasmids from S. flexneri 06-2384 and 06-3102, respectively, were enriched for ferric enterobactin uptake protein FepE (8/17 and 9/25 annotations for S. flexneri 06-2384 and 06-3102, respectively), suggesting a role in iron acquisition.
Virulence Factor Genes Detected by Whole Genome Analysis
Using VirulenceFinder1.5, virulence factor genes were detected on the chromosome and ~210 kb plasmid sequences from both isolates (Table 2). Additional potential virulence factor genes were also identified by BLASTP from the VFDB, Victors, and PATRIC_VF databases using the specialty genes feature hosted by PATRIC. This included 998 and 1,009 virulence factor genes detected in S. flexneri 06-2384 and 06-3102, respectively, with functions ranging from host adherence and invasion to biofilm formation and iron acquisition (Supplemental Table 3).
Of particular note, several serine protease autotransporters of Enterobacteriaceae (SPATE) enterotoxin genes were identified, which are implicated in diarrheal illness and gastrointestinal cytotoxicity by pathogenic Shigella spp. and E. coli infection (Dautin, 2010; Mattock and Blocker, 2017). For both S. flexneri 06-2384 and 06-3102, these include the chromosomally-encoded Shigella enterotoxin 1 (ShET1), protease involved in intestinal colonization (Pic), and Shigella extracellular protein A (SigA) genes, which have been shown to exert cytotoxicity to epithelial cells, mediate serum resistance, induce fluid secretion, and degrade mucus/mucin (Dautin, 2010; Faherty et al., 2012; Mattock and Blocker, 2017). Two paralogs for ShET2 along with type III secretion system (T3SS) components and conjugative transfer genes were identified on the ~210 kb, IncFII plasmids in both S. flexneri 06-2384 and 06-3102. These ShET2 paralogs are T3SS effector proteins that induce secretory activity by the gastrointestinal epithelium to cause fluid-laden diarrhea (Farfan et al., 2011; Faherty et al., 2012; Mattock and Blocker, 2017).
Discussion
In this study, five novel, multiple-antibiotic resistant S. flexneri isolates were cultured from the feces of three macaques maintained in a biomedical research colony. MIC testing demonstrated these S. flexneri isolates were resistant or have decreased susceptibility to beta-lactam, cephalosporin, aminoglycoside, tetracycline, and quinolone, but were susceptible to macrolide and sulfonamide antibiotic classes. Treatment with trimethoprim-sulfadiazine successfully eradicated S. flexneri infection in two of these animals, while infection in the third appeared to be self-limiting after post-surgical use of enrofloxacin. The purpose of this study was to understand the potential mechanisms of multi-drug resistance in these novel S. flexneri isolates.
PCR of plasmid-enriched DNA detected the qnrS, but not qnrA or qnrB, in all of the S. flexneri isolates. The sequences of the qnrS genes had 100% homology to the plasmid-encoded qnrS1 genes from S. flexneri and other Enterobacteriaceae species with quinolone resistance phenotypes. This suggested quinolone resistance in the novel S. flexneri isolates was mediated by a plasmid-encoded qnrS1 gene. To test this, quinolone-susceptible E. coli strains were introduced with plasmid DNA from the novel S. flexneri isolates via conjugation or transformation and then tested for antibiotic resistance. Plasmid DNA isolated from the transconjugants and transformants was PCR-positive for the qnrS gene. The transconjugants and transformants demonstrated decreased susceptibility to quinolones and resistance to beta-lactam antibiotics compared to their respective naïve E. coli recipient strains. When compared to their respective S. flexneri donor isolates, the transconjugants and transformants in general had lower resistance to the quinolones.
To further support that the novel S. flexneri isolates harbored plasmid-encoded qnrS genes and to identify other mechanisms for quinolone resistance, whole genome sequences were obtained for two representative isolates. Consistent with the MIC results, antibiotic resistance genes for aminoglycosides (aadA1, aac(3)-IId), beta-lactams (blaOXA-1), quinolones (oqxA, oqxB), and tetracycline (tet(B)) were detected in their chromosomes. The catA was also detected in the chromosomes, suggesting these isolates have the potential for chloramphenicol resistance, although this was not experimentally confirmed. The oqxA and oqxB genes have been previously identified in E. coli, K. pneumoniae, and K. oxytoca isolates and encode multi-drug efflux pumps against quinolones that yield low-level resistance (Poirel et al., 2012; Andres et al., 2013; Rodriguez-Martinez et al., 2013).
In both S. flexneri genomes, qnrS was co-associated with blaTEM-1B on plasmid-like sequences that had identical homology and synteny to pAH0376 and pVQS1 from S. flexneri 2b and S. enterica, respectively. Additionally, as observed for pAH0376 and pVQS1, these plasmids could be experimentally transferred to E. coli, conferring the recipients with quinolone and beta-lactam resistance (Hata et al., 2005; Karczmarczyk et al., 2012). The activity of oqxA and oqxB combined with qnrS may enhance overall quinolone resistance in these S. flexneri isolates and may explain why the E. coli recipients demonstrated less resistance to quinolones compared to their donor counterparts.
A recently published study surveyed the prevalence of antimicrobial resistance in zoonotic pathogens cultured from NHPs across several different biomedical research institutions in the United States (Kim et al., 2017). The authors found that enrofloxacin is the primary antibiotic chosen by veterinarians for treating diarrhea and gingivitis caused by presumptive S. flexneri infection, and while diagnostic labs commonly screen S. flexneri isolates for enrofloxacin resistance, no resistant strains were reported. A major caveat of this study is the small number of participating institutions; only seven institutions participated, and antibiotic resistance data was obtained from just three. Likewise, the methods used to detect and characterize antibiotic resistance potential were inconsistent between institutions. Therefore, estimations of resistance to enrofloxacin and other quinolones by S. flexneri could have been underreported or missed.
The World Health Organization (WHO) classifies quinolones as the highest priority of clinically important antibiotics based on it being a limited available therapy option to treat serious bacterial infections with a high risk of zoonosis and an ability to acquire antibiotic resistance genes (WHO, 2017). The Centers for Disease Control and Prevention (CDC) recognizes that Shigella spp. are becoming increasingly resistant to fluoroquinolones and other antibiotics (CDC, 2017). The CDC recommends that fluoroquinolones should be avoided when the MIC for ciprofloxacin is ≥0.12 μg/mL, even though this is classified as “susceptible” according to CLSI criteria. The novel S. flexneri isolates and their E. coli transconjugants/transformants in this study had MICs for ciprofloxacin of 0.25–2 μg/mL, which though below the CLSI criteria for resistance, meet the CDC's standard for excluding fluoroquinolones to treat Shigella spp.
According to the latest report by the National Antimicrobial Resistance Monitoring System (2016), as well as the survey by Kim et al. (2017), S. flexneri isolates from humans and NHP's frequently demonstrate resistance to more than one class of antibiotics. Shigella spp. can also exhibit multi-drug resistance with substantial diversity observed in their resistance profiles. As observed for the S. flexneri isolates in this study, resistance or decreased susceptibility to beta-lactam, cephalosporin, aminoglycoside, and tetracycline antibiotic classes, along with quinolones, was noted. The co-association of qnrS1 and blaTEM-1B and other antibiotic resistance gene clusters poses a particular risk in predicting successful eradication strategies. Therefore, culture for Shigella spp. and other enteric pathogens followed by antibiotic testing should be perform prior to initiation of treatment.
The finding of quinolone-resistant S. flexneri in this study may reflect the common use of enrofloxacin to preemptively treat suspected shigella-induced diarrheal disease commonly encountered in newly imported macaques from Asia (Fox, 1975; Tribe and Fleming, 1983; Line et al., 1992; Banish et al., 1993). Macaques co-housed or maintained in groups can also readily acquire Shigella spp. infections from other infected monkeys via fecal/oral or oral/oral routes (Shipley et al., 2010). Cross-transmission of Shigella spp. could have occurred in our colony because animals were co-housed in adjacent cages within the same animal facility. In two of the three rhesus monkeys, a standard enrofloxacin regimen failed to eradicate the S. flexneri infection, but trimethoprim-sulfadiazine treatment was successful. Trimethoprim-sulfamethoxazole has been reported to successfully eradicate Shigella spp. infection in a colony of wild-caught rhesus monkeys (Pucak et al., 1977). S. flexneri in the third rhesus was eliminated during a 10-day course of enrofloxacin treatment. Shigellosis in humans is known to typically resolve within 5–7 days without antibiotic treatment (Hale and Keusch, 1996), so it is possible infection was self-limiting in this individual.
Humans can become infected with Shigella spp. through contact with NHPs in research or zoo setting or maintained as pets (Fox, 1975; Tribe and Fleming, 1983; Kennedy et al., 1993). Shigella spp. infection in humans can readily transmit from person-to-person or through contaminated food or water (Scallan et al., 2011). Additionally, ciprofloxacin has continued to be one of the most commonly prescribed antibiotics for treating shigellosis in human patients despite the discovery of ciprofloxacin-resistant Shigella spp. and plasmid-mediated quinolone resistant strains of S. flexneri (Bowen et al., 2015; CDC, 2017). Therefore, S. flexneri isolates with the capacity to spread and acquire quinolone resistance genes represent not only an institutional concern when treating NHPs, but also a public health concern considering the zoonotic potential of these pathogens. Strict hygienic principles should also be implemented and always followed by personnel in contact with NHPs in order to reduce risk of acquiring infection.
In conclusion, this study demonstrates for the first time the discovery of plasmid-mediated quinolone resistance genes in multiple-antibiotic drug resistant S. flexneri isolated from research macaques. Given that antibiotic treatments, such as enrofloxacin, are often prescribed empirically and prophylactically, the findings of this study stress the need to test for antibiotic susceptibility in NHPs with suspected Shigella spp. infection beforehand in order to appropriately treat these animals and reduce zoonotic risk.
Ethics Statement
All procedures were performed under animal protocols approved by the Massachusetts Institute of Technology Committee on Animal Care (CAC) and in accordance with the guidelines for animal care at Massachusetts Institute of Technology. Animals were cared for in an AAALAC International-accredited animal facility at MIT under federal, state, local and NIH guidelines for animal care.
Author Contributions
AM, HM, ZS, JD-F, and AG characterized novel Shigella isolates. EB cultured novel Shigella isolates. HM, RM, and MP provided veterinary clinical work up of non-human primates, including sample collection. AM, HM, JD-F, and JF wrote and/or reviewed manuscript.
Funding
This study was supported by NIH grants T32-OD010978-26 (JF), P30-ES002109 (JF).
Conflict of Interest Statement
The authors declare that the research was conducted in the absence of any commercial or financial relationships that could be construed as a potential conflict of interest.
Supplementary Material
The Supplementary Material for this article can be found online at: https://www.frontiersin.org/articles/10.3389/fmicb.2018.00311/full#supplementary-material
References
Andres, P., Lucero, C., Soler-Bistue, A., Guerriero, L., Albornoz, E., Tran, T., et al. (2013). Differential distribution of plasmid-mediated quinolone resistance genes in clinical enterobacteria with unusual phenotypes of quinolone susceptibility from Argentina. Antimicrob. Agents Chemother. 57, 2467–2475. doi: 10.1128/AAC.01615-12
Armitage, G. C., Newbrun, E., Hoover, C. I., and Anderson, J. H. (1982). Periodontal disease associated with Shigella flexneri in rhesus monkeys. Clinical, microbiologic and histopathologic findings. J. Periodontal Res. 17, 131–144. doi: 10.1111/j.1600-0765.1982.tb01139.x
Auch, A. F., Klenk, H. P., and Goker, M. (2010). Standard operating procedure for calculating genome-to-genome distances based on high-scoring segment pairs. Stand. Genomic Sci. 2, 142–148. doi: 10.4056/sigs.541628
Aziz, R. K., Bartels, D., Best, A. A., DeJongh, M., Disz, T., Edwards, R. A., et al. (2008). The RAST Server: rapid annotations using subsystems technology. BMC Genomics 9:75. doi: 10.1186/1471-2164-9-75
Banish, L. D., Sims, R., Sack, D., Montali, R. J., Phillips, L. Jr., and Bush, M. (1993). Prevalence of shigellosis and other enteric pathogens in a zoologic collection of primates. J. Am. Vet. Med. Assoc. 203, 126–132.
Bowen, A., Hurd, J., Hoover, C., Khachadourian, Y., Traphagen, E., Harvey, E., et al. (2015). Importation and domestic transmission of Shigella sonnei resistant to ciprofloxacin - United States, May 2014-February 2015. Morbid. Mortal. Week. Rep. 64, 318–320.
Carattoli, A., Zankari, E., Garcia-Fernandez, A., Voldby Larsen, M., Lund, O., Villa, L., et al. (2014). In silico detection and typing of plasmids using PlasmidFinder and plasmid multilocus sequence typing. Antimicrob. Agents Chemother. 58, 3895–3903. doi: 10.1128/AAC.02412-14
Cattoir, V., Weill, F. X., Poirel, L., Fabre, L., Soussy, C. J., and Nordmann, P. (2007). Prevalence of qnr genes in Salmonella in France. J. Antimicrob. Chemother. 59, 751–754. doi: 10.1093/jac/dkl547
CDC (2017). CDC Recommendations for Diagnosing and Managing Shigella Strains with Possible Reduced Susceptibility to Ciprofloxacin. Atlanta, GA: U.S. Department of Health and Human Services.
Dautin, N. (2010). Serine protease autotransporters of enterobacteriaceae (SPATEs): biogenesis and function. Toxins 2, 1179–1206. doi: 10.3390/toxins2061179
Faherty, C. S., Harper, J. M., Shea-Donohue, T., Barry, E. M., Kaper, J. B., Fasano, A., et al. (2012). Chromosomal and plasmid-encoded factors of Shigella flexneri induce secretogenic activity ex vivo. PLoS ONE 7:e49980. doi: 10.1371/journal.pone.0049980
Farfan, M. J., Toro, C. S., Barry, E. M., and Nataro, J. P. (2011). Shigella enterotoxin-2 is a type III effector that participates in Shigella-induced interleukin 8 secretion by epithelial cells. FEMS Immunol. Med. Microbiol. 61, 332–339. doi: 10.1111/j.1574-695X.2011.00778.x
Fox, J. G. (1975). Transmissible drug resistance in Shigella and Salmonella isolated from pet monkeys and their owners. J. Med. Primatol. 4, 165–171. doi: 10.1159/000459850
Grant, J. R., and Stothard, P. (2008). The CGView Server: a comparative genomics tool for circular genomes. Nucleic Acids Res. 36, W181–W184. doi: 10.1093/nar/gkn179
Hale, T. L., and Keusch, G. T. (1996). “Shigella,” in Medical Microbiology, 4th edn, ed S. Baron (Galveston, TX: University of Texas Medical Branch at Galveston).
Hata, M., Suzuki, M., Matsumoto, M., Takahashi, M., Sato, K., Ibe, S., et al. (2005). Cloning of a novel gene for quinolone resistance from a transferable plasmid in Shigella flexneri 2b. Antimicrob. Agents Chemother. 49, 801–803. doi: 10.1128/AAC.49.2.801-803.2005
Jin, Q., Yuan, Z., Xu, J., Wang, Y., Shen, Y., Lu, W., et al. (2002). Genome sequence of Shigella flexneri 2a: insights into pathogenicity through comparison with genomes of Escherichia coli K12 and O157. Nucleic Acids Res. 30, 4432–4441. doi: 10.1093/nar/gkf566
Joensen, K. G., Scheutz, F., Lund, O., Hasman, H., Kaas, R. S., Nielsen, E. M., et al. (2014). Real-time whole-genome sequencing for routine typing, surveillance, and outbreak detection of verotoxigenic Escherichia coli. J. Clin. Microbiol. 52, 1501–1510. doi: 10.1128/JCM.03617-13
Karczmarczyk, M., Stephan, R., Hachler, H., and Fanning, S. (2012). Complete nucleotide sequence of pVQS1 containing a quinolone resistance determinant from Salmonella enterica serovar Virchow associated with foreign travel. J. Antimicrob. Chemother. 67, 1861–1864. doi: 10.1093/jac/dks158
Kennedy, F. M., Astbury, J., Needham, J. R., and Cheasty, T. (1993). Shigellosis due to occupational contact with non-human primates. Epidemiol. Infect. 110, 247–251. doi: 10.1017/S0950268800068163
Kim, J., Coble, D. J., Salyards, G. W., Bower, J. K., Rinaldi, W. J., Plauche, G. B., et al. (2017). Antimicrobial use for and resistance of zoonotic bacteria recovered from nonhuman primates. Comp. Med. 67, 79–86.
Larsen, M. V., Cosentino, S., Rasmussen, S., Friis, C., Hasman, H., Marvig, R. L., et al. (2012). Multilocus sequence typing of total-genome-sequenced bacteria. J. Clin. Microbiol. 50, 1355–1361. doi: 10.1128/JCM.06094-11
Lee, I., Ouk Kim, Y., Park, S. C., and Chun, J. (2016). OrthoANI: an improved algorithm and software for calculating average nucleotide identity. Int. J. Syst. Evol. Microbiol. 66, 1100–1103. doi: 10.1099/ijsem.0.000760
Line, A. S., Paul-Murphy, J., Aucoin, D. P., and Hirsh, D. C. (1992). Enrofloxacin treatment of long-tailed macaques with acute bacillary dysentery due to multiresistant Shigella flexneri IV. Lab. Anim. Sci. 42, 240–244.
Mammeri, H. M., Van De Loo Poirel, L., Martinez-Martinez, L., and Nordmann, P. (2005). Emergence of plasmid-mediated quinolone resistance in Escherichia coli in Europe. Antimicrob. Agents Chemother. 49, 71–76. doi: 10.1128/AAC.49.1.71-76.2005
Martinez-Martinez, L., Pascual, A., and Jacoby, G. A. (1998). Quinolone resistance from a transferable plasmid. Lancet 351, 797–799. doi: 10.1016/S0140-6736(97)07322-4
Mattock, E., and Blocker, A. J. (2017). How do the virulence factors of shigella work together to cause disease? Front. Cell. Infect. Microbiol. 7:64. doi: 10.3389/fcimb.2017.00064
McClure, H. M., Alford, P., and Swenson, B. (1976). Nonenteric Shigella infections in nonhuman primates. J. Am. Vet. Med. Assoc. 169, 938–939.
National Antimicrobial Resistance Monitoring System (2016). National Antimicrobial Resistance Monitoring System for Enteric Bacteria (NARMS): Human Isolates Surveillance Report for 2014. Final Report, U.S. Department of Health and Human Services, Atlanta, GA.
Poirel, L., Cattoir, V., and Nordmann, P. (2012). Plasmid-mediated quinolone resistance; interactions between human, animal, and environmental ecologies. Front. Microbiol. 3:24. doi: 10.3389/fmicb.2012.00024
Pucak, G. J., Orcutt, R. P., Judge, R. J., and Rendon, F. (1977). Elimination of the Shigella carrier state in rhesus monkeys (Macaca mulatta) by trimethoprim-sulfamethoxazole. J. Med. Primatol. 6, 127–132. doi: 10.1159/000459732
Redgrave, L. S., Sutton, S. B., Webber, M. A., and Piddock, L. J. (2014). Fluoroquinolone resistance: mechanisms, impact on bacteria, and role in evolutionary success. Trends Microbiol. 22, 438–445. doi: 10.1016/j.tim.2014.04.007
Robicsek, A., Strahilevitz, J., Sahm, D. F., Jacoby, G. A., and Hooper, D. C. (2006). qnr prevalence in ceftazidime-resistant Enterobacteriaceae isolates from the United States. Antimicrob. Agents Chemother. 50, 2872–2874. doi: 10.1128/AAC.01647-05
Rodriguez-Martinez, J. M., Diaz de Alba, P., Briales, A., Machuca, J., Lossa, M., Fernandez-Cuenca, F., et al. (2013). Contribution of OqxAB efflux pumps to quinolone resistance in extended-spectrum-β-lactamase-producing Klebsiella pneumoniae. J. Antimicrob. Chemother. 68, 68–73. doi: 10.1093/jac/dks377
Scallan, E., Hoekstra, R. M., Angulo, F. J., Tauxe, R. V., Widdowson, M. A., Roy, S. L., et al. (2011). Foodborne illness acquired in the United States–major pathogens. Emerging Infect. Dis. 17, 7–15. doi: 10.3201/eid1701.P11101
Shen, P., Fan, J., Guo, L., Li, J., Li, A., Zhang, J., et al. (2017). Genome sequence of Shigella flexneri strain SP1, a diarrheal isolate that encodes an extended-spectrum β-lactamase (ESBL). Ann. Clin. Microbiol. Antimicrob. 16:37. doi: 10.1186/s12941-017-0212-2
Shipley, S. T., Panda, A., Khan, A. Q., Kriel, E. H., Maciel, M. Jr., Livio, S., et al. (2010). A challenge model for Shigella dysenteriae 1 in cynomolgus monkeys (Macaca fascicularis). Comp. Med. 60, 54–61.
Clinical and Laboratory Standards Institute (2010). Performance Standards for Antimicrobial Disk Susceptibility Tests, Approved Standard, 10th Edn. Wayne, PA.
Clinical and Laboratory Standards Institute (2012). Methods for Dilution Antimicrobial Susceptibility Tests for Bacteria that Grow Aerobically, Approved Standard, 9th Edn. Wayne, PA.
Clinical and Laboratory Standards Institute (2014). Performance Standards for Antimicrobial Susceptibility Testing; Twenty-Fourth Informational Supplement (M100-S24). Wayne, PA.
Strahilevitz, J., Jacoby, G. A., Hooper, D. C., and Robicsek, A. (2009). Plasmid-mediated quinolone resistance: a multifaceted threat. Clin. Microbiol. Rev. 22, 664–689. doi: 10.1128/CMR.00016-09
Tran, J. H., and Jacoby, G. A. (2002). Mechanism of plasmid-mediated quinolone resistance. Proc. Natl. Acad. Sci. U.S.A. 99, 5638–5642. doi: 10.1073/pnas.082092899
Tran, J. H., Jacoby, G. A., and Hooper, D. C. (2005a). Interaction of the plasmid-encoded quinolone resistance protein Qnr with Escherichia coli DNA gyrase. Antimicrob. Agents Chemother. 49, 118–125. doi: 10.1128/AAC.49.1.118-125.2005
Tran, J. H., Jacoby, G. A., and Hooper, D. C. (2005b). Interaction of the plasmid-encoded quinolone resistance protein QnrA with Escherichia coli topoisomerase IV. Antimicrob. Agents Chemother. 49, 3050–3052. doi: 10.1128/AAC.49.7.3050-3052.2005
Tribe, G. W., and Fleming, M. P. (1983). Biphasic enteritis in imported cynomolgus (Macaca fascicularis) monkeys infected with Shigella, Salmonella and Campylobacter species. Lab. Anim. 17, 65–69. doi: 10.1258/002367783781070957
Wang, M., Sahm, D. F., Jacoby, G. A., and Hooper, D. C. (2004). Emerging plasmid-mediated quinolone resistance associated with the qnr gene in Klebsiella pneumoniae clinical isolates in the United States. Antimicrob. Agents Chemother. 48, 1295–1299. doi: 10.1128/AAC.48.4.1295-1299.2004
Wattam, A. R., Abraham, D., Dalay, O., Disz, T. L., Driscoll, T., Gabbard, J. L., et al. (2014). PATRIC, the bacterial bioinformatics database and analysis resource. Nucleic Acids Res. 42, D581–D591. doi: 10.1093/nar/gkt1099
Wattam, A. R., Davis, J. J., Assaf, R., Boisvert, S., Brettin, T., Bun, C., et al. (2017). Improvements to PATRIC, the all-bacterial bioinformatics database and analysis resource center. Nucleic Acids Res. 45, D535–D542. doi: 10.1093/nar/gkw1017
Wei, J., Goldberg, M. B., Burland, V., Venkatesan, M. M., Deng, W., Fournier, G., et al. (2003). Complete genome sequence and comparative genomics of Shigella flexneri serotype 2a strain 2457T. Infect. Immun. 71, 2775–2786. doi: 10.1128/IAI.71.5.2775-2786.2003
WHO (2017). Critically Important Antimicrobials for Human Medicine, 5th Edn. Geneva: World Health Organization.
Keywords: plasmid-mediated quinolone resistance, enrofloxacin resistance, multi-drug antibiotic resistance, Shigella flexneri, non-human primates, zoonotic risk
Citation: Mannion AJ, Martin HR, Shen Z, Buckley EM, Dzink-Fox JL, Garcia A, Marini RP, Patterson MM and Fox JG (2018) Plasmid-Mediated Quinolone Resistance in Shigella flexneri Isolated From Macaques. Front. Microbiol. 9:311. doi: 10.3389/fmicb.2018.00311
Received: 10 October 2017; Accepted: 09 February 2018;
Published: 05 March 2018.
Edited by:
Yonghong Xiao, Zhejiang University, ChinaReviewed by:
Zhi Ruan, Sir Run Run Shaw Hospital, ChinaRemy A. Bonnin, Université Paris-Saclay, France
Copyright © 2018 Mannion, Martin, Shen, Buckley, Dzink-Fox, Garcia, Marini, Patterson and Fox. This is an open-access article distributed under the terms of the Creative Commons Attribution License (CC BY). The use, distribution or reproduction in other forums is permitted, provided the original author(s) and the copyright owner are credited and that the original publication in this journal is cited, in accordance with accepted academic practice. No use, distribution or reproduction is permitted which does not comply with these terms.
*Correspondence: James G. Fox, jgfox@mit.edu
†Present Address: Heather R. Martin, Sloan Kettering Institute, New York, NY, United States