- 1Morrill Science Center IV, Department of Microbiology, University of Massachusetts, Amherst, MA, United States
- 2Department of Physical and Biological Sciences, Western New England University, Springfield, MA, United States
Insoluble extracellular electron donors are important sources of energy for anaerobic respiration in biogeochemical cycling and in diverse practical applications. The previous lack of a genetically tractable model microorganism that could be grown to high densities under anaerobic conditions in pure culture with an insoluble extracellular electron donor has stymied efforts to better understand this form of respiration. We report here on the design of a strain of Geobacter sulfurreducens, designated strain ACL, which grows as thick (ca. 35 μm) confluent biofilms on graphite cathodes poised at -500 mV (versus Ag/AgCl) with fumarate as the electron acceptor. Sustained maximum current consumption rates were 0.8 A/m2, which is 10-fold higher than the current consumption of the wild-type strain. The improved function on the cathode was achieved by introducing genes for an ATP-dependent citrate lyase, completing the complement of enzymes needed for a reverse TCA cycle for the synthesis of biosynthetic precursors from carbon dioxide. Strain ACL provides an important model organism for elucidating the mechanisms for effective anaerobic growth with an insoluble extracellular electron donor and may offer unique possibilities as a chassis for the introduction of synthetic metabolic pathways for the production of commodities with electrons derived from electrodes.
Introduction
The ability of some microorganisms to accept electrons from insoluble extracellular sources to support anaerobic respiration is of biogeochemical significance and can be harnessed for diverse practical applications. However, elucidating the mechanisms for this type of electron transport has been limited by a lack of genetically tractable anaerobes that can be effectively grown in pure cultures with insoluble electron donors.
Examples of biogeochemically significant anaerobic oxidation of insoluble extracellular electron donors include oxidation of reduced humic substances (Lovley et al., 1999; Coates et al., 2002) and direct interspecies electron transfer (DIET) in which methanogens consume electrons either through direct biological electrical connections (Rotaru et al., 2014a,b; Holmes et al., 2017) or conductive materials (Kato et al., 2012; Liu et al., 2012; Chen et al., 2014a,b).
DIET may be of practical significance during anaerobic digestion of organic wastes (Morita et al., 2011; Rotaru et al., 2014b; Shrestha et al., 2014) and many studies have demonstrated how promoting DIET can accelerate and stabilize the anaerobic digestion process (Cheng and Call, 2016; Lovley, 2017b,c). Direct electron transfer from zero valent iron to microorganisms may be an important mechanism for anaerobic corrosion (Dinh et al., 2004; Uchiyama et al., 2010; Enning et al., 2012; Kato et al., 2014). Feeding microorganisms electrons with an electrode is becoming an increasingly attractive possibility for the production of biofuels and other organic commodities, as well as for bioremediation of organic and metal contamination (Thrash and Coates, 2008; Lovley, 2011; Lovley and Nevin, 2013; Rosenbaum and Henrich, 2014; Tremblay and Zhang, 2015; May et al., 2016; Tremblay et al., 2017).
Some strategies for anaerobic growth with extracellular electron donors are not conducive for detailed molecular investigation of metabolism. For example, growth with humic substances or their analogs yields low maximum cell densities of ≤2 × 107 cells per ml (Lovley et al., 1999; Coates et al., 2002). More biomass can be obtained in co-cultures in which the genetically tractable Geobacter sulfurreducens grows by oxidizing the reduced soluble humics analog anthrahydroquinone-2,6-disulfonate (Smith et al., 2015) or via DIET (Summers et al., 2010; Shrestha et al., 2013a,b). However, the presence of the electron-donating partner in these co-cultures complicates mechanistic studies. Substantial iron mineral crusts are associated with cells growing by accepting electrons from zero valent iron (Dinh et al., 2004; Uchiyama et al., 2010; Enning et al., 2012).
Pure culture studies on electron transfer to electrodes have been a powerful approach to elucidate mechanisms for electron transfer to extracellular electron acceptors because they provide an inert surface with a well-defined redox potential (Lovley, 2012, 2017a; Shi et al., 2016). For example, Geobacter sulfurreducens generates high current densities (Nevin et al., 2008; Yi et al., 2009) and forms thick (ca. 100 μm) biofilms on graphite electrodes serving as an electron acceptor, which has facilitated diverse gene expression, proteomic, and genetic investigations (Holmes et al., 2006; Reguera et al., 2006; Nevin et al., 2009; Franks et al., 2010, 2012; Inoue et al., 2010; Malvankar et al., 2011, 2012a,b; Leang et al., 2013; Chan et al., 2017).
G. sulfurreducens is also capable of growing with electrons derived from graphite electrodes (i.e., cathodes). However, cathode biofilms of wild-type G. sulfurreducens consume 20-fold less current than they produce on anodes and biofilms do not extend beyond a mono-layer on the cathode surface (Gregory et al., 2004; Strycharz et al., 2011). Much higher current consumption by G. sulfurreducens on stainless steel cathodes was reported (Dumas et al., 2008), but we have been unable to replicate those results. Furthermore, even in those studies, cathode biofilms were thin and patchy. Other genetically tractable microorganisms that can also consume electrons from cathodes include G. metallireducens (Gregory et al., 2004), Shewanella oneidensis (Ross et al., 2011), Clostridium ljungdahlii (Nevin et al., 2011), and Rhodopseudomonas palustris (Bose et al., 2014). However, all of these microorganisms grow poorly on cathodes, if at all. The cathode biofilms are sparse and current consumption rates are low.
Here, we report on a strain of G. sulfurreducens constructed to enable autotrophic growth. Surprisingly, this strain grew much more effectively on cathodes than the wild-type strain, providing a robust, genetically tractable microbe for the study of cathode-based growth.
Materials and Methods
Strains and Growth Conditions
G. sulfurreducens strain DL-1 was obtained from our laboratory culture collection and was routinely cultured under anaerobic conditions with acetate as the electron donor and fumarate as the electron acceptor, as previously described (Coppi et al., 2001). In order to construct G. sulfurreducens strain ACL, the genes aclA and aclB, which encode the two subunits for the ATP-citrate lyase of Chlorobium limicola (Kanao et al., 2001), were synthesized with codon optimization for G. sulfurreducens with GenScript (Supplementary Figure S1). A BamHI-EcoRI fragment of the aclA and aclB genes was cloned in pKIapr as previously described (Ueki et al., 2017).
Escherichia coli NEB 10-beta (New England Biolabs) was used for plasmid preparation and grown in LB medium supplemented with appropriate antibiotics, as necessary. The synthetic ATP-citrate lyase genes were introduced into the chromosome adjacent to the gene (GSU1106) for citrate synthase in G. sulfurreducens strain DL-1 (Figure 1) with previously described methods (Ueki et al., 2017). Expression of the introduced genes was induced by isopropyl β-D-1-thiogalactopyranoside (IPTG) at a concentration of 1 mM.
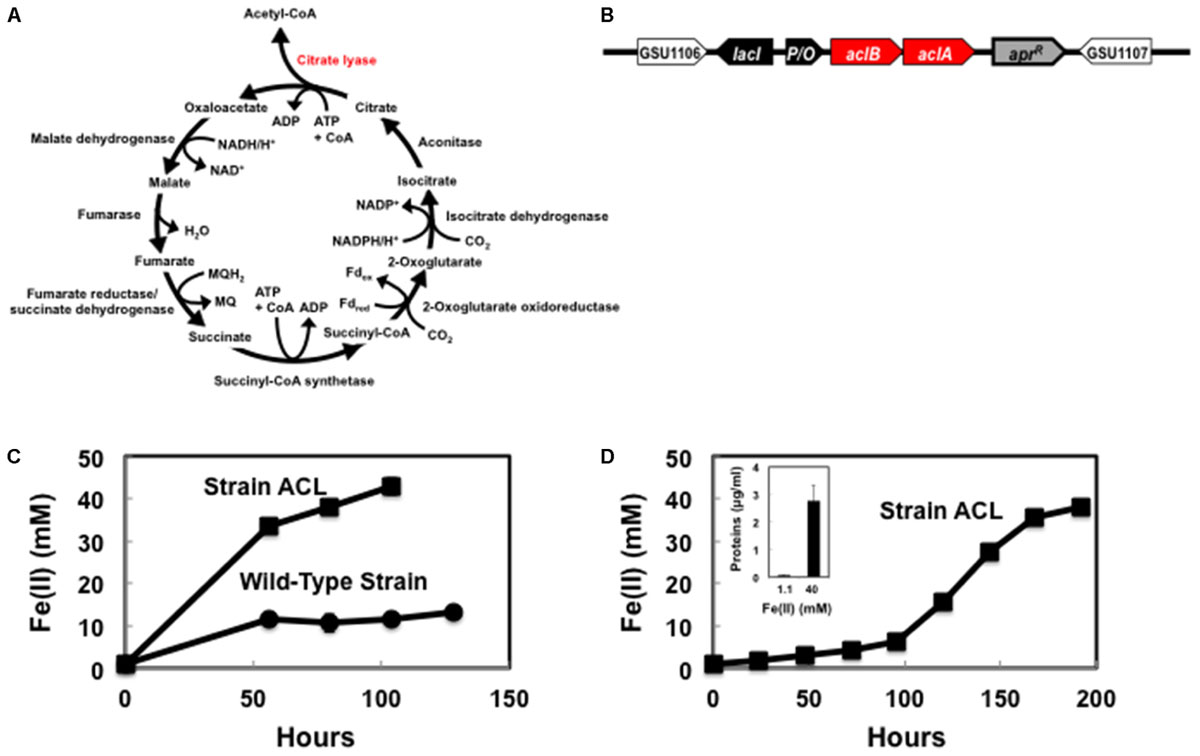
FIGURE 1. Geobacter sulfurreducens strain ACL capable of autotrophic growth. (A) Enzymes for reverse TCA cycle encoded in the native G. sulfurreducens genome and the requirement for ATP-dependent citrate lyase (in red) to complete the pathway. (B) Location of ATP-dependent citrate lyase genes within the G. sulfurreducens strain ACL chromosome. Citrate lyase genes: aclA and aclB; Lac repressor gene; lacI; tac-lac promoter/lac operator (IPTG-inducible): P/O; Apramycin resistance gene: aprR. (C) Fe(II) production from the reduction of Fe(III) with H2 as the electron donor following the first transfer of a 1% inoculum from cultures grown with acetate as the electron donor and Fe(III) as the electron acceptor. (D) Fe(II) production from the reduction of Fe(III) with H2 as the electron donor after a second transfer with a 2% inoculum of strain ACL from (C) into fresh medium. Inset-increase in protein concentration of strain ACL associated with Fe(III) reduction. The results are the means of duplicate cultures.
Cells were grown with H2 as the electron donor and Fe(III) citrate as the electron acceptor as previously described (Coppi et al., 2004). The addition of acetate (1 mM) as a carbon source was necessary to maintain strain DL-1. Fe(II) production was analyzed with ferrozine (Lovley and Phillips, 1987). To estimate cell biomass, total cell extracts were prepared with B-PER Complete Bacterial Protein Extraction Reagent (Thermo Fisher Scientific) and the amount of protein was measured with the Bradford Protein Assay (Bio-Rad) as instructed by the manufacturer.
Growth on Cathodes and Anodes
Cells were grown under anaerobic conditions in two-chambered bioelectrochemical devices with graphite electrodes and potentiostat control of the anode or cathode potential, as previously described with graphite electrodes of 65 cm2 (Nevin et al., 2009; Strycharz et al., 2011). Cathodes were poised at – 500 mV versus Ag/AgCl with fumarate (40 mM) as the electron acceptor. Anodes were poised at +300 mV versus Ag/AgCl with acetate (10 mM) as the electron donor.
Confocal Microscopy
Biofilms on graphite cathodes were prepared using the LIVE/DEAD BacLight viability kit with antifade as previously described, except that isotonic wash buffer was used for rinsing and staining/destaining for 15 min (Nevin et al., 2008; Yi et al., 2009). Confocal scanning laser microscopy was imaged using a Leica TCS SP5 microscope, HCX PL FLUOTAR L 40× (numerical aperture 0.6) objective, and Leica LAS AF software (Yi et al., 2009).
Results and Discussion
In order to construct a strain of G. sulfurreducens that did not require an organic carbon source, we built on previous analysis (Mahadevan et al., 2006; Feist et al., 2014) that suggested that G. sulfurreducens lacks a complete pathway for carbon dioxide fixation. Introduction of an ATP-dependent citrate lyase provides the one enzyme that G. sulfurreducens requires for a complete complement of enzymes necessary for a reverse TCA cycle (Figure 1A and Supplementary Table S1) that has the potential to make necessary biosynthetic precursors from carbon dioxide. Therefore, codon-optimized genes for the two subunits of this enzyme from Chlorobium limicola (Kanao et al., 2001) were introduced into the chromosome adjacent to the gene (GSU1106) for citrate synthase (Figure 1B). This strain was designated G. sulfurreducens strain ACL (ATP-dependent citrate lyase).
As previously reported (Coppi et al., 2004), the wild-type strain of G. sulfurreducens could not sustain metabolism with H2 as the electron donor and Fe(III) citrate as the electron acceptor in the absence of a carbon source (Figure 1C). However, strain ACL grew in repeated transfers into Fe(III) citrate medium with H2 provided as the electron donor and carbon dioxide as the sole carbon source (Figure 1D). Fe(III) reduction was accompanied with an increase in cell protein (Figure 1D, inset). These results demonstrated that expression of the citrate lyase was sufficient to confer the capacity for autotrophic growth.
Strain ACL grew well on cathodes in a medium in which cathodes were the sole electron donor, fumarate was the electron acceptor, and acetate was not added as a carbon source (Figure 2). The improved growth of strain ACL on the cathodes was visible as thick, red biofilms (Figure 2A). In contrast, wild-type cells grew poorly, even with the addition of an acetate carbon source (Figure 2A). Confocal scanning laser microscopy images of the cathodes colonized by strain ACL revealed thick biofilms (ca. 35 μm) that predominantly stained green with Live/Dead stain, suggesting that cells were metabolically active and had intact membranes (Figure 2B). Wild-type cathode biofilms were thinner (<10 μm), very patchy, and stained predominately red, suggesting that many of the cells were moribund (Figure 2C). The growth of strain ACL on cathodes was accompanied by current consumption that consistently maximized at more than 5 mA (Figure 2D and Supplementary Figure S2). This is more than 10-fold the maximum current consumption of ≤0.5 mA typically observed with the wild-type strain (Gregory et al., 2004; Strycharz et al., 2011).
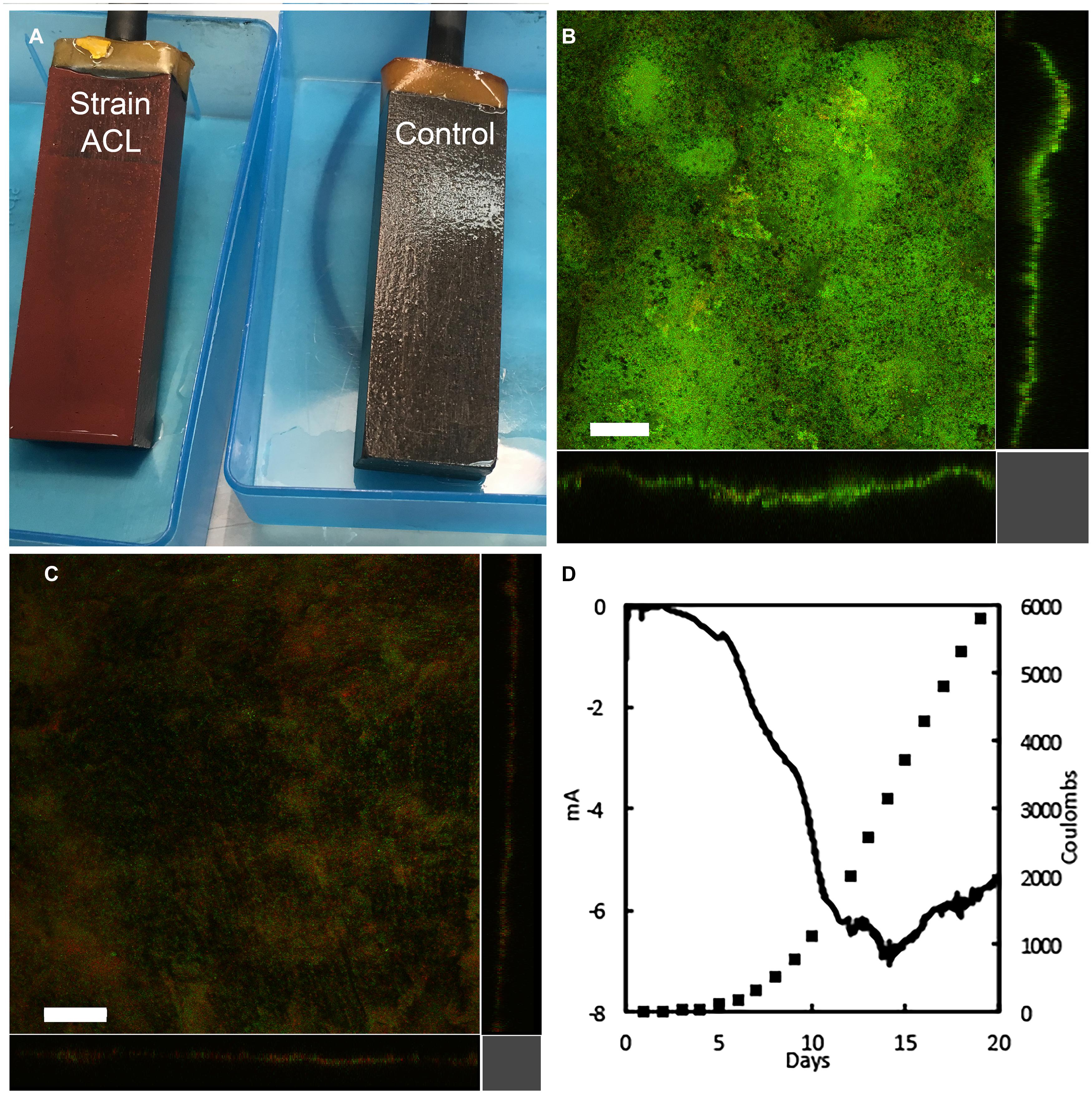
FIGURE 2. Cathode biofilms and current consumption. (A) Appearance of cathode biofilms of strain ACL and the control strain without citrate lyase genes. Confocal scanning laser micrographs of cathode biofilms of ACL (B) and wild-type (C) strains treated with Live/Dead stain. (D) Representative current consumption of ACL strain as current consumption rate (mA) and total cumulative coulombs consumed. Size bar = 50 μm.
The stoichiometry of total current consumption and the simultaneous recovery of electrons in the reduction of fumarate to succinate by mature cathode biofilms of strain ACL was determined over 6-h intervals in three separate bioelectrochemical devices. Coulombs of current consumed from the electrode and coulomb equivalents recovered in succinate production for the three replicate systems were: 40 coulombs consumed/41 equivalents recovered in succinate (recovery 102.5%); 103 coulombs consumed/96 equivalents recovered in succinate (recovery 93.2%); and 139 coulombs consumed/145 equivalents recovered in succinate (recovery 104.3%); yielding a mean and standard deviation of 100 ±6% for electron recovery in the triplicate studies.
Strain ACL generated currents comparable to the wild-type strain with acetate as the electron donor (Supplementary Figure S3). Carbon dioxide fixation by the reverse TCA cycle is not required during growth on acetate. Thus, possession of ATP-dependent citrate lyase would not be expected to enhance current production by strain ACL.
Implications
G. sulfurreducens strain ACL is, to our knowledge, the first pure culture shown to grow anaerobically to high cell densities with electrons derived from a cathode as the sole electron donor. An additional benefit is that methods for genetic manipulation of G. sulfurreducens (Coppi et al., 2001; Chan et al., 2015, 2017; Ueki et al., 2016); a genome-scale understanding of many aspects of central metabolism (Mahadevan et al., 2006, 2011); and a developing model for electron transfer to extracellular electron acceptors (Lovley et al., 2011; Shi et al., 2016) are all available as tools to facilitate elucidation of the mechanisms of electron transfer from extracellular electron donors into cells.
In addition to expressing the required electron carriers, cells accepting electrons from electrodes must also balance carbon and electron flow in a manner that effectively supports growth. The complex regulatory networks in G. sulfurreducens evolved to recognize the availability of natural electron donors (Lovley et al., 2011). They may not be optimized to balance metabolism in wild-type cells presented with both acetate and cathodes, an unnatural source, as electron donors. The ability of strain ACL to grow autotrophically removes the complication of acetate as a second potential electron donor.
Genetic and metabolic modeling tools will aid in further evaluating this hypothesis of metabolic imbalance in wild-type cells and may lead to the design and construction of strains with enhanced ability for electron uptake on cathodes. For example, strains of G. sulfurreducens with faster rates of electron transfer to extracellular electron acceptors have been developed from the understanding of mechanisms for long-range electron transfer to extracellular electron acceptors and central metabolism (Izallalen et al., 2008; Tremblay et al., 2011; Malvankar et al., 2012a; Leang et al., 2013).
As the availability of renewable sources of electricity rises, and costs decline, feeding microorganisms electrons with an electrode is becoming an increasingly attractive possibility for the production of biofuels and other organic commodities (Lovley, 2011; Lovley and Nevin, 2013; Rosenbaum and Henrich, 2014; Tremblay and Zhang, 2015; May et al., 2016; Tremblay et al., 2017). Strain ACL may be the ideal chassis for such endeavors.
Author Contributions
DL, KN, TU, MA, and DH conceived the study. TU constructed the ACL strain and evaluated autotrophic growth on hydrogen. KN and TW conducted the bioelectrochemical growth studies. DL, KN, and TU wrote the manuscript with modifications from all authors.
Funding
This research was supported by Office of Naval Research grants N00014-13-1-0549 and N00014-16-1-2784.
Conflict of Interest Statement
The authors declare that the research was conducted in the absence of any commercial or financial relationships that could be construed as a potential conflict of interest.
Supplementary Material
The Supplementary Material for this article can be found online at: https://www.frontiersin.org/articles/10.3389/fmicb.2018.01512/full#supplementary-material
References
Bose, A., Gardel, E. J., Vidoudez, C., Parra, E. A., and Girguis, P. R. (2014). Electron uptake by iron-oxidizing phototrophic bacteria. Nat. Commun. 5:3391. doi: 10.1038/ncomms4391
Chan, C. H., Levar, C., Zacharoff, L., Badalamenti, J. P., and Bond, D. R. (2015). Scarless genome editing and stable inducible expression vectors for Geobacter sulfurreducens. Appl. Environ. Microbiol. 81, 7178–7186. doi: 10.1128/AEM.01967-15
Chan, C. H., Levar, C. E., Jiménez-Otero, F., and Bond, D. R. (2017). Genome-scale mutational analysis of Geobacter sulfurreducens reveals distinct molecular mechanisms for respiration and sensing of poised electrodes versus Fe(III) oxides. J. Bacteriol. 199:e00340-17. doi: 10.1128/JB.00340-17
Chen, S., Rotaru, A. E., Liu, F., Philips, J., Woodard, T. L., Nevin, K. P., et al. (2014a). Carbon cloth stimulates direct interspecies electron transfer in syntrophic co-cultures. Bioresour. Technol. 173, 82–86. doi: 10.1016/j.biortech.2014.09.009
Chen, S., Rotaru, A. E., Shrestha, P. M., Malvankar, N. S., Liu, F., Fan, W., et al. (2014b). Promoting interspecies electron transfer with biochar. Sci. Rep. 4:5019. doi: 10.1038/srep05019
Cheng, Q., and Call, D. (2016). Hardwiring microbes via direct interspecies electron transfer: mechanisms and applications. Environ. Sci. Process. Impacts 18, 968–980. doi: 10.1039/C6EM00219F
Coates, J. D., Cole, K. A., Chakraborty, R., O’connor, S. M., and Achenbach, L. A. (2002). Diversity and ubiquity of bacteria capable of utilizing humic substances as electron donors for anaerobic respiration. Appl. Environ. Microbiol. 68, 2445–2452. doi: 10.1128/AEM.68.5.2445-2452.2002
Coppi, M. V., Leang, C., Sandler, S. J., and Lovley, D. R. (2001). Development of a genetic system for Geobacter sulfurreducens. Appl. Environ. Microbiol. 67, 3180–3187. doi: 10.1128/AEM.67.7.3180-3187.2001
Coppi, M. V., O’neil, R. O., and Lovley, D. R. (2004). Identification of an uptake hydrogenase required for hydrogen-dependent reduction of Fe(III) and other electron acceptors by Geobacter sulfurreducens. J. Bacteriol. 186, 3022–3028. doi: 10.1128/JB.186.10.3022-3028.2004
Dinh, H. T., Kuever, J., Macbmann, M., Hassel, A. W., Stratmann, M., and Widdel, F. (2004). Iron corrosion by novel anaerobic microorganisms. Nature 427, 829–832. doi: 10.1038/nature02321
Dumas, C., Basseguy, R., and Bergel, A. (2008). Microbial electrocatalysis with Geobacter sulfurreducens biofilm on stainless steel cathodes. Electrochim. Acta 53, 2494–2500. doi: 10.1016/j.electacta.2007.10.018
Enning, D., Venzlaff, H., Garrelfs, J., Dinh, H. T., Meyer, V., Mayrhofer, K., et al. (2012). Marine sulfate-reducing bacteria cause serious corrosion of iron under electroconductive biogenic mineral crust. Environ. Microbiol. 14, 1772–1787. doi: 10.1111/j.1462-2920.2012.02778.x
Feist, A. M., Nagarajan, H., Rotaru, A. E., Tremblay, P. L., Zhang, T., Nevin, K. P., et al. (2014). Constraint-based modeling of carbon fixation and the energetics of electron transfer in Geobacter metallireducens. PLoS Comput. Biol. 10:e1003575. doi: 10.1371/journal.pcbi.1003575
Franks, A. E., Glavin, R. H., and Lovley, D. R. (2012). Real-time spatial gene expression analysis with current-producing biofilms. ChemSusChem 5, 1092–1098. doi: 10.1002/cssc.201100714
Franks, A. E., Nevin, K. P., Glaven, R. H., and Lovley, D. R. (2010). Microtoming coupled with microarray analysis to evaluate potential differences in the metabolic status of Geobacter sulfurreducens at different depths in anode biofilms. ISME J. 4, 509–519. doi: 10.1038/ismej.2009.137
Gregory, K. B., Bond, D. R., and Lovley, D. R. (2004). Graphite electrodes as electron donors for anaerobic respiration. Environ. Microbiol. 6, 596–604. doi: 10.1111/j.1462-2920.2004.00593.x
Holmes, D. E., Chaudhuri, S. K., Nevin, K. P., Mehta, T., Methe, B. A., Liu, A., et al. (2006). Microarray and genetic analysis of electron transfer to electrodes in Geobacter sulfurreducens. Environ. Microbiol. 8, 1805–1815. doi: 10.1111/j.1462-2920.2006.01065.x
Holmes, D. E., Shrestha, P. M., Walker, D. J. F., Dang, Y., Nevin, K. P., Woodard, T. L., et al. (2017). Metatranscriptomic evidence for direct interspecies electron transfer between Geobacter and Methanothrix species in rice paddy soils. Appl. Environ. Microbiol. 83:e00553-17. doi: 10.1128/AEM.00223-17
Inoue, K., Leang, C., Franks, A. E., Woodard, T. L., Nevin, K. P., and Lovley, D. R. (2010). Specific localization of the c-type cytochrome OmcZ at the anode surface in current-producing biofilms of Geobacter sulfurreducens. Environ. Microbiol. Rep. 3, 211–217. doi: 10.1111/j.1758-2229.2010.00210.x
Izallalen, M., Mahadevan, R., Burgard, A., Postier, B., Didonato, R., Sun, J., et al. (2008). Geobacter sulfurreducens strain engineered for increased rates of respiration. Metab. Eng. 10, 267–275. doi: 10.1016/j.ymben.2008.06.005
Kanao, T., Fukui, T., Atomi, H., and Imanaka, T. (2001). ATP-citrate lyase from the green sulfur bacterium Chlorobium limicola is a heteromeric enzyme composed of two distinct gene products. Eur. J. Biochem. 268, 1670–1678. doi: 10.1046/j.1432-1327.2001.02034.x
Kato, S., Hashimoto, K., and Watanabe, K. (2012). Methanogenesis facilitated by electric syntrophy via (semi)conductive iron-oxide minerals. Environ. Microbiol. 14, 1646–1654. doi: 10.1111/j.1462-2920.2011.02611.x
Kato, S., Yumoto, I., and Kamagata, Y. (2014). Isolation of acetogenic bacteria that induce biocorrosion by utilizing metallic iron as the sole electron donor. Appl. Environ. Microbiol. 81, 63–73.
Leang, C., Malvankar, N. S., Franks, A. E., Nevin, K. P., and Lovley, D. R. (2013). Engineering Geobacter sulfurreducens to produce a highly cohesive conductive matrix with enhanced capacity for current production. Energy Environ. Sci. 6, 1901–1908. doi: 10.1039/c3ee40441b
Liu, F., Rotaru, A. E., Shrestha, P. M., Malvankar, N. S., Nevin, K. P., and Lovley, D. R. (2012). Promoting direct interspecies electron transfer with activated carbon. Energy Environ. Sci. 5, 8982–8989. doi: 10.1039/c2ee22459c
Lovley, D., Fraga, J., Coates, J., and Blunt-Harris, E. (1999). Humics as an electron donor for anaerobic respiration. Environ. Microbiol. 1, 89–98. doi: 10.1046/j.1462-2920.1999.00009.x
Lovley, D. R. (2011). Powering microbes with electricity: direct electron transfer from electrodes to microbes. Environ. Microbiol. Rep. 3, 27–35. doi: 10.1111/j.1758-2229.2010.00211.x
Lovley, D. R. (2012). Electromicrobiology. Annu. Rev. Microbol. 66, 391–409. doi: 10.1146/annurev-micro-092611-150104
Lovley, D. R. (2017a). Electrically conductive pili: biological function and potential applications in electronics. Curr. Opin. Electrochem. 4, 190–198. doi: 10.1016/j.coelec.2017.08.015
Lovley, D. R. (2017b). Happy together: microbial communities that hook up to swap electrons. ISME J. 11, 327–336. doi: 10.1038/ismej.2016.136
Lovley, D. R. (2017c). Syntrophy goes electric: direct interspecies electron transfer. Annu. Rev. Microbiol. 71, 643–664. doi: 10.1146/annurev-micro-030117-020420
Lovley, D. R., and Nevin, K. P. (2013). Electrobiocommodities: powering microbial production of fuels and commodity chemicals from carbon dioxide with electricity. Curr. Opin. Biotechnol. 24, 385–390. doi: 10.1016/j.copbio.2013.02.012
Lovley, D. R., and Phillips, E. J. P. (1987). Rapid assay for microbially reducible ferric iron in aquatic sediments. Appl. Environ. Microbiol. 53, 1536–1540.
Lovley, D. R., Ueki, T., Zhang, T., Malvankar, N. S., Shrestha, P. M., Flanagan, K., et al. (2011). Geobacter: the microbe electric’s physiology, ecology, and practical applications. Adv. Microb. Physiol. 59, 1–100. doi: 10.1016/B978-0-12-387661-4.00004-5
Mahadevan, R., Bond, D. R., Butler, J. E., Esteve-Nunez, A., Coppi, M. V., Palsson, B. O., et al. (2006). Characterization of metabolism in the Fe(III)-reducing organism Geobacter sulfurreducens by constraint-based modeling. Appl. Environ. Microbiol. 72, 1558–1568. doi: 10.1128/AEM.72.2.1558-1568.2006
Mahadevan, R., Palsson, B. O., and Lovley, D. R. (2011). In situ to in silico and back: elucidating the physiology and ecology of Geobacter spp. using genome-scale modelling. Nat. Rev. Microbiol. 9, 39–50. doi: 10.1038/nrmicro2456
Malvankar, N. S., Tuominen, M. T., and Lovley, D. R. (2012a). Biofilm conductivity is a decisive variable for high-current-density Geobacter sulfurreducens microbial fuel cells. Energy Environ. Sci. 5, 5790–5797. doi: 10.1039/c2ee03388g
Malvankar, N. S., Tuominen, M. T., and Lovley, D. R. (2012b). Lack of involvement of c-type cytochromes in long-range electron transport in microbial biofilms and nanowires. Energy Environ. Sci. 5, 8651–8659. doi: 10.1039/c2ee22330a
Malvankar, N. S., Vargas, M., Nevin, K. P., Franks, A. E., Leang, C., Kim, B. C., et al. (2011). Tunable metallic-like conductivity in nanostructured biofilms comprised of microbial nanowires. Nat. Nanotechnol. 6, 573–579. doi: 10.1038/nnano.2011.119
May, H. D., Evans, P. J., and Labelle, E. V. (2016). The bioelectrosynthesis of acetate. Curr. Opin. Biotechnol. 42, 225–233. doi: 10.1016/j.copbio.2016.09.004
Morita, M., Malvankar, N. S., Franks, A. E., Summers, Z. M., Giloteaux, L., Rotaru, A. E., et al. (2011). Potential for direct interspecies electron transfer in methanogenic wastewater digester aggregates. mBio 2:e00159-11. doi: 10.1128/mBio.00159-11
Nevin, K. P., Hensley, S. A., Franks, A. E., Summers, Z. M., Ou, J., Woodard, T. L., et al. (2011). Electrosynthesis of organic compounds from carbon dioxide is catalyzed by a diversity of acetogenic microorganisms. Appl. Environ. Microbiol. 77, 2882–2886. doi: 10.1128/AEM.02642-10
Nevin, K. P., Kim, B. C., Glaven, R. H., Johnson, J. P., Woodard, T. L., Methé, B. A., et al. (2009). Anode biofilm transcriptomics reveals outer surface components essential for high current power production in Geobacter sulfurreducens fuel cells. PLoS One 4:e5628. doi: 10.1371/journal.pone.0005628
Nevin, K. P., Richter, H., Covalla, S. F., Johnson, J. P., Woodard, T. L., Jia, H., et al. (2008). Power output and columbic efficiencies from biofilms of Geobacter sulfurreducens comparable to mixed community microbial fuel cells. Environ. Microbiol. 10, 2505–2514. doi: 10.1111/j.1462-2920.2008.01675.x
Reguera, G., Nevin, K. P., Nicoll, J. S., Covalla, S. F., Woodard, T. L., and Lovley, D. R. (2006). Biofilm and nanowire production leads to increased current in Geobacter sulfurreducens fuel cells. Appl. Environ. Microbiol. 72, 7345–7348. doi: 10.1128/AEM.01444-06
Rosenbaum, M. A., and Henrich, A. W. (2014). Engineering microbial electrocatalysis for chemical and fuel production. Curr. Opin. Biotechnol. 29, 93–98. doi: 10.1016/j.copbio.2014.03.003
Ross, D. E., Flynn, J. M., Baron, D. B., Gralnick, J. A., and Bond, D. R. (2011). Towards electrosynthesis in Shewanella: energetics of reversing the Mtr pathway for reductive metabolism. PLoS One 6:e16649. doi: 10.1371/journal.pone.0016649
Rotaru, A. E., Shrestha, P. M., Liu, F., Nevin, K. P., and Lovley, D. R. (2014a). Direct interspecies electron transfer during syntrophic growth of Geobacter metallireducens and Methanosarcina barkeri on ethanol. Appl. Environ. Microbiol. 80, 4599–4605. doi: 10.1128/AEM.00895-14
Rotaru, A. E., Shrestha, P. M., Liu, F., Shrestha, M., Shrestha, D., Embree, M., et al. (2014b). A new model for electron flow during anaerobic digestion: direct interspecies electron transfer to Methanosaeta for the reduction of carbon dioxide to methane. Energy Environ. Sci. 7, 408–415. doi: 10.1039/C3EE42189A
Shi, L., Dong, H., Reguera, G., Beyenal, H., Lu, A., Liu, J., et al. (2016). Extracellular electron transfer mechanisms between microorganisms and minerals. Nat. Rev. Microbiol. 14, 651–662. doi: 10.1038/nrmicro.2016.93
Shrestha, P. M., Malvankar, N. S., Werner, J. J., Franks, A. E., Rotaru, A. E., Shrestha, M., et al. (2014). Correlation between microbial community and granule conductivity in anaerobic bioreactors for brewery wastewater treatment. Bioresour. Technol. 174, 306–310. doi: 10.1016/j.biortech.2014.10.004
Shrestha, P. M., Rotaru, A. E., Aklujkar, M., Liu, F., Shrestha, M., Summers, Z. M., et al. (2013a). Syntrophic growth with direct Interspecies electron transfer as the primary mechanism for energy exchange. Environ. Microbiol. Rep. 5, 904–910. doi: 10.1111/1758-2229.12093
Shrestha, P. M., Rotaru, A. E., Summers, Z. M., Shrestha, M., Liu, F., and Lovley, D. R. (2013b). Transcriptomic and genetic analysis of direct interspecies electron transfer. Appl. Environ. Microbiol. 79, 2397–2404. doi: 10.1128/AEM.03837-12
Smith, J. A., Nevin, K. P., and Lovley, D. R. (2015). Syntrophic growth via quinone-mediated interspecies electron transfer. Front. Microbiol. 6:121. doi: 10.3389/fmicb.2015.00121
Strycharz, S. M., Glaven, R. H., Coppi, M. V., Gannon, S. M., Perpetua, L. A., Liu, A., et al. (2011). Gene expression and deletion analysis of mechanisms for electron transfer from electrodes to Geobacter sulfurreducens. Bioelectrochemistry 80, 142–150. doi: 10.1016/j.bioelechem.2010.07.005
Summers, Z. M., Fogarty, H., Leang, C., Franks, A. E., Malvankar, N. S., and Lovley, D. R. (2010). Direct exchange of electrons within aggregates of an evolved syntrophic co-culture of anaerobic bacteria. Science 330, 1413–1415. doi: 10.1126/science.1196526
Thrash, J. C., and Coates, J. D. (2008). Review: direct and indirect electrical stimulation of microbial metabolism. Environ. Sci. Technol. 42, 3921–3931. doi: 10.1021/es702668w
Tremblay, P. L., Angenent, L. T., and Zhang, T. (2017). Extracellular electron uptake: among autotrophs and mediated by surfaces. Trends Biotechnol. 35, 360–371. doi: 10.1016/j.tibtech.2016.10.004
Tremblay, P. L., Summers, Z. M., Glaven, R. H., Nevin, K. P., Zengler, K., Barrett, C., et al. (2011). A c-type cytochrome and a transcriptional regulator responsible for enhanced extracellular electron transfer in Geobacter sulfurreducens uncovered by adapative evolution. Environ. Microbiol. 13, 13–23. doi: 10.1111/j.1462-2920.2010.02302.x
Tremblay, P. L., and Zhang, T. (2015). Electrifying microbes for the production of chemicals. Front. Microbiol. 6:201. doi: 10.3389/fmicb.2015.00201
Uchiyama, T., Ito, K., Mori, K., Tsurumaru, H., and Harayama, S. (2010). Iron-corroding methanogen isolated from a crude-oil storage tank. Appl. Environ. Microbiol. 76, 1783–1788. doi: 10.1128/AEM.00668-09
Ueki, T., Didonato, L. N., and Lovley, D. R. (2017). Toward establishing minimum requirements for extracellular electron transfer in Geobacter sulfurreducens. FEMS Microbiol. Lett. 364:fnx093. doi: 10.1093/femsle/fnx093
Ueki, T., Nevin, K. P., Woodard, T. L., and Lovley, D. R. (2016). Genetic switches and related tools for controlling gene expression and electrical outputs of Geobacter sulfurreducens. J. Ind. Microbiol. Biotechnol. 43, 1561–1575. doi: 10.1007/s10295-016-1836-5
Keywords: anaerobic respiration, electromicrobiology, bioelectrochemical, citrate lyase, electrosynthesis
Citation: Ueki T, Nevin KP, Woodard TL, Aklujkar MA, Holmes DE and Lovley DR (2018) Construction of a Geobacter Strain With Exceptional Growth on Cathodes. Front. Microbiol. 9:1512. doi: 10.3389/fmicb.2018.01512
Received: 04 May 2018; Accepted: 18 June 2018;
Published: 13 July 2018.
Edited by:
Yang-Chun Yong, Jiangsu University, ChinaCopyright © 2018 Ueki, Nevin, Woodard, Aklujkar, Holmes and Lovley. This is an open-access article distributed under the terms of the Creative Commons Attribution License (CC BY). The use, distribution or reproduction in other forums is permitted, provided the original author(s) and the copyright owner(s) are credited and that the original publication in this journal is cited, in accordance with accepted academic practice. No use, distribution or reproduction is permitted which does not comply with these terms.
*Correspondence: Derek R. Lovley, ZGxvdmxleUBtaWNyb2Jpby51bWFzcy5lZHU=
†Present address: Muktak A. Aklujkar, Applied Biological Materials Inc., Richmond, BC, Canada