- 1Department of Bioscience and Biotechnology, Konkuk University, Seoul, South Korea
- 2NUS Synthetic Biology for Clinical and Technological Innovation (SynCTI), Life Sciences Institute, National University of Singapore, Singapore, Singapore
- 3Bioprocessing Technology Institute, Agency for Science, Technology and Research (A*STAR), Singapore, Singapore
- 4School of Chemical Engineering, Sungkyunkwan University, Suwon, South Korea
Aspergillus oryzae has been commonly used to make koji, meju, and soy sauce in traditional food fermentation industries. However, the metabolic behaviors of A. oryzae during fermentation in various culture environments are largely uncharacterized. Thus, we performed time resolved (0, 4, 8, 12, 16 day) secondary metabolite profiling for A. oryzae KCCM 12698 cultivated on malt extract agar and broth (MEA and MEB) under solid-state fermentation (SSF) and submerged fermentation (SmF) conditions using the ultrahigh performance liquid chromatography-linear trap quadrupole-ion trap-mass spectrometry (UHPLC-LTQ-IT-MS/MS) followed by multivariate analyses. We observed the relatively higher proportions of coumarins and oxylipins in SSF, whereas the terpenoids were abundant in SmF. Moreover, we investigated the antimicrobial efficacy of metabolites that were extracted from SSF and SmF. The SSF extracts showed higher antimicrobial activities as compared to SmF, with higher production rates of bioactive secondary metabolites viz., ketone-citreoisocoumarin, pentahydroxy-anthraquinone, hexylitaconic acid, oxylipins, and saturated fatty acids. The current study provides the underpinnings of a metabolomic framework regarding the growth and bioactive compound production for A. oryzae under the primarily employed industrial cultivation states. Furthermore, the study holds the potentials for rapid screening and MS-characterization of metabolites helpful in determining the consumer safety implications of fermented foods involving Koji mold.
Introduction
Aspergillus species are a ubiquitous mold, which widely affects human life in both deleterious and beneficial ways, since some species are pathogens and the others are used to make fermented foods (Kang et al., 2013). Traditionally, A. oryzae (koji mold) has been used to produce a variety of fermented foods and beverages, such as deonjang, sake, makgeoli, and miso (Lee et al., 2017). The fermented foods are made using spontaneously occurring or inoculated microbial species with selective microflora, including mold (Aspergillus), yeast, and bacterial species to colonize and modulate the food substrates (Chilton et al., 2015). Recently, metabolomics studies of fermented food under different culture conditions have highlighted distinct profiles of metabolites owing to differences in microbial consortia and metabolism (Oh et al., 2016; Lee et al., 2017). Furthermore, the dynamics of microbial communities vary spatiotemporally in a variety of fermentative systems, which have been investigated to determine the quality of end-products (Chen et al., 2014; Yang et al., 2016b). Specifically, these studies have suggested that A. oryzae is among the dominant mold varieties in both the natural as well as the in situ cultivated microenvironments i.e., fermented foods.
Previous studies have established that the physicochemical parameters (pH, aeration, and incubation temperature) and nutrients (carbon and nitrogen sources) categorically affect mold growth and metabolism (Pansuriya and Singhal, 2010). Typically, the microorganisms detect environmental changes and adapt themselves to survive in altered conditions through maneuvering their metabolism, especially the secondary metabolite production (Arumugam et al., 2014). The secondary metabolites syntheses facilitate microbial growth and survival through acting as pathogenicity determinants or extracellular signaling molecules among population of competing microbial communities (Arumugam et al., 2014). In particular, fungal secondary metabolites are imperative compounds for antimicrobial substances, antibiotics, alkaloids, toxins, and pigments syntheses with promising applications in food and pharmaceutical industries (Arumugam et al., 2014). To increase the production of secondary metabolites, many studies have considered optimizing the culture conditions, such as solid-state fermentation (SSF) and submerged fermentation (SmF) (Miranda et al., 2014; Hansen et al., 2015). Previously, we have highlighted the subtle effects of SSF and SmF on the distinct metabolomes and transcriptomes in Penicillium expansum (Kim et al., 2016).
Metabolomics involve unbiased analysis of the overall complements of metabolites in a biological sample under a given set of conditions, serving as a technique to provide the important information of cell, tissue, or whole organism physiologies (Singh et al., 2017). The mass spectrometry (MS)-based metabolomic profiles coupled with multivariate statistical analysis help in determining the effects of different culture conditions as a function of metabolomic and transcriptomic disparity in molds (Kim et al., 2016). Preparative HPLC (prep-HPLC) is the useful tool for the isolation and purification of metabolites such as flavonoid, sterols, and ester, and offers various advantages viz., high method reproducibility and robustness as well as efficient compound recovery useful for screening bioactive fractions (Lee et al., 2013; Dang et al., 2016). A holistic assessment of the metabolomes influenced by two different fermentation states i.e., SSF and SmF, of A. oryzae offers insights into their global and non-targeted metabolic changes. Furthermore, the approach may potentially delineate the chemotaxonomic characterization of koji mold under the two important culture conditions.
In this study, we report the effects of different culture conditions i.e., SSF and SmF, on untargeted secondary metabolite profiles of A. oryzae KCCM 12698. The metabolite profiles were evaluated and tentatively identified using the ultrahigh performance liquid chromatography-linear trap quadrupole-ion trap-mass spectrometry (UHPLC-LTQ-IT-MS/MS) datasets. The study aims to delineate the metabolomic disparity between the two primary cultivation states i.e., SSF and SmF, for A. oryzae. Herein, we highlighted the metabolic disparity between the two primary cultivation states of Aspergillus oryzae (trivially: Koji mold) employed in various fermented food (deonjang, sake, makgeoli, and miso) as well as industrial production of value added products (pharmacologically active, cosmetic, or food additive compounds). Hence, the comprehensive profiling of the secondary metabolites might illuminate the range of secreted metabolic classes helpful in delineating the optimal fermentative conditions and associated implication of secreted end products, either beneficial or detrimental.
Materials and Methods
An illustrative outline of experimental procedure is presented in Supplementary Figure 1.
Chemicals and Reagents
Acetonitrile, ethyl acetate, methanol, and water were purchased from Fisher Scientific (Pittsburgh, PA, USA). Malt extract, yeast extract, tryptic soy agar (TSA), nutrition agar (NA), and peptone were purchased from Becton Dickson (Franklin Lakes, NJ, USA). Agar powder and glucose were purchased from Junsei Chemical (Tokyo, Japan). Metabolite standard 12, 13-DiHOME was purchased from Cayman Chemical (Ann Arbor, MI). Antibiotics ampicillin, amphotericin B were purchased from Sigma-Aldrich (St. Louis, MO, USA).
Strains and Media
A. oryzae KCCM 12698 were obtained from the Korean Culture Center of Microorganisms (KCCM, Republic of Korea). A. oryzae was maintained on malt extract agar (MEA; 20 g malt extract, glucose: 20 g, 1 g peptone and 20 g agar per liter) at 28°C.
For the antimicrobial activity, Candida albicans KACC 30062, Escherichia coli KCTC 1682, Staphylococcus aureus KACC 1621 were used. These strains were obtained from the Korean Agricultural Culture Collection (KACC, Korea) and the Korean Collection for Type Cultures (KCTC, Korea). Tested microbials were maintained on different media. C. albicans was cultured on yeast malt agar (YMA; 3 g yeast extract, 3 g malt extract, 5 g peptone, 10 g glucose, and 20 g agar per liter) at 28°C. E. coli and S. aureus were cultured on nutrition agar (NA; 23g nutrition agar per liter) at 37°C.
Preparation of Spore Suspension
A. oryzae was transferred from frozen stock (−80°C) to MEA plates for pre-culture incubation, prior to the harvesting of viable spores. A 12-day old culture of the strain was treated with 0.01% Tween-20. Spores were collected by washing the surface of the strain and counted using Neubauer chamber. The final spore inoculum size was adjusted to approximately 3.0 × 106 spores/mL.
Culture Condition
For SSF, 100 mL of MEA plates were inoculated with 1 mL of spore suspension (3.0 × 106 spores/mL) into 150 × 25 mm petri dishes and maintained at 28°C. For SmF, 100 mL of malt extract broth (MEB) medium in 250 mL Erlenmeyer flasks was inoculated with 1 mL of spore suspension (3.0 × 106 spores/mL) and incubated at 28°C and 200 rpm. The A. oryzae culture in the two different fermentation states (SSF and SmF) were harvested at regular intervals 0 day to 16 days at every 4 days interval, and the samples immediately stored at deep freezing conditions (−80°C) until analyses (Supplementary Figure 2). Three biological replicates were collected each for SSF and SmF conditions representing each time interval.
Extraction of Fungal Metabolites
Extraction of Fungal metabolites was followed using the method partially adopted from Kim et al. (2016). The metabolites from SSF cultured A. oryzae were extracted by adding 100 mL of ethyl acetate to the chopped agar plugs followed by agitation under a shaking incubator at 200 rpm for 24 h. On the other hand, the metabolites from SmF culture were extracted using solvent-partitioning method with ethyl acetate (1:1, v/v) under similar conditions (200 rpm) for 24 h. The samples were centrifuged (5,000 × g) for 10 min at 4°C (Universal 320 R, Hettich, Zentrifugen, Germany). The partially purified solvent extracts were dried using a speed vacuum concentrator and re-suspended in MeOH at appropriate dilutions. The solvents extracts were filtered using 0.45 μm disposable polytetrafluoroethylene (PTFE) filter prior to LC-MS analysis. The final concentration of each sample was adjusted to 20 mg/mL.
UHPLC-LTQ-IT-MS/MS and UPLC-Q-TOF-MS Analysis
UHPLC-LTQ-IT-MS/MS analysis was performed using the method partially adapted from Oh et al. (2016) on the LTQ-XL ion trap mass spectrometer equipped with an electrospray interface (Thermo Fisher Scientific, San José, CA) coupled with DIONEX UltiMate 3000 RS Pump, RS Autosampler, RS Column Compartment and RS Diode Array Detector (Dionex Corporation, Sunnyvale, USA). The samples were separated on a Thermo Scientific Syncronis C18 UHPLC column with a 1.7 μm particle size. The mobile phase consisted of 0.1% formic acid in water (solvent A) and 0.1% formic acid in acetonitrile (solvent B), with the gradient flow program as follows: the initial solvent condition was 10% of solvent B; the gradient was then gradually increased from 10% solvent B to 100% solvent B over 18 min. Following this, solvent B was decreased to 10% and maintained as such for next 4 min, completing the total run time of 22 min. The flow rate was maintained at 0.3 mL/min with a 10 μL injection volume. The photodiode array detector was set at a wavelength range of 200–600 nm and was managed by the 3D Field. Mass spectra were recorded using electrospray ionization (ESI) at both positive and negative ion modes spanning a mass range of 150–1,000 m/z. The various operational parameters used were as follows: source voltage, ± 5 kV; capillary voltage, 39 V; and capillary temperature, 275°C. Tandem MS analysis was performed by scan-type turbo data-dependent scanning under the conditions used for negative mode MS scanning. The sample analysis was performed for 3 biological replicates. To decrease the effects of systematic error, the samples were analyzed in random blocks of ten runs followed by an intermitted quality control (QC) sample made with pooled blends from each sample extracts (Godzien et al., 2015).
UPLC-Q-TOF-MS analysis was followed by our previous study (Lee et al., 2017). Validations of selected metabolites were performed with a UPLC-Q-TOF-MS analysis. Waters Micromass QTOF Premier used an UPLC ACQUITY system (Waters, Milford, MA) equipped with a binary solvent delivery apparatus, an auto-sampler, and an ultraviolet (UV) detector. The column selected was an ACQUITY UPLC BEH C18 column (100 mm × 2.1 mm × 1.7 μm particle size, Waters Corp.). The operation parameters were set as follows: injection volume, 5 μL; flow rate, 0.3 mL/min; and column temperature, 37°C. The mobile phase consisted of 0.1% formic acid in water (A) and 0.1% formic acid in acetonitrile (B). The gradient program was set as follows: 5% solvent B was maintained initially for 1 min followed by a gradual increase to 100% over 9 min, and then maintained at 100% B for next 1 min, with a subsequent decrease to 5% over final 3 min, maintaining a total runtime of 14 min. The MS data were collected in the range of 100–1,000 m/z using Waters Q-TOF Premier system (Micromass MS Technologies, Manchester, U.K.) under negative- and positive-ion modes. The capillary voltage and cone voltage were set at 2.5 kV and 50 V, respectively. The source temperature was set at 100°C, with the desolvation gas (nitrogen) and cone gas (nitrogen) flow rates tuned to 600 and 50 L/h, respectively.
Data Processing and Statistical Analysis
The UHPLC-LTQ-IT-MS/MS data were obtained from Xcalibur software (version 2.00, Thermo Fisher Scientific), and raw data were subsequently converted to netCDF (*.cdf) format using Xcalibur software. The MS data files were then processed using MetAlign software (RIKILT-Institute of Food Safety, Wageningen, Netherlands) to estimate the retention times, normalized peak intensities, and accurate masses. The results of alignment data were exported to Excel files (Microsoft, Redmond, WA, USA), and multivariate statistical analyses were performed using SIMCA-P+ software (version 12.0, Umetrics, Umea, Sweden). Principal component analysis (PCA), partial least squares discrimination analysis (PLS-DA), and the loading plots were employed to compare distinctive metabolites between SSF and SmF. The variable importance in the projection (VIP) value and analysis of variance (ANOVA) methods were used for the tentative identification of significantly different metabolites detected from UHPLC-LTQ-IT-MS/MS (at VIP > 0.7, p < 0.05) analyses. Statistical analysis for the data involving t-test was performed on PASW Statistical software (version 18.0, SPSS, Inc., Chicago, IL, USA). Tentative metabolite identification procedure was followed by Lynn et al. (2015). The selected secondary metabolites were putatively identified based on various information such as mass spectra, MSn fragment, retention time, UV spectrum, elemental composition, mDa, and i-Fit data obtained from UHPLC-LTQ-IT-MS/MS and UPLC-Q-TOF-MS as well as comparing with standard compound, the published references, Dictionary of Natural Product (CCD, Copyright 2008, Taylor & Francis Group, Boca Raton, FL, USA) and Antibase 3.0 (CambridgeSoft Corporation, Cambridge, MA, USA).
Disk Diffusion Method for Evaluation the Antimicrobial Activity
The antimicrobial bioactivities for A. oryzae SSF and SmF extracts harvested at regular intervals (0, 4, 8, 12, 16 days) against two bacterial (E. coli and S. aureus) and one fungal (C. albicans) species were assayed using the disk diffusion method with few modification (Klančnik et al., 2010; Kang et al., 2013). 100 μL of each microbial suspension was seeded and spread uniformly on NA and YMA plates, respectively. Whatman sterile filter paper disks (6 mm in diameter) were loaded with 10 μL of A. oryzae extract dissolved in 100% methanol at appropriate concentration (10 mg/mL). The extract loaded paper disks were placed onto the agar surface with microbial cultures. Antibiotic (ampicillin and amphotericin B) loaded paper disk were used as positive control and 100% methanol which is the same solvent used to dissolve the Aspergillus extract served as a negative control. The assay plates were incubated for 1 day at 37°C. For each bioactivity assay, pooled three biological replicates were carried out in triplicate.
Preparative HPLC for Purifying Bioactive Compounds
Preparative high-performance liquid chromatography (HPLC) was followed as previously described by Lee et al. (2013) with minor modification. The sample separation was performed on a YMC-Pack Pro C18 reversed-phase column (250 × 4.6 mm × 5 μm particle size) with pump L-2130 and L-2455 diode array detector (Hitachi, Tokyo, Japan) using 5% acetonitrile in water (solvent A) and 100% acetonitrile (solvent B) following the gradient: 5% solvent B was maintained initially for 2 min followed by a gradual increase to 100% over 55 min, then equilibrated at 100% solvent B for 2 min, and sharply decreased to 5% over the final 1 min. The flow rate was 1 mL/min and the peaks were monitored at a wavelength of 220 nm. A total of 60 time-based fractions at every 1 min were collected and dried subsequently using speed vacuum concentrator. Before analyzing preparative HPLC, 4 mg of A. oryzae extracts was injected for HPLC profiling. The flow rate was 1 mL/min and the photodiode array was set at 220–600 nm. Followed by HPLC profiling, 100 mg of A. oryzae extract was injected for preparative HPLC. The flow rate was 1 mL/min and the peaks were monitored at a wavelength of 220 nm (Supplementary Figure 8A). Overall, sixty fractions were collected at the rate of 1 min−1, and dried subsequently by speed vacuum concentrator. The fractions were reconstituted in 100% methanol (1 mg/mL) and the corresponding antimicrobial activities were examined using the method described in previous section for three analytical replicates. The bioactive fractions were analyzed by UHPLC-LTQ-IT-MS/MS and UPLC-Q-TOF-MS methods.
Results
Non-targeted Metabolite Profiling of A. oryzae in SSF and SmF
The time correlated (0, 4, 8, 12, 16 days) metabolite profiling datasets such as LC-MS chromatogram from A. oryzae extracts exhibited different patterns for SSF and SmF cultivation (Supplementary Figure 3). Multivariate analyses including the unsupervised principle component analysis (PCA), as well as the supervised partial least squares discrimination analysis (PLS-DA) were performed to select the discriminant metabolites adding to the observed variance. The PLS-DA score plots based on UHPLC-LTQ-IT-MS/MS profiling data in negative ion mode showed distinct metabolomic patterns between SSF and SmF along PLS1 (23.68%) and PLS2 (9.02%) components (Figure 1B). The statistical variants for the PLS-DA plots were indicated using R2X (0.556) and R2Y (0.990), representing the total sum of squares. Whereas, the fraction of total variation for X and Y components was signified by Q2 (0.961). A similar pattern for metabolomics data was observed using the PCA score plot (Figure 1A). The significantly discriminant metabolites between SSF and SmF were selected using the variable importance in projection value (VIP > 0.7) and p-value (p < 0.05). The selected metabolites were putatively identified using their retention time, mass spectra, λmax, MSn fragment patterns, and elemental compositions based on the UHPLC-LTQ-IT-MS/MS and UPLC-Q-TOF-MS datasets, as well as the published reports and standard compound and presented all of ion extracted chromatogram, MS spectrum, and UV spectrum to clearly understand metabolite identification (Supplementary Figures 6, 7). A total of 26 significantly discriminant metabolites were putatively identified (VIP > 0.7, p < 0.05) and subsequently categorized in to the following secondary metabolite classes, including two coumarins viz., dihydroxy-methoxycoumarin (1), ketone-citreoisocoumarin (2); four terpenoids viz., asperaculin A (3), austalide F (4), austalide H (5), meroterpenoid derivatives (6); seven unsaturated fatty acids viz., 9,12,13-TriHODE (7), 9,12,13-TriHOME (8), 9,10,13-TriHOME (9), 8,11-DiHODE (10), 12,13-DiHODE (11), 12,13-DiHOME (12), 7,10-DiHOME (13); and four miscellaneous metabolites viz., diketopiperazine containing dihydroxy-methoxy-phenylalanine (14), pentahydroxy-anthraquinone (15), dihydroxydodecanoic acid (16), and hexylitaconic acid (18) (Table 1).
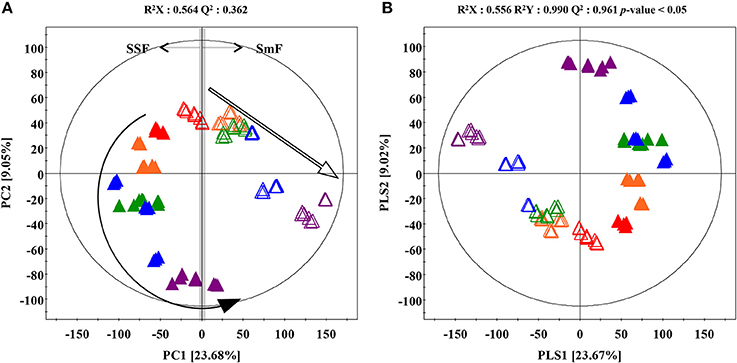
Figure 1. (A) Principal component analysis (PCA) and (B) partial least squares-discriminant analysis (PLS-DA) score plot derived from the UHPLC-LTQ-IT-MS/MS datasets for A. oryzae KCCM 12698 during SSF and SmF conditions. The arrow indicate the flow of times changes. (, Solid-state fermentation;
, Submerged fermentation;
, 0 day;
, 4 days;
, 8 days;
, 12 days;
, 16 days).
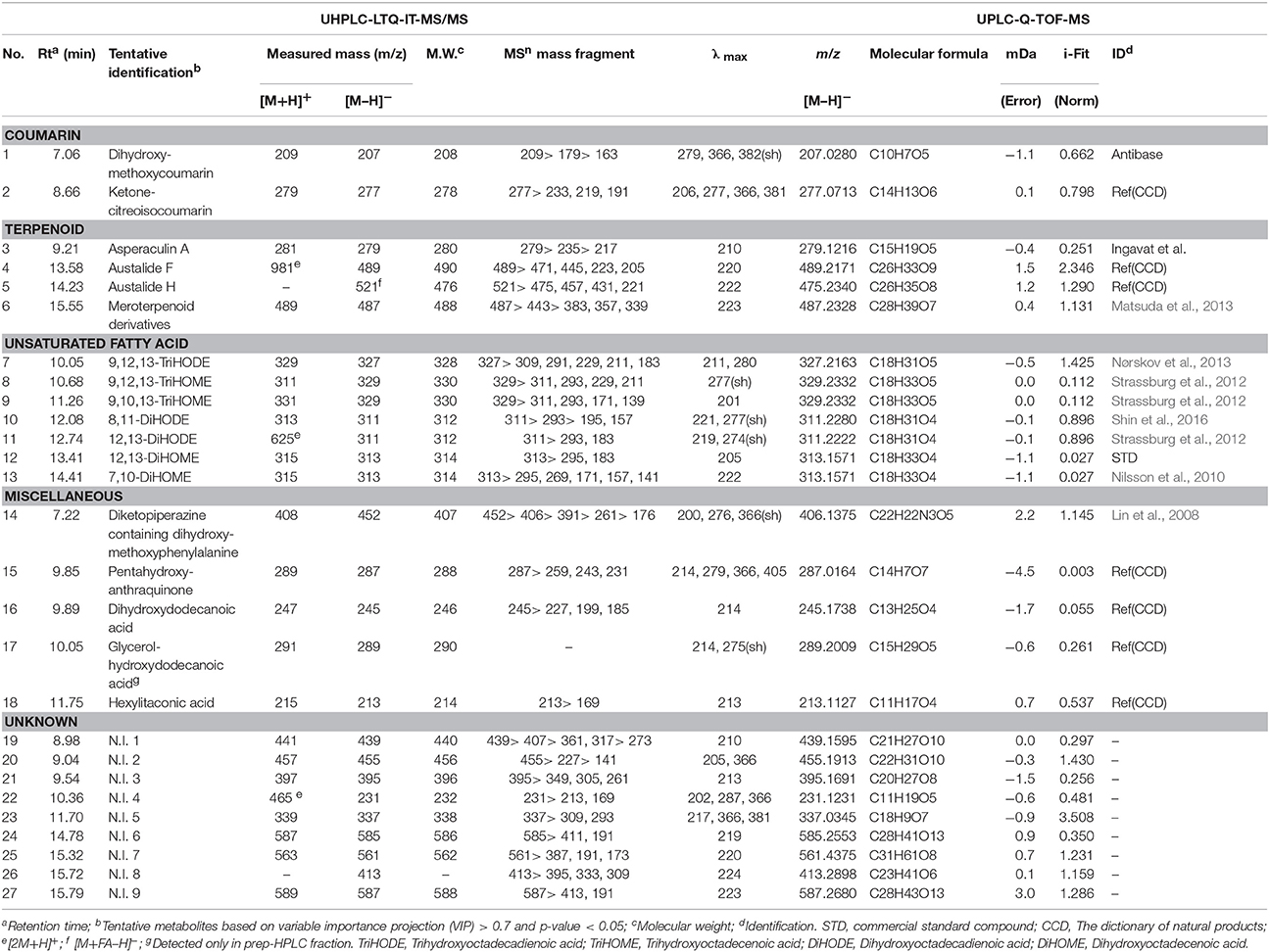
Table 1. Tentative identified metabolites from A. oryzae KCCM 12698 according to different fermentation conditions based on UHPLC-LTQ-IT-MS/MS and UPLC-Q-TOF-MS data.
Evaluation of the Relative Levels of Significantly Discriminant Metabolites Between SSF and SmF
The relative levels of 26 significantly discriminant secondary metabolites were expressed by the corresponding peak area from UHPLC-LTQ-IT-MS/MS chromatograms (Figure 2). Among 26 selected metabolites, 20 metabolites, including coumarins (1, 2), unsaturated fatty acids (7–13), diketopiperazine derivatives (14), pentahydroxy-anthraquinone (15), dihydroxydodecanoic acid (16), and hexylitaconic acid (18), were relatively more abundant in SSF extracts. The remaining six metabolites, including terpenoids (3–6) and two N.I metabolites (N.I. 1 and 9), were comparatively higher in SmF extracts. Further, the loading plots derived from PLS-DA datasets coupled with sub-group of chemical structure for discriminative metabolites and their relative contents showed that coumarins (1–2), unsaturated fatty acids (7–13), diketopiperazine derivatives (11), pentahydroxy-anthraquinone (15), dihydroxydodecanoic acid (16), and hexylitaconic acid (18) were observed mainly in SSF, whereas terpenoids (3–5) were primarily abundant in SmF (Figure 3).
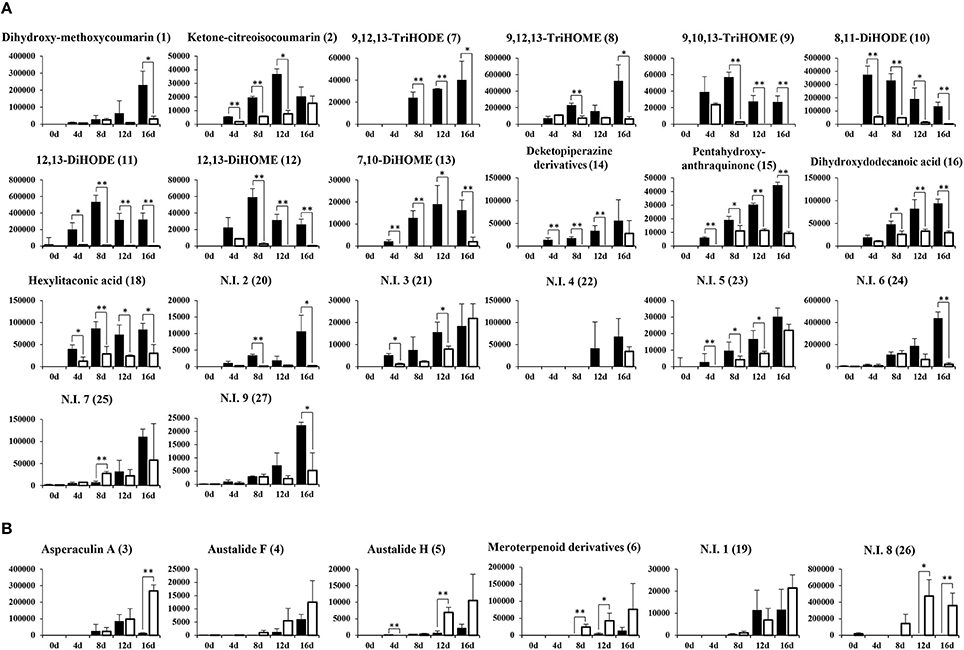
Figure 2. Production of secondary metabolites from solid-state (A) and submerged (B) fermentation of A. oryzae KCCM 12698. The Y-axis of the graphs indicates peak area of each metabolite normalized by volume (100 mL). Data are shown as means ± S.D. (n = 9). Significant differences between the SSF and SmF groups were identified by t-test (*p < 0.05, **p < 0.01). (, Solid-state fermentation;
, Submerged fermentation).
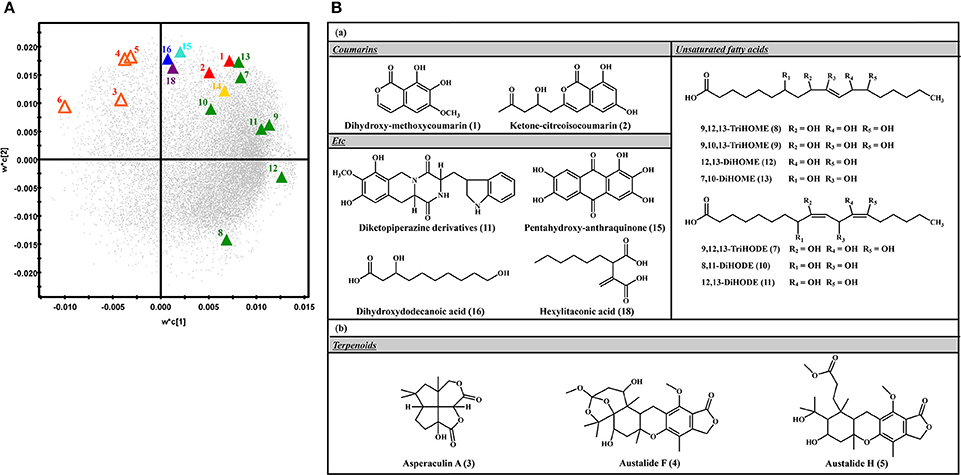
Figure 3. (A) PLS-DA loading plots of SSF and SmF samples analyzed using UHPLC-LTQ-IT-MS/MS. , Solid-state fermentation;
, Submerged fermentation;
, Coumarins;
, Terpenoids;
, Unsaturated fatty acids;
, Diketopiperazine;
, Anthraquinone;
, Saturated fatty acid;
, Organic acid. (B) The structure of compounds significantly produced in (a) solid-state fermentation and (b) submerged fermentation.
Evaluation and Partial Purification of Antimicrobial Metabolites
The potential antimicrobial activities of SSF and SmF extracts from A. oryzae were tested against C. albicans, S. aureus, and E. coli (Figure 4 and Supplementary Figure 4). Intriguingly, an increasing trend of antimicrobial activities was recorded for the metabolites extracted from both the fermentation states. However, the SSF extracts exhibited the relatively higher antimicrobial activity than those of SmF. Based on the time correlated antimicrobial activities, only 8-day incubated A. oryzae SSF extract was further subjected to prep-HPLC analysis and metabolite fractionation (Figure 5). In particular, the fractions collected between 2–3, 20–22, 24–28, 30–31, 34–35, 37–39, 42–43, 51–55 (min) showed notable growth inhibitory activities against S. aureus and E. coli, whereas fractions between 25–28 (min) selectively inhibited C. albicans growth (Figure 5A and Supplementary Figure 5). In particular, we observed that the 25–27 (min) fractions exhibited the highest growth inhibition effects against C. albicans. These fractions were further analyzed by UHPLC-LTQ-IT-MS/MS and UPLC-Q-TOF-MS to identify the antimicrobial metabolites. As a result, seven unsaturated fatty acids (7–13), two saturated fatty acids (16, 17), ketone-citreoisocoumarin (2), pentahydroxy-anthraquinone (15), and hexylitaconic acid (18) were variously identified using standard compounds, molecular weight, elemental composition, MSn fragmentation, and UV λmax (nm) data from the collected prep-HPLC fractions (Figures 5B,C and Supplementary Figure 8).
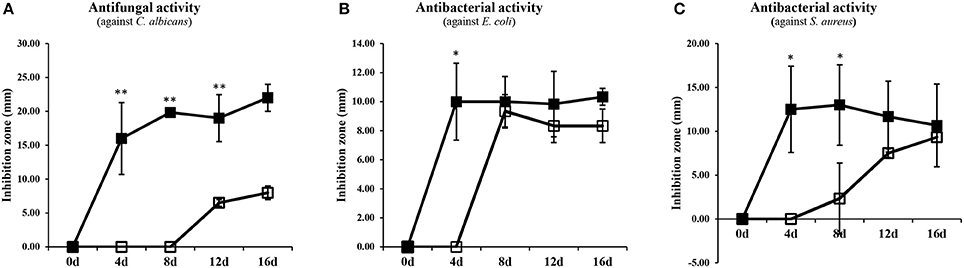
Figure 4. Antimicrobial activity from SSF and SmF extracts (1 mg/100 μL) against (A) Candida albicans, (B) Escherichia coli, and (C) Staphylococcus aureus. The size of disk was 6 mm. Data are shown as means ± S.D (n = 3). Significant differences between the SSF and SmF groups were identified by t-test (*p < 0.05, **p < 0.01). ■, Solid-state fermentation; □, Submerged fermentation.
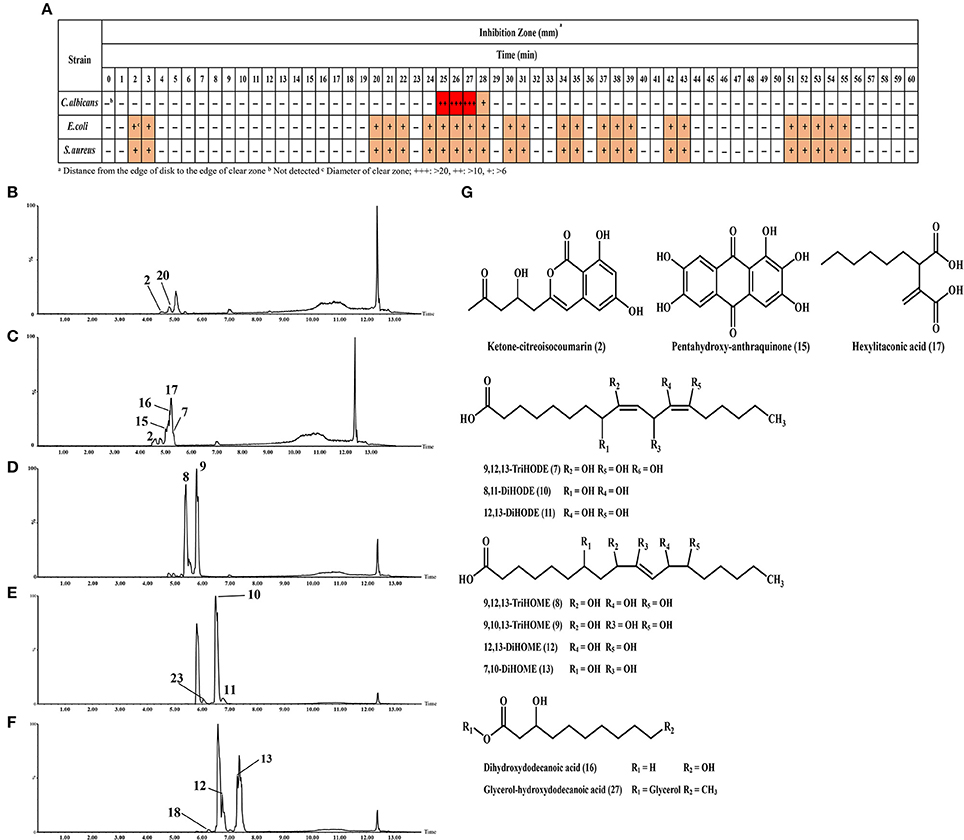
Figure 5. (A) Antimicrobial activities (against C. albicans, E. coli, and S. aureus) for preparative HPLC fractions from the 8 days incubated SSF A. oryzae extracts. Chromatograms for (B) 22 min, (C) 25 min, (D) 27 min, (E) 31 min, and (F) 38 min indicate the bioactive metabolites of each prep fraction. (G) Structure of identified metabolites.
Discussion
In this study, we unraveled a time correlated metabolomic disparity for secondary metabolites production when A. oryzae was cultivated under different culture conditions i.e., SSF and SmF. Moreover, we selected MEA and MEB media to determine the metabolomic differences between SSF and SmF. Both of the medium variants are commonly used for cultivation of filamentous fungi including Aspergillus species providing the fermentation environ rich in nutrients supporting their natural growth and sporulation. In general, the SmF is performed using aqueous nutrients, hence presumably suitable for prokaryotes cultivation with planktonic growth (Subramaniyam and Vimala, 2012). The secondary metabolites have been predominantly produced using SmF, even though the corresponding productivity was lesser compared to SSF (Subramaniyam and Vimala, 2012). The SmF has certain associated advantages viz., efficient downstream processing, relatively tractable scale-up systems, and easy to control process parameters (pH and aeration), in comparison to SSF (Robinson et al., 2001). However, the usage of SSF for the production of secondary metabolites might not be underestimated. The solid state (or substrate) fermentation is typified by a surface cultivation of cultures on nutrient rich solid support. The SSF is generally employed in the recycling or biovalorization of nutrient-rich solid waste substances (Robinson et al., 2001). In general, the SSF is suitable for filamentous fungi or any microorganism that preferably grows under aerobic conditions with low water activity (Subramaniyam and Vimala, 2012). Previously, the comparative studies on enzyme production, including glucoamylase, protease, and cellulolytic enzymes in Aspergillus species under SSF and SmF conditions have been extensively studied (Hansen et al., 2015). Moreover, the studies on primary metabolites, such as organic acids (i.e., succinic acid) and energy sources that directly connect with both cell growth and productivity of secondary metabolites have been investigated (Du et al., 2008). However, a limited number of studies have demonstrated the difference between the nature and the relative abundance of secondary metabolites produced during SSF and SmF involving A. oryzae.
Disparity of Secondary Metabolite Profiles Between SSF and SmF
The comparative metabolomic analysis of SSF and SmF extracts of A. oryzae demonstrated a marked distinction between secondary metabolite profiles (Figure 1). Overall, twenty-six identified metabolites were classified into three classes of compounds that were observed discriminant between the two fermentation conditions (Table 1). Moreover, all of metabolites were tentatively identified except for metabolite with standard compound such as 12,13-DiHOME (12) and 9 non-identifications. In agreement with our results, the coumarins (1–2), terpenoids (3–6), and unsaturated fatty acids (7–13) have been previously reported from Aspergillus species (Schmidt-Dannert, 2015; Costa et al., 2016; Fischer and Keller, 2016). We also examined other secondary metabolites, including diketopiperazines i.e., dihydroxy-methoxyphenylalanine (14), pentahydroxy-anthraquinone (15), dihydroxydodecanoic acid (16), and hexylitaconic acid (18) in A. oryzae, reported in different Aspergillus spp. (Varoglu and Crews, 2000; Lin et al., 2008; Senthilkumar et al., 2014; Fouillaud et al., 2016).
The production of secondary metabolites in filamentous fungi varies remarkably depending on their different culture conditions. Herein, we observed a marked disparity in the nature and abundance of significantly discriminant secondary metabolites produced under SSF and SmF conditions for A. oryzae. In particular, the relative abundance of coumarins (1–2), unsaturated fatty acids (7–13), diketopiperazine derivatives (14), pentahydroxy-anthraquinone (15), dihydroxydodecanoic acid (16), and hexylitaconic acid (18), were observed selectively higher in SSF. Among the different metabolite classes, coumarins broadly are represented by benzopyrone compounds with fused benzene and alpha-pyrone rings. Approximately 1300 coumarins have been identified as secondary metabolites from plants, bacteria, and fungi (Costa et al., 2016). Functionally, coumarins are attractive candidates for combinatorial biosynthesis owing to their established biological activities viz., antioxidant, antibacterial, antifungal, and antiulcer potentials (Costa et al., 2016). Previously, six Aspergillus species were evaluated under different culture conditions i.e., resting (similar to SSF) and submerged culture (relatively disturbed, similar to SmF) states, with the prior one showing higher coumarin bioconversion rates (Costa et al., 2016). Hence, we suggest that the resting state of mycelia in SSF might have resulted in an enhanced biosynthesis of coumarins.
On the other hand, terpenoids were relatively higher in SmF extracts than those in SSF extracts. The terpenoid compounds viz., sesquiterpenoids, diterpenoids, triterpenoids, and meroterpenoids exhibit a range of bioactivities including antibacterial, antifungal, antitumor, and anticancer (Schmidt-Dannert, 2015). In this study, we distinctively identified asperaculin A (sesquiterpenoid) and austalide F and H (meroterpenoids) in A. oryzae. All of these secondary metabolites were previously reported from marine-derived Aspergillus species (Ingavat et al., 2011; Schmidt-Dannert, 2015; Peng et al., 2016). Many reports have been published on the optimization of submerged fermentation to produce bioactive terpenoids (Xiao and Zhong, 2016). In particular, it was reported that the titer of ganoderic acid (GA) and oxygenated lanostane-type triterpenoid was affected by pH shifts and dissolved oxygen tension (DOT) levels, illustrating the effects of cultivation parameters and physiological states on metabolite production (Xiao and Zhong, 2016). Typically, at the beginning of submerged fermentation, the amount of oxygen is high, but as the fermentation progresses, the pH and oxygen level decrease during SmF, and hence the terpenoid contents are expected increase during later stage of SmF (Tang et al., 2009; Vendruscolo et al., 2012; Arumugam et al., 2014).
The unsaturated fatty acids play important roles in prokaryotic and eukaryotic organism, such as cell proliferation, apoptosis, tissue repair, inflammation, and immune cell behavior (Tsitsigiannis and Keller, 2007). The oxygen containing unsaturated fatty acids, trivially the oxylipins, mediate crucial biological activities, including both the intra- and inter-cellular signaling in plants, vertebrates, invertebrates, and fungi (Tsitsigiannis and Keller, 2007). Functionally, the oxylipins are known to exhibit many pharmacological activities viz., antimicrobial and anti-inflammatory (Tsitsigiannis and Keller, 2007). In fungi, the oxylipins regulate cell growth, modulation of spore shape, and germination rate, as well as maintain the ratio of sexual to asexual spore development (Fischer and Keller, 2016). Although the comparative studies profiling the oxylipin class metabolites between SSF and SmF are limited, the levels of the index of unsaturated fatty acids (IUFA, a fatty acid precursor of oxylipins) were reportedly higher in SSF than SmF, and hence the concentration of oxylipin ought to be relatively higher in SSF (Zhang et al., 2015).
Moreover, several miscellaneous discriminant metabolites viz., diketopiperazine derivatives (14), pentahydroxy-anthraquinone (15), dihydroxydodecanoic acid (16), and hexylitaconic acid (18) were observed relatively abundant in SSF. Although, the studies describing the effects of culture conditions on these discriminant metabolites are limited, the higher production of bio-pigments was reported in SSF compared to SmF, potentially justifying the relatively higher abundance of pentahydroxy-anthraquinone (a natural pigment) under SSF in the present study (Fouillaud et al., 2016; Sehrawat et al., 2017). In addition, the rest of the three distinctive metabolites might also have conferred some adaptive and cryptic function in cultivated molds. Most of these metabolites were previously reported from Aspergillus species, reportedly exhibiting a wide range of bioactivities (Varoglu and Crews, 2000; Lin et al., 2008; Senthilkumar et al., 2014). However, the adaptive functions and physiological alteration under different culture conditions need to be studied further in terms of individual functions of metabolite classes.
Antimicrobial Compounds and Their Efficacies
We examined overall 12 metabolites, identified from the active prep-HPLC fractions of SSF extracts, positively exhibiting antimicrobial activities. Positively exhibiting antimicrobial active metabolites were also identified tentatively except for metabolite with standard compound. Owing to the relatively higher and earlier display of antimicrobial activities (Figure 4), we selectively performed prep-HPLC fractionation for 8-day incubated SSF extracts toward the separation of bioactive compounds. Recently, the coumarins related to ketone-citreoisocoumarin (2) were isolated from various organisms, and their antimicrobial activities were established against E. coli and C. albicans (Costa et al., 2016). The parent “coumarin” structure has not only the ability of non-covalent interactions (hydrophobic and electrostatic interactions, hydrogen bonds, and van der Waals force, among others) with many active sites, but also pharmacological activities, such as antimicrobial and antioxidant, among others (Barot et al., 2015). Earlier, it was reported that the hydroxylated coumarins at the C-6, C-7, and C-8 position inhibit bacterial growth through damaging the cell membrane (Yang et al., 2016a).
In agreement with our results, the oxygenated fatty acids (oxylipins) were previously reported to exert antibacterial and antifungal effects (Tsitsigiannis and Keller, 2007). Recently, Ma et al. (2016) have established the antifungal mechanisms for synthetic compounds mimicking the natural oxylipins, proposed that the linear aliphatic compound with more -OH groups efficiently interact with fungal cytoderm showing higher inhibitory activity. Among the saturated fatty acids, dihydroxydodecanoic acid (16) and glycerol-hydroxydodecanoic acid (27) were previously reported to exhibit antibacterial activity (Smith et al., 2008). The saturated fatty acid with a C-9 straight-chain, dodecanoic acid (lauric acid), had associated antibacterial activity, which specifically disrupts bacterial growth. Moreover, the esterification of dodecanoic acid with glyceryl to form glycerides also demonstrated antimicrobial activities (Smith et al., 2008).
Among the polyketides, anthraquinone and hydroxyanthraquinone, specifically exhibited high antimicrobial activities. As previously reported, anthraquinone derivatives related to pentahydroxy-anthraquinone (15) inhibited the growth of S. aureus and E. coli (Fouillaud et al., 2016). Anthraquinone belongs to a class in the quinone family, with its structure containing three benzene rings coupled with two ketone groups attached to the central ring. The higher degree of oxidative stress induced by these compounds might be linked to the reported antibacterial and antifungal activities (Fouillaud et al., 2016). Further, hexylitaconic acid (18) from an endophytic fungus, Eupenicillium sp. LG41, exhibited noticeable efficacy against Acinetobacter sp. BD4, S. aureus, and E. coli (Li et al., 2014). Additionally, the N.I. 2 (20) might be inhibited the growth of S. aureus and E. coli. Based on the relatively higher abundance of bioactive metabolite production in SSF and their established antimicrobial potentials, we can speculate why Aspergillus emerges as the dominant genus during the course of fermentation and exhibiting a similar effect as a biopreservative in fermented food. We putatively identified the specific bioactive metabolites, which could confer a competitive edge to A. oryzae, during colonization of fermentative substrates. However, the present hypothesis needs to be firmly established through more comprehensive metabolite profiling and correlation with their functional and antimicrobial activities in additional studies using diverse strains relevant to the commercial fermentation practices.
In conclusion, we evaluated the non-targeted metabolomic profiles using LC-ESI-MS/MS and antimicrobial activities of A. oryzae 12698 under different fermentation states (SSF and SmF). This approach demonstrated that the production of secondary metabolites in A. oryzae was significantly affected by different cultivation conditions with SSF observed to be noteworthy in relation to antimicrobial metabolite production. In addition, specific bioactive metabolites were putatively identified that could confer a competitive edge to A. oryzae during colonization on fermentative substrates. This study provides a metabolomic framework underpinning the growth and bioactive compound production in A. oryzae in different culture conditions. Furthermore, the comprehensive profiling of the secondary metabolites might illuminate the range of secreted metabolic classes helpful in delineating the optimal fermentative conditions and associated implications of secreted end products, either beneficial or detrimental, especially in relation to fermented foods and beverages involving Koji mold.
Author Contributions
CL designed this research. SS performed the experiments and data analysis. SS, SL, DS, N-RL, and D-YL conducted the data interpretation. The samples of Aspergillus oryzae KCCM 12698 were procured from the Korean Culture Center of Microorganism. The remainder of microorganisms, of Candida albicans KACC 30062, Staphylococcus aureus KACC 1621, and Escherichia coli KCTC 1682, were provided by the Korean Agricultural Culture Collection and the Korean Collection for Type Cultures. SS wrote the paper. All authors approved the final manuscript.
Conflict of Interest Statement
The authors declare that the research was conducted in the absence of any commercial or financial relationships that could be construed as a potential conflict of interest.
Acknowledgments
This research was supported by Traditional Culture Convergence Research Program through the National Research Foundation of Korea (NRF) funded by the Ministry of Science, ICT and Future Planning (NRF-2017M3C1B5019303) and WTU Joint Research Grants of Konkuk University in 2017.
Supplementary Material
The Supplementary Material for this article can be found online at: https://www.frontiersin.org/articles/10.3389/fmicb.2018.01076/full#supplementary-material
Abbreviations
SSF, Solid state fermentation; SmF, Submerged fermentation; UHPLC-LTQ-IT-MS/MS, Ultra high-performance liquid chromatography-linear trap quadrupole-ion trap-tandem mass spectrometry; UPLC-Q-TOF-MS, Ultraperformance liquid chromatography-quadrupole-time of flight-mass spectrometry; PCA, Principal component analysis; PLS-DA, Partial least squares projection to latent structures discriminant analysis
References
Arumugam, G. K., Srinivasan, S. K., Joshi, G., Gopal, D., and Ramalingam, K. (2014). Production and characterization of bioactive metabolites from piezotolerant deep sea fungus Nigrospora sp. in submerged fermentation. J. Appl. Microbiol. 118, 99–111. doi: 10.1111/jam.12693
Barot, K. P., Jain, S. V., Kremer, L., Singh, S., and Ghate, M. D. (2015). Recent advances and therapeutic journey of coumarins: current status and perspectives. Med. Chem. Res. 24, 2771–2798. doi: 10.1007/s00044-015-1350-8
Chen, T., Wang, M., Li, S., Wu, Q., and Wei, H. (2014). Molecular identification of microbial community in surface and undersurface douchi during postfermentation. J. Food Sci. 79:4. doi: 10.1111/1750-3841.12417
Chilton, S. N., Burton, J. P., and Reid, G. (2015). Inclusion of fermented foods in food guides around the world. Nutrient 7, 390–404. doi: 10.3390/nu7010390
Costa, T. M., Tavares, L. B. B., and de Oliveira, D. (2016). Fungi as a source of natural coumarins production. Appl. Microbiol. Biotechnol. 100, 6571–6584. doi: 10.1007/s00253-016-7660-z
Dang, J., Shao, Y., Zhao, J., Mei, L., Tao, Y., Wang, Q., et al. (2016). Two-dimensional hydrophilic interaction chromatography × reversed-phase liquid chromatography for the preparative isolation of potential anti-hepatitis phenylpropanoids from Salvia prattii. J. Sep. Sci. 39, 3327–3338. doi: 10.1002/jssc.201600401
Du, C., Lin, S. K. C., Koutinas, A., Wang, R., Dorado, P., and Webb, C. (2008). A wheat biorefining strategy based on solid-state fermentation for fermentative production of succinic acid. Bioresource Technol. 99, 8310–8315. doi: 10.1016/j.biortech.2008.03.019
Fischer, G. J., and Keller, N. P. (2016). Production of cross-kingdom oxylipins by pathogenic fungi: an update on their role in development and pathogenicity. J. Microbiol. 54, 254–264. doi: 10.1007/s12275-016-5620-z
Fouillaud, M., Venkatachalam, M., Girard-Valenciennes, E., Caro, Y., and Dufossé, L. (2016). Anthraquinones and derivatives from marine-derived fungi: structural diversity and selected biological activities. Mar. Drugs 14, 1–64. doi: 10.3390/md14040064
Godzien, J., Alonso-Herranz, V., Barbas, C., and Armitage, E. G. (2015). Controlling the quality of metabolomics data: new strategies to get the best out of the QC sample. Metabolomics 11, 518–528. doi: 10.1007/s11306-014-0712-4
Hansen, G. H., Lübeck, M., Frisvad, J. C., Lübeck, P. S., and Andersen, B. (2015). Production of cellulolytic enzymes from ascomycetes: comparison of solid state and submerged fermentation. Process Biochem. 50, 1327–1341. doi: 10.1016/j.procbio.2015.05.017
Ingavat, N., Mahidol, C., Ruchirawat, S., and Kittakoop, P. (2011). Asperaculin A, a sesquiterpenoid from a marine-derived fungus, Aspergillus aculeatus. J. Nat. Prod. 74, 1650–1652. doi: 10.1021/np200221w
Kang, D., Son, G. H., Park, H. M., Kim, J., Choi, J. N., Kim, H. Y., et al. (2013). Culture condition-dependent metabolite profiling of Aspergillus fumigatus with antifungal activity. Fungal Biol. 117, 211–219. doi: 10.1016/j.funbio.2013.01.009
Kim, H. Y., Park, H. M., Singh, D., and Lee, C. H. (2016). Metabolomic and transcriptomic comparison of solid-state and submerged fermentation of Penicillium expansum KACC 40815. PLoS ONE 11:e0149012. doi: 10.1371/journal.pone.0149012
Klančnik, A., Piskernik, S., Jeršek, B., and MoŽina, S. S. (2010). Evaluation of diffusion and dilution methods to determine the antibacterial activity of plant extracts. J. Microbiol. Methods 81, 121–126. doi: 10.1016/j.mimet.2010.02.004
Lee, M. Y., Park, H. M., Son, G. H., and Lee, C. H. (2013). Liquid chromatography-mass spectrometry-based chemotaxonomic classification of Aspergillus spp. and evaluation of the biological activity of its unique metabolite, neosartorin. J Microbiol Biotechnol. 23, 932–941. doi: 10.4014/jmb.1212.12068
Lee, S., Singh, D., Jang, E. S., Shin, H. W., Moon, B. S., and Lee, C. H. (2017). Time-resolved comparative metabolomes for Koji fermentation with brown-, white-, and giant embryo-rice. Food Chem. 231, 258–266. doi: 10.1016/j.foodchem.2017.03.119
Li, G., Kusari, S., Lamshöft, M., Schüffler, A., Laatsch, H., and Spiteller, M. (2014). Antibacterial secondary metabolites from an endophytic fungus, Eupenicillium sp. LG41. J. Nat. Prod. 77, 2335–2341. doi: 10.1021/np500111w
Lin, A., Fang, Y., Zhu, T., Gu, Q., and Zhu, W. (2008). A new diketopiperazine alkaloid isolated from an algicolous Aspergillus flavus strain. Pharmazie 63, 323–324. doi: 10.1691/ph.2008.7700
Lynn, K. S., Cheng, M. L., Chen, Y. R., Hsu, C., Chen, A., Lih, T. M., et al. (2015). Metabolite identification for mass spectrometry-based metabolomics using multiple types of correlated ion information. Anal. Chem. 87, 2143–2151. doi: 10.1021/ac503325c
Ma, J., Li, Y., Chen, H., Zeng, Z., Li, Z. L., and Jiang, H. (2016). Synthesis of oxylipin mimics and their antifungal activity against the Citrus postharvest pathogens. Molecules 21:2. doi: 10.3390/molecules21020254
Matsuda, Y., Awakawa, T., and Abe, I. (2013). Reconstituted biosynthesis of fungal meroterpenoid andrastin A. Tetrahedron 69, 8199–8204. doi: 10.1016/j.tet.2013.07.029
Miranda, R. U., Gómez-Quiroz, L. E., Mendoza, M., Pérez-Sánchez, A., Fierro, F., and Barrios-González, J. (2014). Reactive oxygen species regulate lovastatin biosynthesis in Aspergillus terreus during submerged and solid-state fermentations. Fungal Biol. 118, 979–989. doi: 10.1016/j.funbio.2014.09.002
Nilsson, T., Martínez, E., Manresa, A., and Oliw, E. H. (2010). Liquid chromatography/tandem mass spectrometric analysis of 7, 10-dihydroxyoctadecenoic acid, its isotopomers, and other 7, 10-dihydroxy fatty acids formed by Pseudomonas aeruginosa 42A2. Rapid Commun. Mass Spectrom. 24, 777–783. doi: 10.1002/rcm.4446
Nørskov, N. P., Hedemann, M. S., Lærke, H. N., and Knudsen, K. E. B. (2013). Multicompartmental nontargeted LC–MS metabolomics: Explorative study on the metabolic responses of rye fiber versus refined wheat fiber intake in plasma and urine of hypercholesterolemic pigs. J. Proteome Res. 12, 2818–2832. doi: 10.1021/pr400164b
Oh, D. G., Jang, Y. K., Woo, J. E., Kim, J. S., and Lee, C. H. (2016). Metabolomics reveals the effect of garlic on antioxidant-and protease-activities during Cheonggukjang (fermented soybean paste) fermentation. Food Res. Int. 82, 86–94. doi: 10.1016/j.foodres.2016.01.019
Pansuriya, R. C., and Singhal, R. S. (2010). Response surface methodology for optimization of production of lovastatin by solid state fermentation. Braz. J. Microbiol. 41, 164–172. doi: 10.1590/S1517-83822010000100024
Peng, J., Zhang, X., Wang, W., Zhu, T., Gu, Q., and Li, D. (2016). Austalides SU, New Meroterpenoids from the Sponge-Derived Fungus Aspergillus aureolatus HDN14-107. Mar. Drugs 14, 131–140. doi: 10.3390/md14070131
Robinson, T., Singh, D., and Nigam, P. (2001). Solid-state fermentation: a promising microbial technology for secondary metabolite production. Appl. Microbiol. Biotechnol. 55, 284–289. doi: 10.1007/s002530000565
Schmidt-Dannert, C. (2015). Biosynthesis of terpenoid natural products in fungi. Adv. Biochem. Eng. Biotechnol. 148, 19–61. doi: 10.1007/10_2014_283
Sehrawat, R., Panesar, P. S., Panesar, R., and Kumar, A. (2017). Biopigment produced by Monascus purpureus MTCC 369 in submerged and solid state fermentation: a comparative study. Pigm. Resin. Technol. 46, 425–432. doi: 10.1108/PRT-10-2016-0095
Senthilkumar, N., Murugesan, S., and Babu, D. S. (2014). Metabolite profiling of the extracts of endophytic fungi of entomopathogenic significance, Aspergillus flavus and Nigrospora sphaerica isolated from tropical tree species of India, Tectona grandis L. J. Agri. Life Sci. 1, 108–114.
Shin, K. C., Seo, M. J., and Oh, D. K. (2016). Characterization of a novel 8R, 11S-linoleate diol synthase from Penicillium chrysogenum by identification of its enzymatic products. J. Lipid Res. 57, 207–218. doi: 10.1194/jlr.M061341
Singh, D., Lee, S., and Lee, C. H. (2017). Metabolomics for empirical delineation of the traditional Korean fermented foods and beverages. Trends Food Sci. Tech. 61, 103–115. doi: 10.1016/j.tifs.2017.01.001
Smith, A., Nobmann, P., Henehan, G., Bourke, P., and Dunne, J. (2008). Synthesis and antimicrobial evaluation of carbohydrate and polyhydroxylated non-carbohydrate fatty acid ester and ether derivatives. Carbohydr. Res. 343, 2557–2566. doi: 10.1016/j.carres.2008.07.012
Strassburg, K., Huijbrechts, A. M., Kortekaas, K. A., Lindeman, J. H., and Kalkhoven, E. (2012). Quantitative profiling of oxylipins through comprehensive LC-MS/MS analysis: application in cardiac surgery. Anal. Bioanal. Chem. 404, 1413–1426. doi: 10.1007/s00216-012-6226-x
Subramaniyam, R., and Vimala, R. (2012). Solid state and submerged fermentation for the production of bioactive substances: a comparative study. Int. J. Sci. Nat. 3, 480–486.
Tang, Y. J., Zhang, W., and Zhong, J. J. (2009). Performance analyses of a pH-shift and DOT-shift integrated fed-batch fermentation process for the production of ganoderic acid and Ganoderma polysaccharides by medicinal mushroom Ganoderma lucidum. Bioresour. Technol. 100, 1852–1859. doi: 10.1016/j.biortech.2008.10.005
Tsitsigiannis, D. I., and Keller, N. P. (2007). Oxylipins as developmental and host-fungal communication signals. Trends Microbiol. 15, 109–118. doi: 10.1016/j.tim.2007.01.005
Varoglu, M., and Crews, P. (2000). Biosynthetically diverse compounds from a saltwater culture of sponge-derived Aspergillus niger. J. Nat. Prod. 63, 41–43. doi: 10.1021/np9902892
Vendruscolo, F., Rossi, M. J., Schmidell, W., and Ninow, J. L. (2012). Determination of oxygen solubility in liquid media. ISRN Chem. Eng. 2012, 1–5. doi: 10.5402/2012/601458
Xiao, H., and Zhong, J. J. (2016). Production of useful terpenoids by higher-fungus cell factory and synthetic biology approaches. Trends Biotechnol. 34, 242–255. doi: 10.1016/j.tibtech.2015.12.007
Yang, L., Ding, W., Xu, Y., Wu, D., Li, S., Chen, J., et al. (2016a). New insights into the antibacterial activity of hydroxycoumarins against Ralstonia solanacearum. Molecules 21:468. doi: 10.3390/molecules21040468
Yang, L., Yang, H. L., Tu, Z. C., and Wang, X. L. (2016b). High-throughput sequencing of microbial community diversity and dynamics during Douchi fermentation. PLoS ONE 11:e0168166. doi: 10.1371/journal.pone.0168166
Keywords: Aspergillus oryzae, solid state fermentation, submerged fermentation, metabolomics/metabolite profiling, antimicrobial activity
Citation: Son SY, Lee S, Singh D, Lee N-R, Lee D-Y and Lee CH (2018) Comprehensive Secondary Metabolite Profiling Toward Delineating the Solid and Submerged-State Fermentation of Aspergillus oryzae KCCM 12698. Front. Microbiol. 9:1076. doi: 10.3389/fmicb.2018.01076
Received: 05 December 2017; Accepted: 07 May 2018;
Published: 25 May 2018.
Edited by:
Ozgur Bayram, Maynooth University, IrelandReviewed by:
Jae-Hyuk Yu, University of Wisconsin-Madison, United StatesNancy Keller, University of Wisconsin-Madison, United States
Copyright © 2018 Son, Lee, Singh, Lee, Lee and Lee. This is an open-access article distributed under the terms of the Creative Commons Attribution License (CC BY). The use, distribution or reproduction in other forums is permitted, provided the original author(s) and the copyright owner are credited and that the original publication in this journal is cited, in accordance with accepted academic practice. No use, distribution or reproduction is permitted which does not comply with these terms.
*Correspondence: Choong H. Lee, Y2hsZWUxMjNAa29ua3VrLmFjLmty