- 1College of Military Medicine, Academy of Military Medical Sciences, Beijing, China
- 2Institute of Disease Control and Prevention, People’s Liberation Army, Beijing, China
- 3Shanghai Municipal Centre for Disease Control and Prevention, Shanghai, China
In this study, we discovered a novel mobilized colistin resistance (mcr-1) gene variant, named mcr-1.9, which was identified in a colistin-resistant enterotoxigenic Escherichia coli (ETEC) strain from a clinical diarrhea case. The mcr-1.9 gene differs from mcr-1 at position 1036 due to a single nucleotide polymorphism (G→A), which results in an aspartic acid residue being replaced by an asparagine residue (Asp346→Asn) in the MCR-1 protein sequence. Antimicrobial susceptibility testing showed that the mcr-1.9-harboring ETEC strain is resistant to colistin at a minimum inhibitory concentration of 4 μg/ml. Plasmid profiling and conjugation experiments also suggest that the mcr-1.9 variant can be successfully transferred into the E. coli strain J53, indicating that the gene is located on a transferable plasmid. Bioinformatics analysis of data obtained from genome sequencing indicates that the mcr-1.9 gene is located on a 64,005 bp plasmid which has been named pEC26. This plasmid was found to have high similarity to the mcr-1-bearing IncI2-type plasmids pWF-5-19C (99% identity and 99% coverage) and pmcr1-IncI2 (99% identity and 98% coverage). The mcr-1.9-harboring ETEC also shows multidrug resistance to nine classes of antibiotics, and contains several virulence and antimicrobial-resistance genes suggested by the genome sequence analysis. Our report is the first to identify a new mcr-1 variant in an ETEC isolated from a human fecal sample, raising concerns about the existence of more such variants in human intestinal flora. Therefore, we believe that an undertaking to identify new mcr-1 variants in the bacterial communities of human intestines is of utmost importance, and that measures need to be taken to control the spread of mcr-1 and its variants in human intestinal microflora.
Introduction
Diarrheal diseases are the second leading cause of death in children under 5 years of age (Qadri et al., 2005). Each year, diarrhea kills ∼525,000 children worldwide (World Health Organization [WHO], 2017). Enterotoxigenic Escherichia coli (ETEC) is an extremely important causative agent of diarrhea in the developing world, due to the prevalence of unhygienic conditions that include the unavailability of clean water and poor sanitation. It is the most frequently encountered bacterial agent causing diarrhea in both children and adults, as well as the most common cause of traveler’s diarrhea in these areas (von Sonnenburg et al., 2000; Bourgeois et al., 2016). ETECs are defined by their ability to produce one or both of two types of enterotoxins [heat-stable (ST) and heat-labile (LT) (So et al., 1978; Rao, 2008)], besides which, they may also produce several colonization factors (CFs) (So et al., 1978) and carry chromosomal determinants associated with ETEC virulence (Del Canto et al., 2011).
Antimicrobial resistance (AMR) has now been recognized as a significant global threat to human and animal health (World Health Organization [WHO], 2018). Due to the widespread and indiscriminate use of antibiotics in countries where diarrhea is endemic, the rate of multidrug resistance (MDR, resistance to three or more classes of antimicrobials) in Enterobacteriaceae such as ETEC, is increasing alarmingly (Lynch et al., 2013). So far, due to this increase in MDR, the usage of successively stronger antimicrobial agents belonging to the tetracycline, sulfonamide, macrolide, and fluoroquinolone families to treat ETEC infections has increased (Diemert, 2006; Rodas et al., 2011). Fluoroquinolones are one of the most recently developed, powerful, and broad-spectrum antibiotics used for treating a wide variety of bacterial infections (Oethinger et al., 1996). Unfortunately, since their introduction in the late 1980s, bacterial resistance to fluoroquinolones has increased markedly (Aguiar et al., 1992). Colistin, which is a polymyxin antibiotic, is currently used as a last-resort antibiotic for treating infections caused by Gram-negative bacteria exhibiting MDR. However, a study conducted by Liu et al. (2015) on E. coli strains from China, has described a new plasmid-mediated colistin-resistance mechanism conferred by the phosphoethanolamine transferase-encoding gene mcr-1 (Liu et al., 2015). Since then, numerous studies on mcr-1 have been undertaken worldwide to investigate the presence of this gene in E. coli strains isolated from various animals, environments, food samples, and even human subjects (Doumith et al., 2016; Khalifa et al., 2016; Perrin-Guyomard et al., 2016; Suzuki et al., 2016; Zhao et al., 2017a).
Since the discovery of mcr-1, several variants of the gene, such as mcr-1.2, mcr-2, mcr-1.3, mcr-1.4, mcr-1.5, mcr-1.6, mcr-1.7, and mcr-1.8 have been discovered in Italy, Belgium, China, and Brunei (Di Pilato et al., 2016; Xavier et al., 2016; Lu et al., 2017; Tijet et al., 2017; Yang et al., 2017; Zhao et al., 2017b). The variants, mcr-2, mcr-1.3, mcr-1.4, mcr-1.5, mcr-1.7, and mcr-1.8 are known to have been identified from E. coli strains isolated from animal, sewage, or human urinary tract samples (the source for mcr-1.8 has not been indicated) (Table 1). In this study, we report a new variant of the mcr-1 gene, which we term mcr-1.9, identified from an E. coli strain (an ETEC) isolated from a diarrheal fecal sample. Apart from analyzing the molecular and AMR characteristics of this ETEC strain, we have also identified the plasmid type in which mcr-1.9 is located, and compared it with other similar mcr-1-harboring plasmids described elsewhere. We believe that this is the first report that identifies a new mcr-1 variant from an ETEC strain isolated from a human fecal sample.
Materials and Methods
Bacterial Isolation, Serotyping, and mcr-1 Gene Screening
A fecal sample was obtained from a 15-month-old male child diagnosed with watery diarrhea and fever, cured with ceftriaxone in 2014 at the Shanghai Children’s Hospital, China. The fecal sample was streaked onto MacConkey agar and incubated overnight at 37°C. Colonies having a pink coloration and a round, neat appearance were selected as suspected E. coli colonies. These were again streaked directly onto MacConkey agar, and incubated overnight at 37°C, following which, the colonies so obtained were further sub-cultured on Luria–Bertani (LB) agar plates at 37°C. Genomic DNA was prepared using the boiling method from the isolated bacteria, and a Diarrhoeagenic E. coli (DEC) detection PCR kit (SSI Diagnostica, Denmark) was used to identify the type of E. coli strain obtained from the fecal sample. The PCR reactions were performed in a 20-μl volume containing 10 μl of PCR ReadyMix, 6 μl of Primer mix, and 4 μl of DNA template. The cycling conditions were 95°C for 2 min, followed by 35 cycles of denaturation at 94°C for 50 s, annealing at 62°C for 40 s, extension at 72°C for 50 s, and a final extension at 72°C for 3 min in an Applied Biosystems PCR cycler. The PCR products are then loaded into 2% agarose gel and electrophoresis. The type of DEC was then identified by the comparison on the fragment size of PCR products and marker in the kit. We screened for the presence of the mcr-1 gene in this isolate (termed SH66) without prior knowledge of its colistin-resistance. The screening was carried out with PCR and Sanger sequencing using previously described primers (Liu et al., 2016).
Informed and written consents were obtained from the patient’s parents for sample collection and usage, as well as for the publication of obtained results in this study. All experiments were approved and authorized by the Ethics Committees of the Institute of Disease Prevention and Control, People’s Liberation Army, China.
Antimicrobial Susceptibility Testing
Minimum inhibitory concentrations (MICs) of colistin for all isolates obtained from the fecal sample were determined using the 96-well plate dilution method, with a maximum colistin concentration of 32 μg/mL, and a minimum concentration of 0.5 μg/mL; the results were interpreted according to the conventions set by the European Committee on Antimicrobial Susceptibility Testing (EUCAST)1. For the mcr-1-positive isolates, we also tested for antimicrobial susceptibility to other antimicrobials via the broth microdilution method using a 96-well microtiter plate (Sensititre, Trek Diagnostic Systems, Thermo Fisher Scientific Inc., West Sussex, United Kingdom). MICs were determined according to the clinical and laboratory standards institute (CLSI, 2014) recommendations (Bennett et al., 2014). The E. coli strain ATCC 25922 was used for quality control.
Plasmid Profiling and Southern Blotting
The DNA of ETEC strain for plasmid profiling was obtained and embedded into agarose according to the pulsed-field gel electrophoresis (PFGE) plug preparation method (Ribot et al., 2006). Then the plug was cut into slices and treated with S1 endonuclease, following which, the DNA fragments were subjected to PFGE according to the PulseNet standard protocols (Ribot et al., 2006). Following this, the membrane transfer, molecular hybridization, and probe detection steps were performed according to a previously reported method (Zou et al., 2015). The mcr-1 probe sequences used in the southern blotting analysis were identical to the mcr-1 primer sequences mentioned in Section “Bacterial Isolation, Serotyping, and mcr-1 Gene Screening.”
Conjugation Experiments
Conjugation experiments to test the transferability of the mcr-1-positive plasmid were carried out using the E. coli strain J53 (LacZ-, AzrR, RifR) as a recipient, and the mcr-1-positive E. coli strain (identified in the fecal sample and named SH66) as a donor. Overnight cultures of donor and recipient bacterial strains (3 mL of each) were mixed together, harvested, and resuspended in 80 μl of LB with a donor:recipient ratio of 1:3. The resultant mixture was spotted into 5 mL of liquid LB broth and incubated 37°C for 12 to 18 h for mating to occur. Following this, a small amount of the mating mixture was spotted on to a Muller-Hinton agar plate (BD Biosciences, San Jose, CA, United States) containing 100 mg/L sodium azide and 2 mg/L polymyxin B; this was used as a selective medium to identify E. coli J53 transconjugants. The identities of putative transconjugants were confirmed using antimicrobial susceptibility testing and PCR detection of the mcr-1.9 gene as described before. The conjugation transfer rate was calculated through the formula “R = number of transconjugants/number of recipients.”
Whole Genome Sequencing and Bioinformatic Analysis
The genomic DNA of the E. coli isolate SH66 was extracted using the QIAamp DNA Mini Kit (Qiagen, Germany), and sequenced using the Illumina MiSeq platform (Illumina Inc., San Diego, CA, United States) according to the manufacturer’s instructions. A DNA library was constructed with inserts of length 600 bp using the MiSeq Reagent Kit v2. The raw sequence data were assembled de novo via the CLC Genomics Workbench 9.0.1 (CLC Bio, Qiagen Bioinformatics, Germany). The mcr-1 plasmid sequence was assembled through the above software and the gaps were filled through combinatorial PCR and Sanger sequencing. The point mutation in mcr-1.9 was verified by the PCR amplification and Sanger sequencing with the primers MCR1-3-F (5′-GGCGTATTCTGTGCCGTGTA-3′) and MCR1-3-R (5′-CGTGATCGCGTCATGGGT-3′) (Supplementary Data Sheet S2). Resistance genes, virulence genes, and other genetic structures were identified through the center for genomic epidemiology (CGE) website2. Sequence alignments were performed using the software Mauve (Darling et al., 2004), and further rounds of PCR amplification and Sanger sequencing were used to fill in the gaps. Complete plasmid sequences were annotated using the online annotation server RAST (Aziz et al., 2008). The plasmid replicons, IS sequences, and AMR genes were identified using the online software PlasmidFinder3, ISfinder4, and ResFinder5, respectively. Sequence comparisons and map generation were performed using BLAST6 and Easyfig (Sullivan et al., 2011), respectively.
Results
mcr-1 Gene Screening and Antimicrobial Susceptibility Testing
One E. coli strain (termed SH66) was isolated from the fecal sample used in this study, and identified as an ETEC using the DEC PCR detection kit. This strain was subsequently transferred to and preserved in our laboratory, where it was recently screened for the presence of the mcr-1 gene with no prior knowledge of its colistin-resistance characteristic. The screening indicated that this strain harbored an mcr-1 gene. According to medical records, the patient who harbored this strain was not treated with polymyxin antibiotics.
The results of the antimicrobial susceptibility testing indicate that the ETEC strain identified in this study is resistant to colistin (MIC, 4 μg/ml), ampicillin (>32 μg/ml), chloramphenicol (>32 μg/ml), trimethoprim/sulfamethoxazole (>4 μg/ml), tetracycline (>32 μg/ml), ciprofloxacin (>4 μg/ml), sulfonazo isoxazole (>256 μg/ml), nalidixic acid (>32 μg/ml), streptomycin (>64 μg/ml), and azithromycin (>16 μg/ml), though it was found to be susceptible to amoxicillin/clavulanic acid (4 μg/ml), ceftriaxone (≤0.25 μg/ml), ceftiofur (0.25 μg/ml), cefoxitin (8 μg/ml), and gentamicin (1 μg/ml) (Table 2). According to the classification standards of the WHO (Collignon et al., 2009), SH66 is an MDR strain showing resistance to nine classes of antimicrobials, including aminoglycosides, amphenicols, folate pathway inhibitors, macrolides, penicillins, polymyxins, quinolones, sulfonamides, and tetracyclines.
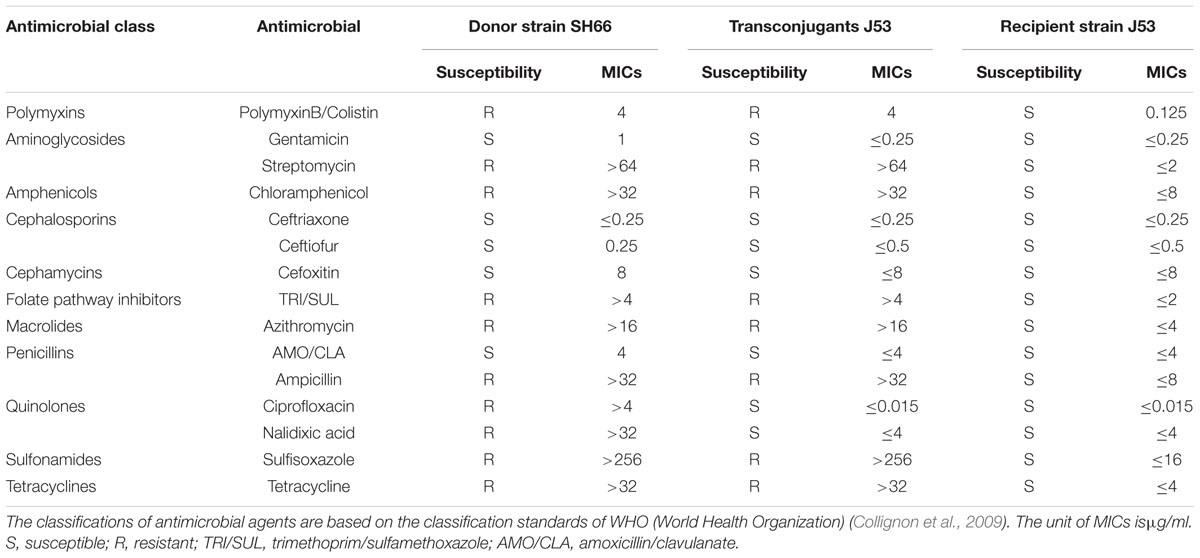
TABLE 2. Comparisons of antimicrobial resistance levels between the donor E. coli strain SH66, recipient strain J53 and the J53 transconjugants.
Conjugation Experiments and Southern Blotting
In order to determine the transferability of the mcr-1 gene carried by SH66, conjugation experiments were carried out using the E. coli strain J53 as a recipient, and SH66 as the donor strain. All transconjugants obtained in this experiment tested positive for the mcr-1 gene via PCR amplification and sequencing analysis. The conjugation transfer rate is 1.6 × 10-5. Colistin susceptibility testing showed that the MIC values for the transconjugants (the J53 strains carrying the mcr-1 gene) increased to 4 μg/ml (Table 2). This is much higher than the colistin resistance levels of the original J53 strains (which have MIC values of 0.125 μg/ml), and indicates that the transconjugants acquire the same colistin resistance gene as the donor strain.
The S1 endonuclease-PFGE and southern blotting analysis revealed that the mcr-1 gene in SH66 was located on a plasmid approximately 60 kb in length (Supplementary Figure S1).
Virulence and Resistance Factors Carried by the mcr-1-Harboring ETEC Strain SH66
The DNA of the E. coli strain SH66 identified in this study were subjected to 600-bp paired-end whole genome sequencing with 73.4-fold coverage using the MiSeq sequencer. A total of 1,364,690 reads and 405,565,025 clean bases were generated, which were assembled into 107 contigs with an N50 length of 216,913 bp. Bioinformatics analyses showed that this strain carries the virulence genes eltA, eltB, astA, eilA, lpfA, ltcA, and stb. Additionally, SH66 also carries several resistance genes, namely, blaTEM-1, mphA, ermB, aph(3’)-Ia, aadA5, strA, strB, sul1, sul2, tetA, tetM, floR, catA1, dfrA17, oqxA, and oqxB, along with the mcr-1 gene, all of which could mediate resistance to β-lactams, macrolides, aminoglycosides, sulphonamides, tetracyclines, phenicols, trimethoprim, quinolones, and colistin (Supplementary Table S1). The presence of these genes also explains the increase in resistance to several antibiotics observed in the E. coli J53 transconjugants after the conjugation experiment (Table 2). Analyses of the gyrA and parC point mutations carried by SH66 also showed that gyrA contained the Ser83→Leu and Asp87→Asn substitutions, along with the parC mutations Ser57→Thr and Ser80→Ile.
Identification and Analysis of the Plasmid Carrying the Novel Variant, mcr-1.9
An mcr-1-carrying plasmid of length 64,005 bp, which we have named pEC26, was identified in SH66 through sequence analysis. Interestingly, the mcr-1 gene identified on this plasmid was found to be a variant of the original mcr-1 due to an SNP (Supplementary Figure S2). The SNP was located at position 1036 (where a G→A transition had occurred), which results in the replacement of an aspartic acid residue with an asparagine residue (Asp346→Asn). Since the position of this SNP is different from those of all previously discovered mcr-1 variants, we named this variant mcr-1.9. The sequence analysis shows that there is no resistance gene in addition to mcr-1.9 located in plasmid pEC26.
The plasmid pEC26 belongs to the IncI2 group, the same plasmid incompatibility group as pHNSHP45, which was the first plasmid reported to harbor mcr-1 (Liu et al., 2015); pEC26 also harbors 86 predicted open reading frames (ORFs), and has a GC content of 42.55% with a plasmid backbone very similar to that of other IncI2-type plasmids, such as pmcr1-IncI2 (99% identity and 98% coverage, GenBank accession no. KU761326) (Li et al., 2016) and pWF-5-19C (99% identity and 99% coverage, KX505142) (Liu et al., 2017). A comparison of these plasmids and pHNSHP45 (KP347127) (Liu et al., 2015) shows that pEC26, pmcr1-IncI2, and pWF-5-19C contain an IS200 element before the mok gene instead of the IS683 found in pHNSHP45. However, the IS200 element in these plasmids is inserted before a 363 bp fragment that precedes the IS683 element in pHNSHP45 (Figures 1, 2A). This indicates that although these two IS elements are inserted into the same plasmid backbone, their positions are not the same. Like all the other plasmids mentioned here, pEC26 also contains the 2,607 bp DNA segment that includes the mcr (mcr-1 or mcr-1.9) and pap2 genes, which are integrated downstream of the nikB gene. Besides this, the mcr-1-associated ISApl1 element was not found in the pEC26 plasmid (Figures 1, 2B). The sequence of pEC26 (Supplementary Data Sheet S1) has been deposited with GenBank under the accession number MG946761.
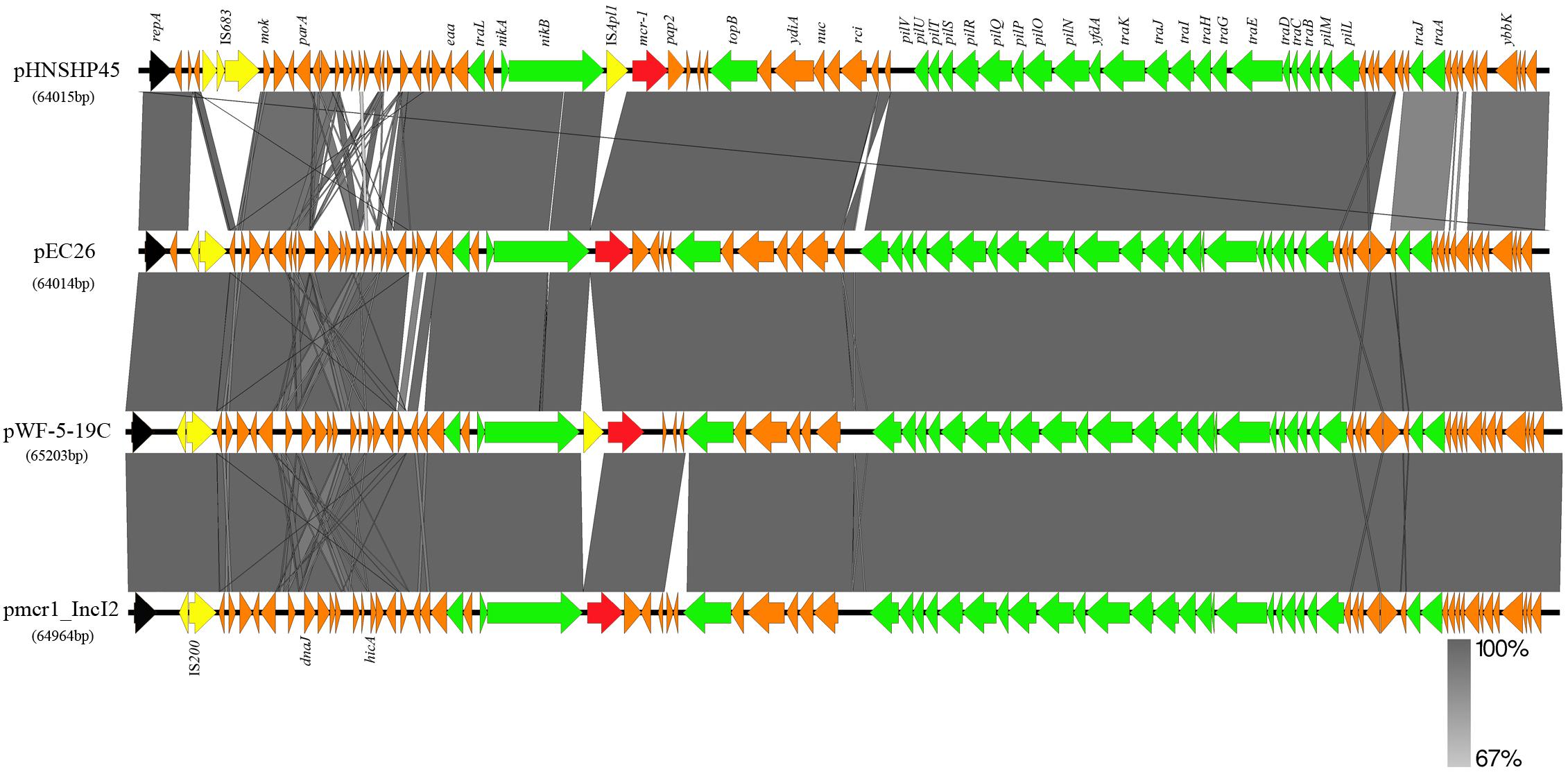
FIGURE 1. Linear comparisons of complete sequences of the IncI2-type plasmids pHNSHP45 (accession no. KP347127), pmcr1_IncI2 (KU761326), pWF-5-19C (KX505142), and pEC26 (MG946761). Colored arrows represent open reading frames, with black, yellow, green, red, and orange arrows representing replication genes, mobile elements, plasmid transfer genes, the mcr-1 gene, and plasmid backbone genes, respectively. Gray shading denotes regions of shared homology amongst the different plasmids.
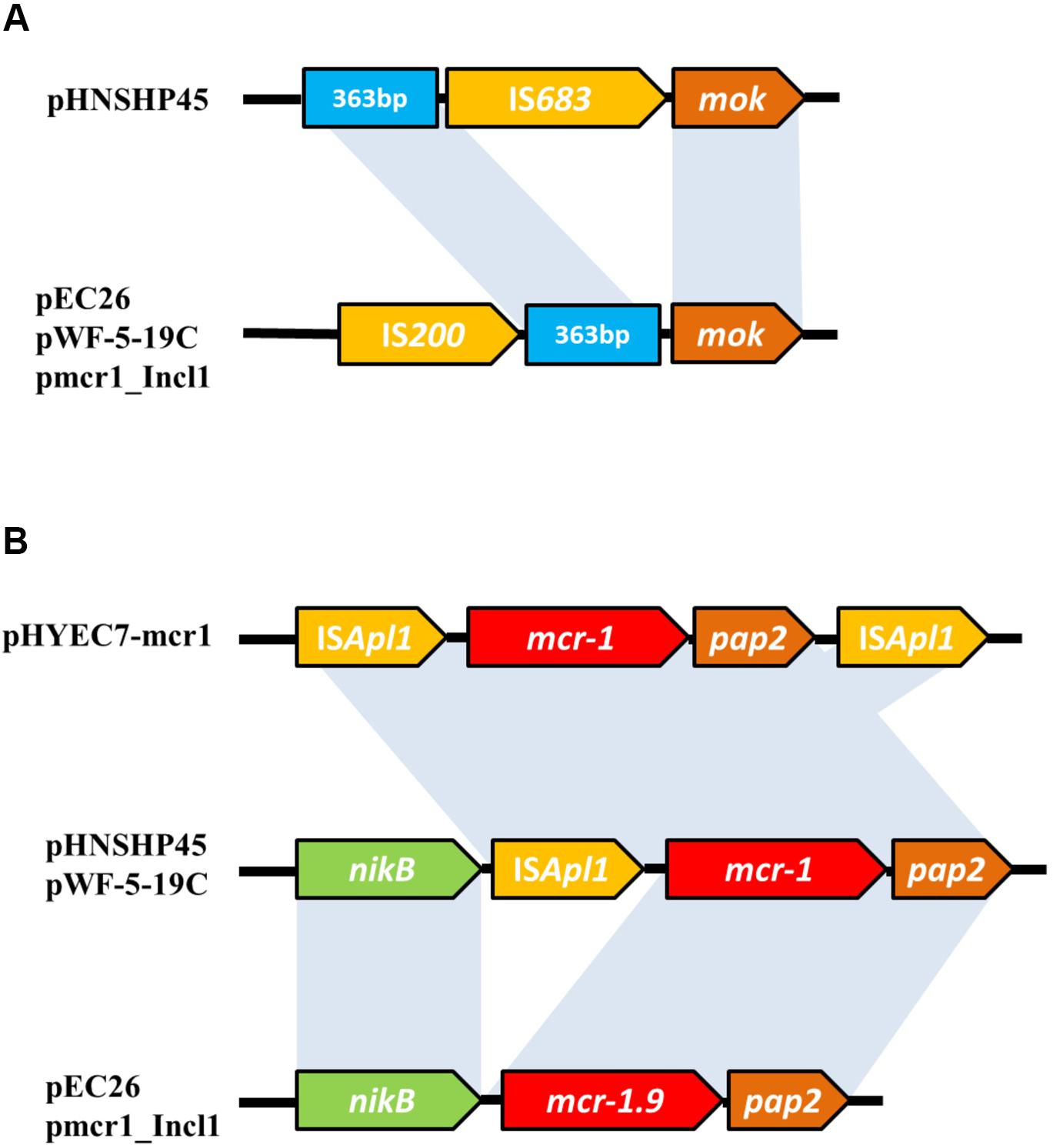
FIGURE 2. A comparison of the genetic environments of IS683 and IS200 (A), along with mcr-1 and mcr-1.9 (B) in the plasmids pHYEC7-mcr1 (Li et al., 2017), pHNSHP45, pWF-5-19C, pmcr1_Incl1 and pEC26. Gray shading indicates >99% nucleotide identity.
Discussion
This study reports the identification of a novel mcr-1 gene variant, mcr-1.9, which was harbored in an ETEC strain isolated from the diarrheal fecal sample of a 15-month-old male child. We find that the variant mcr-1.9, differs from the mcr-1 gene at position 1036 (where a G→A transition had occurred), which results in the replacement of an aspartic acid residue with an asparagine residue (Asp346→Asn). The MCR-1 protein consists of a transmembrane domain and a soluble catalytic domain. The transmembrane domain is located on the N-terminal, and is composed of residues 1–214, whereas the soluble catalytic domain is located on the C-terminal, and is composed of residues 215–541 (Stojanoski et al., 2016). Therefore, the Asp346→Asn change detected in mcr-1.9 is located in the catalytic domain. Since four of the known mcr-1 gene variants, mcr-1.4–1.7, also contain point mutations in the catalytic domain (Table 1), it appears that this domain has a higher mutation rate than the transmembrane domain. However, although the currently identified mutations in the mcr-1 gene have so far not showed obvious increases in colistin resistance, the presence of two or more point mutations in AMR genes can occasionally lead to changes in the strength of resistance imparted by the gene, such as the resistance increase to fluoroquinolones by the additional mutations in the gyrA and/or parC genes (Vila et al., 1994; Ruiz et al., 2002).
Apart from mutations causing heightened resistance to known antimicrobials, another concern regarding resistance genes is their spread through bacterial populations via horizontal transfer. The occurrence of the mcr-1 variant mcr-1.9 in an ETEC strain isolated from a human fecal sample is a cause of much concern; as the human intestine contains a complex collection of microflora where the incidence of horizontal gene transfer can occur at a high rate, the spread of this gene can cause widespread resistance to colistin. Added to this, several studies have shown that the mcr-1 gene can be ubiquitously disseminated in the Enterobacteriaceae (Du et al., 2016; Malhotra-Kumar et al., 2016; Yang et al., 2016). Furthermore, the mcr-1 gene is usually associated with the highly active transposon element ISApl1 that can occur either on one, or both sides of the gene (Li et al., 2017; Snesrud et al., 2017) (Figure 2B). The presence of ISApl1 could further enhance the probability of this gene being transferred horizontally; in vitro mobilization assays have already proved that the mcr-1 gene can be mobilized by ISApl1-mediated transposition (Poirel et al., 2017). Moreover, the transfer of mcr-1 via ISApl1-mediated transposition has been shown to generate mutations, leading to the formation of new mcr-1 gene variants (Poirel et al., 2017). We therefore hypothesize that mcr-1.9 and perhaps other variants of mcr-1 may have been generated during horizontal transfer events involving transposable elements.
The first reported mcr-1 gene was identified in an IncI2 plasmid (Liu et al., 2015). As of now, the mcr-1 gene and its variants have been reported in various plasmid backbones, including the IncI2, IncHI2, IncF, IncP, IncFIP, and IncX4 (Liu et al., 2015; Doumith et al., 2016; Falgenhauer et al., 2016; Nordmann et al., 2016), all of which are known to be vehicles disseminating antibiotic resistance genes among the Enterobacteriaceae. The mcr-1.9 gene identified by us was found on an IncI2-type plasmid, which has a broad host range (Lv et al., 2013). Furthermore, three [mcr-1.3 (KU934208), mcr-1.5 (KY471308), and mcr-1.7 (KY829117)] of the six mcr-1 variants [mcr-1.8 (KY683842) has no description of plasmid type] previously discovered are located on IncI2-type plasmids. Besides this, the plasmid most similar to pEC26 is pWF-5-19C (KX505142), which is found in Cronobacter sakazakii isolated from chicken (Liu et al., 2017). Based on this information, we speculate that pEC26 and plasmids similar to it have a strong ability to facilitate horizontal transfer of the mcr-1 gene, and that this gene may have a higher mutation rate when carried by IncI2-type plasmids than when they are present in other plasmid types. However, since only a few such instances have been identified so far, this hypothesis needs much further study for verification and confirmation.
Besides harboring the novel mcr-1 gene variant, mcr-1.9, the ETEC that we have isolated and described in this study was found to carry various virulence genes, namely, heat-stable enterotoxin (estA), heat-labile enterotoxin (eltB), EAST1 (enteroaggregative E. coli heat-stable toxin 1) gene (astA), adherence related gene (eilA), long polar fimbriae (lpfA), heat-labile enterotoxin A subunit (ltcA), and heat-stale enterotoxin II (stb). The strain was also found to carry several antimicrobial-resistance genes and shows MDR to at least nine classes of antibiotics (Table 2). These results indicate that this strain, called SH66, probably has high AMR and pathogenicity. Since mcr-1 variants have begun to appear in human intestinal bacteria, especially in diarrheal E. coli strains, it is very possible that the spread of this gene in human intestinal microflora may cause issues in the effective treatment of diarrheal conditions induced by bacterial infections.
Overall, our findings highlight the possibility of a widespread dissemination of mcr-1 gene variants, along with various virulence factors and resistance genes in the bacterial flora of the human intestine. Therefore, we believe that it is of utmost importance to track and identify mutations in the mcr-1 gene, and to control its transmission. We also believe that the judicious use of antibiotics by physicians is crucial to reduce the rate of emergence of MDR bacterial strains.
Author Contributions
HS, YS, and CY designed the experiments. HL and BZ performed the experiments. SQ, YX, LJ, and BL analyzed the data. PL, YL, and LY prepared the tables and figures. CY, YX, JX, and LW prepared the manuscript. All authors read and approved the final manuscript.
Funding
This work was supported by the National Key R&D Program of China (no. 2017YFC1600105), Mega-projects of Science and Technology Research, China (no. 2018ZX10714002), and the National Nature Science Foundation of China (nos. 81673237 and 81473023).
Conflict of Interest Statement
The authors declare that the research was conducted in the absence of any commercial or financial relationships that could be construed as a potential conflict of interest.
Supplementary Material
The Supplementary Material for this article can be found online at: https://www.frontiersin.org/articles/10.3389/fmicb.2018.00815/full#supplementary-material
FIGURE S1 | Plasmid profiling and southern blotting of SH66. The red box marks the plasmid pEC26. The genome DNA of Salmonella ser. Braenderup H9812 were restriction digested with XbaI enzyme and the products were used as the markers. The sizes of products are showed on the left with the unit “kb.”
FIGURE S2 | Nucleotide and protein sequence alignments of the mcr-1 gene with its different variants. Only the alignment blocks carrying mutations found in mcr-1.9 are shown. The mutations in the base and amino acid sequences are denoted by the use of a different color. In mcr-1.9, G was mutated to A in nucleotide position 1036, leading to the replacement of an Aspartic acid residue with an asparagine residue at amino acid position 346.
TABLE S1 | List of resistance and virulence factors/genes found in the mcr-1.9-harboring ETEC identified in this study.
DATA SHEET S1 | The complete sequence of mcr-1.9 harboring plasmid pEC26 (GenBank accession number is MG946761).
DATA SHEET S2 | The verification result files of the point mutation in mcr-1.9 by the PCR amplification and Sanger sequencing.
Footnotes
- ^ http://www.eucast.org/clinicalbreakpoints/
- ^ http://www.genomicepidemiology.org/
- ^ https://cge.cbs.dtu.dk/services/PlasmidFinder/
- ^ https://www-is.biotoul.fr/search.php
- ^ https://cge.cbs.dtu.dk/services/ResFinder/
- ^ http://blast.ncbi.nlm.nih.gov
References
Aguiar, J. M., Chacon, J., Canton, R., and Baquero, F. (1992). The emergence of highly fluoroquinolone-resistant Escherichia coli in community-acqntred urinary tract infections. J. Antimicrob. Chemother. 29, 349–350. doi: 10.1093/jac/29.3.349
Aziz, R. K., Bartels, D., Best, A. A., DeJongh, M., Disz, T., Edwards, R. A., et al. (2008). The RAST server: rapid annotations using subsystems technology. BMC Genomics 9:75. doi: 10.1186/1471-2164-9-75
Bennett, J. E., Dolin, R., and Blaser, M. J. (2014). Principles and Practice of Infectious Diseases. New York City, NY: Elsevier.
Bourgeois, A. L., Wierzba, T. F., and Walker, R. I. (2016). Status of vaccine research and development for enterotoxigenic Escherichia coli. Vaccine 34, 2880–2886. doi: 10.1016/j.vaccine.2016.02.076
CLSI (2014). Performance Standards for Antimicrobial Susceptibility Testing. CLSI Document M100-S24. Wayne, PA: Clinical and Laboratory Standards Institute.
Collignon, P., Powers, J. H., Chiller, T. M., Aidara-Kane, A., and Aarestrup, F. M. (2009). World Health Organization ranking of antimicrobials according to their importance in human medicine: a critical step for developing risk management strategies for the use of antimicrobials in food production animals. Clin. Infect. Dis. 49, 132–141. doi: 10.1086/599374
Darling, A. C., Mau, B., Blattner, F. R., and Perna, N. T. (2004). Mauve: multiple alignment of conserved genomic sequence with rearrangements. Genome Res. 14, 1394–1403. doi: 10.1101/gr.2289704
Del Canto, F., Valenzuela, P., Cantero, L., Bronstein, J., Blanco, J. E., Blanco, J., et al. (2011). Distribution of classical and nonclassical virulence genes in enterotoxigenic Escherichia coli isolates from Chilean children and tRNA gene screening for putative insertion sites for genomic islands. J. Clin. Microbiol. 49, 3198–3203. doi: 10.1128/JCM.02473-10
Di Pilato, V., Arena, F., Tascini, C., Cannatelli, A., Henrici De Angelis, L., Fortunato, S., et al. (2016). mcr-1.2, a New mcr variant carried on a transferable plasmid from a colistin-resistant KPC carbapenemase-producing Klebsiella pneumoniae strain of sequence type 512. Antimicrob. Agents Chemother. 60, 5612–5615. doi: 10.1128/AAC.01075-16
Diemert, D. J. (2006). Prevention and self-treatment of traveler’s diarrhea. Clin. Microbiol. Rev. 19, 583–594. doi: 10.1128/CMR.00052-05
Doumith, M., Godbole, G., Ashton, P., Larkin, L., Dallman, T., Day, M., et al. (2016). Detection of the plasmid-mediated mcr-1 gene conferring colistin resistance in human and food isolates of Salmonella enterica and Escherichia coli in England and Wales. J. Antimicrob. Chemother. 71, 2300–2305. doi: 10.1093/jac/dkw093
Du, H., Chen, L., Tang, Y. W., and Kreiswirth, B. N. (2016). Emergence of the mcr-1 colistin resistance gene in carbapenem-resistant Enterobacteriaceae. Lancet Infect. Dis. 16, 287–288. doi: 10.1016/S1473-3099(16)00056-6
Falgenhauer, L., Waezsada, S. E., Yao, Y., Imirzalioglu, C., Kasbohrer, A., Roesler, U., et al. (2016). Colistin resistance gene mcr-1 in extended-spectrum beta-lactamase-producing and carbapenemase-producing Gram-negative bacteria in Germany. Lancet Infect. Dis. 16, 282–283. doi: 10.1016/S1473-3099(16)00009-8
Khalifa, H. O., Ahmed, A. M., Oreiby, A. F., Eid, A. M., Shimamoto, T., and Shimamoto, T. (2016). Characterisation of the plasmid-mediated colistin resistance gene mcr-1 in Escherichia coli isolated from animals in Egypt. Int. J. Antimicrob. Agents 47, 413–414. doi: 10.1016/j.ijantimicag.2016.02.011
Li, A., Yang, Y., Miao, M., Chavda, K. D., Mediavilla, J. R., Xie, X., et al. (2016). Complete sequences of mcr-1-harboring plasmids from extended-spectrum-beta-Lactamase- and Carbapenemase-Producing Enterobacteriaceae. Antimicrob. Agents Chemother. 60, 4351–4354. doi: 10.1128/AAC.00550-16
Li, R., Xie, M., Lv, J., Wai-Chi Chan, E., and Chen, S. (2017). Complete genetic analysis of plasmids carrying mcr-1 and other resistance genes in an Escherichia coli isolate of animal origin. J. Antimicrob. Chemother. 72, 696–699. doi: 10.1093/jac/dkw509
Liu, B. T., Song, F. J., Zou, M., Hao, Z. H., and Shan, H. (2017). Emergence of colistin resistance gene mcr-1 in Cronobacter sakazakii producing NDM-9 and in Escherichia coli from the Same Animal. Antimicrob. Agents Chemother. 61, e01444–16. doi: 10.1128/AAC.01444-16
Liu, Y. Y., Wang, Y., Walsh, T. R., Yi, L. X., Zhang, R., Spencer, J., et al. (2015). Emergence of plasmid-mediated colistin resistance mechanism MCR-1 in animals and human beings in China: a microbiological and molecular biological study. Lancet Infect. Dis. 16, 161–168. doi: 10.1016/S1473-3099(15)00424-7
Liu, Y.-Y., Wang, Y., Walsh, T. R., Yi, L.-X., Zhang, R., Spencer, J., et al. (2016). Emergence of plasmid-mediated colistin resistance mechanism MCR-1 in animals and human beings in China: a microbiological and molecular biological study. Lancet Infect. Dis. 16, 161–168. doi: 10.1016/S1473-3099(15)00424-7
Lu, X., Hu, Y., Luo, M., Zhou, H., Wang, X., Du, Y., et al. (2017). MCR-1.6, a new MCR variant carried by an IncP plasmid in a colistin-resistant Salmonella enterica serovar Typhimurium isolate from a healthy individual. Antimicrob. Agents Chemother. 61, e02632-16. doi: 10.1128/AAC.02632-16
Lv, L., Partridge, S. R., He, L., Zeng, Z., He, D., Ye, J., et al. (2013). Genetic characterization of IncI2 plasmids carrying blaCTX-M-55 spreading in both pets and food animals in China. Antimicrob. Agents Chemother. 57, 2824–2827. doi: 10.1128/AAC.02155-12
Lynch, J. P. III, Clark, N. M., and Zhanel, G. G. (2013). Evolution of antimicrobial resistance among Enterobacteriaceae (focus on extended spectrum beta-lactamases and carbapenemases). Expert Opin. Pharmacother. 14, 199–210. doi: 10.1517/14656566.2013.763030
Malhotra-Kumar, S., Xavier, B. B., Das, A. J., Lammens, C., Hoang, H. T., Pham, N. T., et al. (2016). Colistin-resistant Escherichia coli harbouring mcr-1 isolated from food animals in Hanoi, Vietnam. Lancet Infect. Dis. 16, 286–287. doi: 10.1016/S1473-3099(16)00014-1
Nordmann, P., Lienhard, R., Kieffer, N., Clerc, O., and Poirel, L. (2016). Plasmid-mediated colistin-resistant Escherichia coli in bacteremia in Switzerland. Clin. Infect. Dis. 62, 1322–1323. doi: 10.1093/cid/ciw124
Oethinger, M., Conrad, S., Kaifel, K., Cometta, A., Bille, J., Klotz, G., et al. (1996). Molecular epidemiology of fluoroquinolone-resistant Escherichia coli bloodstream isolates from patients admitted to European cancer centers. Antimicrob. Agents Chemother. 40, 387–392.
Perrin-Guyomard, A., Bruneau, M., Houee, P., Deleurme, K., Legrandois, P., Poirier, C., et al. (2016). Prevalence of mcr-1 in commensal Escherichia coli from French livestock, 2007 to 2014. Euro Surveill. 21:30135. doi: 10.2807/1560-7917.ES.2016.21.6.30135
Poirel, L., Kieffer, N., and Nordmann, P. (2017). In vitro study of ISApl1-mediated mobilization of the colistin resistance gene mcr-1. Antimicrob. Agents Chemother. 61, e00127-17. doi: 10.1128/aac.00127-17
Qadri, F., Svennerholm, A. M., Faruque, A. S., and Sack, R. B. (2005). Enterotoxigenic Escherichia coli in developing countries: epidemiology, microbiology, clinical features, treatment, and prevention. Clin. Microbiol. Rev. 18, 465–483. doi: 10.1128/cmr.18.3.465-483.2005
Rao, M. C. (2008). Toxins which activate guanylate cyclase: heat-stable enterotoxins. Ciba Found. Symp. 112, 74–93.
Ribot, E. M., Fair, M. A., Gautom, R., Cameron, D. N., Hunter, S. B., Swaminathan, B., et al. (2006). Standardization of pulsed-field gel electrophoresis protocols for the subtyping of Escherichia coli O157:H7, Salmonella, and Shigella for PulseNet. Foodborne Pathog. Dis. 3, 59–67. doi: 10.1089/fpd.2006.3.59
Rodas, C., Mamani, R., Blanco, J., Blanco, J. E., Wiklund, G., Svennerholm, A. M., et al. (2011). Enterotoxins, colonization factors, serotypes and antimicrobial resistance of enterotoxigenic Escherichia coli (ETEC) strains isolated from hospitalized children with diarrhea in Bolivia. Braz. J. Infect. Dis. 15, 132–137.
Ruiz, J., Gomez, J., Navia, M. M., Ribera, A., Sierra, J. M., Marco, F., et al. (2002). High prevalence of nalidixic acid resistant, ciprofloxacin susceptible phenotype among clinical isolates of Escherichia coli and other Enterobacteriaceae. Diagn. Microbiol. Infect. Dis. 42, 257–261. doi: 10.1016/S0732-8893(01)00357-1
Snesrud, E., Ong, A. C., Corey, B., Kwak, Y. I., Clifford, R., Gleeson, T., et al. (2017). Analysis of serial isolates of mcr-1-positive Escherichia coli reveals a highly active ISApl1 transposon. Antimicrob. Agents Chemother. 61, e00056-17. doi: 10.1128/AAC.00056-17
So, M., Dallas, W. S., and Falkow, S. (1978). Characterization of an Escherichia coli plasmid encoding for synthesis of heat-labile toxin: molecular cloning of the toxin determinant. Infect. Immun. 21, 405–411.
Stojanoski, V., Sankaran, B., Prasad, B. V., Poirel, L., Nordmann, P., and Palzkill, T. (2016). Structure of the catalytic domain of the colistin resistance enzyme MCR-1. BMC Biol. 14:81. doi: 10.1186/s12915-016-0303-0
Sullivan, M. J., Petty, N. K., and Beatson, S. A. (2011). Easyfig: a genome comparison visualizer. Bioinformatics 27, 1009–1010. doi: 10.1093/bioinformatics/btr039
Suzuki, S., Ohnishi, M., Kawanishi, M., Akiba, M., and Kuroda, M. (2016). Investigation of a plasmid genome database for colistin-resistance gene mcr-1. Lancet Infect. Dis. 16, 284–285. doi: 10.1016/S1473-3099(16)00008-6
Tijet, N., Faccone, D., Rapoport, M., Seah, C., Pasteran, F., Ceriana, P., et al. (2017). Molecular characteristics of mcr-1-carrying plasmids and new mcr-1 variant recovered from polyclonal clinical Escherichia coli from Argentina and Canada. PLoS One 12:e0180347. doi: 10.1371/journal.pone.0180347
Vila, J., Ruiz, J., Marco, F., Barcelo, A., Goñi, P., Giralt, E., et al. (1994). Association between double mutation in gyrA gene of ciprofloxacin-resistant clinical isolates of Escherichia coli and MICs. Antimicrob. Agents Chemother. 38, 2477–2479. doi: 10.1128/aac.38.10.2477
von Sonnenburg, F., Tornieporth, N., Waiyaki, P., Lowe, B., Peruski, L. F., DuPont, H. L., et al. (2000). Risk and aetiology of diarrhoea at various tourist destinations. Lancet 356, 133–134. doi: 10.1016/S0140-6736(00)02451-X
World Health Organization [WHO] (2018). Antimicrobial Resistance. Geneva: World Health Organization.
Xavier, B. B., Lammens, C., Ruhal, R., Kumar-Singh, S., Butaye, P., Goossens, H., et al. (2016). Identification of a novel plasmid-mediated colistin-resistance gene, mcr-2, in Escherichia coli, Belgium, June 2016. Euro Surveill. 21:30280. doi: 10.2807/1560-7917.ES.2016.21.27.30280
Yang, Y. Q., Li, Y. X., Song, T., Yang, Y. X., Jiang, W., Zhang, A. Y., et al. (2017). Colistin resistance gene mcr-1 and its variant in Escherichia coli isolates from chickens in China. Antimicrob. Agents Chemother. 61, e01204-16. doi: 10.1128/AAC.01204-16
Yang, Y. Q., Zhang, A. Y., Ma, S. Z., Kong, L. H., Li, Y. X., Liu, J. X., et al. (2016). Co-occurrence of mcr-1 and ESBL on a single plasmid in Salmonella enterica. J. Antimicrob. Chemother. 71, 2336–2338. doi: 10.1093/jac/dkw243
Zhao, F., Feng, Y., Lu, X., McNally, A., and Zong, Z. (2017a). IncP plasmid carrying colistin resistance gene mcr-1 in Klebsiella pneumoniae from hospital sewage. Antimicrob. Agents Chemother. 61, e02229-16.
Zhao, F., Feng, Y., Lu, X., McNally, A., and Zong, Z. (2017b). Remarkable Diversity of Escherichia coli carrying mcr-1 from hospital sewage with the identification of two new mcr-1 variants. Front. Microbiol. 8:2094. doi: 10.3389/fmicb.2017.02094
Keywords: mobilized colistin resistance (mcr-1) gene, novel mcr-1 variant, clinical diarrhea, enterotoxigenic Escherichia coli (ETEC), multidrug resistance (MDR)
Citation: Liu H, Zhu B, Liang B, Xu X, Qiu S, Jia L, Li P, Yang L, Li Y, Xiang Y, Xie J, Wang L, Yang C, Sun Y and Song H (2018) A Novel mcr-1 Variant Carried by an IncI2-Type Plasmid Identified From a Multidrug Resistant Enterotoxigenic Escherichia coli. Front. Microbiol. 9:815. doi: 10.3389/fmicb.2018.00815
Received: 17 February 2018; Accepted: 10 April 2018;
Published: 25 April 2018.
Edited by:
Dongsheng Zhou, Beijing Institute of Microbiology and Epidemiology, ChinaReviewed by:
Xinglin Zhang, Zhejiang University, ChinaMeiying Yan, National Institute for Communicable Disease Control and Prevention, China
Séamus Fanning, University College Dublin, Ireland
Xianming Shi, Shanghai Jiao Tong University, China
Copyright © 2018 Liu, Zhu, Liang, Xu, Qiu, Jia, Li, Yang, Li, Xiang, Xie, Wang, Yang, Sun and Song. This is an open-access article distributed under the terms of the Creative Commons Attribution License (CC BY). The use, distribution or reproduction in other forums is permitted, provided the original author(s) and the copyright owner are credited and that the original publication in this journal is cited, in accordance with accepted academic practice. No use, distribution or reproduction is permitted which does not comply with these terms.
*Correspondence: Chaojie Yang, jettyang@163.com Yansong Sun, sunys1964@hotmail.com Hongbin Song, hongbinsong@263.net
†These authors have contributed equally to this work.