- 1Department of Infectious Diseases, Robert Koch Institute, Berlin, Germany
- 2Postgraduate Training for Applied Epidemiology, Robert Koch Institute, Affiliated to the European Programme for Intervention Epidemiology Training, European Centre for Disease Prevention and Control (ECDC), Stockholm, Sweden
- 3Department of Infection Control, Medical Disaster Control and Environmental Health Control, Department of Public Health, Berlin, Germany
- 4MEDIAN Klinik Berlin-Kladow, Berlin, Germany
- 5Medical Care Centre Labor 28 GmbH, Berlin, Germany
- 6National Reference Centre for Multidrug-Resistant Gram-Negative Bacteria, Department for Medical Microbiology, Ruhr-University Bochum, Berlin, Germany
- 7Immunology and Laboratory Medicine, Institute for Microbiology, HELIOS Klinikum Emil von Behring, Berlin, Germany
- 8Leibniz Institute DSMZ, German Collection of Microorganisms and Cell Culture, Braunschweig, Germany
Extended-spectrum β-lactamase (ESBL) producing Klebsiella pneumoniae pose an important threat of infection with increased morbidity and mortality, especially for immunocompromised patients. Here, we use the rise of multidrug-resistant K. pneumoniae in a German neurorehabilitation center from April 2015 to April 2016 to dissect the benefit of whole genome sequencing (WGS) for outbreak analyses. In total, 53 isolates were obtained from 52 patients and examined using WGS. Two independent analysis strategies (reference-based and -free) revealed the same distinct clusters of two CTX-M-15 producing K. pneumoniae clones (ST15, n = 31; ST405, n = 7) and one CTX-M-15 producing Klebsiella quasipneumoniae strain (ST414, n = 8). Additionally, we determined sequence variations associated with antimicrobial resistance phenotypes in single isolates expressing carbapenem and colistin resistance, respectively. For rapid detection of the major K. pneumoniae outbreak clone (ST15), a selective triplex PCR was deduced from WGS data of the major outbreak strain and K. pneumoniae genome data deposited in central databases. Moreover, we introduce two novel open-source applications supporting reference genome selection (refRank; https://gitlab.com/s.fuchs/refRank) and alignment-based SNP-filtering (SNPfilter; https://gitlab.com/s.fuchs/snpfilter) in NGS analyses.
Introduction
Extended-spectrum β-lactamase (ESBL)-producing Klebsiella pneumoniae are important nosocomial pathogens (Gupta et al., 2003). In particular, immunocompromised persons are susceptible to severe infections caused by K. pneumoniae (Podschun and Ullmann, 1998). Nosocomial outbreaks with K. pneumoniae are frequently reported from (neonatal) intensive care units (Calbo and Garau, 2015; Stapleton et al., 2016). Within the last few years, the benefit of whole genome sequencing (WGS) to analyse, confirm, and understand better outbreak scenarios has been shown for distinct settings (Quainoo et al., 2017). For instance, three temporally separated case clusters in a Nepali hospital were found to be in fact caused by a single K. pneumoniae strain using WGS-based analysis strategies (Stoesser et al., 2014). Furthermore, WGS-based analysis of an ongoing detection of ESBL-producing K. pneumoniae revealed prevalence of the outbreak strain over a period of several months while extensive infection control measures have been performed. In addition, WGS analysis allowed identification of the origin of the outbreak years before the first clinical and supposed index case was notified (Haller et al., 2015). Nevertheless, most of these studies were performed retrospectively. Although real-time WGS analysis during nosocomial outbreaks is, in principle, a realistic scenario nowadays, limitations in terms of costs, quick access to relevant techniques and qualified personnel and other factors exist in practice. Deducing quick, simple but reliable and specific tests such as PCR-based assays using WGS data would be one of the possible solutions to existing restrictions.
Formerly, K. pneumoniae has been divided into three phylogroups (KpI, KpII, KpIII) and meanwhile these groups have been classified as distinct species (K. pneumoniae, Klebsiella quasipneumoniae, and Klebsiella variicola; Brisse and Verhoef, 2001; Brisse et al., 2004, 2014; Rosenblueth et al., 2004). Accordingly, the term K. pneumoniae sensu stricto refers to isolates belonging to KpI, while the term K. pneumoniae sensu lato comprises isolates of all three phylogroups/species. In previous studies, the majority of clinical Klebsiella isolates was found to belong to KpI (69–82%), while KpII and KpIII represent a remarkably smaller proportion (6–8 and 11–24%, respectively; Brisse et al., 2004; Maatallah et al., 2014). A similar composition was reported for ESBL-producing Klebsiella isolates from a Spanish hospital (Valverde et al., 2008). In contrast to standard diagnostic procedures (MALDI TOF MS) and phenotypic assays for species prediction, only genotypic approaches based on allelic sequences of gyrA and parC or chromosomal β-lactamase genes (bla) allow a reliable differentiation between these species (Brisse and Verhoef, 2001; Brisse et al., 2004). K. pneumoniae was shown to be associated with blaSHV, K. quasipneumoniae with blaOKP and K. variicola with blaLEN (Haeggman et al., 2004). This genotypic information could also be deduced from WGS data. In a recent report Long and colleagues used WGS of 1,777 clinical ESBL-producing Klebsiella isolates, all initially determined as K. pneumoniae by MALDI TOF MS, to further resolve their subspecies into 13 K. variicola and 15 K. quasipneumoniae (0.7 and 0.8%, respectively; Long et al., 2017). In addition, specific PCRs were developed for the identification of K. pneumoniae sensu stricto and K. variicola, respectively (Bialek-Davenet et al., 2014; Garza-Ramos et al., 2015). However, these methods are usually not part of the standard diagnostic routine in clinical laboratories.
From April 2015 onwards, an increased incidence of patients colonized or infected with ESBL-producing K. pneumoniae was observed in a neurorehabilitation center in Germany. Here, we report the WGS-based analysis of isolates from this setting including deduction of a selective PCR screening for rapid detection of the major K. pneumoniae clone and relevant resistance characteristics. Furthermore, we introduce refRank and SNPfilter, two bioinformatics' tools developed for mapping-based approaches to identify the best matching reference genome and to filter single nucleotide polymorphisms (SNPs) in sequence alignments, respectively.
Materials and Methods
Collection of Isolates
Isolation of bacteria and primary diagnostics including biochemical and automated species identification via MALDI-TOF MS (MALDI Biotyper, Bruker Daltonik, Bremen, Germany) as well as antibiotic susceptibility testing (minimum inhibitory concentration determined using BD Phoenix System) were performed by the routine diagnostic laboratory of the hospital. ESBL-positive isolates were identified using Chromagar ESBL (Mast Group, Reinfeld, Germany). A total of 53 bacterial isolates (52 patients) collected between April 2015 and April 2016 were sent to the Robert Koch Institute, Germany and the National Reference Centre for multidrug-resistant gram-negative bacteria for molecular typing (Table 1).
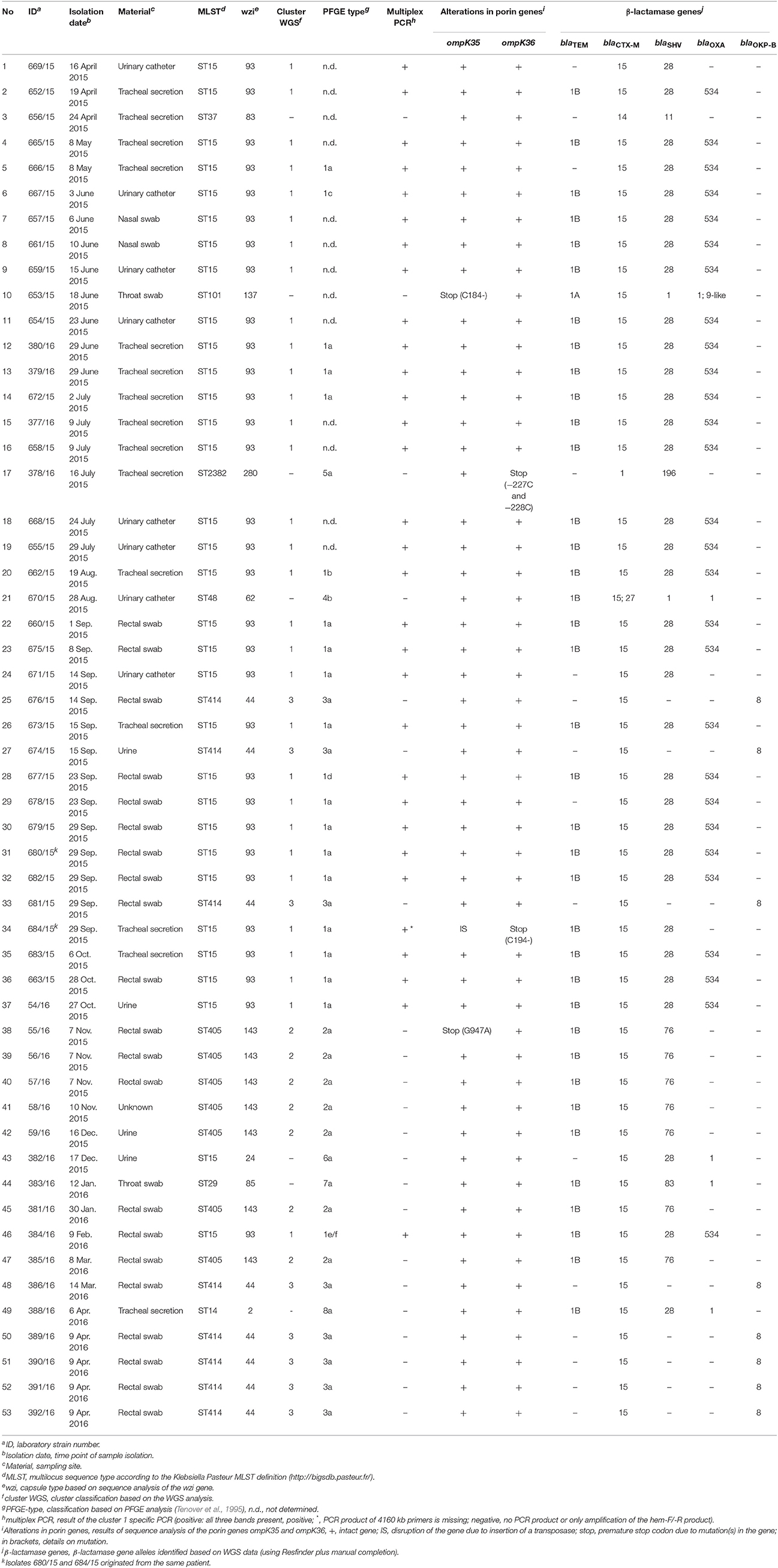
Table 1. Overview of characteristics of all Klebsiella isolates from the neurohabilitation centre, April 2015–April 2016.
Phenotypic Resistance Testing
Antibiotic susceptibilities were determined using the VITEK 2 system (card AST-N248, bioMérieux) and results were interpreted according to the EUCAST breakpoints (version 7.1). In addition, Etest (bioMérieux) for imipenem, ertapenem, meropenem, and colistin was used (Table S1). For a set of 12 out of 53 isolates representing all major clonal variants the MIC for colistin was determined using broth microdilution according to EUCAST.
Whole Genome Sequencing
Bacteria were cultivated in Brain Heart Infusion (BHI) broth. DNA was extracted from overnight cultures using the MagAttract Kit (Qiagen, Hilden, Germany) and the DNeasy Blood & Tissue Kit (Qiagen) in line with the manufacturer's instructions. Qubit dsDNA HS Assay Kit (Invitrogen/Thermo Fisher Scientific, Karlsruhe, Germany) was used for DNA quantification. Sequencing libraries were prepared applying the Nextera XT Kit (Illumina, San Diego, USA) and sequenced on an Illumina Miseq using v3 chemistry (2 × 300 bp) according to the manufacturer's protocol.
Mapping-Based WGS Data Analysis
Raw reads were trimmed applying Trimmomatic (version 0.32, parameters: illuminaclip off, slidingwindow 4:15, leading 3, trailing 3, crop off, headcrop off, minlen 36, avgqual off; Bolger et al., 2014) and mapped to the reference genome of K. pneumoniae PMK-1 (NZ_CP008929) using BWA-SW (version 0.7.13-r1126, default parameters) (Li and Durbin, 2009). The reference genome was selected using refRank (version 1.0.0; see below). SNPs were called using Varscan v2.3 (Koboldt et al., 2012) and variant positions were filtered using SNPfilter (version 2.2.0; see below). Based on the aligned variant positions maximum-likelihood trees were calculated using RAxML (version 8.2.7, model GTR GAMMA, 100 bootstraps; Stamatakis, 2014). The genome sequence of Klebsiella oxytoca KONIH1 (accession: CP008788) was included to root the tree displaying the phylogenetic relationship of all sequenced isolates. For this purpose, artificial Illumina reads were generated from the FASTA-sequence downloaded from NCBI using ART (version 2.5.8, parameters: system MiSeq v3, read length 250, paired end, fragment length 600, standard deviation 300, coverage 100; Huang et al., 2012) and mapped to the reference genome as described above for the sequenced isolates.
Reference Genome Selection Using refRank
To optimize the selection of a reference sequence for NGS read mapping, we created a Python-based application called refRank which provides a coverage-based reference ranking (Figure S1). For this purpose, datasets of single or paired-end reads can be aligned against a collection of defined reference sequences using BWA-SW (Li and Durbin, 2009) or BWA-MEM [arXiv:1303.3997v2 (q-bio.GN)]. Computational costs can be reduced by using only a fraction of randomly picked (paired-end) reads of each dataset (e.g., 10% of all reads). Per base coverage is then determined using SAMtools (Li et al., 2009) and normalized to the reference length and the number of total (mapped and unmapped) reads according to formula (1).
L is reference sequence length, ci is coverage at base i, N is total read number.
Dataset-specific reference ranking is based on the calculated C scores. Additionally, a global reference ranking based on all datasets is provided by calculating the grand average of reference-specific C scores. The source code for refRank is freely available under the terms of the GNU General Public License v3.0 (https://gitlab.com/s.fuchs/refRank).
In the present study, refRank (version 1.0.0) was used with default parameters. The entire collection of completed genomic sequences of K. pneumoniae (n = 63) and K. quasipneumoniae (n = 1) available on RefSeq (accessed at 19th July 2016) was used as reference dataset. All 53 raw read datasets were trimmed using Trimmomatic (Bolger et al., 2014; see above). According to the reference ranking (not shown), the genomic sequence of K. pneumoniae PMK-1 (NZ_CP008929) has been selected as a reference for further analysis.
Variant Site Filtering Using SNPfilter
A python-based application called SNPfilter was developed to condense the alignment of all reconstructed sequences to variant positions only and, thus, to significantly reduce computational costs of subsequent phylogenetic analyses. Optionally, sites can be excluded based on (i) ambiguous base calls and/or deletions, (ii) SNP accumulation (based on exclusion distance), and (iii) user-defined regions (based on genomic coordinates). Importantly, circular replicon topologies can be considered when applying an exclusion distance for accumulated variant positions. The output of SNPfilter provides different files containing (i) aligned variant sites that meet the filter criteria (FASTA format), (ii) general information such as sequence names, number of sites containing ambiguous base calls or gaps, number of variants before and after filtering (TXT format), and (iii) coordinate-specific filter status and sequence information (CSV format). The source code for SNPfilter is freely available under the terms of the GNU General Public License v3.0 (https://gitlab.com/s.fuchs/snpFilter).
In the present study sites containing gaps or ambiguous base calls were excluded. To exclude SNPs in repetitive regions, the reference sequence (NZ_CP008929) was analyzed using the repeat analysis tool in Kodon (Applied Maths) version 3.62 PHASTER (Arndt et al., 2016). Additionally, SNPs in regions of annotated phages and transposases were rejected (not shown).
Maximum Common Genome
Phylogenetic relationship was determined on the basis of the Maximum Common Genome (MCG) (von Mentzer et al., 2014), the set of orthologous genes that are present in all 54 genomes. A gene prediction was performed on the de novo assembled contigs by use of the Prokaryotic Dynamic Programming Genefinding Algorithm (Prodigal) (Hyatt et al., 2010). The obtained coding sequences where then subsequently clustered using USEARCH v7 (Edgar, 2010) with thresholds of 70% similarity on nucleotide level and 90% coverage to determine the set of orthologous genes (n = 1,117) of all genomes. This set was then used as a reference to extract the corresponding allelic variants of the MCG genes from the 54 genomes using PLAST v2.3.1 (Nguyen and Lavenier, 2009). Afterwards the alleles for each gene were aligned with MUSCLE v3.8.31 (Edgar, 2004) and concatenated.
The resulting alignment (1.161 Mbp in length) was used to infer a maximum likelihood phylogeny with RAxML version 8.1.14 using a General Time Reversible model and gamma correction for among site rate variation (Stamatakis, 2014).
De Novo Assembly
Raw reads were trimmed applying Trimmomatic (version 0.32, parameters: Illuminaclip off, maxinfo 50:0.8, leading 3, trailing 3, crop off, headcrop off, minlen 36, avgqual off; Bolger et al., 2014). Trimmed reads were de novo assembled using A5-miseq (version 20150522) with default parameter (Coil et al., 2015).
Cluster Specific Multiplex PCR
The multiplex PCR was designed to allow a rapid identification of isolates belonging to the major outbreak strain (cluster 1). To achieve sufficient discriminatory power three targets had to be considered: (i) a region specific for ST15, (ii) a signature specific for cluster 1 isolates, and (iii) a unique region within a plasmid present in cluster 1 isolates (Table 2). The first primer pair (hem-F/-R) amplifies a presumably ST15-specific region in the ST15 reference genome (NZ_CP008929), which was only covered by reads obtained from cluster 1 isolates (BWA-SW, default parameters). The respective primer pair binds upstream and inside of the hemolysin secretion/activation protein CDS (locus tag PMK1_RS11760) and BLAST search (web BLAST, nucleotide collection, March 2016) of the amplified region did not reveal any further hits in K. pneumoniae isolates (Altschul et al., 1990). Reads of isolate 652/15 that did not map onto the reference genome were de novo assembled in Geneious (version 9.1.6, Geneious assembler, Biomatters Ltd., 30% of data; Kearse et al., 2012). The second primer pair (unique-F/-R) was designed to amplify a region within a contig derived from de novo assembly exhibiting neither BLAST hits within the Klebsiella species nor in plasmid sequences. The third primer pair (4.160 kb-F/-R) was designed to amplify a region within a 4.160-kb plasmid (see section Results) without any BLAST hits. A number of unrelated K. pneumoniae isolates including three ST15 isolates of our institute's strain collection (10 isolates with ESBL production and 10 carbapenemase-producing isolates from diverse hospitals in Germany) was chosen to evaluate the specificity of the multiplex PCR (Table S2).
WGS-Based Conduction of MLST, wzi Type, and Resistance Pattern
WGS data of all isolates were analyzed using Resfinder 2.1 (Zankari et al., 2012) and the MLST tool (Larsen et al., 2012) provided by the Centre for Genomic Epidemiology (https://cge.cbs.dtu.dk/services/). Presence of mcr-1-5 genes were tested using ResFinder 3.0. Using Geneious (Biomatters Ltd.) the contigs derived from de novo assembly using A5-miseq were checked for alterations within the porin genes ompK35 and ompK36 (possibly associated with carbapenem resistance, Martínez-Martínez, 2008), the mgrB gene, the pmrA/pmrB genes, phoQ/phoP genes, and the crrB gene (possibly associated with colistin resistance, Olaitan et al., 2014b; Wright et al., 2015; Cheng et al., 2016). The mutations observed for the colistin and carbapenem resistant isolates were deduced from NGS data of assembled contigs and confirmed by PCR and subsequent Sanger sequencing (primer sequences: Table S3). Determination of capsular type for Klebsiella strains was conducted by wzi gene sequencing extracted from contigs derived from de novo assembly (Brisse et al., 2013).
Genetic Environment of blaCTX−M−15
In order to investigate the genetic environment of blaCTX−M−15, all blaCTX−M−15-containing contigs derived from de novo assembly were aligned (Geneious). Transposases were identified using IS-Finder (https://www-is.biotoul.fr/; Siguier et al., 2006). The transposase upstream of blaCTX−M−15 in cluster 2 isolates was not completely reconstructed by de novo assembly, but by PCR amplification and subsequent Sanger-sequencing (using primers: CTX-M-15-R3: GGTATTGCCTTTCATCCATGTCACCAGC and ST405-R3: CCTTACATTTCAAAAACTCTGCTTACCAGG).
Pulsed-Field Gel-Electrophoresis (PFGE)
PFGE was performed by the National Reference Laboratory for multidrug-resistant gram-negative bacteria as previously described with some modifications (Ribot et al., 2006). Briefly, genomic DNA was digested with XbaI, the initial switch time was 5 s, the final switch time was 50 s, the run time was 20 h, the voltage was 6 V/cm and the temperature was 14°C. Results were interpreted according to the known criteria (Tenover et al., 1995). After treatment with S1 nuclease, resulting DNA fragment pattern were analyzed to predict the size and content of plasmids in the K. pneumoniae strains (Barton et al., 1995).
Results and Discussion
Setting
During April and May 2015 ESBL-producing K. pneumoniae were detected in nine different patients who just came from the intensive care unit of the neurorehabilitation clinic with overall 202 beds. Taking an average number of three ESBL-K. pneumoniae isolates per month in 2014 into account, the infection control specialists suspected an outbreak and an outbreak investigation was started. ESBL-producing K. pneumoniae isolates have been detected in 52 patients of whom 20 developed a clinical infection. All ESBL-producing Klebsiella isolates collected during the time of the outbreak contained CTX-M-15. Since April 2015, at least one case of each outbreak strain was present on five affected wards (Figure 1). No ESBL-producing K. pneumoniae were detected in the environment. Systematic case search with microbiological screening for colonized patients and cohorting of all carriers of ESBL-producing K. pneumoniae led to cessation of transmission. After the discharge of the last involved patients in May 2016, no new cases were registered.
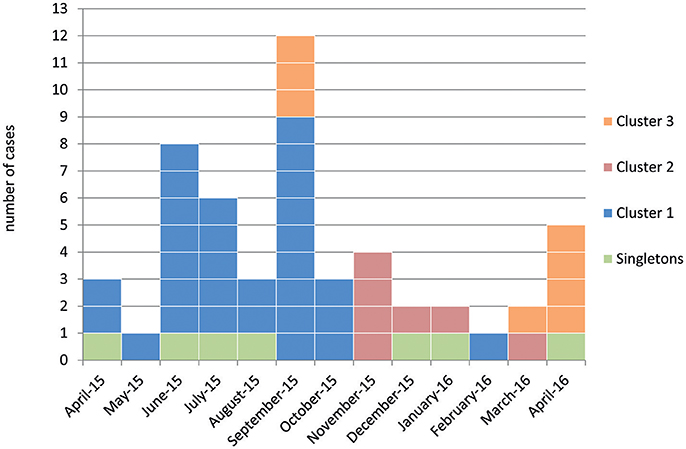
Figure 1. Isolation date of samples received from patients that were tested positive for ESBL-producing Klebsiella pneumoniae (n = 52) during the outbreak. Colors represent the three outbreak strain clusters and singletons, respectively.
Phylogenetic Analysis
Two different strategies were used to analyse the phylogenetic relations between all 53 Klebsiella isolates. First, we applied a reference-based approach by mapping sequence reads to a common reference sequence. As reference we selected K. pneumoniae PMK-1 (NZ_CP008929) using refRank (see section Materials and Methods for detailed information on reference selection; Figure S1). Second, WGS data of the single isolates were de novo assembled and compared using a MCG approach. Subsequent phylogenetic analyses revealed three clonal clusters with 46 isolates and seven unrelated isolates, in total (Figure 2A, Figure S2). Importantly, both the reference-based and -free approach assigned the isolates to the same clusters. Clustering has been also confirmed by PFGE analysis (Table 1).
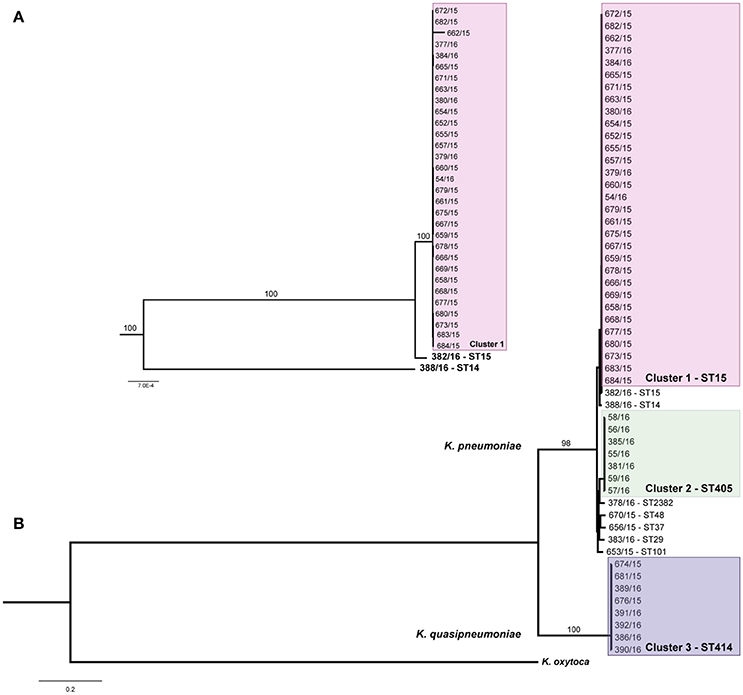
Figure 2. Phylogenetic relationship of all sequenced isolates. (B) The maximum likelihood tree was calculated based on 468,250 SNPs filtered after mapping to the reference genome. K. oxytoca KONIH1 was included to root the tree. Values on branches display support values (100 bootstraps). The three outbreak clusters are depicted by colored boxes. (A) Detailed view including support values of the cluster 1 subtree and the two ST14 and ST15 single isolates. Isolates 680/15 and 684/15 originated from the same patient.
The major cluster (cluster 1) was formed by 31 isolates belonging to ST15, cluster 2 consisted of seven isolates belonging to ST405 and cluster 3 was formed by eight isolates belonging to ST414. The latter were identified as K. quasipneumoniae subspecies similipneumoniae due to the presence of the chromosomal β-lactamase OKP-B (Fevre et al., 2005; Brisse et al., 2014). The classification as K. quasipneumoniae was confirmed by in silico analysis of the gyrA and parC genes (Brisse and Verhoef, 2001; Brisse et al., 2004; data not shown). In line with this finding, all ST414 isolates clustered separately. Among the seven non-clustered isolates, one isolate harbored a novel pgi allele and was therefore assigned to the novel sequence type ST2382 (http://bigsdb.pasteur.fr/). The other six singletons were belonging to ST15 (different from the outbreak clone), ST14, ST101, ST48, ST29, and ST37.
Cluster 1 K. pneumoniae isolates (n = 31) belonged to ST15 and were detected nearly during the whole outbreak period (from April 2015 to February 2016). In addition, cluster 1 isolates were received mainly from infections whereas cluster 2 and 3 isolates were almost exclusively collected from rectal swabs. ST15 represents a widely spread international lineage commonly associated with ESBL and carbapenemase genes (Damjanova et al., 2008; Lee et al., 2011; Breurec et al., 2013; Rodrigues et al., 2014). Correspondingly, there are several reports on outbreaks caused by ST15 isolates (Novais et al., 2012; Stoesser et al., 2014; Chung The et al., 2015; Zhou et al., 2016). As depicted in Figure 2B, there was a single isolate also being ST15 but not belonging to the outbreak cluster. While the cluster isolates harbored the capsular gene wzi93 (associated K type K60), the single isolate harbored wzi24 (associated K type K24; Brisse et al., 2013). These two types represent the two clades previously observed within the ST15 population (Bruchmann et al., 2015; Zhou et al., 2016).
Cluster 2 K. pneumoniae isolates (n = 7) belonged to ST405 and were detected from November 2015 till March 2016. ST405 also represents an internationally distributed sequence type. In the last years, carbapenemase-producing ST405 have been reported several times in various countries in Europe (e.g., in Spain, France, Italy, and Belgium) and in Yemen (Glupczynski et al., 2012; Gharout-Sait et al., 2014; Liapis et al., 2014; Del Franco et al., 2015; Palacios-Baena et al., 2016; Ruiz-Garbajosa et al., 2016). However, reports on ESBL-producing ST405 are rare; to date there is only one further report on a CTX-M-15-producing ST405 K. pneumoniae strain that has caused an outbreak in Spain (Machuca et al., 2016).
Cluster 3 isolates (n = 8) belonged to ST414, a member of the species K. quasipneumoniae, and occurred in September 2015 (n = 2) and from March till April 2016 (n = 5) in the clinic. Conspicuously, the occurrence of clinical multidrug resistant K. quasipneumoniae is far less frequently reported than the occurrence of K. pneumoniae. To the best of our knowledge no cases of K. quasipneumoniae outbreaks or clustering have been published so far. According to previous studies, K. quasipneumoniae accounts for less than 10 % of the clinical K. pneumoniae sensu lato population (Brisse et al., 2004; Maatallah et al., 2014), and hence their potential to cause outbreaks might be proportionally smaller compared to K. pneumoniae. However, during the last 2 years there were several announcements of whole genome sequenced clinical K. quasipneumoniae isolates and case reports that depict the potential of K. quasipneumoniae to cause severe infections (Arena et al., 2015; Breurec et al., 2016; Elliott et al., 2016; Garza-Ramos et al., 2016; Ozer et al., 2016). Underreporting might also be a relevant point related to K. quasipneumoniae outbreaks since routine diagnostic methods for species identification such as MALDI-TOF MS provide no reliable differentiation between K. pneumoniae, K. quasipneumoniae, and K. variicola (Long et al., 2017). Thus, identification of these species requires genotyping (Brisse et al., 2004; Alves et al., 2006; Garza-Ramos et al., 2015) and with regard to the here reported outbreak, the presence of K. quasipneumoniae isolates would not have been detected without whole genome-sequencing.
Antibiotic Resistances
Isolates of all three clusters encoded for the ESBL gene blaCTX−M−15. Further, the three β-lactamase genes blaSHV−28, blaTEM−1, and blaOXA−534, a new allele variant (NCBI accession number KX714285) were found in 26 of 31 isolates belonging to cluster 1 (Table 1). OXA-534 belongs to the OXA-1 group and thus is most likely a narrow spectrum β-lactamase. Cluster 1 isolates displayed resistance to piperacillin, cefotaxime, ceftazidime, cefepime, aztreonam, tobramycin, ciprofloxacin, moxifloxacin, and trimethoprim-sulfamethoxazole, but remained susceptible to imipenem, meropenem, and colistin. Exceptions were two cluster 1 isolates. One isolate (675/125) was resistant to colistin (Vitek 2 MIC >8 mg/L, Etest MIC 16-24 mg/L), although there was no evidence of colistin treatment of the respective patient. Since colistin susceptibility testing using Vitek2 or Etest might be less sensitive, we also performed broth microdilution for a subset of 12 isolates representing all MLST types of CTX-M-15 producing K. pneumoniae (Table S1). Only a single isolate (675/15) was colistin-resistant whereas all the other 11 isolates were colistin-susceptible (MICs between 0.125 and 0.5 mg/L). The recently described plasmid-encoded colistin resistance genes mcr-1-5 (Falgenhauer et al., 2016; Liu et al., 2016; Xavier et al., 2016; Borowiak et al., 2017) could not be detected in this isolate using ResFinder (version 3.0). The crrB gene was absent in all cluster 1 strains and thus, alterations in this gene were omitted as a possible source of colistin resistance in our case. Further, there were no alterations in the pmrB and the phoP genes in this isolate compared to the colistin-susceptible cluster 1 strains. The mgrB gene, however, showed a premature stop codon resulting in a putatively truncated protein of 29 amino acids. The same truncation of MgrB was previously detected in three colistin resistant K. pneumoniae isolates described in two studies and was reported as a probable source of the colistin resistance (Olaitan et al., 2014a; Poirel et al., 2015). Another isolate of cluster 1 (684/15) showed a non-susceptibility to carbapenem antibiotics (Etest: MIC ertapenem >32 mg/L; MIC meropenem 8 mg/L; MIC imipenem 3 mg/L). This isolate originated from a patient that was treated with meropenem (3 × 2 gram, 8 days, intravenously) after being tested positive for a cluster 1 strain isolate (680/15). Five days after the end of this treatment (= 13 days after the first meropenem dose), the carbapenem-resistant isolate was detected. No carbapenemase genes were detected in the WGS data using ResFinder. However, analysis of the porin genes ompK35 and ompK36 exhibited alterations compared to the initial isolate (680/15). The ompK35 gene was disrupted by insertion of a transposase gene (ISEcp1) after 80 nucleotides, while a single nucleotide deletion (C194-) in the ompK36 gene resulting in a frameshift and thus a preliminary stop codon at amino acid position 71. Porin loss after meropenem treatment was previously observed in Klebsiella isolates belonging to an outbreak in Spain in 2008. After 13 days of treatment, a carbapenem-resistant isolate exhibiting a point mutation resulting in a premature stop codon in the ompK36 gene was observed (López-Camacho et al., 2014). In our study, all sequenced isolates were checked for alterations in their ompK genes. There was no additional isolate with alterations in both the ompK35 and the ompK36 genes, but three isolates revealed one single mutated gene each (Table 1). The detected mutations did not influence the carbapenem resistance phenotype or resulted only in slightly increased carbapenem MIC values, respectively (Table S1). This is in concordance with the assumption that increased resistance requires loss of both porins OmpK35 and OmpK36 (Martínez-Martínez, 2008).
Plasmid Content and the Genetic Environment of blaCTX−M−15
De novo assemblies of Illumina raw reads revealed presence of three small plasmids in all but three cluster 1 isolates. Interestingly, two of these small plasmids were virtually identical to published plasmids. The 3,223 kb-plasmid shared 3,222/3,223 bp with the Citrobacter freundii-plasmid pCAV1321-3223 which encodes the multidrug transporter EmrE (Genbank accession: CP011604). This plasmid seems to be very stable and widespread since the isolate which contained the published plasmid had been collected in Virginia/USA in 2010 (Sheppard et al., 2016), and more plasmids of the same size and with a maximum difference of three nucleotides have been reported from various genera, including Serratia (CP011637), Salmonella (CP016867), Enterobacter (KU302804, CP011569, CP011658), and Klebsiella (CP003994, CP01299, CP014305). The 3.559 kb-plasmid shared 3,558/3,559 bp with the K. pneumoniae-plasmid pKp_Goe_917-7 (CP018446). The isolate that contained the published plasmid originates, like the isolates of this study, from a German hospital but was collected about 2 years earlier, in 2013. As the annotated genes encode either proteins involved in plasmid replication (replication initiation protein) or hypothetical proteins, conclusions on function and the potential benefit of carrying this plasmid require further analyses. In order to determine the content and the size of plasmids >30 kb, S1 nuclease digestion and subsequent PFGE analysis were exemplarily performed for three cluster 1 isolates. All three isolates revealed presence of plasmids which, however, differed in their content (data not shown). Isolate 652/15 contained one plasmid (approximately 200 kb), isolate 662/15 contained two plasmids (approximately 70 and 200 kb), and isolate 666/15 harbored one plasmid (approximately 230 kb). Thus, there was no evidence for an identical (blaCTX-M-15-carrying) plasmid harbored by all cluster 1 isolates. Although blaCTX-M-15 is primarily found on plasmids, there are also reports of chromosomal localizations (Coelho et al., 2010; Mshana et al., 2015). To investigate the localization of blaCTX-M-15 in the outbreak isolates, the genetic environment of the ESBL gene was compared (Figure S3).
All isolates belonging to cluster 1 and cluster 3 showed an ISEcp1 transposase gene (identical to accession AJ242809) upstream of blaCTX-M-15. In contrast, all cluster 2 isolates revealed presence of an IS15DIV transposase gene (identical to X13616) upstream of blaCTX-M-15. In most cases the assembled contigs were too small to resolve whether the blaCTX-M-15 gene was located on a plasmid or on the chromosome. However, for 11 isolates of cluster 1 blaCTX-M-15 was found on a contig that also contained the chromosomal encoded dnaA gene, arguing for a chromosomal integration. In contrast, in one of the first sampled isolates (652/15) the blaCTX-M-15 gene was located on the same contig as the plasmid encoded replication initiation gene repB (IncR1; Figure S3). The possible existence of both localizations in cluster 1 isolates suggests either a mobilization of the blaCTX-M-15 during the outbreak or the simultaneous occurrence of both, a chromosomal copy and a plasmid encoded copy of blaCTX-M-15, as recently described for K. pneumoniae (Hudson et al., 2014; Zhou et al., 2015).
A Selective PCR Set Successfully Identifies Isolates Belonging to Cluster 1
Since cluster 1 represented the dominant clone during almost the whole outbreak period, an introduction by re-admittance of colonized patients is imaginable. Therefore, a specific multiplex PCR was established allowing rapid detection of emerging cluster 1 isolates to support the hospital laboratory routine (Figure 3). Based on whole genome data of the primarily sequenced 32 isolates, whereof 27 were found to belong to cluster 1, three primer pairs were designed (hem-F/-R, unique-F/-R, and 4.160 kb-F/-R, see section Materials and Methods and Table 2). Isolates revealing presence of all three PCR products were considered to belong to the outbreak cluster/clone (Figure 3A). Isolates with presence of both the hem and the unique PCR product were considered as probable outbreak isolates since the third primer pair amplifies a plasmid encoded gene and, thus, the absence of the product might be a result of plasmid loss or structural alterations. The latter was the case for cluster 1 isolate 684/15. The PCR succeeded in classifying all further isolates, i.e., the PCR results corresponded with the results of the subsequent whole-genome-analysis (Figure 3B). In order to provide proof of the specificity of the multiplex PCR, 20 unrelated K. pneumoniae isolates (Table S2) were tested and results are given in Figure 3C. None of the isolates tested revealed presence of all three bands. The three ST15 isolates showed amplification of the hem product, but the absence of the other two targets allowed to discriminate between unrelated ST15 isolates and cluster 1 isolates. We therefore conclude that the designed triplex PCR is a reliable tool for the identification of cluster 1 isolates and suggest for similar situations a combination of two to three discriminating targets for increasing the specificity of a corresponding diagnostic or screening test. Furthermore, the amplification of the hem product in all tested ST15 isolates and the absence in all other isolates supports the idea of hem-F/-R as an ST15-specific primer pair. The report of a WGS-based analysis of a K. pneumoniae outbreak in a Dutch hospital was published during the outbreak study reported here. The Dutch study included reconstruction of transmission routes and identification of virulence and antibiotic resistance genes, but whole genome sequence data was also used to develop an outbreak-specific triplex PCR (Zhou et al., 2016). Despite differences in the approach applied for the PCR set-up, both studies illustrate the potential of WGS to identify signature regions in outbreak strains.
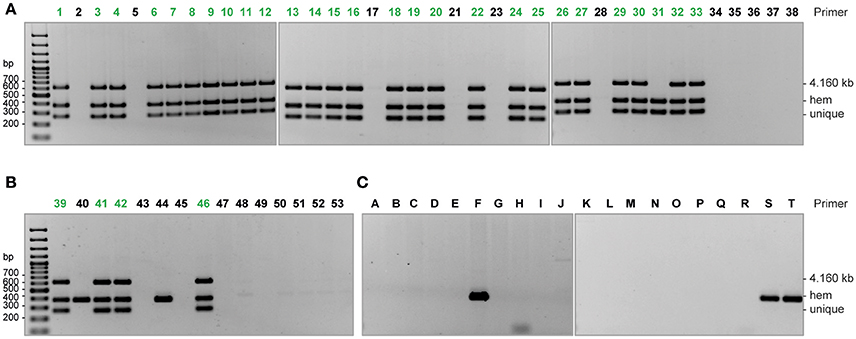
Figure 3. Gel electrophoresis of the cluster 1 specific PCR products (inverted image). PCR products (3 μl) were separated on 1.4% agarose gels. Isolates belonging to the major outbreak strain (cluster 1) are highlighted in green. (A) Clinical isolates from the neurorehabilitation clinic that were used to setup the PCR. (B) Further clinical isolates that occurred from June 2015 till April 2016 in the neurorehabilitation clinic, and were PCR-typed prior WGS. (C) Ten epidemiologically independent ESBL-producing (A–J) and carbapenemase-producing (K–T) K. pneumoniae isolates, respectively (see Table S3), used to check the specificity of the PCR. Interpretation: all three bands present: isolate belongs to cluster 1 (“outbreak strain”); all bands present but PCR product of 4,160 kb missing: isolate probably belongs to cluster 1; no PCR product or only amplification of the hem product: isolate does not belong to cluster 1.
Conclusions
WGS-based analysis allowed the successful elucidation of a one-year outbreak with CTX-M-15 producing K. pneumoniae in a neurorehabilitation center. Using NGS data and two independent bioinformatics' strategies, we were capable of dividing the scenario into three different outbreak clusters of CTX-M-15 producing Klebsiella strain types including one cluster caused by isolates of K. quasipneumoniae. We performed comprehensive database searches and genomic comparisons to finally deduce a cluster-specific triplex PCR for the main outbreak strain type which (i) was further validated on a valuable set of test and wildtype strains and (ii) allowed a real-time allocation of new Klebsiella cases to outbreak and non-outbreak isolates. We did not find experimental proof for a horizontally acquired CTX-M-15 element among strains of the three different WGS clusters considering the different genetic environments as deduced from NGS data. In addition, WGS analysis allowed predicting important antibiotic resistance phenotypes for colistin and carbapenem non-susceptibilities.
Additional Requirements
Sequence data were submitted to the European Nucleotide Archive (http://www.ebi.ac.uk/ena) and are available under study accession number PRJEB18059.
Ethics Statement
No informed consent or ethical approval was required since all isolates were generated and analyzed as part of microbiological diagnostics (therapeutic purposes) and/or infection prevention and control requirements and measures. The outbreak investigation was conducted in accordance with article 25, section 1 of the German Infection Protection Act of 2001.
Author Contributions
LB, SF, YP, SG, HR, and GW: designed the experiments. LB, SF, YP, and TS: performed the experiments. LB, SF, YP, TS, GK, SH, TE, MK, and SG: collected and analyzed the data. LB, MS, TS, and SF: performed bioinformatic analysis. MS and SF: designed and created the Python applications. TE, GK, DS, MF, EZ, CD, M-LT, HR, and SH: were part of the outbreak team, conducted the on-site investigations, implemented control measures, and systematically collected the samples. LB, SF, YP, and GW: wrote the manuscript. All authors reviewed the manuscript.
Funding
This project has received funding from the German Federal Ministry of Health (IIA5-2513NIK006/321-4471-02/129) and from the EU project COMPARE (http://www.compare-europe.eu).
Conflict of Interest Statement
The authors declare that the research was conducted in the absence of any commercial or financial relationships that could be construed as a potential conflict of interest.
Acknowledgments
We thank Kirstin Ganske and Sibylle Müller-Bertling for excellent technical assistance. We thank the team of curators of the Institut Pasteur MLST and whole genome MLST databases for curating the data and making them publicly available (http://bigsdb.pasteur.fr/). We would like to thank Hans-Peter Blank and Jan Walter for data extraction and their support in the outbreak investigation. The authors want to thank the personnel of the clinic, without whom outbreak investigation and control would not have been possible. They want to thank all other members of the outbreak investigation team for their support.
Supplementary Material
The Supplementary Material for this article can be found online at: https://www.frontiersin.org/articles/10.3389/fmicb.2018.00322/full#supplementary-material
References
Altschul, S. F., Gish, W., Miller, W., Myers, E. W., and Lipman, D. J. (1990). Basic local alignment search tool. J. Mol. Biol. 215, 403–410. doi: 10.1016/S0022-2836(05)80360-2
Alves, M. S., Dias, R. C., de Castro, A. C., Riley, L. W., and Moreira, B. M. (2006). Identification of clinical isolates of indole-positive and indole-negative Klebsiella spp. J. Clin. Microbiol. 44, 3640–3646. doi: 10.1128/JCM.00940-06
Arena, F., Henrici De Angelis, L., Pieralli, F., Di Pilato, V., Giani, T., Torricelli, F., et al. (2015). Draft genome sequence of the first hypermucoviscous Klebsiella quasipneumoniae subsp. quasipneumoniae isolate from a bloodstream infection. Genome Announc. 3:e00952-15. doi: 10.1128/genomeA.00952-15
Arndt, D., Grant, J. R., Marcu, A., Sajed, T., Pon, A., Liang, Y., et al. (2016). PHASTER: a better, faster version of the PHAST phage search tool. Nucleic Acids Res. 44, W16–W21. doi: 10.1093/nar/gkw387
Barton, B. M., Harding, G. P., and Zuccarelli, A. J. (1995). A general method for detecting and sizing large plasmids. Anal. Biochem. 226, 235–240. doi: 10.1006/abio.1995.1220
Bialek-Davenet, S., Criscuolo, A., Ailloud, F., Passet, V., Nicolas-Chanoine, M. H., Decre, D., et al. (2014). Development of a multiplex PCR assay for identification of Klebsiella pneumoniae hypervirulent clones of capsular serotype K2. J. Med. Microbiol. 63(Pt 12), 1608–1614. doi: 10.1099/jmm.0.081448-0
Bolger, A. M., Lohse, M., and Usadel, B. (2014). Trimmomatic: a flexible trimmer for Illumina sequence data. Bioinformatics 30, 2114–2120. doi: 10.1093/bioinformatics/btu170
Borowiak, M., Fischer, J., Hammerl, J. A., Hendriksen, R. S., Szabo, I., and Malorny, B. (2017). Identification of a novel transposon-associated phosphoethanolamine transferase gene, mcr-5, conferring colistin resistance in d-tartrate fermenting Salmonella enterica subsp. enterica serovar Paratyphi B. J. Antimicrob. Chemother. 72, 3317–3324. doi: 10.1093/jac/dkx327
Breurec, S., Guessennd, N., Timinouni, M., Le, T. A., Cao, V., Ngandjio, A., et al. (2013). Klebsiella pneumoniae resistant to third-generation cephalosporins in five African and two Vietnamese major towns: multiclonal population structure with two major international clonal groups, CG15 and CG258. Clin. Microbiol. Infect. 19, 349–355. doi: 10.1111/j.1469-0691.2012.03805.x
Breurec, S., Melot, B., Hoen, B., Passet, V., Schepers, K., Bastian, S., et al. (2016). Liver abscess caused by infection with community-acquired Klebsiella quasipneumoniae subsp. quasipneumoniae. Emerg. Infect. Dis. 22, 529–531. doi: 10.3201/eid2203.151466
Brisse, S., and Verhoef, J. (2001). Phylogenetic diversity of Klebsiella pneumoniae and Klebsiella oxytoca clinical isolates revealed by randomly amplified polymorphic DNA, gyrA and parC genes sequencing and automated ribotyping. Int. J. Syst. Evol. Microbiol. 51(Pt 3), 915–924. doi: 10.1099/00207713-51-3-915
Brisse, S., Passet, V., and Grimont, P. A. (2014). Description of Klebsiella quasipneumoniae sp. nov., isolated from human infections, with two subspecies, Klebsiella quasipneumoniae subsp. quasipneumoniae subsp. nov. and Klebsiella quasipneumoniae subsp. similipneumoniae subsp. nov., and demonstration that Klebsiella singaporensis is a junior heterotypic synonym of Klebsiella variicola. Int. J. Syst. Evol. Microbiol. 64(Pt 9), 3146–3152. doi: 10.1099/ijs.0.062737-0
Brisse, S., Passet, V., Haugaard, A. B., Babosan, A., Kassis-Chikhani, N., Struve, C., et al. (2013). wzi Gene sequencing, a rapid method for determination of capsular type for Klebsiella strains. J. Clin. Microbiol. 51, 4073–4078. doi: 10.1128/JCM.01924-13
Brisse, S., van Himbergen, T., Kusters, K., and Verhoef, J. (2004). Development of a rapid identification method for Klebsiella pneumoniae phylogenetic groups and analysis of 420 clinical isolates. Clin. Microbiol. Infect. 10, 942–945. doi: 10.1111/j.1469-0691.2004.00973.x
Bruchmann, S., Muthukumarasamy, U., Pohl, S., Preusse, M., Bielecka, A., Nicolai, T., et al. (2015). Deep transcriptome profiling of clinical Klebsiella pneumoniae isolates reveals strain and sequence type-specific adaptation. Environ. Microbiol. 17, 4690–4710. doi: 10.1111/1462-2920.13016
Calbo, E., and Garau, J. (2015). The changing epidemiology of hospital outbreaks due to ESBL-producing Klebsiella pneumoniae: the CTX-M-15 type consolidation. Future Microbiol. 10, 1063–1075. doi: 10.2217/fmb.15.22
Cheng, Y. H., Lin, T. L., Lin, Y. T., and Wang, J. T. (2016). Amino acid substitutions of CrrB responsible for resistance to colistin through CrrC in Klebsiella pneumoniae. Antimicrob. Agents Chemother. 60, 3709–3716. doi: 10.1128/AAC.00009-16
Chung The, H., Karkey, A., Pham Thanh, D., Boinett, C. J., Cain, A. K., Ellington, M., et al. (2015). A high-resolution genomic analysis of multidrug-resistant hospital outbreaks of Klebsiella pneumoniae. EMBO Mol. Med. 7, 227–239. doi: 10.15252/emmm.201404767
Coelho, A., González-López, J. J., Miro, E., Alonso-Tarres, C., Mirelis, B., Larrosa, M. N., et al. (2010). Characterisation of the CTX-M-15-encoding gene in Klebsiella pneumoniae strains from the Barcelona metropolitan area: plasmid diversity and chromosomal integration. Int. J. Antimicrob. Agents 36, 73–78. doi: 10.1016/j.ijantimicag.2010.03.005
Coil, D., Jospin, G., and Darling, A. E. (2015). A5-miseq: an updated pipeline to assemble microbial genomes from Illumina MiSeq data. Bioinformatics 31, 587–589. doi: 10.1093/bioinformatics/btu661
Damjanova, I., Tóth, A., Paszti, J., Hajbel-Vekony, G., Jakab, M., Berta, J., et al. (2008). Expansion and countrywide dissemination of ST11, ST15 and ST147 ciprofloxacin-resistant CTX-M-15-type beta-lactamase-producing Klebsiella pneumoniae epidemic clones in Hungary in 2005–the new ‘MRSAs’? J. Antimicrob. Chemother. 62, 978–985. doi: 10.1093/jac/dkn287
Del Franco, M., Paone, L., Novati, R., Giacomazzi, C. G., Bagattini, M., Galotto, C., et al. (2015). Molecular epidemiology of carbapenem resistant Enterobacteriaceae in Valle d'Aosta region, Italy, shows the emergence of KPC-2 producing Klebsiella pneumoniae clonal complex 101 (ST101 and ST1789). BMC Microbiol. 15:260. doi: 10.1186/s12866-015-0597-z
Edgar, R. C. (2004). MUSCLE: multiple sequence alignment with high accuracy and high throughput. Nucleic Acids Res. 32, 1792–1797. doi: 10.1093/nar/gkh340
Edgar, R. C. (2010). Search and clustering orders of magnitude faster than BLAST. Bioinformatics 26, 2460–2461. doi: 10.1093/bioinformatics/btq461
Elliott, A. G., Ganesamoorthy, D., Coin, L., Cooper, M. A., and Cao, M. D. (2016). Complete genome sequence of Klebsiella quasipneumoniae subsp. similipneumoniae strain ATCC 700603. Genome Announc. 4:e00438-16. doi: 10.1128/genomeA.00438-16
Falgenhauer, L., Waezsada, S. E., Yao, Y., Imirzalioglu, C., Kasbohrer, A., Roesler, U., et al. (2016). Colistin resistance gene mcr-1 in extended-spectrum beta-lactamase-producing and carbapenemase-producing Gram-negative bacteria in Germany. Lancet Infect. Dis. 16, 282–283. doi: 10.1016/S1473-3099(16)00009-8
Fevre, C., Passet, V., Weill, F. X., Grimont, P. A., and Brisse, S. (2005). Variants of the Klebsiella pneumoniae OKP chromosomal beta-lactamase are divided into two main groups, OKP-A and OKP-B. Antimicrob. Agents Chemother. 49, 5149–5152. doi: 10.1128/AAC.49.12.5149-5152.2005
Garza-Ramos, U., Silva-Sanchez, J., Catalan-Najera, J., Barrios, H., Rodriguez-Medina, N., Garza-Gonzalez, E., et al. (2016). Draft genome sequence of a hypermucoviscous extended-spectrum-beta-lactamase-producing Klebsiella quasipneumoniae subsp. similipneumoniae clinical Isolate. Genome Announc. 4:e00475-16. doi: 10.1128/genomeA.00475-16
Garza-Ramos, U., Silva-Sanchez, J., Martinez-Romero, E., Tinoco, P., Pina-Gonzales, M., Barrios, H., et al. (2015). Development of a multiplex-PCR probe system for the proper identification of Klebsiella variicola. BMC Microbiol. 15:64. doi: 10.1186/s12866-015-0396-6
Gharout-Sait, A., Alsharapy, S. A., Brasme, L., Touati, A., Kermas, R., Bakour, S., et al. (2014). Enterobacteriaceae isolates carrying the New Delhi metallo-beta-lactamase gene in Yemen. J. Med. Microbiol. 63(Pt 10), 1316–1323. doi: 10.1099/jmm.0.073767-0
Glupczynski, Y., Huang, T. D., Bouchahrouf, W., Rezende de Castro, R., Bauraing, C., Gerard, M., et al. (2012). Rapid emergence and spread of OXA-48-producing carbapenem-resistant Enterobacteriaceae isolates in Belgian hospitals. Int. J. Antimicrob. Agents 39, 168–172. doi: 10.1016/j.ijantimicag.2011.10.005
Gupta, A., Ampofo, K., Rubenstein, D., and Saiman, L. (2003). Extended spectrum beta lactamase-producing Klebsiella pneumoniae infections: a review of the literature. J. Perinatol. 23, 439–443. doi: 10.1038/sj.jp.7210973
Haeggman, S., Lofdahl, S., Paauw, A., Verhoef, J., and Brisse, S. (2004). Diversity and evolution of the class A chromosomal beta-lactamase gene in Klebsiella pneumoniae. Antimicrob. Agents Chemother. 48, 2400–2408. doi: 10.1128/AAC.48.7.2400-2408.2004
Haller, S., Eller, C., Hermes, J., Kaase, M., Steglich, M., Radonic, A., et al. (2015). What caused the outbreak of ESBL-producing Klebsiella pneumoniae in a neonatal intensive care unit, Germany 2009 to 2012? Reconstructing transmission with epidemiological analysis and whole-genome sequencing. BMJ Open 5:e007397. doi: 10.1136/bmjopen-2014-007397
Huang, W., Li, L., Myers, J. R., and Marth, G. T. (2012). ART: a next-generation sequencing read simulator. Bioinformatics 28, 593–594. doi: 10.1093/bioinformatics/btr708
Hudson, C. M., Bent, Z. W., Meagher, R. J., and Williams, K. P. (2014). Resistance determinants and mobile genetic elements of an NDM-1-encoding Klebsiella pneumoniae strain. PLoS ONE 9:e99209. doi: 10.1371/journal.pone.0099209
Hyatt, D., Chen, G. L., Locascio, P. F., Land, M. L., Larimer, F. W., and Hauser, L. J. (2010). Prodigal: prokaryotic gene recognition and translation initiation site identification. BMC Bioinformatics 11:119. doi: 10.1186/1471-2105-11-119
Kearse, M., Moir, R., Wilson, A., Stones-Havas, S., Cheung, M., Sturrock, S., et al. (2012). Geneious Basic: an integrated and extendable desktop software platform for the organization and analysis of sequence data. Bioinformatics 28, 1647–1649. doi: 10.1093/bioinformatics/bts199
Koboldt, D. C., Zhang, Q., Larson, D. E., Shen, D., McLellan, M. D., Lin, L., et al. (2012). VarScan 2: somatic mutation and copy number alteration discovery in cancer by exome sequencing. Genome Res. 22, 568–576. doi: 10.1101/gr.129684.111
Larsen, M. V., Cosentino, S., Rasmussen, S., Friis, C., Hasman, H., Marvig, R. L., et al. (2012). Multilocus sequence typing of total-genome-sequenced bacteria. J. Clin. Microbiol. 50, 1355–1361. doi: 10.1128/JCM.06094-11
Lee, M. Y., Ko, K. S., Kang, C. I., Chung, D. R., Peck, K. R., and Song, J. H. (2011). High prevalence of CTX-M-15-producing Klebsiella pneumoniae isolates in Asian countries: diverse clones and clonal dissemination. Int. J. Antimicrob. Agents 38, 160–163. doi: 10.1016/j.ijantimicag.2011.03.020
Li, H., and Durbin, R. (2009). Fast and accurate short read alignment with burrows-wheeler transform. Bioinformatics 25, 1754–1760. doi: 10.1093/bioinformatics/btp324
Li, H., Handsaker, B., Wysoker, A., Fennell, T., Ruan, J., Homer, N., et al. (2009). The sequence alignment/map format and SAMtools. Bioinformatics 25, 2078–2079. doi: 10.1093/bioinformatics/btp352
Liapis, E., Pantel, A., Robert, J., Nicolas-Chanoine, M. H., Cavalie, L., van der Mee-Marquet, N., et al. (2014). Molecular epidemiology of OXA-48-producing Klebsiella pneumoniae in France. Clin. Microbiol. Infect. 20, O1121–O1123. doi: 10.1111/1469-0691.12727
Liu, Y. Y., Wang, Y., Walsh, T. R., Yi, L. X., Zhang, R., Spencer, J., et al. (2016). Emergence of plasmid-mediated colistin resistance mechanism MCR-1 in animals and human beings in China: a microbiological and molecular biological study. Lancet Infect. Dis. 16, 161–168. doi: 10.1016/S1473-3099(15)00424-7
Long, S. W., Linson, S. E., Ojeda Saavedra, M., Cantu, C., Davis, J. J., Brettin, T., et al. (2017). Whole-genome sequencing of human clinical Klebsiella pneumoniae isolates reveals misidentification and misunderstandings of Klebsiella pneumoniae, Klebsiella variicola, and Klebsiella quasipneumoniae. mSphere 2:e00290-17. doi: 10.1128/mSphereDirect.00290-17
López-Camacho, E., Gomez-Gil, R., Tobes, R., Manrique, M., Lorenzo, M., Galvan, B., et al. (2014). Genomic analysis of the emergence and evolution of multidrug resistance during a Klebsiella pneumoniae outbreak including carbapenem and colistin resistance. J. Antimicrob. Chemother. 69, 632–636. doi: 10.1093/jac/dkt419
Maatallah, M., Vading, M., Kabir, M. H., Bakhrouf, A., Kalin, M., Naucler, P., et al. (2014). Klebsiella variicola is a frequent cause of bloodstream infection in the stockholm area, and associated with higher mortality compared to K. pneumoniae. PLoS ONE 9:e113539. doi: 10.1371/journal.pone.0113539
Machuca, J., López-Cerero, L., Fernandez-Cuenca, F., Gracia-Ahufinger, I., Ruiz-Carrascoso, G., Rodriguez-Lopez, F., et al. (2016). Characterization of an outbreak due to CTX-M-15-producing Klebsiella pneumoniae lacking the blaOXA-48 gene belonging to clone ST405 in a neonatal unit in southern Spain. J. Antimicrob. Chemother. 71, 2353–2355. doi: 10.1093/jac/dkw137
Martínez-Martínez, L. (2008). Extended-spectrum beta-lactamases and the permeability barrier. Clin. Microbiol. Infect. 14(Suppl. 1), 82–89. doi: 10.1111/j.1469-0691.2007.01860.x
Mshana, S. E., Fritzenwanker, M., Falgenhauer, L., Domann, E., Hain, T., Chakraborty, T., et al. (2015). Molecular epidemiology and characterization of an outbreak causing Klebsiella pneumoniae clone carrying chromosomally located bla(CTX-M-15) at a German University-Hospital. BMC Microbiol. 15:122. doi: 10.1186/s12866-015-0460-2
Nguyen, V. H., and Lavenier, D. (2009). PLAST: parallel local alignment search tool for database comparison. BMC Bioinformatics 10:329. doi: 10.1186/1471-2105-10-329
Novais, A., Rodrigues, C., Branquinho, R., Antunes, P., Grosso, F., Boaventura, L., et al. (2012). Spread of an OmpK36-modified ST15 Klebsiella pneumoniae variant during an outbreak involving multiple carbapenem-resistant Enterobacteriaceae species and clones. Eur. J. Clin. Microbiol. Infect. Dis. 31, 3057–3063. doi: 10.1007/s10096-012-1665-z
Olaitan, A. O., Diene, S. M., Kempf, M., Berrazeg, M., Bakour, S., Gupta, S. K., et al. (2014a). Worldwide emergence of colistin resistance in Klebsiella pneumoniae from healthy humans and patients in Lao PDR, Thailand, Israel, Nigeria and France owing to inactivation of the PhoP/PhoQ regulator mgrB: an epidemiological and molecular study. Int. J. Antimicrob. Agents 44, 500–507. doi: 10.1016/j.ijantimicag.2014.07.020
Olaitan, A. O., Morand, S., and Rolain, J.-M. (2014b). Mechanisms of polymyxin resistance: acquired and intrinsic resistance in bacteria. Front. Microbiol. 5:643. doi: 10.3389/fmicb.2014.00643
Ozer, E. A., Morris, A. R., Krapp, F., Henry, C. S., Tyo, K. E., Lathem, W. W., et al. (2016). Draft genome sequence of a multidrug-resistant Klebsiella quasipneumoniae subsp. similipneumoniae isolate from a clinical source. Genome Announc. 4:e00422-16. doi: 10.1128/genomea.00422-16
Palacios-Baena, Z. R., Oteo, J., Conejo, C., Larrosa, M. N., Bou, G., Fernandez-Martinez, M., et al. (2016). Comprehensive clinical and epidemiological assessment of colonisation and infection due to carbapenemase-producing Enterobacteriaceae in Spain. J. Infect. 72, 152–160. doi: 10.1016/j.jinf.2015.10.008
Podschun, R., and Ullmann, U. (1998). Klebsiella spp. as nosocomial pathogens: epidemiology, taxonomy, typing methods, and pathogenicity factors. Clin. Microbiol. Rev. 11, 589–603.
Poirel, L., Jayol, A., Bontron, S., Villegas, M. V., Ozdamar, M., Turkoglu, S., et al. (2015). The mgrB gene as a key target for acquired resistance to colistin in Klebsiella pneumoniae. J. Antimicrob. Chemother. 70, 75–80. doi: 10.1093/jac/dku323
Quainoo, S., Coolen, J. P. M., van Hijum, S., Huynen, M. A., Melchers, W. J. G., van Schaik, W., et al. (2017). Whole-genome sequencing of bacterial pathogens: the future of nosocomial outbreak analysis. Clin. Microbiol. Rev. 30, 1015–1063. doi: 10.1128/CMR.00016-17
Ribot, E. M., Fair, M. A., Gautom, R., Cameron, D. N., Hunter, S. B., Swaminathan, B., et al. (2006). Standardization of pulsed-field gel electrophoresis protocols for the subtyping of Escherichia coli O157:H7, salmonella, and shigella for pulsenet. Foodborne Pathog. Dis. 3, 59–67. doi: 10.1089/fpd.2006.3.59
Rodrigues, C., Machado, E., Ramos, H., Peixe, L., and Novais, A. (2014). Expansion of ESBL-producing Klebsiella pneumoniae in hospitalized patients: a successful story of international clones (ST15, ST147, ST336) and epidemic plasmids (IncR, IncFIIK). Int. J. Med. Microbiol. 304, 1100–1108. doi: 10.1016/j.ijmm.2014.08.003
Rosenblueth, M., Martinez, L., Silva, J., and Martinez-Romero, E. (2004). Klebsiella variicola, a novel species with clinical and plant-associated isolates. Syst. Appl. Microbiol. 27, 27–35. doi: 10.1078/0723-2020-00261
Ruiz-Garbajosa, P., Hernandez-Garcia, M., Beatobe, L., Tato, M., Mendez, M. I., Grandal, M., et al. (2016). A single-day point-prevalence study of faecal carriers in long-term care hospitals in Madrid (Spain) depicts a complex clonal and polyclonal dissemination of carbapenemase-producing Enterobacteriaceae. J. Antimicrob. Chemother. 71, 348–352. doi: 10.1093/jac/dkv355
Sheppard, A. E., Stoesser, N., Wilson, D. J., Sebra, R., Kasarskis, A., Anson, L. W., et al. (2016). Nested Russian doll-like genetic mobility drives rapid dissemination of the carbapenem resistance gene blaKPC. Antimicrob. Agents Chemother. 60, 3767–3778. doi: 10.1128/AAC.00464-16
Siguier, P., Perochon, J., Lestrade, L., Mahillon, J., and Chandler, M. (2006). ISfinder: the reference centre for bacterial insertion sequences. Nucleic Acids Res. 34, D32–D36. doi: 10.1093/nar/gkj014
Stamatakis, A. (2014). RAxML version 8: a tool for phylogenetic analysis and post-analysis of large phylogenies. Bioinformatics 30, 1312–1313. doi: 10.1093/bioinformatics/btu033
Stapleton, P. J., Murphy, M., McCallion, N., Brennan, M., Cunney, R., and Drew, R. J. (2016). Outbreaks of extended spectrum beta-lactamase-producing Enterobacteriaceae in neonatal intensive care units: a systematic review. Arch. Dis. Child. Fetal Neonatal ed. 101, F72–F78. doi: 10.1136/archdischild-2015-308707
Stoesser, N., Giess, A., Batty, E. M., Sheppard, A. E., Walker, A. S., Wilson, D. J., et al. (2014). Genome sequencing of an extended series of NDM-Klebsiella pneumoniae neonatal infections in a Nepali hospital characterizes the extent of community versus hospital-associated transmission in an endemic setting. Antimicrob. Agents Chemother. 58, 7347–7357. doi: 10.1128/AAC.03900-14
Tenover, F. C., Arbeit, R. D., Goering, R. V., Mickelsen, P. A., Murray, B. E., Persing, D. H., et al. (1995). Interpreting chromosomal DNA restriction patterns produced by pulsed-field gel electrophoresis: criteria for bacterial strain typing. J. Clin. Microbiol. 33, 2233–2239.
Valverde, A., Coque, T. M., Garcia-San Miguel, L., Baquero, F., and Canton, R. (2008). Complex molecular epidemiology of extended-spectrum beta-lactamases in Klebsiella pneumoniae: a long-term perspective from a single institution in Madrid. J. Antimicrob. Chemother. 61, 64–72. doi: 10.1093/jac/dkm403
von Mentzer, A., Connor, T. R., Wieler, L. H., Semmler, T., Iguchi, A., Thomson, N. R., et al. (2014). Identification of enterotoxigenic Escherichia coli (ETEC) clades with long-term global distribution. Nat. Genet. 46, 1321–1326. doi: 10.1038/ng.3145
Wright, M. S., Suzuki, Y., Jones, M. B., Marshall, S. H., Rudin, S. D., van Duin, D., et al. (2015). Genomic and transcriptomic analyses of colistin-resistant clinical isolates of Klebsiella pneumoniae reveal multiple pathways of resistance. Antimicrob. Agents Chemother. 59, 536–543. doi: 10.1128/AAC.04037-14
Xavier, B. B., Lammens, C., Ruhal, R., Kumar-Singh, S., Butaye, P., Goossens, H., et al. (2016). Identification of a novel plasmid-mediated colistin-resistance gene, mcr-2, in Escherichia coli, Belgium, June 2016. Euro Surveill. 21:30280. doi: 10.2807/1560-7917.ES.2016.21.27.30280
Zankari, E., Hasman, H., Cosentino, S., Vestergaard, M., Rasmussen, S., Lund, O., et al. (2012). Identification of acquired antimicrobial resistance genes. J. Antimicrob. Chemother. 67, 2640–2644. doi: 10.1093/jac/dks261
Zhou, K., Lokate, M., Deurenberg, R. H., Arends, J., Lo-Ten Foe, J., Grundmann, H., et al. (2015). Characterization of a CTX-M-15 producing Klebsiella Pneumoniae outbreak strain assigned to a novel sequence type (1427). Front. Microbiol. 6:1250. doi: 10.3389/fmicb.2015.01250
Zhou, K., Lokate, M., Deurenberg, R. H., Tepper, M., Arends, J. P., Raangs, E. G., et al. (2016). Use of whole-genome sequencing to trace, control and characterize the regional expansion of extended-spectrum beta-lactamase producing ST15 Klebsiella pneumoniae. Sci. Rep. 6:20840. doi: 10.1038/srep20840
Keywords: ESBL, outbreak analysis, NGS, strain typing, CTX-M-15
Citation: Becker L, Fuchs S, Pfeifer Y, Semmler T, Eckmanns T, Korr G, Sissolak D, Friedrichs M, Zill E, Tung M-L, Dohle C, Kaase M, Gatermann S, Rüssmann H, Steglich M, Haller S and Werner G (2018) Whole Genome Sequence Analysis of CTX-M-15 Producing Klebsiella Isolates Allowed Dissecting a Polyclonal Outbreak Scenario. Front. Microbiol. 9:322. doi: 10.3389/fmicb.2018.00322
Received: 26 September 2017; Accepted: 12 February 2018;
Published: 23 February 2018.
Edited by:
Teresa M. Coque, Instituto Ramón y Cajal de Investigación Sanitaria, SpainReviewed by:
Ørjan Samuelsen, University Hospital of North Norway, NorwayAngela Novais, Universidade do Porto, Portugal
Copyright © 2018 Becker, Fuchs, Pfeifer, Semmler, Eckmanns, Korr, Sissolak, Friedrichs, Zill, Tung, Dohle, Kaase, Gatermann, Rüssmann, Steglich, Haller and Werner. This is an open-access article distributed under the terms of the Creative Commons Attribution License (CC BY). The use, distribution or reproduction in other forums is permitted, provided the original author(s) and the copyright owner are credited and that the original publication in this journal is cited, in accordance with accepted academic practice. No use, distribution or reproduction is permitted which does not comply with these terms.
*Correspondence: Guido Werner, wernerg@rki.de
†Present Address: Martin Kaase, Division of Infection Control and Infectious Diseases, University Medical Center Göttingen, Göttingen, Germany