- 1Key Laboratory of Freshwater Biodiversity Conservation, Ministry of Agriculture, Yangtze River Fisheries Research Institute, Chinese Academy of Fishery Sciences, Wuhan, China
- 2College of Fisheries, Huazhong Agricultural University, Wuhan, China
- 3College of Marine Sciences, Shanghai Ocean University, Shanghai, China
Ammonia-oxidizing bacteria (AOB) and archaea (AOA) play important roles in nitrogen removal in aquaculture ponds, but their distribution and the environmental factors that drive their distribution are largely unknown. In this study, we collected surface sediment samples from Ctenopharyngodon idellus ponds in three different areas in China that practice aquaculture. The community structure of AOB and AOA and physicochemical characteristics in the ponds were investigated. The results showed that AOA were more abundant than AOB in all sampling ponds except one, but sediment AOB and AOA numbers varied greatly between ponds. Correlation analyses indicated a significant correlation between the abundance of AOB and arylsulfatase, as well as the abundance of AOA and total nitrogen (TN) and arylsulfatase. In addition, AOB/AOA ratio was found to be significantly correlated with the microbial biomass carbon. AOB were grouped into seven clusters affiliated to Nitrosospira and Nitrosomonas, and AOA were grouped into six clusters affiliated to Nitrososphaera, Nitrososphaera sister group, and Nitrosopumilus. AOB/AOA diversity in the surface sediments of aquaculture ponds varied according to the levels of total organic carbon (TOC), and AOB and AOA diversity was significantly correlated with arylsulfatase and β-glucosidase, respectively. The compositions of the AOB communities were also found to be significantly influenced by sediment eutrophic status (TOC and TN levels), and pH. In addition, concentrations of acid phosphatase and arylsulfatase in surface sediments were significantly correlated with the prominent bacterial amoA genotypes, and concentrations of TOC and urease were found to be significantly correlated with the prominent archaeal amoA genotype compositions. Taken together, our results indicated that AOB and AOA communities in the surface sediments of Ctenopharyngodon idellus aquaculture ponds are regulated by organic matter and its availability to the microorganisms.
Introduction
Globally, aquaculture has been continuously growing in the past 50 years to around 73.8 million tons (not including aquatic plants) in 2014 (FAO, 2016). China has played a major role in its growth and represents more than 60 percent of the global aquaculture production (FAO, 2016). According to the Bureau of Fisheries, 31.79 million tons of freshwater aquaculture products were generated in China in 2016, with an estimated value of US$91.51 billion (Bureau of Fisheries, 2017). However, along with these remarkable achievements, concerns have been raised about the negative environmental effects of increasing productivity which is largely based on high-density pond cultivation. Fertilizers and supplementary feedstuffs are largely used in intensive aquaculture to achieve higher productivity, resulting in the accumulation of nitrogen in pond sediments owing to both the excrements of the species cultivated and excessive residual matter (Avnimelech and Ritvo, 2003). Excessive nitrogen in sediments not only spoils the aquaculture environment and leads to the death of the cultivated species (Camargo and Alonso, 2006), but also affects the surrounding water bodies when discharged (Cao et al., 2007). Thus, the removal of excess nitrogen from the pond systems of high-density aquaculture is of great importance.
Nitrification is considered an essential process during nitrogen loss in ecosystems (Wu et al., 2017). This process consist of two steps, where ammonia is first oxidized to nitrite and subsequently to nitrate, which are thought to be implemented either by separate groups of microorganisms (Vlaeminck et al., 2011) or by a single group of microorganisms, the latter only having been discovered in recent years (known as “comammox”; van Kessel et al., 2015). Microbial ammonia oxidation is the first and rate-limiting step of the two-step nitrification, and has been intensively investigated. Ammonia oxidation is known to be mediated by both ammonia-oxidizing bacteria (AOB) and archaea (AOA) (Venter et al., 2004; Treusch et al., 2005). Terrestrial AOB are classified into two genera Nitrosospira and Nitrosomonas based on the gene sequences of 16S rRNA (Head et al., 1993; Teske et al., 1994) and ammonia monooxygenase (amoA) (Rotthauwe et al., 1997; Purkhold et al., 2000). AOA were only identified in recent years but were found to be ubiquitous. AOA consist of Nitrosopumilus cluster (group I.1a), Nitrosotalea cluster (group I.1a-associated), Nitrososphaera cluster (group I.1b), Nitrososphaera sister cluster, and Nitrosocaldus cluster (ThAOA) (Pester et al., 2012). AOB and AOA have been found to be common in various environments, including estuaries (Beman and Francis, 2006; Sahan and Muyzer, 2008), soils (Leininger et al., 2006), rivers (Sun et al., 2013), lakes (Liu et al., 2015), reservoirs (Wang X. et al., 2014), and wetlands (Yang et al., 2014). The distribution of AOB and AOA varied according to the different habitats being studied, and responded to different environmental factors. For instance, pH, salinity, and nutrients could affect AOB community composition (Li et al., 2015), while water depth, redox variation, and concentration of ammonium drive AOA distribution (Flood et al., 2015). Although their wide distribution and high abundance have been reported (Lu et al., 2016), our current understanding of the distribution characteristics of AOB and AOA in aquaculture ponds is still very limited.
Aquaculture ponds are unique anthropogenic aquatic ecosystems characterized by regular nutrient input. Incomplete assimilation by cultivated species cause continuous accumulation of nutrients in pond sediments, mainly in the form of organic matter (Jimenez-Montealegre et al., 2005). With the decomposition of organic matter, oxygen is depleted and large quantities of ammonia accumulate in the sediment layer (Hargreaves, 1998). The overnutrition and the highly reduced environment in aquaculture ponds can cause significant effect on the nitrification process and may tend to favor specific microbial communities. Although the diversity and abundance of pond sediment AOB and AOA have been characterized in previous studies (Lu et al., 2015, 2016; Zhou et al., 2017), these studies were conducted in single pond systems, and the distribution characteristics of AOB and AOA in aquaculture pond sediments, as well as the driving factors of this distribution, are still unclear.
In this study, we collected surface sediment samples from Ctenopharyngodon idellus aquaculture ponds located in three different areas: Heilongjiang, Changjiang, and Zhujiang; these are considered to be the leading freshwater aquaculture-producing areas in North and South China (Wang et al., 2015). By comparing the AOB and AOA communities and the environmental factors between the different sampling areas, as well as correspondingly analyzing the microorganisms and the factors, the present study aims to characterize the distribution of AOB and AOA in aquaculture pond sediments, and determine possible driving environmental factors.
Materials and Methods
Site Description and Sample Collection
Water and sediment samples were collected from Ctenopharyngodon idellus aquaculture ponds located in three different areas in China: Changjiang (30°18′34′′-30°18′45′′N, 112°03′53′′-112°04′17′′E), Heilongjiang (46°23′32′′-46°23′50′′N, 126°44′25′′-126°44′42′′E), and Zhujiang (22°36′33′′-22°36′37′′N, 113°30′49′′-113°30′58′′E) aquaculture areas. Three ponds were sampled in each area. The sampling ponds had a surface area of ∼7000–27000 m2 and a depth of ∼1.5–3.5 m. In the sampled ponds, Ctenopharyngodon idellus intercropped with Hypophthalmichthys molitrix, and Hypophthalmichthys nobilis were raised for commercial use. Detailed information of Ctenopharyngodon idellus culturing is listed in Supplementary Table S1.
Surface sediment samples (0–5 cm) were collected in triplicate from the aquaculture ponds between May and June 2017 using a core sampler. In each pond, the sediments were mixed and placed in sterile plastic bags, then sealed and transported to the laboratory on ice. One portion was used for the determination of ammonia oxidation activities immediately after arrival, and another portion was used for the analysis of physicochemical characteristics. Subsamples were stored at -80°C for subsequent DNA extractions and molecular analysis. Water samples were also collected for characterization. Water temperature, dissolved oxygen (DO), and pH were recorded in situ using a handheld smarTROLL Multiparameter (In-Situ Inc., United States).
Physicochemical Analysis
For the water samples, ammonium (NH4+), nitrite (NO2-), nitrate (NO3-), total nitrogen (TN), and total phosphorus (TP) were determined according to standard protocols (China Environmental Protection Agency, 2002). Sediment pH was determined by mixing the sample thoroughly with water in a ratio of 1:2.5 (m/v). NH4+, NO2- and NO3- were extracted from the sediment with 1 mol/L KCl solution and measured by spectrophotometric methods (Lu et al., 2016). The TN and TP of the sediment were measured using the semi-micro Kjeldahl method and the alkali fusion - Mo-Sb anti spectrophotometric method, respectively. Sediment organic carbon (TOC) content was determined by using the potassium dichromate oxidation spectrophotometric method (Song et al., 2012). The concentrations of urea were measured according to the method of Therkildsen et al. (1996), with small modifications. Briefly, urea was extracted from ∼35 g of sediment with 15 ml of 1M KCl. The slurry was centrifuged to terminate the extraction after 10 min. Urea concentrations in the supernatant were then measured using the diacetylmonoxime method (Price and Harrison, 1987). The concentrations of water- and acid-soluble sulfate in the sediment were determined by the gravimetric method (American Society for Testing and Materials, 1973).
Microbial Biomass and Soluble Carbon
Microbial biomass carbon (MBC) in the sediment was determined using the fumigation-extraction method (Vance et al., 1987). Briefly, fresh sediments were incubated with chloroform for 24 h, then extracted with 0.5 mol/L potassium sulfate solution, and the difference of the carbon content between the fumigated and the unfumigated samples was determined to be the carbon content of microorganisms in the sediments. The non-fumigated fraction was directly used as the dissolved organic carbon (DOC).
Sediment Enzymes
The activities of four enzymes indicative of C-cycling (β-galactosidase), N-cycling (urease), P-cycling (acid phosphatase) and S-cycling (arylsulfatase) were evaluated with 4-nitrophenyl beta-D-glucopyranoside, urea, 4-nitrophenyl phosphate disodium, and 4-nitrophenyl sulfate potassium as the substrates, respectively, according to Bowles et al. (2014). Briefly, the enzyme activities were assayed using 1 g of air-dried soil which was incubated with the substrate for 1 h or 24 h (37°C) at the optimal pH. Enzyme activities were assayed in duplicate with one control, to which the substrate was added after incubation and subtracted from the sample value. Air-dried sediments were used for enzyme analysis as previous studies have indicated that no differences were detected between assays with air-dried and field-moist samples, or resulted in the same ranking of treatments between field-moist and air-dried samples for the enzymes we evaluated (e.g., Bandick and Dick, 1999; Li and Sarah, 2003).
Potential Ammonia Oxidation Rates (PNRs)
The potential ammonia oxidation in sediments was determined by the chlorate inhibition method (Kurola et al., 2005). Briefly, sediment samples were mixed with a test medium containing 0.04 g/L KH2PO4, 0.18 g/L K2HPO4, 1.06 g/L NaClO3, and 0.2 g/L (NH4)2SO4 to form slurries. The slurries were then incubated upright in an orbital shaking incubator (175 rpm) thermostatically controlled at 25°C for 6 h. Samples of the sediment slurry were collected after 2 and 6 h of incubation, and the nitrite levels were determined. The potential ammonia oxidation rates were calculated based on the change in nitrite concentration.
Nucleic Acid Extraction and amoA Gene Amplification
Approximately 1 g (wet weight) of sediment was transferred into a sterile 1.5 ml centrifuge tube. Total genomic DNA was extracted using the DNeasy® PowerSoil® Kit (QIAGEN, Hilden, Germany) according to the manufacturer’s instructions. Briefly, the sediment was defrosted and homogenized in lysis buffer, then subjected to disruption by bead-beating, and finally centrifuged at 10,000 g for 30 s. The supernatant was then added with extraction buffer and extracted twice. An additional cleaning step was conducted using the MB Spin Column. The extracted DNA was eluted in 50 μl of ultra clean water and stored at -20°C for further analyses.
The primers and reaction conditions for amoA gene amplification are listed in Supplementary Table S2. PCR amplification was performed in a 25 μl reaction volume containing 1.5 μl of DNA template (50–200 ng per reaction), 2X Utaq master mix (ZOMANBIO Inc., Beijing, China), and 1.5 μl of forward and reverse primers (10 μM).
Quantitative Analysis by Real-Time PCR
DNA extracts were used as templates to quantify bacterial and archaeal amoA in the SG Fast qPCR Master Mix (High Rox) (BBI, Sangon Biotech, Shanghai, China) by real-time PCR (ABI StepOnePlus, ABI, Life Tech., Foster City, CA, United States). The primer sets used here were the same as those used in the general PCR (Supplementary Table S2). qPCRs were conducted in duplicate in total volumes of 20 μl containing 10 μl SybrGreen qPCR Master Mix, 2 μl template DNA (10–40 ng per reaction), and 0.4 μl of forward and reverse primers (10 μM). The reaction conditions are provided in Supplementary Table S3.
Inhibition by co-extracted compounds was evaluated by qPCR with different dilutions of the DNA template. Briefly, sediment DNA with 10 times dilutions was used as the template for the qPCR assay with the amoA primers (Supplementary Table S2), and the inhibition was validated by comparing the threshold cycle (Ct) values between different dilutions. The qPCR assay was performed following the same procedure described above. The results indicated that inhibition was not detected or was negligible in most cases.
Plasmids extracted from the AOB or AOA clones (see the following section) were used as a standard for the quantitative analysis of the bacterial or archaeal amoA gene, respectively. The plasmid concentration was determined using an UV-vis Spectrophotometer (ND-2000, Thermo Scientific, DE), and was used to calculate the copy numbers of amoA genes. The plasmids of the amoA gene clones with known concentrations were diluted to produce the standard curve over six orders of magnitude. Melting curve analysis was performed to verify the specificity of the amplification.
Clone Library Construction and Sequencing
Clone libraries were constructed using the amplified amoA genes. PCR products were obtained and purified from size-verified gel bands using SanPrep Column PCR Product Purification kits (Sangon Biotech, Shanghai, China). The purified DNA was ligated to a pUCm-T vector (Beyotime, Shanghai, China), then transformed into Escherichia coli DH5α competent cells for colone library construction. Thirty to sixty colonies from each clone library were randomly selected. Plasmids were extracted from each colony after culturing overnight at 37°C using Uniq-10 Column Plasmid Minipreps Kits (Sangon, Shanghai, China). Plasmids of the correct size, verified by enzyme digestion and gel electrophoresis, were subjected to an ABI 3730xl DNA analyzer (Applied Biosystems, Foster City, CA, United States) for sequence analysis.
Molecular Analysis
Chimera-free amoA gene sequences with similarities equal to or greater than 97% were grouped into the same operational taxonomic units (OTUs). The coverage of each clone library, the OTU-based richness and diversity indices, and the rarefaction analyses were calculated using mothur v.1.39.5 (Schloss et al., 2009). The weighted UniFrac distances between different communities were calculated using mothur. Principal Coordinates Analysis (PCoA) based on the weighted UniFrac distance were performed using the ape package (Paradis et al., 2004), and redundancy analysis (RDA) and canonical correlation analysis (CCA) were performed using the vegan package (Oksanen et al., 2018) in R v. 3.4.3 (R Core Team, 2017). Plots of heatmaps, RDA and CCA were performed using the ggplot2 (Wickham, 2009) package in R.
The bacterial and archaeal sequences recovered from clone libraries were blasted in GenBank using the BLAST1. The most closely related sequences and additional reference sequences were retrieved and subsequently aligned with representative clones in CLUSTALX (version 2.0.11). Phylogenetic neighbor-joining tree were constructed by MEGA (version 7.0.26).
Statistical Analysis
All statistical analyses were performed with the IBM SPSS software package version 19 (IBM Corp. Armonk, NY, United States). The significance of differences between two or multiple groups was evaluated by Student’s t-test and one-way ANOVA. Data were expressed as the mean ± standard deviation (SD) and considered significantly different at p < 0.05. The correlation between two variables was tested by Pearson product-moment correlation method and was considered significant at p < 0.05.
Nucleotide Sequence Accession Numbers
The representative bacterial and archaeal amoA gene sequences reported in this study have been deposited in GenBank under accession numbers MH316770–MH316814 and MH316815-MH316856.
Results
Physicochemical Characteristics of Different Aquaculture Ponds
The water characteristics of the ponds in the different sampling areas are listed in Supplementary Table S4. The water temperature and NO2- in the Heilongjiang ponds were significantly lower (p < 0.05), but pH, DO, and NH4+ were significantly higher (p < 0.05) compared to ponds in other areas. The water pH values in the Heilongjiang ponds ranged from 8.9 to 9.38, indicating an alkaline environment in Heilongjiang pond water.
Despite the water being alkaline, the sediment in the Heilongjiang ponds was relatively neutral, with a pH range from 7.44 to 7.86, and showed no significant difference to ponds in other areas. Sediment TN in different ponds ranged from 1438.40 to 2528.12 mg/kg, TP ranged from 798.43 to 2291.96 mg/kg, NO3- ranged from 0.40 to 2.32 mg/kg, NH4+ ranged from 22.57 to 547.62 mg/kg, DOC ranged from 67.71 to 949.75 mg/kg, urea ranged from 1.45 to 2.90 μg/g, and water- and acid-soluble sulfate ranged from 84.70 to 2188.87 and 0 to 7154.50 mg/kg, respectively. The differences of these parameters in different pond areas were not significant, however sediment NO2-, TOC, MBC and enzymes showed significant differences among the ponds in different areas (Table 1).
The nitrite concentrations in Zhujiang pond sediments were significantly higher than in other areas (p < 0.05), ranging from 0.21 to 0.36 mg/kg. Sediment TOC and MBC in the Zhujiang ponds were significantly higher compared to the Changjiang and Heilongjiang ponds (p < 0.05), respectively, indicating relatively higher organic matter content in Zhujiang pond sediments. β-glucosidase in Heilongjiang pond sediments was significantly higher than in Changjiang ponds (p < 0.05), ranging from 67.20 to 79.89 mg p-nitrophenol kg-1 soil h-1, indicating relatively higher C-cycling activities in Heilongjiang pond sediments. Urease was significantly higher in Zhujiang pond sediments compared to ponds in other areas (p < 0.05), ranging from 1957.06 to 2252.74 mg NH4+ kg-1 soil 24 h-1, which suggests higher rates of nitrogen decomposition in Zhujiang pond sediments. The values of sediment arylsulfatase in the Zhujiang ponds ranged from 576.28 to 897.54 mg p-nitrophenol kg-1 soil h-1, and were significantly higher than in the Changjiang ponds (p < 0.05), indicating relatively higher S-cycling activities in Zhujiang pond sediments.
amoA Gene Abundances and Potential Ammonia Oxidation Rates
The copy number of AOB amoA genes ranged from 1.07 × 105 to 2.90 × 107 copies/g dry soil, while AOA ranged from 1.04 × 106 to 1.30 × 108 copies/g dry soil (Figure 1). AOA were more abundant than AOB in Changjiang and Heilongjiang ponds. AOA and AOB numbers were not significantly different in Zhujiang pond sediments. The ratios of AOA/AOB amoA gene copies ranged from 0.61 to 76.45. Compared to other sampled areas, there were more AOB copies in the Zhujiang ponds and more AOA copies in the Heilongjiang ponds. However, AOB and AOA copies in different sampling areas were not significantly different, possibly due to large variations between different ponds.
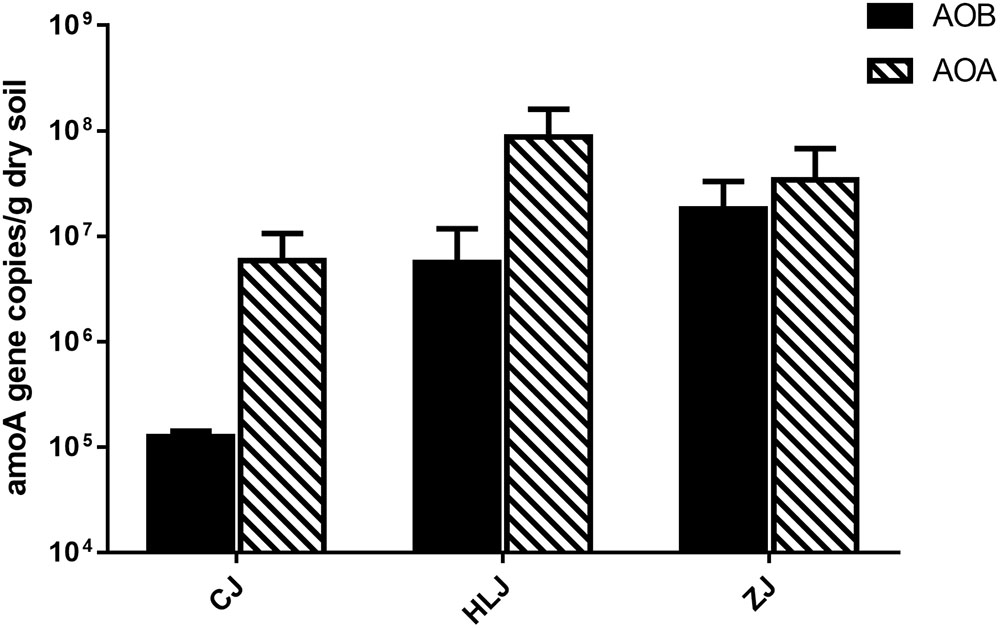
FIGURE 1. Abundance of bacterial and archaeal amoA gene in different sampling areas. Pond samples: CJ, Changjiang; HLJ, Heilongjiang; ZJ, Zhujiang.
The potential ammonia oxidation rates (PNRs) in different sampling ponds were determined to assess possible ammonia oxidation activities in the sediment. PNRs ranged from 6.37 to 63.76 ng NO2-N/g dry mass of soil/h, but were not significantly different between ponds in different areas. Correlation analyses indicate significant correlation between the abundance of AOB and arylsulfatase (R2 = 0.679, p < 0.05), as well as the abundance of AOA and TN (R2 = 0.791, p < 0.05) and arylsulfatase (R2 = 0.716, p < 0.05). Additionally, the AOB/AOA ratio was found to be significantly correlated with MBC (R2 = 0.699, p < 0.05). However, no significant correlations were detected between the AOA/AOB abundance and PNRs as well as NO2-, NO3-, and NH4+.
Phylogeny of Bacterial and Archaeal amoA Genes
Ammonia-Oxidizing Bacteria (AOB)
A total of 45 AOB OTUs were detected in all pond sediments based on a 3% divergence cutoff, including 11, 29, and 23 OTUs in the Changjiang, Heilongjiang and Zhujiang ponds, respectively. The representative OTUs were grouped into seven clusters with robust phylogenetic support based on their relative similarity to phylogenetic reference sequences, covering two out of the three main lineages in the AOB phylogeny (Figure 2).
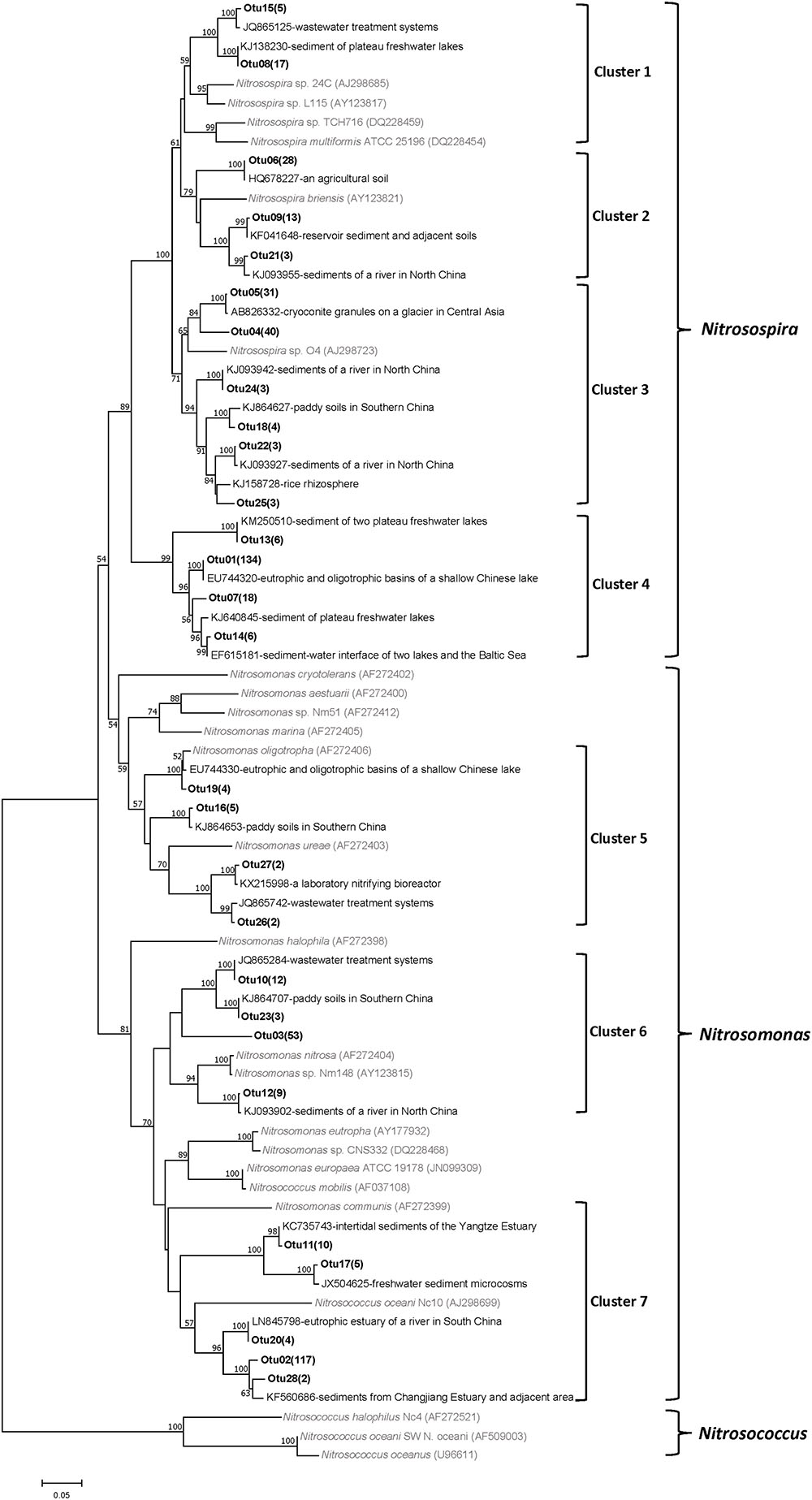
FIGURE 2. Phylogenetic tree of representative AOB OTU and reference sequences from GenBank. The bold number in parentheses behind OTU represents the numbers of the sequences detected. Numbers at the nodes indicate the levels of bootstrap support based on neighbor-joining analysis of 1000 resampled datasets. Only bootstrap values > 50% are represented. The scale bar represents 5% sequence divergence.
Sequences identified as belonging to Nitrosospira were grouped into Cluster 1 (85.8% similar to Nitrosospira multiformis ATCC 25196), Cluster 2 (88.2–89.2% similar to Nitrosospira briensis), Cluster 3 (84.8–86.7% similar to Nitrosospira briensis), and Cluster 4 (74.9–78.9% similar to Nitrosospira briensis). Sequences grouped into Cluster 1 and Cluster 4 were mainly detected in the Changjiang and Heilongjiang ponds. Sequences grouped into Cluster 2 were detected in the Heilongjiang and Zhujiang ponds. Sequences grouped into Cluster 3 were mainly detected in the Heilongjiang ponds.
Sequences identified as belonging to Nitrosomonas were grouped into Cluster 5 (84.2–98.5% similar to Nitrosomonas oligotropha or Nitrosomonas ureae), Cluster 6 (83.6–90.7% similar to Nitrosomonas nitrosa), and Cluster 7 (74.9–82.4% similar to Nitrosomonas communis). Sequences grouped into Cluster 5 were detected in the Heilongjiang and Zhujiang ponds. Sequences grouped into Cluster 6 and Cluster 7 were detected in all sampling areas.
Ammonia-Oxidizing Archaeal (AOA)
A total of 42 AOA OTUs were detected in all pond sediments based on a 3% divergence cutoff, including 17, 11, and 22 OTUs in the Changjiang, Heilongjiang, and Zhujiang ponds, respectively. The representative OTUs were grouped into six clusters based on their relative similarity to the reference sequences, covering three out of the five main lineages in the AOA phylogeny (Figure 3).
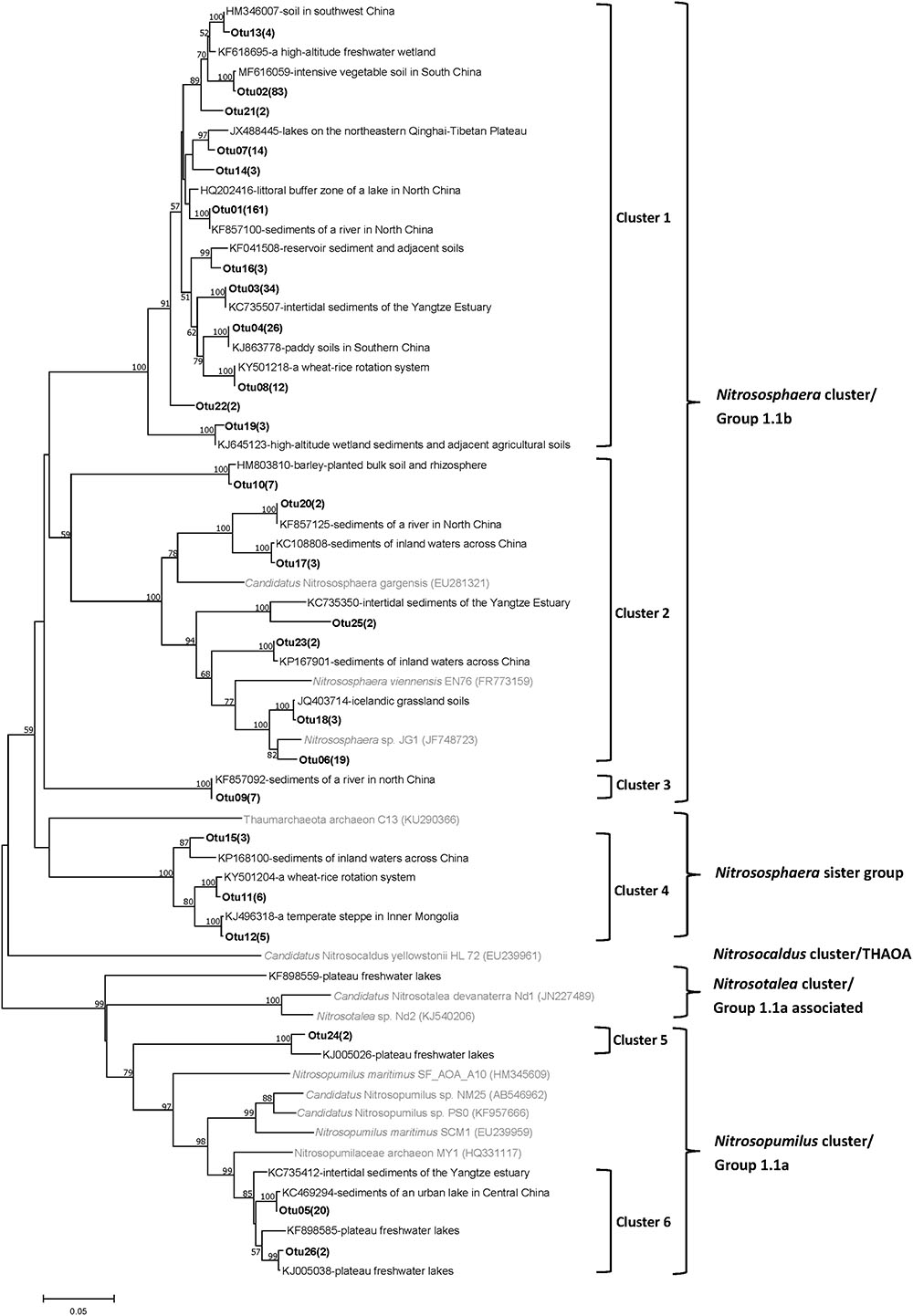
FIGURE 3. Phylogenetic tree of representative AOA OTU and reference sequences from GenBank. The bold number in parentheses behind OTU represents the numbers of the sequences detected. Numbers at the nodes indicate the levels of bootstrap support based on neighbor-joining analysis of 1000 resampled datasets. Only bootstrap values > 50% are represented. The scale bar represents 5% sequence divergence.
Sequences identified as belonging to Nitrososphaera cluster/Group 1.1b were grouped into Cluster 1 (74.4–79.3% similar to Nitrososphaera viennensis EN76 or Candidatus Nitrososphaera gargensis), Cluster 2 (74.2%–91.3% similar to Nitrososphaera viennensis EN76 or Candidatus Nitrososphaera gargensis), and Cluster 3 (only one OTU, 75% or 77.5% similar to Nitrososphaera viennensis EN76 or Candidatus Nitrososphaera gargensis, respectively). Sequences grouped into Cluster 3 were only detected in the Zhujiang ponds, and Cluster 1 and Cluster 2 included sequences from all sampling areas.
Cluster 4 fell into a phylogenetic group known as the Nitrososphaera sister group which was first proposed in 2011 (Pester et al., 2012). Sequences were grouped with an assembled amoA gene sequence (with similarity of 78.1–79.1%) from a novel AOA belonging to the Nitrososphaera sister cluster (Lehtovirta-Morley et al., 2016). The sequences in Cluster 4 were detected in the Changjiang and Heilongjiang but not the Zhujiang ponds.
The remaining sequences were identified as Nitrosopumilus Cluster/Group 1.1a AOA and were divided into two clusters, Cluster 5 and Cluster 6. Cluster 5 contained only one OTU (78.7% similar to Nitrosopumilus maritimus SCM1) and was detected in only the Changjiang and Zhujiang ponds. Cluster 6 sequences showed relatively higher identities to Nitrosopumilus maritimus SCM1 (88.6–88.8%), and was distributed in only the Chanjiang and Zhujiang ponds.
AOB and AOA Diversity and Community Structure
Rarefaction analysis of bacterial and archaeal amoA gene libraries (Supplementary Figure S1) showed an average coverage of 94 and 91%, respectively, and no significant differences were found in coverage between the different sampling areas.
The average values of AOB richness and the diversity indices of pond sediments in the different sampling areas are presented in Supplementary Table S5. The OTU numbers and diversity of AOB were slightly lower in the Changjiang ponds compared to those in other areas, but the difference was not statistically significant. However, the OTU numbers and diversity of AOA were significantly different (p < 0.05), particularly between the Heilongjiang and Zhujiang ponds (Table 2). The Zhujiang ponds had higher AOA richness and abundance than the Heilongjiang ponds. The values of Chao1 (19–21.5), and Shannon (1.66–2.33) in the Zhujiang ponds indicated a relatively high AOA diversity in Zhujiang pond sediments.
Statistical analyses indicated that the AOB Shannon index was significantly correlated with TOC (R2 = 0.691, p < 0.05), PNR (R2 = 0.740, p < 0.05), and arylsulfatase (R2 = 0.734, p < 0.05), while the AOA Shannon index was significantly correlated with MBC (R2 = 0.673, p < 0.05) and β-glucosidase.
The representative amoA gene sequences were used to make comparisons between the microbial community structures of different sampling areas through principal coordinates analysis (PCoA) based on weighted UniFrac distances (Figure 4). The AOB communities of Changjiang pond sediments deviated from those of the Zhujiang and Heilongjiang ponds, as they were distributed along separate areas of the axes with a total explanation percentage of 87.7%. However, the AOB communities of Zhujiang and Heilongjiang pond sediments tended to group together (Figure 4A). Clustering analysis using OTU sequences from the pond samples also indicated a similarity between Zhujiang and Heilongjiang AOB communities (Supplementary Figure S2), probably due to shared OTUs between the Zhujiang and Heilongjiang samples (a total of 13 OTUs were found to be shared between the two areas).
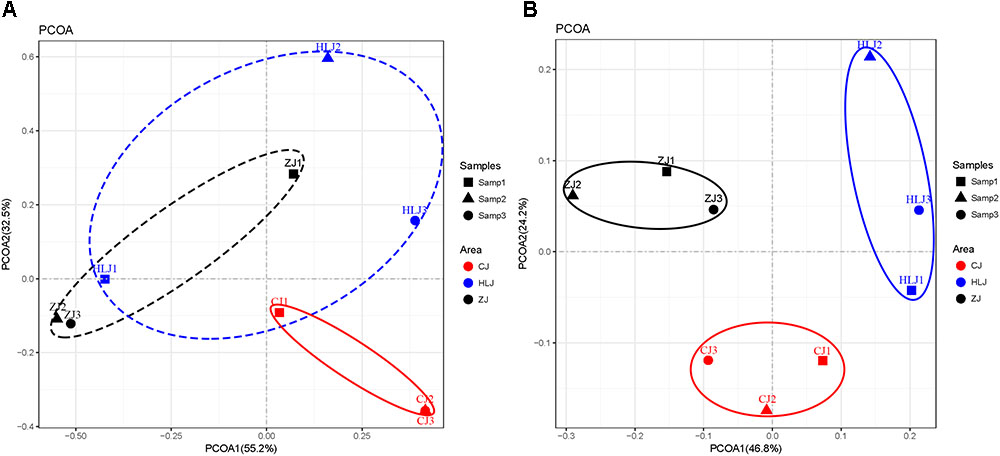
FIGURE 4. Principal coordinates analysis (PCoA) using weighted UniFrac algorithm of AOB (A) and AOA (B) communities revealed by amoA genes. Pond samples: CJ, Changjiang; HLJ, Heilongjiang; ZJ, Zhujiang.
However, AOA communities from different areas were distinctively separated along the axes with a total explanation percentage of 71% (Figure 4B), indicating the presence of distinctive AOA communities in the different sampling areas. Similarly, the AOA OTUs of different areas were also clearly separated by clustering analysis (Supplementary Figure S2).
AOB and AOA Community Compositions and Potential Environmental Drivers
AOB and AOA Community Compositions
The representative AOB OTUs were displayed in a heatmap to show the microbial compositions of pond sediments in the different sampling areas (Figure 5A). The identified OTUs of AOB were further referred back to their phylogenetic structure and grouped into species. The proportions of the detected species in each pond sample are shown in Figure 5B. In Changjiang pond sediments, Nitrosospira cluster 4 was the most prominent AOB cluster/species, mainly in the form of Otu01. Nitrosomonas cluster 7 and Nitrosospira cluster 3 were the most prominent clusters in Heilongjiang pond sediments, mainly consisting of Otu02, Otu04, and Otu05. Nitrosospira cluster 2 and Nitrosomonas cluster 7 were the most prominent clusters in Zhujiang ponds, mainly containing Otu06 and Otu02.
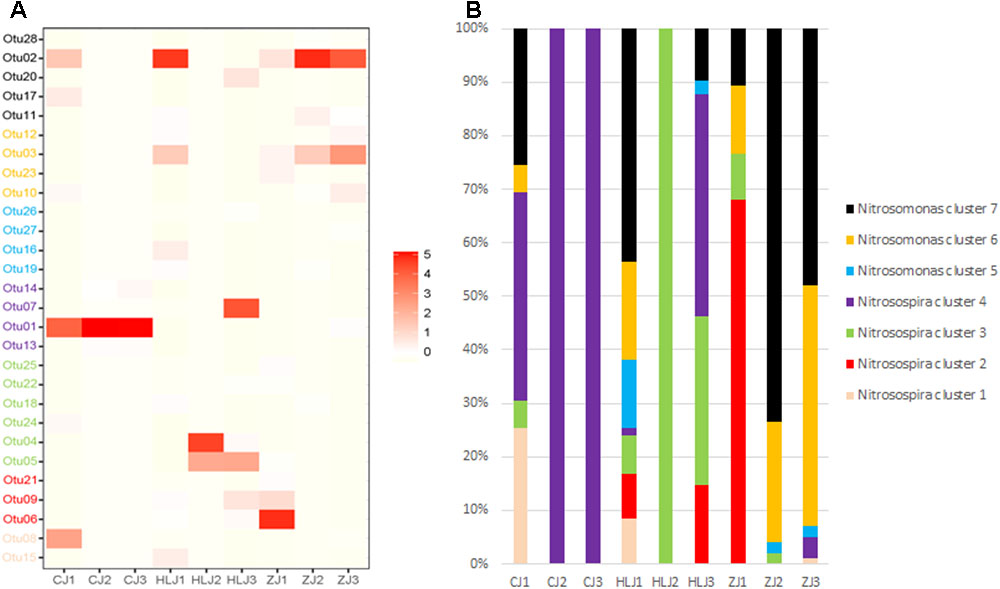
FIGURE 5. Heatmap showing the relative abundance of AOB representative OTUs among different ponds (A) and frequency of each identified AOB cluster/species in different sampling areas (B). The colors in the heatmap represent the lowest to highest frequency of OTUs, from white to red, respectively. Pond samples: CJ, Changjiang; HLJ, Heilongjiang; ZJ, Zhujiang.
For AOA OTUs, the most prominent cluster/species was Nitrososphera Cluster 1 for all pond sediments in the different sampling areas (Figure 6A). Additionally, Nitrososphera Cluster 1 also constituted large percentages of the species compositions (Figure 6B). Otu01 and Otu02 were the most prominent AOA cluster/species in Changjiang pond sediments. Otu01 was the most prominent AOA in Heilongjiang pond sediments. For the Zhujiang ponds, in addition to Nitrososphera Cluster 1 cluster/species (Otu01, Otu03, and Otu04), Otu06 from Nitrososphaera Cluster 2 also contributed significantly to the AOA species composition.
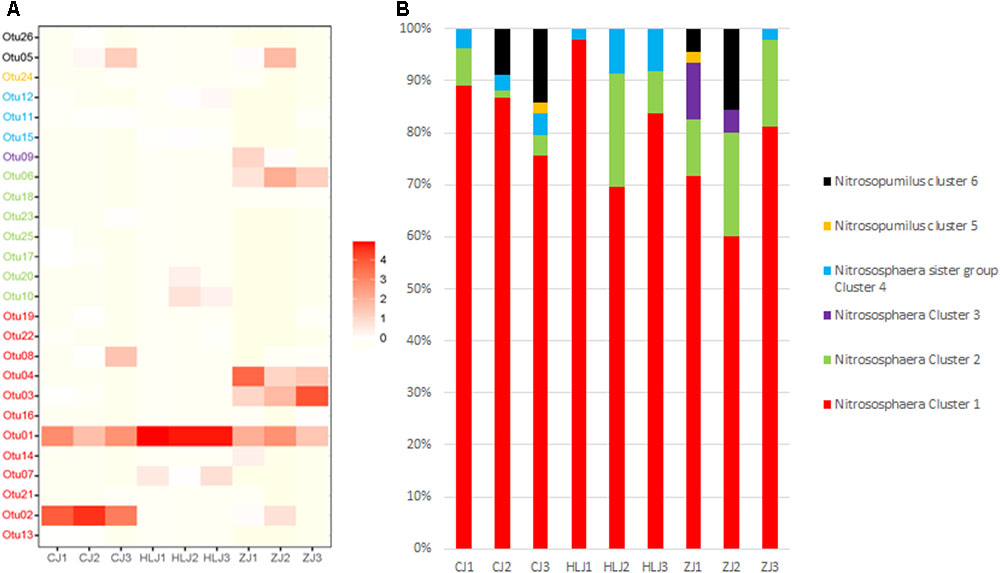
FIGURE 6. Heatmap showing the relative abundance of AOA representative OTUs among different ponds (A) and frequency of each identified AOA cluster/species in different sampling areas (B). The colors in the heatmap represent the lowest to highest frequency of OTUs, from white to red, respectively. Pond samples: CJ, Changjiang; HLJ, Heilongjiang; ZJ, Zhujiang.
Potential Environmental Drivers
The correlations between AOB and AOA community compositions and the associated environmental variables were analyzed by multivariate analysis CCA and RDA, respectively (Figure 7). The environmental variables in the first two dimensions of CCA and RDA explained 50.07 and 46.89%, respectively, of the total variance in the bacterial (Figure 7A) and archaeal (Figure 7B) prominent amoA genotype compositions (OTUs with sequence numbers ≥ 7).
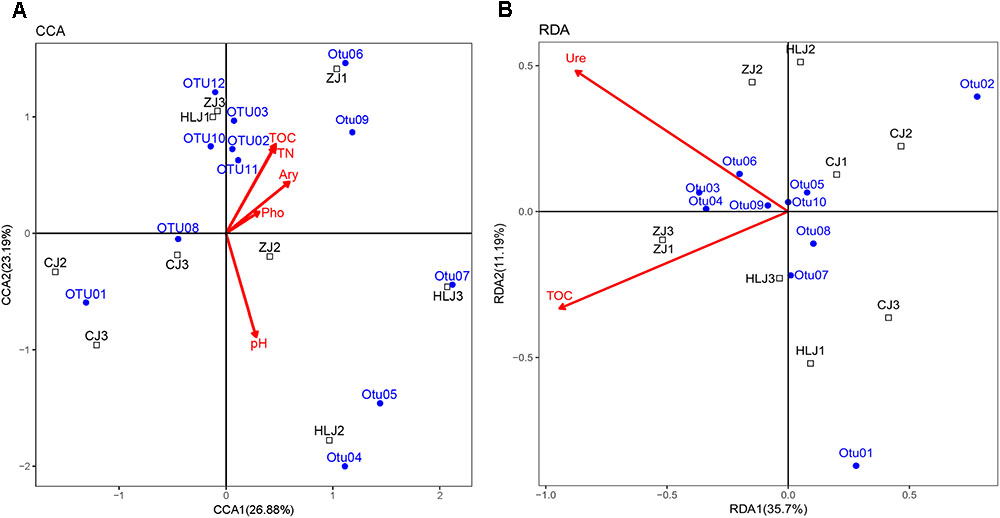
FIGURE 7. CCA and RDA ordination plots for the relationship between AOB (A) and AOA (B) prominent OTUs (sequence numbers ≥ 7) with the environmental parameters in different ponds. Pond samples: CJ, Changjiang; HLJ, Heilongjiang; ZJ, Zhujiang. TOC, total organic carbon; TN, total nitrogen; Ary, arylsulfatase; Pho, acid phosphatase; Glu, β-glucosidase; Ure, urease.
Sediment TOC, TN, pH, acid phosphatase and arylsulfatase were significantly correlated with the prominent AOB amoA genotypes (Supplementary Table S6). These factors provided 26.88, 23.19, 11.19, 5.69, and 0.58% of the total CCA explanatory power. Most OTUs of AOB were positively correlated with sediment TOC, TN, acid phosphatase, and arylsulfatase levels, but negatively correlated with sediment pH.
For AOA, TOC and urease were found to be significantly correlated with the prominent amoA genotype compositions (Supplementary Table S7), and provided 35.70 and 11.19% of the total RDA explanatory power. Most of the AOA OTUs were positively correlated with TOC, including Otu01, which was detected in all the sampled ponds. However, several of the dominant OTUs were found to be negatively correlated with urease, including Otu01 and Otu02.
Discussion
AOB and AOA Abundance in Surface Sediments of Aquaculture Ponds
Our understanding on AOB and AOA abundance in aquaculture pond sediments is still very limited. The predominance of AOB or AOA varies between different freshwater sediments (Yang et al., 2016), and inconsistent results have been found in previous aquaculture environment studies. For example, AOB abundance was found to be higher than AOA in Mandarin fish pond sediments (Zhou et al., 2017), while the abundance of AOA was nearly an order of magnitude higher than AOB in polyculture pond sediments (Lu et al., 2015, 2016). In this study, AOA were predominant in all the aquaculture ponds except one, but great variations in AOB and AOA abundance were found between different ponds. Although a much higher abundance of AOB (up to 2.90 × 107 copies/g dry soil) and AOA (up to 1.30 × 108 copies/g dry soil) were detected in some of our aquaculture ponds compared to the aforementioned studies, or even compared to some soils (Wang J. et al., 2014; Tago et al., 2015), the abundance of AOB and AOA was up to two orders of magnitude lower in other ponds. Considering the significant differences between aquaculture ponds (Table 1), the large variations in terms of abundance could be due to the different trophic statuses.
Due to the large amounts of decomposition during culturing resulting from feeding debris and species excrements, the surface sediments of aquaculture ponds are usually rich in organic substances and may exhibit high NH4+ concentrations. Ammonia concentration is considered an important factor that might affect the abundance of AOB/AOA amoA genes, due to their different affinities to NH4+ (Martens-Habbena et al., 2009). However, our results indicated that the abundance of AOA/AOB was not significantly correlated with NH4+. By comparing our results with those of other studies, we found that the concentrations of NH4+ in our study (up to 547.62 mg/kg) were much higher than in other environments (lower than 50 mg/kg, like soils e.g., Wang et al., 2015; and sediments e.g., Zheng et al., 2013). Such high concentrations may indicate that NH4+ might not be a limiting factor for AOB/AOA in aquaculture pond sediments, which correlates with similar results found in a previous study on aquaculture ponds (Lu et al., 2016). In fact, lower concentrations of NH4+ (28.40–72.93 mg/kg) have been found to be more than what is required to support AOA communities in forest soils (Wu et al., 2017). Thus, we deduced that NH4+ might not be a significant regulating factor for AOB/AOA in aquaculture pond sediments with high NH4+ concentrations. Organic substances, on the other hand, are another important indicator of trophic state and have been shown to greatly affect AOB/AOA abundance in trophic freshwater sediments (e.g., Herrmann et al., 2009; Wu et al., 2010; Hou et al., 2013). Due to the possible inhibition of AOA by organic compounds (Könneke et al., 2005), AOB tended to outnumber AOA in organic-rich environments, as illustrated by the negative correlation between the abundance of AOA and TOC (e.g., Wu et al., 2010). In contrast, a positive correlation between AOA abundance and TOC was found in an aquaculture pond (Lu et al., 2016), indicating that very different conditions might exist in aquaculture pond sediments, however, the mechanisms of which are unknown. In the present study, we used sediment enzymes to evaluate the dynamics of organic substances in aquaculture pond sediments. Similarly, AOA/AOB abundance was found to be positively correlated with TOC in our study. A significantly positive correlation was found between the abundance of AOB and the concentration of arylsulfatase (R2 = 0.679, p < 0.05), as well as the abundance of AOA and TN (R2 = 0.791, p < 0.05) and arylsulfatase (R2 = 0.716, p < 0.05). To our knowledge, this is the first study to report correlations between arylsulfatase in sediment and ammonia-oxidizing microorganisms in freshwater ecosystems.
Enzymes, suggested to be good indicators of soil quality because of their important role in the decomposition of organic matter as well as in nutrient cycling (Aon et al., 2001), are commonly analyzed components of in soil (e.g., Li and Sarah, 2003; Bowles et al., 2014). Air-drying is an important practice in soil characterization because it allows for easy handling (Zornoza et al., 2006; Lopes et al., 2015) and could possibly eliminate the activity of unstable enzymes and leave only stable enzymes, which could have an important ecological effect on soil quality (Nannipieri et al., 1990). However, the evaluation of enzymes in sediments is still very limited. In this study, air-dried sediments were used to evaluate four enzymes which have been proved stable and important in nutrient cycling (Bandick and Dick, 1999; Li and Sarah, 2003). Among these, arylsulfatase catalyzes the hydrolysis of organic sulfate esters and is primarily produced by sediment microorganisms, and may constitute a rate-limiting stage in S cycling (Tabatabai and Bremner, 1970; Chröst, 1991). In this study, arylsulfatase was analyzed to evaluate the process of S-related organic nutrients, considering the high input of protein-rich feed which release S after being decomposed. Indeed, sulfate, as a decomposition product of arylsulfatase, could be detected in the sediments of our aquaculture ponds. Thus, the correlation of AOA and AOB abundance to arylsulfatase may indicate a relationship between ammonia oxidizers and the availability of organic sulfur compounds to microorganisms in aquaculture pond sediments. We further deduced that organic matter (especially organic sulfur) related microorganisms may contribute to the positive correlation between AOB/AOA abundance and TOC in aquaculture pond sediments. Indeed, our results also showed a significant correlation between AOB/AOA ratio and MBC, indicating a relationship between AOB/AOA and sediment microorganisms. However, limited information is known about the impact of organic substance dynamics on microorganisms as well as their interconnections in aquaculture pond sediments. Further studies are needed to elucidate the contribution of TOC on the abundance of ammonia-oxidizing microorganisms in aquaculture ponds.
Potential Ammonia Oxidation Rate in Surface Sediments of Aquaculture Ponds
Considering the unnecessary correlation between the amoA gene and the activity of ammonia oxidation in organisms (Prosser and Nicol, 2008; Veuger et al., 2013), ammonia oxidation rate has usually been determined to obtain more comprehensive information. Many studies use the 15NH4+ isotope dilution technique to detect ammonia oxidation rates in water (Ward, 2011) and sediment (Chen et al., 2016). The addition of chlorate to soil slurry is another method for determining ammonium oxidation, which is suggested by the International Organization for Standardization [ISO 15685:2012(E)]. The chlorate inhibition method has been successfully applied in the past to determine potential ammonia oxidation in soil (e.g., Kurola et al., 2005; Hu et al., 2015; Hayashi et al., 2016) and sediment environments (e.g., Hall, 1984; Kitidis et al., 2011; Laverock et al., 2013). In addition, a recent study on aquaculture pond sediments also used this method to determine the potential ammonia oxidation rates (Lu et al., 2016). Thus, we deduced that the method for determining potential ammonia oxidation rates using chlorate inhibition in our study could produce reliable and comparable results. Indeed, the PNRs detected in this study correlated with those of the above-mentioned studies, that is relatively lower than detected in the soils (Kurola et al., 2005; Hu et al., 2015), but higher than in the sediment of an aquaculture pond (Lu et al., 2016). In addition, a relatively smaller range of PNRs was detected in our study compared to those in soils, possibly indicating ubiquitous inhibition on the activity of nitrifiers in aquaculture pond sediments, especially considering the similarity of low PNRs between pond samples of different areas.
AOB and AOA Diversity in Surface Sediments of Aquaculture Ponds
Like with abundance, there are few reports regarding AOB and AOA diversity in aquaculture pond sediments. Zhou et al. (2017) reported higher AOB diversity than AOA in the sediment of ponds with Mandarin fish. In the present study, different results were observed between ponds. AOA OTU richness and diversity were higher than AOB in the sediments of Changjiang and Zhujiang ponds, but AOB was higher in Heilongjiang pond sediments (see Table 2 and Supplementary Table S5), indicating varied AOB/AOA diversity in different aquaculture pond sediments. The eutrophic level of freshwater sediments have been suggested to positively influence ammonia-oxidizing microbial populations (Yang et al., 2016). Correlation analysis of different ponds in our study also suggested that sediment AOB diversity was positively affected by TOC (R2 = 0.691, p < 0.05). Moreover, AOB and AOA diversity were also significantly correlated with arylsulfatase (R2 = 0.734, p < 0.05) and β-glucosidase (R2 = 0.673, p < 0.05), respectively. These are important extracellular enzymes during transformation of organic matter, and are primarily produced by microbial organisms in sediment. As such, the activity of microorganisms decomposing organic matter might influence ammonia oxidizers in aquaculture pond sediments. Indeed, AOA diversity was also significantly correlated with MBC in our sampling of pond sediments.
AOB and AOA Community Structure in Surface Sediments of Aquaculture Ponds
The archaeal and bacterial amoA gene sequences in this study were found to be closely related with those detected in soil and freshwater environments (Figures 2, 3), indicating a high homology of ammonia oxidizers in aquaculture pond sediments and other environments. All AOB were affiliated to Nitrosospira and Nitrosomonas clusters, similar to previous studies in aquaculture pond sediments (Lu et al., 2016) and freshwater lake sediments (Bao et al., 2016). However, there were also large variations between different AOB OTUs (similarity to known isolates varied from 74.9 to 98.5%), and different AOB clusters were distributed in our sampling areas, indicating large variations in the AOB community in aquaculture pond sediment. Similarly, different AOA community compositions were also detected in the sampling areas. AOA were classified into the Nitrososphaera cluster, Nitrososphaera sister cluster, and Nitrosopumilus cluster according to Pester et al. (2012), which was in accordance with studies on freshwater sediments (Zheng et al., 2013; Yang et al., 2016), but large variations were found between different clusters (similarity to known isolates varied from 74.2 to 91.3%). Pond sediments in different areas were also distributed with varying AOA clusters.
PCoA analysis with representative amoA gene sequences further identified AOB and AOA community variations in the pond sediments of different sampling areas (Figure 4). Although the levels of sediment AOB showed some similarity between the Heilongjiang and Zhujiang ponds, most ponds in these areas were distinctly different. Indeed, sediment AOB communities in these areas were dominated by different amoA gene sequences (Figure 5A), and different AOB phylogenetic clusters were detected in the sampling areas (Figure 5B). The proportions of Nitrosospira and Nitrosomonas were found to be varied in lake sediments (Liu et al., 2015). In aquaculture environments, both Nitrosospira and Nitrosomonas could be predominant (Lu et al., 2016; Zhou et al., 2017). In the present study, the Nitrosospira cluster was prominent in the Changjiang ponds, while the Nitrosomonas and Nitrosospira clusters were prominent in the Heilongjiang and Zhujiang ponds. This suggests that niche separation might exist for AOB species in aquaculture pond sediments. Previous studies on freshwater sediments have indicated that AOB components were differently distributed along the trophic gradients from mesotrophic to eutrophic (Hou et al., 2013; Yang et al., 2016). In this study, AOB were also found to be significantly influenced by sediment eutrophic status (TOC and TN levels), and pH (Figure 7A). In addition, acid phosphatase and arylsulfatase in sediments were significantly correlated with AOB prominent amoA genotypes, indicating that the bio-availability of organic P and S might significantly affect AOB communities in aquaculture pond sediments.
For AOA, the Nitrososphaera cluster was dominant in all the sampling pond sediments (Figure 6), a similar result seen in previous studies on aquaculture pond sediments (Lu et al., 2016; Zhou et al., 2017), but more AOA clusters were found in our study (e.g., the Nitrosopumilus Cluster). Nitrososphaera AOA were found to be dominant in mesotrophic lakes compared to oligotrophic lakes (Bollmann et al., 2014; Yang et al., 2016). The dominance of the Nitrososphaera cluster in our ponds may be due to the high eutrophic levels of aquaculture pond sediments compared to those of other environments. In addition, although the predominance of Nitrososphaera cluster was ubiquitous, the sediment AOA species and phylogenetic compositions varied in different sampling areas. RDA analysis using representative archaeal amoA gene sequences indicated that TOC significantly contributed to the variety in AOA communities observed in different areas. However, dominant Nitrososphaera cluster sequences (Otu01 and Otu02) were found to be negatively correlated with urease, which is important in N-cycling in sediments, indicating a negative effect of nitrogen transformation on Nitrososphaera cluster species in aquaculture ponds. Previous study on the soils of different geographic regions have also indicated the negative effect of soil nitrogen on the AOA Nitrososphaera cluster (Pester et al., 2012). However, further ecophysiological studies based on cultivation are needed to better understand the ecology of these ammonia oxidizers.
Conclusion
In conclusion, this study revealed that AOB and AOA were abundant in Ctenopharyngodon idellus aquaculture pond sediments. The predominance of AOB or AOA varied between the sediments of different aquaculture ponds according to the levels of TOC. In particular, the abundance of AOB and AOA was significantly correlated with arylsulfatase, indicating a relationship between ammonia oxidizers and the availability of organic sulfur compounds in the surface sediment of aquaculture ponds. AOB and AOA diversity were also significantly correlated with arylsulfatase and β-glucosidase, respectively, in aquaculture pond sediments, indicating a correlation between AOB and AOA diversity and the activity of microorganisms decomposing organic matter. In addition, the AOA and AOB community structures were significantly correlated with TOC. Taken together, our results suggest that AOB and AOA communities in the surface sediments of Ctenopharyngodon idellus aquaculture ponds were regulated by the organic matter found in the ponds, as well as its availability to the microorganisms.
Author Contributions
XL, LT, and GL performed the design of the initial studies. LD designed the experiments and wrote the draft manuscript. LY revised the manuscript. CS and LP performed the sampling work. LD and CL performed the laboratory work. All the authors have read and approved the final draft of the article.
Funding
This work was financially supported by Special Scientific Research Funds for Central Non-profit Institutes, Chinese Academy of Fishery Sciences (2016PT08 and 2016ZD07).
Conflict of Interest Statement
The authors declare that the research was conducted in the absence of any commercial or financial relationships that could be construed as a potential conflict of interest.
Acknowledgments
The authors would like to thank Zhao Zhigang in Heilongjiang Fisheries Research Institute, Chinese Academy of Fishery Sciences, and Wang Guangjun and Zhang Kai in Pearl River Fisheries Research Institute for their great help during the sampling.
Supplementary Material
The Supplementary Material for this article can be found online at: https://www.frontiersin.org/articles/10.3389/fmicb.2018.02290/full#supplementary-material
Footnote
References
American Society for Testing and Materials (1973). Book of ASTM Standards. Part 23. Water: Atmospheric Analysis. Philadelphia, PA: ASTM, 425–431.
Aon, M. A., Cabello, M. N., Sarena, D. E., Colaneri, A. C., Franco, M. G., Burgos, J. L., et al. (2001). I. Spatio-temporal patterns of soil microbial and enzymatic activities in an agricultural soil. Appl. Soil Ecol. 18, 239–254. doi: 10.1016/S0929-1393(01)00153-6
Avnimelech, Y., and Ritvo, G. (2003). Shrimp and fish pond soils: processes and management. Aquaculture 220, 549–567. doi: 10.1016/S0044-8486(02)00641-5
Bandick, A., and Dick, R. P. (1999). Field management effects on soil enzyme activities. Soil Biol. Biochem. 31, 1471–1479. doi: 10.1016/S0038-0717(99)00051-6
Bao, L., Wang, X., and Chen, Y. (2016). Abundance and distribution of ammonia-oxidizing microorganisms in the sediments of Beiyun River, China. Ann. Microbiol. 66, 1075–1086. doi: 10.1007/s13213-016-1191-9
Beman, J. M., and Francis, C. A. (2006). Diversity of ammonia-oxidizing Archaea and bacteria in the sediments of a hypernutrified subtropical estuary: Bahia del Tobari, Mexico. Appl. Environ. Microbiol. 72, 7767–7777. doi: 10.1128/AEM.00946-06
Bollmann, A., Bullerjahn, G. S., and McKay, R. M. (2014). Abundance and diversity of ammonia-oxidizing Archaea and bacteria in sediments of trophic end members of the Laurentian Great Lakes, Erie and Superior. PLoS One 9:e97068. doi: 10.1371/journal.pone.0097068
Bowles, T. M., Acosta-Martínez, V., Calderón, F., and Jackson, L. E. (2014). Soil enzyme activities, microbial communities, and carbon and nitrogen availability in organic agroecosystems across an intensively-managed agricultural landscape. Soil Biol. Biochem. 68, 252–262. doi: 10.1016/j.soilbio.2013.10.004
Camargo, J. A., and Alonso, A. (2006). Ecological and toxicological effects of inorganic nitrogen pollution in aquatic ecosystems: a global assessment. Environ. Int. 32, 831–849. doi: 10.1016/j.envint.2006.05.002
Cao, L., Wang, W., Yang, Y., Yang, C., Yuan, Z., Xiong, S., et al. (2007). Environmental impact of aquaculture and countermeasures to aquaculture pollution in China. Environ. Sci. Pollut. Res. 14, 452–462. doi: 10.1065/espr2007.05.426
Chen, S., Peng, X. T., Xu, H. C., and Ta, K. W. (2016). Nitrification of Archaeal ammonia oxidizers in a high-temperature hot spring. Biogeosciences 13, 2051–2060. doi: 10.5194/bg-13-2051-2016
China Environmental Protection Agency (2002). Methods for Water and Wastewater Determination. Beijing: China Environmental Science Press.
Chröst, R. J. (1991). “Environmental control of the synthesis and activity of aquatic microbial ectoenzyme,” in Microbial Enzymes in Aquatic Environments, ed. R. J. Chröst (New York, NY: Springer), 29–53. doi: 10.1007/978-1-4612-3090-8_3
FAO (2016). The State of World Fisheries and Aquaculture 2016. Contributing to food Security and Nutrition for All. Rome: FAO, 200.
Flood, M., Frabutt, D., Floyd, D., Powers, A., Ezegwe, U., Devol, A., et al. (2015). Ammonia-oxidizing bacteria and Archaea in sediments of the Gulf of Mexico. Environ. Technol. 36, 124–135. doi: 10.1080/09593330.2014.942385
Hall, G. H. (1984). Measurement of nitrification rates in lake sediments: comparison of the nitrification inhibitors nitrapyrin and allylthiourea. Microb. Ecol. 10, 25–36. doi: 10.1007/BF02011592
Hargreaves, J. A. (1998). Nitrogen biogeochemistry of aquaculture ponds 1 Approved for publication as Journal Article No. J-9356 of the Mississippi agricultural and forestry experiment station, Mississippi state university. 1. Aquaculture 166, 181–212. doi: 10.1016/S0044-8486(98)00298-1
Hayashi, K., Shimomura, Y., Morimoto, S., Uchida, M., Nakatsubo, T., and Hayatsu, M. (2016). Characteristics of ammonia oxidation potentials and ammonia oxidizers in mineral soil under Salix polaris-moss vegetation in Ny-Ålesund, Svalbard. Polar Biol. 39, 725–741. doi: 10.1007/s00300-01501829-2
Head, I. M., Hiorns, W. D., Embley, T. M., McCarthy, A. J., and Saunders, J. R. (1993). The phylogeny of autotrophic ammonia-oxidizing bacteria as determined by analysis of 16S ribosomal RNA gene sequences. J. Gen. Microbiol. 139(Pt 6), 1147–1153. doi: 10.1099/00221287-139-6-1147
Herrmann, M., Saunders, A. M., and Schramm, A. (2009). Effect of lake trophic status and rooted macrophytes on community composition and abundance of ammonia-oxidizing prokaryotes in freshwater sediments. Appl. Environ. Microbiol. 75, 3127–3136. doi: 10.1128/AEM.02806-08
Hou, J., Song, C., Cao, X., and Zhou, Y. (2013). Shifts between ammonia-oxidizing bacteria and Archaea in relation to nitrification potential across trophic gradients in two large Chinese lakes (Lake Taihu and Lake Chaohu). Water Res. 47, 2285–2296. doi: 10.1016/j.watres.2013.01.042
Hu, H. W., Zhang, L. M., Yuan, C. L., Zheng, Y., Wang, J. T., Chen, D., et al. (2015). The large-scale distribution of ammonia oxidizers in paddy soils is driven by soil pH, geographic distance, and climatic factors. Front. Microbiol. 6:938. doi: 10.3389/fmicb.2015.00938
Jimenez-Montealegre, R., Verdegem, M. C. J., Dam, A., and Verreth, J. A. (2005). Effect of organic nitrogen and carbon mineralization on sediment organic matter accumulation in fish ponds. Aquac. Res. 36, 983–995. doi: 10.1111/j.1365-2109.2005.01307.x
Kitidis, V., Laverock, B., McNeill, L. C., Beesley, A., Cummings, D., Tait, K., et al. (2011). Impact of ocean acidification on benthic and water column ammonia oxidation. Geophys. Res. Lett. 38, 1–5. doi: 10.1029/2011GL049095
Könneke, M., Bernhard, A. E., de la Torre, J. R., Walker, C. B., Waterbury, J. B., and Stahl, D. A. (2005). Isolation of an autotrophic ammonia-oxidizing marine archaeon. Nature 437, 543–546. doi: 10.1038/nature03911
Kurola, J., Salkinoja-Salonen, M., Aarnio, T., Hultman, J., and Romantschuk, M. (2005). Activity, diversity and population size of ammonia-oxidising bacteria in oil-contaminated landfarming soil. FEMS Microbiol. Lett. 250, 33–38. doi: 10.1016/j.femsle.2005.06.057
Laverock, B., Kitidis, V., Tait, K., Gilbert, J. A., Osborn, A. M., and Widdicombe, S. (2013). Bioturbation determines the response of benthic ammonia-oxidizing microorganisms to ocean acidification. Philos. Trans. R. Soc. B 368:20120441. doi: 10.1098/rstb.2012.0441
Lehtovirta-Morley, L. E., Ross, J., Hink, L., Weber, E. B., Gubry-Rangin, C., Thion, C., et al. (2016). Isolation of ‘Candidatus Nitrosocosmicus franklandus’, a novel ureolytic soil Archaeal ammonia oxidiser with tolerance to high ammonia concentration. FEMS Microbiol. Ecol. 92:fiw057. doi: 10.1093/femsec/fiw057
Leininger, S., Urich, T., Schloter, M., Schwark, L., Qi, J., Nicol, G. W., et al. (2006). Archaea predominate among ammonia-oxidizing prokaryotes in soils. Nature 442, 806–809. doi: 10.1038/nature04983
Li, J., Nedwell, D. B., Beddow, J., Dumbrell, A. J., McKew, B. A., Thorpe, E. L., et al. (2015). amoA Gene abundances and nitrification potential rates suggest that benthic ammonia-oxidizing bacteria and not Archaea dominate N cycling in the Colne Estuary, United Kingdom. Appl. Environ. Microbiol. 81, 159–165. doi: 10.1128/AEM.02654-14
Li, X., and Sarah, P. (2003). Enzyme activities along a climatic transect in the Judean Desert. Catena 53, 349–363. doi: 10.1016/S0341-8162(03)00087-0
Liu, Y., Zhang, J., Zhao, L., Li, Y., Dai, Y., and Xie, S. (2015). Distribution of sediment ammonia-oxidizing microorganisms in plateau freshwater lakes. Appl. Microbiol. Biotechnol. 99, 4435–4444. doi: 10.1007/s00253-014-6341-z
Lopes, A. A. C., Sousa, D. M. G., Reis, F. B. Jr., and Mendes, I. C. (2015). Air-drying and long-term storage effects on β-glucosidase, acid phosphatase and arylsulfatase activities in a tropical Savannah Oxisol. Appl. Soil Ecol. 93, 68–77. doi: 10.1016/j.apsoil.2015.04.001
Lu, S., Liao, M., Xie, C., He, X., Li, D., He, L., et al. (2015). Seasonal dynamics of ammonia-oxidizing microorganisms in freshwater aquaculture ponds. Ann. Microbiol. 65, 651–657. doi: 10.1007/s13213-014-0903-2
Lu, S., Liu, X., Ma, Z., Liu, Q., Wu, Z., Zeng, X., et al. (2016). Vertical segregation and phylogenetic characterization of ammonia-oxidizing bacteria and Archaea in the sediment of a freshwater aquaculture pond. Front. Microbiol. 6:1539. doi: 10.3389/fmicb.2015.01539
Martens-Habbena, W., Berube, P. M., Urakawa, H., de la Torre, J. R., and Stahl, D. A. (2009). Ammonia oxidation kinetics determine niche separation of nitrifying Archaea and Bacteria. Nature 462, 976–979. doi: 10.1038/nature08465
Nannipieri, P., Greco, S., and Ceccanti, B. (1990). “Ecological significance of the biological activity in soil,” in Soil Biochemistry, eds J. M. Bollag and G. Stotzky (New York, NY: Marcel Dekker Inc), 293–355.
Oksanen, J., Blanchet, F. G., Friendly, M., Kindt, R., Legendre, P., McGlinn, D., et al. (2018). vegan: Community Ecology Package. R package version 2.4-6. Available at: https://CRAN.R-project.org/package=vegan
Paradis, E., Claude, J., and Strimmer, K. (2004). APE: analyses of phylogenetics and evolution in R language. Bioinformatics 20, 289–290. doi: 10.1093/bioinformatics/btg412
Pester, M., Rattei, T., Flechl, S., Grongroft, A., Richter, A., Overmann, J., et al. (2012). amoA-based consensus phylogeny of ammonia-oxidizing Archaea and deep sequencing of amoA genes from soils of four different geographic regions. Environ. Microbiol. 14, 525–539. doi: 10.1111/j.1462-2920.2011.02666.x
Price, P. P., and Harrison, P. J. (1987). Comparison of methods for the analysis of dissolved urea in seawater. Mar. Biol. 94, 307–317. doi: 10.1007/BF00392945
Prosser, J., and Nicol, G. (2008). Relative contributions of Archaea and bacteria to aerobic ammonia oxidation in the environment. Environ. Microbiol. 10, 2931–2941. doi: 10.1111/j.1462-2920.2008.01775.x
Purkhold, U., Pommerening-Röser, A., Juretschko, S., Schmid, M. C., Koops, H.-P., and Wagner, M. (2000). Phylogeny of all recognized species of ammonia oxidizers based on comparative 16S rRNA and amoA sequence analysis: implications for molecular diversity surveys. Appl. Environ. Microbiol. 66, 5368–5382. doi: 10.1128/aem.66.12.5368-5382.2000
R Core Team (2017). R: A Language and Environment for Statistical Computing. Vienna: R Foundation for Statistical Computing.
Rotthauwe, J. H., Witzel, K. P., and Liesack, W. (1997). The ammonia monooxygenase structural gene amoA as a functional marker: molecular fine-scale analysis of natural ammonia-oxidizing populations. Appl. Environ. Microbiol. 63, 4704–4712.
Sahan, E., and Muyzer, G. (2008). Diversity and spatio-temporal distribution of ammonia-oxidizing Archaea and Bacteria in sediments of the Westerschelde estuary. FEMS Microbiol. Ecol. 64, 175–186. doi: 10.1111/j.1574-6941.2008.00462.x
Schloss, P. D., Westcott, S. L., Ryabin, T., Hall, J. R., Hartmann, M., Hollister, E. B., et al. (2009). Introducing mothur: open-source, platform-independent, community-supported software for describing and comparing microbial communities. Appl. Environ. Microbiol. 75, 7537–7541. doi: 10.1128/AEM.01541-09
Song, H., Li, Z., Du, B., Wang, G., and Ding, Y. (2012). Bacterial communities in sediments of the shallow Lake Dongping in China. J. Appl. Microbiol. 112, 79–89. doi: 10.1111/j.1365-2672.2011.05187.x
Sun, W., Xia, C., Xu, M., Guo, J., Wang, A., and Sun, G. (2013). Distribution and abundance of Archaeal and bacterial ammonia oxidizers in the sediments of the Dongjiang River, a drinking water supply for Hong Kong. Microbes Environ. 28, 457–465. doi: 10.1264/jsme2.ME13066
Tabatabai, M. A., and Bremner, J. M. (1970). Arylsulfatase activity of soils. Proc. Soil Sci. Am. 34, 225–229. doi: 10.2136/sssaj1970.03615995003400020016x
Tago, K., Okubo, T., Shimomura, Y., Kikuchi, Y., Hori, T., Nagayama, A., et al. (2015). Environmental factors shaping the community structure of ammonia-oxidizing bacteria and Archaea in sugarcane field soil. Microbes Environ. 30, 21–28. doi: 10.1264/jsme2.ME14137
Teske, A., Alm, E., Regan, J. M., Toze, S., Rittmann, B. E., and Stahl, D. A. (1994). Evolutionary relationships among ammonia- and nitrite-oxidizing bacteria. J. Bacteriol. 176, 6623–6630. doi: 10.1128/jb.176.21.6623-6630.1994
Therkildsen, M. S., King, G. M., and Lomstein, B. A. (1996). Urea production and turnover following the addition of AMP, CMP, RNA and a protein mixture to a marine sediment. Aquat. Microb. Ecol. 10, 173–179. doi: 10.3354/ame010173
Treusch, A. H., Leininger, S., Kletzin, A., Schuster, S. C., Klenk, H. P., and Schleper, C. (2005). Novel genes for nitrite reductase and Amo-related proteins indicate a role of uncultivated mesophilic crenarchaeota in nitrogen cycling. Environ. Microbiol. 7, 1985–1995. doi: 10.1111/j.1462-2920.2005.00906.x
van Kessel, M. A., Speth, D. R., Albertsen, M., Nielsen, P. H., Op den Camp, H. J. M., et al. (2015). Complete nitrification by a single microorganism. Nature 528, 555–559. doi: 10.1038/nature16459
Vance, E. D., Brookes, P. C., and Jenkinson, D. S. (1987). An extraction method for measuring soil microbial biomass C. Soil Biol. Biochem. 19, 703–707. doi: 10.1016/0038-0717(87)90052-6
Venter, J. C., Remington, K., Heidelberg, J. F., Halpern, A. L., Rusch, D., Eisen, J. A., et al. (2004). Environmental genome shotgun sequencing of the Sargasso Sea. Science 304, 66–74. doi: 10.1126/science.1093857
Veuger, B., Pitcher, A., Schouten, S., Sinninghe Damsté, J. S., and Middelburg, J. J. (2013). Nitrification and growth of autotrophic nitrifying bacteria and Thaumarchaeota in the coastal North Sea. Biogeosciences 10, 1775–1785. doi: 10.5194/bg-10-1775-2013
Vlaeminck, S. E., Hay, A. G., Maignien, L., and Verstraete, W. (2011). In quest of the nitrogen oxidizing prokaryotes of the early Earth. Environ. Microbiol. 13, 283–295. doi: 10.1111/j.1462-2920.2010.02345.x
Wang, J., Wang, W., and Gu, J. D. (2014). Community structure and abundance of ammonia-oxidizing Archaea and bacteria after conversion from soybean to rice paddy in albic soils of Northeast China. Appl. Microbiol. Biotechnol. 98, 2765–2778. doi: 10.1007/s00253-013-5213-2
Wang, X., Wang, C., Bao, L., and Xie, S. (2014). Abundance and community structure of ammonia-oxidizing microorganisms in reservoir sediment and adjacent soils. Appl. Microbiol. Biotechnol. 98, 1883–1892. doi: 10.1007/s00253-013-5174-5
Wang, Q. D., Cheng, L., Liu, J. S., Li, Z. J., Xie, S. Q., and De Silva, S. S. (2015). Freshwater aquaculture in PR China: trends and prospects. Rev. Aquac. 7, 283–302. doi: 10.1111/raq.12086
Ward, B. (2011). Measurement and distribution of nitrification rates in the ocean. Methods Enzymol. 486, 307–323. doi: 10.1016/B978-0-12-381294-0.00013-4
Wickham, H. (2009). ggplot2: Elegant Graphics for Data Analysis. New York, NY: Springer-Verlag. doi: 10.1007/978-0-387-98141-3
Wu, R. N., Meng, H., Wang, Y. F., Lan, W., and Gu, J. D. (2017). A more comprehensive community of ammonia-oxidizing Archaea (AOA) revealed by genomic DNA and RNA analyses of amoA gene in subtropical acidic forest soils. Microb. Ecol. 74, 910–922. doi: 10.1007/s00248-017-1045-4
Wu, Y., Xiang, Y., Wang, J., Zhong, J., He, J., and Wu, Q. L. (2010). Heterogeneity of Archaeal and bacterial ammonia-oxidizing communities in Lake Taihu, China. Environ. Microbiol. Rep. 2, 569–576. doi: 10.1111/j.1758-2229.2010.00146.x
Yang, Y., Shan, J., Zhang, J., Zhang, X., Xie, S., and Liu, Y. (2014). Ammonia- and methane-oxidizing microorganisms in high-altitude wetland sediments and adjacent agricultural soils. Appl. Microbiol. Biotechnol. 98, 10197–10209. doi: 10.1007/s00253-014-5942-x
Yang, Y., Zhang, J., Zhao, Q., Zhou, Q., Li, N., Wang, Y., et al. (2016). Sediment ammonia-oxidizing microorganisms in two plateau freshwater lakes at different trophic states. Microb. Ecol. 71, 257–265. doi: 10.1007/s00248-015-0642-3
Zheng, Y., Hou, L., Liu, M., Lu, M., Zhao, H., Yin, G., et al. (2013). Diversity, abundance, and activity of ammonia-oxidizing bacteria and Archaea in Chongming eastern intertidal sediments. Appl. Microbiol. Biotechnol. 97, 8351–8363. doi: 10.1007/s00253-012-4512-3
Zhou, Z., Li, H., Song, C., Cao, X., and Zhou, Y. (2017). Prevalence of ammonia-oxidizing bacteria over ammonia-oxidizing Archaea in sediments as related to nutrient loading in Chinese aquaculture ponds. J. Soils Sediments 17, 1928–1938. doi: 10.1007/s11368-017-1651-2
Keywords: ammonia-oxidizing bacteria, ammonia-oxidizing archaea, Ctenopharyngodon idellus, aquaculture pond, sediment, organic matter
Citation: Dai L, Liu C, Yu L, Song C, Peng L, Li X, Tao L and Li G (2018) Organic Matter Regulates Ammonia-Oxidizing Bacterial and Archaeal Communities in the Surface Sediments of Ctenopharyngodon idellus Aquaculture Ponds. Front. Microbiol. 9:2290. doi: 10.3389/fmicb.2018.02290
Received: 28 May 2018; Accepted: 07 September 2018;
Published: 24 September 2018.
Edited by:
Dennis A. Bazylinski, University of Nevada, Las Vegas, United StatesReviewed by:
Willm Martens-Habbena, University of Florida, United StatesWei Xie, Tongji University, China
Copyright © 2018 Dai, Liu, Yu, Song, Peng, Li, Tao and Li. This is an open-access article distributed under the terms of the Creative Commons Attribution License (CC BY). The use, distribution or reproduction in other forums is permitted, provided the original author(s) and the copyright owner(s) are credited and that the original publication in this journal is cited, in accordance with accepted academic practice. No use, distribution or reproduction is permitted which does not comply with these terms.
*Correspondence: Gu Li, bGlndUB5ZmkuYWMuY24=