- 1Centro de Investigación en Estructuras Microscópicas, Universidad de Costa Rica, San Pedro, Costa Rica
- 2Department of the Geophysical Sciences, University of Chicago, Chicago, IL, United States
- 3Departamento de Bioquímica, Escuela de Medicina, Universidad de Costa Rica, San Pedro, Costa Rica
- 4Centro de Investigación en Biología Celular y Molecular, Universidad de Costa Rica, San Pedro, Costa Rica
- 5Department of Conservation and Research, Zoo Miami, Miami, FL, United States
- 6Department of Biology, University of Massachusetts, Boston, MA, United States
- 7Smithsonian Tropical Research Institute, Panama City, Panama
- 8Department of Biology, University of South Dakota, Vermillion, SD, United States
- 9Department of Ecology and Evolutionary Biology, University of Colorado, Boulder, Boulder, CO, United States
Amphibian skin is a suitable environment for rich communities of microorganisms, both beneficial and detrimental to the host. The amphibian cutaneous microbiota has been hypothesized to play an important role as symbionts, protecting their hosts against disease. Costa Rica has one of the most diverse assemblages of amphibians in the world and we know very little about the microbiota of these tropical animals. For comparison with other studies, we explore the diversity of the skin bacterial communities employing16S rRNA amplicon sequencing of swab samples from twelve species of frogs at La Selva Biological Station in Sarapiquí, Heredia province. The predominant phylum detected in our studies was Proteobacteria, followed by Bacteroidetes and Actinobacteria, with these three phyla representing 89.9% of the total bacterial taxa. At the family level, Sphingobacteriaceae and Comamonadaceae were highly represented among samples. Our results suggest that host species and host family are significant predictors of the variation in microbiota composition. This study helps set the foundation for future research about microbiota composition and resilience to unfavorable conditions, leading to improvement in managing strategies for endangered amphibian species.
Introduction
Dramatic declines in amphibian populations around the world have been a subject of research in recent years. Since the 1980s, amphibian populations have declined to the point where 42% of the 7854 species described worldwide (AmphibiaWeb, 2018) have experienced some form of population declines (Stuart et al., 2004; Whittaker et al., 2013). Population declines in amphibians have been attributed to several factors, mainly related to human activities such as habitat destruction, pesticide abuse and climate change, among others (Whitfield et al., 2007; Kerby et al., 2011). However, population declines in protected areas, so called “enigmatic declines,” have drawn the attention to the emerging disease chytridiomycosis, caused by the fungus Batrachochytrium dendrobatidis (Bd) (Lips et al., 2006; Whitfield et al., 2007, 2012; Lips, 2016).
Costa Rica has become a focus for amphibian research. In the late 1990s, a study revealed declines of up to 40% of amphibian populations in the Monteverde Cloud Forest Reserve (Pounds et al., 1997). Also, Lips (1998) documented population declines in Costa Rican amphibians at Las Tablas. Another hotspot for amphibian studies in Costa Rica is La Selva Biological Station in Heredia Province. This is a 16 km2 private protected area, managed by the Organization of Tropical Studies, where amphibians have been studied for almost 40 years. Whitfield and collaborators analyzed data collected for over 35 years and they found that all species of terrestrial amphibians declined approximately 75% in this site. Interestingly, that study showed a correlation between population declines and a climate driven reduction of the quantity of leaf litter, but no correlation to Bd emergence was detected (Whitfield et al., 2007).
Over the last years, next generation sequencing technologies allowed scientists to explore oceans, soils, humans and a variety of host related microbiota (Jani and Briggs, 2014). These technologies can be implemented to study amphibians, specifically their skin microbiota. Skin is especially important for amphibian physiology; it is responsible for the exchange of respiratory gasses and osmoregulation (Voyles et al., 2009). Furthermore, the skin is in continuous exposure to microorganisms in the environment, hence these potentially symbiotic microbes may be involved in different physiological processes and interacting with pathogens such as Bd (Madison et al., 2017). Knowledge of the ecology of bacterial communities associated with amphibians has only a few years of development (Bletz et al., 2017a). Previous research showed that bacterial communities of amphibian skin were significantly different to their environments and one of the most important factors driving the structure of the microbiota was host species (McKenzie et al., 2012; Kueneman et al., 2013; Sabino-Pinto et al., 2016). More recently, it has been suggested that environmental factors may also play an important role in the variation of the amphibian bacterial microbiota (Muletz et al., 2017; Bird et al., 2018; Varela et al., 2018). It has also been reported that bacterial communities can vary according to geography (Abarca et al., 2018; Rebollar et al., 2018), ecomorphs (Bletz et al., 2017a) and ontogeny (Longo et al., 2015).
The discovery of bacteria with antagonistic activity to Bd (Harris et al., 2006, 2009) opens the possibility of employing new strategies to control the pathogen; however, due to the rapid loss of species and habitat, it is necessary to speed up the search for beneficial bacteria to be employed in captivity or in wildlife (Flechas et al., 2017). To accelerate this search, the use of culture independent techniques can be a very efficient tool to describe local bacterial communities (Rebollar et al., 2016a). Due to the variation in the inhibitory response of bacteria to Bd (Antwis and Harrison, 2018), and because skin bacterial communities differed strongly from those of the surrounding environment (Bletz et al., 2017a), it has been recommended to implement mitigation strategies at the local level, using bacteria to counteract the circulating pathogen in the same region (Flechas et al., 2017). The aim of this study is to explore and compare the diversity of the skin bacterial communities of twelve tropical amphibian species at La Selva Biological Station in Costa Rica. Further, these communities are compared to previous studies with other amphibian species and were examined for potential anti-Bd function. Our goal is to describe this host-associated microbiota and set the foundation to understand microbiota composition and potential interaction with Bd, as well as to describe host specific relationships between amphibians and microbes in Costa Rica.
Materials and Methods
Study Site, Species and Sampling
All sampling was performed at La Selva Biological Station in Sarapiquí, Heredia Province, northeastern Costa Rica (10°26′ N, 83°59′ W), between January 18th–19th, 2013. La Selva is a private biological reserve with elevation ranging from 35 m to 137 m.a.s.l., managed by the Organization for Tropical Studies for over 45 years and has been described as lowland wet forest. About 73% of the station territory is primary forest, the rest of the reserve includes forests regenerating from pasture and other agricultural uses (Whitfield et al., 2012). We sampled 66 specimens within twelve species of frogs from nine different families. These included 8 terrestrial species, 3 arboreal and one arboreal-aquatic species (Table 1 and Supplementary Table 1). We sampled by visual encounter on the trails, every specimen was kept in individual clean plastic bags. Once in the lab, each amphibian individual was handled with new sterile nitrile gloves. Each frog was washed for 7 s using 50 ml of sterile distilled water. Sterile swabs were rubbed 10 times on the ventral and dorsal areas and 5 more times on each side (Boyle et al., 2004; Bird et al., 2018). After skin swabbing, frogs were released to their natural environment in less than 3 h. The swabs were placed directly in dry labeled 1.5 ml tubes, transported to the laboratory the same day in an ice-filled container and stored at -80°C until DNA was extracted 2 days later.
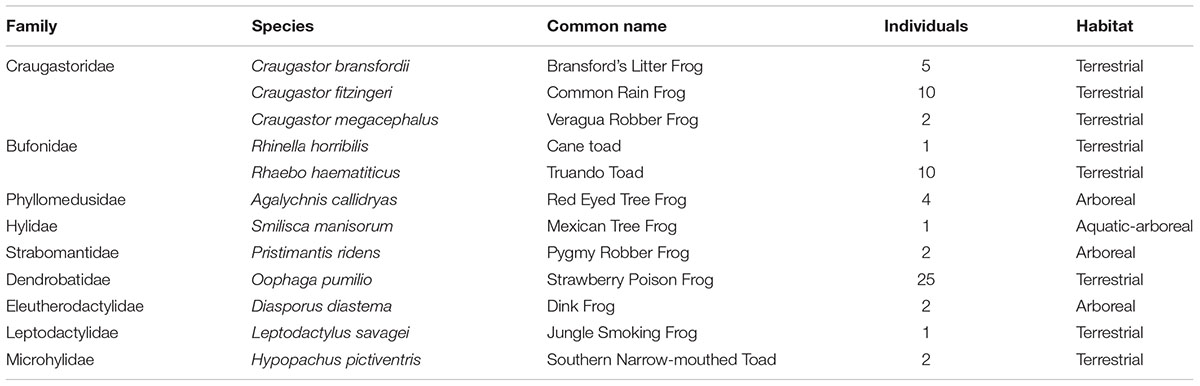
TABLE 1. Amphibian samples processed for skin bacteria community analysis at La Selva Biological Station.
DNA Extraction and Sequencing
DNA extractions were performed using the MOBIO PowerSoil Extraction kit (MO BIO, Carlsbad, CA, United States) according to manufacturer’s protocols, with the following modifications: samples were incubated at 65°C after the addition of reagent C1, the powerbead tubes were vortexed horizontally for 2 min (Lauber et al., 2008), and the reagent C6 was left on the filter for 5 min before the final elution. Eighteen negative controls were extracted from sterile swabs. DNA extractions were transported in ice packs to the University of Colorado Boulder and stored at -80°C for 15 days until barcoding addition. DNA was sequenced 3 weeks later. PCR reactions contained 11 μL PCR water, 10 μL of 5 Prime Master Mix (5 PRIME GmbH), 1.0 μL each of the forward and reverse primers (at 10 μM), 1.0 μL MgCl2, and 1.0 μL genomic DNA. PCR primers (F515/R806) were used to target the V4 region of 16S rRNA. This primer pair amplifies the 533–786 region in Escherichia coli strain 83972 genome sequence (Greengenes accession no. prokMSA_id:470367). The reverse PCR primer contained a 12-base Golay error-correcting barcode originally developed by Caporaso et al. (2011). PCR conditions consisted of an initial denaturation step of 94°C for 3 min, followed by 35 cycles at 94°C for 45 s, 50°C for 60 s, and 72°C for 90 s; and final extension of 10 min at 72°C. From 18 negative controls, five yielded PCR bands, these samples were sequenced as described below and filtered out from the sequence analysis. Each sample was amplified in triplicate and combined. Amplicons were quantified using the Quant-IT Picogreen dsDNA reagent in 1X TE buffer. A composite sample for sequencing was created by combining equimolar ratios of amplicons from the individual samples and cleaned using the MO BIO UltraClean PCR clean up DNA purification kit. Purity and DNA concentration were determined using a NanoDrop spectrophotometer (Thermo Scientific). Samples were processed on an Illumina MiSeq Sequencer (Illumina Inc., San Diego, CA, United States) at the Next Generation Sequencing Facility located at the University of Colorado Boulder. The resulting raw sequences for each sample were deposited as Sequence Read Archive (SRA) in the NCBI BioProject ID PRJNA470570.
Sequence Analyses
Quantitative Insights Into Microbial Ecology (QIIME v1.9.0) (Caporaso et al., 2010b) was employed for all sequence analyses. Sequences were filtered for quality and assigned to their respective sample using default settings. The resulting 3,551,436 reads were clustered into 89,504 operational taxonomic units (OTUs) according to the subsampling open reference protocol using the Greengenes May 2013 97% reference sequences1. The reads that did not match the reference dataset were clustered into de novo OTUs. Chimeric reads, reads matching archaea and mitochondria, as well as low abundance OTUs (lower than 0.00005 of the total read count) were removed from downstream analyses. We detected 116 different OTUs in the negative controls, of which 10 had abundance percentages greater than 1.0%, these OTUs were filtered and removed from further analyses. Samples were subsequently rarefied at 7,800 reads per sample to normalize read counts across samples. Sequences were aligned to the Greengenes (v. gg_13_8) reference alignment using PyNast (Caporaso et al., 2010a). We then constructed a phylogenetic tree using FastTree according to standard procedures within QIIME. Taxonomic summaries at the phylum and genus levels were built by using QIIME’s plot tables L2 and L6, respectively, using the melt function in the RESHAPE2 R package (Wickham, 2007). Then we calculated the core OTUs (OTUs present in 90% of the samples) and the more abundant OTUs, a heatmap of these OTUs was built using heatmap.3 function in R. We determined Shannon diversity index and Faith’s phylogenetic diversity (PD_whole_tree) and employed a parametric ANOVA test to compare the means of the estimators by species and habitat.
Beta diversity analysis was performed with a multidimensional scaling plot (PCoA) using the weighted Unifrac distances and plotted with the ordinate function in the PHYLOSEQ R packages (McMurdie and Holmes, 2013). We also performed analysis of similarity (ANOSIM) and permutational multivariate analysis of variance (PERMANOVA) to examine the contribution of host species, host family and habitat in the variation of bacterial community composition. Additionally, we employed an ADONIS test to examine the simultaneous effects of host species and habitat. We compared the diversity of tropical versus temperate amphibian skin microbiota using our Costa Rica data set and similar datasets of amphibians from California and Virginia (Kueneman et al., 2013; Loudon et al., 2014). Finally, the OTU table was filtered to retain only reads matching sequences of bacteria from the Antifungal Isolates Database (Woodhams et al., 2015) that were previously shown to inhibit the growth of Bd. We calculated the proportion of these putatively anti-Bd bacteria for each sample and tested for differences among species and between habitat types. Non-parametric tests were employed in IBM SPSS Statistics v21 because data did not meet the assumptions of equality of variance.
Results
After rarefaction, we obtained a total of 2,621,143 good quality sequences and 6330 OTUs distributed in 38 bacterial phyla (Figure 1A). Eight out of the twelve species under study were dominated by the phylum Proteobacteria (43.95% of the total OTUs). Other abundant phyla were Bacteroidetes (35.59%) and Actinobacteria (8.79%), while Acidobacteria (3.91%), Firmicutes (2.61%), Verrucomicrobia (1.79%) and Planctomycetes (1.04%) were other phyla represented in at least 1% of the OTUs. Among the four species that were not dominated by Proteobacteria, Hypopachus pictiventris, Leptodactylus savagei, and Rhaebo haematiticus presented a majority of Bacteroidetes OTUs, with 89.02, 69.47, and 39.40%, respectively. The bacterial community associated with Rhinella horribilis showed a dominance of Bacteroidetes (43.23%) and Actinobacteria (42.08%), besides this species, only Smilisca manisorum presented a relative abundance of actinomycete OTUs above 10% (23.72%).
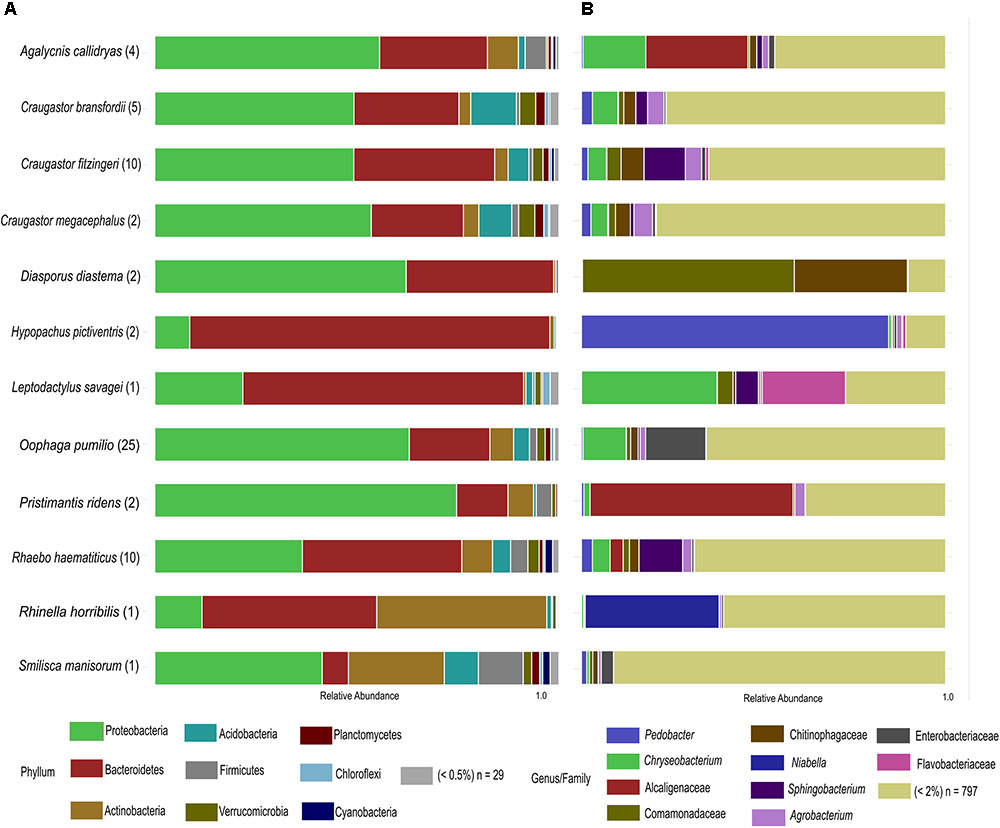
FIGURE 1. Taxonomic profile of skin bacterial communities of Costa Rican frogs from La Selva Biological Station. (A) Phylum-level relative abundance profiles of the host-associated skin bacterial communities. OTUs with abundances lower than 0.5% within each Phylum were clustered together. (B) Genus/family level relative abundance profiles of the frog-associated skin bacterial communities. OTUs with percentages lower than 2% of the microbiota composition within species were clustered together. The number of individuals sampled for each species is shown in parenthesis.
We identified 463 bacterial families in our dataset. In terms of relative abundance of OTUs, the Sphingobacteriaceae family presented the highest percentage (13.82%), followed by Comamonadaceae (9.08%), Weeksellaceae (8.14%), Chitinophagaceae (7.95%), and Alcaligenaceae (7.46%) (Supplementary Table 2). The phylogenetic distribution at family and genus level was noteworthy (Figure 1B), for example, showing the dominance of Pedobacter in the skin microbiota of H. pictiventris (84.34%). In C. bransfordii the bacterial community was weakly dominated by OTUs of the genus Chryseobacterium (7.00%) and Agrobacterium (4.51%). The bacterial community of C. fitzingeri was dominated by OTUs of the genus Sphingobacterium (11.34%) and one OTUs in Chitinophagaceae family (6.28%); C. megacephalus had a bacterial community dominated weakly by Agrobacterium (5.10%). The bacterial community of O. pumilio had a dominance of OTUs related to Enterobacteriaceae (16.54%) and the bacterial community of R. haematiticus was dominated by the genus Sphingobacterium (11.98%). The bacterial community of A. callidryas was dominated by Alcaligenaceae OTUs (28.04%). The Cane toad, R. horribilis, showed an abundance of OTUs in the genus Niabella, family Chitinophagaceae (36.75%). P. ridens was dominated by OTUs in the family Alcaligenaceae (55.7%), while the L. savagei community was dominated by Chryseobacterium (37.21%) followed by another OTU belonging to the family Flavobacteriaceae (22.82%).
Figure 2 shows a heatmap of the core microbiota (OTUs that are present in 90% of the samples), as well as the OTUs that have relative abundances significantly different between the bacterial communities. All amphibian species shared OTUs belonging to the genera Chryseobacterium and Pedobacter, as well as several OTUs assigned to the families Alcaligenaceae, Comamonadaceae and Rhizobiaceae. The three most abundant OTUs detected in this study were assigned to the family Enterobacteriacea and to the genera Methylibium and Pedobacter.
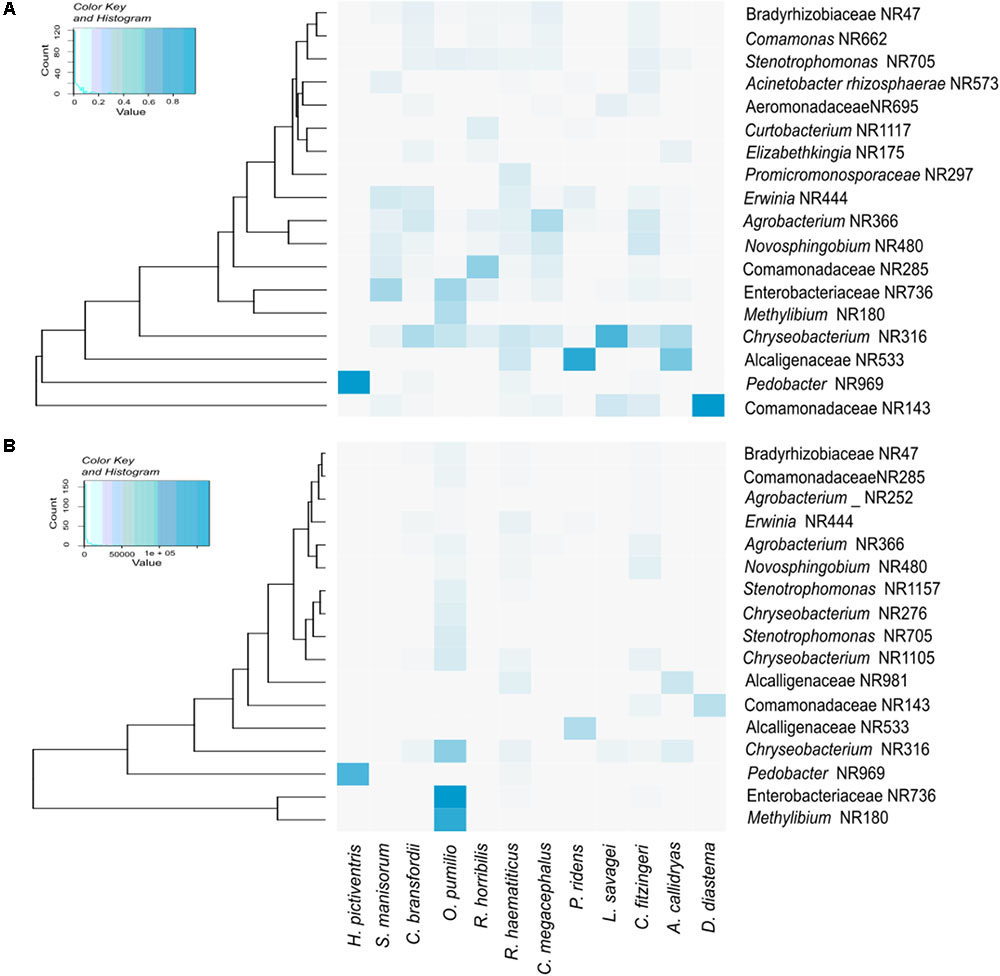
FIGURE 2. Heatmaps depicting the core skin bacteria present in 90% of samples (A), and the OTUs with higher relative abundance (B).
The relative abundance of OTUs varied among individuals within each species, as follows: In O. pumilio (n = 25), 12 individuals were dominated by Enterobacteriaceae OTUs, 7 individuals were dominated by Methylibium OTUs, while the other 6 had higher abundance of Chitinophagaceae and Stenotrophomonas. In C. fitzingeri (n = 10), 3 individuals were dominated by Sphingobacterium OTUs, 2 by Chryseobacterium OTUs and the 5 remaining individuals each had a different most abundant OTU. Regarding R. haematiticus (n = 10), 4 individuals were dominated by Sphingobacterium OTUs, 2 by Chryseobacterium OTUs and the 4 remaining individuals each had a different most abundant OTU. In two species, C. bransfordii (n = 5) and A. callidryas (n = 4), all individuals were dominated by a different OTU. Finally, all species with 2 individuals sampled had the same dominant OTU (G. pictiventris, P. ridens and D. diastema), except for C. megacephalus, in which one individual was dominated by Chryseobacterium and the other by Agrobacterium OTUs.
The beta diversity analysis did not show clear clustering among the different bacterial communities; however, some trends are worth noticing. Figure 3 shows H. pictiventris communities separately from the rest of the frogs. Other species did cluster together such as D. diastema, while some samples of O. pumilio and one of A. callidryas grouped close to them. Most O. pumilio samples are widely distributed around the plot, indicating great variation within this species. Beta-diversity analysis showed that host species (ANOSIM R = 0.416, p = 0.01; PERMANOVA PseudoF = 3.388, p = 0.001) and host family (ANOSIM R = 0.392, p = 0.01; PERMANOVA PseudoF = 3.751, p = 0.001) are significant predictors of the variation in bacterial community composition. Contrary, host habitat did not show significant results (ANOSIM R = 0.171, p = 0.11; PERMANOVA PseudoF = 1.866, p = 0.0599). The combined effect of host species and host habitat also influenced significantly the variation in bacterial community composition (ADONIS F = 3.3884, p = 0.001). Regarding alpha-diversity, both Shannon and phylogenetic diversity indexes presented similar results (Figure 4): they did not vary significantly between amphibian species (P > 0.005 in both indexes). Frogs of the genus Craugastor, O. pumilio, R. haematiticus and S. baudinii have the most diverse skin bacterial communities. On the other hand, D. diastema, P. ridens and H. pictiventris showed lower diversity (Supplementary Table 3). Regarding habitat, there was a significant variation between indexes according to habitat (p < 0.005 in both), showing a higher diversity associated to terrestrial species when compared to arboreal species. On the other hand, there were no differences between diversity indexes when we compared terrestrial species versus aquatic species or arboreal species versus aquatic ones (p > 0.005 in all indexes).
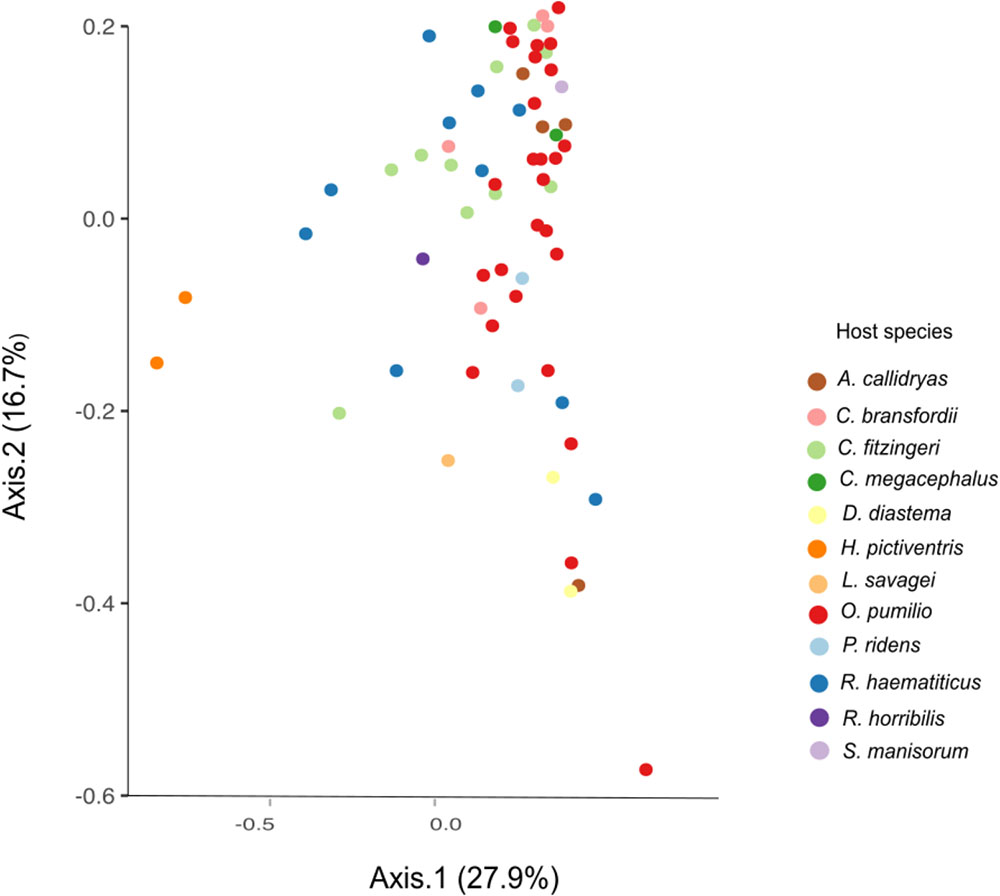
FIGURE 3. Beta diversity PCoA by weighted UNIFRAC of amphibian skin bacterial communities from La Selva Biological Station. Axes show the major components that explain 44.6% of the differences between samples.
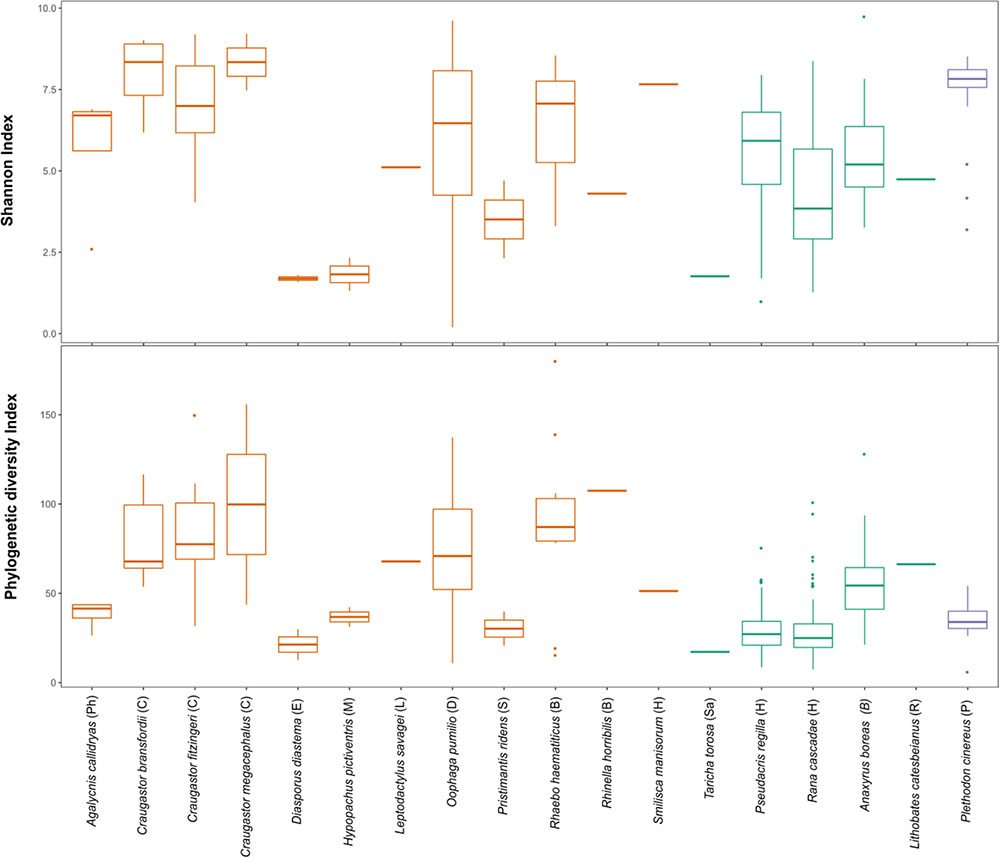
FIGURE 4. Shannon index and Faith Phylogenetic diversity of amphibian skin bacterial communities from Costa Rica (orange), California (green) and Virginia (purple). Letters in parenthesis represent family: Ph, Phyllomedusidae; C, Craugastoridae; E, Eleutherodactylidae; M, Microhylidae; L, Leptodactylidae; D, Dendrobatidae; S, Strabomantidae; B, Bufonidae; H, Hylidae; Sa, Salamandridae; R, Ranidae; P, Plethodontidae.
Finally, we compared tropical and temperate species, but no significant latitudinal differences were found in alpha diversity; furthermore, two overlapping families, Bufonidae and Hylidae, also presented similar alpha diversity values (Figure 5). The proportion of putatively anti-Bd bacteria showed no significant differences among species (Kruskal–Wallis test, p = 0.149) or between arboreal and terrestrial habitat types (Mann–Whitney U-test, 0.714). However, all 12 species and all samples contained putatively anti-Bd bacterial taxa with an average of 13.3% of the 16S rRNA sequencing reads (Figure 5).
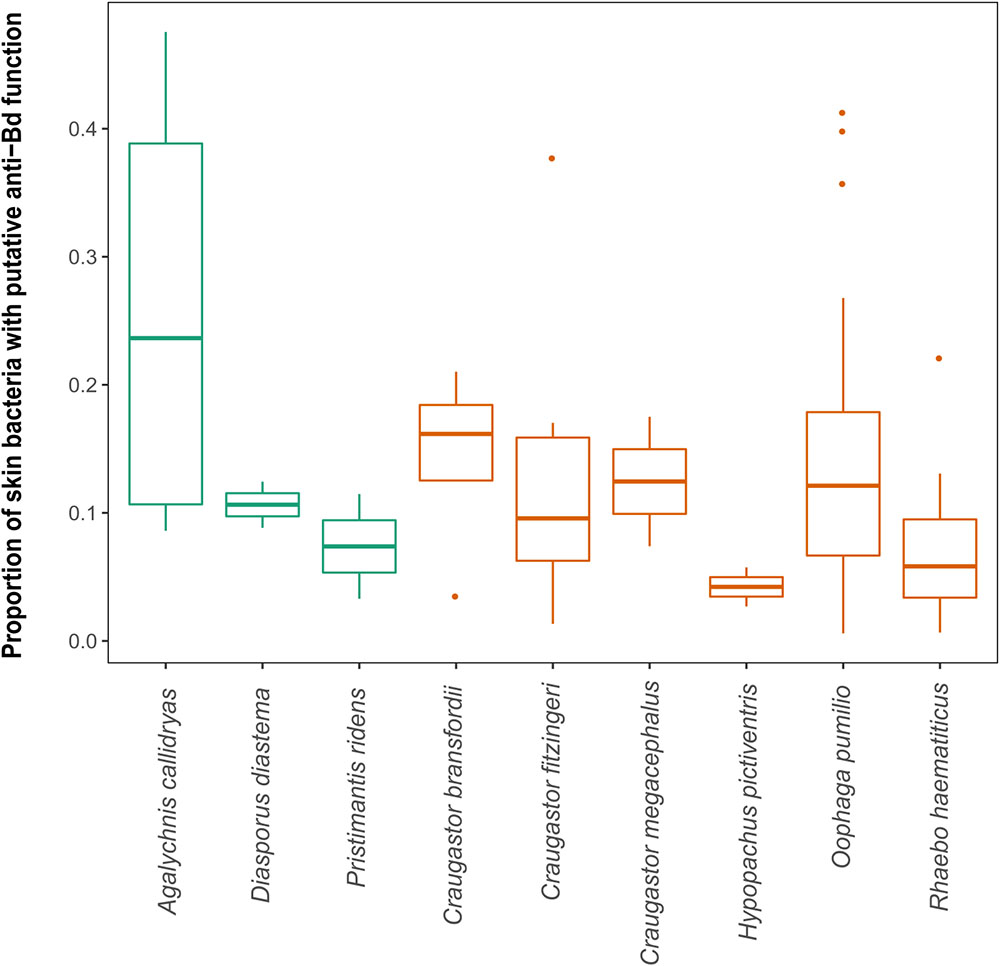
FIGURE 5. Proportion of putatively anti-Bd bacteria from the Antifungal Isolates Database. Note that 9 of the 12 species are shown after removing species with only one sample. No significant differences were found in proportion of putatively anti-Bd bacteria among species or between arboreal (orange) and terrestrial (green) habitat types.
Discussion
Amphibian skin is an important organ for electrolyte and gas exchange with the environment, and many diseases and contaminants can affect amphibians through it (Campbell et al., 2012). Unfortunately, very little is known about amphibian-associated microbiota in a biodiversity hotspot that has suffered from severe amphibian decline such as Costa Rica. To start bridging this knowledge gap, we studied the skin microbiota of several frog species at La Selva Biological Station. We found that skin bacterial communities differ among amphibian species. Species-level differences in the amphibian skin microbiota have been previously documented in temperate (McKenzie et al., 2012; Kueneman et al., 2013; Walke et al., 2014) and tropical systems (Flechas et al., 2012; Belden et al., 2015; Rebollar et al., 2016b). Furthermore, we found that host family is also a good predictor of bacterial community variation. In tropical regions there are more frog families occupying different microhabitats, this is not the case for temperate zones, where host family was not a strong driver of microbiota when compared to host habitat (Kueneman et al., 2013).
It has been reported that host ecology and habitat are more important factors of variation than host taxonomy (Bletz et al., 2017a; Muletz et al., 2017; Bird et al., 2018), and that most amphibian microbiota comes from the environment (Rebollar et al., 2016b). Despite this, we did not find that habitat was a predictor of variation in the bacterial community of the population under study. This result may be caused by the limited sampling range, or it could reflect amphibian ecology at La Selva, where species transition between different habitats. For example, O. pumilio is principally terrestrial but they climb trees to bring tadpoles to bromeliads, R. haematiticus can be seen in the forest floor as well as in rivers or even in low vegetation and C. fitzingeri can be observed in forest leaf litter, vegetation, near rivers and open areas with pastures (Savage, 2002). However, further research is needed to confirm these results, including analyzing more species from aquatic habitats.
Proteobacteria was the most commonly detected phylum and was more abundant in leaf litter species. This is the main phylum recovered from soils (Kim et al., 2014), tree leaves (Kembel et al., 2014) and amphibians (Belden et al., 2015; Bletz et al., 2017a; Varela et al., 2018) in the tropics. The other three dominant phyla agree with the main four phyla of bacteria associated with amphibians in temperate or tropical areas (Lauer et al., 2008; McKenzie et al., 2012; Walke et al., 2014; Becker et al., 2014; Belden et al., 2015). However, we found that Comamonadaceae and Alcaligenaceae were the most common Proteobacteria families in the bacterial communities of the amphibians sampled, in contrast with other studies in both tropical and temperate zones that identified Pseudomonadaceae as the most abundant family (Lam et al., 2010; Rebollar et al., 2016b, 2018).
Bacteroidetes was the second most abundant phylum in skin of the frogs at La Selva. This phylum is also widely distributed in the environment, including soils, sediments and sea water (Thomas et al., 2011). Bacteroidetes is common in four aquatic and terrestrial frog species. Important genera within this phylum present in the microbiota of amphibians at La Selva were Pedobacter, Chryseobacterium and Niabella. High dominance of Pedobacter OTUs was found in H. pictiventris. The abundance of this genus is important because it has been shown to have inhibitory activity against Bd (Harris et al., 2006; Lauer et al., 2008). Pedobacter has been isolated from multiple habitats and it can dominate aerobic communities in different aquatic and terrestrial environments, due to its high metabolic diversity (Gordon et al., 2009). H. pictiventris spends most of its life underground but in the breeding season they congregate in puddles in the forest (Savage, 2002). This sudden change of microclimate could affect the skin bacterial community allowing Pedobacter to thrive over other genera. However, further research is needed to establish whether these two organisms maintain a symbiotic relationship.
Craugastor spp., R. haematiticus, R. horribilis, and O. pumilio had higher overall bacterial diversity compared with the rest of species; P. ridens and H. pictiventris have the least bacterial diversity. At La Selva, alpha diversity metrics as Shannon and Phylogenetic diversity indexes show that typical leaf-litter dwelling families (Craugastoridae, Bufonidae and Dendrobatidae) have greater bacterial diversity than arboreal or aquatic families such as Hylidae, Phyllomedusidae, Leptodactylidae, Microhylidae or Eleutherodactylidae. It is possible that more soil bacteria can colonize amphibians in the leaf litter than in arboreal or aquatic habitats, this pattern agrees with previous studies (Belden et al., 2015; Rebollar et al., 2016b; Bletz et al., 2017a).
Our results show differences with similar studies performed in nearby Panama. In those studies, different frog species were analyzed (except for C. fitzingeri and A. callidryas), suggesting that differences in bacterial composition between countries may be due to different host species tested. One important difference is that Actinobacteria was the second most abundant phylum in frogs of Panama (Belden et al., 2015; Rebollar et al., 2016b), while in Costa Rica this group was in third place. For shared species, however, the alpha diversity indexes appear to be similar, with higher values for C. fitzingeri. The microbiota of C. fitzingeri from La Selva is enriched with OTUs of the Sphingobacteriaceae family, while those of Panama are enriched with other families such as Comamonadaceae and Moraxellaceae (Rebollar et al., 2018). The Shannon index of C. fitzingeri in Costa Rica is similar to that of C. fitzingeri in “Bd-naive” sites in Panamá (Rebollar et al., 2016b), although La Selva is a “Bd-endemic” site where it has been reported that C. fitzingeri along with other species of amphibians carry Bd (Whitfield et al., 2012). Additionally, in our study, in every species with more than 4 amphibians sampled, the most abundant OTU was not the same in all of them. Both observations suggest that there are intraspecific variations in amphibian bacterial communities, these variations are possibly associated with environmental factors, presence of Bd or internal population variations of amphibian species, as has been reported by other studies (Muletz et al., 2017; Abarca et al., 2018; Rebollar et al., 2018).
We found similarities in Shannon diversity index and phylogenetic diversity between temperate zones and tropical zones. At bacterial composition level, temperate zones frogs appear to have more Firmicutes than tropical ones (Kueneman et al., 2013; Loudon et al., 2014; Belden et al., 2015). It is important to point out that the temperate studies included mostly aquatic species, and only one terrestrial family: Bufonidae, while at La Selva we studied mostly terrestrial and arboreal species; therefore, we can only compare two families between Costa Rica and temperate zones: Bufonidae and Hylidae. Those families showed similar richness and phylogenetic diversity. In Bufonidae, Kueneman et al. (2013) found similar abundance of three phyla, the same found in R. horribilis at La Selva. Both in Kueneman et al. (2013) and in Belden et al. (2015) the aquatic temperate species was Lithobates catesbeianus, and they found different microbiota composition between tropical and temperate populations. To establish a better comparison, it is necessary to characterize the bacterial community of a fully aquatic tropical frog, ideally in the Family Ranidae. Even though the tropics present very different conditions than temperate zones, it is possible that the skin texture and habits of certain species may represent a more homogeneous microhabitat for some bacterial genera regardless of geographical location.
We recovered several sequences related to putatively anti-Bd bacterial strains in the Antifungal Isolates Database (Woodhams et al., 2015). This database has been used to determine proportions of OTUs of anti-Bd bacteria among other species of amphibians (Kueneman et al., 2016), as well as to study associations between the degrees of infection and the presence of OTUs related to inhibitory bacteria (Jani et al., 2017). Chryseobacterium and Pseudomonas comprised the majority of putatively anti-Bd bacteria from all species examined. These two genera have been reported as the most abundant among inhibitory Bd bacteria within their phylum (Park et al., 2014; Becker et al., 2015). In this study over 13% of sequences were related to putatively anti-Bd strains. This percentage of putatively anti-Bd bacteria is similar to that found using culture-based techniques in Costa Rican amphibians (11.1% in cell-free supernatant assays) (Madison et al., 2017). The presence of OTUs associated with putatively anti-Bd bacteria suggests the existence of bacteria that can help fight the disease, but this is still a hypothetical value. For this reason, inhibition tests with cultivable bacteria from each amphibian species (Antwis et al., 2015; Bletz et al., 2017b; Burkart et al., 2017) are necessary to confirm if some amphibians indeed carry higher proportions of anti Bd bacteria.
Understanding the microbial community in each region is one of the first steps to implement mechanisms to mitigate chytridiomycosis, including employing probiotic bacteria (Woodhams et al., 2016). Here we find that skin bacterial community of amphibians at La Selva varies according to species and family. Within the bacterial community there are potential symbiotic bacteria that should be the focus of future studies. It is important to know and describe local bacterial communities associated with amphibian skin to understand the mechanisms of interaction between these microorganisms and thus implement efficient bioaugmentation strategies (Flechas et al., 2012). This work represents an effort to characterize the bacterial communities of amphibians in Costa Rica. La Selva Biological Station is a tropical forest research center of worldwide recognition, and this research can serve as a foundation for further studies aimed at designing mitigation strategies to control the threat of Bd and human-associated factors as drivers of amphibian extinction.
Ethics Statement
This study was carried out in accordance with the recommendations of the Institutional Commission on Biodiversity at the University of Costa Rica. Our work with amphibians has been reviewed and approved by the Animal Care and Ethics Committee from the University of Costa Rica (Resolution CICUA-029-15).
Author Contributions
SW, JK, VM and AP-T conceived and designed the experiments. JA, GV, IZ, and CM-C performed the experiments. JA, GV, IZ, SW, DW, JK, and VM analyzed the data. JK, VM, and CM-C contributed reagents, materials, and analysis tools. JA, GV, IZ, DW, VM, and AP-T wrote the paper. SW, DW, JK, and CM-C reviewed draft on the paper.
Funding
This research was done with the support of the United States Fish and Wildlife Service (USFWS Number: 46-6003541) and the University of Costa Rica (Project Number: 801-B2-029). The Ministry of Science and Technology (MICIT) of Costa Rica supported JA through a PINN fellowship (849-PINN-2015-I). A National Science Foundation grant to VM (DEB: 1146284) supported 16S sequencing.
Conflict of Interest Statement
The authors declare that the research was conducted in the absence of any commercial or financial relationships that could be construed as a potential conflict of interest.
The reviewer MV declared a past co-authorship with several of the authors VM and DW to the handling Editor.
Acknowledgments
We thank the Organization for Tropical Studies for the access to La Selva Biological Station. Collecting permits were granted by the “Comisión Institucional de Biodiversidad” (Institutional Biodiversity Committee, University of Costa Rica; Resolution 014) and authorized by La Selva Biological Station. Materials were exchanged under a Biological Material Transfer Agreement between UCR and the University of Colorado signed on January 30, 2013. We thank CIEMIC and CIBCM staff at UCR for all their help and support. We thank Holly Archer, Juan Carlos Cambronero and Gilbert Alvarado for field support.
Supplementary Material
The Supplementary Material for this article can be found online at: https://www.frontiersin.org/articles/10.3389/fmicb.2018.02001/full#supplementary-material
Footnotes
References
Abarca, J. G., Zuniga, I., Ortiz-Morales, G., Lugo, A., Viquez-Cervilla, M., Rodriguez-Hernandez, N., et al. (2018). Characterization of the skin microbiota of the cane toad rhinella cf. marina in puerto rico and costa rica. Front. Microbiol. 8:2624. doi: 10.3389/fmicb.2017.02624
AmphibiaWeb (2018). University of California, Berkeley. Available at: http://amphibiaweb.org [accessed May 2, 2018].
Antwis, R. E., and Harrison, X. (2018). Probiotic consortia are not uniformly effective against different amphibian chytrid pathogen isolates. Mol. Ecol. 27, 577–589. doi: 10.1111/mec.14456
Antwis, R. E., Preziosi, R. F., Harrison, X. A., and Garner, T. W. (2015). Amphibian symbiotic bacteria do not show a universal ability to inhibit growth of the global panzootic lineage of Batrachochytrium dendrobatidis. Appl. Environ. Microbiol. 81, 3706–3711. doi: 10.1128/AEM.00010-15
Becker, M. H., Richards-Zawacki, C. L., Gratwicke, B., and Belden, L. K. (2014). The effect of captivity on the cutaneous bacterial community of the critically endangered Panamanian golden frog (Atelopus zeteki). Biol. Cons. 176, 199–206. doi: 10.1016/j.biocon.2014.05.029
Becker, M. H., Walke, J. B., Cikanek, S., Savage, A. E., Mattheus, N., Santiago, C. N., et al. (2015). Composition of symbiotic bacteria predicts survival in Panamanian golden frogs infected with a lethal fungus. Proc. R. Soc. B. 282, 28–81. doi: 10.1098/rspb.2014.2881
Belden, L. K., Hughey, M. C., Rebollar, E. A., Umile, T. P., Loftus, S. C., Burzynski, E., et al. (2015). Panamanian frog species host unique skin bacterial communities. Front. Microbiol. 6:1171. doi: 10.3389/fmicb.2015.01171
Bird, A. K., Prado-Irwin, S. R., Vredenburg, V. T., and Zink, A. G. (2018). Skin microbiomes of california terrestrial salamanders are influenced by habitat more than host phylogeny. Front. Microbiol. 9:442. doi: 10.3389/fmicb.2018.00442
Bletz, M. C., Archer, H., Harris, R. N., McKenzie, V. J., Rabemananjara, F. C. E., Rakotoarison, A., et al. (2017a). Host ecology rather than host phylogeny drives amphibian skin microbial community structure in the biodiversity hotspot of madagascar. Front. Microbiol. 8:1530. doi: 10.3389/fmicb.2017.01530
Bletz, M. C., Perl, R. G., Bobowski, B. T., Japke, L. M., Tebbe, C. C., Dohrmann, B., et al. (2017b). Amphibian skin microbiota exhibits temporal variation in community structure but stability of predicted Bd-inhibitory function. ISME J. 11, 1521–1534. doi: 10.1038/ismej.2017.41
Boyle, D. G., Boyle, D. B., Olsen, V., Skerratt, L. F., Meyer, E., McDonald, K. R., et al. (2004). Rapid quantitative detection of amphibians to pathogens during field studies. Dis. Aquat. Org. 92, 175–185. doi: 10.3354/dao02162
Burkart, D., Flechas, S. V., Vredenburg, V. T., and Catenazzi, A. (2017). Cutaneous bacteria, but not peptides, are associated with chytridiomycosis resistance in Peruvian marsupial frogs. Anim. Conserv. 20, 483–491. doi: 10.1111/acv.12352
Campbell, C. R., Voyles, J., Cook, D. I., and Dinudom, A. (2012). Frog skin epithelium: electrolyte transport and chytridiomycosis. Int. J. Biochem. Cell Biol. 44, 431–434. doi: 10.1016/j.biocel.2011.12.002
Caporaso, J. G., Bittinger, K., Bushman, F. D., DeSantis, T. Z., Andersen, G. L., and Knight, R. (2010a). PyNAST: a flexible tool for aligning sequences to a template alignment. Bioinformatics 26, 266–267. doi: 10.1093/bioinformatics/btp636
Caporaso, J. G., Kuczynski, J., Stombaugh, J., Bittinger, K., Bushman, F. D., Costello, E. K., et al. (2010b). QIIME allows analysis of high-throughput community sequencing data. Nat. Methods. 7, 335–336. doi: 10.1038/nmeth.f.303
Caporaso, J. G., Lauber, C. L., Walters, W. A., Berg-Lyons, D., Lozupone, C. A., Turnbaugh, P., et al. (2011). Global patterns of 16S rRNA diversity at a depth of millions of sequences per sample. Proc. Natl. Acad Sci. U.S.A. 108, 4516–4522. doi: 10.1073/pnas.1000080107
Flechas, S. V., Blasco-Zúñiga, A., Merino-Viteri, A., Ramírez-Castañeda, V., Rivera, M., and Amézquita, A. (2017). The effect of captivity on the skin microbial symbionts in three Atelopus species from the lowlands of Colombia and Ecuador. PeerJ 5:e3594. doi: 10.7717/peerj.3594
Flechas, S. V., Sarmiento, C., Cárdenas, M. E., Medina, E. M., Restrepo, S., and Amézquita, A. (2012). Surviving Chytridiomycosis: differential anti-batrachochytrium dendrobatidis activity in bacterial isolates from three lowland species of Atelopus. PLoS One 7:e44832. doi: 10.1371/journal.pone.0044832
Gordon, N. S., Valenzuela, A., Adams, S. M., Ramsey, P. W., Pollock, J. L., Holben, W. E., et al. (2009). Pedobacter nyackensis sp. nov., Pedobacter alluvionis sp. nov.and Pedobacter borealis sp. nov., isolated from montana flood-plain sediment and forest soil. Int. J. Syst. Evol. Microbiol. 59, 1720–1726. doi: 10.1099/ijs.0.000158-0
Harris, R. N., Brucker, R. M., Walke, J. B., Becker, M. H., Schwantes, C. R., Flaherty, D. C., et al. (2009). Skin microbes on frogs prevent morbidity and mortality caused by a lethal skin fungus. ISME J. 3, 818–824. doi: 10.1038/ismej.2009.27
Harris, R. N., James, T. Y., Lauer, A., Simon, M. A., and Patel, A. (2006). The amphibian pathogen Batrachochytrium dendrobatidis is inhibited by the cutaneous bacteria of amphibian species. Ecohealth 3, 53–56. doi: 10.1007/s10393-005-0009-1
Jani, A. J., and Briggs, C. J. (2014). The pathogen Batrachochytrium dendrobatidis disturbs the frog skin microbiome during a natural epidemic and experimental infection. PNAS Plus 111, 5049–5058. doi: 10.1073/pnas.1412752111
Jani, A. J., Knapp, R. A., and Briggs, C. J. (2017). Epidemic and endemic pathogen dynamics correspond to distinct host population microbiomes at a landscape scale. Proc. R. Soc. B 284:20170944. doi: 10.1098/rspb.2017.0944
Kembel, S. W., O’ Connor, T. K., Arnold, H. K., Hubbell, S. P., Wright, S. J., and Green, J. L. (2014). Relationships between phyllosphere bacterial communities and plant functional traits in a neotropical forest. PNAS 111, 13715–13720. doi: 10.1073/pnas.1216057111
Kerby, J. L., Hart, J. A., and Storfer, A. (2011). Combined effects of virus, pesticides, and predator cue on larval tiger salamander (Ambystoma trigrinum). EcoHealth 8, 46–54. doi: 10.1007/s10393-011-0682-1
Kim, M., Kim, W.-S., Tripathi, B. M., and Adams, J. (2014). Distinct bacterial communities dominate tropical and temperate zone leaf litter. Microb. Ecol. 67, 837–848. doi: 10.1007/s00248-014-0380-y
Kueneman, J. G., Wegener, L., Woodhams, D. C., Archer, H. M., Knight, R., and McKenzie, V. J. (2013). The amphibian skin associated microbiome across species, space and life history stages. Mol. Ecol. 23, 1238–1250. doi: 10.1111/mec.12510
Kueneman, J. G., Woodhams, D. C., Van Treuren, W., Archer, H. M., Knight, R., and McKenzie, V. J. (2016). Inhibitory bacteria reduce fungi on early life stages of endangered Colorado boreal toads (Anaxyrus boreas). ISME J. 10, 934–944. doi: 10.1038/ismej.2015.168
Lam, B. A., Walke, J. B., Vredenburg, V. T., and Harris, R. N. (2010). Proportion of individuals with anti-Batrachochytrium dendrobatidis skin bacteria is associated with population persistence in the frog Rana muscosa. Biol. Cons. 143, 529–531. doi: 10.1016/j.biocon.2009.11.015
Lauber, C. L., Strickland, M. S., Bradford, M. A., and Fierer, N. (2008). The influence of soil properties on the structure of bacterial and fungal communities across land-use types. Soil Biol. Biochem. 40:2407e2415. doi: 10.1016/j.soilbio.2008.05.021
Lauer, A., Simon, M. A., Banning, J. L., Lam, B. A., and Harris, R. N. (2008). Diversity of cutaneous bacteria with antifungal activity isolated from female four-toed salamanders. ISME J. 2, 145–157. doi: 10.1038/ismej.2007.110
Lips, K. R. (1998). Decline of a tropical montane amphibian fauna. Conserv. Biol. 12, 106–107. doi: 10.1046/j.1523-1739.1998.96359.x
Lips, K. R. (2016). Overview of chytrid emergence and impacts on amphibians. Phil. Trans. R. Soc. B 371:20150465. doi: 10.1098/rstb.2015.0465
Lips, K. R., Brem, F., Brenes, R., Reeve, J. D., Alford, R. A., Voyles, J., et al. (2006). Emerging infectious disease and the loss of biodiversity in a Neotropical amphibian community. Proc. Natl. Acad. Sci. U.S.A. 103, 3165–3170. doi: 10.1073/pnas.0506889103
Loudon, A. H., Woodhams, D. C., Parfrey, L. W., Archer, H., Knight, R., Valerie McKenzie, V., et al. (2014). Microbial community dynamics and effect of environmental microbial reservoirs on red-backed salamanders (Plethodon cinereus). ISME J. 8, 830–840. doi: 10.1038/ismej.2013.200
Longo, A. V., Savage, A. E., Hewson, I., and Zamudio, K. R. (2015). Seasonal and ontogenetic variation of skin microbial communities and relationships to natural disease dynamics in declining amphibians. R. Soc. Open Sci. 2:140377. doi: 10.1098/rsos.140377
Madison, J. D., Berg, E. A., Abarca, J. G., Whitfield, S. M., Gorbatenko, O., Pinto, A., et al. (2017). Characterization of Batrachochytrium dendrobatidis inhibiting bacteria from amphibian populations in costa rica. Front. Microbiol. 8:290. doi: 10.3389/fmicb.2017.00290
McKenzie, V. J., Bowers, R. M., Fierer, N., Knight, R., and Lauber, C. L. (2012). Co-habiting amphibian species harbor unique skin bacterial communities in wild populations. ISME J. 6, 588–596. doi: 10.1038/ismej.2011.129
McMurdie, P. J., and Holmes, S. (2013). phyloseq: an R package for reproducible interactive analysis and graphics of microbiome census data. PLoS One 8:e61217. doi: 10.1371/journal.pone.0061217
Muletz, C. R., Yarwood, S. A., Campbell Grant, E. H., Fleischer, R. C., and Lips, K. R. (2017). Effects of host species and environment on the skin microbiome of plethodontid salamanders. J. Anim. Ecol. 87, 341–353. doi: 10.1111/1365-2656.12726
Park, S., Collingwood, A., St-Hilaire, S., and Sheridan, P. P. (2014). Inhibition of Batrachochytrium dendrobatidis caused by bacteria isolated from the skin of boreal toads, Anaxyrus (Bufo) boreas, from grand teton national park, Wyoming, USA. Microbiol. Insights 7, 1–8. doi: 10.4137/MBI.S13639
Pounds, J. A., Fogden, M. P. L., Savage, J. M., and Gorman, G. C. (1997). Tests of null models for amphibian declines on a tropical mountain. Cons. Biol. 11, 1307–1322. doi: 10.1046/j.1523-1739.1997.95485.x
Rebollar, E. A., Antwis, R. E., Becker, M. H., Belden, L. K., Bletz, M. C., Brucker, R. M., et al. (2016a). Using “Omics” and integrated Multi-omics approaches to guide probiotic selection to mitigate chytridiomycosis and other emerging Infectious diseases. Front. Microbiol. 7:68. doi: 10.3389/fmicb.2016.00068
Rebollar, E. A., Gutiérrez-Preciado, A., Noecker, C., Eng, A., Hughey, M. C., Medina, D., et al. (2018). The skin microbiome of the neotropical frog Craugastor fitzingeri: inferring potential bacterial-host-pathogen interactions from metagenomic data. Front. Microbiol. 9:466. doi: 10.3389/fmicb.2018.00466
Rebollar, E. A., Hughey, M. C., Medina, D., Harris, R. H., Ibáñez, R., and Belden, L. K. (2016b). Skin bacterial diversity of Panamanian frogs is associated with host susceptibility and presence of Batrachochytrium dendrobatidis. ISME J. 10, 1–14. doi: 10.1038/ismej.2015.234
Sabino-Pinto, J., Bletz, M. C., Islam, M. M., Shimizu, N., Bhuju, S., Geffers, R., et al. (2016). Composition of the Cutaneous bacterial community in japanese amphibians: effects of captivity. Host Species, and Body Region. Microb. Ecol. 72, 460–469. doi: 10.1007/s00248-016-0797-6
Savage, J. M. (2002). The Amphibians and Reptiles of Costa Rica: A Herpetofauna between two Continents, Between Two Seas. Chicago: The University of Chicago Press.
Stuart, S. N., Chanson, J. S., Cox, N. A., Young, B. E., Rodriguez, A. S. L., Fischman, D. L., et al. (2004). Status and trends of amphibian declines and extinctions worldwide. Science 306, 1783–1786. doi: 10.1126/science.1103538
Thomas, F., Hehemann, J. H., Rebuffet, E., Cjzek, M., and Michel, G. (2011). Enviromental and gut Bacteroidetes: the food connection. Front. Microbiol. 2:93. doi: 10.3389/fmicb.2011.00093
Varela, B. J., Lesbarrères, D., Ibáñez, R., and Green, D. M. (2018). Environmental and host effects on skin bacterial community composition in panamanian frogs. Front. Microbiol. 9:298. doi: 10.3389/fmicb.2018.00298
Voyles, J., Young, S., Berger, L., Campbell, C., Voyles, W. F., Dinudom, A., et al. (2009). Pathogenesis of chytridiomycosis, a cause of catastrophic amphibian declines. Science 326:582e585. doi: 10.1126/science.1176765
Walke, J. B., Becker, M. H., Loftus, S. C., House, L. L., Cormier, G., Jensen, R. V., et al. (2014). Amphibian skin may select for rare environmental microbes. ISME J. 8, 2207–2217. doi: 10.1038/ismej.2014.77
Whitfield, S., Kerby, J., Gentry, L. R., and Donnelly, M. A. (2012). Temporal variation in infection prevalence by the amphibian chytrid fungus in three species of frogs at la selva. Costa Rica. Biotropica 44, 779–784. doi: 10.1111/j.1744-7429.2012.00872.x
Whitfield, S. M., Bell, K. E., Philippi, T., Sassa, M., Bolaños, F., Chaves, G., et al. (2007). Amphibian and reptile declines over 35 years at La Selva. Costa Rica. PNAS 104, 8352–8356.
Whittaker, K., Koo, M. S., Wake, D. B., and Vredenburg, V. T. (2013). “Global Declines of Amphibians,” in Encyclopedia of Biodiversity, 2nd edition, Vol. 3, ed. S. A. Levin (Waltham, MA: Academic Press), 691–699. doi: 10.1016/B978-0-12-384719-5.00266-5
Wickham, H. (2007). Reshaping data with the reshape package. J. Stat. Softw. 21, 1–20. doi: 10.3978/j.issn.2305-5839.2016.01.33
Woodhams, D. C., Alford, R. A., Antwis, R. E., Archer, H., Becker, M. H., Belden, L. K., et al. (2015). Antifungal isolates database of amphibian skin-associated bacteria and function against emerging fungal pathogens. Ecology 96, 595. doi: 10.1890/14-1837.1
Keywords: amphibian, microbiota, La Selva Biological Station, bacterial communities, Batrachochytrium dendrobatidis
Citation: Abarca JG, Vargas G, Zuniga I, Whitfield SM, Woodhams DC, Kerby J, McKenzie VJ, Murillo-Cruz C and Pinto-Tomás AA (2018) Assessment of Bacterial Communities Associated With the Skin of Costa Rican Amphibians at La Selva Biological Station. Front. Microbiol. 9:2001. doi: 10.3389/fmicb.2018.02001
Received: 31 October 2017; Accepted: 08 August 2018;
Published: 03 September 2018.
Edited by:
Michael Thomas-Poulsen, University of Copenhagen, DenmarkReviewed by:
Ed Narayan, Western Sydney University, AustraliaMiguel Vences, Technische Universitat Braunschweig, Germany
Copyright © 2018 Abarca, Vargas, Zuniga, Whitfield, Woodhams, Kerby, McKenzie, Murillo-Cruz and Pinto-Tomás. This is an open-access article distributed under the terms of the Creative Commons Attribution License (CC BY). The use, distribution or reproduction in other forums is permitted, provided the original author(s) and the copyright owner(s) are credited and that the original publication in this journal is cited, in accordance with accepted academic practice. No use, distribution or reproduction is permitted which does not comply with these terms.
*Correspondence: Adrián A. Pinto-Tomás, YWRyaWFuLnBpbnRvQHVjci5hYy5jcg==