- 1Water Research Institute, IRSA–CNR, Rome, Italy
- 2Department of Chemistry, Sapienza University of Rome, Rome, Italy
This study investigated the organohalide-respiring bacteria (OHRB) and the supporting microbial populations operating in a pilot scale plant employing poly-3-hydroxybutyrate (PHB), a biodegradable polymer produced by bacteria from waste streams, for the in situ bioremediation of groundwater contaminated by chlorinated solvents. The bioremediation was performed in ground treatment units, including PHB reactors as slow release source of electron donors, where groundwater extracted from the wells flows through before the re-infiltration to the low permeability zones of the aquifer. The coupling of the biological treatment with groundwater recirculation allowed to drastically reducing the contamination level and the remediation time by efficiently stimulating the growth of autochthonous OHRB and enhancing the mobilization of the pollutants. Quantitative PCR performed along the external treatment unit showed that the PHB reactor may efficiently act as an external incubator to growing Dehalococcoides mccartyi, known to be capable of fully converting chlorinated ethenes to innocuous end-products. The slow release source of electron donors for the bioremediation process allowed the establishment of a stable population of D. mccartyi, mainly carrying bvcA and vcrA genes which are implicated in the metabolic conversion of vinyl chloride to harmless ethene. Next generation sequencing was performed to analyze the phylogenetic diversity of the groundwater microbiome before and after the bioremediation treatment and allowed the identification of the microorganisms working closely with organohalide-respiring bacteria.
Introduction
Over the past 20 years, intense research efforts have been devoted to elucidate the overall mechanisms underlying the biological reductive dechlorination (RD) process and to develop effective technologies for the in situ remediation of contaminated sites (Lorenz and Löffler, 2016).
The RD process is performed by specialized organohalide-respiring bacteria (OHRB) which enable the complete and efficient detoxification of a variety of aliphatic and aromatic chlorinated pollutants. Among the many bacterial species that are now known to reductively transform organohalides, Dehalococcoides mccartyi is considered as the biomarker of the process due to the unique ability of members of this genus to fully convert chlorinated solvents to harmless products through the activity of a class of enzymes called reductive dehalogenases (RDases) (Richardson, 2013). They are involved in the metabolic dechlorination of PCE or TCE to VC (TceA), of cis-DCE to VC and ethene (BvcA and VcrA) and are coded by the corresponding genes tceA, bvcA, and vcrA (Lee et al., 2006).
Recently, several site investigations showed the relevance of the geochemical monitoring integrated with the biomolecular analysis of both OHRB and flanking microbial communities (Kotik et al., 2013; Kao et al., 2016; Atashgahi et al., 2017). The use of an integrated monitoring approach may reduce uncertainties about the ongoing groundwater processes and allow an efficient long-term management of the remedial action (Majone et al., 2015).
In situ stimulation of native OHRB through the addition of fermentable substrates represents one of the main approaches used for remediating chlorinated solvents contaminated aquifers (Steffan and Schaefer, 2016). Recently, poly-β-hydroxy-butyrate (PHB) was shown to be effective as a slow-release electron donor for the RD process (Aulenta et al., 2008; Pierro et al., 2017). PHB is an inert, biocompatible and fully biodegradable material which has been proposed for several attractive biotechnological applications (Williams and Martin, 2005). It is a polyester synthesized as a carbon and energy reserve material by a wide number of prokaryotes. More than 300 species, mainly of bacteria, have been reported to produce these polymers (Olivera et al., 2001; Chanprateep, 2010; Centeno-Leija et al., 2014). PHB is industrially produced by microbial fermentation using bacterial strains, cultivated on inexpensive carbon sources such as beet and cane molasses, corn starch, alcohols, and vegetable oils (Lee, 1996; Chen, 2009, 2010; Chanprateep, 2010; Peña et al., 2011, 2014).
In order to act as slow-release electron donor, PHB is enzymatically hydrolyzed to 3-hydroxybutyrate (HB) which is then converted to acetate and H2 through β-oxidation.
To date, only a few laboratory studies investigated the efficacy of PHB as electron donor in the RD process (Aulenta et al., 2008; Baric et al., 2012, 2014) and the first pilot scale PHB application was only recently documented (Pierro et al., 2017). A combination of a groundwater circulation wells (GCWs) with an external treatment unit, including a PHB reactor allowing continuous delivering of electron donor in the contaminated aquifer, was installed at a chlorinated solvent contaminated aquifer where partial biological RD quantitatively transformed higher chlorinated ethenes and ethanes (utilized at the site in industrial degreasing operation) in the less chlorinated cis-DCE and VC.
The effectiveness of PHB as a suitable electron donor source for enhancing the in situ RD of cis-DCE to non-toxic ethene in low permeability contaminated aquifer was clearly demonstrated during the plant operation and the RD process was found to be mirrored by the occurrence of both D. mccartyi and reductive dehalogenase genes (Pierro et al., 2017).
As an unexpected effect of the continuous recirculation of the contaminated groundwater through the external treatment unit, the occurrence of a biological dechlorination activity was revealed, after around 200 days of operation, both in the PHB and zero valent iron (ZVI) reactors with the almost quantitative removal of the extracted cis-DCE and VC. The stimulation of the biological reductive activity resulted from the in situ enhancement of the RD by the GCW operation. By this regard, extracted groundwater, enriched with OHRB as result of the electron donor continuous amendment, passes through the external reactors in the treatment unit where dechlorinating microorganisms find the optimal growth conditions (reductive redox conditions and electron donor concentration in the PHB and ZVI reactors).
In this paper, we report the throughout investigation on the microbial changes and the behavior of OHRB along the external operation unit of the pilot plant, focusing in particular on the PHB reactor and on the structure and role of the microbial community involved in the RD process driven by the fermentation of this slow release carbon source.
Materials and Methods
Pilot Field Test
As described in Pierro et al. (2017), the process is carried out by extracting contaminated water through a multi-screened 30-m-deep IEG-GCW® and transferring it to an external treatment unit which comprises a sand tank (for suspended solid filtration), a reactor containing the fermentable PHB polymer and one with ZVI for the removal of the extracted contaminants before reinjection. Valves were installed along the plant for sample collection and analyses (Figure 1). IEG-GCW® plant was designed based on geological and hydrogeological conditions of the site and it consists in a single borehole screened at three different depths separated by packers (first permeable layer, low permeability intermediate layer, and deep permeable layer). Three centrifugal pumps were installed on the surface. Two pumps extracted groundwater from the deep permeable layer (from 22 to 26 m below the ground surface, bgs) and the low permeability intermediate layer, where a significant mass of chlorinated solvents was strongly retained by aging phenomena (from 15 to 19 m bgs). After passing through an external treatment unit, pumped groundwater is discharged back into the aquifer through the upper screened section (from 8 to 12 m bgs), thus creating two circulation groundwater cells (Figure 1). Groundwater recirculation by GCW coupled with electron donor continuous amendment (by the PHB reactor) effectively allowed chlorinated solvents mobilization and in situ RD stimulation in the low accessible heavily contaminated low permeable aquifer zones.
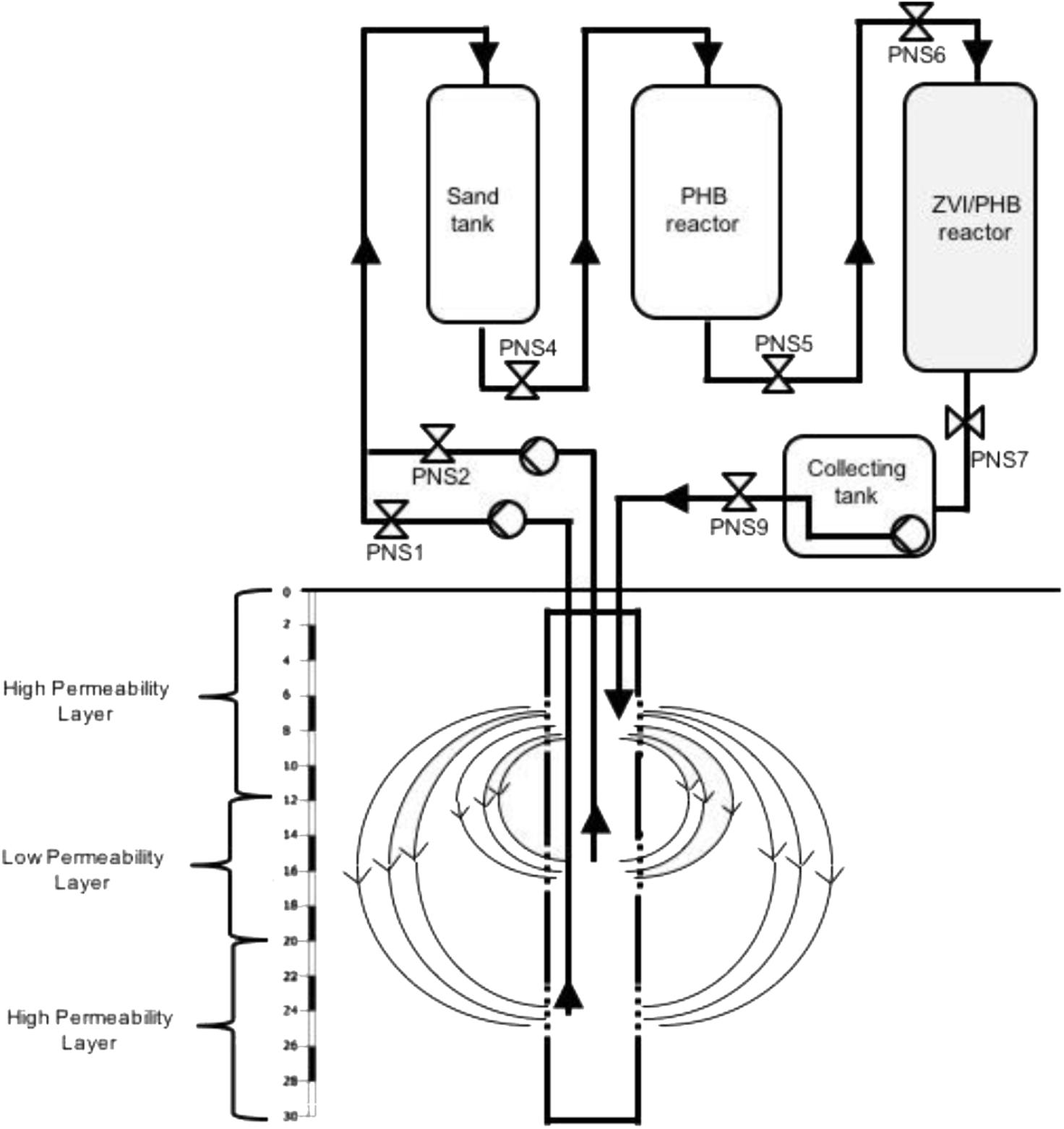
FIGURE 1. Schematic illustration showing the pilot plant (GCW and external treatment unit) and the position of the sampling valves (PN).
Chemical Analysis
Chlorinated solvents were determined by headspace gas chromatographic analysis. Groundwater samples were collected directly from gate valves (PNS1-7) in 10 ml pyrex vials. Each vial was completely filled to leave almost no headspace, sealed with a Teflon-faced butyl septum and transported to the laboratory. In order to create headspace and to perform gas chromatographic analysis, 1 ml of each groundwater sample was transferred into a second 10 ml pyrex vial sealed with a Teflon-faced butyl septum. Headspace analysis of chlorinated compounds was performed using a gas chromatograph (Master DANI) equipped with a DANI 86.50 headspace autosampler. The chromatograph was fitted with a TRB 624 (75 m × 0.53 mm i.d) capillary column and a flame ionization detector (FID, 300°C). Sample injection was operated in splitless mode, where the injector temperature was set at 180°C. Helium was used as carrier gas at a constant flow of 10 mL min-1 and the GC oven was temperature programmed as follows: 50°C for 0.5 min increasing at 15°C min-1 to 180°C for 0 min then increasing at 40°C min-1 to 210°C for 0 min. The headspace autosampler conditions were: oven temperature 80°C, manifold temperature 120°C, transfer line temperature 180°C, shaking soft. The GC was previously calibrated with standard cis-DCE and VC concentrations over a linear response range.
Sampling for Biomolecular Analysis
Samples for biomolecular analysis (500 mL of groundwater) were collected before the bioremediation treatment (T = 0) from the groundwater wells PNS1 (groundwater from high permeable zone with low concentration of cis-DCE and VC) and PNS2 (groundwater from the low permeable zone with high concentration of cis-DCE and VC). Water samples were taken also after 570 days of plant operation from PNS1, PNS2 and from sampling points located in the external unit of the pilot plant PNS4 (out of the sand tank), PNS5, and PNS6 (out of the PHB reactor unit), PNS7 (out of the ZVI/PHB reactor unit).
Each groundwater sample was filtered on polycarbonate filters (pore size 0.22 μm, 47 mm diameter, Nuclepore) to harvest the biomass and DNA was extracted by Power Soil DNA extraction kit (MoBio, Italy) following the manufacturer’s instructions. Purified DNA from each sample was eluted in 100 μL sterile Milli-Q and stored at -20°C until further analysis.
Quantitative PCR
Quantitative PCR (qPCR) targeting D. mccartyi 16S rRNA genes and reductive dehalogenase genes tceA, bvcA, vcrA was conducted on DNA extracted from PNS1 and PNS2 before the bioremediation treatment and from the sampling points located in the external unit of the treatment plant (PNS1, PNS2, PNS4, PNS5, PNS7) after 570 days of operation. qPCR absolute quantification with TaqMan® chemistry was applied. Reactions were performed in 20 μL total volume of SsoAdvancedTM Universal Probes Supermix (Bio-Rad, Italy), including 3 μL of DNA as template, 300 nM of each primer and 300 nM of TaqMan® probe composed by 6-carboxyfluorescein (FAM) as the 5′ end reporter fluorophore and N,N,N,N,-tetramethyl-6-carboxyrhodamine (TAMRA) as the 3′ end quencher. Primers and probes used for each reaction are listed in Supplementary Table S1. Standard curves for the absolute quantification were constructed by using the long amplicons method previously reported in Matturro et al. (2013). Each reaction was performed in triplicate with CFX96 TouchTM Real-Time PCR Detection System (Bio-Rad, Italy). Quantitative data were expressed as gene copy numbers L-1 and error bars were calculated with Microsoft Excel® on triplicate reactions for each sample.
Next Generation Sequencing (NGS)
Next generation sequencing (NGS) was performed on groundwater samples collected at PNS1 and PNS2 before the PHB treatment (T = 0) and at the outlet of the PHB reactor (PNS5) after the bioremediation treatment (570 days of plant operation).
10 ng of DNA extracted from each groundwater sample (500 mL) was used for NGS analysis. 16S rRNA Amplicon Library Preparation (V1–3) was performed as detailed in Matturro et al. (2017). The procedure for bacterial 16S rRNA amplicon sequencing targeting the V1–3 variable regions is based on Caporaso et al. (2012), using primers adapted from the Human Gut Consortium (Ward et al., 2012). PCR reactions were performed in 25 μL reaction volume containing Phusion Master Mix High Fidelity (Thermo Fisher Scientific, United States) and 0.5 μM final concentration of the library adaptors with V1–3 primers (27F: 5′-AGAGTTTGATCCTGGCTCAG-3′; 534R: 5′-ATTACCGCGGCTGCTGG-3′). All PCR reactions were run in duplicate and pooled afterward. The amplicon libraries were purified using the Agencourt® AMpure XP bead protocol (Beckmann Coulter, United States). Library concentration was measured with Qubit 3.0 fluorometer (Thermo Fisher Scientific, United States). The purified libraries were pooled in equimolar concentrations and diluted to 4 nM. 10% Phix control library was spiked in to overcome low complexity issue often observed with amplicon samples. The samples were paired end sequenced (2 × 301 bp) on a MiSeq (Illumina) using a MiSeq Reagent kit v3, 600 cycles (Illumina, United States) following the standard guidelines for preparing and loading samples on the MiSeq.
Next generation sequencing secondary data were processed and analyzed using QIIME2 software tools 2018.2 release (Caporaso et al., 2010). The reads were demultiplexed using demux plugin1, denoized, dereplicated and chimera-filtered using DADA2 algorithm (Callahan et al., 2016). The taxonomic analysis was based on a Naïve–Bayes classifier trained on 16S rRNA gene OTUs clustered at 99% similarities within the Silva 128 database (Quast et al., 2013). The alpha-diversity analysis was performed by PAST version 2.17 (Hammer et al., 2001) using total OTUs generated from each sample. Rarefaction curves were computed using the Vegan R package.
16S rRNA Gene Clone Library
A 16S rRNA gene clone library was obtained from DNA extracted at PNS5 sampling point after 570 days of plant operation. DNA was amplified using primers 27f (5′-AGAGTTTGATCMTGGCTCAG-3′) and 1492r (5′-TACGGYTACCTTGTTACGACTT-3′) using Hot Start Taq98 (Lucigen, Italy). PCR reactions were performed with the following cycles: 2 min at 98°C, 38 cycles for 30 s at 98°C, 30 s at 58°C, 1 min at 72°C and final 15 min at 72°C. PCR products were purified using the QIAquick® PCR purification kit (Qiagen, Milan, Italy). Cloning of PCR products was carried out using pGEM-T Easy Vector System (Promega, Italy) into Escherichia coli JM109 competent cells (Promega, Italy) according to the manufacturer’s instructions. Positive inserts were amplified from recombinant plasmids obtained from white colonies by PCR using the sequencing primers T7f (5′-TAATACGACTCACTATAGGG-3′) and M13r (5′-TCACACAGGAAACAGCTATGAC-3′). PCR amplicons of 1,465 bp length were purified using the QIAquick PCR purification kit (Qiagen, Milan, Italy) and sequenced with primers 530F (5′-GTGCCAGCMGCCGCCG-3′) and 907R (5′-CCGTCAATTCMTTTRAGTTT-3′). A total of 100 clones were screened by PCR and multiple sequence alignments were performed with ClustalW2 to check the similarity among the sequences. The 16S rRNA gene sequences were deposited in the GenBank database under accession numbers from MG251533 to MG251561.
Phylogenetic Analysis
The 16S rRNA sequences were analyzed with the ARB software (Ludwig et al., 2004) using the SILVA 16S rRNA SSU Reference database release 102 (Pruesse et al., 2007). Sequences were screened for chimeras using DECIPHER (Wright et al., 2012). The phylogenetic tree was constructed using the maximum likelihood method implemented in the program RAxML (Stamatakis et al., 2008). Bootstrap analyses were conducted using 1,000 resampling replicates. Planctomycetes was chosen as outgroup.
Results
External Treatment Unit Performances After 200 Days of Operation
Profiles of cis-DCE and VC concentration along the external treatment unit at different operation days are shown in Figure 2. After about 200 days of continuous operations, cis-DCE concentration starts to decline in the PHB reactor and was almost quantitatively removed at the outlet of the ZVI/PHB reactor. VC concentration slightly increased in the PHB reactor and was then completely removed after passing through the ZVI/PHB reactor. Considering that no chemical reductants were present in the PHB reactor and that VC is not efficiently reduced by ZVI reactor, this behavior could be explained only by the occurrence of the biological RD of both cis-DCE and VC.
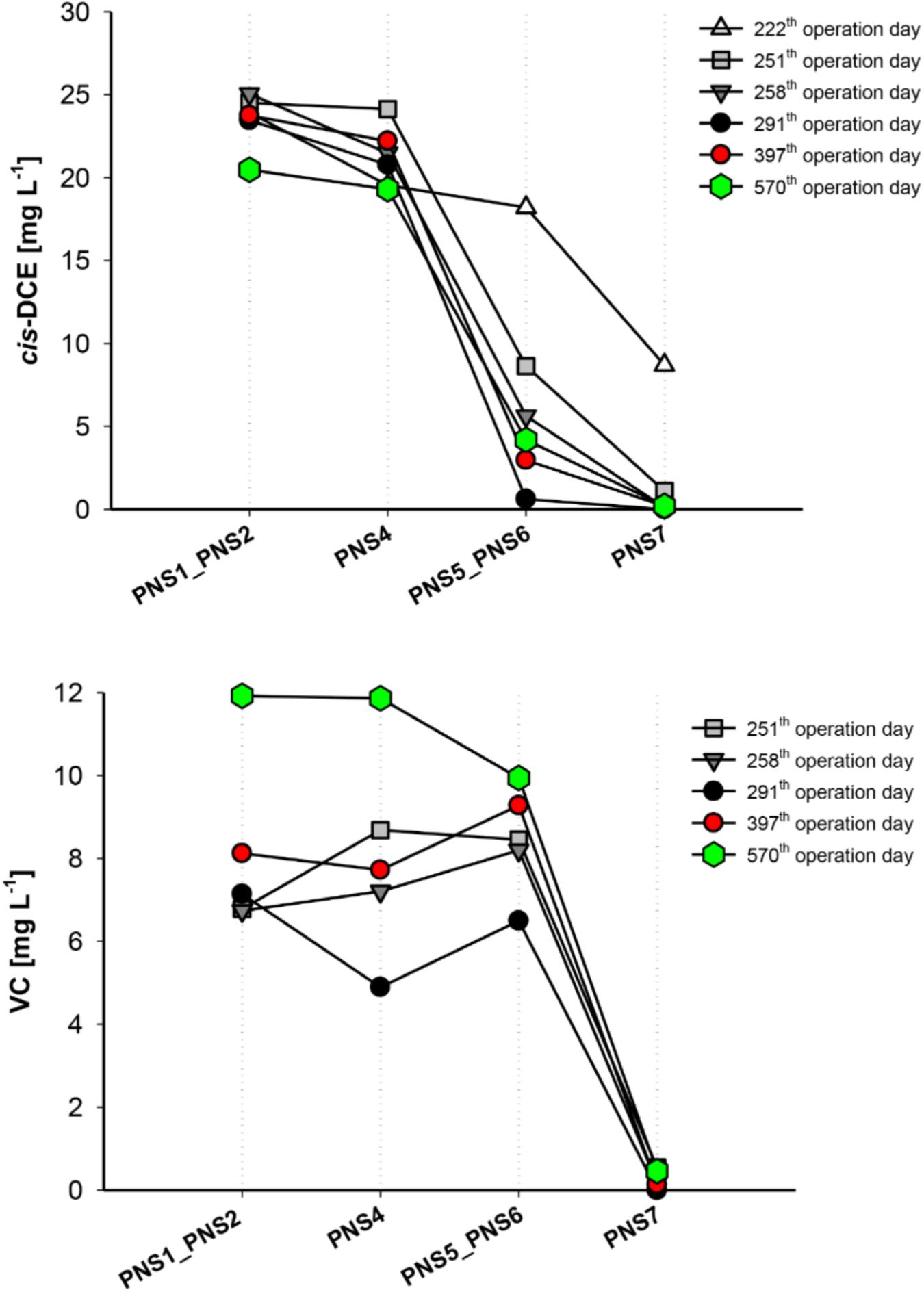
FIGURE 2. cis-DCE and VC reductive dechlorination at different sampling times over long-term plant operation. PNS1 and PNS2 average concentrations are shown.
D. mccartyi and Reductive Dehalogenase Genes Quantification
The biomarkers of RD process, including D. mccartyi 16S rRNA and functional RDase genes tceA, bvcA, and vcrA, were quantified by qPCR in the groundwater samples collected before the treatment and after 19 months of plant operation (T = 570 days) at different sampling points along the external unit of the pilot plant (PNS1, PNS2, PNS4, PNS5, PNS6, PNS7). As shown in Supplementary Figure S1, similar D. mccartyi abundances were found at PNS1 and PNS2 before treatment (2.24E + 06 and 4.02E + 06 16S rRNA gene copies L-1, respectively). They were mainly composed by D. mccartyi strains carrying tceA and vcrA (PNS1: 2.02E + 06 tceA gene copies L-1; 1.12E + 06 vcrA gene copies L-1; PNS2: 3.06E + 06 tceA gene copies L-1; 3.04E + 06 vcrA gene copies L-1).
At the end of the treatment, D. mccartyi increased in both samples (3.12E + 07 and 7E + 07 gene copies L-1 in PNS1 and PNS2, respectively) and remained quite unvaried after the groundwater flew through the inert sand tank PNS4 (6.10E + 07 gene copies L-1). Interestingly, D. mccartyi increased at the outlet of the PHB reactor (PNS5) where a total of 2.54E + 08 gene copies L-1 was found. At the sampling point located before the ZVI/PHB reactor (sampling point PNS6) D. mccartyi abundance was similar to PNS5 (2.49E + 08 gene copies L-1) and slightly decreased to 6.72E + 07 gene copies L-1 before the re-inoculation of the groundwater into the aquifer (sampling point PNS7).
In line with chemical data, D. mccartyi strains carrying bvcA genes represented the main component with only a limited occurrence of vcrA and tceA genes along the all external treatment unit (Figure 3).
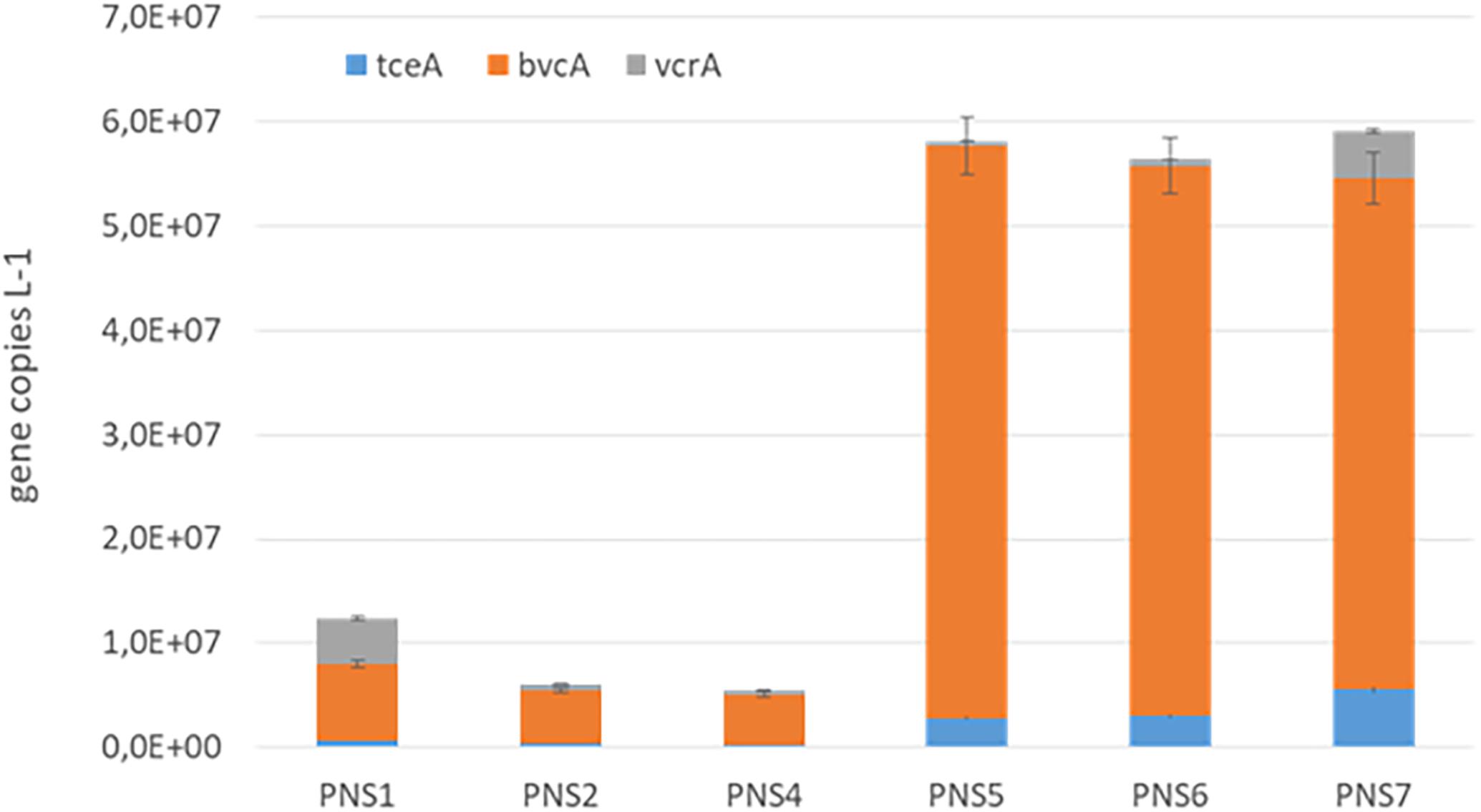
FIGURE 3. Quantification of D. mccartyi strains carrying tceA, bvcA, and vcrA reductive dehalogenase genes along the external units of the pilot plant at 570 days of the plant operation.
Microbial Community Composition of the PHB Reactor
The impact of the treatment on the structure and composition of the groundwater indigenous microbial communities was assessed by 16S rRNA gene high-throughput sequencing.
The analysis performed on groundwater samples collected from PNS1, PNS2, and PNS5 yielded between 63,546 and 108,169 sequence reads after bioinformatic processing (Supplementary Table S2). Rarefaction curves showed that the reads obtained were sufficient to capture sample diversity (Supplementary Figure S2).
As shown in Supplementary Figure S3, the contaminated groundwater before plant operation was mainly composed by Proteobacteria (∼50% of total OTUs in PNS1 and ∼67% of total OTUs in PNS2) whereas members of Chloroflexi phylum represented max ∼2% of total OTUs in both groundwater samples. Interestingly, sequences affiliated to Parcubacteria were found at high abundance (∼22%) in the groundwater from high permeable zone with low concentration of chlorinated contaminants (PNS1). Members of Parcubacteria were recently identified by phylogenetic analysis of 16S rRNA genes recovered from environmental samples and metabolic predictions indicate that they have very restricted metabolic potential, largely based on fermentation (Castelle et al., 2017).
After long-term operation (570 days), the microbiome composition of groundwater drastically changed with the marked increase of Chloroflexi (Figure 4). In detail, Chloroflexi represented up to 32% of total OTUs and included members of Dehalococcoidaceae (21% of total OTUs, mostly composed by sequences affiliated to D. mccartyi genus) and Anaerolinaceae (9% of total OTUs, mainly Leptolinea and Pelolinea genera) families (Figure 4). Other phyla found in PNS5 were affiliated to Proteobacteria (20% of total OTUs, including 9% of Epsilonproteobacteria, and 5% of Deltaproteobacteria), Bacteroidetes (15%), Firmicutes (7%), Spirochaetes (6%), Cloacimonetes (5%), and Actinobacteria (3%). Low-abundance OTUs were affiliated to Microgenomates (2.5%), Alphaproteobacteria (2.0%), Caldithrix (2%) and to a variety of taxa occurring at <1% of total OTUs (∼12%) including sequences affiliated to poorly characterized bacterial lineages such as Aminicenantes, Caldiserica, Parcubacteria, and Peregrinibacteria.
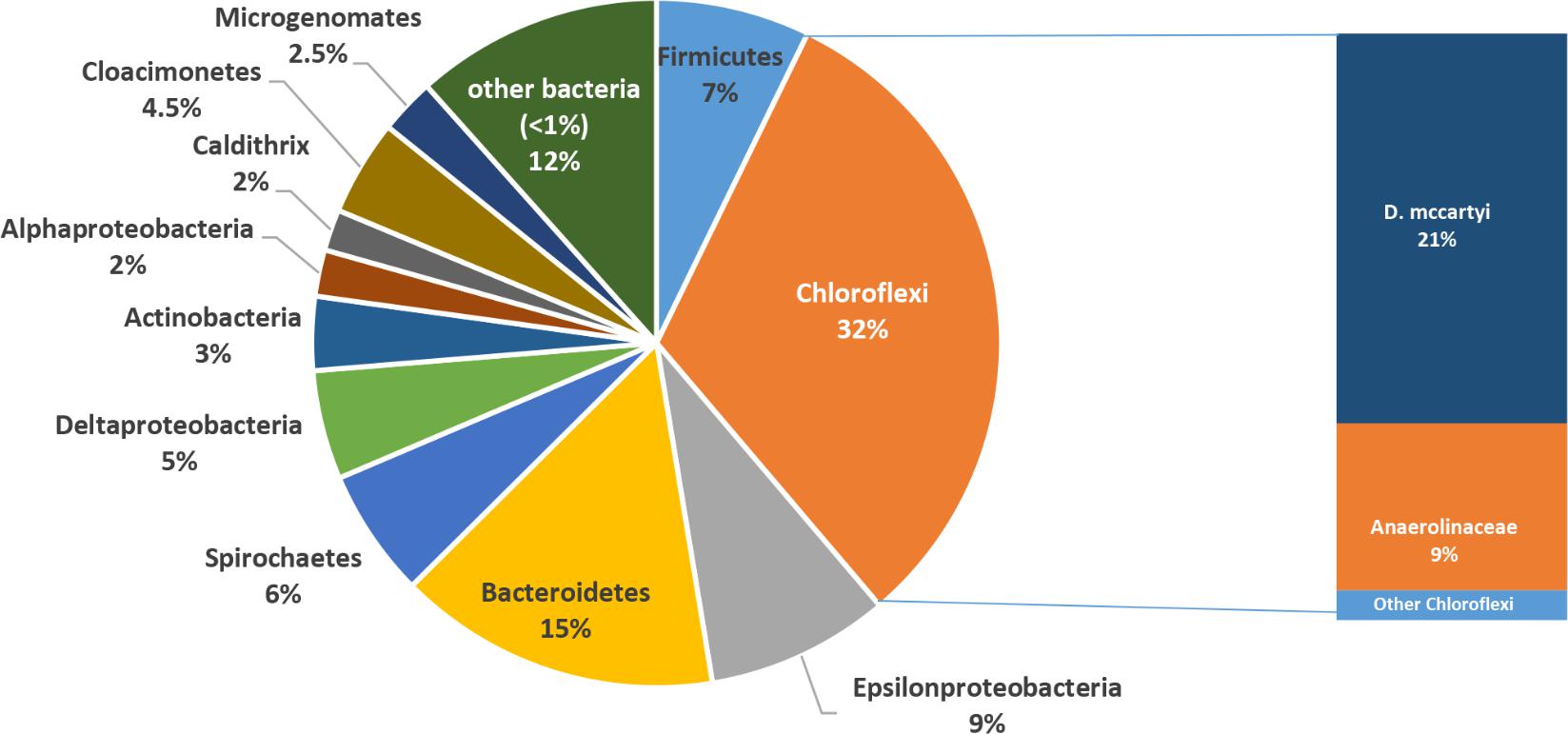
FIGURE 4. Microbial community structure of the treated groundwater (PNS5 sampling point) after long-term plant operation.
16S rDNA Clone Library
The characterization of the microbial communities selected in the PHB reactor was further performed by constructing 16S rDNA clone library to recover near-full-length 16S rRNA gene sequences. A total of 100 clones containing inserts with the expected size (about 1.5 kb) were selected from the total library, partially sequenced and sequence identities determined (Table 1). Partial sequences differing by ≤2% were considered a single relatedness group and representatives of most of these groups were fully sequenced on both strands. Figure 5 shows the phylogenetic tree of representative full-length sequences in the contest of currently recognized bacterial phyla. The analysis of the sequences showed the occurrence of microorganisms mostly with low 16S rRNA gene similarity (<90%) to already previously identified and/or cultured neighbors (Figure 5). The highest number of the screened clones belonged to Lentimicrobiaceae (20%) and Nitrospiraceae (20%) families followed by Dehalococcoidaceae (15%), Prolixibacteraceae (14%), Syntrophaceae (8%), Coriobacteriaceae (6%), Desulfovibrionaceae (4%) and Desulfuromonadaceae (4%) families.
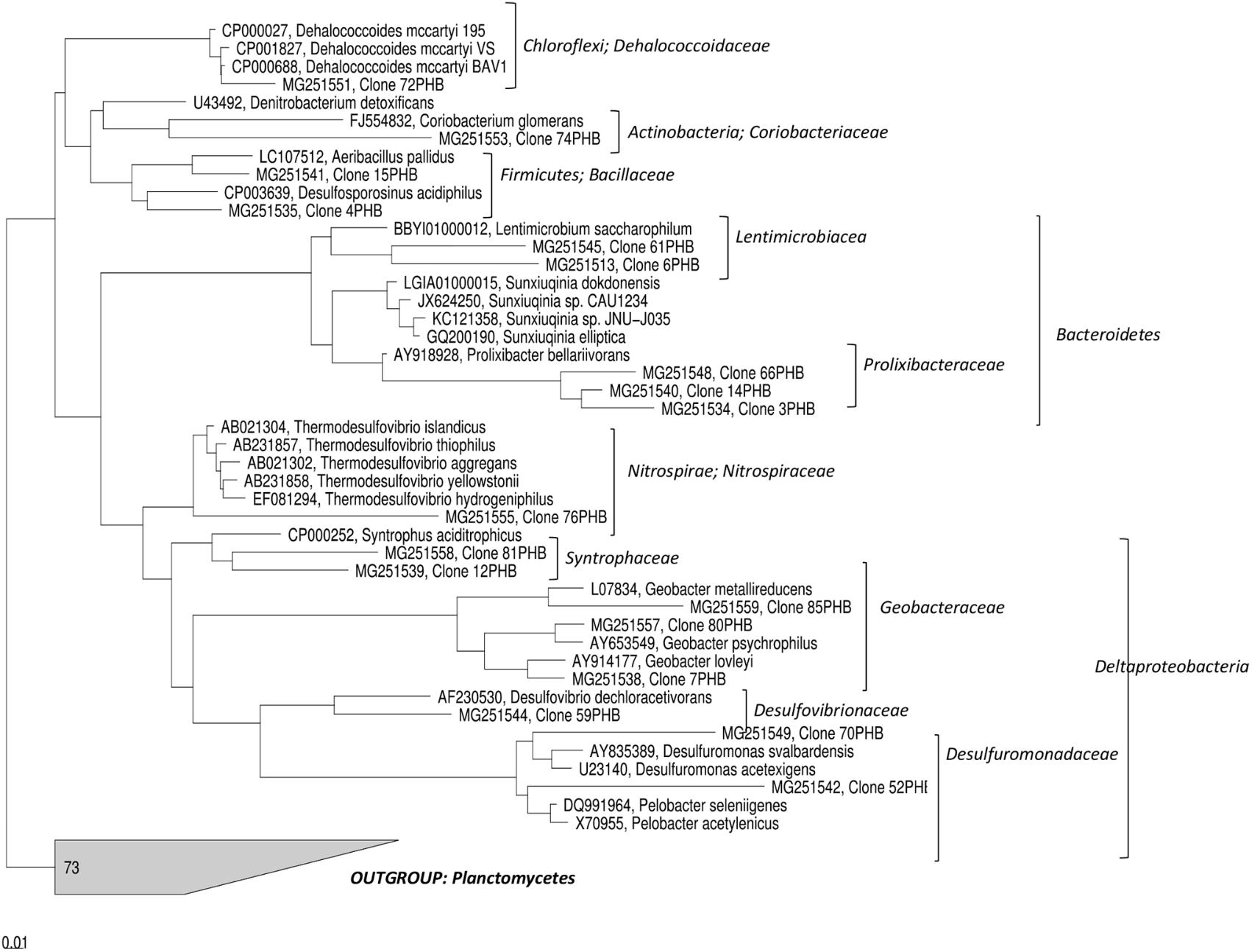
FIGURE 5. Phylogenetic tree of 16S rRNA gene sequences obtained from the clone library constructed with DNA extracted from PNS5 sampling point after 570 days of treatment.
In addition, other bacterial taxa present in decreasing abundance were Geobacter species, Dehalococcoides mccartyi, and Aeribacillus pallidus (Table 1).
Discussion
The reliable and robust application of bioremediation strategies requires the comprehension of the microorganisms responsible for the contaminant degradation as well as the role of the flanking microbial communities. The detailed description of the groundwater microbial community able to reduce chlorinated solvents by using PHB as a slow-release electron donor source is reported in this study. The pilot plant was installed in a site heavily contaminated by cis-DCE and was composed by a GCW system connected to an external treatment unit where a PHB tank was installed to deliver fermentation products directly through the low permeable zones and improve the distribution of soluble electron donors. This coupled system allowed to create an effective three-dimensional circulation cell in the aquifer reaching less permeable layers where significant masses of contaminants are potentially accumulated (Pierro et al., 2017).
Overall, the treatment changed the groundwater microbiome with the remarkable increase of specialized OHRB able to dechlorinate cis-DCE and VC to ethene. This finding is in line with the evidences obtained from a preliminary laboratory treatability study, performed on soil core and groundwater samples taken from the same site, which indicated PHB as an effective source of electron donor able to stimulate the RD until the formation of ethene via transient VC formation (Pierro et al., 2017).
The analysis conducted along the external unit of the pilot plant employing PHB for the biological RD has demonstrated that the PHB reactor may efficiently act as an external incubator to growing D. mccartyi. Indeed, a marked increase of D. mccartyi strains, able to dechlorinate VC metabolically and more efficiently produce ethene, was found in the outlet streams of the PHB and ZVI/PHB reactors.
Additionally, the treatment stimulated the growth of a wide range of putative fermentative bacteria. High throughput sequencing revealed the occurrence of sequences belonging to Leptolinea genus. L. tardivitalis, the sole isolate available so far, is an obligate anaerobe able to ferment sugars and proteinaceous carbon sources and produce as by-products a variety of volatile fatty acids and hydrogen (Yamada et al., 2006). Other Chloroflexi found after the PHB treatment were affiliated to Pelolinea, previously described to have several phenotypic traits in common with members of the class Anaerolineae, e.g., strictly anaerobic growth and chemo-organotrophic metabolism with sugars and polysaccharides (Imachi et al., 2014). Additionally, sequences belonging to Bacteroidetes phylum were found at high abundance by NGS (∼15% of total OTUs) mainly affiliated to Lentimicrobiaceae. The further analysis of the near-full-length 16S rRNA gene sequences, generated by the clonal analysis, showed that most of them have a low similarity to already previously identified and/or cultured neighbors (≤90% 16S rRNA gene similarity). Among others, the screened clones were indeed mostly affiliated to Lentimicrobiaceae and Prolixibacteraceae (Bacteroidetes phylum) (Table 1). Lentimicrobiaceae family contains strictly anaerobic and slow-growing bacteria able to ferment a wide range of compounds to acetate, malate, propionate, formate and hydrogen (Sun et al., 2016). Within this family, Lentimicrobium saccharophilum, an anaerobic bacterium isolated from a reactor treating high-strength starch-based organic wastewater (Sun et al., 2016), displayed the highest sequence similarity (89–90%) with clones 6PHB and 61PHB. The sequences belonging to Prolixibacteraceae shared low levels of 16S rDNA sequence similarity (<90%) to known members of this family such as Sunxiuqinia, retrieved in anaerobic deep-sea environments (Qu et al., 2011) or Prolixibacter, fermentative bacterium isolated from a marine-sediment fuel cell (Holmes et al., 2007a), suggesting that they may belong to a novel taxonomic group at the genus level.
Additionally, a variety of sequences falling into taxonomic groups containing known sulfate- and Fe(III)- reducing bacteria was retrieved by the clonal analysis. They include members of Nitrospiraceae, mostly affiliated to the genus Thermodesulfovibrio (20% of the screened clones), and Syntrophaceaea family sharing 93–94% of sequence similarity with Syntrophus aciditrophicus, a fermenting bacterium able to grow on benzoate and certain fatty acids alone or in syntrophic association with hydrogen-consuming microorganisms (Elshahed and McInerney, 2001; Mouttaki et al., 2007). Furthermore, sequences related to Denitrobacterium genus (Coriobacteriaceae family) were found. This genus comprises a single species, D. detoxificans, able to grow via anaerobic respiration oxidizing hydrogen, formate or lactate for reduction of various oxidized nitrogen compounds (Anderson et al., 2000). Both NGS and clonal analysis revealed the occurrence of Geobacteraceae often found dominating in iron-reducing settings particularly in environments affected by anthropogenic influences (Holmes et al., 2007b). The Geobacter genus comprises anaerobic, non-fermenting chemoorganotrophic microorganisms able to reduce insoluble Fe(III) and Mn(IV) by employing several mechanisms including extracellular electron transfer via electric conductive nanowires. Being Geobacter species physiologically versatile, they are employed in environmental biotechnology, such as the natural attenuation of organic matter, bioremediation of aromatic hydrocarbons, heavy metals and organohalides, and generating bioenergy in microbial fuel cells and microbial electrolysis cells (Holmes et al., 2007b).
To lesser extent (4% of total clone library), sequences affiliated to Desulfovibrionaceae family were found sharing 97% of 16S rRNA sequence similarity with Desulfovibrio dechloroacetivorans, a microorganism able to coupling acetate oxidation to RD for growth (Sun et al., 2000). This group comprises strictly anaerobic members with a respiratory type or fermentative type of metabolism and most species are chemoorganoheterotrophs. Additionally, the analysis revealed the occurrence of sequences affiliated to Desulfuromonadaceae family, whose closest relatives were members of Desulfuromonas genus, such as Desulfuromonas svalbardensis able to reduce Fe(III) using common fermentation products such as acetate, lactate, propionate, formate or hydrogen as electron donors (Vandieken et al., 2006), and Pelobacter genus including microorganisms with the ability to grow fermentatively on short-chain organic acids such as lactate, citrate and pyruvate such as Pelobacter seleniigenes (Narasingarao and Häggblom, 2007).
It is worth noting the occurrence of low-abundance OTUs affiliated to poorly characterized bacterial lineages such as Aminicenantes, Microgenomates, and Cloacimonetes phyla previously found in a wide variety of anaerobic environments including oil reservoirs and hydrocarbon-impacted sites (Farag et al., 2014; Hu et al., 2016; Stolze et al., 2017).
Conclusion
The study confirmed the efficacy of the technology to remediate aquifers contaminated by chloroethenes and gave access the less represented species of the contaminated aquifer likely interacting with dechlorinators for the removal of chlorinated compounds through a wide range of metabolic reactions driven by the availability of PHB fermentation by-products. The results of this work clearly sustain the use of PHB as environmentally sustainable source of electron donor for bioremediation treatment of sites contaminated by chlorinated compounds and indicate the importance to further gain more insights into the metabolism networks of groundwater bacteria stimulated by the presence of PHB for the biological RD.
Author Contributions
BM conducted qPCR, pyrosequencing, and analyzed the whole set of biological data. EF performed the bacterial 16S rRNA gene clonal analysis. LP performed the chemical analysis of the water samples and contributed to the interpretation of chemical data. SR and MPP conceived and coordinated the study. All authors contributed to the writing of the manuscript.
Conflict of Interest Statement
The authors declare that the research was conducted in the absence of any commercial or financial relationships that could be construed as a potential conflict of interest.
Supplementary Material
The Supplementary Material for this article can be found online at: https://www.frontiersin.org/articles/10.3389/fmicb.2018.01664/full#supplementary-material
Footnotes
References
Anderson, R. C., Rasmussen, M. A., Jensen, N. S., and Allison, M. J. (2000). Denitrobacterium detoxificans gen. nov., sp. nov., a ruminal bacterium that respires on nitrocompounds. Int. J. Syst. Evol. Microbiol. 50, 633–638. doi: 10.1099/00207713-50-2-633
Atashgahi, S., Lu, Y., Zheng, Y., Saccenti, E., Suarez-Diez, M., Ramiro-Garcia, J., et al. (2017). Geochemical and microbial community determinants of reductive dechlorination at a site biostimulated with glycerol. Environ. Microbiol. 19, 968–981. doi: 10.1111/1462-2920.13531
Aulenta, F., Fuoco, M., Canosa, A., Petrangeli Papini, M., and Majone, M. (2008). Use of poly-beta-hydroxy-butyrate as a slow-release electron donor for the microbial reductive dechlorination of TCE. Water Sci. Technol. 57, 921–925. doi: 10.2166/wst.2008.073
Baric, M., Majone, M., Beccari, M., and Petrangeli Papini, M. (2012). Coupling of polyhydroxybutyrate (PHB) and zero valent iron (ZVI) for enhanced treatment of chlorinated ethanes in permeable reactive barriers (PRBs). Chem. Eng. J. 19, 22–30. doi: 10.1016/j.cej.2012.04.026
Baric, M., Pierro, L., Pietrangeli, B., and Papini, M. P. (2014). Polyhydroxyalkanoate (PHB) as a slow-release electron donor for advanced in situ bioremediation of chlorinated solvent-contaminated aquifers. New Biotechnol. 31, 377–382. doi: 10.1016/j.nbt.2013.10.008
Callahan, B. J., McMurdie, P. J., Rosen, M. J., Han, A. W., Johnson, A. J., and Holmes, S. P. (2016). DADA2: high-resolution sample inference from Illumina amplicon data. Nat. Methods 13, 581–583. doi: 10.1038/nmeth.3869
Caporaso, J. G., Kuczynski, J., Stombaugh, J., Bittinger, K., Bushman, F. D., Costello, E. K., et al. (2010). QIIME allows analysis of high-throughput community sequencing data. Nat. Methods 7, 335–336. doi: 10.1038/nmeth.f.303
Caporaso, J. G., Lauber, C. L., Walters, W. A., Berg-Lyons, D., Huntley, J., Fierer, N., et al. (2012). Ultra-high- throughput microbial community analysis on the Illumina HiSeq and MiSeq platforms. ISME J. 6, 1621–1624. doi: 10.1038/ismej.2012.8
Castelle, C. J., Brown, C. T., Thomas, B. C., Williams, K. H., and Banfield, J. F. (2017). Unusual respiratory capacity and nitrogen metabolism in a Parcubacterium (OD1) of the candidate phyla radiation. Sci. Rep. 7:40101. doi: 10.1038/srep40101
Centeno-Leija, S., Huerta-Beristain, G., Giles-Gómez, M., Bolivar, F., Gosset, G., and Martinez, A. (2014). Improving poly-3-hydroxybutyrate production in Escherichia coli by combining the increase in the NADPH pool and acetyl-CoA availability. Antonie Van Leeuwenhoek 105, 687–696. doi: 10.1007/s10482-014-0124-5
Chanprateep, S. (2010). Current trends in biodegradable polyhydroxyalkanoates. J. Biosci. Bioeng. 110, 621–632. doi: 10.1016/j.jbiosc.2010.07.014
Chen, G. (2009). A microbial polyhydroxyalkanoates (PHA) based bio-and materials industry. Chem. Soc. Rev. 38, 2434–2446. doi: 10.1039/b812677c
Chen, G. Q. (2010). “Plastics completely synthesized by bacteria: polyhydroxyalkanoates,” in Plastics from Bacteria: Natural Functions and Applications Microbiology Monographs, ed. G.-Q. Chen (Berlin: Springer-Verlag), 17–37.
Elshahed, M. S., and McInerney, M. J. (2001). Benzoate fermentation by the anaerobic bacterium Syntrophus aciditrophicus in the absence of hydrogen-using microorganisms. Appl. Environ Microbiol. 67, 5520–5525. doi: 10.1128/AEM.67.12.5520-5525.2001
Farag, I. F., Davis, J. P., Youssef, N. H., and Elshahed, M. S. (2014). Global patterns of abundance, diversity and community structure of the Aminicenantes (Candidate Phylum OP8). PLoS One 9:e92139. doi: 10.1371/journal.pone.0092139
Hammer,Ø., David Harper, A. T., and Ryan, P. D. (2001). PAST: paleontological statistics software package for education and data analysis. Palaeontol. Electron. 4:9.
Holmes, D. E., Nevin, K. P., Woodard, T. L., Peacock, A. D., and Lovley, D. R. (2007a). Prolixibacter bellariivorans gen. nov., sp. nov., a sugar-fermenting, psychrotolerant anaerobe of the phylum Bacteroidetes, isolated from a marine-sediment fuel cell. Int. J. Syst. Evol. Microbiol. 57, 701–707. doi: 10.1099/ijs.0.64296-0
Holmes, D. E., O’Neil, R. A., Vrionis, H. A., N’Guessan, L. A., Ortiz-Bernad, I., Larrahondo, M. J., et al. (2007b). Subsurface clade of Geobacteraceae that predominates in a diversity of Fe(III)-reducing subsurface environments. ISME J. 1, 663–677. doi: 10.1038/ismej.2007.85
Hu, P., Tom, L., Singh, A., Thomas, B. C., Baker, B. J., Piceno, Y. M., et al. (2016). Genome-resolved metagenomic analysis reveals roles for candidate phyla and other microbial community members in biogeochemical transformations in oil reservoirs. mBio 7:e01669-15. doi: 10.1128/mBio.01669-15
Imachi, H., Sakai, S., Lipp, J. S., Miyazaki, M., Saito, Y., Yamanaka, Y., et al. (2014). Pelolinea submarina gen. nov., sp. nov., an anaerobic, filamentous bacterium of the phylum Chloroflexi isolated from subseafloor sediment. Int. J. Syst. Evol. Microbiol. 64, 812–818. doi: 10.1099/ijs.0.057547-0
Kao, C. M., Liao, H. Y., Chien, C. C., Tseng, Y. K., Tang, P., Lin, C. E., et al. (2016). The change of microbial community from chlorinated solvent-contaminated groundwater after biostimulation using the metagenome analysis. J. Hazard. Mater. 302, 144–150. doi: 10.1016/j.jhazmat.2015.09.047
Kotik, M., Davidova, A., Voriskova, J., and Baldrian, P. (2013). Bacterial communities in tetrachloroethene-polluted groundwaters: a case study. Sci. Total Environ. 45, 517–527. doi: 10.1016/j.scitotenv.2013.02.082
Lee, P. K. H., Johnson, D. R., Holmes, V. F., He, J. Z., and Alvarez-Cohen, L. (2006). Reductive dehalogenase gene expression as a biomarker for physiological activity of Dehalococcoides spp. Appl. Environ. Microbiol. 72, 6161–6168. doi: 10.1128/AEM.01070-06
Lee, S. Y. (1996). Bacterial polyhydroxyalkanoates. Biotechnol. Bioeng. 49, 1–14. doi: 10.1002/(SICI)1097-0290(19960105)49:1<1::AID-BIT1>3.0.CO;2-P
Ludwig, W., Strunk, O., Westram, R., Richter, L., Meier, H., Yadhukumar, A., et al. (2004). ARB: a software environment for sequence data. Nucleic Acids Res. 32, 1363–1371. doi: 10.1093/nar/gkh293
Majone, M., Verdini, R., Aulenta, F., Rossetti, S., Tandoi, V., Kalogerakis, N., et al. (2015). In situ groundwater and sediment bioremediation: barriers and perspectives at European contaminated sites. N. Biotechnol. 32, 133–146. doi: 10.1016/j.nbt.2014.02.011
Matturro, B., Frascadore, E., and Rossetti, S. (2017). High-throughput sequencing revealed novel Dehalococcoidia in dechlorinating microbial enrichments from PCB-contaminated marine sediments. FEMS Microbiol. Ecol. 93:fix134. doi: 10.1093/femsec/fix134
Matturro, B., Heavner, G. L., Richardson, R. E., and Rossetti, S. (2013). Quantitative estimation of Dehalococcoides mccartyi at laboratory and field scale: comparative study between CARD-FISH and real time PCR. J. Microbiol. Methods 93, 127–133. doi: 10.1016/j.mimet.2013.02.011
Mouttaki, H., Nanny, M. A., and McInerney, M. J. (2007). Cyclohexane carboxylate and benzoate formation from crotonate in Syntrophus aciditrophicus. Appl. Environ. Microbiol. 73, 930–938. doi: 10.1128/AEM.02227-06
Narasingarao, P., and Häggblom, M. M. (2007). Pelobacter seleniigenes sp. nov., a selenate-respiring bacterium. Int. J. Syst. Evol. Microbiol. 57, 1937–1942. doi: 10.1099/ijs.0.64980-0
Olivera, E., Carnicero, D., Jodra, R., Miñambres, B., García, B., Abraham, G., et al. (2001). Genetically engineered Pseudomonas: a factory of new bioplastics with broad applications. Environ. Microbiol. 3, 612–618. doi: 10.1046/j.1462-2920.2001.00224.x
Peña, C., Castillo, T., García, A., Millán, M., and Segura, D. (2014). Biotechnological strategies to improve production of microbial poly-(3-hydroxybutyrate): a review of recent research work. Microb. Biotechnol. 7, 278–293. doi: 10.1111/1751-7915.12129
Peña, C., Castillo, T., Nuñez, C., and Segura, D. (2011). Bioprocess Design: Fermentation Strategies for Improving the Production of Alginate and Poly-β-Hydroxyalkanoates (PHAs) by Azotobacter vinelandii. Rijeka: INTECH, 217–242.
Pierro, L., Matturro, B., Rossetti, S., Sagliaschi, M., Sucato, S., Alesi, E., et al. (2017). Polyhydroxyalkanoate as a slow-release carbon source for in situ bioremediation of contaminated aquifers: from laboratory investigation to pilot-scale testing in the field. N. Biotechnol. 37, 60–68. doi: 10.1016/j.nbt.2016.11.004
Pruesse, E., Quast, C., Knittel, K., Fuchs, B. M., Ludwig, W., Peplies, J., et al. (2007). SILVA: a comprehensive online resource for quality checked and aligned ribosomal RNA sequence data compatible with ARB. Nucleic Acids Res. 35, 7188–7196. doi: 10.1093/nar/gkm864
Qu, L., Zhu, F., Hong, X., Gao, W., Chen, J., and Sun, X. (2011). Sunxiuqinia elliptica gen. nov., sp. nov., a member of the phylum Bacteroidetes isolated from sediment in a sea cucumber farm. Int. J. Syst. Evol. Microbiol. 61, 2885–2889. doi: 10.1099/ijs.0.026971-0
Quast, C., Pruesse, E., Yilmaz, P., Gerken, J., Schweer, T., Yarza, P., et al. (2013). The SILVA ribosomal RNA gene database project: improved data processing and web-based tools. Nucleic Acids Res. 41, 590–596. doi: 10.1093/nar/gks1219
Richardson, R. E. (2013). Genomic insights into organohalide respiration. Curr. Opin. Biotechnol. 24, 498–505. doi: 10.1016/j.copbio.2013.02.014
Stamatakis, A., Hoover, P., and Rougemont, J. (2008). A rapid bootstrap algorithm for the RAxML Web servers. Syst. Biol. 57, 758–771. doi: 10.1080/10635150802429642
Steffan, R. J., and Schaefer, C. E. (2016). “Current and future bioremediation applications: bioremediation from a practical and regulatory perspective,” in Organohalide-Respiring Bacteria, eds L. Adrian and F. E. Löffler (Berlin: Springer-Verlag), 517–540.
Stolze, Y., Bremges, A., Maus, I., Puhler, A., Sczyrba, A., and Schluter, A. (2017). Targeted in situ metatranscriptomics for selected taxa from mesophilic and thermophilic biogas plants. Microb. Biotechnol. 11, 667–679. doi: 10.1111/1751-7915.12982
Sun, B., Cole, J. R., Sanford, R. A., and Tiedje, J. M. (2000). Isolation and characterization of Desulfovibrio dechloracetivorans sp. nov., a marine dechlorinating bacterium growing by coupling the oxidation of acetate to the reductive dechlorination of 2-chlorophenol. Appl. Environ. Microbiol. 66, 2408–2413. doi: 10.1128/AEM.66.6.2408-2413.2000
Sun, L., Toyonaga, M., Ohashi, A., Tourlousse, D. M., Matsuura, N., Meng, X. Y., et al. (2016). Lentimicrobium saccharophilum gen. nov., sp. nov., a strictly anaerobic bacterium representing a new family in the phylum Bacteroidetes, and proposal of Lentimicrobiaceae fam. nov. Int. J. Syst. Evol. Microbiol. 66, 2635–2642. doi: 10.1099/ijsem.0.001103
Vandieken, V., Mussmann, M., Niemann, H., and Jørgensen, B. B. (2006). Desulfuromonas svalbardensis sp. nov. and Desulfuromusa ferrireducens sp. nov., psychrophilic, Fe(III)-reducing bacteria isolated from Arctic sediments, Svalbard. Int. J. Syst. Evol. Microbiol. 56, 1133–1139. doi: 10.1099/ijs.0.63639-0
Ward, D. V., Gevers, D., Giannoukos, G., Earl, A. M., Methé, B. A., Sodergren, E., et al. (2012). Evaluation of 16S rDNA-based community profiling for human microbiome research. PLoS One 7:e39315. doi: 10.1371/journal.pone.0039315
Williams, S., and Martin, D. (2005). Applications of polyhydroxyalkanoates (PHA) in medicine and pharmacy. Biolym. Online 2005, 91–103. doi: 10.1002/3527600035.bpol4004
Wright, E. S., Yilmaz, L. S., and Noguera, D. R. (2012). DECIPHER, a search-based approach to chimera identification for 16S rRNA sequences. Appl. Environ. Microbiol. 78, 717–725. doi: 10.1128/AEM.06516-11
Yamada, T., Sekiguchi, Y., Hanada, S., Imachi, H., Ohashi, A., Harada, H., et al. (2006). Anaerolinea thermolimosa sp. nov., Levilinea saccharolytica gen. nov., sp. nov. and Leptolinea tardivitalis gen. nov., sp. nov., novel filamentous anaerobes, and description of the new classes Anaerolineae classis nov. and Caldilineae classis nov. in the bacterial phylum Chloroflexi. Int. J. Syst. Evol. Microbiol. 56, 1331–1340. doi: 10.1099/ijs.0.64169-0
Keywords: reductive dehalogenation, D. mccartyi, PHB, reductive dehalogenase genes, groundwater circulation wells, bioremediation
Citation: Matturro B, Pierro L, Frascadore E, Petrangeli Papini M and Rossetti S (2018) Microbial Community Changes in a Chlorinated Solvents Polluted Aquifer Over the Field Scale Treatment With Poly-3-Hydroxybutyrate as Amendment. Front. Microbiol. 9:1664. doi: 10.3389/fmicb.2018.01664
Received: 30 March 2018; Accepted: 04 July 2018;
Published: 24 July 2018.
Edited by:
Shanquan Wang, Sun Yat-sen University, ChinaReviewed by:
Maurizio Petruccioli, Università degli Studi della Tuscia, ItalyHaitham Sghaier, Centre National des Sciences et Technologies Nucléaires, Tunisia
Copyright © 2018 Matturro, Pierro, Frascadore, Petrangeli Papini and Rossetti. This is an open-access article distributed under the terms of the Creative Commons Attribution License (CC BY). The use, distribution or reproduction in other forums is permitted, provided the original author(s) and the copyright owner(s) are credited and that the original publication in this journal is cited, in accordance with accepted academic practice. No use, distribution or reproduction is permitted which does not comply with these terms.
*Correspondence: Simona Rossetti, cm9zc2V0dGlAaXJzYS5jbnIuaXQ=