- 1Microbial Ecology, Groningen Institute for Evolutionary Life Sciences, University of Groningen, Groningen, Netherlands
- 2Department of Microbiology, University of Haripur, Haripur, Pakistan
- 3Molecular Microbiology, Groningen Biomolecular Sciences and Biotechnology Institute, University of Groningen, Groningen, Netherlands
Fungal-associated Paraburkholderia terrae strains in soil have been extensively studied, but their sensing strategies to locate fungi in soil have remained largely elusive. In this study, we investigated the behavior of five mycosphere-isolated P. terrae strains [including the type-3 secretion system negative mutant BS001-ΔsctD and the type strain DSM 17804T] with respect to their fungal-sensing strategies. The putative role of oxalic acid as a signaling molecule in the chemotaxis toward soil fungi, as well as a potential carbon source, was assessed. First, all P. terrae strains, including the type strain, were found to sense, and show a chemotactic response toward, the different levels of oxalic acid (0.1, 0.5, and 0.8%) applied at a distance. The chemotactic responses were faster and stronger at lower concentrations (0.1%) than at higher ones. We then tested the chemotactic responses of all strains toward exudates of the soil fungi Lyophyllum sp. strain Karsten and Trichoderma asperellum 302 used in different dilutions (undiluted, 1:10, 1:100 diluted) versus the control. All P. terrae strains showed significant directed chemotactic behavior toward the exudate source, with full-strength exudates inciting the strongest responses. In a separate experiment, strain BS001 was shown to be able to grow on oxalate-amended (0.1 and 0.5%) mineral medium M9. Chemical analyses of the fungal secretomes using proton nuclear magnetic resonance (1H NMR), next to high-performance liquid chromatography (HPLC), indeed revealed the presence of oxalic acid (next to glycerol, acetic acid, formic acid, and fumaric acid) in the supernatants of both fungi. In addition, citric acid was found in the Lyophyllum sp. strain Karsten exudates. Given the fact that, next to oxalic acid, the other compounds can also serve as C and energy sources for P. terrae, the two fungi clearly offer ecological benefits to this bacterium. The oxalic acid released by the two fungi may have primarily acted as a signaling molecule, and, as a “second option,” a carbon source for P. terrae strains like BS001.
Introduction
Bacteria in soil, most often, encounter conditions of nutrient (carbon source) scarcity (Van Elsas et al., 2007). Hence, the hospitable microhabitats at plants and fungi, where carbonaceous compounds become available, constitute attractive refuges. To localize such potential niches, soil bacteria depend on the genetic traits that enable them to perceive potential signals, leading to chemotaxis and guiding them toward hospitable microenvironments. Bacteria that have a symbiotic phase included in their lifestyle are particularly dependent on processes of recognition and positive movement. In previous studies, several fungi in soil have been found to release carbon- and energy-yielding compounds like oxalic acid (Sullivan et al., 2012). Such compounds are used by soil fungi to scavenge metals and release inorganic nutrients (Van Hees et al., 2006; Adeleke et al., 2012). In some plant-associated fungi as well as in fungi involved in the decay of litter and wood, oxalic acid can even form complexes – in the form of oxalate bound to cations like Ca2+ – on the hyphal tips (Dutton and Evans, 1996; Bhatti et al., 1998; Harrold and Tabatabai, 2006; Van Hees et al., 2006; Heller and Witt-Geiges, 2013). A limited range of soil bacteria is known to be able to use oxalic acid and its complexes as a potential carbon source, in sites under the direct influence of fungi (Bravo et al., 2013) or plants (Franceschi and Nakata, 2005). Interestingly, oxalic acid has recently been shown to act as a signaling molecule for bacteria of the genus Collimonas (Rudnick et al., 2015). In addition, it was shown to serve as a carbon source for several Paraburkholderia species, including the plant-associated Paraburkholderia phytofirmans PsJN (Kost et al., 2014).
The genus Paraburkholderia encompasses different species, of which some can interact with soil fungi. Examples are Paraburkholderia terrae, in particular strain BS001 and the related strains BS110, BS007, and BS437 (Nazir et al., 2012, 2014). These strains have been shown to harbor genetic systems that furnish the capacity to obtain ecological benefits from fungi in soil (Nazir et al., 2012; Haq et al., 2014). Among such systems, those responsible for chemotaxis and flagellar movement are of prime importance (Yang et al., 2017), as they underlie the active exploration by the bacterium, via fungal-assisted migration, of the soil for hospitable space, in which sensing the putative chemoattractants released by soil fungi can play a role. Boersma et al. (2010) had already shown that Lyophyllum sp. strain Karsten could release glycerol into the medium it is grown in. Later, Nazir et al. (2013) provided evidence for the contention that the interaction between Lyophyllum sp. strain Karsten and P. terrae BS001 (in liquid microcosms) promoted the release of glycerol by the fungus, spurring the growth of the bacterium. Strain BS001 was also able to grow actively in minimal medium supplemented with glycerol as the sole carbon source (Nazir et al., 2013). However, evidence for the production of oxalic acid by Lyophyllum sp. strain Karsten (as well as Trichoderma asperellum 302), and its putative role in their interactions with P. terrae strains BS001, BS110, BS007, and BS437, has been lacking.
In this study, we tested the hypothesis that Lyophyllum sp. strain Karsten and T. asperellum 302 exude different small carbonaceous compounds, including oxalic acid, which attract, and offer ecological benefits to, the fungal-interactive P. terrae strains BS001, BS110, BS007, and BS437, next to the type strain DSM 17804T. We used simple microcosm setups to test bacterial behavior, and performed proton nuclear magnetic resonance (1H NMR) and high-performance liquid chromatography (HPLC) to investigate the nature of the fungal-released chemical compounds. We further hypothesized that oxalic acid acts primarily as a signaling molecule for the fungal-associated B terrae strains.
Materials and Methods
Bacterial Strains, Growth Conditions, and Media
In this study, we used four fungal-interactive Paraburkholderia strains, i.e., BS001, BS110, BS007, and BS437, next to a type-3 secretion system (TTSS) (ΔsctD) mutant of strain BS001 (Yang et al., 2016) and type strain DSM 17804T. All strains were maintained at -80°C, and then kept as inoculum sources on R2A agar at 25°C. All P. terrae cultures used were grown overnight in Luria–Bertani (LB) broth at 28°C, with shaking at 180 rpm. At harvest, the cultures were subjected to centrifugation at 12,000 ×g for 3 min. The bacterial pellets were then double-washed with sterile saline (0.85% NaCl solution). For all experiments on chemotaxis, cultures were centrifuged at 1,057 ×g for 20 min and the resulting pellets washed twice with morpholine ethane sulfonic (MES) acid buffer, containing 1 g of KH2PO4 and 1 g (NH4)2SO4 per L; pH 5.6 (Rudnick et al., 2015).
Chemotaxis Assays
The chemotaxis assays were performed in M9-G agar Petri plates, with the final concentration of the agar set at 0.25% [M9 agar for testing of swimming motility (M9A-S)]. M9-G medium consisted of 6.76 g of Na2HPO4, 3 g of KH2PO4, 0.5 g NaCl, and 1 g of NH4Cl per L MilliQ water (pH 6.8), supplemented with 0.5% glycerol and 2 mL (per L) of filter-sterilized (0.2 μm) 1 M MgSO4 and 100 μL (per L) of 1 M CaCl2.
To set up the experiments with oxalic acid as well as fungal exudates, molten M9-G agar [1.2% (w/v)] was mixed 1:1 with either oxalic acid [final levels 0.1–0.5 and 0.8% (w/v)] or different levels of fungal exudates (undiluted, 1:10, and 1:100 diluted). Controls contained either no oxalic acid (oxalic acid control) or no exudates (exudate control). Following solidification of the agar, rectangular (15 mm × 5 mm) stripes were severed from the media and introduced as “target stripes” in the chemotaxis assay Petri plates.
The washed bacterial cells resuspended in MES buffer were adjusted to establish approximate cell numbers of 107 CFU/mL. The suspensions were then introduced as 5-μL stripes onto the Petri plates at distances of about 15 mm from, and parallel with, the agar stripes containing either oxalic acid [0 (controls), 0.1, 0.5, and 0.8% (w/v)] or fungal exudates [zero (controls), undiluted, 1:10, and 1:100 diluted]. The Petri plates were incubated at 25°C for up to 48 h, and readings [distances traveled in millimeters, in the forward (“toward”) and backward (“away from”) directions] were taken manually at set times.
Growth of P. terrae Strain BS001 on Oxalate as the Sole Carbon and Energy Source
Washed cells of P. terrae strain BS001 were introduced, at estimated levels of 107 cells/mL, into triplicate (per treatment) 100-mL Erlenmeyer flasks containing 20 mL of M9 medium supplemented with zero (negative control), 0.1, or 0.5% of oxalate or glucose (positive control). The flasks were incubated at 28°C, with shaking at 150 rpm, and samples were aseptically taken at set times for CFU enumerations. The samples were dilution-plated on LB agar plates and CFU counts determined following incubation of the plates at 28°C for up to 1 week.
Fungal Strains, Growth Media, and Conditions to Obtain Exudates
For long-term maintenance, Lyophyllum sp. strain Karsten and T. asperellum 302 were maintained in autoclaved distilled water. For routine use, the fungi were refreshed once a month on oat flake agar (OFA) plates. For analysis of their respective secretomes, both fungal strains were first grown for 48–72 h in oat flake medium at 28°C with shaking. The well-grown fungal cultures were then centrifuged at 2,655 ×g (FA-45-30-11 rotor, Eppendorf) for 10 min and the pellets washed twice with M9. M9 medium supplemented with 1% [w/v] sodium propionate (pH 4.8) was then used for fungal growth for the preparation of fungal secretomes. Thus, Erlenmeyer flasks containing 50 mL of medium were inoculated with the respective strains and incubated for two weeks at 28°C, with shaking.
Harvesting Fungal Exudates and Sample Preparation for 1H NMR
Well-grown 50-mL cultures of both Lyophyllum sp. strain Karsten and T. asperellum 302 were harvested by centrifugation at 4,226 × g (F-35-6-30 rotor, Eppendorf) for 10 min. The resulting supernatants were then filtered through 0.2 μm filters (Whatman; FP 30/0.2 CA-S), to remove fungal hyphae and debris. The filtrates containing the exudates were stored at -20°C. For 1H NMR analyses, samples were subsequently defrosted at room temperature. To 570 μL of filtrate, 30 μL of D2O containing 3-(trimethylsilyl)-1-propanesulfonic acid sodium salt (DSS; 0.1 mM final concentration) were added (as internal standard). To minimize pH-based peak shifts, the pH for all samples was adjusted to 7.0 with either HCl or NaOH. For 1H NMR data acquisition, aliquots of 600 μL were transferred to Bruker NMR tubes.
1H NMR Data Acquisition and Analysis
The 1H NMR spectra were acquired on a Bruker DRX400 spectrometer 500-MHz (Bruker Spectrospin, Toronto, ON, Canada). The 1H NMR spectra were further processed and analyzed using MestReNova (version 9.0.0-12821) software. For identification, spectra obtained were compared with spectra of known compounds using the Human Metabolome Database (HMDB).
High-Performance Liquid Chromatography (HPLC)-Based Analysis of Fungal Exudates
Oxalic acid levels were determined in the exudates of both fungi, using a C18 reverse phase column (id 5 μm; d 4.6 mm × 250 mm) on a Shimadzu UPLC device (Shimadzu Corp., Kyoto, Japan). An isocratic method was chosen, using a 25 mM K-phosphate buffer with a pH of 2.4 as the mobile phase. The pump flow was set at 1.5 mL/min and the column temperature at 30°C. Then, samples at 4°C (20 μL) were run [sample injection followed 30 s after run initiation with a total length of 14 min]. The chromatogram was subsequently evaluated at 210 nm. Peak areas were extracted using the Shimadzu software. The oxalic acid concentration was derived using an external standard at concentrations of 0.01, 0.05, 0.10, 0.25, 0.5, and 1.0% (w/v). Corresponding peaks were further identified using 0.1% oxalic acid internal sample spiking.
Analysis of Putative Oxalate Degradation Pathways in Paraburkholderia Genomes
We used the MetaCyc database and specifically examined the available P. terrae genomes (strains BS001, BS110, BS007, and BS437) for oxalate degradation pathways. The P. terrae BS001 genome was published in Haq et al. (2014), and the genomes of strains BS007, BS110, and BS437 in Pratama et al. (2017). We did a directed search in the aforementioned genomes hosted at MicroScope (Vallenet et al., 2013) for the presence and absence of any of the five known pathways (I–V) of oxalate degradation.
Statistical Analyses
All chemotaxis experiments had four biological replications. Full factorial ANOVA was performed using RStudio Version 0.99.893 – © 2009–2016 RStudio, Inc. The input data consisted of the distances (measured in mm) traveled by the bacterial strains in two directions (“toward” and “away”). The confidence interval of 95% was used in all the analyses.
Results
Chemotactic Behavior of Fungal-Interactive Paraburkholderia terrae Strains Exposed to Various Concentrations of Oxalic Acid
To understand the ecological behavior at fungi of the selected P. terrae strains, we tested their chemotactic (swimming) responses (Figures 1–3 and Supplementary Figure S1) on M9-G agar. Oxalic acid in different concentrations [0.1, 0.5, 0.8, and 0% (control)] was used as the putative chemoattractant in the used agar stripes. Full factorial ANOVA (Supplementary Table S1) of the collective data revealed that the factors oxalic acid level and movement type (toward or away from the attractant) were the key drivers of the chemotactic responses of all P. terrae strains. This included the type strain, which a priori had not been selected for fungal interactivity. First, without any added oxalate, the swimming behavior of all strains was “random” in the sense that the movement “toward” versus “away” was never different (P > 0.05). Second, the chemotactic responses of all strains toward the oxalic acid source were significantly faster and higher (P < 0.05) than away from it, clearly suggesting that positive chemotaxis took place. Remarkably, the responses were stronger at the lowest concentration of oxalic acid (0.1%) than at the two higher concentrations (P < 0.05).
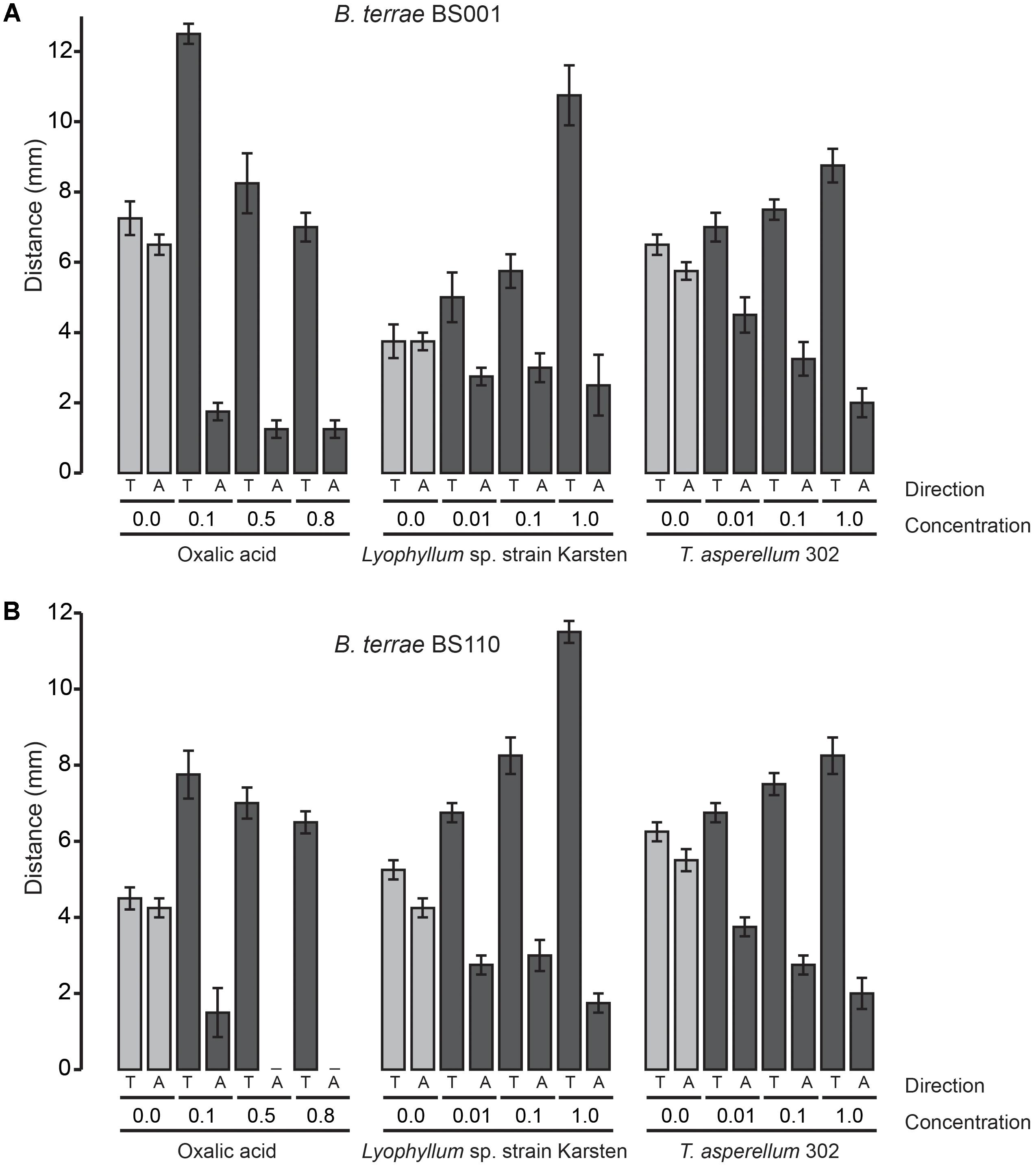
FIGURE 1. Chemotaxis (swimming) of P. terrae strains BS001 and BS110 toward oxalate and exudates of Lyophyllum sp. strain Karsten and T. asperellum 302 on 0.25% (w/v) M9 medium. (A) The response of P. terrae BS001 to either oxalate or exudates of both fungal strains. (B) The response of P. terrae BS110 either oxalate or exudates of both fungal strains. The Y-axis represents the distance in mm, whereas the X-axis shows the direction of taxis (“toward” is denoted by “T” and “away” by “A”) and concentration of oxalate or levels of fungal exudates. Data are representative of four biological replicates.
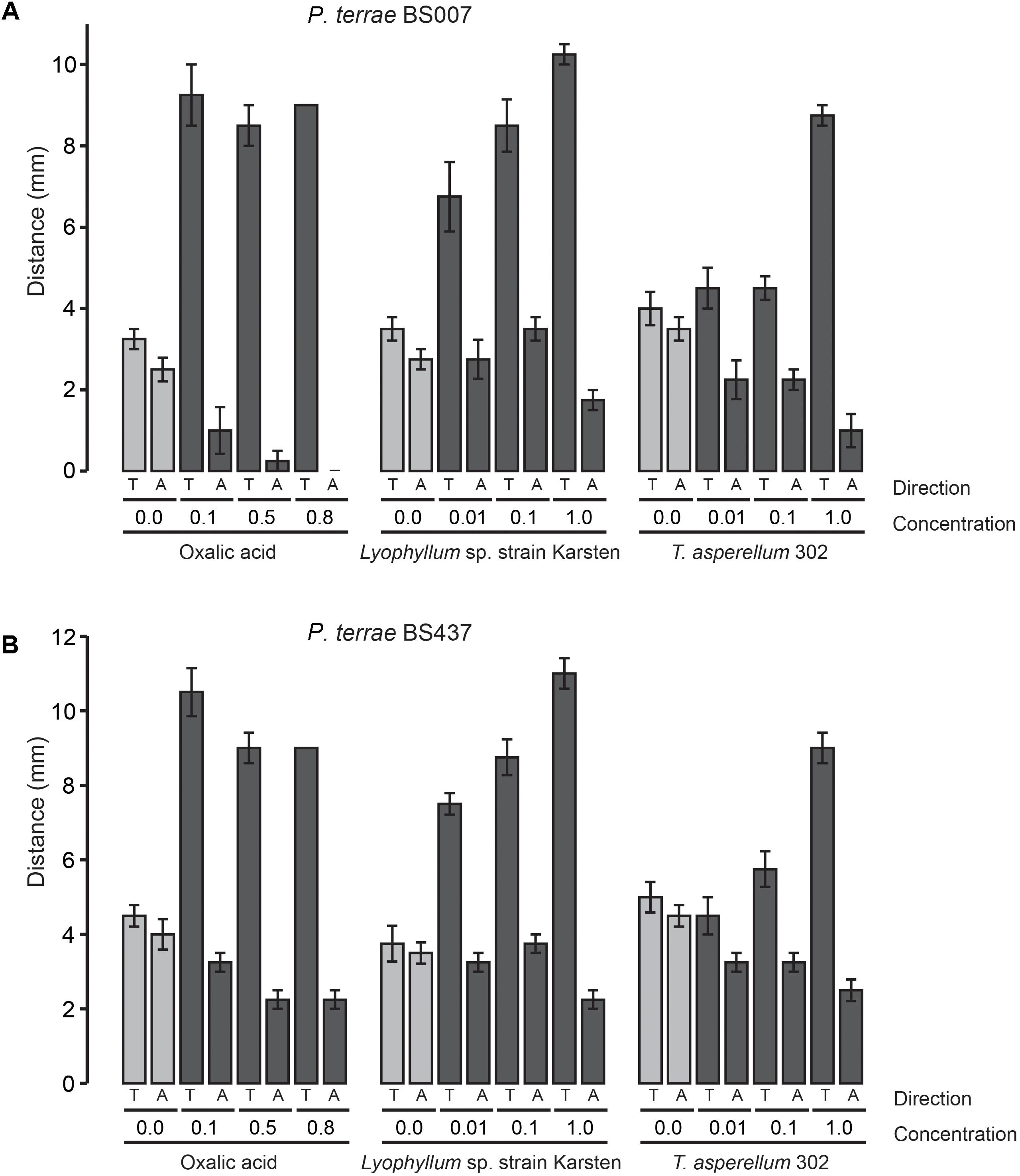
FIGURE 2. Chemotactic (swimming) behavior of P. terrae strains BS007 and BS437 toward oxalate and exudates of Lyophyllum sp. strain Karsten and T. asperellum 302 on 0.25% (w/v) M9 medium. (A) The response of P. terrae BS007 to either oxalate or exudates of both fungal strains. (B) The response of P. terrae BS437 to either oxalate or exudates of both fungal strains. The Y-axis represents distance in mm, whereas the X-axis shows the direction of taxis (toward denoted by “T” and away denoted by “A”) and concentration of oxalate or levels of exudates. Data are representative of four biological replicates.
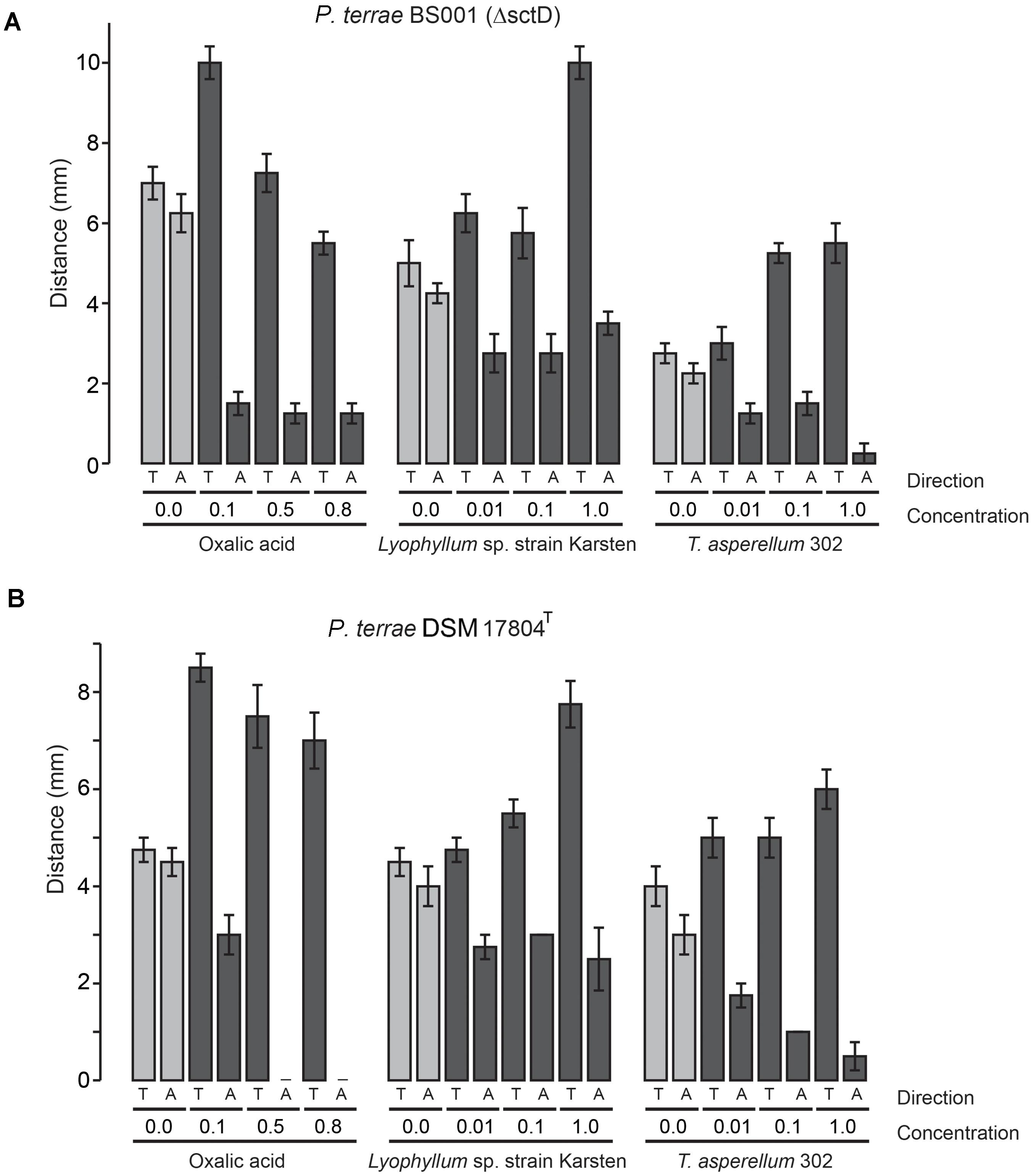
FIGURE 3. Chemotactic (swimming) behavior of P. terrae strains BS001-ΔsctD and DSM 17804T toward oxalate and exudates of Lyophyllum sp. strain Karsten and T. asperellum 302 on 0.25% (w/v) M9 medium. (A) The response of P. terrae BS001-ΔsctD to either oxalate or exudates of both fungal strains. (B) The response of P. terrae DSM 17804T to either oxalate or exudates of both fungal strains The Y-axis represents the distance in mm, whereas the X-axis shows the direction of taxis (toward denoted by “T” and away denoted by “A”) and concentration of oxalate or levels of exudates. Data are representative of four biological replicates.
Chemotactic Responses of Fungal-Interactive Paraburkholderia terrae Strains Toward Exudates of Lyophyllum sp. Strain Karsten and T. asperellum 302
We then tested the chemotactic responses of all P. terrae strains upon confrontation with exudates from Lyophyllum sp. strain Karsten as well as T. asperellum 302, at the different levels. First, the swimming behavior of all strains in response to control stripes (no fungal exudate added) was undirected, as all strains apparently explored the milieu around the inoculum similarly in both directions (toward versus away; P > 0.05). Interestingly, all P. terrae strains showed a strong chemotactic response toward the M9 agar stripes containing fungal exudates (full-strength, 1:10 diluted and 1:100 diluted), as compared to the movement away (P < 0.05). A full factorial ANOVA (Supplementary Table S2) of the data showed that the movement type (“toward” versus “away”), fungal type, bacterial strain and exudate level in the stripes were the factors driving the chemotactic behavior of all P. terrae strains (including the type strain DSM 17804T). The chemotactic responses were stronger toward the exudates of Lyophyllum sp. strain Karsten than to those of T. asperellum 302 (data of all strains are shown in Figures 1–3). There were differences in the movement of the strains toward the exudates (full-strength) of both fungi, with strains BS110, BS437, BS001, and BS007 being more “proactive” than strain DSM 17804T.
With respect to the responses to T. asperellum 302, the different P. terrae strains reacted differently to the same concentrations of the exudates, with strains BS110, BS437, BS001, and BS007 being more “avid” responders than strains DSM 17804T and BS001-ΔsctD. ANOVA (Supplementary Table S2) of the data revealed that three factors, i.e., exudate level, taxis direction (toward/away) and P. terrae strain, were the key variables that drove the chemotactic responses. Thus, there were significant differences (P < 0.05) in the responses of all P. terrae strains toward full-strength exudates (1%) compared to the diluted ones (0.01 and 0.1%) and the control (0%). At higher concentration (1%), the bacterial taxis was significantly one-directional (toward the stripes) and stronger (P < 0.05).
Identification and Quantification of Oxalic Acid in the Exudates of Lyophyllum sp. Strain Karsten and T. asperellum 302
Previous work (Boersma et al., 2010) already analyzed Lyophyllum sp. strain Karsten exudates by 1H NMR; however, this method cannot detect oxalic acid. Hence, here we examined the exudates of Lyophyllum sp. strain Karsten as well as T. asperellum 302 for the presence of oxalic acid using HPLC analyses over a C18 reverse phase column (id 5 μm; d 4.6 mm × 250 mm). Control runs of progressively increasing levels of pure oxalic acid revealed the appearance of progressively bigger peaks, at retention time 2.4 min, in the resulting chromatograms (Supplementary Figure S2). The exudates from both fungi were then examined. Both showed the appearance of peaks at the exact same retention time, and thus oxalic acid was presumably produced. Quantification of the peaks revealed that considerable amounts had been released by both fungi into the propionate-amended M9 medium, with final oxalic acid levels being in the range 0.075–0.093% (w/v; Table 1). To validate the identification of the respective peaks in the chromatogram, we spiked the respective fungal exudates with 0.1% (w/v) of oxalic acid in the same HPLC run (Supplementary Figure S2). Indeed, the added 0.1% oxalic acid about doubled the peak weight and so the measured amount of oxalic acid. Together, our results suggest that oxalic acid is indeed released by both fungal types, in rather similar quantities, under the applied conditions.
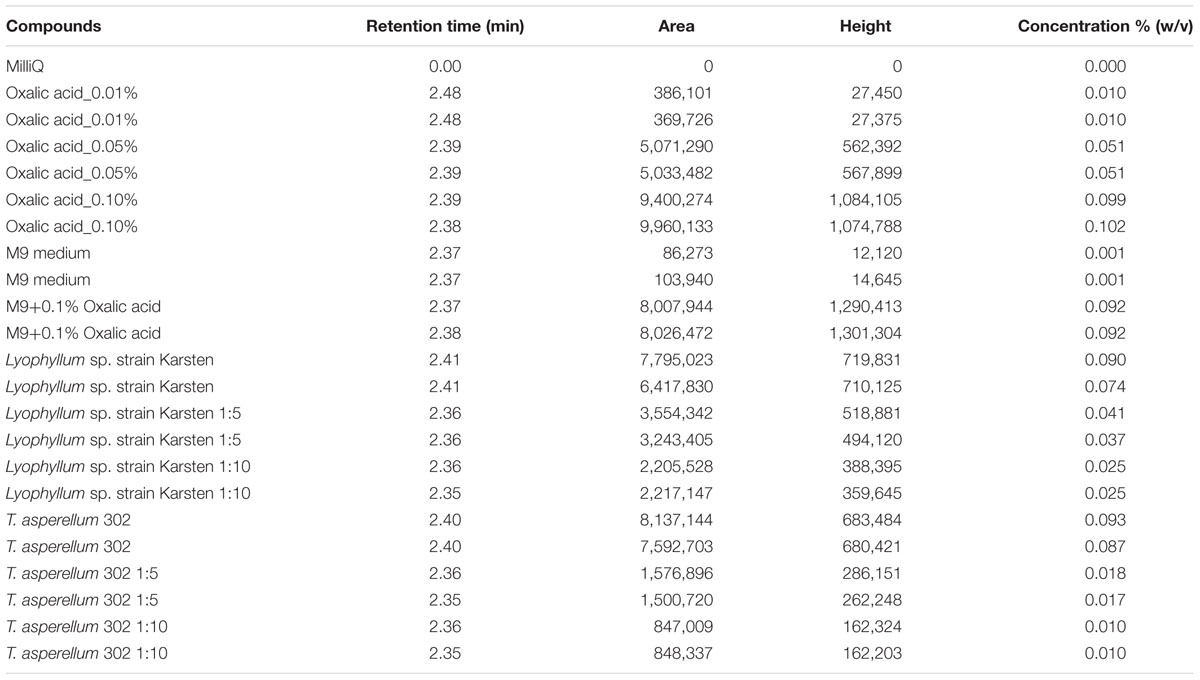
TABLE 1. Quantitative analysis of the exudates of Lyophyllum sp. strain Karsten and Trichoderma asperellum 302 for the identification of oxalic acid, using high-performance liquid chromatography (HPLC). Oxalic acid was used as an external standard and M9 medium as a negative control.
1H NMR-Based Analyses of the Exudates Released by Lyophyllum sp. Strain Karsten in Propionate-Amended M9 Medium
To underpin the chemotaxis findings, we then re-analyzed the exudates of Lyophyllum sp. strain Karsten, using 1H NMR. The resulting spectra showed the existence of various peaks, indicating that Lyophyllum sp. strain Karsten had released various small compounds. The control M9+propionate medium, as well as that containing potential fungal exudates, both showed two large peaks at 1.04 and 2.16 ppm, respectively, which were both attributed to propionate (Figure 4A). The potential exudate spectra further revealed a multiplet corresponding to glycerol, as found earlier (Boersma et al., 2010; from 3.54 to 3.80 ppm). Expectedly, acetic acid (1.91 ppm) and formic acid (8.44 ppm) were also detected (Figure 4B). Moreover, another multiplet (2.51; 2.54 and 2.65/2.67 ppm) was identified as citric acid/citrate. Finally, a peak probably representing fumaric acid (6.51 ppm) stood out. Both citric and fumaric acid had not been detected previously (Boersma et al., 2010). Several other small peaks could not be attributed to any known compound or metabolite. Thus, Lyophyllum sp. strain Karsten releases a suite of compounds/metabolites, including glycerol, acetate, formate, citrate, and fumarate, into its milieu that might be perceived and used by the fungal-interactive bacteria P. terrae BS001, BS110, BS007, and BS437 as potential carbon and energy sources.
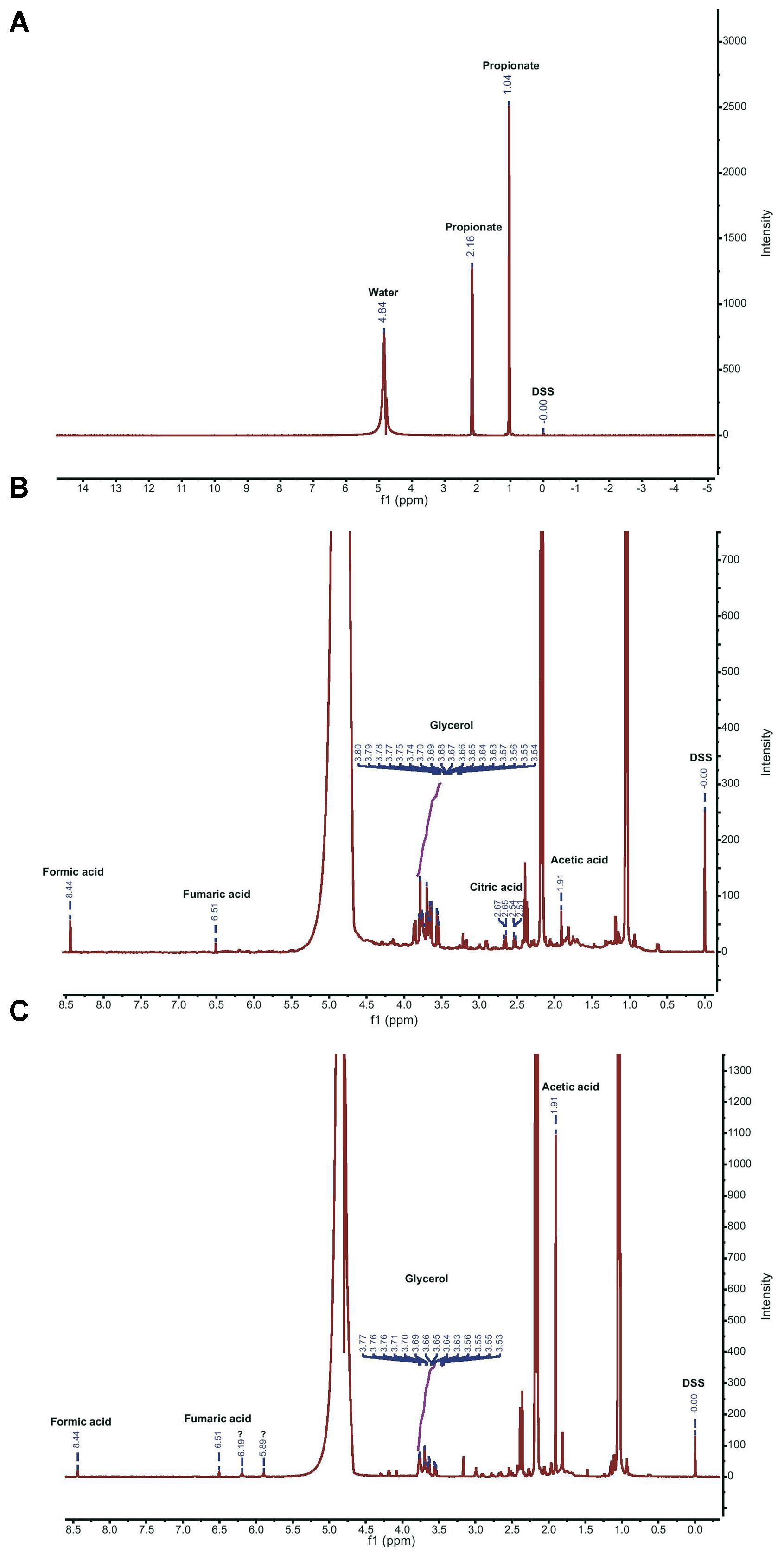
FIGURE 4. Proton NMR spectra of M9 medium, and fungal exudates. (A) M9 spectrum showing peaks corresponding to water and propionate. (B) Full spectrum of exudates of Lyophyllum sp. strain Karsten. (C) Full spectrum of exudates of T. asperellum 302. 3-(trimethylsilyl)-1-propanesulfonic acid sodium salt (DSS) was used as the internal standard (0.0 ppm). Corresponding peaks of compounds in exudates of Lyophyllum sp. strain Karsten (B) and T. asperellum 302 (C) are annotated with their names. Unknown peaks are denoted by (?).
1H NMR-Based Analysis of the Exudates Released by Trichoderma asperellum 302 Into Propionate-Amended M9 Medium
We then analyzed the exudates of T. asperellum 302, using 1H NMR, in order to find any commonalities and/or differences with the exudates of Lyophyllum sp. strain Karsten. First, glycerol was also found to be released by T. asperellum 302, as evidenced by the finding of a multiplet in the range reported in the foregoing (Figure 4C). Then, we also found peaks representative of acetic acid (1.91 ppm) and formic acid (8.44 ppm). Interestingly, fumaric acid/fumarate (6.51 ppm) was, presumably, also detected. Next to these broad commonalities in the metabolites released by both fungi, there were two unknown and as-yet-unidentified peaks (at 5.89 and 6.19 ppm), which were absent from the spectra obtained with Lyophyllum sp. strain Karsten. On the other hand, we did not find the multiplet representing citric acid/citrate. Thus, the ecological opportunities offered by T. asperellum 302 to its associated P. terrae strains were, to some extent, similar to those of Lyophyllum sp. strain Karsten, with some differences as suggested by the presence of unidentified peaks.
Growth of Paraburkholderia terrae BS001 on Oxalate-Supplemented M9 Medium
We then tested whether or not oxalic acid could serve as the sole carbon and energy source for the growth of P. terrae BS001 in M9 medium. Unsupplemented M9 medium was used as the negative and M9 medium supplemented with (0.5%) glucose as the positive control. The negative control did not support growth of strain BS001, as the CFU counts – from the initial 5.30 log 10 CFU/mL – increased only slightly over the 66 h incubation time, indicating residual growth (data not shown). P. terrae BS001 grown in M9+0.5% glucose showed a significantly increased (P < 0.05) final population density of 7.89 log 10 CFU/mL after 66 h of incubation. On 0.5% oxalate, we observed a progressive increase in the initial cell density, from about 5.30 log 10 to 6.20 log 10 CFU/mL (after 18 h) and then to 7.68 log 10 CFU/mL (42 h), after which it slightly dropped back to 6.86 log 10 CFU/mL at 66 h of incubation (data not shown). On M9 medium supplemented with 0.1% oxalate (w/v), the CFU counts of strain BS001, from an initial 5.30 log 10 CFU/mL, also increased significantly (P < 0.05), reaching 7.33 log 10 CFU/mL after 66 h of incubation (Figure 5). The overall results suggest that P. terrae BS001 can use oxalic acid as a carbon and energy source when supplied as a single carbonaceous compound in M9 medium.
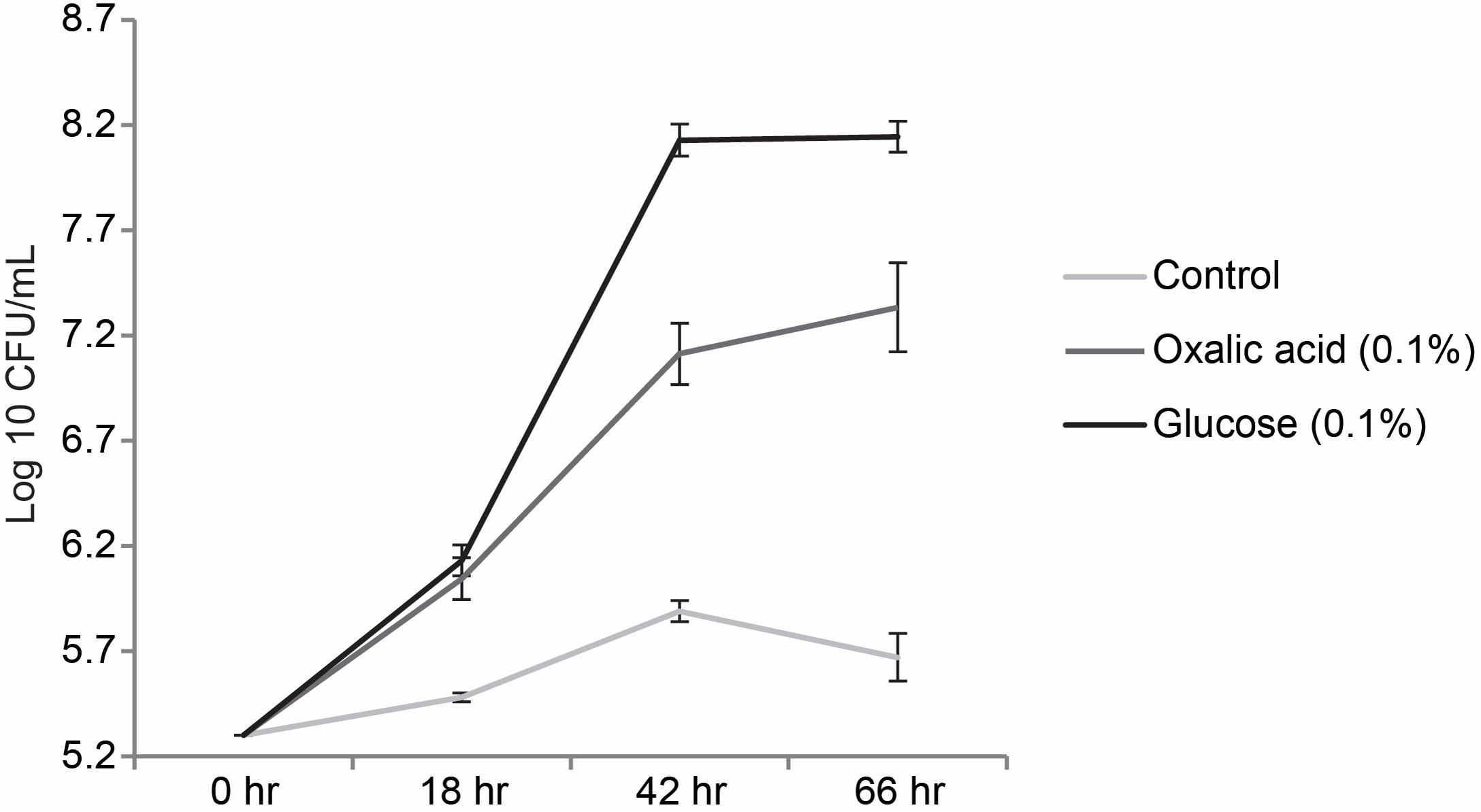
FIGURE 5. Growth curve of P. terrae BS001 grown on M9 medium supplemented with oxalate; represents the growth patterns of P. terrae BS001 on M9 medium supplemented with 0.1% of glucose, 0.1% oxalate [w/v] and no carbon source. The Y-axis represents CFU/mL on a log 10 scale, while the X-axis shows the times in hours. Data are representative of three biological replicates.
Genetic Potential for Oxalate Utilization in P. terrae Strains
We analyzed the genomes of P. terrae BS001, BS110, BS437, and BS007 to find genes that are potentially involved in the utilization and uptake of oxalic acid or its complexes (pathways I–V). Although we did not find any genes (as per the annotation) that are predicted to encode enzymes involved in oxalate degradation pathway I, we found genes encoding enzymes involved in pathways II and III in all P. terrae strains (Table 2). Moreover, we did find a cluster of five genes, which was recently reported to be upregulated in the transcriptome of strain BS001 during its interaction (physical contact stage) with Lyophyllum sp. strain Karsten (Haq et al., 2017), across all P. terrae strains (recently described by Pratama et al., 2017). The cluster contains a gene encoding a so-called “cupin” protein that was postulated to be acting as oxalate oxidase and/or oxalate carboxylase and may potentially be involved in the degradation of oxalate to formate and H2O2 as per the pathways IV and V.
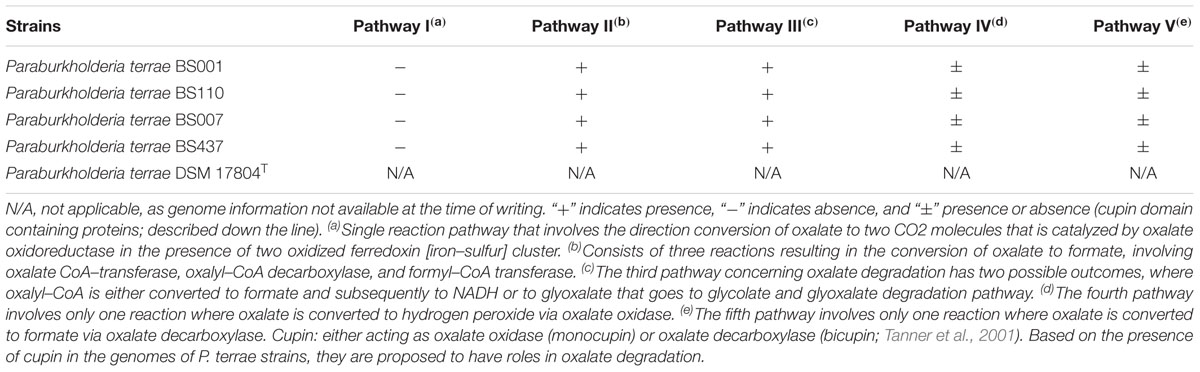
TABLE 2. The list of oxalate degrading pathways according to MetaCyc. The potential presence (+) or absence (-) of oxalate degradation pathways is shown in the respective Paraburkholderia terrae strains. Cupin domain containing proteins are postulated to be acting either as oxalate oxidase or oxalate decarboxylase.
Discussion
The ecological benefits offered by soil fungi to bacteria living in their vicinity have been addressed previously (De Boer et al., 2005; Warmink and van Elsas, 2009; Nazir et al., 2010; Pion et al., 2013; Stopnisek et al., 2016). A key model “system,” featuring P. terrae BS001 and a range of soil fungi (Nazir et al., 2014), including Lyophyllum sp. strain Karsten, has previously been interrogated with respect to the potential mechanisms involved in the bacterial–fungal interaction (Warmink and van Elsas, 2008, 2009; Nazir et al., 2013; Haq et al., 2014). However, questions on the fungal responsiveness of other P. terrae strains, such as BS110, BS007, and BS437, have remained unanswered. Moreover, any information regarding the type strain DSM 17804T has been lacking so far. Hence, there is a perceived lack of information with respect to the commonality of fungotropic chemotactic behavior within the species P. terrae. In this study, we thus examined the chemotactic behavior of a range (BS110, BS007, BS437, BS001, BS001-ΔsctD and DSM 17804T) of P. terrae strains toward exudates collected from the basidiomycete Lyophyllum sp. strain Karsten and the ascomycete T. asperellum 302, as well as oxalate. In recent findings, by Yang et al. (2016), the TTSS was found to have a helper effect in the migration of P. terrae toward fungal mycelia in soil microcosms, and therefore the inclusion of the TTSS-impaired mutant in the current study was deemed necessary to ascertain its potential role in chemotaxis. Concerning the signaling mechanism that might be employed, there are examples of a wide number of methyl-accepting chemotaxis proteins (MCPs) in bacteria such as VfcB and VfcB2 of Vibrio fischeri, that act as fatty acid chemoreceptors (Nikolakakis et al., 2016).
First, the analyses of the fungal exudates using proton 1H NMR and HPLC revealed the two fungi to release remarkably similar amounts of a few detectable small carbonaceous compounds, including the major compounds oxalate, glycerol, acetate, and formate, and presumably fumarate. The finding of oxalate was revealing and added this compound to the list of potential chemoattractants, and possibly carbon sources, for P. terrae (further discussed below). On the other hand, we found the differential presence of citrate (at Lyophyllum sp. strain Karsten) and an unidentified compound (at T. asperellum 302). Overall, one may conclude that a suite of compounds, next to glycerol, are released by both fungi, potentially serving as chemoattractants for P. terrae.
Remarkably, all P. terrae strains, including the type strain DSM 17804T, indeed were found to move, in a concerted fashion, toward the exudates of both fungi, with lower responses at the higher dilutions, and an overall difference between the two fungi. A slight decrease of migrational competence was found in the TTSS mutant strain, the exact cause of which remained unexplored. Further, the fact that the migrational behavior of the type strain was akin that of the other P. terrae strains indicated that – within the limitations of this study – P. terrae, in contrast to what was previously thought, might constitute a “tight” group of fungal-interactive organisms. This is opposed to the hypothesis that strain BS001 and its relatives are outliers within this species (compared to the type strain), as – in contrast to the type strain – they were isolated on the basis of their fungal interactivity (Warmink and van Elsas, 2008; Nazir et al., 2013, 2014).
Furthermore, given the fact that the two fungi indeed may release several of the aforementioned carbonaceous compounds into the soil, fungal-responsive Paraburkholderia species (broadly speaking, the species P. terrae) have likely adapted to migrate toward the fungal mycelia, thus reaping the ecological benefits of the utilization of the different compounds (e.g., glycerol, acetate, formate, fumarate, and oxalate; see below), for growth and survival.
Concerning the role of oxalic acid as a determinant of the behavior of the tested P. terrae strains, the data clearly indicated that all P. terrae strains responded to the oxalic acid/oxalate stemming from the agar stripes in the chemotaxis setup, even in the presence of glycerol in the M9 agar medium. Thus, even with a highly utilizable source of carbon and energy, the P. terrae cells migrated toward the source of oxalate. A question with respect to oxalate availability arises here, as (dissociated) oxalate was the most prominent chemical form under our experimental conditions (pH 6.8). The finding that the oxalate showed the highest attractant activity when at the lower “stripe” concentration (0.1%) was difficult to explain. However, the chemical interplay with (bivalent) cations present in the agar (affecting oxalate diffusion), in particular Ca2+ (0.1 mM), is complex and difficult to model. Remarkably, in a recent study with Collimonas fungivorans Ter331, an increasing concentration of oxalate in the medium was found to prompt a “lethargic” chemotactic response by bacteria, compared to a more active one at lower concentration (Rudnick et al., 2015). Notwithstanding its clear effect as a chemoattractant for all P. terrae strains when present as the sole carbonaceous compound, we did not address here to what extent the fungal-released oxalate was a major or minor chemoattractant in the presence of the other fungal-released compounds (such as glycerol). Clearly, this poses a complex question, which was not answered here.
The potential oxalotrophy of P. terrae was then investigated on the basis of growth experiments with P. terrae BS001. Indeed, we found that P. terrae BS001 was able to grow on M9 medium supplemented with defined concentrations of oxalic acid. This stands in contrast to previous findings (using Biolog assays) where P. terrae strains were reportedly unable to utilize oxalate (Nazir et al., 2012). However, Kost et al. (2014) reported that a range of plant-beneficial Burkholderia species (now split into two genera, Burkholderia and Paraburkholderia), including close relatives of P. terrae, namely P. phytofirmans, P. xenovorans, P. hospita, and P. caribensis, were able to grow on minimal medium containing oxalic acid as the sole carbon source. In contrast, the plant-pathogenic Burkholderia glumae, B. gladioli, and B. plantarii and human-pathogenic strains of the B. cepacia complex were unable to use oxalate as the carbon source. The here collected data (Table 2) suggest that P. terrae should be added to the list of oxalotrophic organisms. What are the ecological consequences of this finding? In the light of the rather poor energetic value of oxalate (Schneider et al., 2012), it may serve as a last-resort carbon source in the presence of other such sources. However, oxalotrophy should not be excluded as a true option for energy generation under conditions of starvation. In previous work, fungal-released oxalate in soil has been hypothesized to act as a virulence factor (thus promoting cellular activity) and as a helper in lignin degradation (Dutton and Evans, 1996; Heller and Witt-Geiges, 2013). Also, plant-released oxalate may act in calcium homeostasis, in repellance of herbivores or in toxic metal (e.g., aluminum) chelation (Franceschi and Nakata, 2005; Klug and Horst, 2010). Here, we posit that, apart from such anthropogenically inspired function attributions, fungal-released oxalate acts as a key “last-resort” carbon and energy source, selecting bacterial species such as P. terrae in the mycosphere upon the arisal of strongly carbon-limited conditions.
Supportive of the tenet of active oxalate oxidation was the fact that, indeed, in all P. terrae strains, systems for the catabolism (and uptake) of oxalate were found. The presence of genes encoding key enzymes of degradation pathways II and III, and that of the “cupin” (oxalate oxidase/carboxylase) thus suggested all strains have the genetic information enabling them to utilize oxalate. Moreover, we recently observed that the gene encoding the cupin protein was upregulated by P. terrae under the influence of growing mycelia of Lyophyllum sp. strain Karsten under soil-mimicking conditions (Haq et al., 2017). However, with respect to the latter phenomenon, further experiments would be needed to have deeper insight into the specific role of the cupin protein.
On the basis of the data presented herein, we further hypothesize that the oxalate/oxalic acid exuded by fungi in soil may be used by locally occurring P. terrae cells as a signaling molecule which enables to locate a colonizable surface, next to a plethora of nutrients. Concerning the latter, Boersma et al. (2010) previously quantified the glycerol levels in the exudates of Lyophyllum sp. strain Karsten in propionate-amended M9 medium, and found levels of approximately 2 mM. In that study, glycerol was presumed to be the major bacterium-feeding substrate coming from the fungus. Here, the estimated oxalic acid levels were approximately 7.5–7.9 mM, or three- to fourfold more. However, the fact that glycerol holds more energy than oxalate, and can easily diffuse (passively) into bacterial cells, suggests that it serves as a more accessible, and hence preferable, carbon and energy source than oxalate. Similarly, all other compounds released by the two fungi may also serve as “better” nutrients. In this theory, one may envision diauxic trophic behavior of P. terrae strains like BS001 in the mycosphere, with oxalate indeed acting as a last-resort nutrient. Furthermore, in the behavioral response to the two fungi, a chemoattractant/signaling function of oxalic acid cannot also be excluded.
Conclusion
Our results confirm that the nutritional conditions offered by two diverse soil fungi had key commonalities. In the light of the finding of oxalate, glycerol, and three organic acids (next to one or more unknown compounds) in the fungal secretomes, these conditions are of a complex nature. Yet, they offer the baseline against which interactions of the two fungi with P. terrae strains take place. We argue that our findings are indicative of a highly complex interplay, which, to expand it to natural soil environment, needs further investigation. Possibly, several diauxic shifts, as incited by presumably different and varying levels of the fungal-released compounds, occur over time in the fungal-interactive strains, and so it will be a challenge for future studies to precisely determine the sequence of events that take place in the chemotactic behavior of P. terrae at the two fungi.
Author Contributions
IH conceived the idea, performed the experiments, analyzed the data, and wrote the manuscript. RZ performed the HPLC and subsequent analyses. PY provided the mutant strain. JE conceived the idea and wrote the manuscript.
Funding
IH was financially supported by the TRAINBIODIVERSE project [European Marie Sklodowska-Curie Initial Training Networks (ITN) program, project number: 289949] as well as the University of Groningen. The China Scholarship Council (CSC) financially supported PY.
Conflict of Interest Statement
The authors declare that the research was conducted in the absence of any commercial or financial relationships that could be construed as a potential conflict of interest.
Acknowledgments
We acknowledge Pieter van der Meulen from Stratingh Institute for Chemistry, University of Groningen, for his help and assistance with 1H NMR data acquisition and preliminary analyses.
Supplementary Material
The Supplementary Material for this article can be found online at: https://www.frontiersin.org/articles/10.3389/fmicb.2018.00989/full#supplementary-material
FIGURE S1 | A representative figure of the chemotactic response of P. terrae toward oxalate.
FIGURE S2 | High-performance liquid chromatography (HPLC) chromatograms representing oxalic acid peaks of: oxalic acid [0.1 and 0.25% (w/v)] as a positive control, M9 medium as a negative control, and M9 medium spiked with oxalic acid [0.1% (w/v)], Lyophyllum sp. strain Karsten exudates and Lyophyllum sp. strain Karsten exudates spiked with oxalic acid (0.1% [w/v]; “sample A” means Lyophyllum sp. strain Karsten exudates sample “A,” Trichoderma asperellum 302 exudates and T. asperellum 302 exudates spiked with oxalic acid [0.1% (w/v)] “Tri B” means T. asperellum 302 exudates “B.” At the end, the calibration curve is provided.
TABLE S1 | Full factorial ANOVA testing for the effects of bacterial strains, oxalic acid concentration, movement direction, and their interaction on the distance traveled in the chemotaxis experiment on M9-G medium. Statistical significance codes: 0 “∗∗∗” 0.001 “∗∗” 0.01 “∗” 0.05 “.” 0.1 “ ” 1.
TABLE S2 | Full factorial ANOVA testing for the effects of bacterial strains, fungal strains, exudates level (conc.), movement direction, and their interaction on the distance traveled in the chemotaxis experiment on M9-G medium. Statistical significance codes: 0 “∗∗∗” 0.001 “∗∗” 0.01 “∗” 0.05 “.” 0.1 “ ” 1.
References
Adeleke, R. A., Cloete, T. E., Bertrand, A., and Khasa, D. P. (2012). Iron ore weathering potentials of ectomycorrhizal plants. Mycorrhiza 22, 535–544. doi: 10.1007/s00572-012-0431-5
Bhatti, J. S., Comerford, N. B., and Johnston, C. T. (1998). Influence of soil organic matter removal and pH on oxalate sorption onto a spodic horizon. Soil Sci. Soc. Am. J. 62, 152–158. doi: 10.2136/sssaj1998.03615995006200010020x
Boersma, F. G. H., Otten, R., Warmink, J. A., Nazir, R., and van Elsas, J. D. (2010). Selection of Variovorax paradoxus?like bacteria in the mycosphere and the role of fungal-released compounds. Soil Biol. Biochem. 42, 2137–2145. doi: 10.1016/j.soilbio.2010.08.009
Bravo, D., Cailleau, G., Bindschedler, S., Simon, A., Job, D., Verrecchia, E., et al. (2013). Isolation of oxalotrophic bacteria able to disperse on fungal mycelium. FEMS Microbiol. Lett. 348, 157–166. doi: 10.1111/1574-6968.12287
De Boer, W., Folman, L. B., Summerbell, R. C., and Boddy, L. (2005). Living in a fungal world: impact of fungi on soil bacterial niche development. FEMS Microbiol. Rev. 29, 795–811. doi: 10.1016/j.femsre.2004.11.005
Dutton, M. V., and Evans, C. S. (1996). Oxalate production by fungi: its role in pathogenicity and ecology in the soil environment. Can. J. Microbiol. 42, 881–895. doi: 10.1139/m96-114
Franceschi, V. R., and Nakata, P. A. (2005). Calcium oxalate in plants: formation and function. Annu. Rev. Plant Biol. 56, 41–71. doi: 10.1146/annurev.arplant.56.032604.144106
Haq, I. U., Dini-Andreote, F., and van Elsas, J. D. (2017). Transcriptional responses of the bacterium Burkholderia terrae BS001 to the fungal host Lyophyllum sp. strain Karsten under soil-mimicking conditions. Microb. Ecol. 6, 1652–1668. doi: 10.1007/s00248-016-0885-7
Haq, I. U., Graupner, K., Nazir, R., and van Elsas, J. D. (2014). The genome of the fungal interactive soil bacterium Burkholderia terrae BS001—A plethora of outstanding interactive capabilities unveiled. Genome Biol. Evol. 6, 1652–1668. doi: 10.1093/gbe/evu126
Harrold, S. A., and Tabatabai, M. A. (2006). Release of inorganic phosphorus from soils by low-molecular-weight organic acids. Commun. Soil Sci. Plant Anal. 37, 1233–1245. doi: 10.1080/00103620600623558
Heller, A., and Witt-Geiges, T. (2013). Oxalic acid has an additional, detoxifying function in Sclerotinia sclerotiorum pathogenesis. PLoS One 8:e72292. doi: 10.1371/journal.pone.0072292
Klug, B., and Horst, W. J. (2010). Oxalate exudation into the root-tip water free space confers protection from aluminum toxicity and allows aluminum accumulation in the symplast in buckwheat (Fagopyrum esculentum). New Phytol. 187, 380–391. doi: 10.1111/j.1469-8137.2010.03288.x
Kost, T., Stopnisek, N., Agnoli, K., Eberl, L., and Weisskopf, L. (2014). Oxalotrophy, a widespread trait of plant-associated Burkholderia species, is involved in successful root colonization of lupin and maize by Burkholderia phytofirmans. Front. Microbiol. 4:421. doi: 10.3389/fmicb.2013.00421
Nazir, R., Tazetdinova, D. I., and van Elsas, J. D. (2014). Burkholderia terrae BS001 migrates proficiently with diverse fungal hosts through soil and provides protection from antifungal agents. Front. Microbiol. 5:598. doi: 10.3389/fmicb.2014.00598
Nazir, R., Warmink, J. A., Boersma, H., and van Elsas, J. D. (2010). Mechanisms that promote bacterial fitness in fungal affected soil microhabitats. FEMS Microbiol. Ecol. 71, 169–185. doi: 10.1111/j.1574-6941.2009.00807.x
Nazir, R., Warmink, J. A., Voordes, D. C., van de Bovenkamp, H. H., and van Elsas, J. D. (2013). Inhibition of mushroom formation and induction of glycerol release-ecological strategies of Burkholderia terrae BS001 to create a hospitable niche at the fungus Lyophyllum sp. strain Karsten. Microb. Ecol. 65, 245–254. doi: 10.1007/s00248-012-0100-4
Nazir, R., Zhang, M. Z., de Boer, W., and van Elsas, J. D. (2012). The capacity to comigrate with Lyophyllum sp. strain Karsten through different soils is spread among several phylogenetic groups within the genus Burkholderia. Soil Biol. Biochem. 50, 221–233. doi: 10.1016/j.soilbio.2012.03.015
Nikolakakis, K., Monfils, K., Moriano-Gutierrez, S., Brennan, C. A., and Ruby, E. G. (2016). Characterization of the Vibrio fischeri fatty acid chemoreceptors, VfcB and VfcB2. Appl. Environ. Microbiol. 82, 696–704. doi: 10.1128/AEM.02856-15
Pion, M., Spangenberg, J. E., Simon, A., Bindschedler, S., Flury, C., Chatelain, A., et al. (2013). Bacterial farming by the fungus Marcello crassipes. Proc. Biol. Sci. R. Soc. 280:20132242. doi: 10.1098/rspb.2013.2242
Pratama, A. A., Haq, I. U., Nazir, R., De Mares, M. C., and van Elsas, J. D. (2017). Draft genome sequences of three fungal-interactive Paraburkholderia terrae strains, BS007, BS110 and BS437. Stand. Genomic Sci. 12:81. doi: 10.1186/s40793-017-0293-8
Rudnick, M. B., van Veen, J. A., and de Boer, W. (2015). Oxalic acid: a signal molecule for fungus-feeding bacteria of the genus Collimonas? Environ. Microbiol. Rep. 7, 709–714. doi: 10.1111/1758-2229.12290
Schneider, K., Skovran, E., and Vorholt, J. A. (2012). Oxalyl-coenzyme A reduction to glyoxylate is the preferred route of oxalate assimilation in Methylobacterium extorquens AM1. J. Bacteriol. 194, 3144–3155. doi: 10.1128/JB.00288-12
Stopnisek, N., Zuhlke, D., Carlier, A., Barberan, A., Fierer, N., Becher, D., et al. (2016). Molecular mechanisms underlying the close association between soil Burkholderia and fungi. ISME J. 10, 253–264. doi: 10.1038/ismej.2015.73
Sullivan, T. S., Gottel, N. R., Basta, N., Jardine, P. M., and Schadt, C. W. (2012). Firing range soils yield a diverse array of fungal isolates capable of organic acid production and pb mineral solubilization. Appl. Environ. Microbiol. 78, 6078–6086. doi: 10.1128/AEM.01091-12
Tanner, A., Bowater, L., Fairhurst, S. A., and Bornemann, S. (2001). Oxalate decarboxylase requires manganese and dioxygen for activity: overexpression and characterisation of Bacillus subtilis YvrK and YoaN. J. Biol. Chem. 276, 43627–43634. doi: 10.1074/jbc.M107202200
Vallenet, D., Belda, E., Calteau, A., Cruveiller, S., Engelen, S., Lajus, A., et al. (2013). MicroScope an integrated microbial resource for the curation and comparative analysis of genomic and metabolic data. Nucleic Acids Res. 41, D636–D647. doi: 10.1093/nar/gks1194
Van Elsas, J. D., Jansson, J. K., and Trevors, J. T. (2007). Modern Soil Microbiology, 2nd Edn. New York, NY: CRC Press.
Van Hees, P. A. W., Rosling, A., Essen, S., Godbold, D. L., Jones, D. L., and Finlay, R. D. (2006). Oxalate and ferricrocin exudation by the extramatrical mycelium of an ectomycorrhizal fungus in symbiosis with Pinus sylvestris. New Phytol. 169, 367–377. doi: 10.1111/j.1469-8137.2005.01600.x
Warmink, J. A., and van Elsas, J. D. (2008). Selection of bacterial populations in the mycosphere of Laccaria proxima: is type III secretion involved? ISME J. 2, 887–900. doi: 10.1038/ismej.2008.41
Warmink, J. A., and van Elsas, J. D. (2009). Migratory response of soil bacteria to Lyophyllum sp. strain Karsten in soil microcosms. Appl. Environ. Microbiol. 75, 2820–2830. doi: 10.1128/AEM.02110-08
Yang, P., Zhang, M., and van Elsas, J. D. (2017). Role of flagella and type four pili in the co-migration of Burkholderia terrae BS001 with fungal hyphae through soil. Sci. Rep. 7:2997. doi: 10.1038/s41598-017-02959-8
Keywords: oxalic acid, chemotaxis, fungal exudates, Paraburkholderia terrae, signaling
Citation: Haq IU, Zwahlen RD, Yang P and van Elsas JD (2018) The Response of Paraburkholderia terrae Strains to Two Soil Fungi and the Potential Role of Oxalate. Front. Microbiol. 9:989. doi: 10.3389/fmicb.2018.00989
Received: 03 February 2018; Accepted: 27 April 2018;
Published: 29 May 2018.
Edited by:
Mohamed Hijri, Université de Montréal, CanadaReviewed by:
Ashu Sharma, University at Buffalo, The State University of New York, United StatesCristina Silva Pereira, Instituto de Tecnologia Química e Biológica (ITQB-NOVA), Portugal
Copyright © 2018 Haq, Zwahlen, Yang and van Elsas. This is an open-access article distributed under the terms of the Creative Commons Attribution License (CC BY). The use, distribution or reproduction in other forums is permitted, provided the original author(s) and the copyright owner are credited and that the original publication in this journal is cited, in accordance with accepted academic practice. No use, distribution or reproduction is permitted which does not comply with these terms.
*Correspondence: Irshad Ul Haq, aS51LmhhcUBydWcubmw=; aXJzaGFkdWxoYXE4NUBnbWFpbC5jb20=; Jan Dirk van Elsas, ai5kLnZhbi5lbHNhc0BydWcubmw=