- 1Division of Bioscience and Bioindustry, Graduate School of Technology, Industrial and Social Sciences, Tokushima University, Tokushima, Japan
- 2Department of Applied Biological Science, Faculty of Agriculture, Kagawa University, Kagawa, Japan
- 3Department of Biomedical Engineering, Faculty of Engineering, Osaka Institute of Technology, Osaka, Japan
We recently identified and characterized a novel broad substrate specificity amino acid racemase (BAR) from the hyperthermophilic archaeon Pyrococcus horikoshii OT-3. Three genes, PH0782, PH1423, and PH1501, encoding homologs exhibiting about 45% sequence identity with BAR were present in the P. horikoshii genome. In this study, we detected pyridoxal 5′-phosphate (PLP)-dependent amino acid racemase activity in the protein encoded by PH0782. The enzyme showed activity toward Ala, Ser, Thr, and Val, but the catalytic efficiency with Thr or Val was much lower than with Ala or Ser. The enzyme was therefore designated Ala/Ser racemase (ASR). Like BAR, ASR was highly stable at high temperatures and over a wide range of pHs, though its hexameric structure differed from the dimeric structure of BAR. No activity was detected in K291A or D234A ASR mutants. This suggests that, as in Ile 2-epimerase (ILEP) from Lactobacillus buchneri JCM1115, these residues are involved in Schiff base formation and substrate interaction, respectively. Unlike BAR, enhanced ASR activity was not detected in P. horikoshii cells cultivated in the presence of D-Ala or D-Ser. This is the first description of a PLP-dependent fold type I ASR in archaea.
Introduction
D-Amino acids play a variety of important roles in many organisms. In mammals, for example, D-Ser and D-Asp are known to be N-methyl-D-Asp (NMDA) receptor co-agonists (Errico et al., 2015), while D-Ala is a major osmolyte responsible for intracellular isosmotic regulation in the tissues of bivalve and kuruma prawn (Abe et al., 2005; Yoshikawa and Yokoyama, 2015). In bacteria, it is well known that D-Ala and D-Glu are components of the peptidoglycan cell wall (Hancock, 1960; Ghuysen, 1961), as are D-Ser and D-Asp (Reynolds and Courvalin, 2005; Veiga et al., 2006). Although free D-amino acids such as D-Asp, D-Ala, and D-Ser have also been detected in hyperthermophilic archaea (Matsumoto et al., 1999; Nagata et al., 1999; Long et al., 2001), the cell walls of archaea such as the Pyrococcus and Thermococcus species are S-layers, composed of hexagonally or tetragonally arranged proteins or glycoproteins and do not contain D-amino acids. The physiological function of D-amino acids in archaeal cells thus remains unclear.
Free D-amino acids in cells are generally produced by amino acid racemases, which catalyze pyridoxal 5′-phosphate (PLP)-dependent or PLP-independent racemization of amino acids. PLP-independent Asp racemases (AspRs) and PLP-dependent AlaR and SerR have been found in archaeal cells and characterized (Matsumoto et al., 1999; Long et al., 2001; Moore and Leigh, 2005; Ohnishi et al., 2008; Aihara et al., 2016; Washio et al., 2016). This includes detailed structural and functional characterization of a PLP-independent AspR from the hyperthermophilic archaeon Pyrococcus horikoshii OT-3 (Liu et al., 2001, 2002a,b; Yoshida et al., 2006; Ohtaki et al., 2008; Kita et al., 2009). We recently detected novel PLP-dependent amino acid racemase activity toward Met, Leu, and Phe in the crude extract of P. horikoshii, identified the enzyme gene (ORF ID: PH0138), and determined the properties of the recombinant protein (Kawakami et al., 2015, 2017). That enzyme showed activity toward 10 amino acids and was therefore named BAR.
This enzyme was originally annotated as a GABA-AT in the genome database, and its primary structure is consistent with a fold-type I PLP-dependent enzyme. Three other genes within the P. horikoshii genome, PH0782, PH1423, and PH1501, encode proteins that are also annotated as GABA-ATs and exhibit high similarity to BAR. This suggests these homologs also function as amino acid racemases. We previously reported that BAR activity is markedly increased in P. horikoshii cells grown on medium supplemented with D-allo-Ile, which suggests BAR mediates utilization of D-amino acids for growth (Kawakami et al., 2015). In the present study, we constructed expression systems for BAR homologs using pET vector and detected amino acid racemase activity toward Ala and Ser in the recombinant PH0782 enzyme. We then characterized the enzyme and identified the residues responsible for its catalytic activity. To better understand the physiological function of this enzyme, we examined the level of AlaR activity in P. horikoshii cells under various growth conditions.
Materials and Methods
Materials
o-Phthalaldehyde and N-tert-butyloxycarbonyl-L-cysteine were from Wako (Osaka, Japan) and Sigma–Aldrich (Tokyo, Japan), respectively. D- and L-amino acids were from Wako and Tokyo Chemical Industry (Tokyo). All other chemicals were of reagent grade.
Construction of Expression Plasmids for BAR Homologs
In this study, we constructed expression plasmids for PH0782, PH1423, and PH1501 genes using a pET system. The three genes were amplified using PCR with P. horikoshii genomic DNA as the template. The primer sets used in the PCR are listed in Supplementary Table S1. Forward and reverse primers introduced an NdeI site overlapping the 5′ initiation codon and a BglII (for PH0782) or BamHI (for PH1423 and PH1501) site proximal to the 3′ end of the termination codon. PCR reactions were run using PrimeStar Max DNA polymerase (Takara, Tokyo) according to the manufacturer’s instructions. Amplified fragments were purified, introduced into pCR4-TOPO (Invitrogen, Tokyo) and sequenced. The resultant TOPO/PH0782, TOPO/PH1423 and TOPO/PH1501 were digested with NdeI and BamHI (BglII for PH0782) and introduced into pET11a (Novagen, Tokyo) to generate pET11a/PH0782, pET11a/PH1423, and pET11a/PH1501, respectively.
For construction of the K291A and D234A mutants, two sets of primers were designed (Supplementary Table S1) and pET11a/PH0782 was used as the template. The non-PCR reaction was run with PrimeStar Max DNA polymerase (Takara, Tokyo) using the standard protocol supplied by the manufacturer. The restriction enzyme DpnI was added to the reaction mixture to digest the template DNA. An aliquot of the reaction mixture was then used to transform TOP 10 cells (Stratagene, Tokyo). To screen for the correct mutation, the plasmids were extracted from the transformants and whole gene sequencing was conducted using a genetic analyzer (Model 3130, Applied Biosystems, Tokyo).
Expression and Purification of Recombinant Enzymes
The procedures used to express and purify PH0782 were similar to those used for BAR (Kawakami et al., 2017), except that Escherichia coli BL21 (DE3) cells (Stratagene) were used as the competent cells. For analysis of the mutant enzymes and substrate screening, enzymes partially purified through heat treatment at 90°C for 20 min were used.
Protein concentrations were determined using the Bradford method (Bradford, 1976). Bovine serum albumin served as the standard.
Subunit and Native Molecular Mass Determination
SDS–PAGE (Laemmli, 1970) was used to determine the subunit molecular mass of the enzymes. Myosin (200 kDa), β-galactosidase (116.3 kDa), phosphorylase B (97.4 kDa), serum albumin (66.2 kDa), ovalbumin (45 kDa), carbonic anhydrase (31 kDa), trypsin inhibitor (21.5 kDa), lysozyme (14.4 kDa), and aprotinin (6.5 kDa) were used as molecular mass standards (Bio-Rad, Tokyo). The native molecular mass of PH0782 was determined using gel filtration chromatography with a HiLoad 26/60 Superdex 200 column (GE Healthcare, Tokyo). Thyroglobulin (670 kDa), γ-globulin (158 kDa), Ovalbumin (44 kDa), and Myoglobin (17 kDa) were used as molecular mass standards (Bio-Rad).
Separation and Determination of DL-Amino Acids Using UPLC
UPLC analyses were performed for quantitative determination of L- or D-amino acids produced in the enzyme assays used for substrate screening, assessment of pH dependency, and kinetic analysis. For the enzyme assays, the standard reaction mixture included 100 mM HEPES (pH 7.0), 10 mM L-amino acid, 0.04 mM PLP, and 1 μg of enzyme in a 100-μL volume, which was incubated at 80°C for 30 min. After incubation, the reaction mixture was immediately cooled, 6% trichloroacetic acid was added, and the precipitate was removed by centrifugation. The supernatant was then neutralized using NaOH, and the amino acids in the mixture were derivatized with OPA and NBC. In the screening assays, the derivatized amino acids were analyzed using an X-pressPak V-C18 column (2.0 mm by 50 mm, Jasco, Tokyo) as described previously (Kawakami et al., 2017). For simultaneous kinetics analysis using Ala, Ser, Val, and Thr as substrates, citrate solutions (5 mM, pH5.8) in 15 and 60% ethanol were used as buffers A and B, respectively, and the gradient program was operated as follows: 0–20% B for 4.5 min, 20–40% B for 2.5 min, 40–70% B for 1.0 min, 70% B for 0.5 min, and 70–0% B for 0.5 min.
Characterization of Enzymes
For substrate screening, 10 μg of each enzyme were added to the reaction mixture and incubated for 60 min. Ala, Val, Leu, Ile, Phe, Met, Trp, Tyr, Ser, Thr, Asn, Gln, and Arg were used as substrates. To determine pH dependency, L-Ala was used as the substrate and the activity was assayed under various buffer conditions (acetate [pH 5.5–6.5], MES [pH 5.5–7.0], phosphate [pH 6.0–8.0], HEPES [pH 6.5–8.5], and CHES [pH 8.5–9.5]; buffer pH was adjusted at 25°C). To determine the kinetic parameters, racemase activity was assayed in the presence of 1–20 mM Ala or Ser (n = 3). Reaction rates were independently calculated, and apparent Vmax and Km values were analyzed with Prism 5.0 (GraphPad Software) using a non-linear regression model.
To assess the pH stability of PH0782, the enzyme (0.1 mg/ml) was incubated at 80°C for 2 h in several buffers (100 mM, acetate [pH 4.0–6.0], phosphate [pH 7.0–8.0], glycine [pH 9.0–10.0], and phosphate [pH 11.0–12.0]), and the residual activity was assayed. The thermostability of PH0782 was assessed by determining the residual activity after incubation at selected temperatures for 30 min and at 80°C for several different incubation times. The residual activities in the temperature and pH stability assays were determined spectrophotometrically using L-Ala as the substrate, as described previously (Kawakami et al., 2015).
Cultivation of P. horikoshii Under Various Conditions and Determination of Racemase Activities
The activity of BAR was greatly increased in P. horikoshii cells grown on medium supplemented with D-allo-Ile (Kawakami et al., 2015). We therefore investigated ASR activity in P. horikoshii cells grown on medium supplemented with D-Ala, D-Ser or D-allo-Ile. Cultivation of P. horikoshii OT-3 with a D-amino acid was previously described by Kawakami et al. (2015).
In the kuruma prawn, D-Ala produced from L-Ala by AlaR reportedly functions as an osmolyte responsible for intracellular isosmotic regulation (Yoshikawa and Yokoyama, 2015). To determine whether ASR is responsible for increasing the NaCl concentration in the medium, P. horikoshii was cultivated for 3 h at several NaCl concentrations (1.5, 2.5, 3.5, and 4.5%) in standard medium after cultivation for 18 h at 90°C in standard medium (2.5% NaCl). The P. horikoshii cells collected by centrifugation were disrupted by sonication, after which the extracts were cleared through another centrifugation, and the supernatants were used for determination of racemase activity. Spectrophotometric assays were performed as described previously (Kawakami et al., 2015), and L-Met and L-Ala were used as substrates for BAR and ASR, respectively.
Results
Screening of Substrates for Racemase Activity of BAR Homologs
Escherichia coli BL21 (DE3) cells harboring expression plasmids encoding BAR homologs (pET11a/PH0782, pET11a/PH1423, and pET11a/PH1501) were grown in LB medium, and gene expression was induced using IPTG. The cells were then disrupted by sonication, and the resultant supernatants were heated at 90°C for 20 min in the presence of 0.1M citrate (pH 5.5) buffer. The heat-treated supernatants were then subjected to SDS–PAGE, and bands derived from BAR homologs were clearly detected at about 50 kDa (data not shown). To screen for amino acid racemase activity, L-forms of Ala, Val, Leu, Ile, Phe, Met, Trp, Tyr, Ser, Thr, Asn, Gln, and Arg were used as substrates with the BAR homologs, and the reaction mixtures were analyzed using UPLC. Peaks for the D-forms of Ala and Ser were clearly detected in the reaction mixture containing PH0782. Weaker peaks for D-Val and D-allo-Thr were also detected with PH0782 (data not shown). Slight peaks for the D-forms of Met, Phe, and Leu were detected in the reaction mixture containing PH1501. No peak corresponding to a D-amino acid was detected in the reaction mixture containing PH1423 (data not shown). In addition, spectrophotometric assay revealed no racemase activity toward Pro in any of the enzymes (data not shown).
Purification and Molecular Mass Determination of PH0782
PH0782 was successfully purified using the same procedure used for BAR (Figure 1A). About 4 mg of purified enzyme were obtained from 3.2 L of LB medium. SDS–PAGE showed the subunit molecular mass of purified PH0782 to be about 54.9 kDa, which approximates the molecular mass (50.5 kDa) calculated from the primary structure. Using gel filtration chromatography, the native molecular mass of PH0782 was estimated to be about 300 kDa, which suggests the enzyme exists as a hexamer.
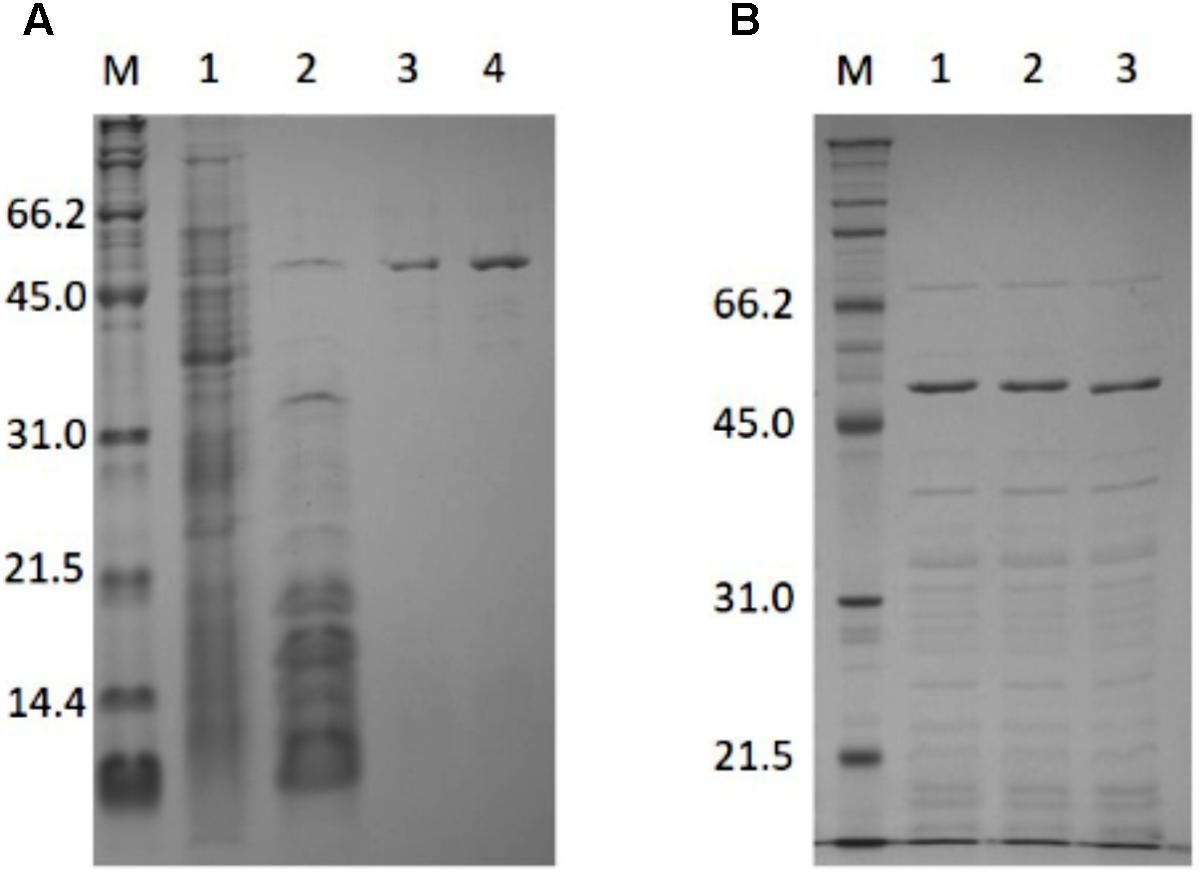
FIGURE 1. SDS–PAGE analysis of the purification of PH0782 (A) and its mutants (B). (A) 15% polyacrylamide gel was used for the analysis. Lanes: M, markers; 1, crude extract (30 μg); 2, heat treatment (6.5 μg); 3, butyl-Toyopearl (1.4 μg); and 4, DEAE-cellurofine (1.4 μg). (B) Crude extracts of cells expressing wild type and mutant PH0782 were heated at 90°C for 20 min with 0.1M citrate (pH 5.5), and the supernatants (each of about 7 μg) were applied to 12% polyacrylamide gel. Lanes: M, markers; 1, wild type; 2, K291A mutant; and 3, D234A mutant.
Characterization of PH0782
The activity of the purified enzyme was doubled by the addition of PLP and completely inhibited by the addition of 1 mM hydroxylamine hydrochloride, a known inhibitor of PLP-dependent enzymes, suggesting PLP is a cofactor. To prepare an apo-protein of PH0782, the enzyme was incubated at 80°C for 18 h in the assay mixture. The apo-enzyme exhibited no activity in the absence of PLP and recovered its activity by addition of PLP.
To assess the thermostability of PH0782, its enzyme activity was determined after incubation at different temperatures for various times. When the enzyme was incubated for 30 min at temperatures between 50 and 100°C, full enzyme activity was retained until 90°C, but about 30% was lost at 100°C (Figure 2A). When the enzyme was incubated at 80°C, full activity was retained for at least 5 h (Figure 2B). When the enzyme was incubated at 80°C for 2 h at various pHs, full enzyme activity was retained at pHs ranging from 6 to 10 (Figure 2C). To determine the optimal pH for enzyme activity, the assay was performed at pHs ranging from 5.5 to 9.5. Highest activity was detected at around pH 6.5–7.0 in MES and HEPES buffers (Figure 2D).
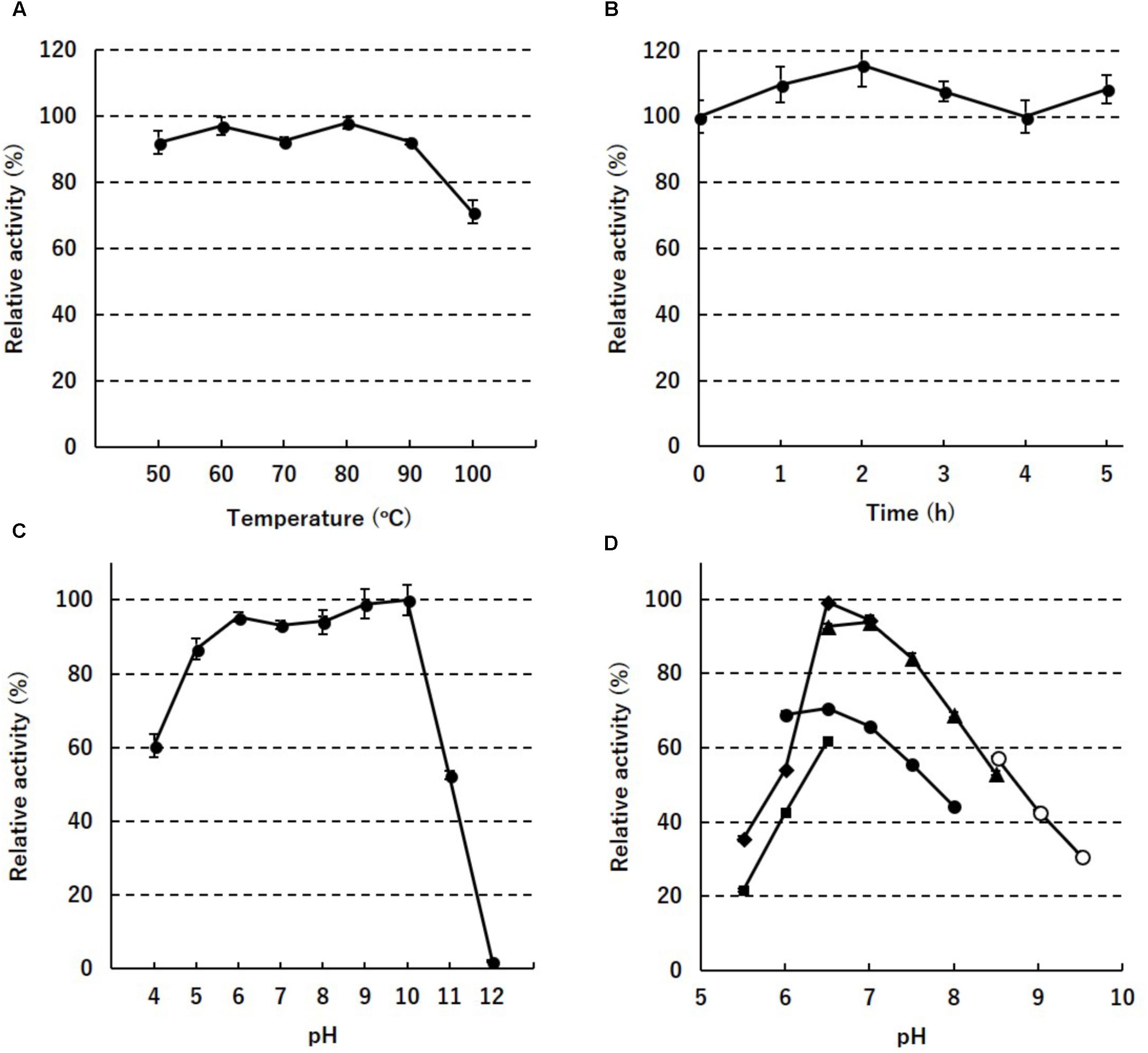
FIGURE 2. Enzyme stability against temperature (A), time (B), and pH (C), and the pH dependency (D) of PH0782. In (D), acetate (squares), MES (diamonds), phosphate (closed circles), HEPES (triangles), and CHES (open circles) were used as buffers.
To determine the kinetic parameters of PH0782 enzyme activity toward Ala and Ser, the initial velocities of both D-amino acid formation and L-amino acid formation were measured at concentrations ranging from 1 to 20 mM. The relation between substrate concentration and initial velocity fitted well into a non-linear regression model. The calculated Vmax and Km values for PH0782 are shown in Table 1. The Vmax values toward D-Ala (49.5 ± 2.09 μmol/min/mg) and D-Ser (41.2 ± 1.10 μmol/min/mg) were 1.7 and 1.3 times higher than those toward L-Ala (28.2 ± 0.76 μmol/min/mg) and L-Ser (31.4 ± 0.80 μmol/min/mg), respectively. The Km values for L-Ala (3.3 ± 0.22 mM) and L-Ser (4.5 ± 0.32 mM) were lower than those for D-Ala (5.5 ± 0.59 mM) and D-Ser (5.9 ± 0.40 mM), respectively. The kinetic parameters for Val and Thr were not determined because of the high Km and/or low Vmax predicted from the preliminary analysis.
Mutation Analysis of the Catalytic Residues
Two PH0782 mutants (K291A and D234A) were constructed to confirm that these residues are important for the enzyme’s catalytic activity. Figure 3 shows an alignment of the primary structures of BAR homologs and ILEP from Lactobacillus buchneri JCM1115 (Mutaguchi et al., 2013). The crystal structure of ILEP revealed that K280 and D222 are responsible for the interaction with PLP (Hayashi et al., 2017). These residues corresponded to residues K291 and D234 in PH0782 (Figure 3). After expression in E. coli, the mutant proteins were clearly observed on SDS–PAGE after heat treatment at 90°C (Figure 1B). Spectrophotometric assay using L-Ala as a substrate detected no activity with either the K291A or D234A mutant.
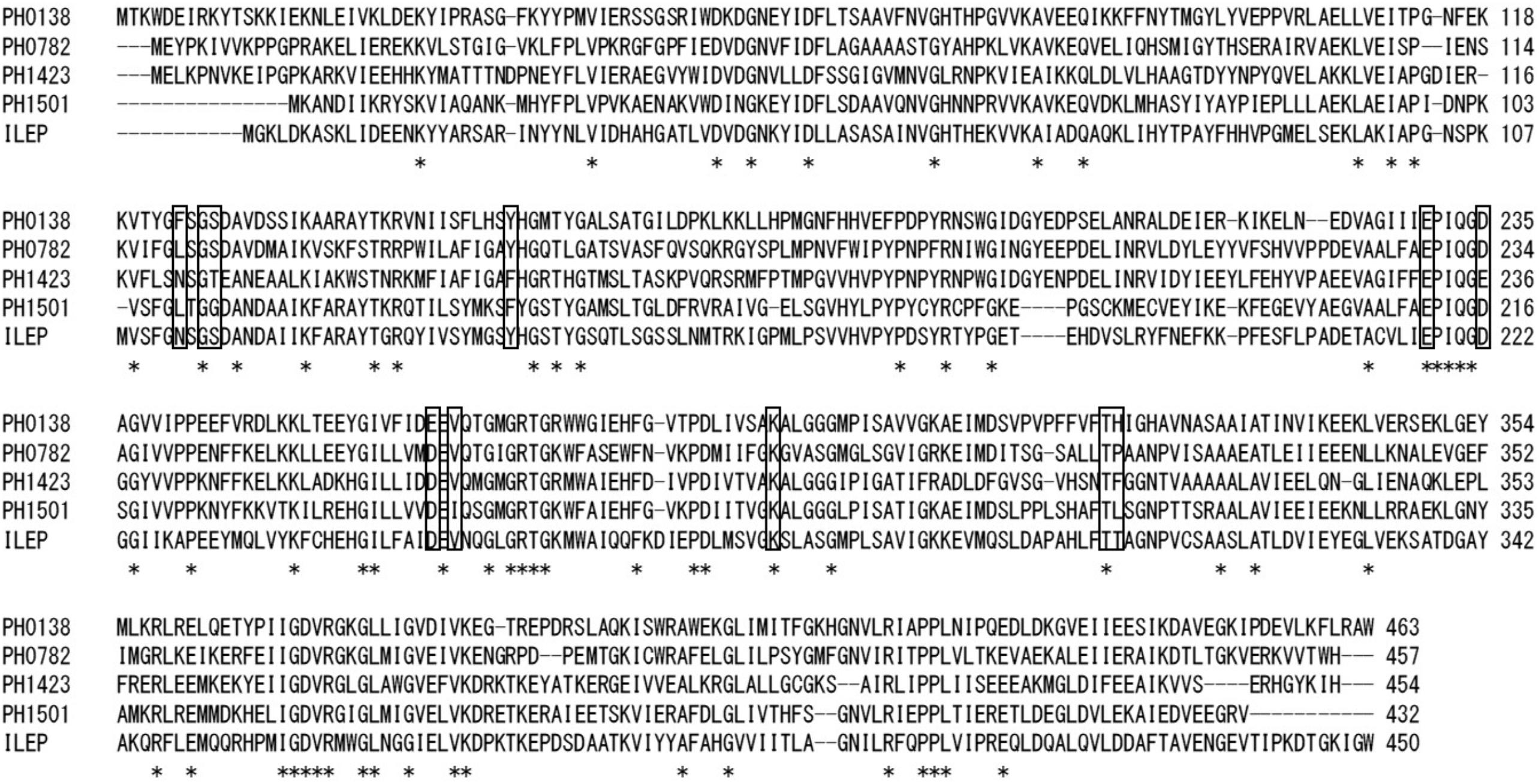
FIGURE 3. Sequence alignment of BAR homologs and ILEP from L. buchneri. Conserved residues are indicated by asterisks. Residues interacting with PLP, which were determined from the structure of ILEP (Hayashi et al., 2017), are boxed.
AlaR Activity in P. horikoshii Cells Grown Under Various Conditions
To determine whether ASR mediates utilization of D-amino acids for growth, P. horikoshii was cultivated in medium containing several D-amino acids. The activity toward Met derived from BAR was detected in all P. horikoshii cells tested. As previously described, the highest activity was detected in cells grown on medium containing D-allo-Ile (Kawakami et al., 2015). By contrast, no AlaR activity was detected in any of the tested cells.
Alanine racemase activity in P. horikoshii cells cultivated at several NaCl concentrations (1.5, 2.5, 3.5, and 4.5%) was determined to test whether ASR is responsible for increasing the NaCl concentration in the medium, but no activity was detected in any of the tested cells.
Discussion
In an earlier study, we revealed that PH0138, which was originally annotated as a putative GABA-AT, catalyzed the racemization of a number of hydrophobic and aromatic amino acids. We therefore designated the enzyme a BAR (Kawakami et al., 2015). In addition, we found that three other genes, PH0782, PH1423, and PH1501, were also annotated as putative GABA-AT genes and had high sequence similarity with BAR (about 45%). In the present study, we succeeded in constructing expression plasmids encoding these genes and found that PH0782 has amino acid racemase activity toward Ala, Ser, Val, and Thr. The racemase activity was completely inhibited by hydroxylamine hydrochloride, but strangely, we did not succeed to prepare apo-protein of PH0782 by dialysis against the buffer containing hydroxylamine hydrochloride. In addition, apo-protein was not obtained even after incubation at 80°C for 18 h with or without hydroxylamine. This suggests that PLP binds tightly to PH0782 and is stabilized in the enzyme complex, although free PLP is known to be thermolabile. On the other hand, after the enzyme was incubated at 80°C for 18 h in the assay mixture containing L-Ala as substrate, it showed no activity but recovered its activity by the addition of PLP. This indicates that apo-protein is probably prepared by incubation in the presence of substrate at 80°C. But further studies might be needed to clarify these observations. The enzyme activity of PH0782 produced equimolar amounts of L-Ala and D-Ala and of L-Ser and D-Ser, and the equilibrium constant for racemization (Keq) was determined to be nearly 1 (Supplementary Figure S1). These results indicate that PH0782 catalyzes the racemization reaction. Kinetic analysis revealed that the catalytic efficiency with Ala (7.19 ± 0.52 /S/MM FOR L-Ala and 7.58 ± 0.87 /s/mM for D-Ala) is comparable to that with Ser (5.87 ± 0.44 /s/mM for L-Ser and 5.88 ± 0.43 /s/mM for D-Ser), but the efficiency was much lower with Val or Thr. We therefore conclude that PH0782 is an ASR, not a BAR. Further analyses will be necessary to identify the substrates and activities of PH1423 and PH1501, as they exhibited little or no activity in the present study.
Alanine racemases and serine racemases are well characterized as PLP-dependent racemases (Yoshimura and Esaki, 2003; Radkov and Moe, 2014; Hernández and Cava, 2016) that generally show strict substrate specificity for Ala and Ser, and are classified as fold type III and type II PLP-dependent enzymes, respectively. By contrast, ASR shows equivalent substrate specificity for Ala and Ser and is classified as fold type I. Although racemase activities toward Ala and Ser have been detected in extract from the hyperthermophilic archaeon Pyrobaculum islandicum, the details remain unclear (Nagata et al., 2002). Recently, ILEP from L. buchneri was found to share 37 and 40% sequence identity with ASR and BAR, respectively, and to be a fold type I enzyme that catalyzes the epimerization and/or racemization of multiple hydrophobic amino acids, including Ile and Val (Mutaguchi et al., 2013). More recently, the crystal structures of ILEP in its apo-form and in complex with PLP, PLP-L-Ile, and PLP-D-allo-Ile were determined at resolutions of 1.94–2.77 Å (Hayashi et al., 2017). The residues in ILEP that interact with PLP are well conserved in both ASR and BAR (Figure 3). Among these residues, K280 (K291 in ASR) forms an internal aldimine (Schiff-base) linkage with PLP, and D222 (D234) is the most proximate to the Cα atom of D-allo-Ile. In addition, the D222A and D222N ILEP mutants exhibit almost no activity (Hayashi et al., 2017). Likewise, in the present study, the K291A and D234A ASR mutants exhibited no activity. These results suggest that, as in ILEP, these residues are involved in Schiff base formation and substrate interaction, and that the monomer structures of ASR and BAR are similar to that of ILEP, though the quaternary structures differ among the three enzymes: BAR is dimeric, ILEP is tetrameric, and ASR is hexameric.
Several free D-amino acids, including D-Asp and D-Ala, are present within the cells of hyperthermophilic archaea, but their functions remain unknown. The presence of ASR in P. horikoshii suggests that D-Ala and/or D-Ser serve some function in these cells. We previously observed that cellular BAR activity in P. horikoshii is dramatically enhanced when the cells are grown on medium supplemented with D-allo-Ile (Kawakami et al., 2015). This was the first clue that D-amino acids are involved with expression of enzyme genes in hyperthermophilic archaea. We therefore investigated ASR activity in P. horikoshii grown on medium supplemented with D-amino acids; however, no activity was detected in any of the cells tested. It is also known that D-Ala acts as an osmolyte to protect tissues in crustaceans from changes in osmotic pressure (Yoshikawa and Yokoyama, 2015). We therefore investigated ASR activity in P. horikoshii cells grown in the presence of high and low salt concentrations, but again no activity was detected. Although the physiological function of ASR remains unclear, the ASR-encoding PH0782 gene forms a cluster with PH0781 gene, which encodes a putative alanine transport protein, and this gene cluster is widely distributed in other Pyrococcus and Thermococcus strains. This suggests the enzyme may function in D-Ala production from L-Ala in these strains.
Author Contributions
RK conceived and designed the study, collected, analyzed and interpreted the data, and drafted the article. TAO carried out, analyzed, and interpreted a pair of experiments. HS and TOO interpreted data and helped draft the manuscript.
Conflict of Interest Statement
The authors declare that the research was conducted in the absence of any commercial or financial relationships that could be construed as a potential conflict of interest.
Acknowledgments
This work was supported in part by JSPS KAKENHI Grant Number JP 15H04490 (to TOO) and a grant from the Institute for Fermentation, Osaka (IFO Grant No. G-2018-2-079 to HS).
Supplementary Material
The Supplementary Material for this article can be found online at: https://www.frontiersin.org/articles/10.3389/fmicb.2018.01481/full#supplementary-material
Abbreviation
AlaR, alanine racemase; ASR, Ala/Ser racemase; BAR, broad substrate specificity amino acid racemase; GABA-AT, γ-aminobutyric acid aminotransferase; IPTG, isopropyl-β-D-thiogalactopyranoside; NBC, N-tert-butyloxycarbonyl-L-cystein; OPA, o-phthalaldehyde; SerR, serine racemase.
References
Abe, H., Yoshikawa, N., Sarower, M. G., and Okada, S. (2005). Physiological function and metabolism of free D-alanine in aquatic animals. Biol. Pharm. Bull. 28, 1571–1577. doi: 10.1248/bpb.28.1571
Aihara, T., Ito, T., Yamanaka, Y., Noguchi, K., Odaka, M., Sekine, M., et al. (2016). Structural and functional characterization of aspartate racemase from the acidothermophilic archaeon Picrophilus torridus. Extremophiles 20, 385–393. doi: 10.1007/s00792-016-0829-7
Bradford, M. M. (1976). A rapid and sensitive method for the quantitation of microgram quantities of protein utilizing the principle of protein-dye binding. Anal. Biochem. 72, 248–254. doi: 10.1016/0003-2697(76)90527-3
Errico, F., Mothet, J. P., and Usiello, A. (2015). D-Aspartate: an endogenous NMDA receptor agonist enriched in the developing brain with potential involvement in schizophrenia. J. Pharm. Biomed. Anal. 116, 7–17. doi: 10.1016/j.jpba.2015.03.024
Ghuysen, J. M. (1961). Précisions sur la structure des complexes disaccharide-peptide libérés des parois de Micrococcus lysodeikticus sous l’action des β (I → 4) N-acetylhexosaminidases. Biochim. Biophys. Acta 47, 561–568. doi: 10.1016/0006-3002(61)90551-0
Hancock, R. (1960). The amino acid composition of the protein and cell wall of Staphylococcus aureus. Biochim. Biophys. Acta 37, 42–46. doi: 10.1016/0006-3002(60)90076-7
Hayashi, J., Mutaguchi, Y., Minemura, Y., Nakagawa, N., Yoneda, K., Ohmori, T., et al. (2017). Crystal structure of the novel amino-acid racemase isoleucine 2-epimerase from Lactobacillus buchneri. Acta Crystallogr. D Struct. Biol. 73, 428–437. doi: 10.1107/S2059798317005332
Hernández, S. B., and Cava, F. (2016). Environmental roles of microbial amino acid racemases. Environ. Microbiol. 18, 1673–1685. doi: 10.1111/1462-2920.13072
Kawakami, R., Ohmori, T., Sakuraba, H., and Ohshima, T. (2015). Identification of a novel amino acid racemase from a hyperthermophilic archaeon Pyrococcus horikoshii OT-3 induced by D-amino acids. Amino Acids 47, 1579–1587. doi: 10.1007/s00726-015-2001-6
Kawakami, R., Sakuraba, H., Ohmori, T., and Ohshima, T. (2017). First characterization of an archaeal amino acid racemase with broad substrate specificity from the hyperthermophile Pyrococcus horikoshii OT-3. J. Biosci. Bioeng. 124, 23–27. doi: 10.1016/j.jbiosc.2017.02.004
Kita, A., Tasaki, S., Yohda, M., and Miki, K. (2009). Crystal structure of PH1733, an aspartate racemase homologue, from Pyrococcus horikoshii OT3. Proteins 74, 240–244. doi: 10.1002/prot.22244
Laemmli, U. K. (1970). Cleavage of structural proteins during the assembly of the head of bacteriophage T4. Nature 227, 680–685. doi: 10.1038/227680a0
Liu, L., Iwata, K., Kawarabayasi, Y., Kikuchi, H., Kita, A., Yohda, M., et al. (2001). Crystallization and preliminary X-ray analysis of aspartate racemase from Pyrococcus horikoshii OT3. Acta Crystallogr. D Biol. Crystallogr. 57, 1674–1676. doi: 10.1107/S0907444901012549
Liu, L., Iwata, K., Kita, A., Kawarabayasi, Y., Yohda, M., and Miki, K. (2002a). Crystal structure of aspartate racemase from Pyrococcus horikoshii OT3 and its implications for molecular mechanism of PLP-independent racemization. J. Mol. Biol. 319, 479–489. doi: 10.1016/S0022-2836(02)00296-6
Liu, L., Iwata, K., Yohda, M., and Miki, K. (2002b). Structural insight into gene duplication, gene fusion and domain swapping in the evolution of PLP-independent amino acid racemases. FEBS Lett. 528, 114–118. doi: 10.1016/S0014-5793(02)03264-7
Long, Z., Lee, J. A., Okamoto, T., Sekine, M., Nimura, N., Imai, K., et al. (2001). Occurrence of d-amino acids and a pyridoxal 5′-phosphate-dependent aspartate racemase in the acidothermophilic archaeon, Thermoplasma acidophilum. Biochem. Biophys. Res. Commun. 281, 317–321. doi: 10.1006/bbrc.2001.4353
Matsumoto, M., Homma, H., Long, Z., Imai, K., Iida, T., Maruyama, T., et al. (1999). Occurrence of free D-amino acids and aspartate racemases in hyperthermophilic archaea. J. Bacteriol. 181,6560–6563.
Moore, B. C., and Leigh, J. A. (2005). Markerless mutagenesis in Methanococcus maripaludis demonstrates roles for alanine dehydrogenase, alanine racemase, and alanine permease. J. Bacteriol. 187, 972–979. doi: 10.1128/JB.187.3.972-979.2005
Mutaguchi, Y., Ohmori, T., Wakamatsu, T., Doi, K., and Ohshima, T. (2013). Identification, purification, and characterization of a novel amino acid racemase, isoleucine 2-epimerase, from Lactobacillus species. J. Bacteriol. 195, 5207–5215. doi: 10.1128/JB.00709-13
Nagata, Y., Tanaka, K., Iida, T., Kera, Y., Yamada, R., Nakajima, Y., et al. (1999). Occurrence of D-amino acids in a few archaea and dehydrogenase activities in hyperthermophile Pyrobaculum islandicum. Biochim. Biophys. Acta 1435, 160–166. doi: 10.1016/S0167-4838(99)00208-3
Nagata, Y., Ito, M., Toizaki, S., Sugisaki, T., and Yamada, T. (2002). A D-amino acid dehydrogenase and an alanine racemase in a hyperthermophile Pyrobaculum islandicum. Viva Orig. 30, 199–203.
Ohnishi, M., Saito, M., Wakabayashi, S., Ishizuka, M., Nishimura, K., Nagata, Y., et al. (2008). Purification and characterization of serine racemase from a hyperthermophilic archaeon, Pyrobaculum islandicum. J. Bacteriol. 190, 1359–1365. doi: 10.1128/JB.01184-07
Ohtaki, A., Nakano, Y., Iizuka, R., Arakawa, T., Yamada, K., Odaka, M., et al. (2008). Structure of aspartate racemase complexed with a dual substrate analogue, citric acid, and implications for the reaction mechanism. Proteins 70, 1167–1174. doi: 10.1002/prot.21528
Radkov, A. D., and Moe, L. A. (2014). Bacterial synthesis of D-amino acids. Appl. Microbiol. Biotechnol. 98, 5363–5374. doi: 10.1007/s00253-014-5726-3
Reynolds, P. E., and Courvalin, P. (2005). Vancomycin resistance in enterococci due to synthesis of precursors terminating in D-alanyl-D-serine. Antimicrob. Agents Chemother. 49, 21–25. doi: 10.1128/AAC.49.1.21-25.2005
Veiga, P., Piquet, S., Maisons, A., Furlan, S., Courtin, P., Chapot-Chartier, M. P., et al. (2006). Identification of an essential gene responsible for D-Asp incorporation in the Lactococcus lactis peptidoglycan crossbridge. Mol. Microbiol. 62, 1713–1724. doi: 10.1111/j.1365-2958.2006.05474.x
Washio, T., Kato, S., and Oikawa, T. (2016). Molecular cloning and enzymological characterization of pyridoxal 5’-phosphate independent aspartate racemase from hyperthermophilic archaeon Thermococcus litoralis DSM 5473. Extremophiles 20, 711–721. doi: 10.1007/s00792-016-0860-8
Yoshida, T., Seko, T., Okada, O., Iwata, K., Liu, L., Miki, K., et al. (2006). Roles of conserved basic amino acid residues and activation mechanism of the hyperthermophilic aspartate racemase at high temperature. Proteins 64, 502–512. doi: 10.1002/prot.21010
Yoshikawa, N., and Yokoyama, M. (2015). Effects of high-salinity seawater acclimation on the levels of D-alanine in the muscle and hepatopancreas of kuruma prawn, Marsupenaeus japonicus. J. Pharm. Biomed. Anal. 116, 53–58. doi: 10.1016/j.jpba.2015.05.003
Keywords: Ala racemase, Ser racemase, PLP-dependent enzyme, hyperthermophilic archaea, Pyrococcus horikoshii OT-3, D-amino acid
Citation: Kawakami R, Ohshida T, Sakuraba H and Ohshima T (2018) A Novel PLP-Dependent Alanine/Serine Racemase From the Hyperthermophilic Archaeon Pyrococcus horikoshii OT-3. Front. Microbiol. 9:1481. doi: 10.3389/fmicb.2018.01481
Received: 12 January 2018; Accepted: 13 June 2018;
Published: 09 July 2018.
Edited by:
Tohru Yoshimura, Nagoya University, JapanCopyright © 2018 Kawakami, Ohshida, Sakuraba and Ohshima. This is an open-access article distributed under the terms of the Creative Commons Attribution License (CC BY). The use, distribution or reproduction in other forums is permitted, provided the original author(s) and the copyright owner(s) are credited and that the original publication in this journal is cited, in accordance with accepted academic practice. No use, distribution or reproduction is permitted which does not comply with these terms.
*Correspondence: Ryushi Kawakami, a2F3YWthbWlAdG9rdXNoaW1hLXUuYWMuanA=