- Earth and Life Institute, Applied Microbiology, Mycology, Université catholique de Louvain, Louvain-la-Neuve, Belgium
Climate change scenarios predict a higher variability in rainfall and an increased risk of water deficits during summers for the coming decades. For this reason, arbuscular mycorrhizal fungi (AMF) and their mitigating effects on drought stress in plants are increasingly considered in crop management. However, the impact of a decrease in water availability on the development of AMF and their ability to take up and transport inorganic phosphorus (Pi) to their hosts remain poorly explored. Here, Medicago truncatula plantlets were grown in association with Rhizophagus irregularis MUCL 41833 in bi-compartmented Petri plates. The system consisted in associating the plant and AMF in a root compartment (RC), allowing only the hyphae to extend in a root-free hyphal compartment (HC). Water availability in the HC was then lowered by increasing the concentration of polyethylene glycol-8000 (PEG-8000) from 0 to 10, 25, and 50 g L-1 (corresponding to a slight decrease in water potential of -0.024, -0.025, -0.030, and -0.056 Mpa, respectively). Hyphal growth, spore production and germination were severely impaired at the lowest water availability. The dynamics of Pi uptake by the AMF was also impacted, although total Pi uptake evaluated after 24 h stayed unchanged. The percentage of metabolically active extraradical hyphae remained above 70%. Finally, at the lowest water availability, a higher P concentration was observed in the shoots of M. truncatula. At reduced water availability, the extraradical mycelium (ERM) development was impacted, potentially limiting its capacity to explore a higher volume of soil. Pi uptake was slowed down but not prevented. The sensitivity of R. irregularis MUCL 41833 to a, even small, decrease in water availability contrasted with several studies reporting tolerance of AMF to drought. This suggests a species or strain-dependent effect and support the necessity to compare the impact of water availability on morpho-anatomy, nutrient uptake and transport capacities of other, potentially more drought-tolerant (e.g., isolated from dry environments) AMF.
Introduction
Drought associated to extreme heat has been responsible for a significant drop in yield of crops and cultivable surfaces over the last 40 years (Lesk et al., 2016). Projected climate changes indicate a higher variability in rainfall in the coming decades, with an increasing risk of water deficits during summers, while water resources for irrigation will be at best maintained. Enhancing crops resilience to drought is thus a priority to secure nowadays and future’s food production (Wheeler and von Braun, 2013).
There are various soils, water and crop management practices which, together with improved genotypes, have the potential to increase water use efficiency of crops. For instance, irrigation is a practical solution to increase crop yield, although an intensification of this method is not viable on the long term as freshwater resources are limited (Elliott et al., 2014). Irrigation methods that avoid salinization of soil, soil leaching, and improve water use efficiency are alternatives, but are not always affordable for smallholders (Wezel et al., 2014; Xie et al., 2014). Soil microorganisms living in close relations with plant roots might also play a role in plant water stress mitigation via various mechanisms (see for review Kim et al., 2012). Among these below-ground inhabitants are the beneficial and naturally occurring arbuscular mycorrhizal fungi (AMF) (Cardoso and Kuyper, 2006; Rodriguez and Sanders, 2014).
Arbuscular mycorrhizal fungi are obligate root symbionts that have co-evolved with plants for an estimate of 400 million years (Parniske, 2008). They are nowadays associated with an approximate of 72% of Angiosperms (Brundrett, 2009) in almost all ecosystems (Smith and Read, 2008). Their beneficial effects on plant nutrition and growth as well as on biotic and abiotic stresses alleviation have been amply described (Miransari, 2014; Abdel Hamed Abdel Latef et al., 2016; Lenoir et al., 2016; Plouznikoff et al., 2016) so that they are today considered as essential components of agro-ecosystems. In the recent years, many studies have focused on the role of AMF in plant water stress mitigation (Aroca and Ruiz-Lozano, 2009; Jayne and Quigley, 2014; Fernández-Lizarazo and Moreno-Fonseca, 2016; Farooq et al., 2017). Under dry environments, AMF have been reported to regulate plant physiology and metabolism (Wu and Zou, 2017) by changing absissic acid/cytokinin hormone balance (Goicoechea et al., 1997) and by protecting the host plants from oxidative damages under osmotic stress conditions (Porcel and Ruiz-Lozano, 2004). They have been shown to improve osmotic adjustment (Wu and Xia, 2006) and water lift from roots to shoots via higher water apoplastic flow (Bárzana et al., 2012). They have also been reported to increase cell to cell water transport through increased expression of aquaporin membrane proteins (Aroca et al., 2007; Ruiz-Lozano et al., 2009). Increased drought tolerance of AMF-colonized plants has also been associated to an improved nutrition, especially inorganic phosphorus (Pi) that is transported by the extraradical hyphae of the fungus to the root cells (Nelsen and Safir, 1982; Bethlenfalvay et al., 1988; Fitter and Nichols, 1988). The extraradical hyphae can increase the access of plants to soil water (Sánchez-Díaz and Honrubia, 1994; Ahmad Khan et al., 2003) and increase soil water retention through glomalin excretion (Augé, 2001; Wu et al., 2008).
Despite the quite large number of studies reporting the effects of AMF on plant physiology and growth under drought stress conditions, little is known on the impact of decreased water availability on the development of the fungus and its capacity to take up and transport Pi to plants, an essential attribute of these fungi. Any change with respect to their growth and development or capacity to provide the host plant with nutrients would indeed partly compromise their agro-ecological value. Today, most studies have been conducted in pots and a few in bi-compartmented systems making it possible to differentiate Pi uptake by the plant from that of the fungus (Thingstrup et al., 2000; Jansa et al., 2003; Merrild et al., 2013). However, these systems do not allow the non-destructive observation of AMF development. Moreover, the dynamics of Pi uptake by the sole extraradical mycelium (ERM) of the fungus is nearly impossible to assess due, among others, to the presence of unwanted microbial contaminants.
In vitro cultivation systems associating excised roots (Declerck et al., 2005) or whole plants (Voets et al., 2005) in mono or bi-compartmented Petri plates (St-Arnaud et al., 1996) are more indicated to overcome some of these issues. They have been used to study the development (Bago et al., 1996, 1998; St-Arnaud et al., 1996; Declerck et al., 2005) and architecture (Bago et al., 1998; de la Providencia et al., 2005; Voets et al., 2006) of the ERM as well as the intimate plant-AMF association under abiotic stress conditions (e.g., heavy metals – Pawlowska and Charvat, 2004; potentially toxic elements such as chromium – Gil-Cardeza et al., 2017; salt – Jahromi et al., 2008; Aroca and Ruiz-Lozano, 2009; fungicides – Zocco et al., 2011; polyaromatic hydrocarbons (PAHs) – Calonne et al., 2012). In particular, the utilization of bi-compartmented Petri plates (with the fungus developing in a root-free compartment) has allowed to monitor Pi uptake and transport in presence of fungicides (Zocco et al., 2011) or PAHs (Calonne et al., 2014).
A few in vitro studies have also been focused on the effects of water stress on the AMF. Indeed, mannitol has been reported to decrease germination and germ tube growth of Funneliformis mosseae (Estaun, 1989) on gelled medium. Glycerol has also been reported to decrease mycelium biomass, spore production and spore size of R. irregularis DAOM 197198 (Hammer and Rillig, 2011). More recently, osmotic stress induced by the application of polyethylene glycol (PEG-8000) in liquid Minimum (M) medium upregulated the expression of the fungal aquaporins GintAQP1 and GintAQP2 of Rhizophagus irregularis DAOM 197198 associated to transformed Daucus carota roots in bi-compartmented Petri plates (Li et al., 2013). PEG is an inert and low toxic polymer (Lawlor, 1970; Mexal and Reid, 1973), thus representing an adequate option to create water shortage in gelled medium. Over the last decades, it has become the principal compound used to study physiological response of plants cultivated in vitro to water stress (Rai et al., 2011). Therefore, it appears to be a good candidate to investigate the role of water deficit on the development and architecture of AMF and on the uptake and transport of Pi.
In the present work, we first conducted a preliminary study to assess the water potential achieved by increasing concentrations of PEG in liquid medium. We then evaluated and compared the effects of PEG-induced decreased water availability on the development (i.e., spore germination and ERM development) and on the Pi uptake and transport of R. irregularis MUCL 41833 associated to Medicago truncatula. Albeit water potential achieved under our experimental conditions are much higher than encountered under dry environments, we hypothesize that slight changes in water availability can trigger differential response of the fungus.
Materials and Methods
Biological Material
Seeds of Medicago truncatula Gaertn. cv. Jemalong A 17 (SARDI, Australia) were surface-disinfected by immersion in sodium hypochlorite (8% active chloride) for 10 min and rinsed in sterilized (121°C for 15 min) deionized water. Seeds were germinated in Petri plates (90 mm diameter) containing 35 ml of modified Strullu–Romand (MSR) medium (Declerck et al., 1998) without vitamins and supplemented with 10 g L-1 sucrose and 3 g L-1 Gelrite (Merck & Co., Kenilworth, NJ, United States). The MSR medium was adjusted to pH 5.5 before sterilization (121°C for 15 min). The Petri plates were incubated at 27°C in the dark. The seedlings were ready to use 4 days following seed germination.
The AMF R. irregularis (Błaszk., Wubet., Renker and Buscot) C. Walker and A. Schüßler comb. nov. MUCL 41833 was supplied by the Glomeromycota in vitro collection (GINCO1). The fungus was grown in bi-compartmented Petri plates (90 mm diameter) in association with M. truncatula plantlets following the method developed by Voets et al. (2009).
Preliminary Experiment: Water Potential of MSR Medium Supplemented With Increasing Concentrations of Polyethylene Glycol
Polyethylene glycol 8000 (PEG-8000; Applichem, Darmstadt, Germany) was used to induce a decrease in water availability. The efficiency of PEG in decreasing the water potential and thus water availability of the MSR medium was determined as follows: liquid MSR medium without PEG or supplemented with 10, 25, or 50 g L-1 PEG was prepared. The dew point at each concentration was determined using a vapor pressure osmometer (VAPRO® 5520, Wescor Inc., Logan, UT, United States). The osmolarities obtained from the different solutions were then converted into water potentials (ψ). Response curve of water potential to PEG concentrations was finally built using a quadratic model in the JMP statistic software (JMP®Pro 12.1.0) from SAS. Quality of fit between the model and the data was evaluated by computation of the R2.
For the various assays described below, PEG at the various concentrations was mixed with sterilized (121°C for 15 min) liquid or solid (with 3% of Gelrite) MSR medium without sucrose and vitamins. Solubilization of PEG in the MSR medium was done with a sterilized magnetic rod and at a temperature of 45°C to prevent medium solidification before pouring in the Petri plates.
Preparation of the Mycelium Donor Plants to Study the Hyphal Development, Spore Production, and Pi Uptake by the AMF
Spores (±100) of R. irregularis MUCL 41833 were associated to a 4-day-old M. truncatula plantlet in the root compartment (RC) of a 90 mm diameter bi-compartmented Petri plate [named Mycelium Donor Plant (MDP) in vitro culture system – see for details Voets et al., 2009]. The roots were plated on the MSR medium with the shoot extending outside the MDP in vitro culture system via a hole cautiously plastered with silicon grease (VWR, Radnor, PA, United States) to avoid contaminations. The RC and adjacent hyphal compartment (HC) were filled with 25 ml MSR medium without sucrose and vitamins and solidified with 3 g L-1 gelrite. The MDP in vitro culture systems were then transferred to a growth chamber at 22/18°C (day/night), 70% relative humidity (RH), a photoperiod of 16 h day-1 and a photosynthetic photon flux (PPF) of 225 μmol m-2 s-1. The systems were covered with dark plastic sheets to protect M. truncatula roots and AMF from the light, while the shoots developed under light conditions. To nourish the plantlet, RC was supplied every week with approximately 10 ml of fresh MSR medium using a 25 ml sterile pipette (VWR). To allow the passage of the pipette, a hole in the lid above the RC was made using a hot cork borer and was closed after pipetting using a double layer of sterilized plaster (MicroporeTM, 3MTM, Maplewood, MN, United States). Hyphae started to cross the partition wall separating the RC from the HC within 8 to 12 weeks. Two 4-day-old M. truncatula plantlets were subsequently transferred in the HC of each system to allow pre-mycorrhization of new plantlets. Roots were plated on the MSR medium without sucrose and vitamins and shoots extended outside the system via two holes, similarly plastered with silicon grease to avoid contaminations. The new plantlets were homogenously colonized within 12 days.
Impact of Decreasing Water Availability on Hyphal Development and Spore Production of R. irregularis MUCL 41833
Single 12-day-old pre-mycorrhized M. truncatula plantlets were plated in the RC of new bi-compartmented Petri plates using the same technique as for the preparation of MDP systems (see above). Using a sterile pipette, the RC was supplied with 15 ml of solid MSR medium without sucrose and vitamins every 2 weeks during the whole experiment. After 9 weeks, 24 systems showing profuse ERM development in the HC were selected. To allow homogenous growth of the AMF in this compartment, the MSR medium was cut 2 mm away from the partition wall and removed. The HC was then supplemented with 25 ml MSR medium lacking sucrose and vitamins and solidified with 3 g L-1 of gelrite in absence (Control-PEG treatment) or supplemented with 10, 25, and 50 g L-1 PEG (PEG10, PEG25, and PEG50 treatments, respectively). Hyphae re-colonized the MSR medium within 4 weeks. Hyphae and spore densities were homogenous along the partition wall, although their respective length and number gradually decreased along the growing front. Hyphal length and spores number were counted under binocular microscope (40× magnification) in three 10 mm2 squares located in the middle of the HC, perpendicular to the partition wall and parallel to the growing axis. Hyphal length (L) was estimated using the Newmann’s formula modified by Marsh (1971):
where N is the number of hyphal intersections with the grid, 11/14 is the ideal size of the grid sides that gives direct estimation of length in cm and grid unit = 1 cm.
Spores number was summed over the three squares (Declerck et al., 2003). Six replicates were considered per treatment.
Impact of Decreasing Water Availability on Spore Germination and Germ Tube Length of R. irregularis MUCL 41833
Spores of a two-month-old MDP in vitro culture of R. irregularis MUCL 41833 were isolated from the MSR medium following solubilization of the gelled medium with 10 mM filter-sterilized sodium citrate buffer (Doner and Becard, 1991). Spores were separated with forceps and placed single with a micropipette in each well of two 24-well tissue culture plates (VWR, Radnor, PA, United States) containing 2 ml of solid MSR medium without sucrose and vitamins and without PEG (Control-PEG treatment) or supplemented with 10, 25, and 50 g L-1 PEG (PEG10, PEG25, and PEG50 treatments, respectively). The 24-well tissue culture plates were then sealed with cellophane (Toppits®, Melitta Group, Germany) and incubated in the dark at 27°C. Spores germination was estimated at regular intervals for 110 days and hyphal length of germinated spores was similarly evaluated at day 110 under binocular microscope (30× magnification).
For Control-PEG, PEG10 and PEG25 but not PEG50 treatment, Gompertz model was fitted to the germination counts by non-linear (NLIN) regression using Gauss–Newton minimization algorithm in SAS software. Effect of the treatment was evaluated by testing a significant reduction of the residual sum of square when parameterizing with and without an effect of the treatment using the Fisher–Snedecor F-test with 1 and t-4 df (Seber and Wild, 1989; Declerck et al., 2001): where SSEr is the residual sum of square in reduced model and SSEf in the full model, MSEf = residual mean square in the full model.
Parameter estimates of the model in the different treatments were further compared to assess an effect on the dynamics of germination in the HC. Confidence intervals were built around the following estimated parameters: coefficient “a” referred to the cumulated number of germinated spores when the plateau phase was reached, “b” referred to the maximum spore germination rate (tangent of the curve at the inflection point), and “c” referred to the lag time (time to the intersection between the tangent at the inflection point and the time axis).
Impact of Decreasing Water Availability on Pi Uptake by R. irregularis MUCL 41833, SDH Activity of Hyphae, Plant Biomass, and Phosphorus Concentration
Phosphorus uptake by R. irregularis MUCL 41833 was measured indirectly by monitoring Pi concentration remaining in the medium of the HC of the MDP in vitro culture systems. This approach cannot exclude that some of the Pi is adsorbed on the hyphae, although most studies conducted in vitro (e.g., Nielsen et al., 2002; Zocco et al., 2011; Calonne et al., 2014; Gil-Cardeza et al., 2017) clearly show that the vast majority of Pi is taken up and transported by the mycelium. Single 12-day-old pre-mycorrhized M. truncatula plantlets were plated in the RC of bi-compartmented Petri plates using the same technique as for the preparation of MDP systems (see above). The RC was supplied with 15 ml of solid MSR medium without sucrose and vitamins every 2 weeks during the whole experiment. A profuse ERM was produced in the RC within 9 weeks and started to cross the partition wall separating the RC from the HC (Figure 1). At that time, the gelled MSR medium in the HC was entirely removed and replaced by 15 ml of liquid MSR medium without sucrose and vitamins (Figure 1). A hole in the lid of the system was made above the HC, similarly to the procedure described in Section “Preparation of the Mycelium Donor Plants to Study the Hyphal Development, Spore Production, and Pi Uptake by the AMF.” Using a 25 ml sterile pipette, the HC was then supplied weekly with approximately 15 ml of liquid MSR medium. Profuse hyphal regrowth in the HC was obtained within 7 weeks. The MDP in vitro culture systems were then separated in four homogenous groups based on the surface covered by the hyphae in the HC. Hyphal surface was estimated following the method described by Voets et al. (2009) by tracing on a transparent sheet the growing front constituted by the hyphal network. This measure confirmed no significant differences (P = 0.6395) between treatments.
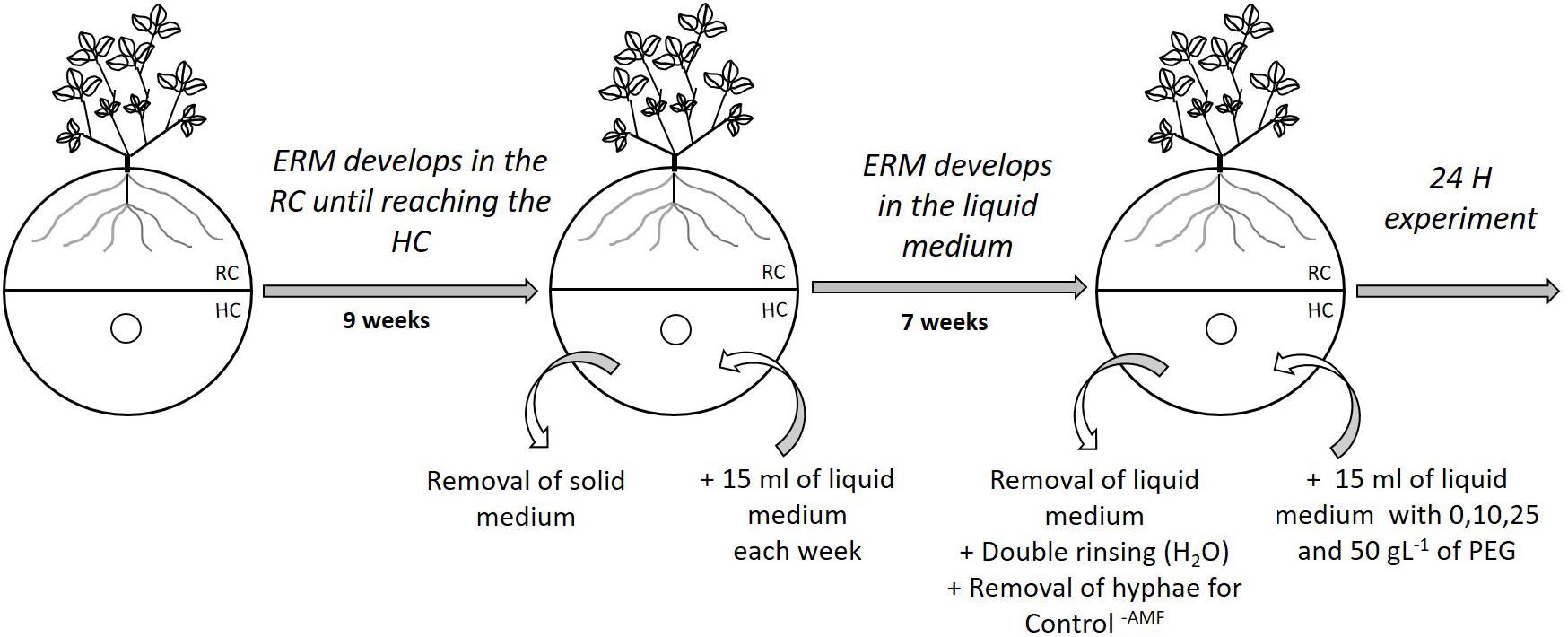
FIGURE 1. Schematic representation of the preparation of bi-compartmented Petri plates to assess the impact of decreasing water availability on the inorganic phosphorus uptake by Rhizophagus irregularis MUCL 41833. The fungus is associated with Medicago truncatula roots in the root compartment (RC) and extraradical mycelium (ERM) extends in the hyphal compartment (HC). Small circles represent the opening made in the lid of the Petri plate used for adding modified Strullu–Romand (MSR) medium. Addition of solid MSR medium in the RC is not represented in the scheme.
Four treatments, each with six replicates, were considered: MDP in vitro culture systems without PEG in the HC (Control-PEG treatment) or supplemented with 10, 25, and 50 mg L-1 PEG (PEG10, PEG25, PEG50 treatments, respectively). A control (six replicates) without mycelium in the HC (Control-AMF treatment) was also considered by cutting and removing the ERM from the HC. Liquid MSR medium was retrieved from the HC and the compartment was rinsed two times with sterilized deionized water (Figure 1). Fifteen ml of fresh liquid MSR medium was added to the Control-AMF and Control-PEG treatments, whereas liquid MSR medium containing 10, 25, and 50 g L-1 PEG was added to the other treatments (Figure 1). The Pi concentration in the HC at the start of experiment was determined by inductively coupled plasma atomic emission spectroscopy (ICP-AES; ICP 6500, Thermo-Scientific). The value was 0.59 mg L-1 and total P content 0.09 mg.
For Pi concentrations analysis in the HC, 0.5 ml of MSR medium was sampled every 2 h during 24 h from the HC of each system. The samples were diluted (1/10) and digested with 20 μl of nitric acid (65%, Suprapur®, Merck, Germany) and finally analyzed by ICP-AES. Data of Pi concentration obtained in ppm were subsequently converted into mg L-1 and standardized as in Garcés-Ruiz et al. (2017). Data were finally transformed into % of Pi remaining in the medium which is the percentage of the Pi concentration remaining in the medium of the HC at time 2, 4, 6, 8, 12, 18, 24 h relative to the Pi concentration in the medium of the HC at time T0:
where [Pix] = Pi concentration in the medium of the HC for the sample x considered
t = time considered (2, 4, 6, 8, 12, 18, or 24 h after the start of the experiment)
t0 = 0 h before the start of the experiment
[Pic] = Mean Pi concentration measured over six replicates in the Control-AMF treatment
At the end of the sampling phase, the ERM in the HC was harvested from the liquid MSR medium and stained for succinate dehydrogenase (SDH) detection. The SDH staining method enabled to evaluate the proportion of metabolically active hyphae (Saito, 1995; Vierheilig et al., 2005). Hyphae were harvested and incubated overnight at room temperature in the dark in a freshly prepared staining solution containing Tris–HCl (50 mM), MgCl2 (0.5 mM), sodium succinate (25.1 mM) and Nitroblue tetrazolium (1.22 mM). The samples were then washed with deionized water, counterstained in 0.1% acid fuchsin in lactic acid for 15 min and transferred to lactoglycerol for de-staining. Hyphae samples were mounted on microscope slides with lactoglycerol. The precipitation of substrate after enzymatic reaction was assessed under a bright field dissecting microscope (Olympus SZ, Olympus Optical, Germany) at 500× magnification. For each sample, an approximate of 100 hyphal intersections was observed. According to Van Aarle et al. (2002), intersections of hyphae were classified as active or non-active following substrate precipitation or not.
Following harvest, shoots and roots were separated and dry weights evaluated after 48 h at 45°C in an oven. The dried shoots and roots material were then grinded and subsamples heated overnight at a temperature of 60°C with 4 ml of nitric acid 65% followed by an evaporation step at 120°C. Subsamples were then dissolved in 2 ml solution of aqua regia (3/4 HCL 38% for 1/4 HNO3 65%). The solutions were filtered and diluted in 25 ml of purified MilliQ (MilliporeTM) water for P concentration analysis via ICP-AES.
Statistical Analysis
Data analysis was performed with JMP or SAS statistical software (SAS Institute Inc., Cary, NC, United States) with a 5% α-threshold. Pairwise comparisons following chi-square tests were analyzed using Bonferroni’s correction. Significant differences between treatments for water potential measurements, hyphal lengths, shoots and roots dry biomass, shoots and roots P concentrations and content as well as for hyphal surfaces, and relative water contents were tested using analysis of variance with one factor (ANOVA1) followed by a post hoc Tuckey (α = 5%) test. Levene’s test was run prior to the ANOVA analysis to confirm homogeneity of variance in the distribution. Number of spores produced were tested using a likelihood ratio test for negative binomial model. Data corresponding to the % of Pi remaining in the medium were submitted to an arcsin-1 transformation and submitted to repeated-measures ANOVA with REML estimation where “Treatment” was regarded as a fixed factor and “Time” as random factor. Additionally, % of Pi remaining in the medium was compared for each time using ANOVA1 test as described above. Finally, SDH activity and spore germination rates were submitted to a chi-squared test.
Results
Water Potential of MSR Medium Supplemented With Increasing Concentrations of Polyethylene Glycol
Water potential of the MSR medium was significantly lower in the PEG50 treatment as compared to the Control-PEG, PEG10, and PEG25 treatments (Figure 2). No significant differences were noticed between the Control-PEG treatment and the PEG10 or PEG25 treatments. Modeling of the response by linear regression (data not shown) using a quadratic model showed high statistic fitting (R2= 0.93501).
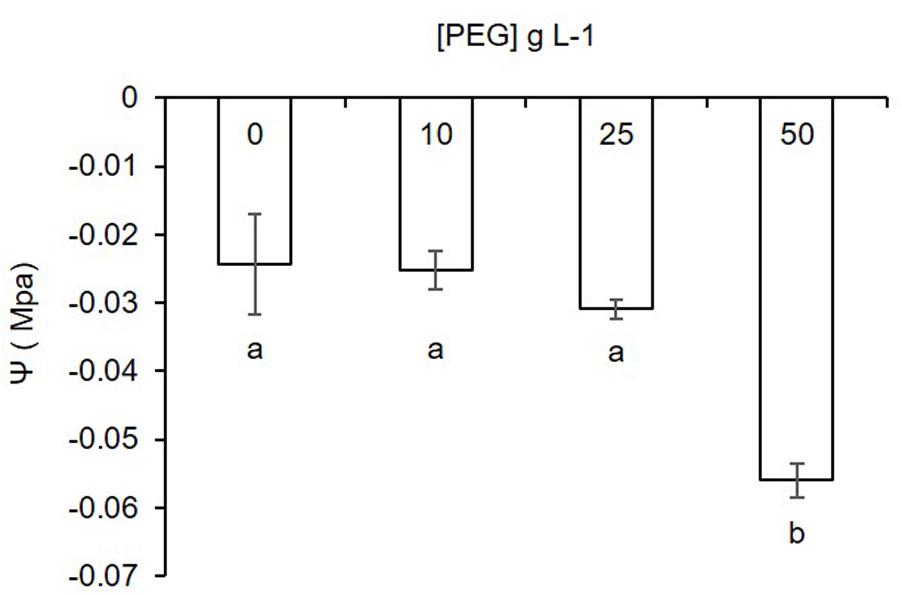
FIGURE 2. Water potential (Ψ) response of the MSR medium supplemented with increasing concentrations of polyethylene glycol (PEG) (0, 10, 25, and 50 g L-1). Data are represented as means ± SD (n = 3). Different letters below bars indicate significant differences between treatments using pair-wised comparison (Tuckey’s test, P < 0.05).
Impact of Decreasing Water Availability on Hyphal Development and Spore Production of R. irregularis MUCL 41833
The hyphal length and number of newly produced spores in presence of increasing concentrations of PEG are presented in Table 1. After 4 weeks of growth in the HC, the hyphal length was significantly higher in the Control-PEG treatment as compared to the PEG50 treatment, but did not differ from the PEG10 and PEG25 treatments (Table 1). No differences were found between the PEG10, PEG25, and PEG50 treatments (Table 1).
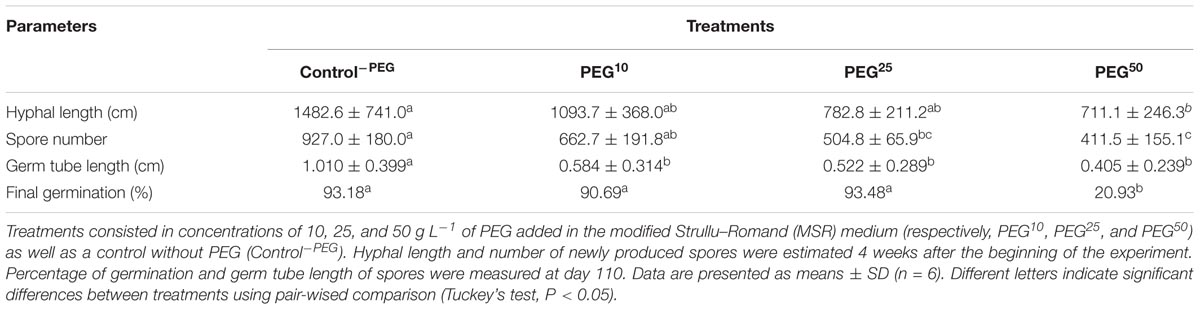
TABLE 1. Effect of decreasing water availability on (i) hyphal length and spore productions of R. irregularis MUCL 41833 developing in the hyphal compartment (HC) of a mycorrhizal donor plant in vitro culture system and on (ii) % of germination and germ tube length of isolated spores.
The number of newly produced spores was significantly higher in the Control-PEG treatment as compared to the PEG25 and PEG50 treatments, but did not differ from the PEG10 treatment (Table 1). Similarly, the number of spores in the PEG10 treatment was significantly higher as compared to the PEG50 treatment but did not differ from the PEG25 treatment (Table 1). No significant difference in number of spores was noticed between the PEG25 and PEG50 treatments (Table 1).
Impact of Decreasing Water Availability on Spore Germination and Germ Tube Length of R. irregularis MUCL 41833
The percentage of germinated spores at the end of the experiment (i.e., day 110) was significantly lower in the PEG50 treatment (i.e., 20.9%) as compared to the Control-PEG (i.e., 93.2%), PEG10 (i.e., 90.7%), and PEG25 (i.e., 93.6%) treatments. No significant differences were noticed between the Control-PEG, PEG10, and PEG25 treatments.
Comparison of modeled germination curves among Control-PEG, PEG10, and PEG25 treatments (Figure 3A) demonstrated a significant impact of the treatments (F = 29.99, P < 0.000001). Parameter “a” (referring to the cumulated number of germinated spores when the plateau phase was reached) did not differ between the control-PEG and the PEG10 and PEG25 treatments (Figure 3B). Parameter “b” (corresponding to the maximum spore germination rate) was significantly higher in the Control-PEG treatment as compared to the two other treatments that did not differ amongst them (Figure 3B). Parameter “c” (referring to the lag time) was significantly higher in the PEG25 treatment as compared to the Control-PEG and PEG10 treatments (Figure 3B). No differences were found between Control-PEG and PEG10 treatments (Figure 3B). The germ tube length of germinated spores under decreasing water availabilities, is presented in Table 1. The germ tube length, estimated at 110 days, was significantly lower in the PEG10, PEG25, and PEG50 treatments as compared to the Control-PEG treatment (Table 1). No significant differences were found between the three PEG treatments (Table 1).
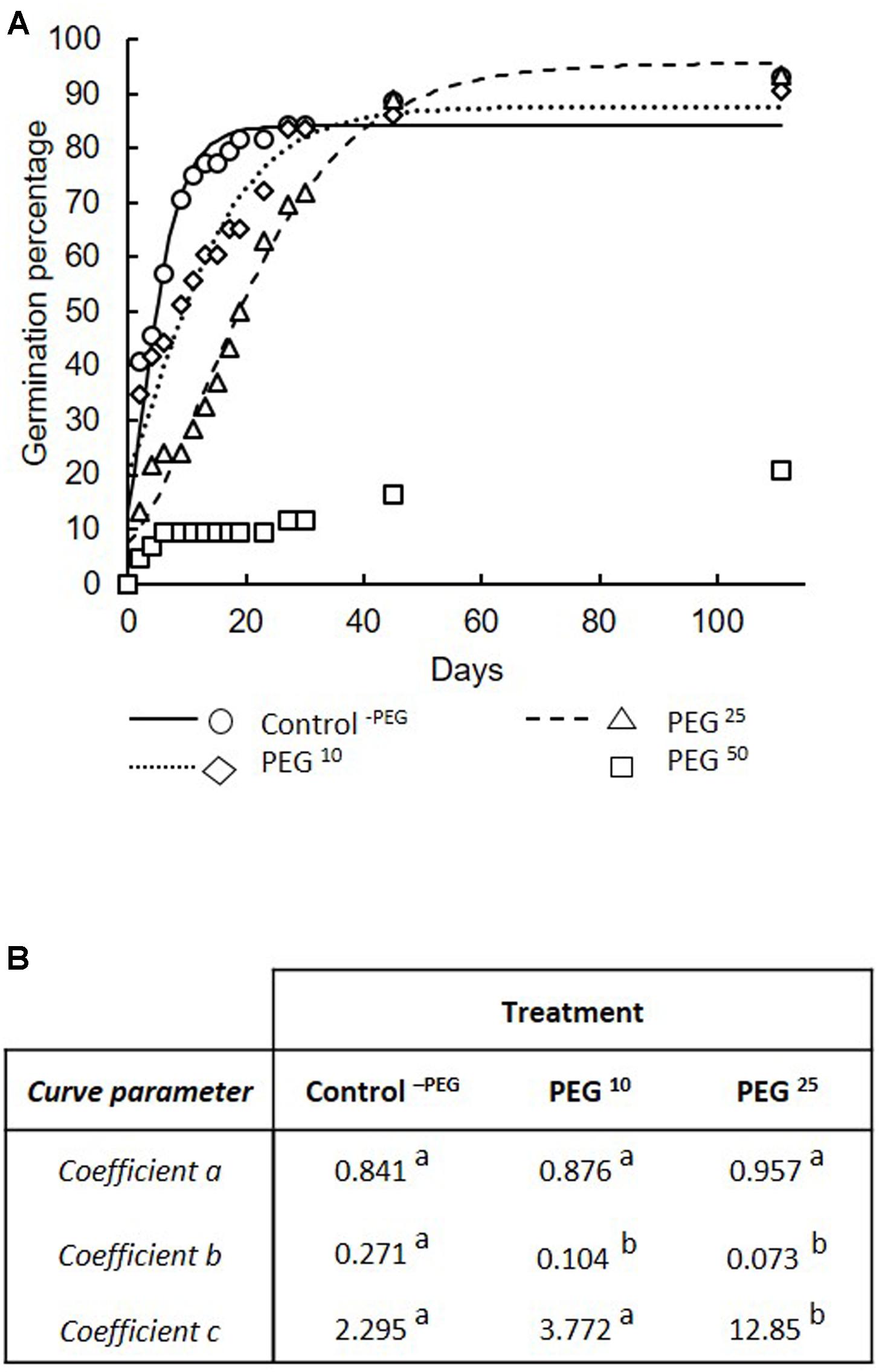
FIGURE 3. Germination percentage of spores of R. irregularis MUCL 41833. (A) in response to decreasing water availability. Treatments consisted in concentrations of 10, 25, and 50 g L-1 of PEG added to the medium (respectively, PEG10, PEG25, and PEG50) as well as a control without PEG (Control-PEG). Germination percentages were modeled using a non-linear regression and a 3-parameter Gompertz model following the equation:y = aexp (–e-b(x-e)). Observed percentages are represented by symbols whereas lines represent modelized curves under Control-PEG, PEG10, and PEG25 treatments. (B) Coefficients of the models are estimated in each treatment. Coefficient “a” referred to the cumulated number of germinated spores when the plateau phase was reached, “b” the maximum spore germination rate, and “c” the lag time. Different letters indicate significant differences among treatments using comparison of confidence intervals (see section “Materials and Methods” for further details).
Impact of Decreasing Water Availability on Pi Uptake by R. irregularis MUCL 41833, SDH Activity of Hyphae and Plant Parameters
Short-term dynamics of Pi uptake (throughout the text expressed as the % of Pi remaining in the medium, see definition in section “Materials and Method”) under decreasing water availabilities is reported in Figure 4. Whatever the treatment (Control-PEG, PEG10, PEG25, PEG50), the % of Pi remaining in the medium decreased over time suggesting an efficient uptake of Pi by the extraradical hyphae (Figure 4).
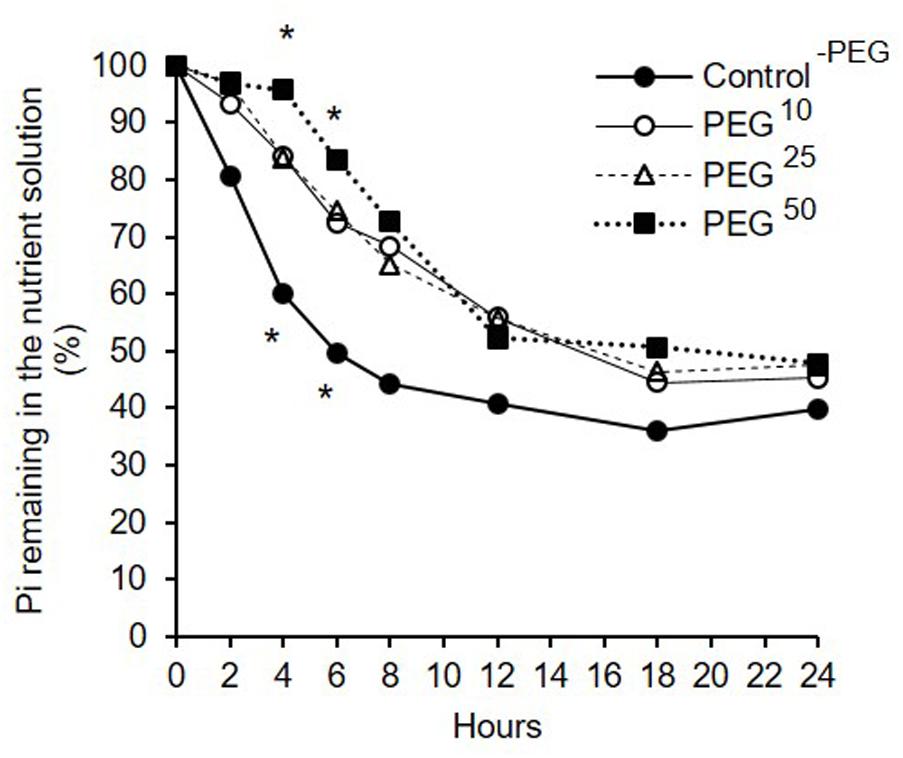
FIGURE 4. Percentage of inorganic phosphorus (Pi) remaining in the medium in presence of R. irregularis MUCL 41833 developing in the HC under decreasing water availabilities during 24 h. Treatments consisted in concentrations of 10, 25, and 50 g L-1 of PEG added to the medium (respectively, PEG10, PEG25, and PEG50) as well as a Control-PEG. Pi concentrations at each sampling time were standardized after Pi concentrations in the Control-AMF treatment and expressed in percentages relative to their T0 values (see section “Materials and Methods” for further details). Data are presented as means. At T4 and T6, stars above marks represent significant differences among treatments after ANOVA1 analysis followed by pair-wised comparisons (Tuckey’s test, P < 0.05, n = 6).
No significant differences in the % of Pi remaining in the medium at T24 was found among treatments. This result is consistent with total SDH activity measured at the end of the experiment (comprised between 72 and 89%) that revealed no significant differences among the treatments (data not shown, P = 0.9127).
Analysis of the % of Pi remaining in the medium with repeated measures over the time showed, however, a significant effect of the factor “treatment” (P < 0.0001) (Figure 4). Further analyses were thus conducted at each time point to compare % of Pi remaining in the medium.
At T4 and T6, the % of Pi remaining in the medium in the Control-PEG treatment was significantly lower as compared to the PEG50 treatment but did not differ with PEG10 and PEG25 treatments (Figure 4). No significant differences were found between PEG10, PEG25, and PEG50 treatments (Figure 4).
Finally, at T2, T8, T12, T18, and T24, no significant differences were noticed in the % of Pi remaining in the medium among treatments (respectively P = 0.1302, P = 0.0853, P = 0.5294, P = 0.4609, and P = 0.4699).
At the end of the experiment, no significant differences were noticed in shoot and roots dry weights (P = 0.1557 and P = 0.1224, respectively) and water content (P = 0.1281 and P = 0.119, respectively) (Table 2). P concentration in shoots differed significantly among treatments (P = 0.0049) (Table 2). P concentration in shoots was significantly higher in PEG50 as compared to Control-myco, Control-PEG and PEG10 treatments but did not differ from PEG25 treatment (Table 2). No differences were found between Control-myco, Control-PEG, PEG10 and PEG25 treatments (Table 2). No significant differences were found in roots P concentrations (P = 0.2944) and in shoots and root P contents (P = 0.5672 and P = 0.0681, respectively) (Table 2).
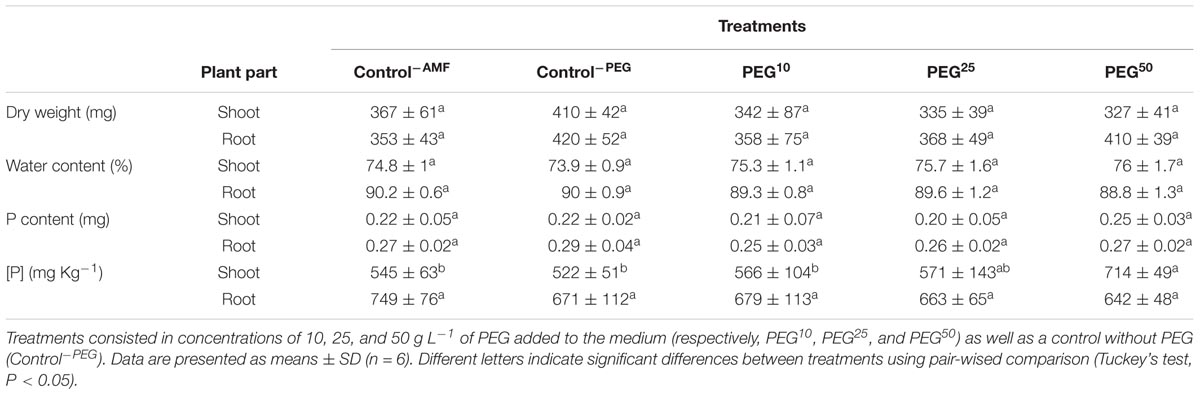
TABLE 2. Shoot and root dry weights, water content and phosphorus content and concentration [P] of M. truncatula plantlets associated to R. irregularis MUCL 41833 developing in a HC under decreased water availabilities during 24 h.
Discussion
Drought stress is an increasing problem in agriculture and methods to alleviate or at least decrease its impact on crops are highly demanded. Plant roots are intimately associated with microorganisms. Some of them (e.g., AMF) have been reported to increase the resistance/tolerance of plants to environmental stresses such as water deficit (Jayne and Quigley, 2014; Fernández-Lizarazo and Moreno-Fonseca, 2016). A few studies conducted within field also reported significant increase of plant biomass under dry environments (Caravaca et al., 2003; Querejeta et al., 2006; Pellegrino et al., 2011). This suggested that the utilization of AMF may potentially represent an innovative approach to address the issue of decreasing crop production under drought stress conditions. If the beneficial effects of AMF on plants under water stress have been reported (Jayne and Quigley, 2014; Fernández-Lizarazo and Moreno-Fonseca, 2016), limited knowledge is available on the impact of decreasing water availability on the development of AMF and their capacity to take up and transport Pi to plants. Here we reported on the impact of decreasing water availability on the development of R. irregularis MUCL 41833 and its capacity to take up and transport Pi under in vitro culture conditions. Water deficit was established via the application of increasing amounts (0, 10, 25, and 50 mg L-1) of PEG to the medium (corresponding to a decrease in water potential in a range from -0.024 to -0.056 Mpa). At the highest concentration of PEG and thus lowest availability of water, hyphal development, and spore production, as well as spore germination were decreased. Similarly, the dynamic of Pi depletion in the root-free HC was impacted at the highest concentration of PEG, even if total depletion was similar at the end of the experiment (i.e., after 24 h) suggesting an effective but slower dynamics of Pi uptake by the ERM.
Establishment of Water Availability Conditions to Study the Impact of PEG on R. irregularis MUCL 41833
Water potential measured in the MSR medium decreased inversely to PEG concentration and accordingly to previous results followed a quadratic model (Michel and Kaufmann, 1973). PEG significantly decreased the water potential of the MSR medium at the concentration of 50 g L-1. Conversely, 10 and 25 g L-1 did not significantly decrease the water potential as compared to the Control-PEG treatment. Water potentials considered in our experiment (from -0.024 to -0.056 Mpa) were above the limit of what is considered a drought stress environment (<-1 Mpa). However, it was sufficient to establish a gradient of water availability to the fungus impacting at the highest concentration development and to a lesser extend Pi uptake by the AMF. Higher concentrations of PEG in the MSR medium were also tested and decreased further the water potential (see Supplementary Figure S1). However, under these conditions, the MSR medium did not solidify completely, probably due to a decreased polymerization of the gelling agent (i.e., Gelrite) as earlier reported by van der Weele et al. (2000). An increased opacity of the medium was also noticed, preventing the easy and rigorous observation of the AMF.
Impact of Decreasing Water Availability on Fungal ERM Development
Hyphal length was significantly decreased in the PEG50 treatment and spore production in the PEG25 and PEG50 treatment as compared to the Control-PEG treatment. These results were consistent with the observations of Khalvati et al. (2005) in a pot experiment conducted in the greenhouse. These authors noticed that the extraradical hyphae density of G. intraradices was decreased under drought conditions. Conversely, Bethlenfalvay et al. (1988) and Davies et al. (1992) noticed an increase in hyphal length of Glomus mosseae and Glomus deserticola, respectively under greenhouse pot experiments. Similar contrasting results were reported for spores production. Drought drastically decreased their number in Gigaspora albida and Scutellospora heterogama (Silva et al., 2015), Entrophospora colombiana and Acaulospora longula, (Sieverding and Toro, 1988), while no effects were noticed on Gigaspora margarita, Glomus clarum, and G. mosseae (Sylvia and Schenck, 1983) under greenhouse pot experiments. Spore germination was also significantly decreased in the PEG50 treatment as compared to the other treatments and germ tube length was significantly decreased at all PEG concentrations as compared to the Control-PEG treatment. This corroborates the results of Sylvia and Schenck (1983) and Douds and Schenck (1991). These authors, respectively, reported a decrease in germination of G. intraradices, G. mosseae, and A. longula spores placed in soil substrate of water potentials equal or lower than -0.5 MPa and a decrease in germination of G. clarum, G. etunicatum, and G. macrocarpum spores placed in soil substrate of water potentials below -0.01 Mpa. Under in vitro conditions, Estaun (1989) observed a negative effect of glycerol-induced osmotic stress on germination and germ tube length of G. mosseae starting at a water potential of -0.21 Mpa.
Noticeably, these results clearly demonstrated that the impact of decreasing water availability can drastically differ between AMF species. Although water potentials in the above-mentioned studies were much lower than in our experiment, R. irregularis MUCL 41833 seemed to be very sensitive to water availability as noticed by the decreasing hyphal growth, spore production and spore germination obtained in the PEG50 treatment (corresponding to -0.056 Mpa). In previous greenhouse pot experiments the effects of drought on the decrease in spore production and extraradical development could not be strictly attributed to a direct impact on the fungus. Indeed, it was not possible to separate fungus from plant and thus direct from indirect effect on the fungus. Nevertheless, the authors suggested that the effects were due to a decrease of the carbon sink constituted by the plants (Augé, 2001; Manoharan et al., 2010; Silva et al., 2015). In our study conducted strictly in vitro, thus avoiding any confounding effects, only the ERM of the fungus was exposed to a decrease in water availability. The results clearly demonstrated that R. irregularis was directly impacted by a decrease in water availability. The effects observed were thus only attributable to the water potential and the resulting osmotic stress.
Impact of Decreasing Water Availability on Pi Uptake and Transport by the AMF
One of the most noticeable attribute of AMF is the ability of the fungus to take up and transport Pi to its host. The in vitro culture system used in this study was particularly adequate to investigate Pi uptake by the AMF as earlier demonstrated (Nielsen et al., 2002; Zocco et al., 2011; Calonne et al., 2014) but was never applied under conditions of decreased water availability. Under PEG-induced decrease in water availability, Pi uptake by the fungus was significantly impacted at the lowest water availability (PEG50), while no impact was noticed for PEG10 and PEG25. Indeed, within the first 6 h, the % of Pi remaining in the medium was lower in the HC of the Control-PEG treatment as compared to the PEG50 treatment indicating a probable effect of lower water availability on the Pi uptake and possibly on the Pi translocation to the plant. Interestingly, at the end of the experiment, no differences were noticed in % of Pi remaining in the medium whatever the water availability. Since SDH activity of the mycelium was similar between the different treatments, the decrease in Pi uptake by the fungus within the first hours cannot be clearly attributed to the death of a substantial proportion of hyphae. We hypothesized that the decreased rate of Pi uptake by the fungus could be related with a lower diffusion of Pi due to the viscosity of PEG solution or to an impact on the Pi transporters in the hyphae. It was, indeed, reported that under lower phosphate concentrations in the environment, the expression of the fungal Pi transporter GintPT was decreased (Maldonado-Mendoza et al., 2001). PEG could decrease water mass-flow and Pi diffusion to the hyphae causing GintPT to decrease its expression. The lower Pi uptake may be accompanied by a slower translocation within the hyphae, although this needs to be demonstrated.
Effect of Decreasing Water Availability on Plant Parameters of M. truncatula
No significant difference in shoots and roots P content was found in the absence (Control-AMF) or in the presence (Control-PEG, PEG10, PEG25, and PEG50 treatment) of mycelium in the HC. These results were expected as no differences in the Pi uptake by the mycelium could be detected among treatments after 24 h. Furthermore, it is unlikely that Pi uptake by the hyphae could directly impact P content in plants. Indeed, Pi quantities taken up by the hyphae are very small compared to the whole plant P content. Moreover, the short time of experiment might not be sufficient to observe a complete translocation of Pi to the plant. Indeed, it has been demonstrated that Pi accumulated in symbiotic structures in the RC only after 37 h (Nielsen et al., 2002).
Surprisingly, we found that P concentrations in shoots were significantly increased under PEG50 treatment compared to the Control-AMF (×1.32), Control-PEG (×1.37), and PEG10 (×1.26), treatments. Shoot P content was expected to follow the same trend given that shoot biomass was not found significantly different among treatments. However, shoot P content did not differ significantly among treatment, probably because of the impact of high standard deviations. As no difference was found in root P concentration among treatments, increased P concentration in shoots under the PEG50 treatment might be due to a higher root to shoot P translocation compared to the other treatments. In a similar experiment (Gil-Cardeza et al., 2017), an increased translocation of P from root to the shoot was also observed. Pi uptake by the mycelium was monitored in response to formaldehyde applied in the HC. After 24 h, P concentration in shoots of M. truncatula was significantly increased (×1.9) as compared to the treatment without stress. This effect was explained by a plant response to the high stress applied on the mycelium in the HC. Although the role of AM symbiosis in the plant P translocation is still barely known, some studies point the interaction of AM symbiosis long distance signals with the plant P homeostasis regulation. Analysis of miRNA expression pattern in mycorrhized M. truncatula plants under low phosphate conditions showed that AMF could increase the expression of miR399 (Branscheid et al., 2010), a P starvation signal involved in the regulation of root-shoot P compartmentation in the Arabidopsis thaliana model (Doerner et al., 2008). Moreover, AM symbiosis can up or down regulate the expression of numerous Pht1-type P transporter in plants and are believed to play a possible role in P translocation (Javot et al., 2007).
It was demonstrated that extraradical hyphae could directly transfer soil water to the plant and improve plant water status (Khalvati et al., 2005). However, the presence of ERM in the HC seemed not to improve water status of the plant as no significant increase in shoots and roots water content of M. truncatula were found. Similarly, no effect was found by adding PEG while Li et al. (2013) demonstrated that PEG addition in the HC enhanced expression of the fungal aquaporin genes GintAQPF1 and GintAQPF2 of R. irregularis and may increase water absorption by the hyphae. The absence of effect of the treatments on water content, in shoots and roots of M. truncatula in our experiment was expected because of the relative short time application of stress (24 h). Another explanation is that PEG concentrations tested were not as severe as water potential level found under a drought environment.
Conclusion
The present study cannot be compared per se with drought conditions in natural environments as the water potentials tested in the in vitro system were not low enough to induce drought stress in soil. However, the in vitro culture system was adequate to investigate the impact of a gradient of water availability on various fungal parameters and function (i.e., Pi uptake and transport) under strict controlled conditions. PEG-induced decrease in water availability impacted hyphal development and spore production, spore germination and Pi uptake dynamics at the highest concentration suggesting a probable higher effect under even more limiting water conditions. The in vitro system used appeared adequate to investigate various parameters of the AMF. It opens the door for comparative studies including AMF strains isolated from drought environments as well as for molecular studies involving expression of aquaporin genes or genes involved in stress tolerance.
Author Contributions
OLP contributed to the development of experiments, data collection, analysis, and interpretation of the data, drafted the work, and gave the final approval and agreed with all aspects of the work. SD made substantial contributions to the conception and design of the experiments, interpretation of the data, draft corrections, and gave the final approval and agreed with all aspects of the work.
Funding
This research was funded by the Prix Pierre et Colette Bauchau 2013.
Conflict of Interest Statement
The authors declare that the research was conducted in the absence of any commercial or financial relationships that could be construed as a potential conflict of interest.
Acknowledgments
The authors thank the “Support en Méthodologie et Calcul Statistique” (especially Catherine Rasse for her advice in section “Statistical Analysis”) and the “Groupe de Recherche en Physiologie Végétale” (for providing some analytical instruments – i.e., the osmometer) of the Université catholique de Louvain. A special thank is also addressed to François Ferrais for technical assistance.
Supplementary Material
The Supplementary Material for this article can be found online at: https://www.frontiersin.org/articles/10.3389/fmicb.2018.01254/full#supplementary-material
FIGURE S1 | Water potential (Ψ) response of the Modified Strullu–Romand (MSR) medium supplemented with sugar (3 g L-1) and increasing concentrations of Polyethylene glycol 8000 (0, 50, 75, 130, and 200 g L-1). Data are represented as means ± SD (n = 3).
Footnotes
References
Abdel Hamed Abdel Latef, A., Hashem, A., Rasool, S., Fathi Abd, E., Egamberdieva, D., Jan, S., et al. (2016). Arbuscular mycorrhizal symbiosis and abiotic stress in plants: a review. J. Plant Biol. 59, 407–426. doi: 10.1007/s12374-016-0237-7
Ahmad Khan, I., Ahmad, S., and Mirza, S. (2003). Yield and water use efficiency (WUE) of Avena sativa as influenced by vesicular arbuscular mycorrhizae (VAM). Asian J. Plant Sci. 4, 371–373.
Aroca, R., Porcel, R., and Ruiz-Lozano, J. M. (2007). How does arbuscular mycorrhizal symbiosis regulate root hydraulic properties and plasma membrane aquaporins in Phaseolus vulgaris under drought, cold or salinity stresses? New Phytol. 173, 808–816. doi: 10.1111/j.1469-8137.2006.01961.x
Aroca, R., and Ruiz-Lozano, J. M. (2009). Climate Change, Intercropping, Pest Control and Beneficial Microorganisms, ed. E. Lichtfouse (Dordrecht: Springer), doi: 10.1007/978-90-481-2716-0
Augé, R. M. (2001). Water relations, drought and vesicular-arbuscular mycorrhizal symbiosis. Mycorrhiza 11, 3–42. doi: 10.1007/s005720100097
Bago, B., Azcon-Aguilar, C., and Piche, Y. (1998). Architecture and developmental dynamics of the external mycelium of the arbuscular mycorrhizal fungus Glomus intraradices grown under monoxenic conditions. Mycologia 90, 52–62. doi: 10.2307/3761011
Bago, B., Vierheilig, H., Piche, Y., and Azcon-Aguilar, C. (1996). Nitrate depletion and pH changes induced by the extraradical mycelium of the arbuscular mycorrhizal fungus Glomus intraradices grown in monoxenic culture. New Phytol. 133, 273–280. doi: 10.1111/j.1469-8137.1996.tb01894.x
Bárzana, G., Aroca, R., Paz, J. A., Chaumont, F., Martinez-Ballesta, M. C., Carvajal, M., et al. (2012). Arbuscular mycorrhizal symbiosis increases relative apoplastic water flow in roots of the host plant under both well-watered and drought stress conditions. Ann. Bot. 109, 1009–1017. doi: 10.1093/aob/mcs007
Bethlenfalvay, G. J., Brown, M. S., Ames, R. N., and Thomas, R. S. (1988). Effects of drought on host and endophyte development in mycorrhizal soybeans in relation to water use and phosphate uptake. Physiol. Plant. 72, 565–571. doi: 10.1111/j.1399-3054.1988.tb09166.x
Branscheid, A., Sieh, D., Pant, B. D., May, P., Devers, E. A., Elkrog, A., et al. (2010). Expression pattern suggests a role of MiR399 in the regulation of the cellular response to local Pi increase during arbuscular mycorrhizal symbiosis. Mol. Plant Microbe Interact. 23, 915–926. doi: 10.1094/MPMI-23-7-0915
Brundrett, M. C. (2009). Mycorrhizal associations and other means of nutrition of vascular plants: understanding the global diversity of host plants by resolving conflicting information and developing reliable means of diagnosis. Plant Soil 320, 37–77. doi: 10.1007/s11104-008-9877-9
Calonne, M., Fontaine, J., Tisserant, B., Dupré de Boulois, H., Grandmougin-Ferjani, A., Declerck, S., et al. (2014). Polyaromatic hydrocarbons impair phosphorus transport by the arbuscular mycorrhizal fungus Rhizophagus irregularis. Chemosphere 104, 97–104. doi: 10.1016/j.chemosphere.2013.10.070
Calonne, M., Sahraoui, A. L., Campagnac, E., Debiane, D., Laruelle, F., Grandmougin-Ferjani, A., et al. (2012). Propiconazole inhibits the sterol 14α-demethylase in Glomus irregulare like in phytopathogenic fungi. Chemosphere 87, 376–383. doi: 10.1016/j.chemosphere.2011.12.027
Caravaca, F., Barea, J. M., Palenzuela, J., Figueroa, D., Alguacil, M. M., and Roldán, A. (2003). Establishment of shrub species in a degraded semiarid site after inoculation with native or allochthonous arbuscular mycorrhizal fungi. Appl. Soil Ecol. 22, 103–111. doi: 10.1016/S0929-1393(02)00136-1
Cardoso, I. M., and Kuyper, T. W. (2006). Mycorrhizas and tropical soil fertility. Agric. Ecosyst. Environ. 116, 72–84. doi: 10.1016/j.agee.2006.03.011
Davies, F. T., Potter, J. R., and Linderman, R. G. (1992). Mycorrhiza and repeated drought exposure affect drought resistance and extraradical hyphae development of pepper plants independent of plant size and nutrient content. J. Plant Physiol. 139, 289–294. doi: 10.1016/S0176-1617(11)80339-1
de la Providencia, I. E., de Souza, F. A., Fernández, F., Delmas, N. S., and Declerk, S. (2005). Arbuscular mycorrhizal fungi reveal distinct patterns of anastomosis and hyphal formation mechanisms healing between different phylogenic groups. New Phytol. 165, 261–271. doi: 10.1111/j.1469-8137.2004.01236.x
Declerck, S., De Boulois, H. D., Bivort, C., and Delvaux, B. (2003). Extraradical mycelium of the arbuscular mycorrhizal fungus Glomus lamellosum can take up, accumulate and translocate radiocaesium under root-organ culture conditions. Environ. Microbiol. 5, 510–516. doi: 10.1046/j.1462-2920.2003.00445.x
Declerck, S., D’or, D., Cranenbrouck, S., and Le Boulengé, E. (2001). Modelling the sporulation dynamics of arbuscular mycorrhizal fungi in monoxenic culture. Mycorrhiza 11, 225–230. doi: 10.1007/s005720100124
Declerck, S., Strullu, D.-G., and Fortin, J.-A. (2005). Vitro Culture of Mycorrhizas, eds D. S. Strullu and J. André Fortin (Berlin: Springer). doi: 10.1007/b138925
Declerck, S., Strullu, D. G., and Plenchette, C. (1998). Monoxenic culture of the intraradical forms of Glomus sp. isolated from a tropical ecosystem: a proposed methodology for germplasm collection. Mycologia 90, 579–585. doi: 10.2307/3761216
Doerner, P., Reed, J., and Bartel, B. (2008). Phosphate starvation signaling: a threesome controls systemic Pi homeostasis This review comes from a themed issue on cell signalling and gene regulation Edited. Curr. Opin. Plant Biol. 11, 536–540. doi: 10.1016/j.pbi.2008.05.006
Doner, L. W., and Becard, G. (1991). Solubilization of gellan gels by chelation of cations. Biotechnol. Tech. 5, 25–28. doi: 10.1007/BF00152749
Douds, D. D., and Schenck, N. C. (1991). Germination and hyphal growth of vam fungi during and after storage in soil at five matric potentials. Soil Biol. Biochem. 23, 177–183. doi: 10.1016/0038-0717(91)90132-4
Elliott, J., Deryng, D., Müller, C., Frieler, K., Konzmann, M., Gerten, D., et al. (2014). Constraints and potentials of future irrigation water availability on agricultural production under climate change. Proc. Natl. Acad. Sci. U.S.A. 111, 3239–3244. doi: 10.1073/pnas.1222474110
Estaun, M. V. (1989). Effect of sodium chloride and mannitol on germination and hyphal growth of the vesicular-arbuscular mycorrhizal fungus Glomus mossae. Agric. Ecosyst. Environ. 29, 123–129. doi: 10.1016/0167-8809(90)90266-G
Farooq, M., Gogoi, N., Barthakur, S., Baroowa, B., Bharadwaj, N., Alghamdi, S. S., et al. (2017). Drought stress in grain legumes during reproduction and grain filling. J. Agron. Crop Sci. 203, 81–102. doi: 10.1111/jac.12169
Fernández-Lizarazo, J. C., and Moreno-Fonseca, L. P. (2016). Mechanisms for tolerance to water-deficit stress in plants inoculated with arbuscular mycorrhizal fungi. A review. Agron. Colomb. 34, 179–189. doi: 10.15446/agron.colomb.v34n2.55569
Fitter, A. H., and Nichols, R. (1988). The use of benomyl to control infection by vesicular-arbuscular mycorrhizal fungi. New Phytol. 110, 201–206. doi: 10.1111/j.1469-8137.1988.tb00253.x
Garcés-Ruiz, M., Calonne-Salmon, M., Plouznikoff, K., Misson, C., Navarrete-Mier, M., Cranenbrouck, S., et al. (2017). Dynamics of short-term phosphorus uptake by intact mycorrhizal and non-mycorrhizal maize plants grown in a circulatory semi-hydroponic cultivation system. Front. Plant Sci. 8:1471. doi: 10.3389/fpls.2017.01471
Gil-Cardeza, M. L., Calonne-Salmon, M., Gómez, E., and Declerck, S. (2017). Short-term chromium (VI) exposure increases phosphorus uptake by the extraradical mycelium of the arbuscular mycorrhizal fungus Rhizophagus irregularis MUCL 41833. Chemosphere 187, 27–34. doi: 10.1016/j.chemosphere.2017.08.079
Goicoechea, N., Antolin, M. C., and Sanchez-Diaz, M. (1997). Gas exchange is related to the hormone balance in mycorrhizal or nitrogen-fixing alfalfa subjected to drought. Physiol. Plant. 100, 989–997. doi: 10.1111/j.1399-3054.1997.tb00027.x
Hammer, E. C., and Rillig, M. C. (2011). The influence of different stresses on glomalin levels in an arbuscular mycorrhizal fungus-salinity increases glomalin content. PLoS One 6:e2846. doi: 10.1371/journal.pone.0028426
Jahromi, F., Aroca, R., Porcel, R., and Ruiz-Lozano, J. M. (2008). Influence of salinity on the in vitro development of Glomus intraradices and on the in vivo physiological and molecular responses of mycorrhizal lettuce plants. Microb. Ecol. 55, 45–53. doi: 10.1007/s00248-007-9249-7
Jansa, J., Mozafar, A., and Frossard, E. (2003). Long-distance transport of P and Zn through the hyphae of an arbuscular mycorrhizal fungus in symbiosis with maize. Agronomie 23, 481–488. doi: 10.1051/agro:2003013
Javot, H., Pumplin, N., and Harrison, M. J. (2007). Phosphate in the arbuscular mycorrhizal symbiosis: transport properties and regulatory roles. Plant Cell Environ. 30, 310–322. doi: 10.1111/j.1365-3040.2006.01617.x
Jayne, B., and Quigley, M. (2014). Influence of arbuscular mycorrhiza on growth and reproductive response of plants under water deficit: a meta-analysis. Mycorrhiza 24, 109–119. doi: 10.1007/s00572-013-0515-x
Khalvati, M. A., Hu, Y., Mozafar, A., and Schmidhalter, U. (2005). Quantification of water uptake by arbuscular mycorrhizal hyphae and its significance for leaf growth, water relations, and gas exchange of barley subjected to drought stress. Plant Biol. 7, 706–712. doi: 10.1055/s-2005-872893
Kim, Y.-C., Glick, B. R., Bashan, Y., and Ryu, C.-M. (2012). “Enhancement of plant drought tolerance by microbes,” in Plant Responses to Drought Stress, ed. R. Aroca (Berlin: Springer), 383–413. doi: 10.1007/978-3-642-32653-0_15
Lawlor, D. W. (1970). Absorption of polyethylene glycols by plants and their effects on plant growth. New Phytol. 69, 501–513. doi: 10.1111/j.1469-8137.1970.tb02446.x
Lenoir, I., Fontaine, J., and Lounès-Hadj Sahraoui, A. (2016). Arbuscular mycorrhizal fungal responses to abiotic stresses: a review. Phytochemistry 123, 4–15. doi: 10.1016/j.phytochem.2016.01.002
Lesk, C., Rowhani, P., and Ramankutty, N. (2016). Influence of extreme weather disasters on global crop production. Nature 529, 84–87. doi: 10.1038/nature16467
Li, T., Hu, Y., Hao, Z., Li, H., and Chen, B. (2013). Aquaporin genes GintAQPF1 and GintAQPF2 from Glomus intraradices contribute to plant drought tolerance. Plant Signal. Behav. 8:e24030. doi: 10.4161/psb.24030
Maldonado-Mendoza, I. E., Dewbre, G. R., and Harrison, M. J. (2001). A phosphate transporter gene from the extraradical mycelium of an arbuscular mycorrhizal fungus Glomus intraradices is regulated in response to phosphate in the environment. Mol. Plant Microbe Interact. 14, 1140–1148. doi: 10.1094/MPMI.2001.14.10.1140
Manoharan, P. T., Shanmugaiah, V., Balasubramanian, N., Gomathinayagam, S., Sharma, M. P., and Muthuchelian, K. (2010). Influence of AM fungi on the growth and physiological status of Erythrina variegata Linn. grown under different water stress conditions. Eur. J. Soil Biol. 46, 151–156. doi: 10.1016/j.ejsobi.2010.01.001
Marsh, B. A. B. (1971). Measurement of length in random arrangements of lines. J. Appl. Ecol. 8, 265–267. doi: 10.2307/2402144
Merrild, M. P., Ambus, P., Rosendahl, S., and Jakobsen, I. (2013). Common arbuscular mycorrhizal networks amplify competition for phosphorus between seedlings and established plants. New Phytol. 200, 229–240. doi: 10.1111/nph.12351
Mexal, J., and Reid, C. P. P. (1973). The growth of selected mycorrhizal fungi in response to induced water stress. Can. J. Bot. 51, 1579–1588. doi: 10.1139/b73-201
Michel, B. E., and Kaufmann, M. R. (1973). The osmotic potential of polyethylene glycol 6000. Plant Physiol. 51, 914–916. doi: 10.1104/pp.51.5.914
Miransari, M. (2014). Use of Microbes for the Alleviation of Soil Stresses, Vol. 1, ed. M. Miransari (New York, NY: Springer), doi: 10.1007/978-1-4614-9466-9
Nelsen, C. E., and Safir, G. R. (1982). Increased drought tolerance of mycorrhizal onion plants caused by improved phosphorus nutrition. Planta 154, 407–413. doi: 10.1007/BF01267807
Nielsen, J. S., Joner, E. J., Declerck, S., Olsson, S., Jakobsen, I., and Jakobsen, I. (2002). Phospho-imaging as a tool for visualization and noninvasive measurement of P transport dynamics in arbuscular mycorrhizas. New Phytol. 154, 809–819. doi: 10.1046/j.1469-8137.2002.00412.x
Parniske, M. (2008). Arbuscular mycorrhiza: the mother of plant root endosymbioses. Nat. Rev. Microbiol. 6, 763–775. doi: 10.1038/nrmicro1987
Pawlowska, T. E., and Charvat, I. (2004). Heavy-metal stress and developmental patterns of arbuscular mycorrhizal fungi. Appl. Environ. Microbiol. 70, 6643–6649. doi: 10.1128/AEM.70.11.6643
Pellegrino, E., Bedini, S., Avio, L., Bonari, E., and Giovannetti, M. (2011). Field inoculation effectiveness of native and exotic arbuscular mycorrhizal fungi in a Mediterranean agricultural soil. Soil Biol. Biochem. 43, 367–376. doi: 10.1016/j.soilbio.2010.11.002
Plouznikoff, K., Declerck, S., and Calonne-Salmon, M. (2016). “Mitigating abiotic stresses in crop plants by arbuscular mycorrhizal fungi,” in Belowground Defence Strategies in Plants, Signaling and Communication in Plants, eds C. M. Vos and K. Kazan (Berlin: Springer), 341–400. doi: 10.1007/978-3-319-42319-7_15
Porcel, R., and Ruiz-Lozano, J. M. (2004). Arbuscular mycorrhizal influence on leaf water potential, solute accumulation, and oxidative stress in soybean plants subjected to drought stress. J. Exp. Bot. 55, 1743–1750. doi: 10.1093/jxb/erh188
Querejeta, J. I., Allen, M. F., Caravaca, F., and Roldán, A. (2006). Differential modulation of host plant delta 13C and delta 18O by native and nonnative arbuscular mycorrhizal fungi in a semiarid environment. New Phytol. 169, 379–387. doi: 10.1111/j.1469-8137.2005.01599.x
Rai, M. K., Kalia, R. K., Singh, R., Gangola, M. P., and Dhawan, A. K. (2011). Developing stress tolerant plants through in vitro selection-An overview of the recent progress. Environ. Exp. Bot. 71, 89–98. doi: 10.1016/j.envexpbot.2010.10.021
Rodriguez, A., and Sanders, I. R. (2014). The role of community and population ecology in applying mycorrhizal fungi for improved food security. ISME J. 9, 1053–1061. doi: 10.1038/ismej.2014.207
Ruiz-Lozano, J. M., del Mar Alguacil, M., Bárzana, G., Vernieri, P., and Aroca, R. (2009). Exogenous ABA accentuates the differences in root hydraulic properties between mycorrhizal and non mycorrhizal maize plants through regulation of PIP aquaporins. Plant Mol. Biol. 70, 565–579. doi: 10.1007/s11103-009-9492-z
Saito, M. (1995). Enzyme activities of the internal hyphae and germinated spores of an arbuscular mycorrhizal fungus. Gigaspora margarita Becker & Hall. New Phytol. 129, 425–431. doi: 10.1111/j.1469-8137.1995.tb04313.x
Sánchez-Díaz, M., and Honrubia, M. (1994). “Water relations and alleviation of drought stress in mycorrhizal plants,” in Impact of Arbuscular Mycorrhizas on Sustainable Agriculture and Natural Ecosystems, eds S. Gianinazzi and H. Schiiepp (Basel: Birkhäuser Basel), doi: 10.1007/978-3-0348-8504-1_13
Seber, G. A. F., and Wild, C. J. (1989). Nonlinear Regression. Hoboken, NJ: John Wiley & Sons, Inc. doi: 10.1002/0471725315
Sieverding, E., and Toro, T. S. (1988). Influence of soil water regimes on VA mycorrhiza. J. Agron. Crop Sci. 161, 322–332. doi: 10.1111/j.1439-037X.1988.tb00676.x
Silva, E. M., Maia, L. C., Menezes, K. M. S., Braga, M. B., Melo, N. F., and Yano-Melo, A. M. (2015). Water availability and formation of propagules of arbuscular mycorrhizal fungi associated with sorghum. Appl. Soil Ecol. 94, 15–20. doi: 10.1016/j.apsoil.2015.05.004
St-Arnaud, M., Hamel, C., Vimard, B., Caron, M., and Fortin, J. A. (1996). Enhanced hyphal growth and spore production of the arbuscular mycorrhizal fungus Glomus intraradices in an in vitro system in the absence of host roots. Mycol. Res. 100, 328–332. doi: 10.1016/S0953-7562(96)80164-X
Sylvia, D. M., and Schenck, N. C. (1983). Germination of chlamydospores of three Glomus species as affected by soil matric potential and fungal contamination. Mycologia 75, 30–35. doi: 10.2307/3792920
Thingstrup, I., Kahiluoto, H., and Jakobsen, I. (2000). Phosphate transport by hyphae of field communities of arbuscular mycorrhizal fungi at two levels of P fertilization. Plant Soil 221, 181–187. doi: 10.1023/A:1004721626216
Van Aarle, I. M., Olsson, P. A., and Söderström, B. (2002). Arbuscular mycorrhizal fungi respond to the substrate pH of their extraradical mycelium by altered growth and root colonization. New Phytol. 155, 173–182. doi: 10.1046/j.1469-8137.2002.00439.x
van der Weele, C. M., Spollen, W. G., Sharp, R. E., and Baskin, T. I. (2000). Growth of Arabidopsis thaliana seedlings under water deficit studied by control of water potential in nutrient-agar media. J. Exp. Bot. 51, 1555–1562. doi: 10.1093/jexbot/51.350.1555
Vierheilig, H., Schweiger, P., and Brundrett, M. (2005). An overview of methods for the detection and observation of arbuscular mycorrhizal fungi in roots. Physiol. Plant. 125, 393–404. doi: 10.1111/j.1399-3054.2005.00564.x
Voets, L., De La Providencia, I. E., and Declerck, S. (2006). Glomeraceae and Gigasporaceae differ in their ability to form hyphal networks. New Phytol. 172, 185–188. doi: 10.1111/j.1469-8137.2006.01873.x
Voets, L., de la Providencia, I. E., Fernandez, K., Ijdo, M., Cranenbrouck, S., and Declerck, S. (2009). Extraradical mycelium network of arbuscular mycorrhizal fungi allows fast colonization of seedlings under in vitro conditions. Mycorrhiza 19, 347–356. doi: 10.1007/s00572-009-0233-6
Voets, L., Dupré de Boulois, H., Renard, L., Strullu, D.-G., and Declerck, S. (2005). Development of an autotrophic culture system for the in vitro mycorrhization of potato plantlets. FEMS Microbiol. Lett. 248, 111–118. doi: 10.1016/j.femsle.2005.05.025
Wezel, A., Casagrande, M., Celette, F., Vian, J. F., Ferrer, A., and Peigné, J. (2014). Agroecological practices for sustainable agriculture. A review. Agron. Sustain. Dev. 34, 1–20. doi: 10.1007/s13593-013-0180-7
Wheeler, T., and von Braun, J. (2013). Climate change impacts on global food security. Science 341, 508–513. doi: 10.1126/science.1239402
Wu, Q.-S., and Xia, R.-X. (2006). Arbuscular mycorrhizal fungi influence growth, osmotic adjustment and photosynthesis of citrus under well-watered and water stress conditions. J. Plant Physiol. 163, 417–425. doi: 10.1016/j.jplph.2005.04.024
Wu, Q.-S., Xia, R.-X., and Zou, Y.-N. (2008). Improved soil structure and citrus growth after inoculation with three arbuscular mycorrhizal fungi under drought stress. Eur. J. Soil Biol. 44, 122–128. doi: 10.1016/j.ejsobi.2007.10.001
Wu, Q.-S., and Zou, Y.-N. (2017). “Arbuscular mycorrhizal fungi and tolerance of drought stress in plants,” in Arbuscular Mycorrhizas and Stress Tolerance of Plants, ed. Q. S. Wu (Singapore: Springer), 25–41. doi: 10.1007/978-981-10-4115-0_2
Xie, H., You, L., Wielgosz, B., and Ringler, C. (2014). Estimating the potential for expanding smallholder irrigation in Sub-Saharan Africa. Agric. Water Manag. 131, 183–193. doi: 10.1016/j.agwat.2013.08.011
Keywords: arbuscular mycorrhizal fungi, polyethylene glycol (PEG), drought stress, hyphal development, phosphorus uptake, phosphorus transport
Citation: Le Pioufle O and Declerck S (2018) Reducing Water Availability Impacts the Development of the Arbuscular Mycorrhizal Fungus Rhizophagus irregularis MUCL 41833 and Its Ability to Take Up and Transport Phosphorus Under in Vitro Conditions. Front. Microbiol. 9:1254. doi: 10.3389/fmicb.2018.01254
Received: 17 February 2018; Accepted: 24 May 2018;
Published: 11 June 2018.
Edited by:
Mohamed Hijri, Université de Montréal, CanadaReviewed by:
Franck Stefani, Agriculture and Agri-Food Canada (AAFC), CanadaJan Jansa, Institute of Microbiology (AVCR), Czechia
Copyright © 2018 Le Pioufle and Declerck. This is an open-access article distributed under the terms of the Creative Commons Attribution License (CC BY). The use, distribution or reproduction in other forums is permitted, provided the original author(s) and the copyright owner are credited and that the original publication in this journal is cited, in accordance with accepted academic practice. No use, distribution or reproduction is permitted which does not comply with these terms.
*Correspondence: Stéphane Declerck, c3RlcGhhbi5kZWNsZXJja0B1Y2xvdXZhaW4uYmU=