- 1Laboratorio de Bacteriología General, Instituto de Patobiología, Centro Nacional de Investigaciones Agropecuarias, Instituto Nacional de Tecnología Agropecuaria, Buenos Aires, Argentina
- 2Consejo Nacional de Investigaciones Científicas y Tecnológicas, Buenos Aires, Argentina
- 3Laboratorio de Resistencia Bacteriana, Cátedra de Microbiología, Universidad de Buenos Aires, Facultad de Farmacia y Bioquímica, Buenos Aires, Argentina
The use of antimicrobial growth promoters (AGPs) in sub-therapeutic doses for long periods promotes the selection of resistant microorganisms and the subsequent risk of spreading this resistance to the human population and the environment. Global concern about antimicrobial resistance development and transference of resistance genes from animal to human has been rising. The goal of our research was to evaluate the susceptibility pattern to different classes of antimicrobials of colistin-resistant Escherichia coli from poultry production systems that use AGPs, and characterize the resistance determinants associated to transferable platforms. E. coli strains (n = 41) were obtained from fecal samples collected from typical Argentine commercial broiler farms and susceptibility for 23 antimicrobials, relevant for human or veterinary medicine, was determined. Isolates were tested by PCR for the presence of mcr-1, extended spectrum β-lactamase encoding genes and plasmid-mediated quinolone resistance (PMQR) coding genes. Conjugation and susceptibility patterns of the transconjugant studies were performed. ERIC-PCR and REP-PCR analysis showed a high diversity of the isolates. Resistance to several antimicrobials was determined and all colistin-resistant isolates harbored the mcr-1 gene. CTX-M-2 cefotaximase was the main mechanism responsible for third generation cephalosporins resistance, and PMQR determinants were also identified. In addition, co-transference of the qnrB determinant on the mcr-1-positive transconjugants was corroborated, which suggests that these resistance genes are likely to be located in the same plasmid. In this work a wide range of antimicrobial resistance mechanisms were identified in E. coli strains isolated from the environment of healthy chickens highlighting the risk of antimicrobial abuse/misuse in animals under intensive production systems and its consequences for public health.
Introduction
Antimicrobial agents have been used extensively for prevention and treatment of infectious diseases in food animals (Dibner and Richards, 2005; Niewold, 2007). The concomitant risk of spreading antibiotic resistance to human population through the food supply chain and the environment is important since many classes of these antimicrobial agents are also used in human medicine. Therefore, increased global concern regarding development of antimicrobial resistance and transference of resistance genes from animals to humans has been rising (Ljungquist et al., 2016; Madec et al., 2017; Wang et al., 2017).
Various antimicrobials have been widely used by the poultry industry as antibiotic growth promoters (AGPs) since the 1950s. To reduce costs of production, AGPs have been added into feed to promote weight gain by optimizing feed conversion ratios (Moore and Evenson, 1946; Jukes et al., 1950). In contrast to therapeutic usages of antimicrobials that are administered at high doses for a limited period of time, AGPs are used in sub-therapeutic doses during longer periods. This situation is particularly favorable for the selection of resistant microorganisms (Diarra et al., 2007).
Any use of antimicrobial agents may contribute to clinical relevant antimicrobial resistance. One of the first findings that led to strong recommendations (and even banning) for the use of AGP in the European Union (EU) was the finding that administration of avoparcin, a glycopeptide AGP, was involved in emerging glycopeptide-resistant bacteria (Howarth and Poulter, 1996). In the same way, use of colistin as an AGP in livestock led to the emergence and silent dissemination of plasmid-mediated mechanisms involved with polymyxin resistance (Rhouma et al., 2016). International organizations responsible for human, animal health, and food production (World Health Organization-WHO/World Organization for Animal Health-OIE/ Food and Agriculture Organization-FAO) carried out systematic evaluations on the impact of veterinary antimicrobial resistance on public health, and they stated that the misuse and overuse of antimicrobials is accelerating the processes of antimicrobial resistance. As a result, this topic is now considered as one of the critical issues in developed and developing countries as indicated by the United Nations General Assembly in 2016.
As part of a technical support program to national poultry producers, our team conducted studies to understand the antimicrobial resistance evolution in food-borne bacteria under commercial production systems in Argentina. Our studies included the selection of Escherichia coli as an indicator microorganism and concluded that almost 50% of the strains were found to be resistant to colistin used as AGP (Dominguez et al., 2017) which was much higher than reported in studies published previously. Therefore, the aim of this work was to evaluate the susceptibility pattern to different classes of antimicrobials of colistin-resistant E. coli isolated from poultry production systems that use AGP, and to characterize the resistance determinants associated to transmissible elements.
Materials and Methods
Sampling and E. coli Isolation
Fresh fecal samples were collected from 129 commercial broiler farms located in the most relevant production areas of Argentina (Entre Rios and Buenos Aires Provinces). At the moment of the sampling, healthy 4–6 week-old broiler chickens were at the end of the rearing cycle in the farms (Dominguez et al., 2017). Each E. coli strain was isolated from a pool of 10 feces samples collected in different sections of each barn. All samples were placed into boxes containing ice packs and immediately transported to the laboratory to isolate the microorganism by culture on non-antibiotic-supplemented MacConkey agar plates at 37°C for 18–24 h. Isolates were initially selected by the morphology of the colonies and further identified by standard biochemical tests (Brenner and Farmer, 2015). According to the size of the farms, a fixed number of isolates were arbitrarily selected: 2 isolates from small (less than 50,000 birds), 3 from medium (between 50,000 and 150,000 birds) and 6 from large (more than 150,000 birds) farms. Overall 304 E. coli isolates were obtained (Dominguez et al., 2017). In the present study a subset of 31 strains resistant to colistin and 10 susceptible -according to EUCAST criteria- were analyzed (EUCAST 2017)1. These strains were isolated from 11 farms belonging to 3 different integrated companies located at Entre Rios and Buenos Aires Provinces.
Phenotypic Antimicrobial Susceptibility Testing
Antibiotic susceptibility was determined by agar disk diffusion test against 23 antibiotics representing seven antimicrobial classes, commonly used in human and veterinary medicine. Antimicrobial susceptibility was determined for the following agents:
• ß-lactams including:
■ Penicillins: Ampicillin (AMP), Amoxicillin-Clavulanic Acid (AMC)
■ Second generation cephalosporins: Cefuroxime (CXM)
■ Third generation cephalosporins (TGC): Ceftiofur (CFT), Cefotaxime (CTX), Ceftriaxone (CRO), Ceftazidime (CAZ)
■ Cephamycins: Cefoxitin (FOX)
■ Fourth generation cephalosporins: Cefepime (FEP)
■ Monobactams: Aztreonam (ATM)
■ Carbapenems: Imipenem (IMI), Meropenem (MEM)
• Aminoglycosides: Kanamycin (KAN), Gentamicin (GEN), Amikacin (AMI), Streptomycin (STR)
• Tetracyclines: Tetracycline (TET)
• Quinolones: Nalidixic Acid (NAL), Ciprofloxacin (CIP), Enrofloxacin (ENR)
• Sulfonamides: Trimethoprim-Sulfamethoxazole (SXT)
• Phenicols: Chloramphenicol (CLR)
• Polymyxins: Colistin (COL)
The results were interpreted according to the Clinical and Laboratory Standards Institute (CLSI) criteria, (CLSI, 2017) and (CLSI, 2013). Susceptibility to colistin was evaluated by broth microdilution and results were interpreted according to the European Committee on Antimicrobial Susceptibility Testing guidelines (EUCAST).
E. coli strains resistant to three or more antimicrobial classes were categorized as multidrug resistant (MDR). Phenotypic screening for extended spectrum β-lactamase (ESBL) and plasmid mediated AmpC (pAmpC) was conducted performing synergy test using cefotaxime/clavulanic acid (CTX/CLA, 30/10 μg), ceftazidime/clavulanic acid (CAZ/CLA, 30/10 μg) and phenyl-boronic acid (PBA, 300 μg) containing disks, respectively (Yagi et al., 2005; CLSI, 2017). E. coli ATCC 25922 and E. coli ATCC 35218 were included as control.
Molecular Analysis of Resistance
All strains were tested by PCR for the presence of transferable resistance markers (mcr-1, ESBL, pAmpC, and plasmid mediated quinolone resistance—PMQR- coding- genes) using primers listed in Table 1. In the case of mcr-1 detection, the full mcr-1 gene was amplified and sequenced by using CLR5-F in combination with MCR1-R (5′-TGCGGTCTTTGACTTTGTC) (this study). Total DNA was obtained by boiling bacterial suspensions and plasmid DNA was purified according to Kado and Liu method (Kado and Liu, 1981).
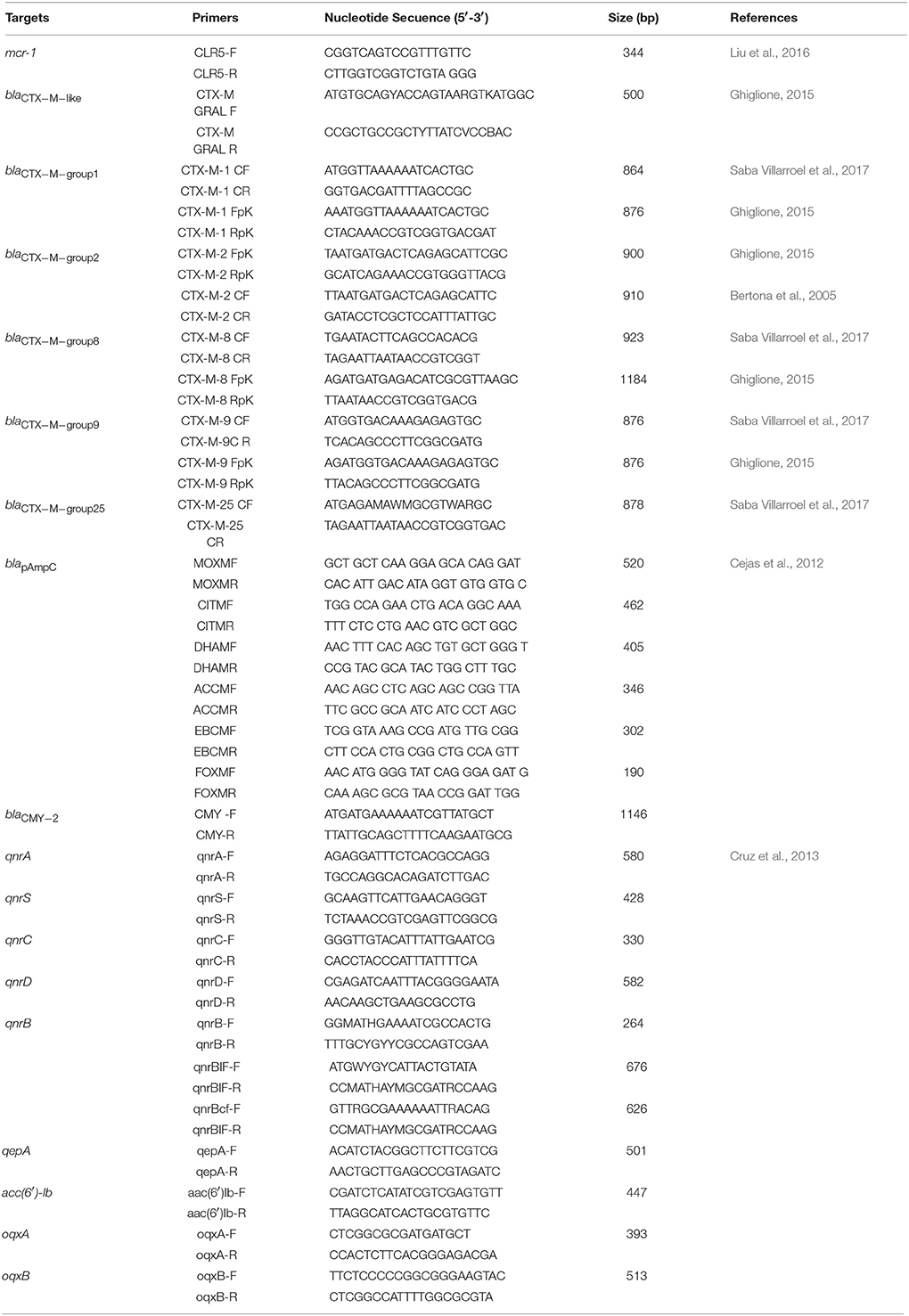
Table 1. Targets, primers, sequence, and product size used for PCR and sequencing of mcr-1, BLEE, ESBL, AmpC, and PMQR genes.
Plasmid Conjugation Studies
To assess mcr-1 plasmid transferability, conjugation studies by liquid mating were performed. Salmonella M1744 and E. coli J53 strains were used as recipient and randomly chosen mcr-1-positive strains from each farm were used as donors. After the conjugation, the transconjugants obtained from Salmonella M1744 were selected in TSA media supplemented with colistin (2 μg/mL), whereas those obtained from E. coli J53 were selected with sodium azide (200 μg/mL) and colistin (1 μg/mL). To confirm successful conjugation, colonies obtained in the selective media were screened for mcr-1 gene by PCR and then colistin MIC was determined for both transconjugant and parental E. coli strains by the broth microdilution as described before. In addition, co-resistance to other antimicrobials was assessed by agar disk diffusion method as previously described.
Molecular Typing by PCR-Based Techniques
Clonality of the isolates was determined by the homology relationships among fragments amplified by ERIC-PCR (Enterobacterial Repetitive Intergenic Consensus) and REP-PCR (Repetitive Extragenic Palindromic) according to Versalovic et al. (1991). Dendrograms were constructed by GelJ 1.0 program, using UPGMA algorithm and applying the DICE correlation coefficient.
Statistical Analysis
Significant differences (p < 0.05) in the association among strains according to the presence of genes were determined by Pearson's Chi-squared test with Yates continuity correction using Epidat software (version 4.1).
Results and Discussion
Resistance to Colistin and mcr−1 Gene Detection
The presence of mcr-1 in Argentina was already detected in E. coli isolates recovered from invasive infections in humans (Rapoport et al., 2016) and has also been found in bacteria isolated from domestic animals (Dominguez et al., 2017). The E. coli strains included in the present report were classified in the base of their susceptibility to colistin following the recommendations of the European Committee on Antimicrobial Susceptibility Testing (EUCAST, 2017). All strains considered resistant to colistin harbored the mcr-1 gene as demonstrated by PCR, and the sequenced gene was identical to the previously published sequence, accession number KP347127.1 (Liu et al., 2016). Additionally, from the 10 strains classified as colistin-susceptible, 3 of them were positive for the mcr-1 gene (Table 2).
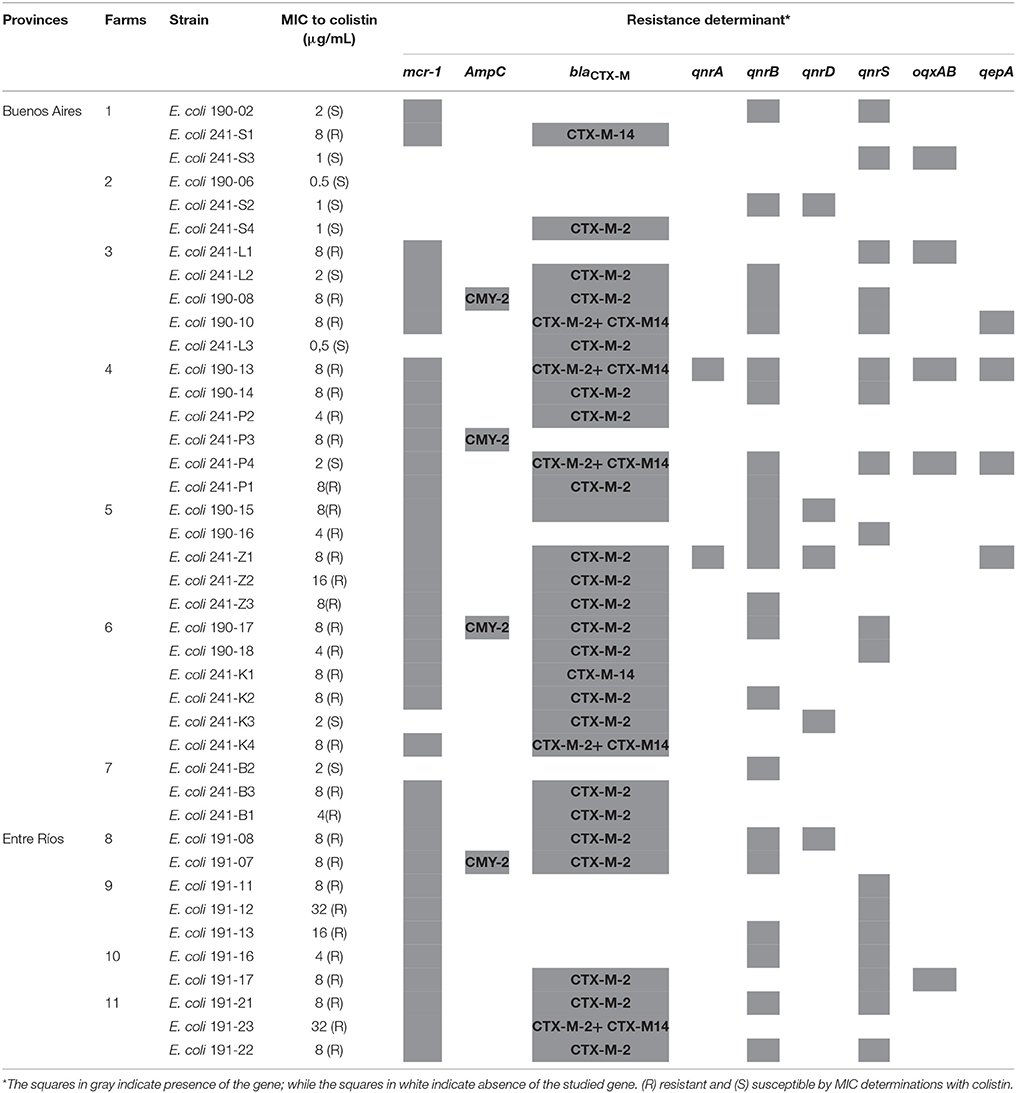
Table 2. Characteristics of Escherichia coli recovered from different farms in Buenos Aires and Entre Ríos, Argentina, 2014.
From the 31 colistin resistant/mcr-1-positive E. coli strains, 28 showed MICs ranging from 4 to ≥ 32 μg/mL. Although the disk diffusion method (10 μg colistin disk) is not yet standardized for polymyxins, all 28 strains displayed colistin inhibition zone ≤11 mm. The remaining strains (3/31) showed MICs between 4 and 8 μg/mL but nevertheless displayed inhibition zones ≥ 11 mm with colistin. Although molecular detection is the most appropriate technique for mcr-1 identification, a strong association with the phenotypic methodologies to detect mcr-1-mediated colistin resistance was observed.
Previous works describe the transferable nature of the mcr-1 gene (Liu et al., 2016). In the present work, the mcr-1-mediated colistin resistance was successfully transferred by conjugation to both recipient laboratory strains (E. coli J53 and Salmonella M1744). Two out of ten mcr-1-positive strains from each farm (Table 3) were obtained from liquid mating experiments performed using poultry E. coli strains as plasmid donors. Plasmids carrying the mcr-1 gene conjugated at a transfer frequency of ~1.5 × 10−3 transconjugants per donor cell. Accordant to results obtained by Liu et al. (2016), we found that MIC for colistin of the transconjugants increased four- and eight-fold compared to the original recipient strains.
Molecular typing analysis by the ERIC-PCR and REP-PCR showed that all E. coli carrying mcr-1 gene from this study had high clonal diversity and thus considered as genetically unrelated Figure S1. These results are in concordance with previous reports that describe the wide distribution of the mcr-1 gene among E. coli isolates independently of bacterial source or host species, suggesting a non-clonal spread of colistin resistance (Fernandes et al., 2016; Rapoport et al., 2016). In addition, this study reports a successful plasmid–gene combination of these E. coli strains in healthy broiler chickens, which may play a role in the emergence and spread of this gene.
Resistance to Other Antimicrobials
Resistance to Fluoroquinolones and Detection of Plasmid-Mediated Quinolone Resistant (PMQR) Genes
Further determinations of antimicrobial susceptibility of mcr-1-positive strains demonstrated high rates of multidrug resistance, since 85% (29/34) of the tested strains were resistant to at least three different classes of antimicrobial agents (Figure 1).
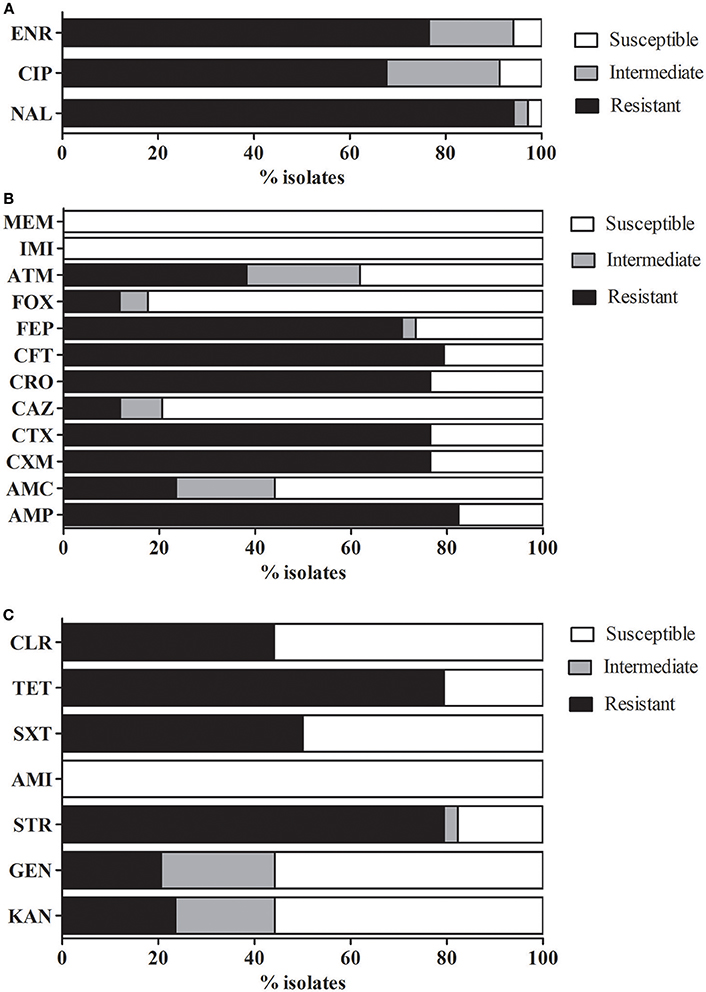
Figure 1. Antimicrobial susceptibility profiles. Percentage of antimicrobial susceptibility in the isolates analyzed. (A) Fluoroquinolones, (B) β-lactams, and (C) Other antimicrobials. AMP, ampicillin; AMC, amoxicillin-clavulanic acid; CXM, cefuroxime; CTX, cefotaxime; CAZ, ceftazidime; CRO, ceftriaxone; CFT, ceftiofur; FEP, cefepime; FOX, cefoxitin; ATM, aztreonam; IMI, imipenem; MEM, meropenem; NAL, nalidixic acid; CIP, ciprofloxacin; ENR, Enrofloxacin; KAN, kanamycin; GEN, gentamicin; STR, streptomycin; AMI, amikacin; SXT, trimethoprim-sulfamethoxazole; TET, tetracycline; and CLR, chloramphenicol.
Simultaneous resistance to colistin and quinolones or fluoroquinolones was relatively high (Figure 1A), since 94% (32/34) of the mcr-1-positive strains were resistant to nalidixic acid (NAL), 67.6% (23/34) to ciprofloxacin (CIP), and 76.5% (26/34) to enrofloxacin (ENR). Almost three from every four strains (76.5%) harbored a PMQR marker and the most prevalent determinants were qnrS (20/34) and qnrB (18/34). Almost three from every four strains (76.5%) harbored a PMQR marker and the most prevalent determinants were qnrS (20/34) and qnrB (18/34). Other PMQRs such as qnrA (2/34), qnrD (1/34) and the efflux pumps oqxAB (5/34) and qepA (5/34) were also identified. These results are consistent with the analysis made by Huang et al. (2009) in isolates from China, who also found a high ratio of E. coli strains harboring PMQR determinants and the authors suggest that this fact may be related to the extended use or misuse of antimicrobials in poultry.
Although no significant genotypic relation (p > 0.05) was found between mcr-1-positive strains and plasmid mediated quinolone resistance genes (PMQR), results obtained in conjugation experiments suggest that fluoroquinolone and colistin resistance can be simultaneously co-transferred, since both transconjugants (EC 190-14 TC and EC 191-07 TC or EC 191-07 TCS) displayed decreased susceptibility to NAL and were positive for qnrB gene detection (Table 3). However, the large number of strains carrying genetic determinants for fluoroquinolones in healthy broilers was relatively high; this scenario suggests that other selective forces such as colistin used as AGP (Morales et al., 2012) or therapeutic antimicrobial misuse are driving the selection of fluoroquinolone-resistant bacteria.
Resistance to β-Lactams and Detection of Extended Spectrum β-Lactamase (ESBL) and Plasmidic AmpC β-Lactamase
The antimicrobial susceptibility analysis showed a relatively high percentage of AMP resistance 82.4% (28/34) among the mcr-1-positive strains and a strong relation between susceptibility to both antimicrobials as determined by disk diffusion tests (R: 0.33, p < 0.05). Considering the susceptibility showed to AMP, a high percentage of resistance (between 76.5 and 79.4%) was also observed in oxyimino-cephalosporins (CTX, CRO and CFT, a cephalosporin used in veterinary medicine) and FEP (70.6%). However, very little resistance to CAZ and FOX was detected, while all isolates remained susceptible to carbapenems (IMI and MEM) (Figure 1B). In contrast, most clinical E. coli strains were found to be susceptible to a wide range of antimicrobials, including carbapenems (Lai et al., 2017).
CTX-M-producing enterobacteria are widespread among human population and an increasing number of reports describes their presence in livestock environments as well as in food from animal origin (Lazarus et al., 2015). Our findings demonstrate that also healthy birds may act as a reservoir of blaCTX−M−2 and blaCTX−M−14 genes. In the recent past, CTX-M-2 was the dominant ESBL group among human clinical Enterobacteriaceae isolates in South America (Quinteros et al., 2003; Minarini et al., 2007; Saba Villarroel et al., 2017). From 26 extended- spectrum cephalosporins (ESC)-resistant and mcr-1-positive strains, 18 strains (18/34, 56%) were CTX-M-2 producers and two produce CTX-M-14. Five strains harbored both CTX-M genes. CMY-2 was identified in 4 strains (3 were also CTX-M-2 producers) (Table 2). According to these results, the main mechanism responsible for TGC resistance was the production of CTX-M cefotaximases which explained the low resistance rates to FOX and CAZ. ESBLs from groups CTX-M-2 and CTX-M-14 were previously identified in mcr-1-carrying E. coli recovered from human samples (Rapoport et al., 2016) and from wild birds (kelp gulls) in the south of Argentina (Liakopoulos et al., 2016).
The cosmopolitan CTX-M-15 variant belonging to the CTX-M-1 subfamily, which is also widespread in human clinical isolates from Argentina, (Sennati et al., 2012), could not be found in this study. This finding was unexpected since reports from Brazil, where poultry productive systems are similar to Argentina (Botelho et al., 2015), described the presence of the CTX-M-15 ESBL and the coexistence of CTX-M-8 and CMY-2 in E. coli isolates recovered from chicken meat.
Many studies in E. coli strains, most of them involving isolates from animals, have demonstrated the presence of mcr-1 gene together with ESBL (Rhouma and Letellier, 2017). In the present work, despite the presence of the CTX-M-2 gene in the parental strain, no co-selection of ESC-resistant was observed in the transconjugants (Table 3).
Although 50% of the E. coli strains analyzed carried both sets of ESBL and PMQR genes, no association between the presence of ESBL and a specific PMQR mechanism (p > 0.05) was observed. Additionally, aac (6′)-Ib-cr gene was not detected. It is remarkable the absence of aac (6′)-Ib-cr gene, and the lack of association between ESBL and PMQR which is usually found in some Enterobacteriaceae isolated from human (Andres et al., 2013; Cruz et al., 2013) in Argentina.
A large variability of PMQR determinants was also observed in TGC-sensitive (without ESBL) and mcr-1-positive strains with a similar proportion of qnrB and qnrS genes. To a lesser extent, some of these strains also showed oqxAB gene. According to the results of this study, we suggest that E. coli strains from broiler chickens could be the reservoir not only of the mcr-1 gene, but also of PMQR and ESBL genes.
Resistance to Other Antimicrobials-Multiple Drug Resistance (MDR)
Most of the mcr-1-positive strains were determined to carry ESBL or PMQR-genes and also most of them were resistant to other classes of antimicrobial agents. This is probably due to the fact that the aforementioned genes are commonly found in mobile elements such as conjugative plasmids that also harbor resistant determinants to different groups of antimicrobials and confer the MDR phenotype. It is of particular concern that 39/41 (95.1%) strains considered in this study (including mcr-1-negative strains) expressed a multi-resistance phenotype.
The percentage of strains resistant to aminoglycosides and mcr-1-positive strains was variable and drug dependent. Resistance rates to this family was STR>KAN (79.4%, 23.6%) and GEN (20.6%). All strains remained susceptible to AMI (Figure 1C). Resistance to TET (79.4%) was relatively high, as expected considering the extensive use in animal medicine, which is in concordance with previous studies were TET resistance markers are frequently found in E. coli strains (Argudín et al., 2017). To a lesser extent, also resistance to SXT (50%) and CLR (44%) was also detected.
Conclusions
The results highlight that commercial broiler farms can be an important reservoir of mcr-1-carrying E. coli strains. In fact, the high occurrence of E. coli isolates (76%) carrying the mcr-1 gene is alarming and has not been reported in any other part of the world (Delgado-Blas et al., 2016; Fernandes et al., 2016; Kawanishi et al., 2017; Meinersmann et al., 2017; Monte et al., 2017; Whang et al., 2018). These differences could be associated with the method of screening used in the present report since a higher number of unrelated farms separated from a relatively high distance were considered. Although this may be related to particularities of the productive system, the local practices are quite similar to the ones from other countries in South America that were administering colistin without any restriction. A potential combination of antibiotics used in the productive system, climatic variations and other variables, may influence the spread of mcr-1 and these scenarios could also contribute to the selection of multi-resistant bacteria.
In this study, we determined the presence of resistance determinants in colistin-resistant E. coli strains from the environment of an intensive production system such as broiler chickens destined to consumption. A wide range of phenotypic resistance to both antibiotics in veterinary and human medicine was identified and resistance to colistin, quinolones and β-lactams was observed in the analyzed strains. The proportion of resistance to other antimicrobial families (SXT, TET, CLR, and aminoglycoside) was relatively high, underlining the presence of a large number of isolates with a MDR profile. The ability the co-transference of the qnrB determinant on the mcr-1-positive transconjugants was corroborated, which suggests that these resistance genes are likely to be located in the same plasmid thus transforming it into a more successful clone.
Author Contributions
JD, LR, GG, JC, and MF participated in the design of the study. JD, RF, and DC performed the experiments. JD, LR, JC, and MF analyzed the data. JD, LR, PC, and MF collected E. coli strains. JD, LR, PC, JC, and MF wrote the paper. All authors contributed to the critical revision of the manuscript and have seen and approved the final draft. All authors read and approved the final manuscript.
Funding
This work was supported by a grant from the Instituto Nacional de Tecnología Agropecuaria, [INTA PNSA PE-1115056] and the Agencia Nacional de Promoción Científica y Tecnológica; [PICT 0737-2012 and PIP-CONICET 11220120100400CO].
Conflict of Interest Statement
The authors declare that the research was conducted in the absence of any commercial or financial relationships that could be construed as a potential conflict of interest.
Acknowledgeements
We thank Gonzalez, L.M., for invaluable help at the lab; Losada Eaton, D. and Arregui, M.E. and Pierson, E.M. for their manuscript correction; and Diaz Carrasco, J.M. for their technical assistance with the images. JD and RF, R.A. are supported by research fellowships from Consejo Nacional de Investigaciones Científicas y Tecnológicas (CONICET).
Supplementary Material
The Supplementary Material for this article can be found online at: https://www.frontiersin.org/articles/10.3389/fmicb.2018.01679/full#supplementary-material
Figure S1. Phylogenetic tree and patterns of ERIC-PCR on agarose gel electrophoresis.
Footnotes
References
Andres, P., Lucero, C., Soler-Bistué, A., Guerriero, L., Albornoz, E., Tran, T., Petroni, A., et al. (2013). Differential distribution of plasmid-mediated quinolone resistance genes in clinical enterobacteria with unusual phenotypes of quinolone susceptibility from Argentina. Antimicrob. Agents Chemother. 57, 2467–2475. doi: 10.1128/AAC.01615-12
Argudín, M. A., Deplano, A., Meghraoui, A., Dodémont, M., Heinrichs, A., Denis, O., et al. (2017). Bacteria from animals as a pool of antimicrobial resistance genes. Antibiotics (Basel) 6:E12. doi: 10.3390/antibiotics6020012
Bertona, E., Radice, M., Rodríguez, C. H., Barberis, C., Vay, C., Famiglietti, A., et al. (2005). Phenotipic and genotypic characterization of resistance to third-generation cephalosporins in Enterobacter spp. Rev. Argent. Microbiol. 37, 203–208.
Botelho, L. A., Kraychete, G. B., Silva, J. L., Regis, D. V., Picão, R. C., Moreira, B. M., et al. (2015). Widespread distribution of CTX-M and plasmid-mediated AmpC β-lactamases in Escherichia coli from Brazilian chicken meat. Mem. Inst. Oswaldo Cruz 110, 249–254. doi: 10.1590/0074-02760140389
Brenner, D. J., and Farmer, J. (2015). “Enterobacteriaceae.” in Bergey's Manual of Systematics of Archaea and Bacteria, eds W. B. Whitman, F. Rainey, P. Kämpfer, M. Trujillo, J. Chun, P. DeVos, B. Hedlund, and S. Dedysh, 1–24. doi: 10.1002/9781118960608.fbm00222
Cejas, D., Fernández Canigia, L., Quinteros, M., Giovanakis, M., Vay, C., Lascialandare, S., et al. (2012). Plasmid-Encoded AmpC (pAmpC) in Enterobacteriaceae: epidemiology of microorganisms and resistance markers. Rev. Argent. Microbiol. 44, 182–186.
CLSI (2013). “Performance standards for antimicrobial disk and dilution susceptibility test for bacteria isolated from animals; approved standard,” in CLSI Document VET01-A4, 4th Edn. (Wayne, PA: Clinical and Laboratory Standards Institute).
CLSI (2017). “Performance standards for antimicrobial susceptibility testing,” in CLSI Supplement M100, 27th Edn. (Wayne, PA: Clinical and Laboratory Standards Institute).
Cruz, G. R., Radice, M., Sennati, S., Pallecchi, L., Rossolini, G. M., Gutkind, G., et al. (2013). Prevalence of plasmid-mediated quinolone resistance determinants among oxyiminocephalosporin-resistant Enterobacteriaceae in Argentina. Mem. Inst. Oswaldo Cruz 108, 924–927. doi: 10.1590/0074-0276130084
Delgado-Blas, J. F., Ovejero, C. M., Abadia-Patiño, L., and Gonzalez-Zorn, B. (2016). Coexistence of mcr-1 and blaNDM−1 in Escherichia coli from Venezuela. Antimicrob. Agents Chemother. 60, 6356–6358. doi: 10.1128/AAC.01319-16
Diarra, M. S., Silversides, F. G., Diarrassouba, F., Pritchard, J., Masson, L., Brousseau, R., et al. (2007). Impact of feed supplementation with antimicrobial agents on growth performance of broiler chickens, Clostridium perfringens and enterococcus counts, and antibiotic resistance phenotypes and distribution of antimicrobial resistance determinants in Escherichia coli isolates. Appl. Environ. Microbiol. 73, 6566–6576.doi: 10.1128/AEM.01086-07.
Dibner, J. J., and Richards, J. D. (2005). Antibiotic growth promoters in agriculture: history and mode of action. Poult. Sci. 84, 634–643. doi: 10.1093/ps/84.4.634
Dominguez, J. E., Figueroa Espinosa, R. A., Redondo, L. M., Cejas, D., Gutkind, G. O., Chacana, P. A., et al. (2017). Plasmid-mediated colistin resistance in Escherichia coli recovered from healthy poultry. Rev. Argent. Microbiol. 49, 297–298. doi: 10.1016/j.ram.2017.02.001
Fernandes, M. R., Moura, Q., Sartori, L., Silva, K. C., Cunha, M. P., Esposito, F., et al. (2016). Silent dissemination of colistin-resistant Escherichia coli in South America could contribute to the global spread of the mcr-1 gene. Euro Surveill. 21:30214. doi: 10.2807/1560-7917.ES.2016.21.17.30214
Ghiglione, B. (2015). Estudio de las Bases Bioquímicas y Moleculares de la Actividad Hidrolítica de Variantes Salvajes y Mutacionales de β-Lactamasas CTX-M Sobre Oximino-Cefalosporinas. Papel de mutaciones puntuales en la expansión del espectro de hidrólisis. Ph.D. thesis, Universidad de Buenos Aires.
Howarth, F., and Poulter, D. (1996). Vancomycin resistance: time to ban avoparcin. Lancet 347:1047. doi: 10.1016/S0140-6736(96)90187-7
Huang, S. Y., Dai, L., Xia, L. N., Du, X. D., Qi, Y. H., Liu, H. B., et al. (2009). Increased prevalence of plasmid-mediated quinolone resistance determinants in chicken Escherichia coli isolates from 2001 to 2007. Foodborne Pathog. Dis. 6, 1203–1209. doi: 10.1089/fpd.2009.0348
Jukes, T. H., Stokstad, E. L. R., Tayloe, R. R., Cunha, T. J., Edwards, H. M., and Meadows, G. B. (1950). Growth-promoting effect of aureomycin on pigs. Arch. Biochem. 26, 324–325.
Kado, C. I., and Liu, S. T. (1981). Rapid procedure for detection and isolation of large and small plasmids. J. Bacteriol. 145, 1365–1373.
Kawanishi, M., Abo, H., Ozawa, M., Uchiyama, M., Shirakawa, T., Suzuki, S., et al. (2017). Prevalence of colistin resistance gene mcr-1 and absence of mcr-2 in escherichia coli isolated from healthy food-producing animals in japan. Antimicrob. Agents Chemother. 61:e02057-16. doi: 10.1128/AAC.02057-16
Lai, C. C., Chuang,. Y. C., Chen,. C. C., and Tang, H. J. (2017). Coexistence of MCR-1 and NDM-9 in a clinical carbapenem-resistant Escherichia coli isolate. Int. J. Antimicrob. Agents 49, 517–518. doi: 10.1016/j.ijantimicag.2017.02.001
Lazarus, B., Paterson, D. L., Mollinger, J. L., and Rogers, B. A. (2015). Do human extraintestinal Escherichia coli infections resistant to expanded-spectrum cephalosporins originate from food-producing animals? A systematic review. Clin. Infect. Dis. 60, 439–452. doi: 10.1093/cid/ciu785
Liakopoulos, A., Mevius, D. J., Olsen, B., and Bonnedahl, J. (2016). The colistin resistance mcr-1 gene is going wild. J. Antimicrob. Chemother. 71, 2335–2336. doi: 10.1093/jac/dkw262
Liu, Y. Y., Wang, Y., Walsh, T. R., Yi, L. X., Zhang, R., Spencer, J., et al. (2016). Emergence of plasmid-mediated colistin resistance mechanism MCR-1 in animals and human beings in China: a microbiological and molecular biological study. Lancet Infect. Dis. 16, 161–168. doi: 10.1016/S1473-3099(15)00424-7
Ljungquist, O., Ljungquist, D., Myrenås, M., Rydén, C., Finn, M., and Bengtsson, B. (2016). Evidence of household transfer of ESBL/pAmpC-producing Enterobacteriaceae between humans and dogs – a pilot study. Infect. Ecol. Epidemiol. 6:31514. doi: 10.3402/iee.v6.31514
Madec, J. Y., Haenni, M., Nordmann, P., and Poirel, L. (2017). Extended-spectrum β-lactamase/ AmpC- and carbapenemase-producing Enterobacteriaceae in animals: a threat for humans? Clin. Microbiol. Infect. 23, 826–833. doi: 10.1016/j.cmi.2017.01.013
Meinersmann, R. J., Ladely, S. R., Plumblee, J. R., Cook, K. L., and Thacker, E. (2017). Prevalence of mcr-1 in the cecal contents of food animals in the United States. Antimicrob. Agents Chemother. 61:e02244–e02216. doi: 10.1128/AAC.02244-16.
Minarini, L. A., Gales, A. C., Palazzo, I. C., and Darini, A. L. (2007). Prevalence of community-occurring extended spectrum beta-lactamase-producing Enterobacteriaceae in Brazil. Curr. Microbiol. 54, 335–341. doi: 10.1007/s00284-006-0307-z
Monte, D. F., Mem, A., Fernandes, M. R., Cerdeira, L., Esposito, F., Galvão, J. A., et al. (2017). Chicken meat as a reservoir of colistin-resistant Escherichia coli strains carrying mcr-1 Genes in South America. Antimicrob. Agents Chemother. 61:e02718-16. doi: 10.1128/AAC.02718-16
Moore, P. R., and Evenson, A. (1946). Use of sulfasuxidine, steptothricin, and streptomycin in nutritional studies with the chick. J. Biol. Chem. 165, 437–441.
Morales, A. S., Fragoso de Araújo, J., de Moura Gomes, V. T., Reis Costa, A. T., dos Prazeres Rodrigues, D., Porfida Ferreira, T. S., et al. (2012). Colistin resistance in Escherichia coli and Salmonella enterica strains isolated from swine in Brazil. ScientificWorldJournal 2012:109795. doi: 10.1100/2012/109795
Niewold, T. A. (2007). The nonantibiotic anti-Inflammatory effect of antimicrobial growth promoters, the real mode of action? A hypothesis. Poult. Sci. 86, 605–609. doi: 10.1093/ps/86.4.605
Quinteros, M., Radice, M., Gardella, N., Rodriguez, M. M., Costa, N., Korbenfeld, D., et al. (2003). Extended-spectrum β-lactamases in enterobacteriaceae in buenos aires, argentina, public hospitals. Antimicrob. Agents Chemother. 47, 2864–2867. doi: 10.1128/AAC.47.9.2864-2867.2003
Rapoport, M., Faccone, D., Pasteran, F., Ceriana, P., Albornoz, E., Petroni, A., et al. (2016). First Description of mcr-1-mediated colistin resistance in human infections caused by Escherichia coli in Latin America. Antimicrob. Agents Chemother. 60, 4412–4413. doi: 10.1128/AAC.00573-16
Rhouma, M., Beaudry, F., Thériault, W., and Letellier, A. (2016). Colistin in pig production: chemistry, mechanism of antibacterial action, microbial resistance emergence, and one health perspectives. Front. Microbiol. 7:1789.doi: 10.3389/fmicb.2016.01789
Rhouma, M., and Letellier, A. (2017). Extended-spectrum β-lactamases, carbapenemases and the mcr-1 gene: is there a historical link? Int. J. Antimicrob. Agents 49, 269–271. doi: 10.1016/j.ijantimicag.2016.11.026
Saba Villarroel, P. M., Gutkind, G. O., Di Conza, J. A., and Radice, M. A. (2017). First survey on antibiotic resistance markers in Enterobacteriaceae in Cochabamba, Bolivia. Rev. Argent. Microbiol. 49, 50–54. doi: 10.1016/j.ram.2016.10.002
Sennati, S., Santella, G., Di Conza, J., Pallecchi, L., Pino, M., Ghiglione, B., et al. (2012). Changing epidemiology of extended-spectrum β-lactamases in Argentina: emergence of CTX-M-15. Antimicrob. Agents Chemother. 56, 6003–6005. doi: 10.1128/AAC.00745-12
Versalovic, J., Koeuth, T., and Lupski, J. R. (1991). Distribution of repetitive DNA sequences in eubacteria and application to fingerprinting of bacterial genomes. Nucleic Acids Res. 19, 6823 −6831. doi: 10.1093/nar/19.24.6823
Wang, J., Ma, Z. B., Zeng, Z. L., Yang, X. W., Huang, Y., and Liu, J. H. (2017). The role of wildlife (wild birds) in the global transmission of antimicrobial resistance genes. Zool. Res. 38, 55–80. doi: 10.24272/j.issn.2095-8137.2017.024
Whang, R., Liu, Y., Zhang, Q., Jin,. L., Wang,. Q., Zhang,. Y., et al. (2018). The prevalence of colistin resistance in Escherichia coli and Klebsiella pneumoniae isolated from food animals in China: coexistence of mcr-1 and blaNDM with low fitness cost. Int. J. Antimicrob. Agents 51:739–744. doi: 10.1016/j.ijantimicag.2018.01.023
Yagi, T., Wachino, J., Kurokawa, H., Suzuki, S., Yamane, K., Doi, Y., et al. (2005). Practical methods using boronic acid compounds for identification of class C beta-lactamase-producing Klebsiella pneumoniae and Escherichia coli. J. Clin. Microbiol. 43, 2551–2558. doi: 10.1128/JCM.43.6.2551-2558.2005.
Keywords: Colistin, mcr-1, food-borne bacteria, Escherichia coli, CTX-M-2, qnrB, multi drug resistance
Citation: Dominguez JE, Redondo LM, Figueroa Espinosa RA, Cejas D, Gutkind GO, Chacana PA, Di Conza JA and Fernández Miyakawa ME (2018) Simultaneous Carriage of mcr-1 and Other Antimicrobial Resistance Determinants in Escherichia coli From Poultry. Front. Microbiol. 9:1679. doi: 10.3389/fmicb.2018.01679
Received: 05 February 2018; Accepted: 05 July 2018;
Published: 25 July 2018.
Edited by:
Patrícia Poeta, University of Trás-os-Montes and Alto Douro, PortugalReviewed by:
Catherine M. Logue, University of Georgia, United StatesLiang Li, Los Angeles Biomedical Research Institute, United States
Copyright © 2018 Dominguez, Redondo, Figueroa Espinosa, Cejas, Gutkind, Chacana, Di Conza and Fernández Miyakawa. This is an open-access article distributed under the terms of the Creative Commons Attribution License (CC BY). The use, distribution or reproduction in other forums is permitted, provided the original author(s) and the copyright owner(s) are credited and that the original publication in this journal is cited, in accordance with accepted academic practice. No use, distribution or reproduction is permitted which does not comply with these terms.
*Correspondence: José A. Di Conza, jdiconza@gmail.com
Mariano E. Fernández Miyakawa, fernandezmiyakawa.m@inta.gob.ar