- 1Department of Evolutionary and Environmental Biology, The Faculty of Natural Sciences, University of Haifa, Haifa, Israel
- 2Faculty of Medicine, Bar-Ilan University, Ramat Gan, Israel
- 3Clinical Microbiology Laboratory, Baruch Padeh Medical Center, Tiberias, Israel
- 4Department of Biology and Environment, The Faculty of Natural Sciences, University of Haifa, Haifa, Israel
Vibrio cholerae serogroups O1 and O139 are the causative agents of cholera disease. There are more than 200 serogroups in this species that are termed V. cholerae non-O1/non-O139. Non-O1/non-O139 strains can cause gastroenteritis and cholera like diarrhea, wound infections, external otitis, and bacteraemia that may lead to mortality. Previous antimicrobial susceptibility studies were conducted mainly on O1/O139 serogroups and on clinical isolates. Our aim was to study and compare the antimicrobial susceptibilities of non-O1/non-O139 environmental strains isolated from chironomids, fish, and waterfowl. Significant differences were found in the antimicrobial susceptibilities between the environmental strains that were isolated from three different reservoir habitats. Significant increase in minimum inhibitory concentrations (MICs) of ampicillin and chloramphenicol was found in chironomid isolates from 2009 compared to those from 2005. V. cholerae isolates from different waterfowl species displayed the highest MIC values to chloramphenicol and trimethoprim-sulfamethoxazole (SXT), while chironomid isolates demonstrated the highest MIC values toward ampicillin. Isolates from fish and waterfowl showed high MIC values toward doxycycline. No significant differences were found between the MICs of isolates from the different waterfowl species. The percentage of antimicrobial resistance among V. cholerae isolates from waterfowl was the highest compared to the abundance of antimicrobial resistant isolates from chironomids or fish. The antimicrobial resistance genes can be carried on mobile genetic elements, thus, waterfowl may act as reservoirs for these elements and may spread them all over the globe. Data regarding treatment with antimicrobial agents toward V. cholerae non-O1/non-O139 serogroups is lacking and therefore further studies are needed.
Introduction
Vibrio cholerae is a Gram-negative rod with more than 200 different serogroups. Only serogroups O1 and O139 are considered as the causative agents of the epidemic diarrheal disease cholera. Cholera is an endemic disease in many Asian and African countries. Non-O1/non-O139 strains share the same environmental niche with the pathogenic strains. They can cause intestinal infections such as gastroenteritis and cholera-like diarrhea, and also extra-intestinal infections such as wound infections, otitis media, external otitis, and bacteraemia that sometimes can cause mortality in humans (Sack et al., 2004; Bier et al., 2015; Kechker et al., 2017).
Antibiotics are not required to treat diarrheal cholera or diarrheal cholera-like symptoms. The main treatment for cholera is oral rehydration therapy. However, in severe cholera cases, a combination of oral rehydration therapy with antibiotic treatment is recommended since the antibiotics decrease the volume of the diarrhea (Kitaoka et al., 2011). Nevertheless, antimicrobial agent therapy is required for treating extra-intestinal infections caused by non-O1/non-O139 strains. For example, non-O1/non-O139 V. cholerae bacteremia is a life-threatening infection (Petsaris et al., 2010). Mortality occurred in 33% out of 350 non-O1/non-O139 bacteraemia cases (Deshayes et al., 2015). It was described that non-O1/non-O139 strains are usually susceptible to most antimicrobial agents (Petsaris et al., 2010; Ceccarelli et al., 2015). However, resistances of non-O1/non-O139 strains to aminoglycosides, ampicillin and carbapenems was reported (Ottaviani et al., 2003; Bier et al., 2015).
According to the guidelines of the Clinical and Laboratory Standards Institute (CLSI, 2018) there are some antibiotics that were recommended for treating V. cholerae infections (these recommendations do not distinguish between serogroups). The recommended antimicrobial agents are: ampicillin that acts as a cell growth inhibitor; azithromycin, doxycycline, and chloramphenicol that inhibit protein synthesis; and trimethoprim-sulfamethoxazole (SXT) that inhibits folic acid metabolism (CLSI, 2018).
Chironomids (Diptera, Chironomidae), also known as non-biting midges, are the most abundant insects in freshwater aquatic ecosystems (Laviad and Halpern, 2016). Chironomids were found as natural reservoirs of V. cholerae (Broza and Halpern, 2001; Halpern et al., 2004; Senderovich and Halpern, 2013).
Fish are also reservoirs of V. cholerae. V. cholerae was isolated from about 30 fish species (reviewed in Halpern and Izhaki, 2017). It was isolated mainly from healthy fish intestines; however, it was also detected in the fish gills, skin, kidney, liver, and brain tissues (Halpern and Izhaki, 2017). V. cholerae O1 and O139 were isolated from fish mucus and scales in Mozambique, from tilapia gills in Tanzania, and from two marine fish in India (du Preez et al., 2010; Kumar and Lalitha, 2013; Hounmanou, 2015). Moreover, many studies found correlations between the occurrence of cholera and fish consumption or handling (e.g., Acosta et al., 2001; Schürmann et al., 2002).
Although V. cholerae has been studied since 1884 (Koch, 1884), the mechanism that enables the bacteria to disseminate around the globe is still an enigma. Halpern et al. (2008) suggested that waterfowl can be the vector responsible for this dissemination. Additionally, they explained that waterfowl can either acquire the bacterium from zooplankton (chironomids or copepods) or from fish consumption. Fish can get infected with V. cholerae via zooplankton consumption (chironomids or copepods) (Halpern et al., 2008). Recently, we have demonstrated that V. cholerae can be identified from wild waterfowl intestines (Laviad-Shitrit et al., 2018) and that great cormorants got infected with V. cholerae after they were fed with tilapia fish that were naturally infected with V. cholerae (Laviad-Shitrit et al., 2017).
As far as we know, data regarding susceptibility of different V. cholerae non-O1/non-O139 environmental serogroups is very rare. Knowledge of their antimicrobial resistance is of global health interest as they are dispersed all over the world and share the same niche with the pathogenic strains (Halpern and Izhaki, 2017; Laviad-Shitrit et al., 2017, 2018).
Here we studied and compared the minimum inhibitory concentration (MIC) of different environmental V. cholerae non-O1/non-O139 serogroups that were isolated from three different reservoirs of V. cholerae: chironomid egg masses and fish and waterfowl intestines.
Materials and Methods
Vibrio cholerae Isolates
Isolates were obtained from previous studies. 40 isolates were obtained from chironomid egg masses (Senderovich et al., 2008; Shaked, 2011), 49 isolates from fish intestine samples (Senderovich et al., 2010; Laviad-Shitrit et al., 2017), and 47 isolates from waterfowl intestine samples (Laviad-Shitrit et al., 2017, 2018). Details regarding the sampling sites, sampling dates and the identity of the sampled fish and waterfowl species, can be found in Supplementary Table S1.
The isolation and Identification of V. cholerae was described in each of the references that were mentioned above. Briefly, V. cholerae was isolated directly on Thiosulfate-citrate-bile salts-sucrose (TCBS) agar plates (Difco, United States), without any enrichment (e.g., Laviad-Shitrit et al., 2017). Isolates’ identities were verified by multiplex PCR assay that amplified the ompW gene that encodes an outer membrane protein specific to V. cholerae and ctxA gene that encodes the cholera enterotoxin subunit A, according to Nandi et al. (2000). The identity of O1 and O139 serogroups was examined by slide agglutination with the use of two specific antisera: (1) a poly antiserum specific for O1 surface antigen (Difco), and (2) an antiserum specific for O139 surface antigen (Ministry of Health, Israel). Moreover, V. cholerae isolates from waterfowl underwent somatic O antigen serogrouping (Laviad-Shitrit et al., 2018). The procedure of somatic O antigen serogrouping is described in details in Shimada et al. (1994). The non-O1/non-O139 serogroups identities are described in Supplementary Table S2.
Determination of Minimum Inhibitory Concentration (MIC)
All isolates were tested for their antimicrobial susceptibilities using the E-test gradient. Each isolate was classified as resistant (R), intermediately resistant (I), or susceptible (S), according to the guidelines of the Clinical and Laboratory Standards Institute (Supplementary Table S3 and CLSI, 2018). Isolates were spread on CHROMagar plate (HyLaboratories, Rehovot, Israel) and then incubated at 37°C for 24 h. Colonies were suspended in 0.45% NaCl solution to 0.5 McFarland turbidity and subjected to MIC test strip (Liofilchem S.r.l., Italy) on Mueller Hinton agar plates (BD Diagnostics, Sparks, MD, United States). The antibiotics that were tested were chosen according to the guidelines of the Clinical and Laboratory Standards Institute (CLSI, 2018): ampicillin, azithromycin, chloramphenicol, doxycycline, and trimethoprim-sulfamethoxazole (hereinafter SXT). Plates were incubated for 24 h at 37°C. MIC was calculated for each antibiotic as the lowest concentration of the antibiotic at which the zone of inhibition intersected the strip.
Statistical Analysis
Repeated measures ANOVA with Bonferroni post hoc test was used to determine the differences between the MICs of the five antimicrobial agents (n = 147 strains). Data sphericity was not assumed (Mauchly’s test: p < 0.001) and Huynh-Feldt correction was applied (E = 0.375). One-way ANOVA tests with Tukey HSD post hoc were used to determine the differences between MICs of V. cholerae strains isolated from chironomids, fish, and waterfowl, and from different waterfowl species (herons, egrets, and cormorants). Independent samples Student t-tests were applied to compare the MICs for V. cholerae strains that were isolated from chironomids at different years (2005 and 2009).
Results
Antibiotic susceptibilities of 147 V. cholerae environmental non-O1/non-O139 strains were studied for five antimicrobial agents that are used to treat cases of V. cholerae infections. The strains were isolated from: (i) chironomid egg masses (50 strains; Senderovich et al., 2008; Shaked, 2011), (ii) fish intestines (50 strains; Senderovich et al., 2010; Laviad-Shitrit et al., 2017), and (iii) waterfowl intestines (47 strains; Laviad-Shitrit et al., 2018). Overall, significant differences were observed in V. cholerae sensitivities to the different antimicrobial agents [Repeated measures ANOVA: F(1.5,205.8) = 4.1, p = 0.029]. MIC values of doxycycline were the highest, with an average MIC of 9.34 ± 3.31 μg/ml (Figure 1) and MIC90 of 8.0 μg/ml (Table 1). No significant differences were observed between the MIC values of the other four antimicrobial agents that were studied (p > 0.05).
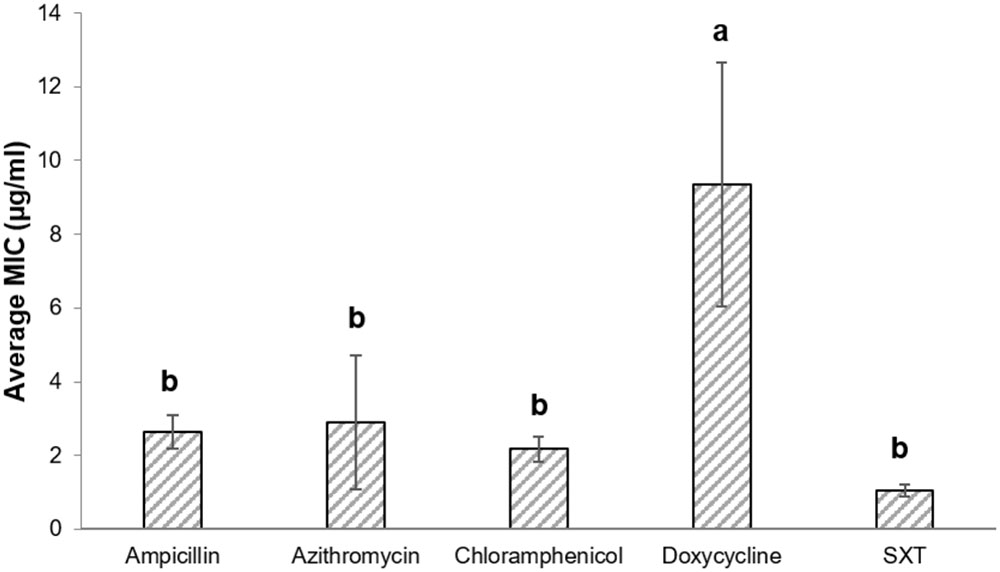
FIGURE 1. MIC (average ± std. error) of the studied antimicrobial agents toward all V. cholerae strains. Ampicillin (n = 146), azithromycin (n = 141), chloramphenicol (n = 146), doxycycline (n = 144); SXT (n = 146). Bars with different letters are significantly different by repeated measures ANOVA with Bonferroni post hoc test (p < 0.05). SXT, Trimethoprim (TMP) and sulfamethoxazole.
Vibrio cholerae strains exhibited substantial variations in their susceptibilities toward the tested antimicrobial agents (Figure 2). High differences were found in the ampicillin MIC90 values between isolates from chironomid egg masses (12.4 μg/ml), from waterfowl species (1.75 μg/ml), and from fish (1 μg/ml). Isolates from fish and waterfowl intestine samples that were exposed to doxycycline showed high MIC90 values (16.0 and 14.4 μg/ml, respectively) compared to the isolates from chironomids (only 1.0 μg/ml). Additionally, isolates from waterfowls showed the highest MIC90 values after exposure to chloramphenicol and SXT (12.0 and 5.0 μg/ml, respectively) compared to isolates from fish (2.0 and 1.5 μg/ml, respectively) and from chironomid egg masses (1.5 and 0.75 μg/ml, respectively) (Table 1).
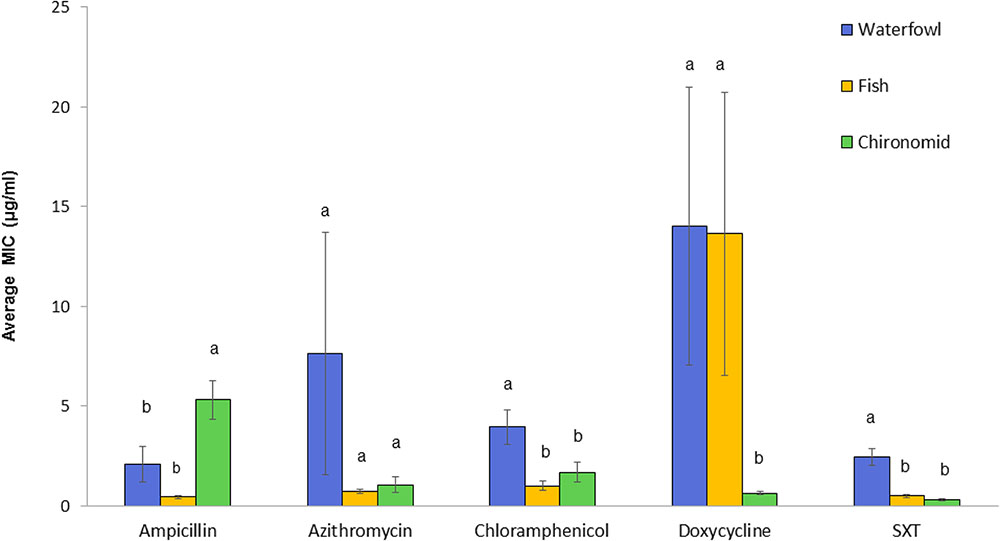
FIGURE 2. Comparative analysis of MICs (average ± std. error) for each of the studied antimicrobial agents toward V. cholerae strains that were isolated from the three environmental reservoirs (waterfowl n = 47, fish n = 50, and chironomids n = 50). Bars with different letters are significantly different by One-way ANOVA with Tukey HSD test (p < 0.05). SXT, Trimethoprim (TMP) and sulfamethoxazole.
One-way ANOVA revealed significant differences in the average MIC of ampicillin, chloramphenicol, doxycycline, and SXT between V. cholerae strains that were isolated from chironomids, fish, and waterfowl; ampicillin [F(2, 143) = 11.03, p < 0.001], chloramphenicol [F(2, 143) = 7.11, p < 0.001], doxycycline [F(2, 141) = 8.52, p < 0.001], and SXT [F(2, 143) = 23.21, p < 0.001]. No significant difference was observed between MIC values of azithromycin (p = 0.24) (Figure 2). V. cholerae isolated from waterfowl were significantly more resistant to SXT and chloramphenicol with average MIC concentrations of 2.44 ± 0.42 and 3.95 ± 0.86 μg/ml, respectively (Figure 2). In contrast, V. cholerae isolates from chironomids were more resistant to ampicillin with average MIC value of 5.33 ± 0.98 μg/ml and a MIC90 value of 12.4 μg/ml (Table 1).
Vibrio cholerae strains isolated from chironomids during 2009 showed significantly higher MICs of ampicillin, chloramphenicol, and SXT compared with the strains that were isolated during 2005 (Figure 3) [Students t-test: ampicillin: t(36.3) = 4.67, p < 0.001; chloramphenicol: t(31.9) = 2.25, p = 0.032; SXT: t(48) = 2.05, p = 0.046]. No significant differences were found between V. cholerae strains isolated from chironomids at the Kishon River vs. WSP (independent samples t-tests: p > 0.05).
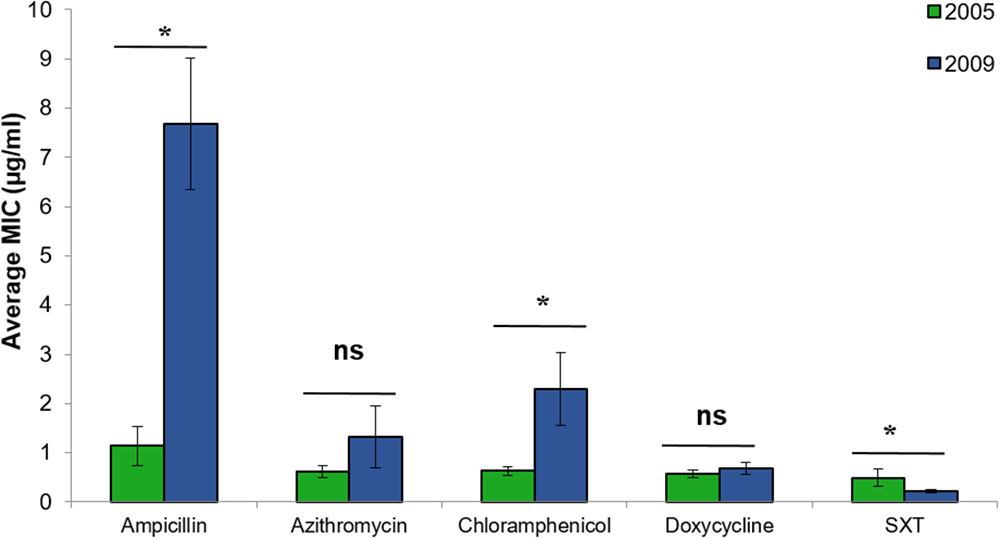
FIGURE 3. Comparative analysis of the MIC (average ± std. error) for each of the studied antimicrobial agents toward V. cholerae strains that were isolated from chironomids in 2005 (green bars, n = 18), and 2009 (blue bars, n = 32). Asterisks represent significant differences in MICs by Student’s t-test (p < 0.05). ns, no significant difference; SXT, Trimethoprim (TMP) and sulfamethoxazole.
No significant differences in MIC concentrations were found between strains isolated from the different waterfowl species (herons, egrets, and cormorants, p > 0.05) (Figure 4). Four strains that were isolated from two different individual herons were resistant even to the highest concentration of azithromycin that was studied (256 μg/ml) (Table 2).
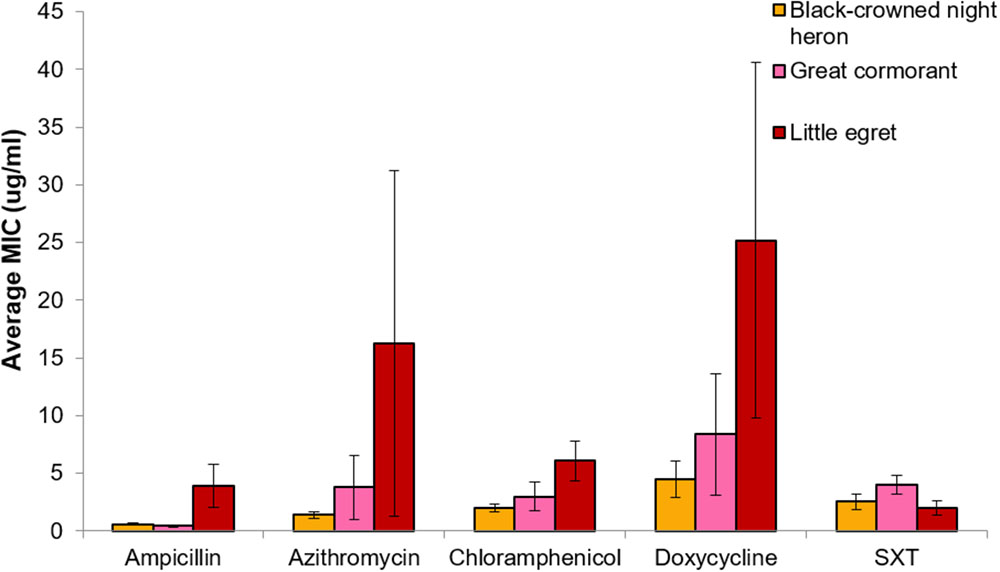
FIGURE 4. Comparative analysis of MIC (average ± std. error) for each of the studied antimicrobial agents toward V. cholerae strains that were isolated from the three waterfowl species [black-crowned night herons (orange, n = 21), great cormorants (pink, n = 5), and little egrets (red, n = 22)]. No significant differences were found between MIC concentrations of the different strains that were isolated from different waterfowl species (p > 0.05).
Significant differences were observed between the average MIC values of SXT of strains that belonged to different serogroups [F(3, 38) = 4.00, p = 0.014]. Serogroup O6 showed the highest MIC of SXT, with a MIC50 of 4 μg/ml and MIC90 of 5.58 ± 1.74 μg/ml. No significant differences in MICs of the other antibiotics were observed between serogroups (Supplementary Table S2).
Discussion
Antimicrobial agent effectiveness is decreasing due to a significant increase of antimicrobial resistance in bacteria. Medicine achievements in major surgery, organ transplantation, and treatment of preterm babies, cancer chemotherapy, and many other clinical procedures cannot be successful without effective antibiotic treatments (Laxminarayan et al., 2013). It was estimated that bacteria resistant to antimicrobial compounds kill 25,000 people annually in Europe. In the United States, the estimated numbers for sickness and death due to antibiotic-resistant bacterial strains are 2 × 106 and 2.3 × 104 people every year (CDC, 2013).
It was found that different serogroups of V. cholerae use multidrug efflux pumps in order to export chemically and structurally unrelated components such as antibiotics (Paulsen et al., 1996), e.g., tetracycline, Norfloxacin, ciprofloxacin, doxorubicin, chloramphenicol, and nalidixic acid (Kitaoka et al., 2011). Moreover, antibiotic resistance can occur in V. cholerae via spontaneous mutations, horizontal gene transfer, and by conjugative plasmids (Kitaoka et al., 2011). V. cholerae strains that are resistant to the recommended antimicrobial treatment compounds (e.g., co-trimoxazole, chloramphenicol, sulphonamides, and nalidixic acid), were reported in South Asia and Africa. Resistance to cotrimoxazole and furazolidone in sub Saharan Africa and Bangladesh is increasing while resistance to tetracycline varies from year to year (Laxminarayan et al., 2013).
Antimicrobial susceptibilities studies of V. cholerae are mainly conducted on O1 and O139 clinical strains. Studying environmental strains is important because humans are usually infected from the environment. Here we studied antimicrobial susceptibilities of environmental V. cholerae non-O1/non-O139 strains that were isolated from chironomids, fish, and waterfowl species that are considered their natural reservoirs and vectors. Pathogenic V. cholerae strains share the same niche with the non-pathogenic strains as was found for V. cholerae in fish and waterfowl (Halpern and Izhaki, 2017; Laviad-Shitrit et al., 2018). Thus, resistance to antimicrobial compounds can be transferred from the non-pathogenic to the pathogenic strains inside their mutual host.
Isolates from waste water were found to be significantly more resistant to antimicrobial compounds relative to surface water. Waste water facilities are considered as hotspots for horizontal resistance gene transfer (Bouki et al., 2013). Acinetobacter strains isolated from waste water were resistant to amoxicillin/clavulanic acid, chloramphenicol, and rifampin, but not to ciprofloxacin, colistin, gentamicin, rifampin, sulfisoxazole, and trimethoprim (Zhang et al., 2009). Amoxicillin, ciprofloxacin, tetracycline, and sulfamethoxazole residues were detected in raw and treated waste water (Novo et al., 2013). In the current study, chironomid egg masses were sampled from a waste water stabilization plant. Isolates from chironomids showed higher ampicillin MIC values compared to waterfowl and fish isolates. Nevertheless, only one isolate was resistant to ampicillin and two were resistant to azithromycin (Table 3). V. cholerae is embedded in a biofilm within the gelatinous matrix that surrounds the egg mass, and thus it is probably protected from exposure to the antimicrobial compounds that are present in the waste water. Moreover, significant differences were obtained in MIC values of ampicillin, chloramphenicol, and SXT between the 2 years of sampling (2005 and 2009).
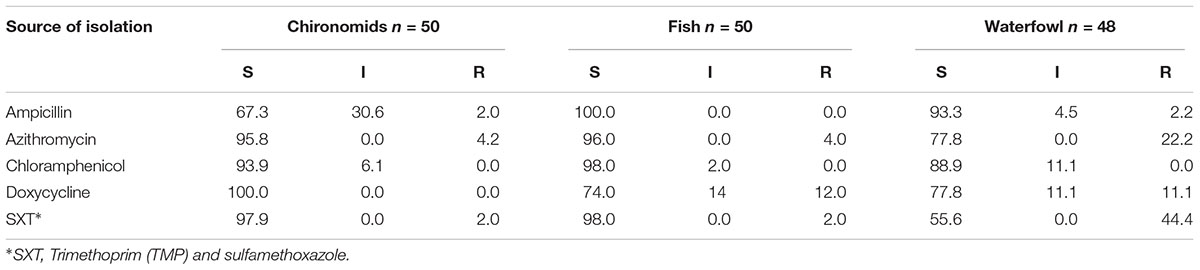
TABLE 3. Percentage of isolates that were found susceptible (S), intermediate (I), or resistant (R) to different antimicrobial agents in accordance to the Clinical and Laboratory Standards (CLSI, 2018; see also Supplementary Table S3).
Since 1940, antimicrobial agents have been added to animals’ food in order to reduce production costs and improve growth (Aarestrup, 2000). To date there is still a massive use of antimicrobial agents in animals’ food. In Israel, fish food in fish ponds is usually supplemented with one of the following antimicrobial compounds: oxytetracycline hydrochloride, sulfadiazine trimethoprim, and florfenicol. When transferring juvenile fish (weighing up to 100 g) between pools, the water is supplemented with oxytetracycline hydrochloride that is an analog of tetracycline (doxycycline) and is also used in humans (An et al., 2015). Indeed, the MIC90 of doxycycline in the fish isolates was the highest compared to the isolates from the other environmental reservoirs tested in the current study (Table 1). Isolates that were resistant to doxycycline were isolated in the current study from the following fish pond species; tilapia, grass carps, and Jordan mouthbrooder (Table 2 and Supplementary Table S1). Although there is a minor difference between the different antimicrobial agents used for humans or animals, in some countries the amounts of antimicrobial agents that are used in agriculture and aquaculture are four times higher than that used in medicine (Laxminarayan et al., 2013).
Different antimicrobial drug resistance mechanisms were found in V. cholerae strains (reviewed in Kitaoka et al., 2011). For example, antimicrobial resistance can be carried on mobile genetic elements like conjugative plasmids, SXT elements and integrons. Integrating conjugative elements (ICEs) are transferred by cell contact and then integrate into the chromosome. The first Integrating conjugative element carrying antibiotic resistance genes was described in O139 strain isolated in India, in 1992 (Waldor et al., 1996). This ICE was named SXT element as it carried genes for SXT resistance (Beaber et al., 2004; Kitaoka et al., 2011). Since then, many strains that acquired SXT elements were detected all around the globe (Hochhut et al., 2001; Burrus et al., 2006; Kitaoka et al., 2011). Interestingly, in the current study we found that the percentage of antimicrobial resistant V. cholerae isolates from waterfowl intestines was the highest, compared to antimicrobial resistant isolates from chironomids and fish (Table 3). Thus, it may be that waterfowl act as reservoirs and vectors of V. cholerae strains which carry resistant conjugative elements and that waterfowl are spreading these genetic elements, globally.
Conclusion
Studying the susceptibility of environmental pathogenic bacteria to antimicrobial agents is very important because V. cholerae causes disease originating from human exposure to environmental strains. Waterfowl species may be the vectors by which antimicrobial resistant strains and or resistant conjugative elements are spread all over the globe. Further study is needed to understand the bacterial antimicrobial resistance profiles and the dissemination of antimicrobial resistance strains via waterfowl.
Author Contributions
SL-S, II, AP, and MH conceived and designed the experiments. SL-S and AP performed the experiments. AP, II, and MH contributed reagents, materials, and analysis tools. SL-S and YS analyzed the data. SL-S and MH wrote the paper. All authors discussed the results, reviewed, and commented.
Funding
This work was supported in part by a grant from the Israel Science Foundation (ISF Grant No. 296/16) and in part by the Binational Science Foundation (BSF Grant No. 2015103).
Conflict of Interest Statement
The authors declare that the research was conducted in the absence of any commercial or financial relationships that could be construed as a potential conflict of interest.
Supplementary Material
The Supplementary Material for this article can be found online at: https://www.frontiersin.org/articles/10.3389/fmicb.2018.01726/full#supplementary-material
References
Aarestrup, F. M. (2000). Occurrence, selection and spread of resistance to antimicrobial agents used for growth promotion for food animals in Denmark. Acta Pathol. Microbiol. Immunol. Scand 101, 1–48.
Acosta, C. J., Galindo, C. M., Kimario, J., Senkoro, K., Urassa, H., Casals, C., et al. (2001). Cholera outbreak in southern Tanzania: risk factors and patterns of transmission. Emerg. Infect. Dis. 7, 583–587. doi: 10.3201/eid0707.017741
An, X., Zhuo, S., Zhang, P., and Zhu, C. (2015). Carbon dots based turn-on fluorescent probes for oxytetracycline hydrochloride sensing. RSC Adv. 5, 19853–19858. doi: 10.1039/C4RA16456C
Beaber, J. W., Hochhut, B., and Waldor, M. K. (2004). SOS response promotes horizontal dissemination of antibiotic resistance genes. Nature 427, 72–74. doi: 10.1038/nature02241
Bier, N., Schwartz, K., Guerra, B., and Strauch, E. (2015). Survey on antimicrobial resistance patterns in Vibrio vulnificus and Vibrio cholerae non-O1/non-O139 in Germany reveals carbapenemase-producing Vibrio cholerae in coastal waters. Front. Microbiol. 6:1179. doi: 10.3389/fmicb.2015.01179
Bouki, C., Venieri, D., and Diamadopoulos, E. (2013). Detection and fate of antibiotic resistant bacteria in wastewater treatment plants: a review. Ecotoxicol. Environ. Saf. 91, 1–9. doi: 10.1016/j.ecoenv.2013.01.016
Broza, M., and Halpern, M. (2001). Chironomid egg masses and Vibrio cholerae. Nature 412:40. doi: 10.1038/35083691
Burrus, V., Marrero, J., and Waldor, M. K. (2006). The current ICE age: biology and evolution of SXT-related integrating conjugative elements. Plasmid 55, 173–183. doi: 10.1016/j.plasmid.2006.01.001
CDC (2013). Antibiotic Resistance Threats in the United States. Washington, DC: US Department of Health and Human Services.
Ceccarelli, D., Chen, A., Hasan, N. A., Rashed, S. M., Huq, A., and Colwell, R. R. (2015). Non-O1/Non-O139 Vibrio cholerae carrying multiple virulence factors and V. cholerae O1 in the Chesapeake Bay. Maryland Appl. Environ. Microbiol. 81, 1909–1918. doi: 10.1128/AEM.03540-14
CLSI (2018). M45. Methods for Antimicrobial Dilution and Disk Susceptibility Testing of Infrequently Isolated or Fastidious Bacteria; Proposed Guideline, 3rd. Edn. Wayne, PA: CLSI.
Deshayes, S., Daurel, C., Cattoir, V., Parienti, J. J., Quilici, M. L., and de La Blanchardière, A. (2015). Non-O1, non-O139 Vibrio cholerae bacteraemia: case report and literature review. Springerplus 4:575. doi: 10.1186/s40064-015-1346-3
du Preez, M., van der Merwe, M. R., Cumbana, A., and le Roux, W. (2010). A survey of Vibrio cholerae O1 and O139 in estuarine waters and sediments of Beira, Mozambique. Water SA 36, 615–620. doi: 10.4314/wsa.v36i5.61995
Halpern, M., Broza, Y. B., Mittler, S., Arakawa, E., and Broza, M. (2004). Chironomid egg masses as a natural reservoir of Vibrio cholerae non-O1 and non-O139 in freshwater habitats. Microb. Ecol. 47, 341–349. doi: 10.1007/s00248-003-2007-6
Halpern, M., and Izhaki, I. (2017). Fish as hosts of Vibrio cholerae. Front. Microbiol. 8:282. doi: 10.3389/fmicb.2017.00282
Halpern, M., Senderovich, Y., and Izhaki, I. (2008). Waterfowl—The missing link in epidemic and pandemic cholera dissemination? PLoS Pathog. 4:e1000173. doi: 10.1371/journal.ppat.1000173
Hochhut, B., Lotfi, Y., Mazel, D., Faruque, S. M., Woodgate, R., and Waldor, M. K. (2001). Molecular analysis of antibiotic resistance gene clusters in Vibrio cholerae O139 and O1 SXT constins. Antimicrob. Agents Chemother. 45, 2991–3000. doi: 10.1128/AAC.45.11.2991-3000.2001
Hounmanou, Y. M. G. (2015). “Virulence characteristics and antibiotic susceptibility of Vibrio cholerae in low quality water, fish and vegetables,” in Proceedings of the Second International One Health Conference, Morogoro.
Kechker, P., Senderovich, Y., Ken-Dror, S., Laviad-Shitrit, S., Arakawa, E., and Halpern, M. (2017). Otitis media caused by Vibrio cholerae O100: a case report and review of the literature. Front. Microbiol. 8:1619. doi: 10.3389/fmicb.2017.01619
Kitaoka, M., Miyata, S. T., Unterweger, D., and Pukatzki, S. (2011). Antibiotic resistance mechanisms of Vibrio cholerae. J. Med. Microbiol. 60, 397–407. doi: 10.1099/jmm.0.023051-0
Koch, R. (1884). An address on cholera and its bacillus. Br. Med. J. 2, 403–407. doi: 10.1136/bmj.2.1235.403
Kumar, R., and Lalitha, K. V. (2013). Prevalence and molecular characterization of Vibrio cholerae O1, Non-O1 and Non-O139 in tropical seafood in Cochin, India. Foodborne Pathog. Dis. 10, 278–283. doi: 10.1089/fpd.2012.1310
Laviad, S., and Halpern, M. (2016). Chironomids’ relationship with Aeromonas species. Front. Microbiol. 7:736. doi: 10.3389/fmicb.2016.00736
Laviad-Shitrit, S., Izhaki, I., Arakawa, E., and Halpern, M. (2018). Wild waterfowl as potential vectors of Vibrio cholerae and Aeromonas species. Trop. Med. Int. Health 23, 758–764. doi: 10.1111/tmi.13069
Laviad-Shitrit, S., Lev-Ari, T., Katzir, G., Sharaby, Y., Izhaki, I., and Halpern, M. (2017). Great cormorants (Phalacrocorax carbo) as potential vectors for the dispersal of Vibrio cholerae. Sci. Rep. 7:7973. doi: 10.1038/s41598-017-08434-8
Laxminarayan, R., Duse, A., Wattal, C., Zaidi, A. K. M., Wertheim, H. F. L., Sumpradit, N., et al. (2013). Antibiotic resistance-the need for global solutions. Lancet Infect. Dis. 13, 1057–1098. doi: 10.1016/S1473-3099(13)70318-9
Nandi, B., Nandy, R. K., Mukhopadhyay, S., Nair, G. B., Shimada, T., and Ghose, A. C. (2000). Rapid method for species-specific identification of Vibrio cholerae using primers targeted to the gene of outer membrane protein OmpW. J. Clin. Microbiol. 38, 4145–4151.
Novo, A., André, S., Viana, P., Nunes, O. C., and Manaia, C. M. (2013). Antibiotic resistance, antimicrobial residues and bacterial community composition in urban wastewater. Water Res. 47, 1875–1887. doi: 10.1016/j.watres.2013.01.010
Ottaviani, D., Masini, L., and Bacchiocchi, S. (2003). A biochemical protocol for the isolation and identification of current species of Vibrio in seafood. J. Appl. Microbiol. 95, 1277–1284. doi: 10.1046/j.1365-2672.2003.02105.x
Paulsen, I. T., Brown, M. H., and Skurray, R. A. (1996). Proton-dependent multidrug efflux systems. Microbiol. Rev. 60, 575–608.
Petsaris, O., Nousbaum, J. B., Quilici, M. L., Le Coadou, G., Payan, C., and Abalain, M. L. (2010). Non-O1, non-O139 Vibrio cholerae bacteraemia in a cirrhotic patient. J. Med. Microbiol. 59, 1260–1262. doi: 10.1099/jmm.0.021014-0
Sack, D. A., Sack, R. B., Nair, G. B., and Siddique, A. K. (2004). Cholera. Lancet 363, 223–233. doi: 10.1016/S0140-6736(03)15328-7
Schürmann, D., Ebert, N., Kampf, D., Baumann, B., Frei, U., and Suttorp, N. (2002). Domestic cholera in Germany associated with fresh fish imported from Nigeria. Eur. J. Clin. Microbiol. Infect. Dis. 21, 827–828. doi: 10.1007/s10096-002-0832-z
Senderovich, Y., Gershtein, Y., Halewa, E., and Halpern, M. (2008). Vibrio cholerae and Aeromonas: Do they share a mutual host? ISME J. 2, 276–283. doi: 10.1038/ismej.2007.114
Senderovich, Y., and Halpern, M. (2013). The protective role of endogenous bacterial communities in chironomid egg masses and larvae. ISME J. 7, 2147–2158. doi: 10.1038/ismej.2013.100
Senderovich, Y., Izhaki, I., and Halpern, M. (2010). Fish as reservoirs and vectors of Vibrio cholerae. PLoS One 5:e8607. doi: 10.1371/journal.pone.0008607
Shaked, T. (2011). Evaluation and Assimilation of the Real-Time PCR Method for Detection of Vibrio cholerae in Drinking Water and for Monitoring V. cholerae and Aeromonas spp. Populations in Chironomid Egg Masses. Haifa: University of Haifa.
Shimada, T., Arakawa, E., Itoh, K., Okitsu, T., Matsushima, A., Asai, Y., et al. (1994). Extended serotyping scheme for Vibrio cholerae. Curr. Microbiol. 28, 175–178. doi: 10.1007/BF01571061
Waldor, M. K., Tschäpe, H., and Mekalanos, J. J. (1996). A new type of conjugative transposon encodes resistance to sulfamethoxazole, trimethoprim, and streptomycin in Vibrio cholerae O139. J. Bacteriol. 178, 4157–4165. doi: 10.1128/jb.178.14.4157-4165.1996
Keywords: V. cholerae, antimicrobial susceptibility, resistance, antibiotics, chironomid, fish, waterbird, waterfowl
Citation: Laviad-Shitrit S, Sharaby Y, Izhaki I, Peretz A and Halpern M (2018) Antimicrobial Susceptibility of Environmental Non-O1/Non-O139 Vibrio cholerae Isolates. Front. Microbiol. 9:1726. doi: 10.3389/fmicb.2018.01726
Received: 12 April 2018; Accepted: 11 July 2018;
Published: 02 August 2018.
Edited by:
Daniela Ceccarelli, Wageningen Bioveterinary Research (WBVR), NetherlandsReviewed by:
Durg Vijai Singh, Institute of Life Sciences (ILS), IndiaBen Davies Tall, United States Food and Drug Administration, United States
Copyright © 2018 Laviad-Shitrit, Sharaby, Izhaki, Peretz and Halpern. This is an open-access article distributed under the terms of the Creative Commons Attribution License (CC BY). The use, distribution or reproduction in other forums is permitted, provided the original author(s) and the copyright owner(s) are credited and that the original publication in this journal is cited, in accordance with accepted academic practice. No use, distribution or reproduction is permitted which does not comply with these terms.
*Correspondence: Avi Peretz, YXBlcmV0ekBwb3JpYS5oZWFsdGguZ292Lmls Malka Halpern, bWhhbHBlcm5AcmVzZWFyY2guaGFpZmEuYWMuaWw=