- 1Department of Biology, Faculty of Science, Mahidol University, Bangkok, Thailand
- 2Department of Biochemistry, Faculty of Science, Mahidol University, Bangkok, Thailand
Antibiotic resistance is a major concern in Clostridium difficile, the causative agent of antibiotic-associated diarrhea. Reduced susceptibility to first- and second-line agents is widespread, therefore various attempts have been made to seek alternative preventive and therapeutic strategies against this pathogen. In this work, the antimicrobial properties of asiatic acid were evaluated against C. difficile. Asiatic acid displayed substantial inhibitory effects on 19 C. difficile isolates collected from different sources with minimal inhibitory concentrations ranging from 10 to 20 μg/ml. Time kill analysis and minimal bactericidal concentration revealed potential bactericidal activity of this compound. Asiatic acid induced membrane damages and alterations in morphological ultrastructure in C. difficile, thereby causing the leakage of intracellular substances. Moreover, asiatic acid also displayed an inhibitory effect on cell motility, but did not interfere with biofilm formation and spore germination. Analysis of drug combination showed no synergistic effect between asiatic acid and vancomycin/metronidazole. Altogether, asiatic acid exhibited strong antimicrobial activity against vegetative cells and could serve as an alternative resource for tackling C. difficile.
Introduction
Clostridium difficile infection (CDI) is considered as a major leading cause of infectious diarrhea among hospitalized patients. Over the past decades, there has been a progressive increase in the prevalence and mortality of CDI cases worldwide (Martin et al., 2016). Although patients with CDI may develop the disease from hospitals, potential sources of CDI in humans may include domestic and farm animals since an overlap between isolates from humans and animals has been demonstrated (Janvilisri et al., 2009). As a consequence of long-term antibiotic use, normal gastrointestinal biota is disrupted, allowing scarce population of C. difficile to overgrow and colonize in the gastrointestinal tract. The pathogenicity of C. difficile depends mostly on the toxins A and B. Both toxins induce the disruption of tight junctions of colonic epithelial cells, causing various symptoms ranging from mild diarrhea to severe pseudomembranous colitis (Voth and Ballard, 2005; Kuehne et al., 2010). Vancomycin and metronidazole are normally prescribed for the patients with CDI according to the clinical guideline, however, 25% of the cases continue to suffer from recurrence. In recent years, significant reduction in the susceptibility of C. difficile against vancomycin and metronidazole has been demonstrated in clinical isolates, causing treatment failure for CDI (Peláez et al., 2002, 2008; Freeman et al., 2010; Tickler et al., 2014). This could potentially be due to the fact that the C. difficile develops defensive mechanisms against these prescribed drugs (Harnvoravongchai et al., 2017; Ngernsombat et al., 2017). Although alternative approaches such as fecal transplantation and phage therapy have been introduced for treatment of CDI, however, limitations due to the immunological concern has been addressed (Bakken et al., 2011; Brandt et al., 2012; Sangster et al., 2014). Hence, novel therapeutic options are still in a critical demand. At present, herbs and natural products have regained tremendous attention to be used as promising alternatives for the treatment of bacterial infection. In fact, various active natural extracts against C. difficile have been reported, however, the identification of bioactive lead compounds and characterization of their mode of action are still lacking (Aljarallah, 2016; Finegold et al., 2018). Asiatic acid (AA) is a pentacyclic triterpenoid derived from a tropical plant Centella asiatica, which has been widely used in traditional remedy in Asia. AA has been shown to exhibit the beneficial effects not only for anti-cancer and neuroprotective activities (Krishnamurthy et al., 2009; Wu et al., 2017), but also displays antimicrobial activity against certain Gram-positive and Gram-negative pathogenic bacteria (Djoukeng et al., 2005; Wong et al., 2011; Liu et al., 2015). However, there is still no report regarding the effect of AA against C. difficile. This study therefore aims to investigate the potential inhibitory effect and the mode of action of AA against C. difficile.
Materials and Methods
Bacterial Strains, Growth Condition and Chemicals
Clostridium difficile strains 630 and R20291 were kindly provided as a gift from Prof. Nigel Minton, University of Nottingham. Human isolates (RA) of C. difficile were previously isolated from diarrheal patients admitted to Ramathibodi Hospital, Thailand during 2010–2011 (Chankhamhaengdecha et al., 2013). The C. difficile NIH isolates were obtained from the National Institute of Health (NIH), Thailand. Food and animal C. difficile isolates were previously obtained (Ojha et al., 2016). All isolates were cultivated in brain heart infusion (BHI) medium at 37°C under anaerobic condition, unless otherwise mentioned. Asiatic acid with the purity of 97% was purchased from Sigma-Aldrich (St. Louis, MO, United States). The structure of AA is shown in Figure 1. The stock AA solution was prepared at 10 mg/ml in dimethyl sulfoxide (DMSO).
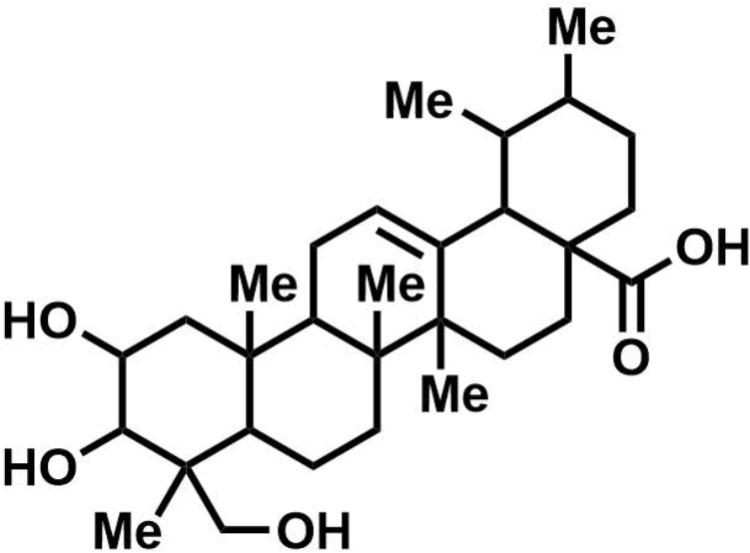
FIGURE 1. Chemical structure of asiatic acid. Figure was created using ChemDraw version 17.0.0.206 (121). Active code: 683F-0185-F588-C2F2.
Determination of Minimal Inhibitory Concentrations and Minimal Bactericidal Concentrations
Minimal inhibitory concentrations (MICs) were determined by broth dilution method. One percent of overnight bacterial culture was transferred into pre-reduced BHI broth, followed by incubation at 37°C for 8–10 h to avoid the cells entering spore stage. Cell density was diluted to the final inoculum of approximately 3 × 105 CFU/ml in 96 well-plate containing antimicrobial agents. Following 48 h of incubation, optical density at 600 nm was measured using a microplate reader (Tecan). To determine the minimal bactericidal concentrations (MBCs), cell suspensions in 96 well-plates used for MIC test were replicated onto BHI agar. Following the incubation at 37°C under anaerobic condition for 48 h, further bacterial growth on the agar was visually observed.
Time-Kill Analysis
The starter was prepared by growing C. difficile strains 630 and R20291 culture overnight. The inoculum was done by transferring one percent of starter culture into BHI broth and incubated for 8-10 h to avoid cells entering spore stage. Time kill assays were carried out with the initial inoculum at approximately 108 CFU/ml. Bacterial growth kinetics was followed against different concentrations of AA. The ratio of the absorbance at 600 nm measured at the time t and time zero was plotted against time.
Fluorescent Microscopic Analysis
Clostridium difficile strain R20291 suspension was prepared as described above. Cell suspension was treated with 20 μg/ml AA for 0, 2, 4 and 6 h. The samples were then washed, mounted on glass slides, and fixed with methanol. To distinguish live and dead bacterial cells, fixed samples were stained with 500 mM propidium iodide (PI), washed and subsequently counterstained with 1 μg/ml Hoechst 33342. Each sample was visualized and analyzed under FV3000 confocal laser scanning microscope (Olympus). To investigate the cell permeability, C. difficile cells were treated with 20 μg/ml AA for 0, 30, 60, and 120 min. The samples then were processed as mentioned previously. After the cells were fixed, each sample was stained with 4′,6-diamidino-2-phenylindole (DAPI), washed and subsequently stained with membrane dye, FM 4-64 (Life technologies). Images were acquired in confocal laser scanning microscope LSM800 with airyscans (Zeiss).
Determination of Protein and Nucleic Acid Leakage
Five ml of C. difficile strain R20291 cells at an optical density of 1.0 were harvested and resuspended in pre-reduced phosphate buffer saline (PBS, pH 7.4). AA was added to a final concentration of 40 μg/ml, the solution was then incubated at 37°C for 6 h under anaerobic condition. Cell debris and pellet were removed by centrifugation, and soluble fraction was retrieved for protein determination using Bradford assay and visualized on SDS-PAGE. For the measurement of nucleic acid leakage, total nucleic acids were purified from the soluble fraction by phenol-chloroform extraction, followed by ethanol precipitation. Quantification of the amount of nucleic acid was monitored by spectrophotometer and further verified by agarose gel electrophoresis.
Scanning Electron Microscopy
The effect of AA on cell morphology and ultrastructure of C. difficile strains 630 and R20291 was observed by scanning electron microscopy (SEM). C. difficile suspension was incubated with AA at 37°C for 6 h, and the suspension was collected and resuspended in PBS pH 7.4. The bacterial cells were fixed with the buffer containing 2.5% glutaraldehyde and 4.0% paraformaldehyde for 4 h, followed by post fixation with a cross-linking reagent, 1.0% osmium tetraoxide, for 1 h. The samples were then dehydrated with a series of ethanol, critical point dried through carbon dioxide, followed by sputter coating with platinum-palladium. The specimens were visualized under Hitachi 2500 scanning electron microscope.
Biofilm Formation and Degradation
One percent of C. difficile strain R20291 suspension (Abs600 of 0.5) was inoculated into 16 × 150 mm screw capped glass culture tube, containing 5 ml BHI supplemented with 0.1 M glucose. The culture was grown for 5 days to allow biofilm development. Different concentrations of AA were added into the bacterial biofilm, followed by further incubation for 24 h. Remained biofilm was measured with crystal violet staining as described previously with slight modifications (Dawson et al., 2012). The samples were washed twice with PBS to remove unbound cells, subsequently stained with 0.2% crystal violet for 30 min, and washed twice with PBS. Absolute methanol was used to extract crystal violet from the biofilm and the solution was monitored at the absorbance of 570 nm.
Evaluation of Bacterial Motility
The effect of AA on swimming motility of C. difficile strain 630 was determined on soft agar. Two microliter of C. difficile culture was stabbed into pre-reduced 0.4% agar BHI medium with different concentrations of AA, followed by the incubation at 37°C for 48 h under anaerobic condition. Swimming motility was quantified by measuring diameter of the spot on soft agar.
Spore Preparation and Germination Kinetics
Overnight culture of C. difficile strains 630 and R20291 was plated onto 70:30 sporulation medium (Fimlaid et al., 2013), followed by the incubation for 3–4 days. Spores were then harvested as previously described (Ojha et al., 2016). Briefly, sporulation induced lawns were collected in distilled water using sterile scarper and washed in distilled water for 5 times. The suspensions were treated with 0.3 mg/ml proteinase K for 1 h, then subjected to heat at 65°C for 1 h to allow efficient inactivation of vegetative cells. Spores were additionally washed with distilled water for 5 times to remove cell debris. Phase contrast microscopy was used to examine the spore purity and spore viability was checked on the agar plate, before being stored at room temperature. To investigate the germination kinetics, germination assay was evaluated as previously described with a few modifications (Bhattacharjee et al., 2015). Spore suspensions were heat-activated at 65°C for 30 min, then subsequently chilled on ice. Activated spores were diluted in BHI supplemented with 0.1% taurocholate. Spore germination was tracked by monitoring the loss of Abs600 at 1 min interval for 1 h. The ratio of the Abs600 at the time t and time zero was plotted against time.
Drug Combination Assay
Bacterial susceptibility to the combination of AA and either vancomycin or metronidazole was tested by checkerboard assay (Magi et al., 2015). Two-fold serial dilutions of AA at the final concentrations ranging from 0.625 to 40 μg/ml were mixed with either vancomycin at a range of 0.25–16 μg/ml or metronidazole at a range of 0.03125–2 μg/ml in 96-well plates. Approximately 3 × 105 CFU/ml of C. difficile strains 630 and R20291 were inoculated, followed by the incubation at 37°C for 48 h under anaerobic condition. To determine the drug combination effect, fractional inhibitory concentration (FIC) index was calculated according to the equation: FIC index = FICA + FICB, where FICA = MICA+B/MICA and FICB = MICB+A/MICB. MICA+B is the MIC of compound A in the combination with compound B and vice versa for MICB+A, whereas MICA or MICB are the MIC of the compound alone. The combination effect is defined as synergy when FIC ≤ 0.5, additive when 0.5 < FIC ≤ 1.0, no interaction when 1.0 < FIC ≤ 4.0, and antagonism when FIC > 4.0 (Páez et al., 2013).
Statistical Analysis
Unless indicated otherwise, all statistical analyses were based on at least 3 independent experiments. The statistical analyses were performed using Student’s t-test with a 95% confidence interval for the sample mean. The p-value less than 0.05 indicates statistically significant difference.
Results
Asiatic Acid Inhibits the Growth of C. difficile
An inhibitory effect of AA on 19 C. difficile strains isolated from different sources was investigated by determining the MIC values. The result of MICs of AA and recommended antibiotics used for CDI treatment including vancomycin and metronidazole were summarized in Table 1. AA exhibited substantial inhibitory effect against C. difficile strains with the MIC value of 10.0 μg/ml, excepted for human isolates 630 and RA156, whose MIC values were 20.0 μg/ml. On the contrary, a broad range of MICs of vancomycin and metronidazole against the tested C. difficile strains was evident. The MICs of vancomycin ranged from 1.0–8.0 μg/ml, while the MICs of metronidazole were from 0.5–16.0 μg/ml. MBC assays were concomitantly performed to demonstrate the bactericidal effect of AA on C. difficile. According to Table 1, MBCs of AA against C. difficile was equal to 10.0 μg/ml, except for the strains 630, R20291 and RA156, which MBC values were shifted up to 20.0 μg/ml.
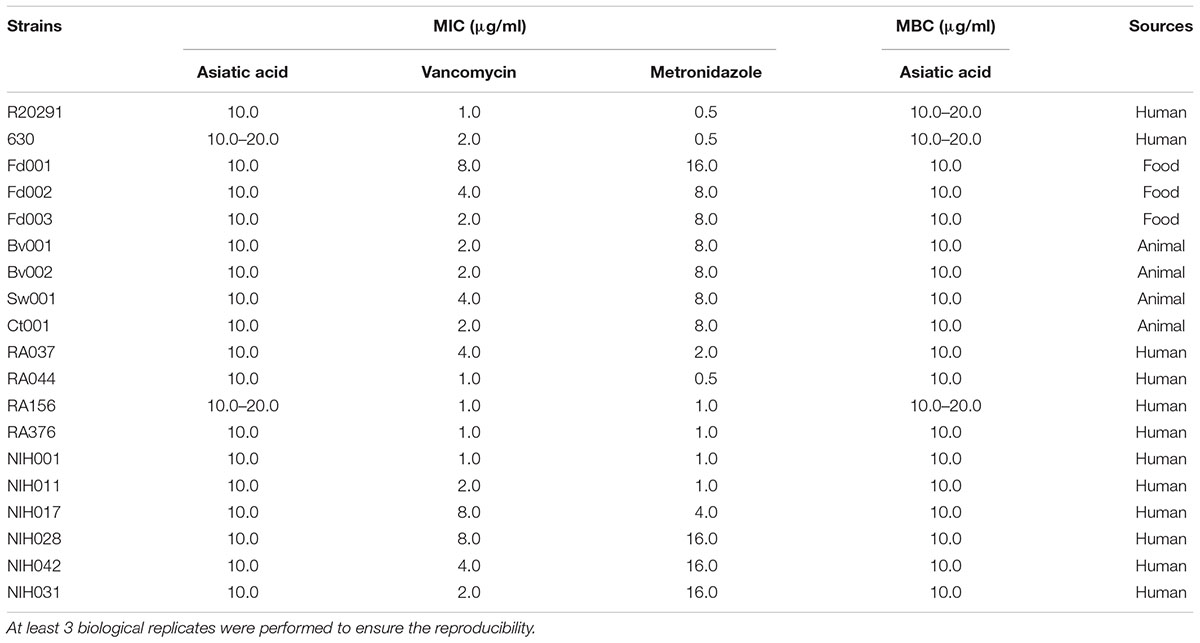
TABLE 1. Antimicrobial activity of asiatic acid, vancomycin, and metronidazole against C. difficile isolated from different sources.
The effect of AA on C. difficile growth was assessed using in vitro time kill experiments. The relative growth as measured by Abs600 at the time t compared to t = 0 was plotted against times. An apparent reduction of bacterial viability was seen as indicated by the decline of the slope (Figure 2). In agreement with MIC/MBC data, the exposure to AA at the concentrations of 10 and 20 μg/ml totally inhibited the growth of both C. difficile strains 630 and R20291. Bactericidal effect of AA was further validated by live and dead cell assays using PI/Hoechst 33342 staining and fluorescent microscopy. PI is a membrane impermeant dye, while Hoechst 33342 exhibits membrane permeability in bacterial cells, therefore only dead cells accumulate and display red intensity. As shown in Figure 3, the levels of red intensity increased in a time-dependent manner, implying the higher population of dead cells over the exposure time. These results were strongly consistent with the bactericidal activity indicated in the time kill curve.
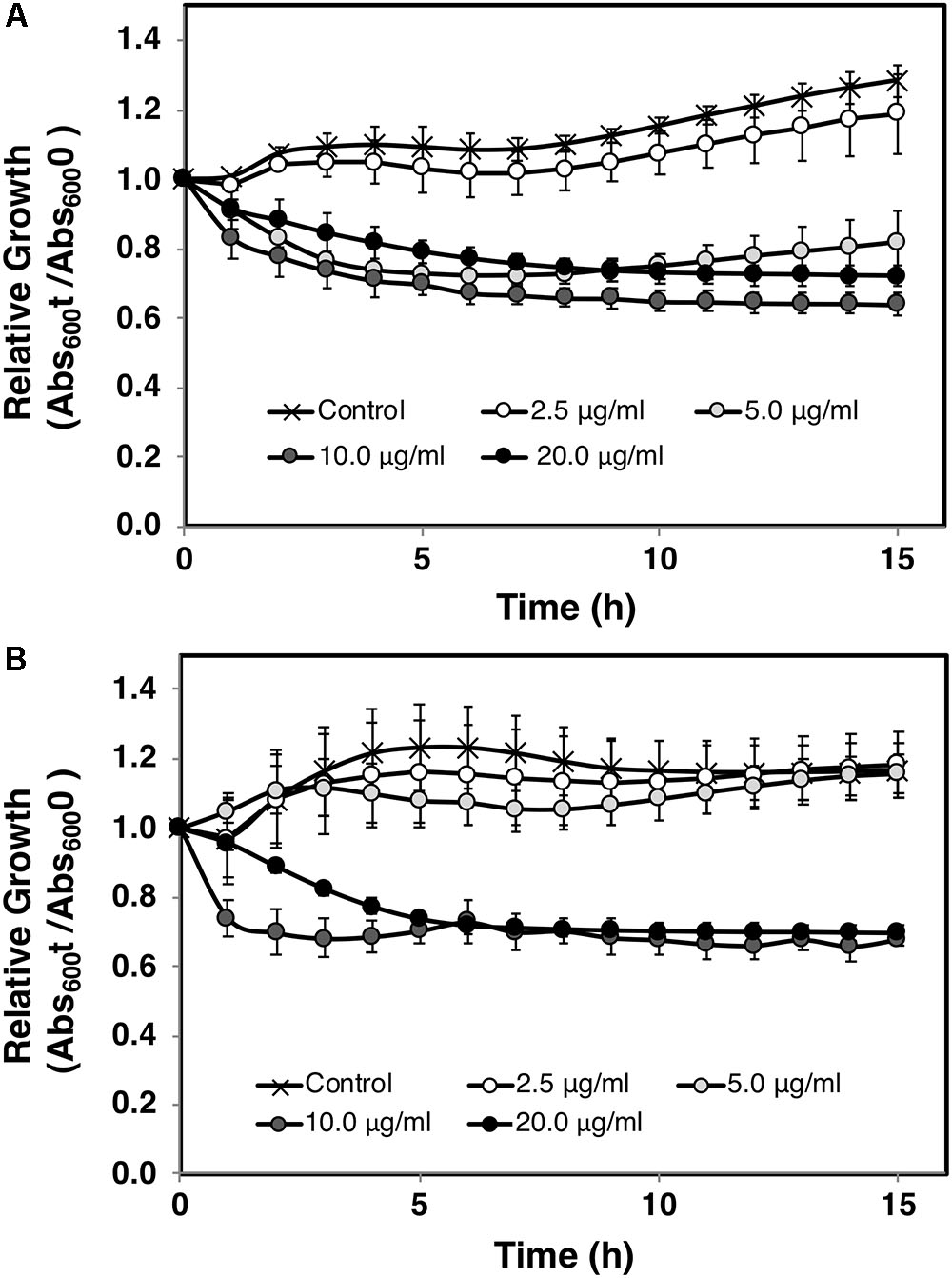
FIGURE 2. Time kill curve of C. difficile treated with asiatic acid. C. difficile strains (A) R20291 and (B) 630 were grown in BHI broth medium with various concentrations of asiatic acid. Growth kinetics was followed for 15 h at 1 h interval. 1% DMSO was added to the control sample. Mean values of at least 3 independent measurements (± standard error) are plotted.
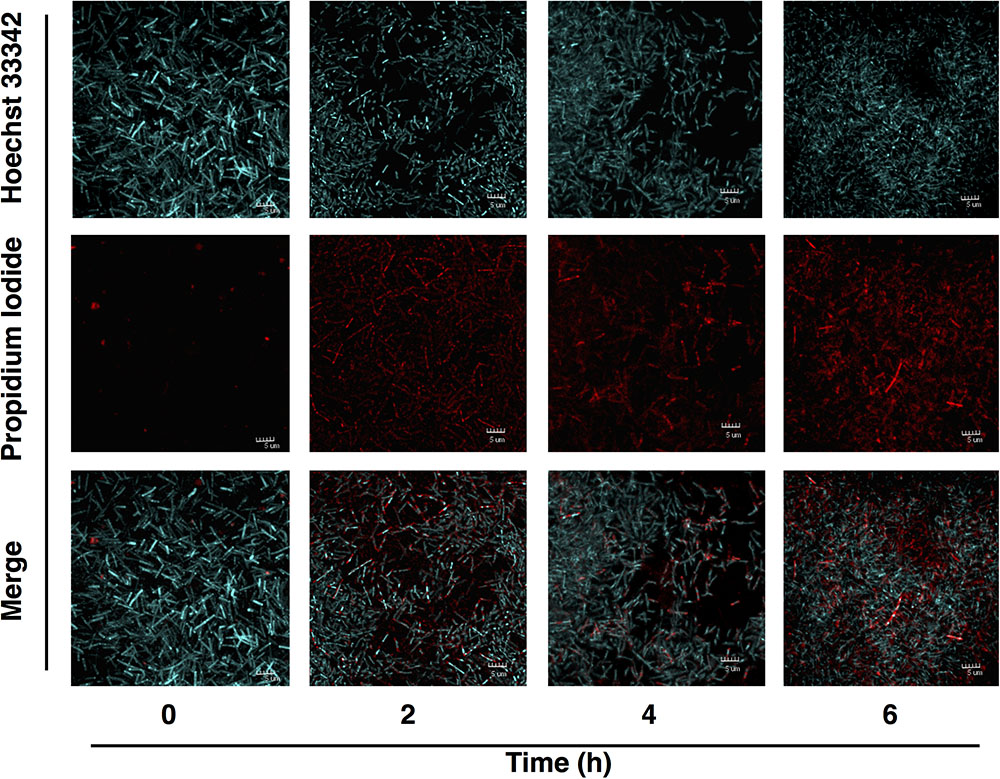
FIGURE 3. Viability of C. difficile following treatment with asiatic acid. Fluorescent images were captured after C. difficile strain R20291 was exposed to AA at 0, 2, 4, and 6 h. Blue-stained bacteria (Hoechst 33342) represented live cell, while red-stained bacteria (propidium iodide) represented dead cell.
Asiatic Acid Disrupts C. difficile Membrane Permeability
Changes in bacterial cellular morphology were observed using FM4-64/DAPI staining and fluorescent microscopy. As shown in Figure 4, leakage of bacterial DNA was observed in C. difficile R20291 following the exposure to AA for 30 min (Figure 4A), and the damages to plasma membranes were observed at the exposure time of 60 and 120 min. In contrast, DNA was tightly packed in the intact cells for untreated control condition (Figure 4B). The leakage of proteins and nucleic acids in the suspension was also evaluated. Up to 2.5-fold of protein leakage was observed in the cell suspension of C. difficile following the AA treatment as shown in Figures 5A,B, and the increase was calculated to be statically significant (p < 0.05). Similarly, the amount of released nucleic acids in the suspension was significantly elevated to ∼3-fold compared to the untreated controls (Figures 5C,D). Alterations in the ultrastructure of C. difficile cells following the exposure to AA were observed using SEM analysis (Figure 6). While the control cells retained their normal rod-shaped structure with smooth and intact surfaces, the exposure to AA caused damages on the bacterial plasma membrane as indicated by the number of craters on the surface and rupture of cells. The intense of damages was correlated well with the concentration of AA.
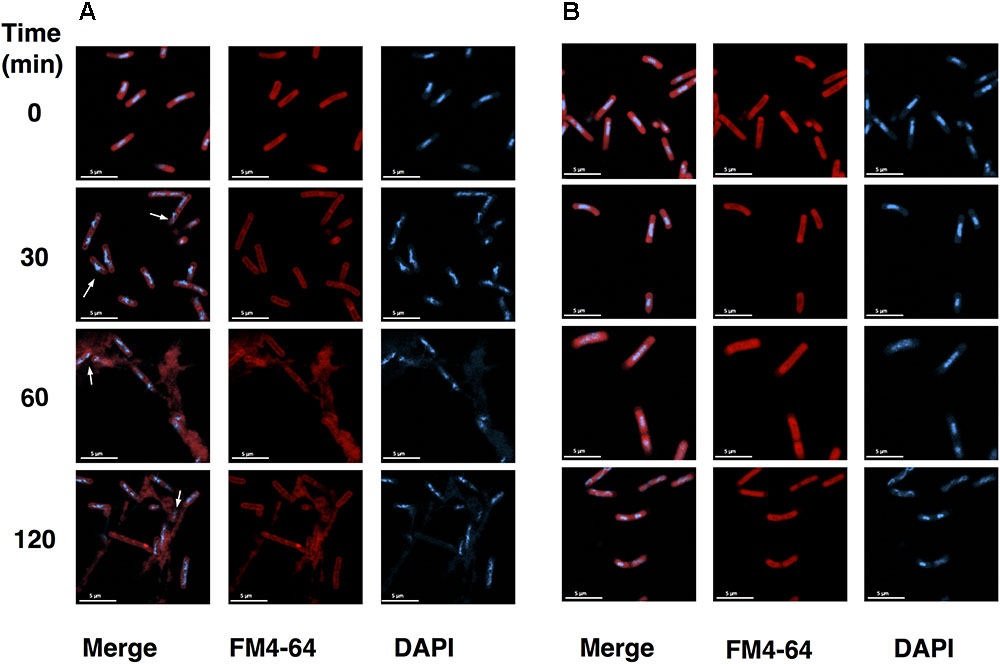
FIGURE 4. C. difficile membrane integrity is compromised in the presence of asiatic acid. Fluorescence microscopic images of (A) C. difficile strain R20291 treated with 20 μg/ml AA at each time interval and (B) untreated samples stained with membrane dye FM4-64 (Red) and DNA dye DAPI (Blue). Red fluorescence indicates membrane disintegration (permeable membrane). Scale bar: 5 μm.
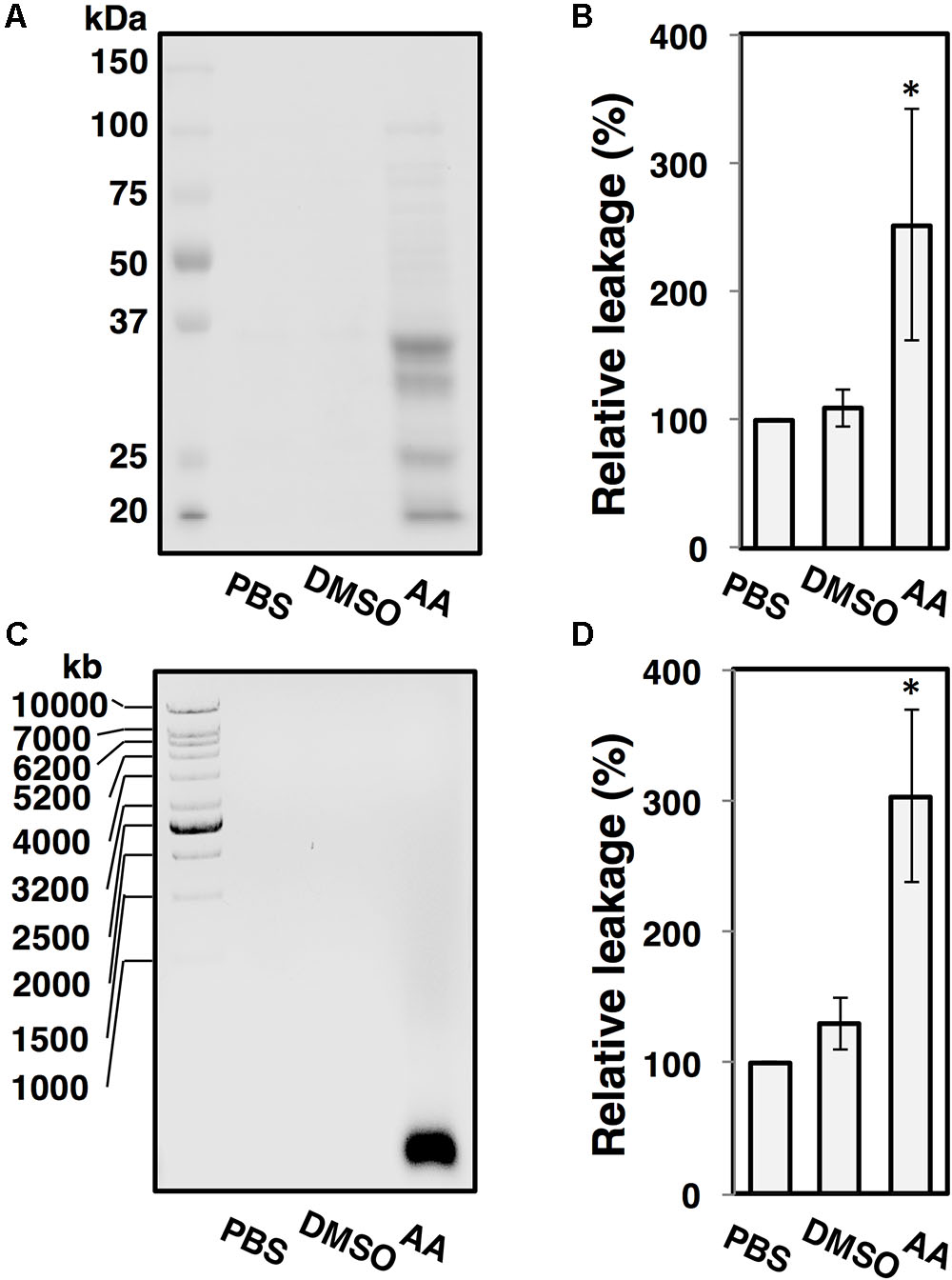
FIGURE 5. Asiatic acid causes bacterial intracellular component leakage. C. difficile strain R20291 grown in BHI medium was anaerobically incubated in PBS buffer, PBS with 1% DMSO and PBS with 40 μg/ml AA. (A) Released proteins from the supernatant fractions were analyzed by SDS-PAGE, (B) Bar graphs represent the relative protein leakage as determined by Bradford assay, (C) Released nucleic acids from the supernatant fractions were analyzed by agarose gel electrophoresis, (D) Bar graphs represent the relative nucleic acid leakage as quantified by UV spectrophotometry. At least 3 independent tests were performed to ensure the reproducibility. The error bars and the asterisks represent the standard errors and the statistical significance with the p-values < 0.05, respectively.
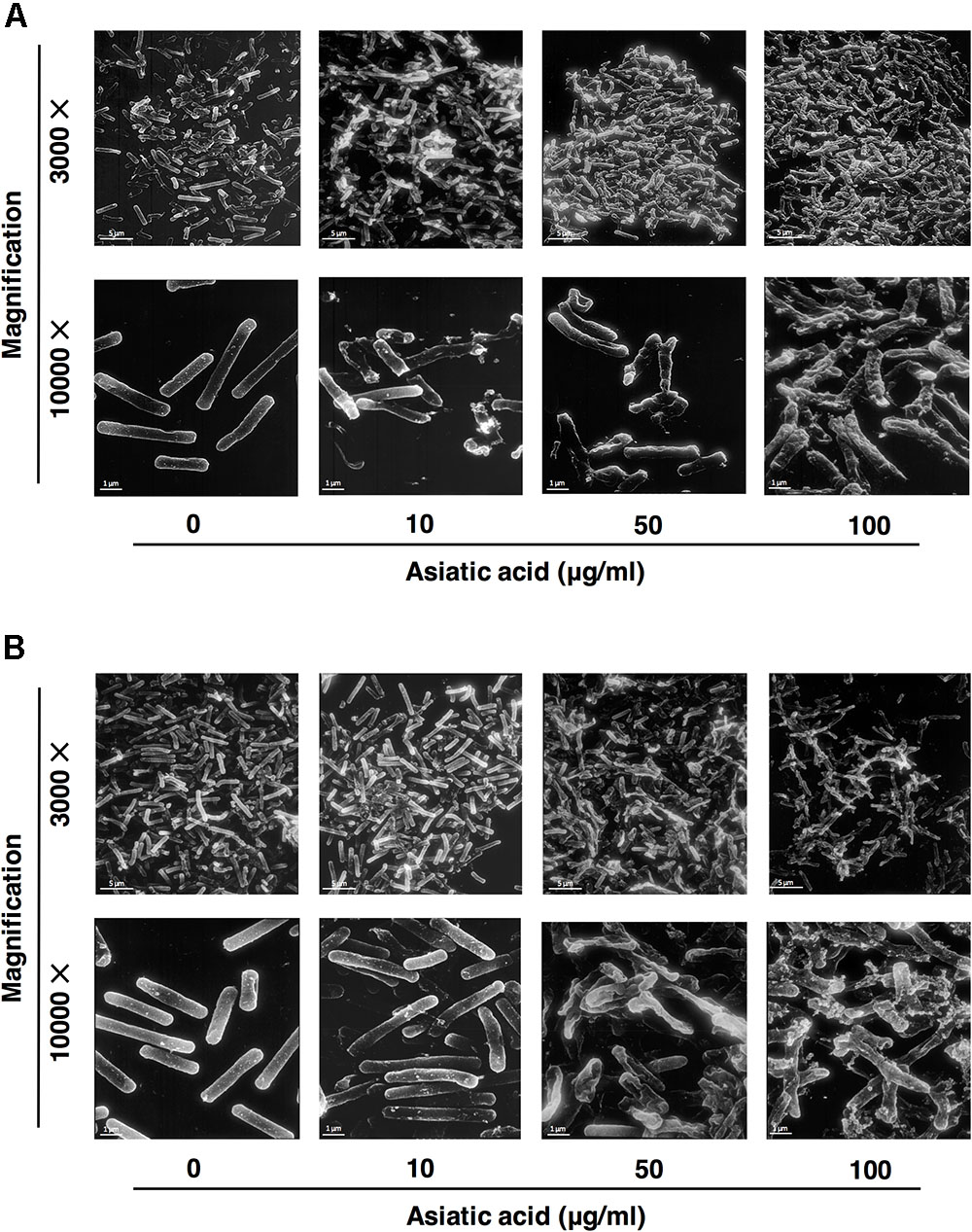
FIGURE 6. Scanning electron microscopic analysis of asiatic acid-treated C. difficile strains (A) R20291 and (B) 630. Bacterial cells were treated with 10, 50, and 100 μg/ml AA, and were then subjected to SEM. Control (untreated) cells displayed intact smooth surfaces as shown in the left panel. Scale bar: 1, 5 μm.
Asiatic Acid Inhibits Cell Motility, but Does Not Interfere Biofilm and Spore Germination
The effects of AA on other virulence factors including biofilm, motility and spore germination were investigated. Ability of C. difficile to adhere and form biofilm causes an increase in bacterial virulence (Dapa and Unnikrishnan, 2013). Biofilm of C. difficile R20291 was grown and subjected to AA. The results revealed that the amount of biofilm was not affected even upon the exposure of high concentration of AA (80 μg/ml) (Figure 7A). For cell motility, the significant reduction in colony diameters of C. difficile 630 was observed when the concentration of AA reached 10 μg/ml (p < 0.05) (Figures 7B,C). It should be noted that no colony formation was seen when the concentration of AA exceeded 10 μg/ml. To determine whether AA could inactivate C. difficile spore, purified spores of C. difficile strains R20291 and 630 were subjected to AA, and germination kinetics was followed by monitoring the changes in Abs600 over a 1 h time period. The relative germination rate was calculated along with the increasing AA concentrations. The results revealed no significant changes in spore germination of both strains at any tested concentrations of AA (Figures 8A–D). Despite no effect of AA on spore germination, ultrastructure of C. difficile R20291 exhibited a few visible damages on the spore surface following the exposure to 10 and 100 μg/ml AA (Figure 8E).
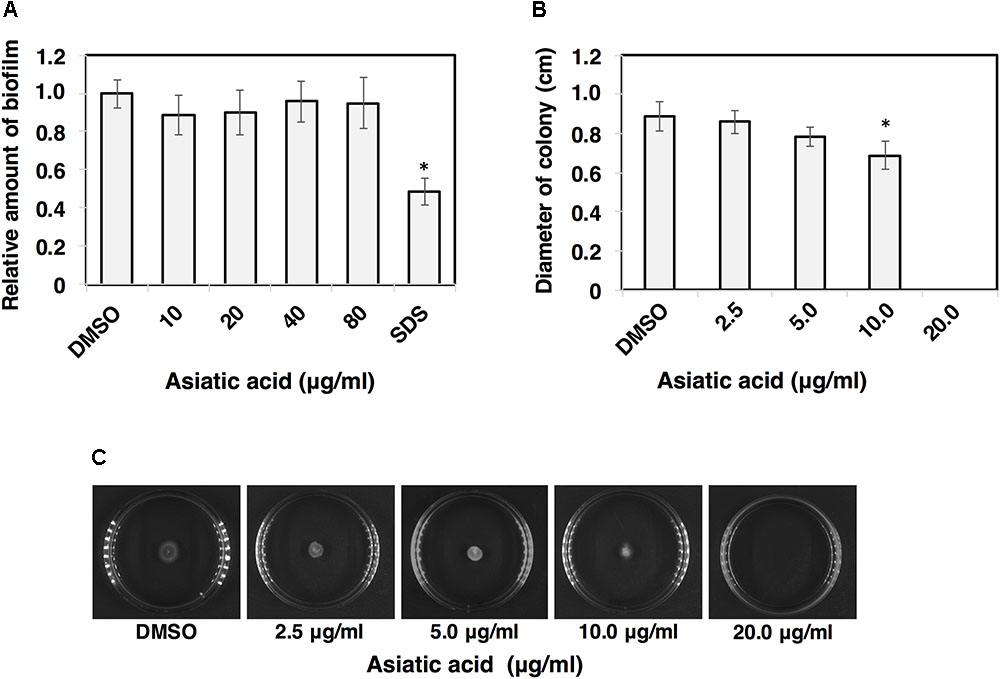
FIGURE 7. Asiatic acid inhibits C. difficile motility, but does not induce biofilm degradation. (A) Effect of AA at different concentrations on biofilm degradation in C. difficile strain R20291. SDS-treated positive control was included. (B) Bar graphs represent migration distance of C. difficile strain 630. (C) Images represent swimming motility of C. difficile strain 630 on BHI soft agar at various concentrations of AA. At least 3 independent experiments were performed. The error bars and the asterisks represent the standard errors and the statistical significance with the p-values < 0.05, respectively.
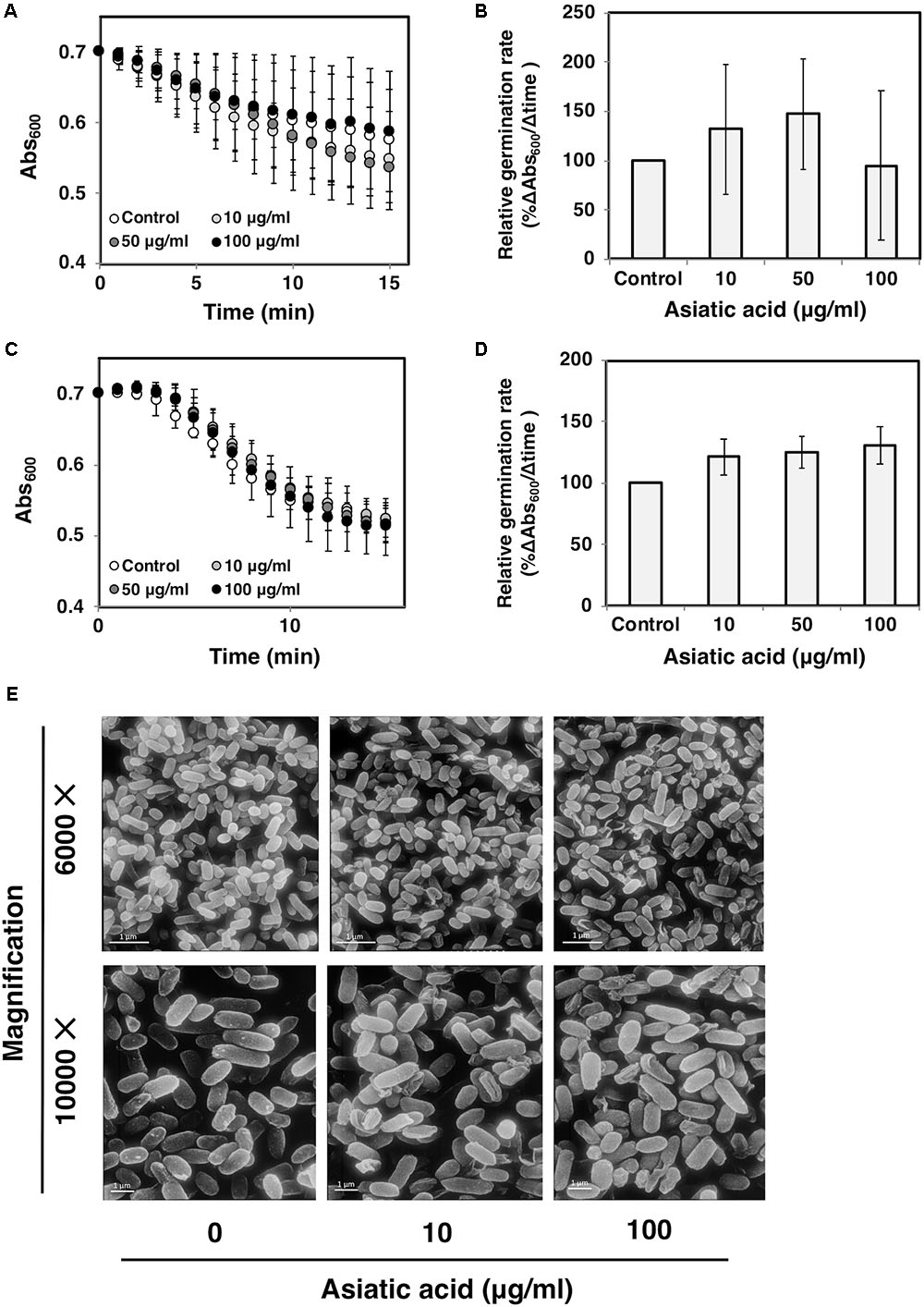
FIGURE 8. Asiatic acid does not inactivate C. difficile spore germination. C. difficile spores from strains (A,B) R20291 and (C,D) 630 were exposed to various concentrations of AA. The germination kinetics was tracked for 15 min at 1 min interval. Bar graphs represent relative germination rate. At least 3 independent tests were performed to ensure the reproducibility. Mean values of at least 3 independent experiments (± standard error) are plotted. (E) Scanning electron micrographs of C. difficile spores from strain R20291 exposed to 10 and 100 μg/ml AA.
Asiatic Acid Exhibits No Synergism With Vancomycin and Metronidazole
The synergistic effect of AA and vancomycin/metronidazole was explored using checkerboard method and the isobolograms were plotted (Supplementary Materials). Based on our results, AA exhibited no synergistic effect with both vancomycin and metronidazole as FIC indices were calculated to fall in a range of 1.0 to 1.5, suggesting that AA acted individually with vancomycin or metronidazole.
Discussion
Prior to the era of antibiotic discovery, folk remedies were widely practiced through the combination of traditional knowledge and implementation of herb medication, health prevention and promotion. Despite the fact that current medication is based on the use of antibiotics, a considerable part of the world population still depends on alternative treatment. In many countries, more than 50% of medical prescriptions rely on traditional medicine, meanwhile 38% of adult patients in the United States preferred herbal medicines as an alternative treatment (Benzie and Wachtel-Galor, 2011). AA, an active constituent mostly present ∼0.5% of dry weight in the leave of Centella asiatica (Rafamantanana et al., 2009), has been proved to possess several pharmacological effects including anti-inflammatory, anti-aging and anti-cancer properties (Huang et al., 2011; Tsao and Yin, 2015; Ren et al., 2016). AA also exhibits an antimicrobial activity against certain pathogenic bacteria (Liu et al., 2015). As the current therapy for C. difficile relies on antibiotic treatment including vancomycin and metronidazole, and the resistance to both drugs is becoming more pronounced, hence we attempted to explore the potential use of AA as an alternative therapeutic against C. difficile.
Susceptibility tests of C. difficile with AA showed consistent MIC value among isolates from different sources (10–20 μg/ml). A lower inhibitory concentration of AA against C. difficile was observed compared to the previously reported MICs of 24–40 μg/ml on other pathogens (Liu et al., 2015). Variations could be resulted from differences in tested bacterial species and experimental conditions. In parallel, MIC values of vancomycin and metronidazole, the antibiotics recommended in the practical guideline for treatment of CDI, were also determined. The MICs of these two antibiotics varied among tested strains. The human isolate NIH028 exhibited the highest MIC against vancomycin and metronidazole (8.0 and 16.0 μg/ml, respectively) implying the possibility of the resistance development during the antibiotic treatment. The resistance to vancomycin and metronidazole in C. difficile strains isolated from the patients in hospitals has been demonstrated (Peláez et al., 2002, 2008; Baines et al., 2008; Spigaglia et al., 2011; Karlowsky et al., 2012). The food isolate Fd001 also exhibited high resistance to both drugs. Because the transmission of C. difficile occurs via fecal-oral route, the emergence of antibiotic resistant isolates from food marks an alarm for critical concerning on the transmission of C. difficile in contaminated diet (Lund and Peck, 2015). Although higher concentration of AA was required to inhibit certain C. difficile isolates, compared to those in vancomycin and metronidazole, however, constant dosage of the compound used for the inhibition allows the determination of precise dose for alternative treatment. MBCs of AA in all tested isolates ranged between 10.0 and 20.0 μg/ml, which do not exceed 4 times of the MICs, indicating the bactericidal activity of the compound. In accordance with the MBC test, time kill kinetics determined in C. difficile strains R20291 and 630 demonstrated a decline in bacterial density as a result of AA exposure. C. difficile strain R20291 exhibited higher sensitivity to AA compared to the strain 630, which is in agreement with those observed in MIC test. It should be noted that the kinetic studies were followed for 16 h, while MIC determinations were performed for 48 h, therefore the dissimilarity of the susceptibility profiles could be clearly demonstrated between each time intervals rather than at one end point. An increase in the population of dead cells following the exposure to AA was evidently time-dependent, which further supports the role of AA as a bactericidal agent. Prior to the drug administration, cytotoxicity of the compounds against host cells is needed to be evaluated (Tharmalingam et al., 2018). IC50 values for AA were reported to be greater than 100 μM (∼50 μg/ml) on human umbilical vein endothelial cells and kidney epithelial Vero cells (Jirasripongpun et al., 2012; Huang et al., 2016), as well as no significant effect of the compound at the same concentration was observed on viability of human gastric mucosa epithelial cells (Jing et al., 2016). Additionally, up to 165 mg/kg of AA was administered to the mice without any signal of side effects (Krishnamurthy et al., 2009). Hence, AA could be a decent therapeutic agent for CDI due to the substantially low MIC against C. difficile compared to the cytotoxicity level.
AA is a terpenoid, an organic compound made up of isoprene units, which is usually found in plant essential oils. It has been demonstrated that terpenoids can cause large-scale membrane thinning on lipid bilayer and could therefore exert their antimicrobial properties via a membrane disruption mechanism (Khandelia et al., 2010). Correspondingly, several studies on terpenoids extracted from plants proposed the properties of the compounds to act on the bacterial plasma membrane, which eventually lead to cell dead (Jasmine et al., 2011; Sathya Bama et al., 2012). To determine the potential mode of action of AA on cell permeability, leakage of intracellular compounds was evaluated. The fluorescence microscopic analyses revealed the rapid action of AA on C. difficile, which caused the leakage of DNA from the bacterial cells within 30 min, while the shape of cell was still retained. Distorted bacterial membrane with no enclosed DNA was later visualized upon the longer incubation period, in accordance with the amount of released proteins and nucleic acids quantified in the supernatant. Additionally, severe damages at the surface including cell rupture and alterations in cellular morphology as demonstrated in SEM micrographs of both 630 and R20291 strains were consistent with the damages previously observed in other bacteria (Hartmann et al., 2010; Goldbeck et al., 2014; Hong et al., 2015). These data suggested that AA potentially acts on cell membrane and causes substantial membrane rupture on C. difficile. As the integrity of bacterial plasma membrane is crucial not only for cell protection but also houses enzymes responsible for cellular processes such as energy production. Thus, bacterial membrane disruption could collapse cellular processes, which in turn affects C. difficile survival and growth.
Ability to motile and adhere involves in the development of disease of C. difficile (Twine et al., 2009; Dingle et al., 2011; Stevenson et al., 2015). Thus, we assessed the inhibitory effect of AA on the motility of C. difficile. The results showed that the motility of C. difficile was significantly reduced following the exposure to AA. Noteworthy, even with a concentration below the inhibitory dose, AA could potentially reduce the infection by impairing colonization and adhesion of C. difficile. Previous reports showed that certain naturally derived compounds including anthocyanins, could inhibit toxin secretion via transcription repression in a gastrointestinal pathogen, Helicobacter pylori, at the sub-inhibitory concentrations (Kim et al., 2012). Further investigations should be addressed for toxin reduction in C. difficile. Following C. difficile colonization, biofilm formation could be initiated. Bacterial biofilm is an aggregated form of bacterial community, in which each individual cell is compacted and connected through the released extracellular polymeric substances. It has been shown that biofilm formation increases the resistance to vancomycin up to 10 folds in C. difficile (Dapa and Unnikrishnan, 2013), reflecting the consequent complication for antibiotic treatment. Although AA exhibited the strong inhibitory effect on C. difficile via the disruption of membrane permeability, degrading activity of the compound was not observed when tested against biofilm. Extracellular polymeric substances are usually composed of different biomolecules such as polysaccharides, proteins, DNA and lipids, which serve as a rigid architecture for bacteria. Hence, AA that is supposed to act on lipid membrane, might not be able to penetrate through the barrier of the complicated extracellular matrix of the biofilm.
Clostridium difficile spores are highly resistant to harsh conditions including heat and toxic chemicals, and play a critical role in the transmission of the disease. Physical and chemical treatments have been reported to inactivate spores and inhibit their germination (Rutala et al., 1993; Perez et al., 2005; Fawley et al., 2007; Francis et al., 2013; Ojha et al., 2016). Here, we also attempted to examine the effect of AA on spore germination. Although AA exhibited an inhibitory effect against C. difficile vegetative cells, however, spore germination did not seem to be significantly influenced by the presence of AA, even at high concentration. On the contrary with the germination data, SEM analysis revealed a detectable spore damage when exposed to 10 μg/ml of AA. It could be explained by the fact that (i) only a few spores were damaged, thus the overall germination rate was not affected, and (ii) spores are encoded by several rigid layers (Paredes-Sabja et al., 2014), therefore the damages at the spore surface may not affect spore germination.
Furthermore, synergism between AA and the first- and second-line agents for CDI including vancomycin and metronidazole was investigated. The FIC values of AA and vancomycin/metronidazole fell into the range of 1.0–1.5, suggesting no synergistic effect between AA and these drugs. This might due to the difference in mode of actions as AA is proposed to disrupt bacterial cell membrane, while vancomycin and metronidazole kill bacteria through the inhibition of cell wall and DNA synthesis, respectively (Huang et al., 2009). Considering no antagonistic effect, CDI patients who have been treated with vancomycin or metronidazole, can be alternatively treated with AA without any contravention.
Conclusion
Asiatic acid exhibited remarkable antimicrobial activity against C. difficile by disrupting permeability of the cell membrane. Nevertheless, the compound was unable to destroy dormant spores or biofilm. Taken into account of the strong inhibition properties of AA and low cytotoxicity level, the compound could be further developed as an alternative treatment to combat CDI.
Author Contributions
PH and TJ conceived and designed the study. PH, SC, and PO performed the experiments. SS and KB helped with the experimental assays. PH and TJ wrote the paper. TJ supervised the project. All authors have read and approved the manuscript.
Funding
This research project was supported by a grant from the government budget to Mahidol University (TJ), and an initiative research grant from Mahidol University (PH).
Conflict of Interest Statement
The authors declare that the research was conducted in the absence of any commercial or financial relationships that could be construed as a potential conflict of interest.
The reviewer PD and handling Editor declared their shared affiliation.
Acknowledgments
We appreciate Methinee Pipatthana and Anupong Bunyacharoenchit for technical assistance.
Supplementary Material
The Supplementary Material for this article can be found online at: https://www.frontiersin.org/articles/10.3389/fmicb.2018.02125/full#supplementary-material
FIGURE S1 | No synergistic effect between asiatic acid and vancomycin/metronidazole. (A) Checkerboard test of C. difficile strains 630 and R20291 on BHI medium supplemented with the combination of AA and either vancomycin or metronidazole. Shading area represents visible growth. (B) Isobolograms of AA plus vancomycin or metronidazole. Dotted lines represent the additive effect of the drug combination (FIC = 1). At least 3 independent tests were performed to ensure the reproducibility.
References
Aljarallah, K. M. (2016). Inhibition of Clostridium difficile by natural herbal extracts. J. Taibah Univ. Med. Sci. 11, 427–431. doi: 10.1016/j.jtumed.2016.05.006
Baines, S. D., O’Connor, R., Freeman, J., Fawley, W. N., Harmanus, C., Mastrantonio, P., et al. (2008). Emergence of reduced susceptibility to metronidazole in Clostridium difficile. J. Antimicrob. Chemother. 62, 1046–1052. doi: 10.1093/jac/dkn313
Bakken, J. S., Borody, T., Brandt, L. J., Brill, J. V., Demarco, D. C., Franzos, M. A., et al. (2011). Treating Clostridium difficile infection with fecal microbiota transplantation. Clin. Gastroenterol. Hepatol. 9, 1044–1049. doi: 10.1016/j.cgh.2011.08.014
Benzie, I. F., and Wachtel-Galor, S. (eds) (2011). Herbal Medicine: Biomolecular and Clinical Aspects. Boca Raton, FL: CRC Press.
Bhattacharjee, D., Francis, M. B., Ding, X., McAllister, K. N., Shrestha, R., and Sorg, J. A. (2015). Re-examining the germination phenotypes of several Clostridium difficile strains suggests another role for the CspC germinant receptor. J. Bacteriol. 198, 777–786. doi: 10.1128/JB.00908-15
Brandt, L. J., Aroniadis, O. C., Mellow, M., Kanatzar, A., Kelly, C., Park, T., et al. (2012). Long-term follow-up of colonoscopic fecal microbiota transplant for recurrent Clostridium difficile infection. Am. J. Gastroenterol. 107, 1079–1087. doi: 10.1038/ajg.2012.60
Chankhamhaengdecha, S., Hadpanus, P., Aroonnual, A., Ngamwongsatit, P., Chotiprasitsakul, D., Chongtrakool, P., et al. (2013). Evaluation of multiplex PCR with enhanced spore germination for detection of Clostridium difficile from stool samples of the hospitalized patients. Biomed. Res. Int. 2013:875437. doi: 10.1155/2013/875437
Dapa, T., and Unnikrishnan, M. (2013). Biofilm formation by Clostridium difficile. Gut Microbes 4, 397–402. doi: 10.4161/gmic.25862
Dawson, L. F., Valiente, E., Faulds-Pain, A., Donahue, E. H., and Wren, B. W. (2012). Characterisation of Clostridium difficile biofilm formation, a role for Spo0A. PLoS One 7:e50527. doi: 10.1371/journal.pone.0050527
Dingle, T. C., Mulvey, G. L., and Armstrong, G. D. (2011). Mutagenic analysis of the Clostridium difficile flagellar proteins, FliC and FliD, and their contribution to virulence in hamsters. Infect. Immun. 79, 4061–4067. doi: 10.1128/IAI.05305-11
Djoukeng, J. D., Abou-Mansour, E., Tabacchi, R., Tapondjou, A. L., Bouda, H., and Lontsi, D. (2005). Antibacterial triterpenes from Syzygium guineense (Myrtaceae). J. Ethnopharmacol. 101, 283–286. doi: 10.1016/j.jep.2005.05.008
Fawley, W. N., Underwood, S., Freeman, J., Baines, S., Saxton, K., Stephenson, K., et al. (2007). Efficacy of hospital cleaning agents and germicides against epidemic Clostridium difficile strains. Infect. Control Hosp. Epidemiol. 28, 920–925. doi: 10.1086/519201
Fimlaid, K. A., Bond, J. P., Schutz, K. C., Putnam, E. E., Leung, J. M., Lawley, T. D., et al. (2013). Global analysis of the sporulation pathway of Clostridium difficile. PLoS Genet. 9:e1003660. doi: 10.1371/journal.pgen.1003660
Finegold, S. M., Summanen, P. H., Corbett, K., Downes, J., Henning, S. M., and Li, Z. (2018). Pomegranate extract exhibits in vitro activity against Clostridium difficile. Nutrition 30, 1210–1212. doi: 10.1016/j.nut.2014.02.029
Francis, M. B., Allen, C. A., and Sorg, J. A. (2013). Muricholic acids inhibit Clostridium difficile spore germination and growth. PLoS One 8:e73653. doi: 10.1371/journal.pone.0073653
Freeman, J., Bauer, M. P., Baines, S. D., Corver, J., Fawley, W. N., Goorhuis, B., et al. (2010). The changing epidemiology of Clostridium difficile infections. Clin. Microbiol. Rev. 23, 529–549. doi: 10.1128/CMR.00082-09
Goldbeck, J. C., Victoria, F. N., Motta, A., Savegnago, L., Jacob, R. G., Perin, G., et al. (2014). Bioactivity and morphological changes of bacterial cells after exposure to 3-(p-chlorophenyl) thio citronellal. LWT Food Sci. Technol. 59, 813–819. doi: 10.1016/j.lwt.2014.05.036
Harnvoravongchai, P., Pipatthana, M., Chankhamhaengdecha, S., and Janvilisri, T. (2017). Insights into drug resistance mechanisms in Clostridium difficile. Essays Biochem. 61, 81–88. doi: 10.1042/EBC20160062
Hartmann, M., Berditsch, M., Hawecker, J., Ardakani, M. F., Gerthsen, D., and Ulrich, A. S. (2010). Damage of the bacterial cell envelope by antimicrobial peptides gramicidin S and PGLa as revealed by transmission and scanning electron microscopy. Antimicrob. Agents Chemother. 54, 3132–3142. doi: 10.1128/AAC.00124-10
Hong, J., Guan, W., Jin, G., Zhao, H., Jiang, X., and Dai, J. (2015). Mechanism of tachyplesin I injury to bacterial membranes and intracellular enzymes, determined by laser confocal scanning microscopy and flow cytometry. Microbiol. Res. 170, 69–77. doi: 10.1016/j.micres.2014.08.012
Huang, G. J., Huang, S. S., Chiu, C. S., Chen, H. J., Hou, W. C., Sheu, M. J., et al. (2011). Antinociceptive activities and the mechanisms of anti-inflammation of asiatic acid in mice. Evid. Based Complement. Altern. Med. 2011:895857. doi: 10.1155/2011/895857
Huang, H., Weintraub, A., Fang, H., and Nord, C. E. (2009). Antimicrobial resistance in Clostridium difficile. Int. J. Antimicrob. Agents 34, 516–522. doi: 10.1016/j.ijantimicag.2009.09.012
Huang, R. Z., Wang, C. Y., Li, J. F., Yao, G. Y., Pan, Y. M., Ye, M. Y., et al. (2016). Synthesis, antiproliferative and apoptosis-inducing effects of novel asiatic acid derivatives containing α-aminophosphonates. RSC Adv. 6, 62890–62906. doi: 10.1039/C6RA11397D
Janvilisri, T., Scaria, J., Thompson, A. D., Nicholson, A., Limbago, B. M., Arroyo, L. G., et al. (2009). Microarray identification of Clostridium difficile core components and divergent regions associated with host origin. J. Bacteriol. 191, 3881–3891. doi: 10.1128/JB.00222-09
Jasmine, R., Selvakumar, B. N., and Daisy, P. (2011). Investigating the mechanism of action of terpenoids and the effect of interfering substances on an Indian medicinal plant extract demonstrating antibacterial. Int. J. Pharm. Stud. Res. 2, 19–24.
Jing, Y., Wang, G., Ge, Y., Xu, M., Tang, S., and Gong, Z. (2016). AA-PMe, a novel asiatic acid derivative, induces apoptosis and suppresses proliferation, migration, and invasion of gastric cancer cells. Onco. Targets Ther. 9, 1605–1621. doi: 10.2147/OTT.S98849
Jirasripongpun, K., Jirakanjanakit, N., Sakulsom, P., Wongekalak, L. O., and Hongsprabhas, P. (2012). Influences of food matrices on cytotoxicity of asiatic acid in mammalian cell models. Int. J. Food Sci. Technol. 47, 1970–1976. doi: 10.1111/j.1365-2621.2012.03058.x
Karlowsky, J. A., Zhanel, G. G., Hammond, G. W., Rubinstein, E., Wylie, J., Du, T., et al. (2012). Multidrug-resistant North American pulsotype 2 Clostridium difficile was the predominant toxigenic hospital-acquired strain in the province of Manitoba, Canada, in 2006-2007. J. Med. Microbiol. 61(Pt 5), 693–700. doi: 10.1099/jmm.0.041053-0
Khandelia, H., Witzke, S., and Mouritsen, O. G. (2010). Interaction of salicylate and a terpenoid plant extract with model membranes: reconciling experiments and simulations. Biophys. J. 99, 3887–3894. doi: 10.1016/j.bpj.2010.11.009
Kim, S.-H., Park, M., Woo, H., Tharmalingam, N., Lee, G., Rhee, K.-J., et al. (2012). Inhibitory effects of anthocyanins on secretion of Helicobacter pylori CagA and VacA toxins. Int. J. Med. Sci. 9, 838–842. doi: 10.7150/ijms.5094
Krishnamurthy, R. G., Senut, M.-C., Zemke, D., Min, J., Frenkel, M. B., Greenberg, E. J., et al. (2009). Asiatic acid, a pentacyclic triterpene from Centella asiatica, is neuroprotective in a mouse model of focal cerebral ischemia. J. Neurosci. Res. 87, 2541–2550. doi: 10.1002/jnr.22071
Kuehne, S. A., Cartman, S. T., Heap, J. T., Kelly, M. L., Cockayne, A., and Minton, N. P. (2010). The role of toxin A and toxin B in Clostridium difficile infection. Nature 467, 711–713. doi: 10.1038/nature09397
Liu, W. H., Liu, T. C., and Mong, M. C. (2015). Antibacterial effects and action modes of asiatic acid. Biomedicine 5:16. doi: 10.7603/s40681-015-0016-7
Lund, B. M., and Peck, M. W. (2015). A possible route for foodborne transmission of Clostridium difficile? Foodborne Pathog. Dis. 12, 177–182. doi: 10.1089/fpd.2014.1842
Magi, G., Marini, E., and Facinelli, B. (2015). Antimicrobial activity of essential oils and carvacrol, and synergy of carvacrol and erythromycin, against clinical, erythromycin-resistant Group A Streptococci. Front. Microbiol. 6:165. doi: 10.3389/fmicb.2015.00165
Martin, J. S., Monaghan, T. M., and Wilcox, M. H. (2016). Clostridium difficile infection: epidemiology, diagnosis and understanding transmission. Nat. Rev. Gastroenterol. Hepatol. 13, 206–216. doi: 10.1038/nrgastro.2016.25
Ngernsombat, C., Sreesai, S., Harnvoravongchai, P., Chankhamhaengdecha, S., and Janvilisri, T. (2017). CD2068 potentially mediates multidrug efflux in Clostridium difficile. Sci. Rep. 7:9982. doi: 10.1038/s41598-017-10155-x
Ojha, S. C., Chankhamhaengdecha, S., Singhakaew, S., Ounjai, P., and Janvilisri, T. (2016). Inactivation of Clostridium difficile spores by microwave irradiation. Anaerobe 38, 14–20. doi: 10.1016/j.anaerobe.2015.10.015
Páez, P. L., Bazán, C. M., Bongiovanni, M. E., Toneatto, J., Albesa, I., Becerra, M. C., et al. (2013). Oxidative stress and antimicrobial activity of chromium(iii) and ruthenium(II) complexes on Staphylococcus aureus and Escherichia coli. Biomed. Res. Int. 2013:906912. doi: 10.1155/2013/906912
Paredes-Sabja, D., Shen, A., and Sorg, J. A. (2014). Clostridium difficile spore biology: sporulation, germination, and spore structural proteins. Trends Microbiol. 22, 406–416. doi: 10.1016/j.tim.2014.04.003
Peláez, T., Alcalá, L., Alonso, R., Rodríguez-Créixems, M., García-Lechuz, J. M., and Bouza, E. (2002). Reassessment of Clostridium difficile susceptibility to metronidazole and vancomycin. Antimicrob. Agents Chemother. 46, 1647–1650. doi: 10.1128/AAC.46.6.1647-1650.2002
Peláez, T., Cercenado, E., Alcalá, L., Marín, M., Martín-López, A., Martínez-Alarcón, J., et al. (2008). Metronidazole resistance in Clostridium difficile is heterogeneous. J. Clin. Microbiol. 46, 3028–3032. doi: 10.1128/JCM.00524-08
Perez, J., Springthorpe, V. S., and Sattar, S. A. (2005). Activity of selected oxidizing microbicides against the spores of Clostridium difficile: relevance to environmental control. Am. J. Infect. Control 33, 320–325. doi: 10.1016/j.ajic.2005.04.240
Rafamantanana, M. H., Rozet, E., Raoelison, G. E., Cheuk, K., Ratsimamanga, S. U., Hubert, P., et al. (2009). An improved HPLC-UV method for the simultaneous quantification of triterpenic glycosides and aglycones in leaves of Centella asiatica (L.) Urb (APIACEAE). J. Chromatogr. B 877, 2396–2402. doi: 10.1016/j.jchromb.2009.03.018
Ren, L., Cao, Q. X., Zhai, F. R., Yang, S. Q., and Zhang, H. X. (2016). Asiatic acid exerts anticancer potential in human ovarian cancer cells via suppression of PI3K/Akt/mTOR signalling. Pharm. Biol. 54, 2377–2382. doi: 10.3109/13880209.2016.1156709
Rutala, W. A., Gergen, M. F., and Weber, D. J. (1993). Inactivation of Clostridium difficile spores by disinfectants. Infect. Control Hosp. Epidemiol. 14, 36–39.
Sangster, W., Hegarty, J. P., and Stewart, D. B. (2014). Phage therapy for Clostridium difficile infection: an alternative to antibiotics? Semin. Colon Rectal Surg. 25, 167–170. doi: 10.1053/j.scrs.2014.05.014
Sathya Bama, S., Jayasurya Kingsley, S., Sankaranarayanan, S., and Bama, P. (2012). Antibacterial activity of different phytochemical extracts from the leaves of T. procumbens Linn.: identification and mode of action of the terpenoid compound as antibacterial. Ind. J. Pharm. Pharm. Sci. 4, 557–564.
Spigaglia, P., Barbanti, F., Mastrantonio, P., Ackermann, G., Balmelli, C., Barbut, F., et al. (2011). Multidrug resistance in European Clostridium difficile clinical isolates. J. Antimicrob. Chemother. 66, 2227–2234. doi: 10.1093/jac/dkr292
Stevenson, E., Minton, N. P., and Kuehne, S. A. (2015). The role of flagella in Clostridium difficile pathogenicity. Trends Microbiol. 23, 275–282. doi: 10.1016/j.tim.2015.01.004
Tharmalingam, N., Port, J., Castillo, D., and Mylonakis, E. (2018). Repurposing the anthelmintic drug niclosamide to combat Helicobacter pylori. Sci. Rep. 8:3701. doi: 10.1038/s41598-018-22037-x
Tickler, I. A., Goering, R. V., Whitmore, J. D., Lynn, A. N. W., Persing, D. H., and Tenover, F. C. (2014). Strain types and antimicrobial resistance patterns of Clostridium difficile isolates from the United States, 2011 to 2013. Antimicrob. Agents Chemother. 58, 4214–4218. doi: 10.1128/AAC.02775-13
Tsao, S., and Yin, M. (2015). Antioxidative and antiinflammatory activities of asiatic acid, glycyrrhizic acid, and oleanolic acid in human bronchial epithelial cells. J. Agric. Food Chem. 63, 3196–3204. doi: 10.1021/acs.jafc.5b00102
Twine, S. M., Reid, C. W., Aubry, A., McMullin, D. R., Fulton, K. M., Austin, J., et al. (2009). Motility and flagellar glycosylation in Clostridium difficile. J. Bacteriol. 191, 7050–7062. doi: 10.1128/JB.00861-09
Voth, D. E., and Ballard, J. D. (2005). Clostridium difficile toxins: mechanism of action and role in disease. Clin. Microbiol. Rev. 18, 247–263. doi: 10.1128/CMR.18.2.247-263.2005
Wong, K. C., Ali, D., and Boey, P. L. (2011). Chemical constituents and antibacterial activity of Melastoma malabathricum L. Nat. Prod. Res. 26, 609–618. doi: 10.1080/14786419.2010.538395
Keywords: Clostridium difficile, asiatic acid, antimicrobials, drug resistance, herb
Citation: Harnvoravongchai P, Chankhamhaengdecha S, Ounjai P, Singhakaew S, Boonthaworn K and Janvilisri T (2018) Antimicrobial Effect of Asiatic Acid Against Clostridium difficile Is Associated With Disruption of Membrane Permeability. Front. Microbiol. 9:2125. doi: 10.3389/fmicb.2018.02125
Received: 01 March 2018; Accepted: 20 August 2018;
Published: 07 September 2018.
Edited by:
Henrietta Venter, University of South Australia, AustraliaReviewed by:
Nagendran Tharmalingam, Alpert Medical School, United StatesPermal Deo, University of South Australia, Australia
Copyright © 2018 Harnvoravongchai, Chankhamhaengdecha, Ounjai, Singhakaew, Boonthaworn and Janvilisri. This is an open-access article distributed under the terms of the Creative Commons Attribution License (CC BY). The use, distribution or reproduction in other forums is permitted, provided the original author(s) and the copyright owner(s) are credited and that the original publication in this journal is cited, in accordance with accepted academic practice. No use, distribution or reproduction is permitted which does not comply with these terms.
*Correspondence: Tavan Janvilisri, dGF2YW4uamFuQG1haGlkb2wuYWMudGg=