- College of Veterinary Medicine, Key Laboratory of Zoonosis of Ministry of Agricultural and Rural Affairs, South China Agricultural University, Guangzhou, China
The purpose of this study was to investigate the occurrence of plasmid-mediated colistin resistance gene mcr-1 in Enterobacteriaceae isolates from companion animals in Guangzhou, China. Enterobacteriaceae isolated from 180 samples collected from cats and dogs were screened for mcr-1 by PCR and sequencing. MCR-1-producing isolates were further characterized by multilocus sequence typing and pulsed-field gel electrophoresis (PFGE). Plasmid characterization was performed by conjugation, replicon typing, S1-PFGE, and Southern blot hybridization. Plasmid pHN6DS2 as a representative IncN1-IncHI2/ST3 plasmid from ST93 E. coli was fully sequenced. pHN6DS2-like plasmids were screened by PCR-mapping and sequencing. The mcr-1 gene was detected in 6.25% (8/128) Escherichia coli isolates, of which, five belonged to E. coli ST93 and had identical PFGE patterns, resistance profiles and resistance genes. mcr-1 genes were located on ∼244.4 kb plasmids (n = 6), ∼70 kb plasmids, and ∼60 kb plasmids, respectively. Among them, five mcr-1-carrying plasmids were successfully transferred to recipient by conjugation experiments, and were classified as IncN1-IncHI2/ST3 (∼244.4 kb, n = 4, all obtained from E. coli ST93), and IncI2 (∼70 kb, n = 1), respectively. Plasmid pHN6DS2 contained a typical IncHI2-type backbone, with IncN1 segment (ΔrepA-Iterons I-gshB-ΔIS1294) inserted into the multiresistance region, and was similar to other mcr-1-carrying IncHI2/ST3 plasmids from Enterobacteriaceae isolates of various origins in China. The remaining five mcr-1-bearing plasmids with sizes of ∼244.4 kb were identified to be pHN6DS2-like plasmids. In conclusion, clonal spread of ST93 E. coli isolates was occurred in companion animals in Guangzhou, China.
Introduction
Colistin has been a last-resort treatment option in human medicine for infections caused by multi-resistant Gram-negative bacteria (Kaye et al., 2016). Resistance to colistin had only been involved with chromosomal mutations until the identification of plasmid-mediated colistin resistance gene mcr-1 from a porcine Escherichia coli isolate in China in 2015 (Liu et al., 2016). The emergence and dissemination of mcr-1 is a significant global concern and poses a serious threat to clinical treatment. Since the discovery of mcr-1, it has been increasingly reported in Enterobacteriaceae from humans, animals, food products, and the environment worldwide, particularly in China (Jeannot et al., 2017; Wang et al., 2018). To date, mcr-1 has been identified on various plasmid types, with IncI2, IncHI2, and IncX4 being the major carriers, and ISApl1 is involved in mcr-1 mobilization between DNA molecules (e.g., plasmid, chromosome) (Matamoros et al., 2017; Li et al., 2018; Wang et al., 2018). Previous studies have demonstrated high mcr-1 prevalence in E. coli isolates from food-producing animals (pigs and chickens) and meat (pork and chicken meat) in China (Liu et al., 2016, 2017; Wu et al., 2018). However, mcr-1 has been rarely reported in companion animals, though 8.7% Enterobacteriaceae isolates were identified to carry mcr-1 among companion animals in Beijing, China, meanwhile, mcr-1-positive E. coli isolates may transfer between companion animals and close contactors in a pet store in Guangzhou, China (Sun et al., 2016; Zhang et al., 2016; Lei et al., 2017). Thus, in this study, we investigated the prevalence and characterization of mcr-1 in Enterobacteriaceae isolates from companion animals in Guangzhou, China, to provide insights into the spread of mcr-1 in companion animals.
Materials and Methods
Sample Collection and mcr-1 Detection
During July to August 2016, 180 samples were collected from cats and dogs at four animal hospitals located in four districts in Guangzhou, China, including 68 feces samples from healthy animals, 112 samples (feces, urine, eye secretion, ear exudates, nasal secretion, and skin) from diseased animals (Table 1). Samples were incubated in LB broth for 16∼24 h and then inoculated on the MacConkey agar. One isolate per sample was selected and identified by MALDI-TOF mass spectrometry or 16S rRNA sequencing (Supplementary Table S1). In all isolates, mcr-1 was detected by PCR and sequencing (Supplementary Table S1).
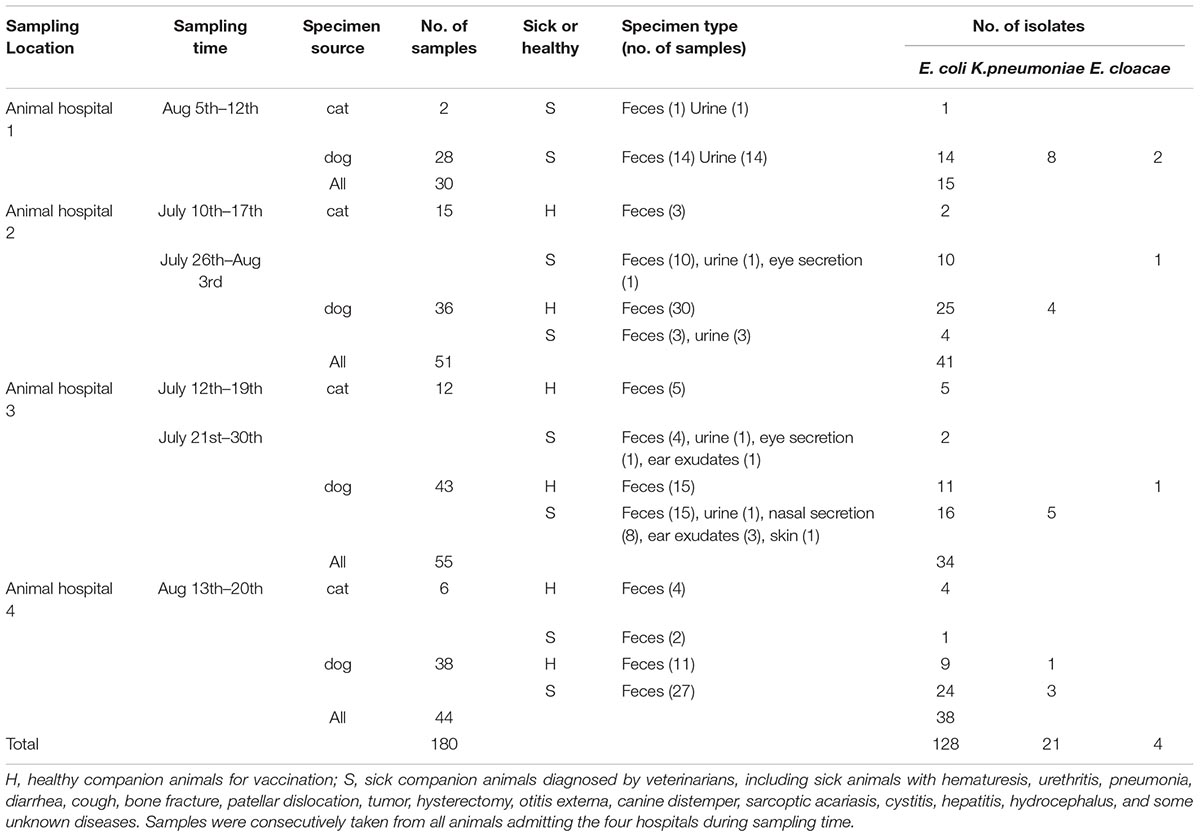
TABLE 1. Source and origin of Enterobacteriaceae isolates obtained from companion animals in Guangzhou, China.
Molecular Typing
The genetic diversity of mcr-1-positive E. coli isolates was characterized by multilocus sequence typing (MLST)1. Five mcr-1-carrying ST93 E. coli isolates in this study and the mcr-1-positive ST93 E. coli strain PET01, that was previously obtained from a cat in Guangzhou, China (Zhang et al., 2016) were further analyzed by pulsed-field gel electrophoresis (PFGE) (Gautom, 1997).
Conjugation/Transformation Experiments and Plasmid Characterization
Conjugation experiments were carried out using streptomycin-resistant E. coli C600 as the recipient strain as previously described (Chen et al., 2007). Transconjugants were selected using 2 mg/L colistin and 3,000 mg/L streptomycin. Transfer frequencies were calculated as the number of transconjugants per recipient, experiments were performed in triplicate. Transformation was conducted by heat-shock and electroporation using E. coli strain DH5α as the recipient strain, and selected by 2 mg/L colistin. The presence of mcr-1 in the transconjugants was confirmed by PCR and sequencing. Transconjugants with a single mcr-1-carrying plasmid, verified by S1-PFGE (Barton et al., 1995) and Southern blot hybridization, were selected for further study. The location of mcr-1 in the original isolates which failed to obtain transconjugants/transformants was determined by S1-PFGE and Southern blot hybridization. All the transconjugants were characterized by PCR-based replicon typing and IncI2 and IncX4 plasmids were screened according to previously described protocols (Carattoli et al., 2005; Johnson et al., 2012; Lv et al., 2013). IncHI2 plasmids were further characterized by plasmid double locus sequence typing (García-Fernández and Carattoli, 2010). The genetic structure of mcr-1 was determined by PCR mapping and sequencing in five transconjugants and three original isolates without transconjugants/transformants (Supplementary Table S2).
Antimicrobial Susceptibility Testing
The original mcr-1-positive E. coli isolates, the recipient strain C600, and transconjugants were tested for their susceptibility to ampicillin, cefotaxime, imipenem, gentamycin, amikacin, tetracycline, chloramphenicol, florfenicol, ciprofloxacin, sulfamethoxazole/trimethoprim, colistin, and fosfomycin by the agar dilution method or the broth microdilution method (limited to colistin). Antimicrobial susceptibility tests were performed and interpreted according to M100, 28th edition of the CLSI (Wayne, PA, United States) (Clinical Laboratory Standards Institute [CLSI], 2018). Colistin ( > 2 mg/L), and florfenicol ( > 16 mg/L) were interpreted according to the clinical breakpoints or epidemiological cutoff values of EUCAST.2 The E. coli strain ATCC 25922 was used for quality control. The mutations within gyrA and parC were detected in ciprofloxacin-resistant mcr-1-positive E. coli isolates (Supplementary Table S1). Other resistance genes, including blaCTX-M, floR, rmtB, oqxAB, and fosA3 were screened in original mcr-1-positive isolates and their transconjugants using the primers listed in Supplementary Table S1.
Plasmid Sequencing
Plasmid pHN6DS2, as a representative IncN1-IncHI2/ST3 plasmid from ST93 E. coli isolate, was selected to extract from the transconjugant using QIAGEN® Plasmid Midi Kit (Qiagen, Hilden, Germany) and sequenced by Illumina Miseq technology (Illumina, San Diego, CA, United States). Sequence reads were assembled into contigs with SOAPdenovo version 2.04. Nine contigs of pHN6DS2 were assembled into the complete plasmid sequence with PCR amplification and Sanger sequencing (Supplementary Table S3) using related mcr-1-carrying plasmids as references by BLAST3. Analysis and annotation of plasmid pHN6DS2 were performed using the RAST server (Aziz et al., 2008), ISfinder4, ResFinder5, RAC6, BLAST7, and the Gene Construction Kit 4.5 (Textco BioSoftware, Inc., Raleigh, NC, United States). The remaining transconjugants or original isolates containing ∼244.4 kb mcr-1-bearing plasmid were examined for pHN6DS2-like plasmids by PCR and sequencing (Supplementary Table S4).
Nucleotide Sequence Accession Number
The nucleotide sequences of plasmid pHN6DS2 has been deposited in the GenBank database under the accession number MH459020.
Results and Discussion
Identification of mcr-1 and Antimicrobial Susceptibility
A total of 128 E. coli, 21 Klebsiella pneumoniae, and 4 Enterobacter cloacae isolates were obtained from 180 samples of companion animals origin (Table 1). Among them, mcr-1 was present in eight (6.25%) E. coli isolates, two from healthy animals and six from diseased animals (Table 2). The isolates from diseased animals (6/72, 8.33%) showed higher mcr-1 prevalence than those from healthy animals (2/56, 3.57%; P > 0.05). However, we did not identify mcr-1 in K. pneumoniae or E. cloacae isolates. Although mcr-1 prevalence in companion animals was greatly lower than that among food-producing animals in China (Liu et al., 2017; Wu et al., 2018), it was similar to the previously described mcr-1 detection in companion animals in Beijing, China (Lei et al., 2017).
As shown in Table 1, all mcr-1-positive strains exhibited minimal inhibitory concentration (MIC) of 4 mg/L to colistin, and showed resistance to ampicillin, tetracycline, sulfamethoxazole/trimethoprim, and fosfomycin, but susceptibility to amikacin and imipenem; seven displayed resistance to gentamycin, chloramphenicol and florfenicol. The mcr-1-positive isolates also harbored other resistance genes, including blaCTX-M (n = 8), fosA3 (n = 8), floR (n = 7), and oqxAB (n = 2) (Table 2). In addition, seven mcr-1-bearing isolates exhibited resistance to ciprofloxacin with mutations in gyrA (S83L and D87Y) and parC (S57T and/or S80I) (Table 2).
Since colistin is not applied to companion animals in China, pet food containing chicken meat might be one source of mcr-1 (Lei et al., 2017). Close contact to food-producing animals in local hog and poultry markets, as well as to humans, might also be the potential origins. Furthermore, the widely use of cephalosporins, aminoglycosides, and fluoroquinolones in companion animal medicine (data not shown) could allow for the co-selection of isolates harboring mcr-1, as well as blaCTX-M and fosA3, conferring resistance to crucial clinical antibiotics.
Molecular Typing
Eight mcr-1-positive E. coli isolates were assigned to ST93 (n = 5), ST1011, ST3285, and a new ST, respectively (Table 2). ST93 has been sporadically described as avian and human extra-intestinal pathogenic or diarrhoeagenic E. coli in humans, animals, and food products worldwide (Chen et al., 2014; Maluta et al., 2014; Vogt et al., 2014), and particularly it has been previously detected as mcr-1 carriers from a pig in Laos (Olaitan et al., 2015, 2016), from a cat in Guangzhou, China (Zhang et al., 2016), and from a patient in Finland (Gröndahl-Yli-Hannuksela et al., 2018). The five mcr-1-carrying ST93 E. coli isolates were obtained from both intestinal and extraintestinal sites from two animal hospitals located within a distance of 7 km. They showed indistinguishable PFGE patterns which differed from previously described mcr-1-harboring ST93 E. coli isolate PET01 from a cat in Guangzhou (Zhang et al., 2016; Figure 1), indicating that clonal spread of mcr-1-harboring E. coli had occurred among companion animals within two hospitals in Guangzhou. The observation that they had identical antimicrobial susceptibility profiles, resistance genes, and mutations within gyrA and parC may further support this hypothesis (Table 2). However, small numbers of samples were collected from four animal hospitals in this study, thus limiting this hypothesis. The prevalence and dissemination mechanisms of mcr-1 in companion animals in Guangzhou should be further investigated by using large scale samples from more animal hospitals. Though rare, it is possible for these two hospitals to exchange animal patients. The possibility of acquisition of mcr-1-harboring ST93 E. coli from a common ancestor could not be ruled out. Although horizontal transfer mediated by mobile elements such as insertion sequence and plasmids has been the major reason for mcr-1 worldwide dissemination, clonal spread of mcr-1-harboring strains, such as E. coli ST93 in the present study, Salmonella Typhimurium ST34 in pigs (Li et al., 2016; Yi et al., 2017) might be another reason accounting for mcr-1 transmission. Most importantly, the potential of mcr-1 transmission mediated by MCR-1-producing clones from companion animals to humans through close contact should not be underestimated, which might have already occurred in China by E. coli ST354 and ST101 clones (Zhang et al., 2016; Lei et al., 2017).
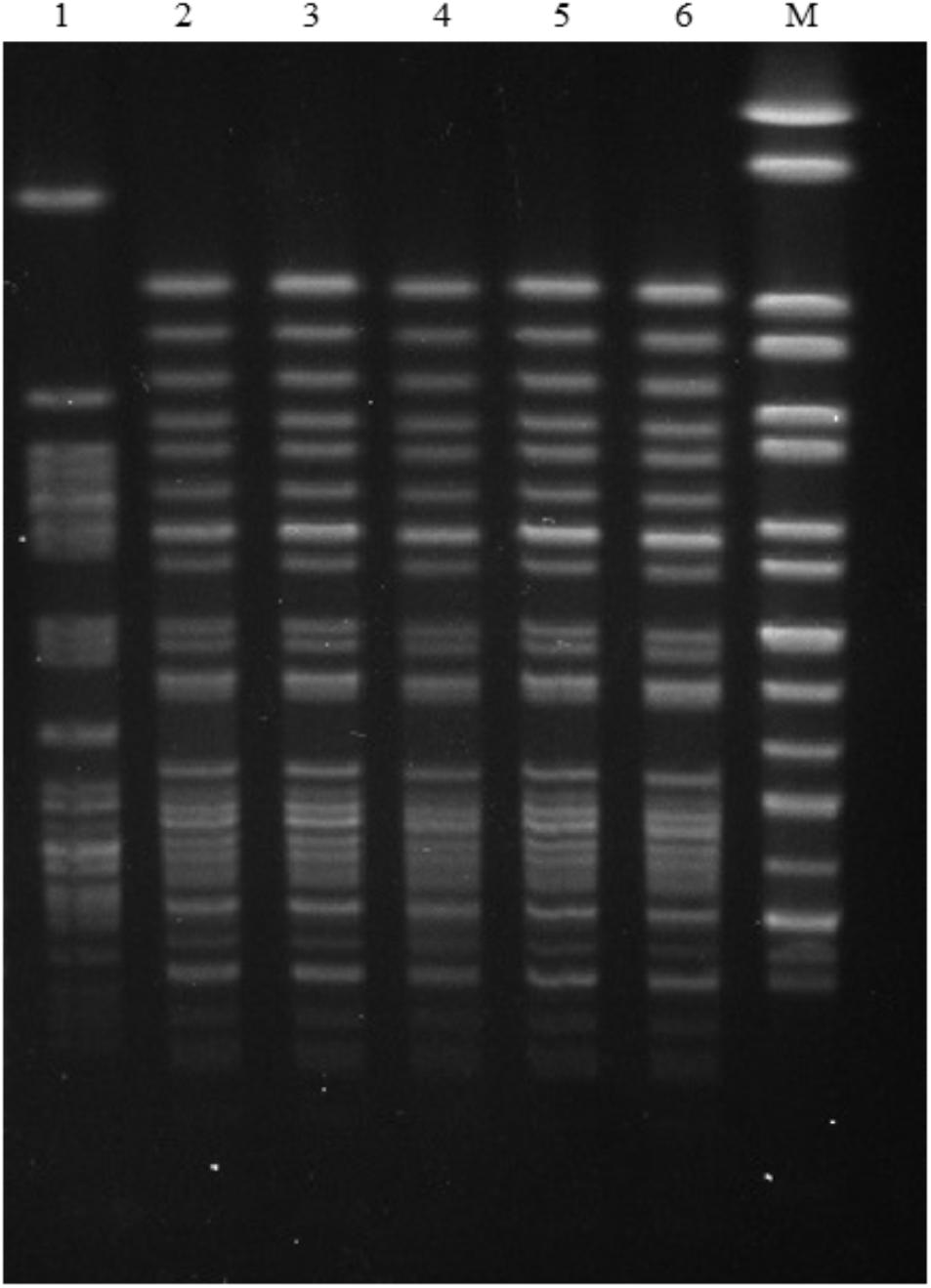
FIGURE 1. PFGE patterns of five mcr-1-carrying E. coli ST93 strains in this study and the mcr-1- carrying E. coli ST93 strain PET01 that was isolated from a cat in a pet shop from Guangzhou (Zhang et al., 2016). Lanes: (1) PET01; (2) GZ6DS2; (3) GZ6DS9; (4) GZ6CS9; (5) GZ6DH17; (6) GZ6DH18; (9) GZ6DS69; (10) GZ6DS68; M Salmonella enterica serovar Braenderup H9812 Marker.
Characterization of mcr-1-Carrying Plasmids
Five strains successfully transferred mcr-1 to E. coli C600 at frequencies of 10-2 to 10-4 transconjugants/recipient (Supplementary Table S5), and the remaining three strains failed to transfer mcr-1 to E. coli C600 or DH5α by conjugation or transformation. S1-PFGE and Southern hybridization indicated that mcr-1 was located on ∼244.4 kb plasmids (n = 6), ∼60 kb plasmids (n = 1), or ∼70 kb plasmid (n = 1) (Table 2). Additionally, five mcr-1-harboring transconjugants with single plasmid were classified as IncN1-IncHI2/ST3 (∼244.4 kb, n = 4) which were all obtained from ST93 E. coli isolates, and IncI2 (∼70 kb, n = 1) (Table 2 and Supplementary Figure S1), which agree with previous observation that IncHI2 and IncI2 plasmids have been the major vectors for mcr-1 global dissemination (Matamoros et al., 2017; Wang et al., 2018). Furthermore, the transconjugants showed elevated MICs for colistin (1∼2 mg/L; 8-16-fold) compared with the recipient E. coli C600. In addition, co-transfer of resistance to ampicillin, cefotaxime, gentamycin, chloramphenicol, florfenciol, sulfamethoxazole/trimethoprim, and fosfomycin was observed in four transconjugants with IncN1-IncHI2/ST3 plasmid from ST93 E. coli isolates, resistance genes blaCTX-M-14, floR and fosA3 were also co-transferred with mcr-1 (Table 2). The co-transfer of blaCTX-M-64 with mcr-1 on an IncI2 plasmid in the remaining transconjugant caused resistance to ampicillin and cefotaxime (Table 2). The presence of other resistance genes co-located on the same plasmid allows for the selection of mcr-1 under pressure posed by other agents, thus facilitating mcr-1 transmission.
It has been hypothesized that mcr-1 is initially captured and mobilized by the composite transposon Tn6330 (ISApl1-mcr-1-pap2-ISApl1), followed by the loss of ISApl1 over time, leading to the formation of mcr-1 in diverse genetic structures, with the structure mcr-1-pap2 being dominant, followed by the structure ISApl1-mcr-1-pap2 (Snesrud et al., 2018; Wang et al., 2018). The genetic structure of mcr-1 in our study was determined by PCR mapping. We did not observe the complete Tn6330, but the presence of ISApl1 upstream was common, identified in six transconjugants or original isolates with ∼244.4 kb mcr-1-carrying plasmids, the structure mcr-1-pap2 was also identified (n = 2) (Table 2). Our results further support that mobile elements (ISApl1, IncHI2, and IncI2 plasmids) play an important role in the mobilization and dissemination of mcr-1 in E. coli from different sources.
Plasmid Sequencing and Comparative Analysis
Plasmid pHN6DS2 had a size of 253, 783 bp, and was organized similarly to other IncHI2 plasmids, containing regions for functions of replication, multiresistance, conjugal transfer, maintenance, and stability (Supplementary Figure S2).
Interestingly, a fragment with the least size of 37, 258-bp including the module. ISApl1-mcr-1-pap2 and a set of tellurite resistance determinants (terYXWZABCDEF) in plasmid pHN6DS2 from canine E. coli was similar to several other IncHI2/ST3 plasmids found in Enterobacteriaceae isolates from various sources in China, such as plasmids pHNTS53-1 (Raoultella ornithinolytica, lettuce, MF135535), pHSHLJ1-MCR1 (S. Typhimurium, human, KX856066), pMCR_WCHEC1613 (E. coli, environment, CP019214) (Zhao et al., 2017), and pASSD2-MCR1 (S. Typhimurium, pig, KX856065) (Figure 2). However, ISApl1 was absent and IS1 was inserted upstream of mcr-1 flanked by 9-bp direct repeats (DRs) in plasmid pASSD2-MCR1 (Figure 2). Furthermore, the fragment was also identical to the corresponding region of the IncHI2-IncF recombinant plasmid pMR0516mcr obtained from clinical E. coli isolate in the United States (McGann et al., 2016; Figure 2). However, the module ISApl1-mcr-1-pap2 was inserted into the backbone of plasmid pHNSHP45-2 (Zhi et al., 2016) with different location and orientation (Figure 2).
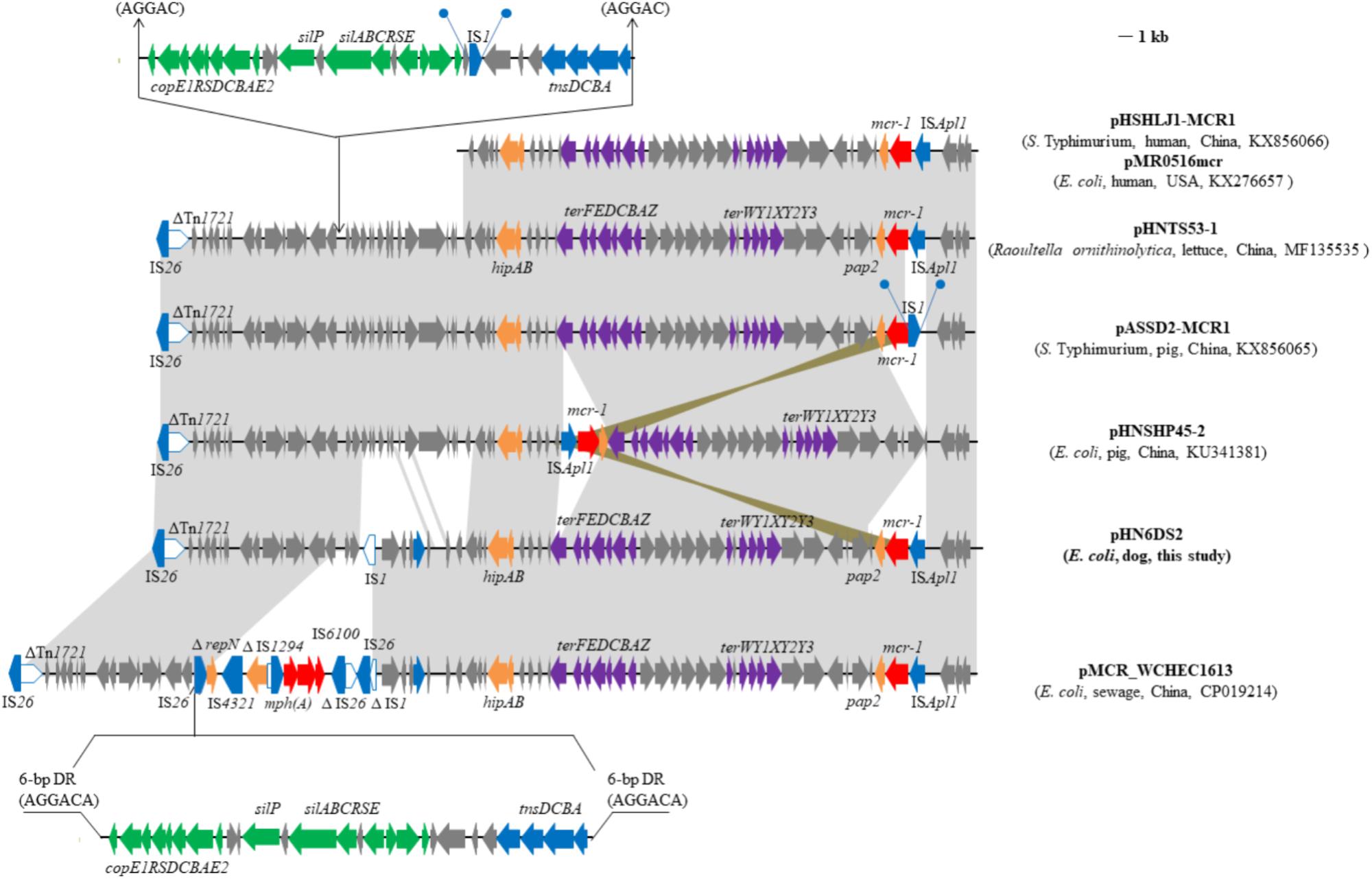
FIGURE 2. Genetic organization of the partial sequence containing mcr-1 of plasmid pHN6DS2, and structural comparison with other mcr-1-carrying plasmids. Arrows indicate the positions of the genes and the direction. Regions with > 99% homology are shaded in gray or azure. Δ indicates a truncated gene or mobile element. Direct repeats are indicated by arrows and blue circles. Tall bars represent the 38 bp inverted repeat (IR) of transposons. The backbone is indicated by dotted lines.
Furthermore, the multiresistance region (MRR) of pHN6DS2 contained numerous resistance genes, such as aphA1, tetM, sul3, aadA1, cmlA1, aadA2, floR, blaCTX-M-14, and fosA3, and complete or truncated insertion sequences and transposons (e.g., IS26, Tn21, IS4321, IS1006, ISCR2, ISAba1, Tn5393, ISEc59, ISEcp1, IS903, and Tn1721) (Figure 3). As a multi-replicon plasmid, pHN6DS2 harbored an approximately 3-kb IncN1 segment (ΔrepA-Iterons I-gshB-ΔIS1294), containing IncN replication initiation gene repA truncated by IS26, five tandem 37-bp repeats within iterons region, gshB encoding glutathione synthetase, and 114 bp of the oriIS end of IS1294 (Figure 3). The similar structure was also observed in plasmid pASSD2-MCR1 with the exception of IS4321 insertion (Figure 3). The macrolide phosphotransferase region harboring several mph genes was located downstream of the IncN segment, and was followed by IS6100, 123-bp of Tn402, incomplete Tn21-like transposon TnchrA, and a 2, 067-bp segment containing tetracycline resistance gene tetM (Figure 3). IS26 was inserted in inverted repeat at the tni end of Tn402, named IRt, flanked by 8-bp DRs (Figure 3).
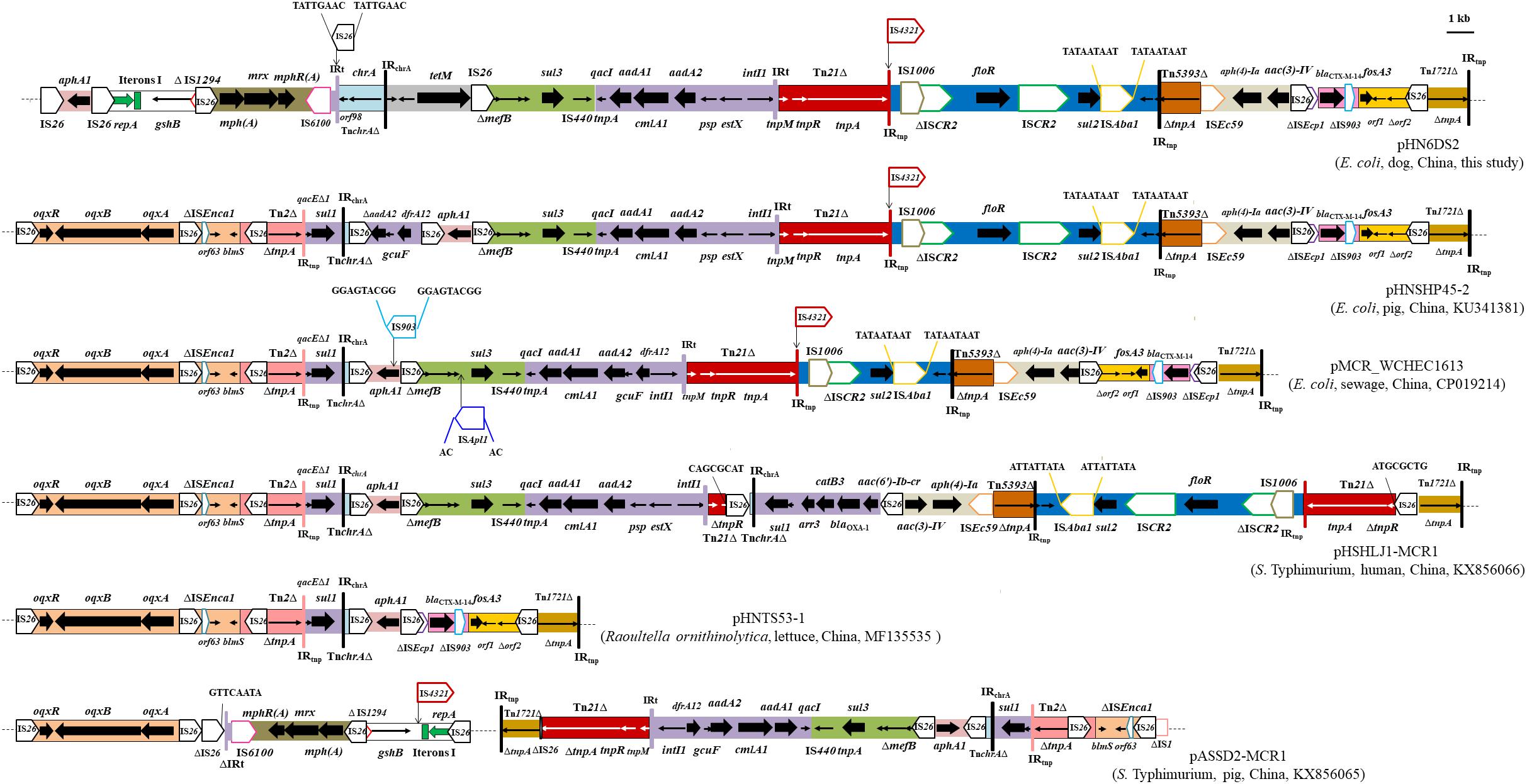
FIGURE 3. Genetic organization of the multiresistance region of plasmid pHN6DS2, and structural comparison with other mcr-1-carrying plasmids. The extents and directions of antibiotic resistance (thick arrows) and other genes are indicated. Δ indicates a truncated gene or mobile element. ISs are shown as boxes labeled with their name. Labeled vertical arrows with IS boxes indicate the insertion sites of IS elements. Direct repeats are indicated by arrows and sequence. Tall bars represent the 38 bp inverted repeat (IR) of transposons. The backbone is indicated by dotted lines.
The MRR of pHN6DS2 was similar to that of pHNSHP45-2, but differed by acquisition of the ∼10.5-kb oqxAB resistance module (Tn6010-ΔISEnca1-orf63-blmS-ΔTn2-IS26-ΔTn2) and an ∼4.3-kb segment harboring the |qacEΔ1|sul1| ΔaadA2|gcuF|dfrA12| cassette array which was interrupted by partial TnchrA and IS26, and by loss of the IncN segment, mph region, and tetM region (Figure 3). Similarly, MRRs of mcr-1-carrying plasmids pMCR_WCHEC1613, pHSHLJ1-MCR1, pHNTS53-1, and pASSD2-MCR1 were related to those of pHN6DS2 and pHNSHP45-2, differed by insertions, deletions, or rearrangement of various regions harboring antimicrobial resistance genes such as oqxAB, sul3, floR, blaCTX-M-14, and fosA3, and mobile element such as Tn21, ISCR2, IS4321 (Figure 3). Notably, IS26, via transposition and homologous recombination, seems to play an important role in the formation of distinct but also related MRRs. The remaining three transconjugants carrying IncN1-IncHI2/ST3 plasmids and two original isolates with ∼244.4 kb mcr-1-bearing plasmids were also examined for pHN6DS2-like plasmids. All five transconjugants or original isolates harbored pHN6DS2-like plasmids with identical insertion of the module ISApl1-mcr-1-pap2 but variable MRRs (Table 3).
These data suggested that similar mcr-1-carrying IncHI2/ST3 plasmids, after acquiring, losing or reorganizing various regions, could spread among Enterobacteriaceae species in livestock, humans, vegetables, and the environment, particularly in different regions in China. The presence of pHN6DS2-like plasmids further supported this hypothesis and highlighted the potential of pHN6DS2-like plasmid to become an efficient vehicle for mcr-1 dissemination between distinct organisms or regions.
Conclusion
In conclusion, the spread of mcr-1 in companion animals in the present study might be mainly attributed to clonal dissemination of E. coli ST93 isolates within two hospitals, associated with IncN1-IncHI2/ST3 plasmids. Although the origin of mcr-1 in companion animals is unclear, it is possible for mcr-1-positive clones or plasmids to transfer from companion animals to humans through close contact, thus the dissemination of mcr-1 among companion animals needs continued vigilance.
Ethics Statement
This study was carried out in accordance with the recommendation of ethical guidelines of South China Agricultural University. Individual written informed consent for the use of samples was obtained from all the animal owners.
Author Contributions
J-HL, Z-LZ, and JW conceived the study. X-YH, Y-BX, JW, Z-WG, Z-BM, M-YY, L-CL, P-LL, J-CY, and J-WH carried out the experiments. JW, X-YH, and Y-BX analyzed the data. JW wrote the manuscript. J-HL and Z-LZ revised the manuscript. All authors read and approved the final manuscript.
Funding
This work was supported in part by the grants from National Natural Science Foundation of China (NSFC) (31625026 and 31830099), National Key Basic Research Program of China (No. 2013CB127200), and the National Training Program of Innovation and Entrepreneurship for Undergraduates (No. 201710564202).
Conflict of Interest Statement
The authors declare that the research was conducted in the absence of any commercial or financial relationships that could be construed as a potential conflict of interest.
Acknowledgments
We thank Jia Long, Yang He, Jun-Jie Weng, and Zhuo-Wen Lu for assistance in sample collection from companion animals. We also thank Guo-Bao Tian for providing ST93 E. coli strain PET01.
Supplementary Material
The Supplementary Material for this article can be found online at: https://www.frontiersin.org/articles/10.3389/fmicb.2018.02989/full#supplementary-material
Footnotes
- ^ http://enterobase.warwick.ac.uk/species/index/ecoli
- ^ https://mic.eucast.org/Eucast2/
- ^ http://blast.ncbi.nlm.nih.gov/Blast.cgi
- ^ https://www-is.biotoul.fr//
- ^ https://cge.cbs.dtu.dk//services/ResFinder/
- ^ https://galileoamr.arcbio.com/mara/
- ^ http://blast.ncbi.nlm.nih.gov/Blast.cgi
References
Aziz, R. K., Bartels, D., Best, A. A., DeJongh, M., Disz, T., Edwards, R. A., et al. (2008). The RAST server: rapid annotations using subsystems technology. BMC Genomics 9:75. doi: 10.1186/1471-2164-9-75
Barton, B. M., Harding, G. P., and Zuccarelli, A. J. (1995). A general method for detecting and sizing large plasmids. Anal. Biochem. 226, 235–240. doi: 10.1006/abio.1995.1220
Carattoli, A., Bertini, A., Villa, L., Falbo, V., Hopkins, K. L., and Threlfall, E. J. (2005). Identification of plasmids by PCR-based replicon typing. J. Microbiol. Methods 63, 219–228. doi: 10.1016/j.mimet.2005.03.018
Chen, L., Chen, Z. L., Liu, J. H., Zeng, Z. L., Ma, J. Y., and Jiang, H. X. (2007). Emergence of RmtB methylase-producing Escherichia coli and Enterobacter cloacae isolates from pigs in China. J. Antimicrob. Chemother. 59, 880–885. doi: 10.1093/jac/dkm065
Chen, Y., Chen, X., Zheng, S., Yu, F., Kong, H., Yang, Q., et al. (2014). Serotypes, genotypes and antimicrobial resistance patterns of human diarrhoeagenic Escherichia coli isolates circulating in southeastern China. Clin. Microbiol. Infect. 20, 52–58. doi: 10.1111/1469-0691.12188
Clinical Laboratory Standards Institute [CLSI] (2018). Performance Standards for Antimicrobial Susceptibility Testing [S]. CLSI Supplement M100, 28th Edn. Wayne, PA: Clinical and Laboratory Standards Institute.
García-Fernández, A., and Carattoli, A. (2010). Plasmid double locus sequence typing for IncHI2 plasmids, a subtyping scheme for the characterization of IncHI2 plasmids carrying extended-spectrum beta-lactamase and quinolone resistance genes. J. Antimicrob. Chemother. 65, 1155–1161. doi: 10.1093/jac/dkq101
Gautom, R. K. (1997). Rapid pulsed-field gel electrophoresis protocol for typing of Escherichia coli O157:H7 and other gram-negative organisms in 1 day. J. Clin. Microbiol. 35, 2977–2980.
Gröndahl-Yli-Hannuksela, K., Lönnqvist, E., Kallonen, T., Lindholm, L., Jalava, J., Rantakokko-Jalava, K., et al. (2018). The first human report of mobile colistin resistance gene, mcr-1, in Finland. APMIS 126, 413–417. doi: 10.1111/apm.12834
Jeannot, K., Bolard, A., and Plésiat, P. (2017). Resistance to polymyxins in Gram-negative organisms. Int. J. Antimicrob. Agents 49, 526–535. doi: 10.1016/j.ijantimicag.2016.11.029
Johnson, T. J., Bielak, E. M., Fortini, D., Hansen, L. H., Hasman, H., Debroy, C., et al. (2012). Expansion of the IncX plasmid family for improved identification and typing of novel plasmids in drug-resistant Enterobacteriaceae. Plasmid 68, 43–50. doi: 10.1016/j.plasmid.2012.03.001
Kaye, K. S., Pogue, J. M., Tran, T. B., Nation, R. L., and Li, J. (2016). Agents of last resort: polymyxin resistance. Infect. Dis. Clin. North. Am. 30, 391–414. doi: 10.1016/j.idc.2016.02.005
Lei, L., Wang, Y., Schwarz, S., Walsh, T. R., Ou, Y., Wu, Y., et al. (2017). mcr-1 in Enterobacteriaceae from companion animals, Beijing, China, 2012-2016. Emerg. Infect. Dis. 23, 710–711. doi: 10.3201/eid2304.161732
Li, R., Yu, H., Xie, M., Chen, K., Dong, N., Lin, D., et al. (2018). Genetic basis of chromosomally-encoded mcr-1 gene. Int. J. Antimicrob. Agents 51, 578–585. doi: 10.1016/j.ijantimicag.2017.11.015
Li, X. P., Fang, L. X., Song, J. Q., Xia, J., Huo, W., Fang, J. T., et al. (2016). Clonal spread of mcr-1 in PMQR-carrying ST34 Salmonella isolates from animals in China. Sci. Rep. 6:38511. doi: 10.1038/srep38511
Liu, B. T., Song, F. J., Zou, M., Zhang, Q. D., and Shan, H. (2017). High incidence of Escherichia coli strains coharboring mcr-1 and blaNDM from chickens. Antimicrob. Agents Chemother. 61: e02347-16. doi: 10.1128/AAC/02347-16
Liu, Y. Y., Wang, Y., Walsh, T. R., Yi, L. X., Zhang, R., Spencer, J., et al. (2016). Emergence of plasmid-mediated colistin resistance mechanism MCR-1 in animals and human beings in China: a microbiological and molecular biological study. Lancet Infect. Dis. 16, 161–168. doi: 10.1016/S1473-3099(15)00424-7
Lv, L., Partridge, S. R., He, L., Zeng, Z., He, D., Ye, J., et al. (2013). Genetic characterization of IncI2 plasmids carrying blaCTX-M-55 spreading in both pets and food animals in China. Antimicrob. Agents Chemother. 57, 2824–2827. doi: 10.1128/AAC.02155-12
Maluta, R. P., Logue, C. M., Casas, M. R., Meng, T., Guastalli, E. A., and Rojas, T. C. (2014). Overlapped sequence types (STs) and serogroups of avian pathogenic (APEC) and human extra-intestinal pathogenic (ExPEC) Escherichia coli isolated in Brail. PLoS One 9:e105016. doi: 10.1371/journal.pone.0105016
Matamoros, S., van Hattem, J. M., Arcilla, M. S., Willemse, N., Melles, D. C., Penders, J., et al. (2017). Global phylogenetic analysis of Escherichia coli and plasmids carrying the mcr-1 gene indicates bacterial diversity but plasmid restriction. Sci. Rep. 7:15364. doi: 10.1038/s41598-017-15539-7
McGann, P., Snesrud, E., Maybank, R., Corey, B., Ong, A. C., Clifford, R., et al. (2016). Escherichia coli harboring mcr-1 and blaCTX-M on a novel IncF plasmid: first report of mcr-1 in the United States. Antimicrob. Agents Chemother. 60, 4420–4421. doi: 10.1128/AAC.01103-16
Olaitan, A. O., Chabou, S., Okdah, L., Morand, S., and Rolain, J. M. (2016). Dissemination of the mcr-1 colistin resistance gene. Lancet Infect. Dis. 16:147. doi: 10.1016/S1473-3099(15)00540-X
Olaitan, A. O., Thongmalayvong, B., Akkhavong, K., Somphavong, S., Paboriboune, P., Khounsy, S., et al. (2015). Clonal transmission of a colistin-resistant Escherichia coli from a domesticated pig to a human in Laos. J. Antimicrob. Chemother. 70, 3402–3404. doi: 10.1093/jac/dkv252
Snesrud, E., McGann, P., and Chandler, M. (2018). The birth and demise of the ISApl1-mcr-1-ISApl1 composite transposon: the vehicle for transferable colistin resitance. mBio 9:e02381-17. doi: 10.1128/mBio.02381-17
Sun, J., Yang, R. S., Zhang, Q., Feng, Y., Fang, L. X., Xia, J., et al. (2016). Co-transfer of blaNDM-5 and mcr-1 by an IncX3-X4 hybrid plasmid in Escherichia coli. Nat. Microbiol. 1:16176. doi: 10.1038/nmicrobiol.2016.176
Vogt, D., Overesch, G., Endimiani, A., Collaud, A., Thomann, A., and Perreten, V. (2014). Occurrence and genetic characteristics of third-generation cephalosporin-resistant Escherichia coli in Swiss retail meat. Microb. Drug. Resist. 20, 485–494. doi: 10.1089/mdr.2013.0210
Wang, R., van Dorp, L., Shaw, L. P., Bradley, P., Wang, Q., Wang, X., et al. (2018). The global distribution and spread of the mobilized colistin resistance gene mcr-1. Nat. Commun. 9:1179. doi: 10.1038/s41467-018-03205-z
Wu, R., Yi, L. X., Yu, L. F., Wang, J., Liu, Y., Chen, X., et al. (2018). Fitness advantage of mcr-1-bearing IncI2 and IncX4 plasmids in vitro. Front. Microbiol. 9:331. doi: 10.3389/fmicb.2018.00331
Yi, L., Wang, J., Gao, Y., Liu, Y., Doi, Y., Wu, R., et al. (2017). mcr-1-harboring Salmonella enterica Serovar Typhimurium sequence type 34 in pigs. China Emerg. Infect. Dis. 23, 291–295. doi: 10.3201/eid2302.161543
Zhang, X. F., Doi, Y., Huang, X., Li, H. Y., Zhong, L. L., Zeng, K. J., et al. (2016). Possible transmission of mcr-1-harboring Escherichia coli between companion animals and human. Emerg. Infect. Dis. 22, 1679–1681. doi: 10.3201/eid2209.160464
Zhao, F., Feng, Y., Lü, X., McNally, A., and Zong, Z. (2017). Remarkable diversity of Escherichia coli carrying mcr-1 from hospital sewage with the identification of two new mcr-1 variants. Front. Microbiol. 8:2094. doi: 10.3389/fmicb.2017.02094
Keywords: colistin resistance, companion animals, Escherichia coli, mcr-1, plasmids
Citation: Wang J, Huang X-Y, Xia Y-B, Guo Z-W, Ma Z-B, Yi M-Y, Lv L-C, Lu P-L, Yan J-C, Huang J-W, Zeng Z-L and Liu J-H (2018) Clonal Spread of Escherichia coli ST93 Carrying mcr-1-Harboring IncN1-IncHI2/ST3 Plasmid Among Companion Animals, China. Front. Microbiol. 9:2989. doi: 10.3389/fmicb.2018.02989
Received: 27 June 2018; Accepted: 19 November 2018;
Published: 04 December 2018.
Edited by:
Dongchang Sun, Zhejiang University of Technology, ChinaReviewed by:
Guo-bao TIAN, Sun Yat-sen University, ChinaYongfei Hu, China Agricultural University, China
Nora Lía Padola, National University of Central Buenos Aires, Argentina
Yonghong Xiao, Zhejiang University, China
Copyright © 2018 Wang, Huang, Xia, Guo, Ma, Yi, Lv, Lu, Yan, Huang, Zeng and Liu. This is an open-access article distributed under the terms of the Creative Commons Attribution License (CC BY). The use, distribution or reproduction in other forums is permitted, provided the original author(s) and the copyright owner(s) are credited and that the original publication in this journal is cited, in accordance with accepted academic practice. No use, distribution or reproduction is permitted which does not comply with these terms.
*Correspondence: Zhen-Ling Zeng, zlzeng@scau.edu.cn Jian-Hua Liu, jhliu@scau.edu.cn
†These authors have contributed equally to this work