- 1Department of Pulmonary and Critical Care Medicine, Air Force General Hospital of PLA, Beijing, China
- 2Department of Infection Management and Disease Control, Chinese PLA General Hospital, Beijing, China
- 3Department of Respiratory Medicine, Chinese PLA General Hospital, Beijing, China
- 4Department of Clinical Pharmacology, Chinese PLA General Hospital, Beijing, China
Stenotrophomonas maltophilia is an emerging nosocomial pathogen with high resistance to most clinically used antimicrobials. Tigecycline is a potential alternative antimicrobial for S. maltophilia infection treatment, but its resistance mechanism in clinical isolates is not fully elucidated. We investigated the antimicrobial susceptibility of 450 S. maltophilia isolated during 2012–2015 from three university hospitals in Beijing, China. These strains exhibited high susceptibility to minocycline (98.44%), sulfamethoxazole/trimethoprim (87.56%), tigecycline (77.78 %), doxycycline (81.33%), levofloxacin (67.56%), and ticarcillin/clavulanate (73.00%). The susceptibility of tigecycline-nonsusceptible strains (TNS) to doxycycline and levofloxacin was much lower than that of tigecycline-susceptible strains (TSS) (25.00% vs. 97.71% for doxycycline, P < 0.001; 17.00% vs. 82.00% for levofloxacin, P < 0.001). We further selected 48 TNS and TSS and compared the detection rate of eight tetracycline-specific genes by PCR and the expression level of six intrinsic multidrug resistance efflux pumps by real-time PCR. Only one tetB and two tetH genes in TNS and three tetH genes in TSS were detected, and the detection rate had no difference. The average expression level of smeD in TNS was higher than that in TSS [20.59 (11.53, 112.54) vs. 2.07 (0.80, 4.96), P < 0.001], while the average expression levels of smeA, smeI, smeO, smeV, and smrA were not significantly different, indicating that smeDEF was the predominant resistance genetic determinant in clinical S. maltophilia. Higher smeD expression was also observed in levofloxacin- and doxycycline-nonsusceptible isolates than in their corresponding susceptible isolates [16.46 (5.83, 102.24) vs. 2.72 (0.80, 6.25) for doxycycline, P < 0.001; 19.69 (8.07, 115.10) vs. 3.01(1.00, 6.03), P < 0.001], indicating that smeDEF was also the resistance genetic determinant to levofloxacin and doxycycline. The consistent resistance profile and common resistance genetic determinant highlight the importance of rational use of tigecycline for preventing the occurrence and spread of multidrug resistance.
Introduction
Stenotrophomonas maltophilia is classified by the World Health Organization as one of the leading multidrug resistant organisms in hospital settings, and an increasing infection rate has been reported in worldwide and nationwide surveillance studies during the past 15 years (Fluit et al., 2001; Brooke, 2012, 2014; Sader et al., 2013; Walkty et al., 2014; Chang et al., 2015). Treatment of S. maltophilia infections is limited due to the extensive resistance displayed to most clinically used antimicrobials, and sulfamethoxazole/trimethoprim (SXT) is the only recommended first-line antimicrobial. However, its use is limited by a high incidence of allergic reactions, intolerance, and increasing resistance mediated by the spread of sul1 and sul2 genes (Toleman et al., 2007; Hu et al., 2011; Chang et al., 2015; Zhao et al., 2016). Tigecycline has been reported to retain good in vitro activity against S. maltophilia in worldwide surveillance and multicenter studies, and Wei et al. (2016) reported that 80.4% of clinical isolates in China, and 72.7% of SXT-resistant isolates were susceptible to tigecycline, making it a promising alternative antimicrobial for infection treatment (Stein and Babinchak, 2013; Rizek et al., 2015; Wei et al., 2015, 2016). Clinical studies revealed the similar effectiveness of tigecycline and SXT in the treatment of nosocomial S. maltophilia infection (Tekce et al., 2012; Wu and Shao, 2014). Therefore, tigecycline could be used for treatment of S. maltophilia infection, but invalid use and overuse of tigecycline might result in emergence and spread of resistance during treatment. At present, the tigecycline resistance mechanism in clinical isolates is unclear, and epidemiological surveys focused on frequency and genetic determinants of tigecycline resistance are needed in clinical isolates of S. maltophilia. These epidemiologic data could help to guide the appropriate use of tigecycline and preventing the occurrence and spread of multidrug resistance.
Resistance to tetracycline in Gram-negative bacteria is usually attributed to two different mechanisms: acquisition of tetracycline-specific resistance genes and increased expression of intrinsic multidrug resistance efflux pumps (Bartha et al., 2011; Nguyen et al., 2014; Chang et al., 2015; Sharma et al., 2016). For tetracycline-specific resistance genes, carriage of the flavin-dependent monooxygenase genes (tetX, tetX1, tetX2) could confer resistance against tigecycline (Bartha et al., 2011). The tetX1 gene has been detected in tigecycline-nonsusceptible Acinetobacter baumannii isolates in China, indicating that the tetX1 gene might play a role in the reduced tigecycline susceptibility in clinical A. baumannii isolates (Deng et al., 2014). The other tetracycline-specific genes, including that encoding for efflux pumps (tetA, tetB, tetH, tet39) and ribosomal protection proteins (tetM), were also commonly detected in Gram-negative bacteria, but their detection rate and potential role in mediating tigecycline resistance in S. maltophilia has not been investigated (Bartha et al., 2011; Nguyen et al., 2014; Chang et al., 2015). A variety of intrinsic efflux pumps were presented in S. maltophilia and the resistance-nodulation-cell division (RND) family were recognized as the most important intrinsic multidrug-resistance efflux pumps (Chang et al., 2015). The K279a sequence carries nine RND-type efflux pump genes (Crossman et al., 2008). Six of these efflux pumps have been characterized, among which SmeDEF, SmeIJK, SmeOP-ToICsm, and SmeVWX can confer resistance to tigecycline or tetracycline (Alonso and Martinez, 2000; Zhang et al., 2001; Cho et al., 2012; Gould et al., 2013; Lin et al., 2014; Chang et al., 2015; Sanchez and Martinez, 2015). Besides these RND efflux pumps, SmrA, a member of the ATP binding cassette family, confers resistance to fluoroquinolones and tetracycline (Al-Hamad et al., 2009). However, most of these studies were performed by genetic introduction, deletion, or mutation in a single reference or clinical strain, and could not be representative of the common clinical scenario. Though all of these intrinsic efflux pumps could mediate tigecycline resistance, which is the predominant genetic determinant in clinical strains is still unclear. Therefore, molecular epidemiological investigations on the tigecycline resistance genetic determinants in clinically isolated S. maltophilia are still needed.
To this end, we investigated the frequency and antimicrobial susceptibility of 450 clinical isolates of S. maltophilia obtained between 2012 and 2015 from three university hospitals in Beijing, China, and further explored the possible genetic determinants of tigecycline resistance. We compared the detection rate of various tetracycline-specific resistance genes by PCR and the expression levels of multidrug-resistance efflux pumps by real-time PCR in tigecycline-nonsusceptible strains (TNS, minimum inhibitory concentration [MIC] for tigecycline > 2 mg/L) vs. tigecycline-susceptible strains (TSS, MIC for tigecycline ≤ 2 mg/L). The epidemiological investigation of the resistance profile and resistance genetic determinants to tigecycline could aid in the rational use of tigecycline and help in the development of targeted prevention measures to contain the occurrence and spread of multidrug resistance.
Materials and Methods
Bacterial Strains and Susceptibility Test
During 2012 to 2015, 450 clinical S. maltophilia isolates were collected from hospitalized patients at Peking Union Medical College Hospital, the Chinese PLA General Hospital, and the Air Force General Hospital in Beijing, China. Tigecycline is classified as a special-use antibacterial drug in China and is approved only for the treatment of complicated skin and soft-tissue infections, complicated intra-abdominal infections, and bacterial pneumonia. These S. maltophilia strains were all isolated from respiratory tract specimens of patients with pulmonary infection. Bacterial species identification was performed using a Vitek II bacterial identification system (bioMérieux, Marcy-l’Étoile, France), and the results were further confirmed by species-specific PCR (Whitby et al., 2000). MIC results for SXT, minocycline, levofloxacin, ticarcillin/clavulanate, ceftazidime, and chloramphenicol were performed by the agar dilution method, and the results were interpreted according to the breakpoints suggested by CLSI (2015). Since there were no specified breakpoints criterion of tigecycline and doxycycline for S. maltophilia, tigecycline susceptibility breakpoint criterion for Enterobacteriaceae and doxycycline susceptibility breakpoint criterion for Acinetobacter spp. were used. Escherichia coli ATCC 25922 and Pseudomonas aeruginosa ATCC 27853 purchased from the American Type Culture Collection (Manassas, VA, United States) were used as quality control strains. SXT, minocycline, levofloxacin, ticarcillin/clavulanate, ceftazidime, and chloramphenicol were purchased from the National Institute for the Control of Pharmaceutical and Biological Products (Beijing, China). Tigecycline and moxifloxacin were obtained from Wyeth Pharmaceutical (Philadelphia, PA, United States) and Bayer Healthcare (Bayer Pharma AG, Wuppertal, Germany), respectively. Mueller-Hinton agar was purchased from Becton Dickinson and Co. (Franklin Lakes, NJ, United States). Antimicrobial solutions were prepared fresh on the day of use as previously described (CLSI, 2015).
Detection of Tetracycline-Specific Genes
Of the 450 isolates detected, 48 TNS and 48 TSS S. maltophilia were selected randomly and screened by PCR reaction for the presence of eight tetracycline-specific resistance genes (tetA, tetB, tetM, tetH, tet39, tetX, tetX1, and tetX2) using specific primers (Table 1) and Taq DNA Polymerase (TaKaRa Bio, Tokyo, Japan). PCR cycling parameters were as follows: initial activation at 95°C for 5 min; 30 amplification cycles at 95°C for 30 s, 55°C for 30 s, and 72°C for 30 s; and a final extension step at 72°C for 7 min. A 50-μL reaction volume was used, and the products were separated by 1% (w/v) agarose gel electrophoresis (Amresco, Solon, OH, United States) followed by ethidium bromide staining. Images were acquired using a Gel DocTM EQ imaging system (Bio-Rad, Hercules, CA, United States).
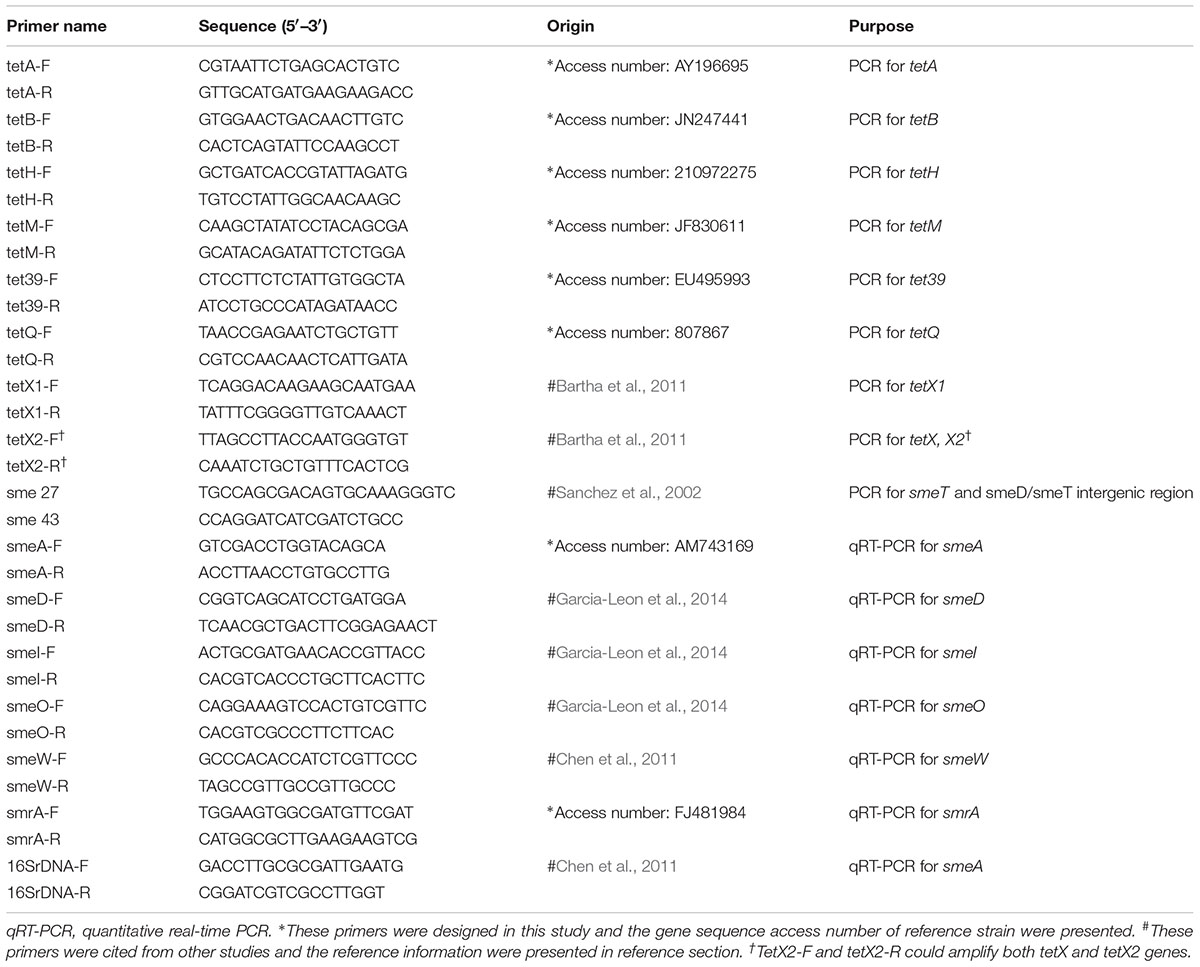
TABLE 1. Primers used for specific amplification of tetracycline-specific resistance genes and intrinsic multidrug-resistance efflux pumps of S. maltophilia.
Detection of Expression of Intrinsic Multidrug Efflux Pumps
The expression levels of six multidrug efflux pump genes (smeA, smeD, smeI, smeO, smeV, and smrA) were assessed by real-time PCR using specific primers (Table 1). S. maltophilia suspensions were prepared and inoculated in Mueller-Hinton broth (Difco, Cockeysville, MD, United States). After overnight culture, total DNase-treated RNA was extracted using the RNeasy kit (Tiangen, Beijing, China). cDNA was synthesized using the primeScript RT-PCR kit (TaKaRa Bio) following the manufacturer’s instructions. Real-time PCR was performed using the DNA Engine Opticon 2 real-time PCR detection system (Bio-Rad) and a SYBR premix EX Taq kit (TaKaRa Bio). Thermocycling conditions were as follows: Taq activation at 95°C for 5 s, followed by 40 cycles at 95°C for 15 s and 58°C for 30 s. Each real-time PCR reaction was conducted in triplicate. Analysis of real-time PCR results was carried out by the 2-ΔΔCT method. The housekeeping gene 16S rDNA was used as an internal control to normalize the expression of target genes and a susceptible S. maltophilia from Air Force General Hospital (K106, MICtigecycline = 0.25 mg/L) was used as an external control to normalize the relative expression levels of target genes from clinical isolates. All real-time PCR experiment amplifying the target gene should also include real-time PCR reaction amplifying 16S rDNA gene for both test strains and external control K106 in the same plate. The Ct values for the 16S rRNA gene in K106 were all within one cycle for each experiment under all conditions tested.
Amplification for smeT and smeT/D Intergenic Region
To identify the sequence variations of the smeT and the smeT/D intergenic region, PCR reactions were performed using primers sme 27 and sme 43 presented by Sanchez et al. (2002; Table 1). The primers were designed for sequence amplification of the smeT and the smeT/D intergenic region in D457R (accession number: AJ316010). The S. maltophilia K279a and ATCC13637 were used as control to ensure the accuracy of the PCR reaction. Sequence of the smeT and the smeT/D intergenic region of D457R were blasted in NCBI database1. All sequence of smeT gene and smeT/D intergenic region presented in NCBI database were downloaded and aligned in Mega 6.
Statistical Analyses
SPSS 22.0 (SPSS Inc., Chicago, IL, United States) was used for statistical analyses. Comparative analyses of antimicrobial susceptibility rate and detection rate of tetracycline-specific resistant genes were performed by the Chi-squared test or Fisher’s exact test, as appropriate. Expression levels of intrinsic multidrug-resistance efflux pumps are presented as median (interquartile range) and compared by Mann-Whitney U test. All comparisons were two-tailed, and P < 0.05 was considered to indicate statistically significant differences.
Results
Antimicrobial Susceptibility Profiles
Tigecycline exhibited good activity (MIC50/MIC90: 1/8 mg/L, susceptibility rate: 77.78%) (Table 2). SXT (87.56%), minocycline (98.44%), and ticarcillin/clavulanate (76.00%) retained high in vitro activity against clinical isolates of S. maltophilia, while ceftazidime (26.44%) and chloramphenicol (12.00%) exhibited poor activity. TSS strains displayed high susceptibility to minocycline (100.00%), SXT (89.71%), doxycycline (97.71%), levofloxacin (82.00%), and ticarcillin/clavulanate (76.86%), while the TNS strains displayed high susceptibility to minocycline (94.00%), SXT (80.00%), and ticarcillin/clavulanate (73.00%), but poor susceptibility to doxycycline (25.00%) and levofloxacin (17.00%). The susceptibility rate of TNS strains to doxycycline and levofloxacin was much lower than that of TSS strains (25.00% vs. 97.71% for doxycycline, P < 0.001; 17.00% vs. 82.00% for levofloxacin, P < 0.001).
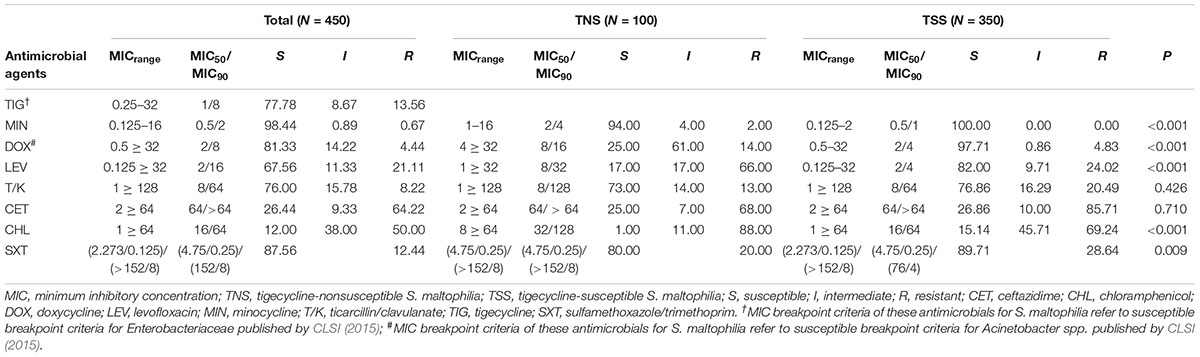
TABLE 2. In vitro susceptibility of 450 clinical isolates of S. maltophilia to eight clinically used antimicrobials.
Detection of Tetracycline-Specific Genes
We screened eight tetracycline-specific resistance genes (tetA, tetB, tetM, tetH, tet39, tetX, tetX1, and tetX2) in 48 selected clinical TNS and 48 TSS clinical S. maltophilia by routine PCR analysis. Only one tetB and two tetH genes in TNS and three tetH genes in TSS were detected, and the detection rate was not different between the two groups.
Expression of Intrinsic Multidrug Efflux Pumps
Real-time PCR showed that the average relative expression levels [median (interquartile range)] of smeA, smeD, smeI, smeO, smeW, and smrA in TNS S. maltophilia were 7.47 (3.64, 16.93), 20.59 (11.53, 112.54), 0.41 (0.20, 2.85), 1.12 (0.30, 1.90), 2.99 (1.44, 6.03), and 1.46 (0.63, 3.57), respectively. The values in TSS S. maltophilia were 8.64 (3.42, 14.68), 2.07 (0.80, 4.96), 0.65 (0.23, 11.61), 1.26 (0.56, 2.10), 4.75 (1.86, 6.61), and 1.74 (0.92, 2.96), respectively. Quantitative analysis (Figure 1) revealed that the average expression level of smeD was higher in the TNS group than in the TSS group [20.59 (11.53, 112.54) vs. 2.07 (0.80, 4.96), P < 0.001]. We further divided the 98 selected S. maltophilia into levofloxacin-susceptible and levofloxacin-nonsusceptible strains (including 47 LSS and 49 LNS), doxycycline-susceptible and doxycycline-nonsusceptible strains (including 50 DSS and 46 DNS). Compared with that in the corresponding LSS and DSS, the expression of smeD was higher in LNS [16.46 (5.83, 102.24) vs. 2.72 (0.80, 6.25), P < 0.001] and DNS [19.69 (8.07, 115.10) vs. 3.01(1.00, 6.03), P < 0.001] (Table 3).
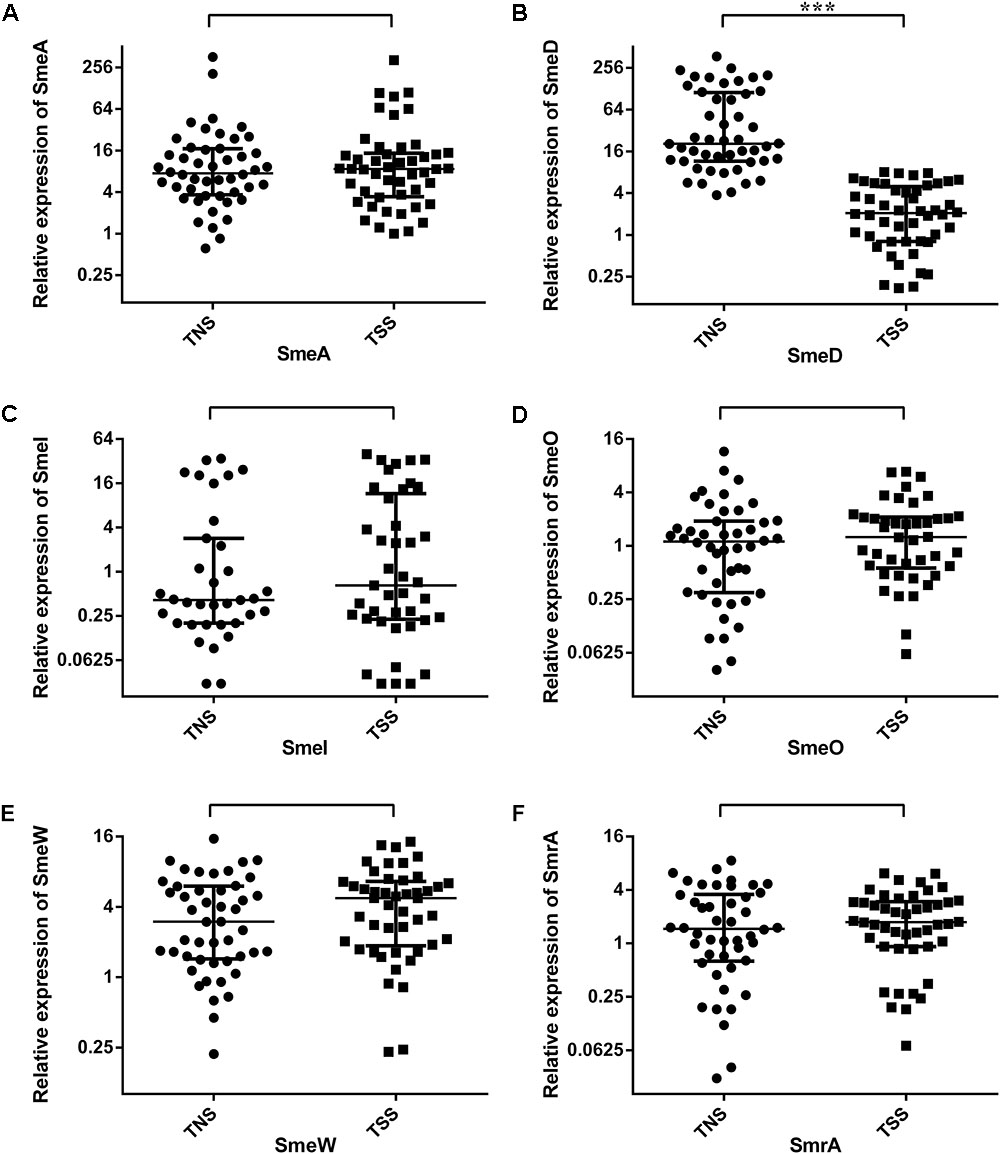
FIGURE 1. Real-time PCR analysis of intrinsic multidrug-resistance efflux pumps gene expression profiles in tigecycline-nonsusceptible and tigecycline-susceptible S. maltophilia. (A–F) Represent the relative expression level of smeA, smeD, smeI, smeO, smeW, and smrA, respectively. TNS, tigecycline-nonsusceptible S. maltophilia; TSS, tigecycline-susceptible S. maltophilia. Each dot corresponds to a clinical isolate. Expression levels of intrinsic multidrug-resistance efflux pumps are presented as median (interquartile range) and compared by non-parametric tests. ∗∗∗P < 0.001.
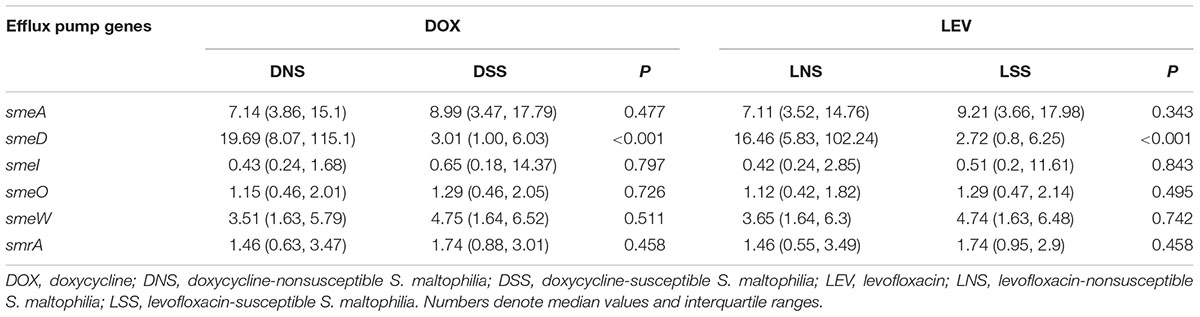
TABLE 3. Expression levels of intrinsic multidrug-resistance efflux pumps genes in S. maltophilia nonsusceptible to doxycycline or levofloxacin and the corresponding susceptible controls.
Amplification for smeT and smeT/D Intergenic Region
Primers sme 27 and sme 43, which designed for amplification of D457R failed to amplify the target gene in most of our clinical strains, and even the K279a and ATCC13637. The sequence of D457R (accession number: AJ316010) had 90–100% identity in smeT gene and 83–100% identity in smeT/D intergenic region with sequences of S. maltophilia strains from NCBI database. There was a variety of sequence variants located on the smeT and smeT/D intergenic region, including original and terminal region of these genes (see Supplementary Material).
Discussion
Stenotrophomonas maltophilia is one of the leading multidrug resistant organisms in hospital settings and can cause severe nosocomial respiratory and bloodstream infections (Looney et al., 2009; Brooke, 2014; Chang et al., 2015). Tigecycline is regarded as a potential alternative antimicrobial for infection treatment (Looney et al., 2009; Chang et al., 2015). Clinical isolates of S. maltophilia in Beijing showed good in vitro susceptibility to tigecycline. The tigecycline susceptibility rate in Beijing was lower than that reported in the Taiwan Surveillance of Antimicrobial Resistance Study from 1998 to 2008 and in the countries in the SENTRY Antimicrobial Surveillance Program from 2009 to 2012, but similar to that reported in SENTRY from 2011 to 2014 (Wu et al., 2012; Sader et al., 2014, 2016). The difference might be partially due to the different geographic origins of the sources and the timing of the studies. More selective pressure caused by the gradual increase in the use of tigecycline in recent years might also contribute to the lower susceptibility rate. The clinical S. maltophilia isolates from Beijing showed high in vitro susceptibility to minocycline, SXT, and ticarcillin/clavulanate, rendering these compounds appropriate antimicrobial treatments for S. maltophilia infection in this area. Ceftazidime and chloramphenicol had poor activity, and they should not be used as empirical treatment. The susceptibility rate against doxycycline and levofloxacin in TNS S. maltophilia was much lower than that in the TSS group. Highly consistent antimicrobial resistance profiles against tigecycline, doxycycline, and levofloxacin indicated that they might share common genetic resistance determinants, and this criterion should be taken into account when deciding on the treatment regimen.
Due to the global spread of carbapenem-resistant A. baumannii, KPC-producing K. pneumoniae, and SXT-resistant S. maltophilia, tigecycline is regarded as the last resort in the control of clinical infections (Tekce et al., 2012; Sun et al., 2013; Deng et al., 2014; Chang et al., 2015; He et al., 2015). Epidemiological investigations have demonstrated that overexpression of the RND efflux pump AdeABC plays the predominant role on the tigecycline resistance in clinically isolated A. baumannii, while the RND efflux pump AdeIJK and flavin-dependent monooxygenase TetX1 are also involved in tigecycline resistance (Deng et al., 2014; Li et al., 2015). In K. pneumonia, the RND efflux pump AcrAB contributes to tigecycline resistance in clinical isolates (Bratu et al., 2009; He et al., 2015). Tigecycline is not effective against Pseudomonas aeruginosa, and the reduced susceptibility is attributed to RND efflux pump MexXY (Dean et al., 2003; Sun et al., 2013). However, the tigecycline resistance mechanism in clinical isolates has not been fully elucidated. To identify the predominant genetic determinants of tigecycline resistance in clinical isolates of S. maltophilia, we compared the detection rate of eight tetracycline-specific resistance genes and the expression levels of six multidrug-resistance efflux pump genes. Among the eight tetracycline-specific resistance genes, only a few tetB or tetH genes were detected in clinical S. maltophilia isolates, and the detection rate was not different between the TNS and TSS groups, indicating that the tetracycline-specific resistance genes were not the predominant tigecycline resistance mechanism.
Regarding the intrinsic efflux pump genes, a variety of efflux pumps have been shown to be associated with resistance to tigecycline or tetracycline by the genetic introduction, deletion, or mutation in a single reference or clinical strain, including SmeDEF, SmeIJK, SmeOP-TolCsm, SmeVW, and SmrA (Alonso and Martinez, 2000; Zhang et al., 2001; Al-Hamad et al., 2009; Cho et al., 2012; Gould et al., 2013; Lin et al., 2014; Sanchez and Martinez, 2015). Considering the common resistance profile between tigecycline and levofloxacin, we also detected the expression level of smeABC, which could mediate the extrusion of quinolones (Li et al., 2002). Quantitative analysis revealed that the average expression level of smeD was higher in TNS S. maltophilia than in TSS strains, while the remaining five intrinsic multidrug efflux genes did not show significant differences. The results indicate that overexpression of smeDEF is the predominant tigecycline resistance mechanism of clinical S. maltophilia isolates in Beijing. SmeDEF was identified as a novel multidrug efflux pump from S. maltophilia in 2000. The pump is formed by an inner membrane protein (SmeE), an outer membrane protein (SmeF), and a membrane fusion protein (SmeD) (Alonso and Martinez, 2000). Zhang et al. (2001) have identified tigecycline as a substrate of SmeDEF in both intrinsic and acquired resistance by genetic deletion and mutation of ULA-511 strain. We confirmed that overexpression of smeDEF is the common genetic determinants of resistance to tigecycline in clinical isolates of S. maltophilia in Beijing. Subsequent studies after Zhang et al. (2001) demonstrated that this pump mediated not only multiple resistance to a variety of structurally unrelated antimicrobial agents, but also a number of disinfectants and heavy-metal ions (Sanchez et al., 2002; Gould and Avison, 2006; Chang et al., 2015). A battery of epidemiological investigations have indicated that quinolone resistance in clinical isolates of S. maltophilia is associated with overexpression of smeDEF, and the extrusion activity of SmeDEF is not abolished by the efflux pump inhibitor Phe-Arg-β-naphthylamide (Sanchez et al., 2002; Jia et al., 2015; Chong et al., 2017). We further compared the expression levels of intrinsic efflux pumps between levofloxacin-nonsensitive and levofloxacin-sensitive, and doxycycline-nonsensitive and doxycycline-sensitive groups. The results revealed a higher expression of smeD in levofloxacin- and doxycycline-nonsensitive isolates than in their corresponding sensitive counterparts, which demonstrated that overexpression of SmeDEF is also the common genetic determinants of multiple resistance to doxycycline, and levofloxacin.
Our results highlight the importance of the rational use of antibiotics to prevent the emergence and spread of multidrug resistance. Due to a variety of intrinsic and acquired antimicrobial resistance mechanisms, therapeutic options for S. maltophilia treatment are limited, especially for patients who cannot tolerate SXT. Tigecycline, doxycycline, and levofloxacin have become the few available potential alternative antimicrobial agents when SXT is unsuitable for the treatment of S. maltophilia owing to contraindications or resistance. However, inappropriate use and overuse of tigecycline might result in the occurrence and spread of tigecycline, and also the emergence of resistance to levofloxacin and doxycycline. A serendipitous mutation in the regulatory smeT gene, such as A/T mutation at position 498, could lead to overexpression of smeDEF and multiple resistances to tigecycline, doxycycline, and levofloxacin (Sanchez et al., 2002). If clinical strains with this mutation were selected and enriched by pressure of prolonged exposure to subinhibitory concentrations, the selection and enrichment would result in emergence of multidrug resistance and the failure of clinical treatment. Using primers sme 27 and sme 43 presented by Sanchez et al. (2002), we tried to identify other sequence variations located on the smeT and the smeT/D intergenic region in clinical S. maltophilia, but the primers designed for amplification of D457R failed to amplify most of our clinical strains, and even the K279a and ATCC13637. We further downloaded all of the sequence of smeT and the smeT/D intergenic region in NCBI database and performed sequence alignment. The result of alignment revealed a variety of sequence variants located on the smeT and smeT/D intergenic region, including original and terminal region of these genes, therefore it was difficult to design general primers that could amplify the complete sequence of smeT in clinical S. maltophilia from different areas and times. Further researches are still needed to identify the possible sequence variations of smeT gene leading to the overexpression of smeDEF in clinical scenario.
Conclusion
Clinical isolates of S. maltophilia in Beijing showed good in vitro susceptibility to tigecycline. S. maltophilia had highly consistent antimicrobial resistance profiles against tigecycline, doxycycline, and levofloxacin, and the smeDEF efflux pumps gene was the common genetic determinant of resistance to these three drugs. The consistent resistance profile and common resistance genetic determinant highlight the importance of rational use of tigecycline for preventing the occurrence and spread of multidrug resistance.
Author Contributions
YNL and BZ conceived and designed the experiments. JZ and YXL executed the experiments. JZ, YL, and DW performed and wrote the manuscript. RW and WN helped to edit the manuscript.
Funding
This work was supported by the Special Grant for the Prevention and Control of Infectious Diseases of China (Nos. 2013ZX10004 805-003, 2013ZX10004 217-002, and 2009ZX10004-204), the major project of the Twelfth Five Year Research Foundation of Military Medical Sciences and Technology (Nos. BWS13J027, 13BJYZ31, and CWS15B049).
Conflict of Interest Statement
The authors declare that the research was conducted in the absence of any commercial or financial relationships that could be construed as a potential conflict of interest.
Acknowledgments
We are grateful to Peking Union Medical College Hospital, Chinese PLA General Hospital, and Air Force General Hospital for kindly providing S. maltophilia strains and helpful information.
Supplementary Material
The Supplementary Material for this article can be found online at: https://www.frontiersin.org/articles/10.3389/fmicb.2018.00549/full#supplementary-material
Footnote
References
Al-Hamad, A., Upton, M., and Burnie, J. (2009). Molecular cloning and characterization of SmrA, a novel ABC multidrug efflux pump from Stenotrophomonas maltophilia. J. Antimicrob. Chemother. 64, 731–734. doi: 10.1093/jac/dkp271
Alonso, A., and Martinez, J. L. (2000). Cloning and characterization of SmeDEF, a novel multidrug efflux pump from Stenotrophomonas maltophilia. Antimicrob. Agents Chemother. 44, 3079–3086. doi: 10.1128/AAC.44.11.3079-3086.2000
Bartha, N. A., Soki, J., Urban, E., and Nagy, E. (2011). Investigation of the prevalence of tetQ, tetX and tetX1 genes in Bacteroides strains with elevated tigecycline minimum inhibitory concentrations. Int. J. Antimicrob. Agents 38, 522–525. doi: 10.1016/j.ijantimicag.2011.07.010
Bratu, S., Landman, D., George, A., Salvani, J., and Quale, J. (2009). Correlation of the expression of acrB and the regulatory genes marA, soxS and ramA with antimicrobial resistance in clinical isolates of Klebsiella pneumoniae endemic to New York City. J. Antimicrob. Chemother. 64, 278–283. doi: 10.1093/jac/dkp186
Brooke, J. S. (2012). Stenotrophomonas maltophilia: an emerging global opportunistic pathogen. Clin. Microbiol. Rev. 25, 2–41. doi: 10.1128/CMR.00019-11
Brooke, J. S. (2014). New strategies against Stenotrophomonas maltophilia: a serious worldwide intrinsically drug-resistant opportunistic pathogen. Expert Rev. Anti Infect. Ther. 12, 1–4. doi: 10.1586/14787210.2014.864553
Chang, Y. T., Lin, C. Y., Chen, Y. H., and Hsueh, P. R. (2015). Update on infections caused by Stenotrophomonas maltophilia with particular attention to resistance mechanisms and therapeutic options. Front. Microbiol. 6:893. doi: 10.3389/fmicb.2015.00893
Chen, C. H., Huang, C. C., Chung, T. C., Hu, R. M., Huang, Y. W., and Yang, T. C. (2011). Contribution of resistance-nodulation-division efflux pump operon smeU1-V-W-U2-X to multidrug resistance of Stenotrophomonas maltophilia. Antimicrob. Agents Chemother. 55, 5826–5833. doi: 10.1128/aac.00317-11
Cho, H. H., Sung, J. Y., Kwon, K. C., and Koo, S. H. (2012). Expression of Sme efflux pumps and multilocus sequence typing in clinical isolates of Stenotrophomonas maltophilia. Ann. Lab. Med. 32, 38–43. doi: 10.3343/alm.2012.32.1.38
Chong, S. Y., Lee, K., Chung, H. S., Hong, S. G., Suh, Y., and Chong, Y. (2017). Levofloxacin efflux and smeD in clinical isolates of Stenotrophomonas maltophilia. Microb. Drug Resist. 23, 163–168. doi: 10.1089/mdr.2015.0228
CLSI (2015). Performance Standards for Antimicrobial Susceptibility Testing; Twenty-Fifth Informational Supplement. Wayne, PA: Clinical and Laboratory Standards Institute.
Crossman, L. C., Gould, V. C., Dow, J. M., Vernikos, G. S., Okazaki, A., et al. (2008). The complete genome, comparative and functional analysis of Stenotrophomonas maltophilia reveals an organism heavily shielded by drug resistance determinants. Genome Biol. 9:R74. doi: 10.1186/gb-2008-9-4-r74
Dean, C. R., Visalli, M. A., Projan, S. J., Sum, P. E., and Bradford, P. A. (2003). Efflux-mediated resistance to tigecycline (GAR-936) in Pseudomonas aeruginosa PAO1. Antimicrob. Agents Chemother. 47, 972–978. doi: 10.1128/aac.47.3.972-978.2003
Deng, M., Zhu, M. H., Li, J. J., Bi, S., Sheng, Z. K., Hu, F. S., et al. (2014). Molecular epidemiology and mechanisms of tigecycline resistance in clinical isolates of Acinetobacter baumannii from a Chinese university hospital. Antimicrob. Agents Chemother. 58, 297–303. doi: 10.1128/AAC.01727-13
Fluit, A. C., Schmitz, F. J., Verhoef, J., and European SENTRY Participant Group (2001). Frequency of isolation of pathogens from bloodstream, nosocomial pneumonia, skin and soft tissue, and urinary tract infections occurring in European patients. Eur. J. Clin. Microbiol. Infect. Dis. 20, 188–191. doi: 10.1007/s100960100455
Garcia-Leon, G., Hernandez, A., Hernando-Amado, S., Alavi, P., Berg, G., and Martinez, J. L. (2014). A function of SmeDEF, the major quinolone resistance determinant of Stenotrophomonas maltophilia, is the colonization of plant roots. Appl. Environ. Microbiol. 80, 4559–4565. doi: 10.1128/aem.01058-14
Gould, V. C., and Avison, M. B. (2006). SmeDEF-mediated antimicrobial drug resistance in Stenotrophomonas maltophilia clinical isolates having defined phylogenetic relationships. J. Antimicrob. Chemother. 57, 1070–1076. doi: 10.1093/jac/dkl106
Gould, V. C., Okazaki, A., and Avison, M. B. (2013). Coordinate hyperproduction of SmeZ and SmeJK efflux pumps extends drug resistance in Stenotrophomonas maltophilia. Antimicrob. Agents Chemother. 57, 655–657. doi: 10.1128/AAC.01020-12
He, F., Fu, Y., Chen, Q., Ruan, Z., Hua, X., Zhou, H., et al. (2015). Tigecycline susceptibility and the role of efflux pumps in tigecycline resistance in KPC-producing Klebsiella pneumoniae. PLoS One 10:e0119064. doi: 10.1371/journal.pone.0119064
Hu, L. F., Chang, X., Ye, Y., Wang, Z. X., Shao, Y. B., Shi, W., et al. (2011). Stenotrophomonas maltophilia resistance to trimethoprim/sulfamethoxazole mediated by acquisition of sul and dfrA genes in a plasmid-mediated class 1 integron. Int. J. Antimicrob. Agents 37, 230–234. doi: 10.1016/j.ijantimicag.2010.10.025
Jia, W., Wang, J., Xu, H., and Li, G. (2015). Resistance of Stenotrophomonas maltophilia to fluoroquinolones: prevalence in a university hospital and possible mechanisms. Int. J. Environ. Res. Public Health 12, 5177–5195. doi: 10.1016/j.resmic.2015.04.002
Li, H., Wang, X., Zhang, Y., Zhao, C., Chen, H., Jiang, S., et al. (2015). The role of RND efflux pump and global regulators in tigecycline resistance in clinical Acinetobacter baumannii isolates. Future Microbiol. 10, 337–346. doi: 10.2217/fmb.15.7
Li, X. Z., Zhang, L., and Poole, K. (2002). SmeC, an outer membrane multidrug efflux protein of Stenotrophomonas maltophilia. Antimicrob. Agents Chemother. 46, 333–343. doi: 10.1128/AAC.46.2.333-343.2002
Lin, C. W., Huang, Y. W., Hu, R. M., and Yang, T. C. (2014). SmeOP-TolCSm efflux pump contributes to the multidrug resistance of Stenotrophomonas maltophilia. Antimicrob. Agents Chemother. 58, 2405–2408. doi: 10.1128/AAC.01974-13
Looney, W. J., Narita, M., and Muhlemann, K. (2009). Stenotrophomonas maltophilia: an emerging opportunist human pathogen. Lancet Infect. Dis. 9, 312–323. doi: 10.1016/S1473-3099(09)70083-0
Nguyen, F., Starosta, A. L., Arenz, S., Sohmen, D., Donhofer, A., and Wilson, D. N. (2014). Tetracycline antibiotics and resistance mechanisms. Biol. Chem. 395, 559–575. doi: 10.1515/hsz-2013-0292
Rizek, C., Ferraz, J. R., van der Heijden, I. M., Giudice, M., Mostachio, A. K., Paez, J., et al. (2015). In vitro activity of potential old and new drugs against multidrug-resistant gram-negatives. J. Infect. Chemother. 21, 114–117. doi: 10.1016/j.jiac.2014.10.009
Sader, H. S., Castanheira, M., Farrell, D. J., Flamm, R. K., Mendes, R. E., and Jones, R. N. (2016). Tigecycline antimicrobial activity tested against clinical bacteria from Latin American medical centres: results from SENTRY Antimicrobial Surveillance Program (2011-2014). Int. J. Antimicrob. Agents 48, 144–150. doi: 10.1016/j.ijantimicag.2016.04.021
Sader, H. S., Farrell, D. J., Flamm, R. K., and Jones, R. N. (2014). Antimicrobial susceptibility of Gram-negative organisms isolated from patients hospitalised with pneumonia in US and European hospitals: results from the SENTRY Antimicrobial Surveillance Program, 2009-2012. Int. J. Antimicrob. Agents 43, 328–334. doi: 10.1016/j.ijantimicag.2014.01.007
Sader, H. S., Flamm, R. K., and Jones, R. N. (2013). Tigecycline activity tested against antimicrobial resistant surveillance subsets of clinical bacteria collected worldwide (2011). Diagn. Microbiol. Infect. Dis. 76, 217–221. doi: 10.1016/j.diagmicrobio.2013.02.009
Sanchez, M. B., and Martinez, J. L. (2015). The efflux pump SmeDEF contributes to trimethoprim-sulfamethoxazole resistance in Stenotrophomonas maltophilia. Antimicrob. Agents Chemother. 59, 4347–4348. doi: 10.1128/AAC.00714-15
Sanchez, P., Alonso, A., and Martinez, J. L. (2002). Cloning and characterization of SmeT, a repressor of the Stenotrophomonas maltophilia multidrug efflux pump SmeDEF. Antimicrob. Agents Chemother. 46, 3386–3393. doi: 10.1128/AAC.46.11.3386-3393.2002
Sharma, V. K., Johnson, N., Cizmas, L., McDonald, T. J., and Kim, H. (2016). A review of the influence of treatment strategies on antibiotic resistant bacteria and antibiotic resistance genes. Chemosphere 150, 702–714. doi: 10.1016/j.chemosphere.2015.12.084
Stein, G. E., and Babinchak, T. (2013). Tigecycline: an update. Diagn. Microbiol. Infect. Dis. 75, 331–336. doi: 10.1016/j.diagmicrobio.2012.12.004
Sun, Y., Cai, Y., Liu, X., Bai, N., Liang, B., and Wang, R. (2013). The emergence of clinical resistance to tigecycline. Int. J. Antimicrob. Agents 41, 110–116. doi: 10.1016/j.ijantimicag.2012.09.005
Tekce, Y. T., Erbay, A., Cabadak, H., and Sen, S. (2012). Tigecycline as a therapeutic option in Stenotrophomonas maltophilia infections. J. Chemother. 24, 150–154. doi: 10.1179/1120009X12Z.00000000022
Toleman, M. A., Bennett, P. M., Bennett, D. M., Jones, R. N., and Walsh, T. R. (2007). Global emergence of trimethoprim/sulfamethoxazole resistance in Stenotrophomonas maltophilia mediated by acquisition of sul genes. Emerg. Infect. Dis. 13, 559–565. doi: 10.3201/eid1304.061378
Walkty, A., Adam, H., Baxter, M., Denisuik, A., Lagace-Wiens, P., Karlowsky, J. A., et al. (2014). In vitro activity of plazomicin against 5,015 gram-negative and gram-positive clinical isolates obtained from patients in Canadian hospitals as part of the CANWARD study, 2011-2012. Antimicrob. Agents Chemother. 58, 2554–2563. doi: 10.1128/AAC.02744-13
Wei, C., Ni, W., Cai, X., and Cui, J. (2015). A Monte Carlo pharmacokinetic/pharmacodynamic simulation to evaluate the efficacy of minocycline, tigecycline, moxifloxacin, and levofloxacin in the treatment of hospital-acquired pneumonia caused by Stenotrophomonas maltophilia. Infect. Dis. (Lond.) 47, 846–851. doi: 10.3109/23744235.2015.1064542
Wei, C., Ni, W., Cai, X., Zhao, J., and Cui, J. (2016). Evaluation of trimethoprim/sulfamethoxazole (SXT), minocycline, tigecycline, moxifloxacin, and ceftazidime alone and in combinations for SXT-susceptible and SXT-resistant Stenotrophomonas maltophilia by in vitro time-kill experiments. PLoS One 11:e0152132. doi: 10.1371/journal.pone.0152132
Whitby, P. W., Carter, K. B., Burns, J. L., Royall, J. A., LiPuma, J. J., and Stull, T. L. (2000). Identification and detection of Stenotrophomonas maltophilia by rRNA-directed PCR. J. Clin. Microbiol. 38, 4305–4309.
Wu, H., Wang, J. T., Shiau, Y. R., Wang, H. Y., Lauderdale, T. L., and Chang, S. C. (2012). A multicenter surveillance of antimicrobial resistance on Stenotrophomonas maltophilia in Taiwan. J. Microbiol. Immunol. Infect. 45, 120–126. doi: 10.1016/j.jmii.2011.09.028
Wu, Y., and Shao, Z. (2014). High-dosage tigecycline for Stenotrophomonas maltophilia bacteremia. Chin. Med. J. 127:3199.
Zhang, L., Li, X. Z., and Poole, K. (2001). SmeDEF multidrug efflux pump contributes to intrinsic multidrug resistance in Stenotrophomonas maltophilia. Antimicrob. Agents Chemother. 45, 3497–3503. doi: 10.1128/aac.45.12.3497-3503.2001
Keywords: Stenotrophomonas maltophilia, tigecycline, resistance, susceptibility, efflux pump, smeDEF
Citation: Zhao J, Liu Y, Liu Y, Wang D, Ni W, Wang R, Liu Y and Zhang B (2018) Frequency and Genetic Determinants of Tigecycline Resistance in Clinically Isolated Stenotrophomonas maltophilia in Beijing, China. Front. Microbiol. 9:549. doi: 10.3389/fmicb.2018.00549
Received: 03 December 2017; Accepted: 12 March 2018;
Published: 26 March 2018.
Edited by:
Xian-Zhi Li, Health Canada, CanadaReviewed by:
Favre-Bonté Sabine, Claude Bernard University Lyon 1, FranceYuji Morita, Aichi Gakuin University, Japan
Copyright © 2018 Zhao, Liu, Liu, Wang, Ni, Wang, Liu and Zhang. This is an open-access article distributed under the terms of the Creative Commons Attribution License (CC BY). The use, distribution or reproduction in other forums is permitted, provided the original author(s) and the copyright owner are credited and that the original publication in this journal is cited, in accordance with accepted academic practice. No use, distribution or reproduction is permitted which does not comply with these terms.
*Correspondence: Bo Zhang, emhhbmdib2FpcmZvcmNlQDE2My5jb20= Youning Liu, bGl1eW91bmluZ3BsYTMwMUAxNjMuY29t
†These authors have contributed equally to this work.