- 1State Key Laboratory of Agrobiotechnology and Key Laboratory of Soil Microbial, Ministry of Agriculture, College of Biological Sciences, China Agricultural University, Beijing, China
- 2Escuela Nacional de Ciencias Biológicas, Instituto Politécnico Nacional, Mexico City, Mexico
Quorum sensing (QS) plays an important role in the growth, nodulation, and nitrogen fixation of rhizobia. In this study, we show that water-soluble humic materials (WSHM) repress the expression of the QS related genes sinI, sinR, and expR in Sinorhizobium meliloti. This decreased the production of N-acetyl homoserine lactones (AHL) and exopolysaccharides (EPS), and ultimately increased S. meliloti cell density. We also identified a novel regulator, SMc03890 (renamed QsrR), which binds directly to the expR promoter. Deletion of qsrR increased expR expression. WSHM repressed the expression of expR by augmenting the interaction between QsrR and the expR promoter; this was determined by a bacterial-one-hybrid assay. These effects of WSHM on the QS system in S. meliloti may be the underlying mechanism by which WSHM increase the symbiotic nitrogen fixation of Medicago sativa inoculated with S. meliloti. This study provides the first evidence that humic acids regulate the QS of rhizobia and suggests that WSHM could be used as fertilizers to improve the efficiency of symbiotic nitrogen fixation.
Introduction
Quorum sensing (QS) is a bacterial communication mechanism in which cell physiology and behavior are coordinated with population density (Bogino et al., 2015). In symbiotic nitrogen-fixing bacteria (rhizobia), QS plays a key role in their growth and the formation of symbiosis with their legume hosts (Bogino et al., 2015; Koul et al., 2016). In the QS system of Sinorhizobium meliloti 8530 (Figure 1), SinI is responsible for synthesis of the QS signaling molecules, N-acetyl homoserine lactones (AHL). The expression of sinI is induced by SinR; meanwhile, ExpR either mediates a positive regulatory feedback loop by inducing the expression of sinI or a negative feedback regulation by down-regulating the expression of sinR, depending on the AHL concentration (Charoenpanich et al., 2013; Calatrava-Morales et al., 2018). In addition to regulating the genes involved in nodulation and nitrogen fixation (Hoang et al., 2004), AHL-ExpR complex also up-regulate the expression of exp operon, which is involved in EPS II synthesis, and down-regulate genes related to bacterial motility, such as visN, visR, and rem (Gurich and Gonzalez, 2009; Mueller and González, 2010). The number of pink nodules induced by the sinI mutant decreased compared with the WT strain due to the inability of the sinI mutant to repress the expression of motility genes at high cell densities (Gurich and Gonzalez, 2009). The expR mutant, S. meliloti 1021, grows more rapidly and has an increased nodule occupancy (10∼20% higher than the WT strain, S. meliloti 8530) (Charoenpanich et al., 2015).
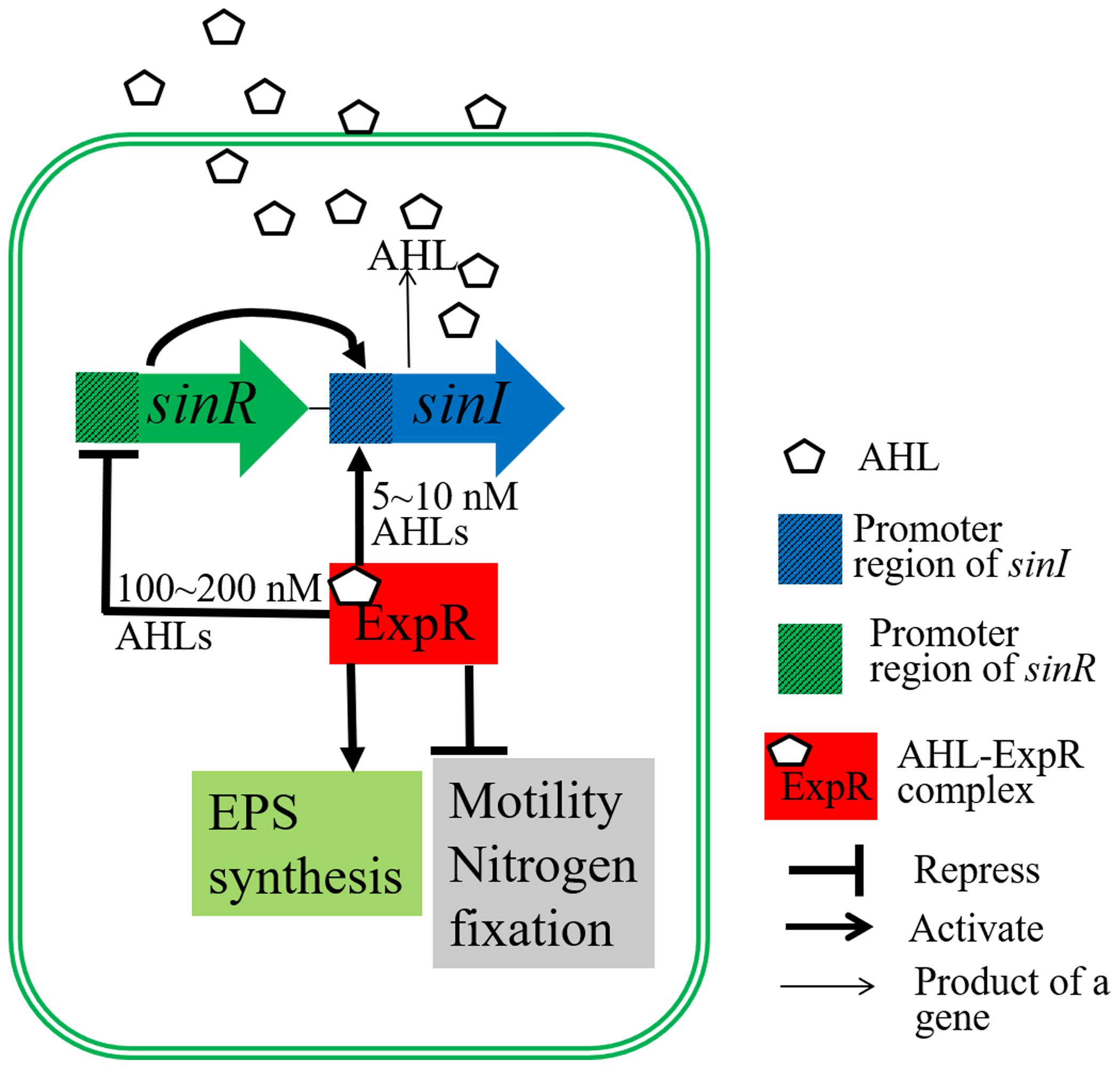
Figure 1. Regulatory diagram of the S. meliloti quorum sensing system. The transcription of sinI, which encodes AHL synthase, is induced by SinR. sinI transcription can also be induced by the AHL-ExpR complex at low AHL concentrations (5∼10 nM). The AHL-ExpR complex represses sinR expression at high AHL concentrations (100∼200 nM). Furthermore, the AHL-ExpR complex induces the expression of genes related to EPS biosynthesis, and represses the expression of genes important for motility, nodulation, and nitrogen fixation.
Many compounds have been reported to interfere with the bacterial QS systems (Kalia, 2013; Gonzalez-Ortiz et al., 2014). For rhizobia, L-canavanine, which is extracted from alfalfa seed exudates, inhibits the expression of S. meliloti expR gene (Keshavan et al., 2005), while the flavonoids induce the expression of AHL synthesis genes in Sinorhizobium fredii SMH12 and in Rhizobium etli ISP42 (Pérez-Montaño et al., 2011). Generally, compounds that interfere with microbial QS systems are heterocyclic compounds containing groups such as furan, pyridine, butyrolactone, benzene ring, and quinoline (Gonzalez and Keshavan, 2006; Christensen et al., 2013). These compounds have chemical structures similar to those of humic materials (Gao T.G. et al., 2015); thus, it is possible that humic materials may also interfere with microbial QS systems.
Humic materials are supramolecules derived from the residues of degraded plant, animal and microbial cells (Hayes and Wilson, 1997), with structures of relatively small self-assembled molecules that are held together by multiple weak interactions, such as hydrogen and van der Waals bonds. Generally, humic materials include, but are not limited to, n-alkanoic acids, n-alkanols, hydrocarbons, hydroxyacids, aromatic compounds, polyhydroxylated compounds, steroids, terpenoids, and N-heterocyclic compounds (Nebbioso and Piccolo, 2012). Humic materials are the most biological active compounds in soil that could stimulate plant growth as phytohormones (Traversa et al., 2013; Savy et al., 2017), enhance ATPase activity, promote nutrient utilization of plant (Jannin et al., 2012; Canellas et al., 2015), and stimulate the growth of bacteria (Tikhonov et al., 2010). In a previous study, we revealed that the water-soluble humic materials (WSHM) that are produced by lignite biodegradation enhanced the growth, cell metabolism, and nutrient transport of Bradyrhizobium liaoningense CCBAU05525, as well as its nodulation with soybean (12∼26% increased yield in soybean grains) (Gao T.G. et al., 2015). Therefore, WSHM could be a potential fertilizer to improve the legume yield.
In the present study, we examined the effects of WSHM on the QS system and the symbiotic nitrogen fixation of S. meliloti, and revealed that WSHM enhance the growth and nitrogen fixation of S. meliloti by regulating the QS system. This is the first report showing the effects of WSHM on the QS system in S. meliloti. Finally, we identified QsrR as a novel repressor of expR in this bacterium.
Materials and Methods
WSHM, Bacterial Strains, and Plasmids
Water-soluble humic materials were extracted from lignite collected from a Coal Mine in Inner Mongolia of China according to previously described methods (Dong et al., 2006; Jiang et al., 2013). Briefly, the lignite powder was inoculated with Penicillium sp. P6 and Bacillus sp. Y7 and incubated at 28°C for 2 weeks. Biodegraded lignite was diluted tenfold in deionized water, stirred, and centrifuged three times at 9000 ×g for 15 min. The supernatant was filtered through Whatman No. 1 filter paper and the filtrated (WSHM) were dried at 40°C for about 72 h, weighed, and stored in a vacuum-dried chamber. In the WSHM, 68 aromatic, aliphatic, and nitrogen-based compounds were detected by tetramethyl ammonium hydroxide (TMAH)-py-GC/MS (Gao T.G. et al., 2015).
All the bacterial strains and plasmids used in this study are listed in Supplementary Table S1. Among them, S. meliloti 1021 is a native mutant in which the ORF of expR is interrupted by an insertion; while S. meliloti 8530 is a derivative of S. meliloti 1021 by excising this insertion spontaneously. Since most S. meliloti strains harbor a functional expR (Pellock et al., 2002), S. meliloti 8530 was considered as the wild type strain. Agrobacterium tumefaciens KYC55 (pJZ372, pJZ384, pJZ410) was used as a bioassay strain for ultrasensitive detection of AHL.
Growth Response of S. meliloti 8530 to WSHM
Sinorhizobium meliloti 8530 was preincubated in 50 mL of YM broth (Gao T.G. et al., 2015) for 4 days at 28°C with shaking (150 rpm). The culture was used to inoculate 50 mL of either YM broth (control) or YM broth with 500 mg L-1 WSHM at a final OD600 of 0.01. The cultures were incubated at 28°C with shaking (150 rpm) for 5–6 days and samples were plated using serial dilutions every 12 h to evaluate the rhizobia cell density. This assay was performed thrice in triplicate.
Response of AHL Production to WSHM
Agrobacterium tumefaciens KYC55 (pJZ372, pJZ384, pJZ410) was incubated in AT medium [KH2PO4, 10.7 g; MgSO4 ⋅ 7H2O, 160 mg; CaCl2, 78 mg; FeSO4 ⋅ 7H2O, 5 mg; MnSO4 ⋅ H2O, 2.2 mg; (NH4)2SO4, 2 g; glucose, 5 g; in 1 L of distilled water; pH adjusted to 7.3 with K2HPO4; tetracycline, spectinomycin, and gentamycin at final concentrations of 2, 100, and 100 μg/mL respectively] with shaking at 150 rpm for 2 days to yield up to 109 cells mL-1 (Zhu et al., 2003). The AHL production was detected as previously described (Zheng et al., 2006). Briefly, the cell-free supernatant of S. meliloti 1021 or 8530 culture (Figure 2B) was added to fresh AT broth at the ratio of 10% (v/v). The cell-free supernatant of A. tumefaciens strain R10 (pCF218) (Zhu et al., 2003) culture was used as positive control to assess the AHL sensitivity of A. tumefaciens KYC55 under these experimental conditions. The same volume of sterilized water (Control) or WSHM solution (500 mg L-1) was added separately into AT broth as negative controls. All the AT media prepared for tests were inoculated with approximately 107 cells mL-1 of A. tumefaciens KYC55 and incubated with aeration (150 rpm) for 16–20 h at 28°C. The β-galactosidase activity in each culture was quantitatively analyzed to estimate the concentration of AHL (Zhu et al., 2003). β-galactosidase activity (Miller units) was calculated as OD420 × 103/(time of reaction in minutes × volume of culture in milliliters × OD600) (Pérez-Montaño et al., 2011). Assays were conducted in triplicate for three times.
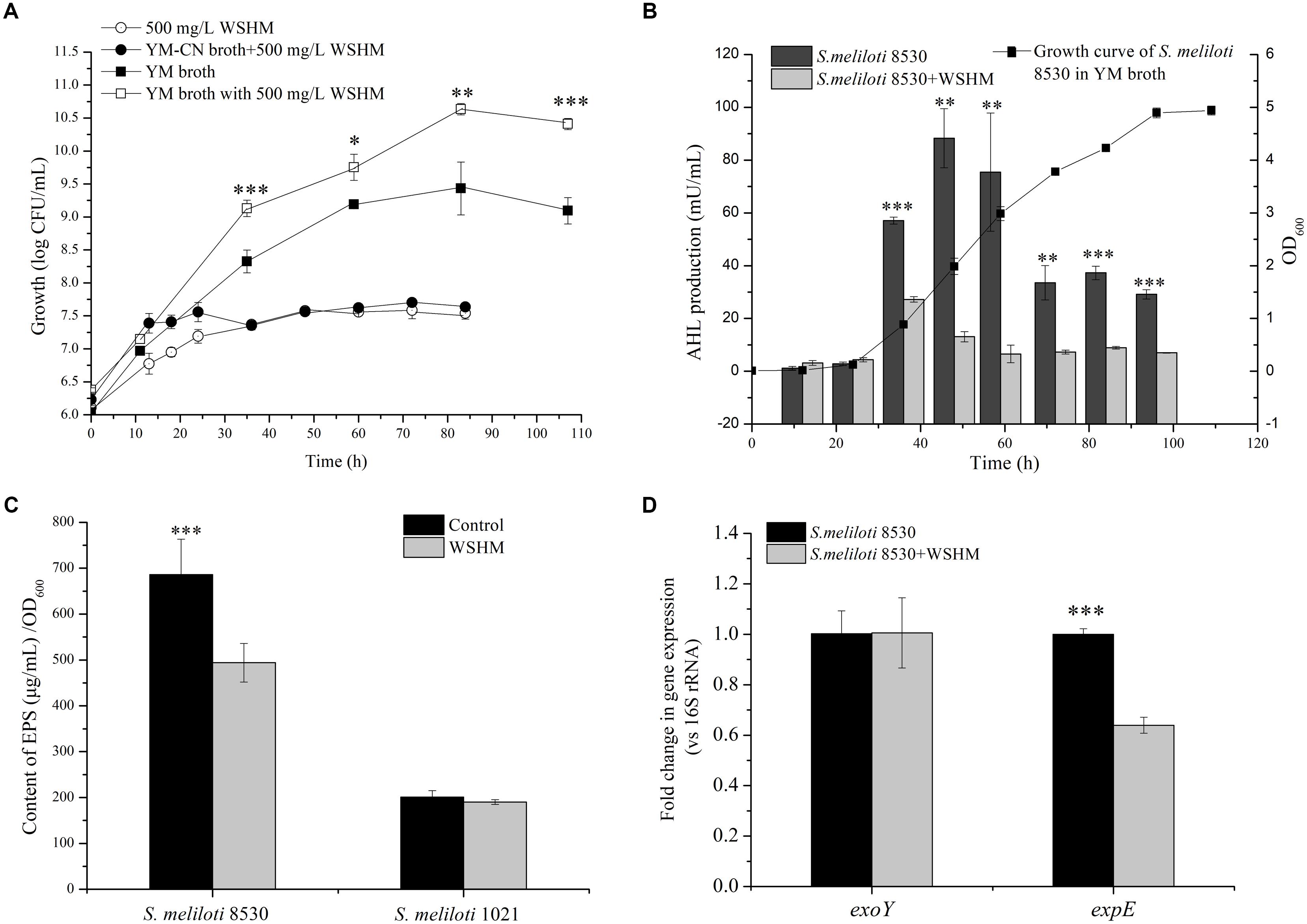
Figure 2. Effects of WSHM on the growth (A), AHL production (B), exopolysaccharide production (C), and exoY and expE gene expression (D) in S. meliloti 8530. All the data are expressed as average ± standard deviation (SD) (n = 3). Statistical significance was assessed by Student’s t-tests (∗P < 0.05; ∗∗P < 0.01; ∗∗∗P < 0.001). For exopolysaccharides production (C), S. meliloti 1021 (ΔexpR) was also included. (A) YM-CN broth: the nitrogen (yeast extract) and carbon (mannitol) source in YM broth were removed.
Response of EPS Synthesis to WSHM Treatment
The effects of WSHM on EPS synthesis were analyzed for the wild type strain S. meliloti 8530, which can produce EPS I (succinoglycan), EPS II (galactoglucan), and linear mixed-linkage β-glucan (MLG) (Pérez-Mendoza et al., 2015), as well as for S. meliloti 1021, which could produce EPS I and extremely low level of EPS II (Pellock et al., 2002). Both strains were cultured in GMS medium (Staehelin et al., 2006) with and without 500 mg L-1 of WSHM for 5 days. EPSs were collected as described by Staehelin et al. (2006) and quantified in triplicate by the anthrone-sulfuric acid method (Jones, 2012).
Expression of exoY and expE Genes in Response to WSHM
The effects of WSHM on the expression of exoY and expE, which are responsible for EPS I and EPS II synthesis, respectively (Mueller and González, 2010), were analyzed by reverse transcription quantitative real-time PCR (RT-qPCR). S. meliloti 8530 was cultured in YM broth (control) or YM broth supplied with 500 mg L-1 WSHM as mentioned above. Samples were collected at the end of exponential phrase (OD600 = 1.5–2.0). Total RNA was isolated using the RNA Pure Bacteria Kit (CWBIO, Beijing, China) according to the manufacturer’s instructions. First strand cDNAs were synthesized using PrimeScript Reverse Transcriptase (RT) (TaKaRa Code: D2680S) according to the manufacturer’s instructions. These cDNA samples were used for RT-qPCR with primers specific to exoY and expE genes (Supplementary Table S2). A 167 bp fragment of 16S rRNA gene was used as an internal control for normalization (Pérez-Montaño et al., 2014). Each 20 μL-reaction contained 10 μL of Power SYBR Green Master Mix (ABI, United States). The PCR program was: 95°C for 10 min, followed by; 40 cycles of 95°C for 15 s and 60°C for 1 min, followed by the melting curve. PCR was performed on an ABI 7500 Thermocycler and data were analyzed using the 2-ΔΔCt method (Jannin et al., 2012). The experiment was performed for three times with four technical replicates.
Expression of QS Genes in Response to WSHM
The bacteria were cultured same as described above (see Expression of exoY and expE Genes in Response to WSHM). The expression of QS genes following WSHM treatment was analyzed by RT-qPCR as mentioned above with primers specific to sinI, sinR, and expR (Supplementary Table S2). The cDNA obtained from cultures of S. meliloti 8530, S. meliloti 1021 (ΔexpR), S. meliloti MG170 (ΔsinR), and S. meliloti MG32 (ΔsinI) (Supplementary Table S1) with or without WSHM were used as templates, and the experiment was performed three times with four replicates.
Deletion of qsrR and Its Effect on expR Expression in Response to WSHM
Agrobacterium tumefaciens interacts with plant hosts similar to those of rhizobia. Thus, in order to analyze the repression mechanisms of WSHM on expR expression, the QS regulation system in A. tumefaciens (Gonzalez and Keshavan, 2006) was compared with that in S. meliloti 8530. AccR of A. tumefaciens can counteract traR repression by directly binding with opines produced by the plant host. Since protein SMc03890 in strain 8530, which was renamed as QsrR in this study, was very similar to AccR (with 31% similarity of amino acid sequence) and were both DeoR family transcriptional regulators, we hypothesized that QsrR could be capable of mediating the effects of WSHM on expR expression. To test this hypothesis, the S. melilotiΔqsrR mutant strain was constructed via homologous recombination. Furthermore, by fusing the expR promoter region with the lacZ structural gene, the change in expR expression caused by the deletion of qsrR was determined by the activity of β-galactosidase. Refer to Supplementary Methods for detailed description of the process.
Previous reports have evidenced that the eukaryotic hosts are capable of interfering with bacterial QS by producing molecular signals, like flavonoid (Kalia, 2013; Nievas et al., 2017), and flavonoid homologs have been detected in WSHM (Gao T.G. et al., 2015). In order to analyze whether WSHM function as a plant signal (like opines) to represses QS in S. meliloti, expR gene expression levels in S. meliloti 8530 or S. melilotiΔqsrR following treatment with either WSHM (500 mg L-1) or alfalfa seed exudates (2%, v/v) were determined by measuring the activity of β-galactosidase (Chai et al., 2010). Alfalfa seed exudates were prepared according to Cai et al. (2009). Assays were conducted in triplicate and repeated three times.
QsrR Purification and Electrophoretic Mobility Shift Assays (EMSAs)
The full-length ORF of qsrR was amplified using the primers qsrR281 and qsrR282 (Supplementary Table S2) and cloned into pET28a. The recombinant plasmid pET28a-qsrR was transformed into Escherichia coli Rosetta (DE3) and cultured in LB medium with 0.4 mM isopropyl-β-D-thiogalactoside (IPTG) for induction at 37°C. The QsrR protein with a His tag (His6-QsrR) was purified with Ni-loaded nitrilotriacetic acid (NTA) resin (GE Healthcare) from cultures of E. coli Rosetta (DE3) carrying the recombinant plasmid. Electrophoretic mobility shift assays (EMSAs) were used to detect the interaction between the QsrR protein and the expR promoter using a DIG Gel Shift Kit, 2nd Generation (Roche), according to the manufacturer’s instructions. The promoter region of expR was amplified with primers expR11 and expR12 (Supplementary Table S2). Binding specificity was evaluated through addition of ∼100-fold excess of unlabeled expR promoter fragments, which competed with the labeled probe to bind with His6-QsrR. A labeled non-specific DNA probe from Streptomyces avermitilis was used as negative control. EMSAs were repeated at least twice.
Bacterial One-Hybrid Assay
The experimental procedure was similar to that of Luo et al. (2014). Briefly, the gene qsrR was amplified with primers 03890BHf and 03890BHr (Supplementary Table S2), excised with Not I/Bgl II, and cloned into the bait plasmid pB1H1 to generate pB1H1-qsrR. Fragments R1 and R4 in the promoter region of expR were cloned into the prey plasmid pH3U3, respectively. pH3U3-R1 and pH3U3-R4 were not self-activating prey confirmed previously (see Supplementary Methods for detail). Then the plasmid pairs pB1H1-qsrR/pH3U3-R1 and pB1H1-qsrR/pH3U3-R4 were transformed into E. coli USO respectively. The growth of the transformants of E. coli USO, including USO: pB1H1-zif268/pH3U3-zif268 as positive control (+/+); USO: pB1H1/pH3U3 (-/-), USO: pB1H1/pH3U3-R1 and USO: pB1H1/pH3U3-R4 as negative controls (-/+); and two transformants with qsrR, USO: pB1H1-qsrR/pH3U3-R1 and USO: pB1H1-qsrR/pH3U3-R4 (+/+), were determined on NM medium without histidine and with varying concentrations of 3-AT. We expected the transformants with qsrR to survive on NM medium containing 3-AT, as long as QsrR interacts with the expR promoter region to recruit RNA polymerase and activate the transcription of the reporter gene HIS3. Higher levels of HIS3 gene expression enable the bacteria grow on NM medium with higher concentrations of 3-AT. Assays were repeated three times.
Effects of WSHM on Plant Growth and Nodulation by S. meliloti
Sinorhizobium meliloti was cultured aerobically at 28°C in YM broth (Gao T.G. et al., 2015) for 2 days to OD600 = 1.0 (about 108 CFU mL-1) and were used as inoculant. Medicago sativa seeds were surface-sterilized by 3% (v/v) NaClO for 3 min, germinated on 0.7% agar-water plates in the dark at 28°C for 24–48 h. The germinated seeds were planted in pots (three seeds per pot) filled with 300 cm3 of vermiculite and moisturized with low-N nutrient solution (Vincent, 1970). Six treatments with seven replicates (i.e., pots) were included: no inoculation control with and without WSHM (500 mg L-1); inoculation treatments of wild type strain (S. meliloti 8530, 1 mL) with and without WSHM; and inoculation treatments of ΔqsrR strain (1 mL) with and without WSHM (Table 1). Plants were grown in a greenhouse at 25 ± 2°C during the day and 17 ± 2°C at night with 60% relative humidity. Pots were rearranged daily to give a random distribution of growth conditions. After 45 days, all of the alfalfa plants were harvested and the number, fresh weight, and nitrogenase activity of the nodules, as well as the dry weight of the plants, were determined for each pot. The nitrogenase activity was analyzed by acetylene reduction assay, for which the whole roots with nodules in each pot (three plants) were put into a sealed bottle and incubated with acetylene (Suganuma et al., 1998), and the nitrogenase activity was calculated as μmol C2H4 /h ⋅ g of nodule. Then, the number and fresh weight of nodules in each pot were counted. The pooled data for each pot (three plants) were considered as one sample, and statistical analysis was conducted using Duncan test. Finally, several root nodules were sliced and treated for transmission electron microscopy (TEM) according to Bourassa et al. (2017) to evaluate the effects of WSHM treatment on the number and morphology of bacteroids in nodules induced by S. meliloti 8530.
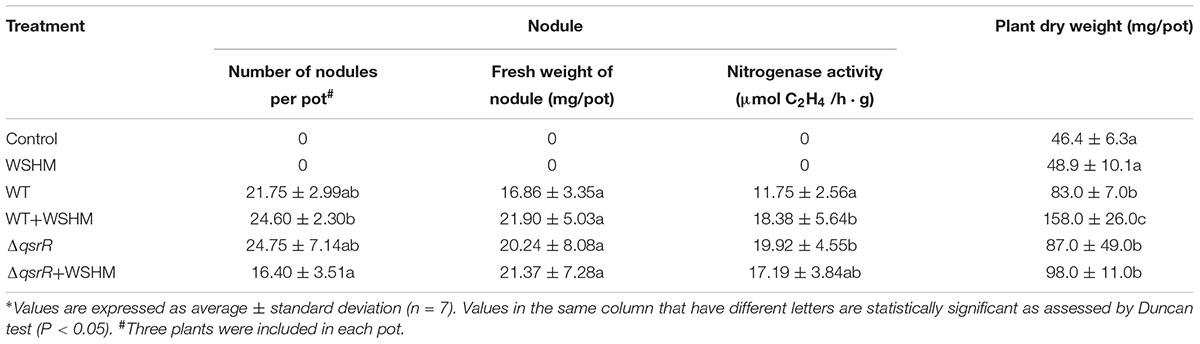
Table 1. Effects of WSHM on nodulation of M. sativa inoculated with S. melilotiΔqsrR or S. meliloti 8530 (WT) in the greenhouse∗.
Results
The Growth of S. meliloti in Response to WSHM
As shown in Figure 2A, the cell density of S. meliloti 8530 was about 3.5 × 107 CFU/mL (83 h) in the WSHM solution or in YM-CN broth (without carbon/nitrogen source) supplied with WSHM. While it was 2.51 × 109 CFU/mL in YM broth and 3.98 × 1010 CFU/mL in YM broth supplied with WSHM, which corresponds to 14.8-fold increase in cell density by WSHM at the stationary phase (Figure 2A). We could calculate from these data that only 0.09% of the total cell number increased in YM broth supplied with WSHM could be attributed to the additional nutrient sources from WSHM. Therefore, this growth enhancement can be mainly attributed to the stimulation effect of WSHM.
Synthesis of AHL in Response to WSHM
In this analysis, the AHL detector strain, A. tumefaciens KYC55 did not respond to WSHM but did respond to the AHL produced by A. tumefaciens R10 (Supplementary Figure S1). Thus, A. tumefaciens KYC55 was used to investigate the effects of WSHM on AHL production in S. meliloti. AHL synthesis in S. meliloti 8530 started in the beginning of exponential phase and reached a peak during the middle of exponential phase (50 h); while the AHL synthesis during the whole potential phase was significantly decreased (60–93%) by WSHM (Figure 2B). WSHM treatment also reduced the production of AHL in S. meliloti 1021 by 57.9% (from 65 to 28 mU/mL, 50 h of incubation) (Supplementary Figure S1). Additionally, increased production of AHL by A. tumefaciens R10 in response to WSHM was observed (Supplementary Figure S1). These results suggest that WSHM could regulate the QS system in S. meliloti.
EPS Synthesis and exoY/expE Expression of S. meliloti in Response to WSHM
Exopolysaccharides synthesis in S. meliloti 1021 was low (<200 μg ml-1) and was not affected by WSHM (Figure 2C); while EPS synthesis in S. meliloti 8530 was high (680 μg ml-1) without WSHM and was decreased by 30.87% (480 μg ml-1) with WSHM treatment.
For expE, a gene involved in EPS II synthesis and regulated by AHL-ExpR, its expression was down-regulated significantly by treatment with WSHM (Figure 2D); meanwhile, the expression of exoY, a gene involved in EPS I synthesis, was not affected by WSHM in S. meliloti 8530. All of these results suggest that WSHM did not affect the synthesis of EPS I, but decreased the production of EPS II and MLG.
Impact of WSHM on the Expression of Genes Involved in QS
In this analysis, WSHM treatment significantly down-regulated the expression of sinI, sinR, and expR in S. meliloti 8530 (Figure 3A); while in the expR mutant strain, S. meliloti 1021, WSHM only down-regulated sinR expression and did not alternate sinI expression (Figure 3B). In addition, the expression levels of both sinI and expR were down-regulated by WSHM in the sinR mutant (MG170, Figure 3C). These results suggest that the repression of sinI in S. meliloti 8530 by WSHM is due to the repression of expR and is independent of sinR. In the sinI mutant (MG32), the expression levels of the sinR and expR genes were down-regulated by WSHM (Figure 3D); suggesting that the effects of WSHM on sinR and expR expression are independent of sinI. Furthermore, the sinR expression was down-regulated by WSHM in both S. meliloti 8530 and S. meliloti 1021 (ΔexpR), suggesting that the repression of sinR expression by WSHM is independent of ExpR.
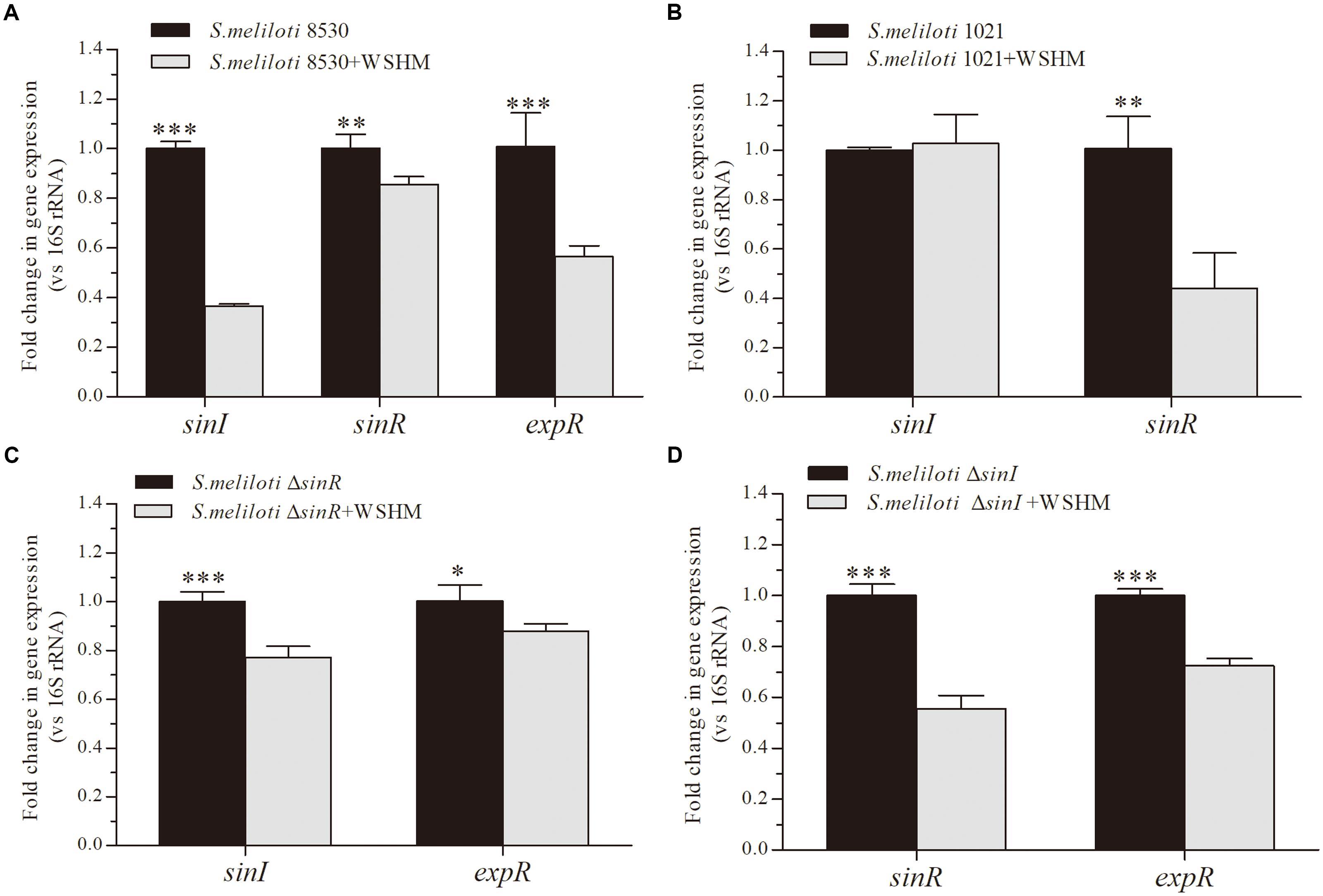
Figure 3. Effects of WSHM on the expression of genes involved in the QS system in S. meliloti 8530 (A), S. meliloti 1021 (B), S. melilotiΔsinR (C), and S. melilotiΔsinI (D). Data are expressed as average ± SD (n = 3). Statistical significance was determined using Student’s t-tests (∗P < 0.05; ∗∗P < 0.01; ∗∗∗P < 0.001).
Effects of WSHM on the Expression of expR in S. meliloti ΔqsrR
The expression level of the expR gene in S. melilotiΔqsrR (SMc03890 deletion, see Section “Deletion of qsrR and Its Effect on expR Expression in Response to WSHM” for detail) was significantly higher than that in the WT (Figure 4), suggesting that QsrR represses expR transcription. Thus, the SMc03890 gene was renamed qsrR, for “quorum sensing regulator expR repressor.” As expected, the expR gene was down-regulated significantly in response to WSHM in S. meliloti 8530, but not in S. melilotiΔqsrR, suggesting that QsrR is responsible for repressing expR expression in response to WSHM. Meanwhile, alfalfa seed exudates significantly repressed expR expression in both S. meliloti 8530 and S. melilotiΔqsrR (Figure 4), suggesting that the repression of expR expression by seed exudates is independent of QsrR.
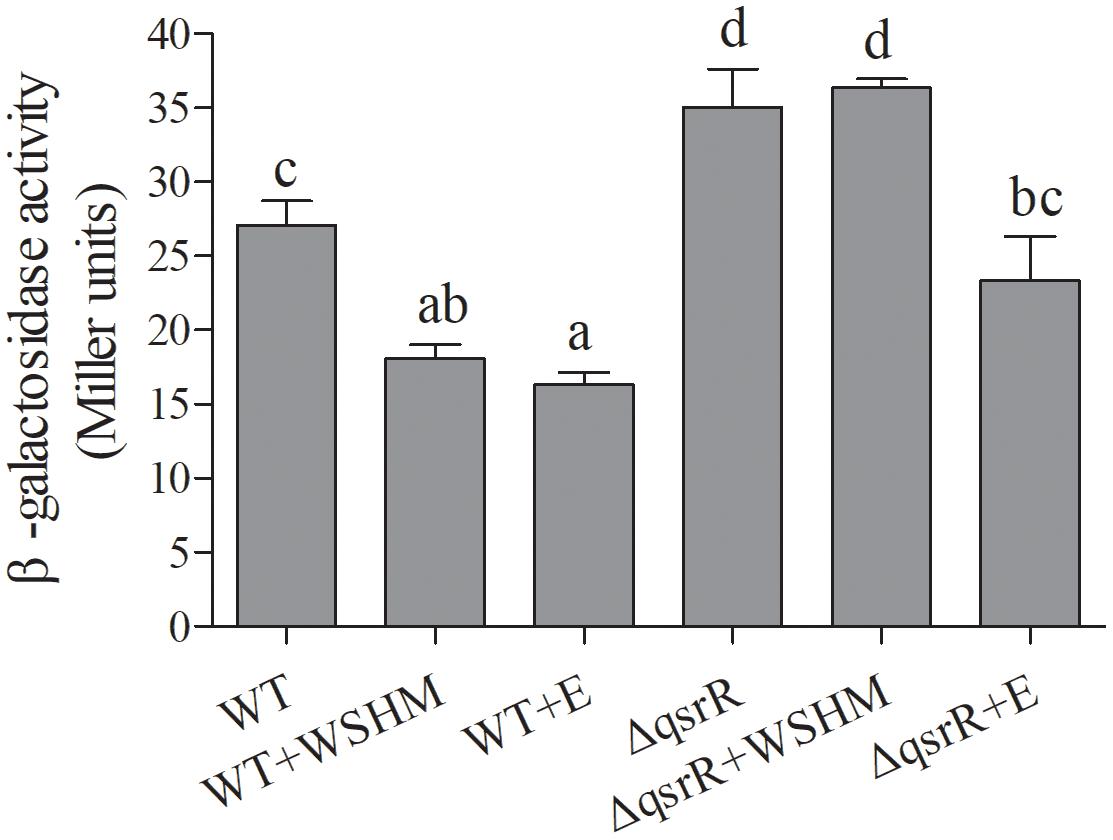
Figure 4. Expression of PexpR-lacZ in S. meliloti 8530 (WT) and S. melilotiΔqsrR mutant treated with either 500 mg L-1 WSHM or 2% alfalfa seed exudates (E), respectively. Alfalfa seed exudates were used to assess whether WSHM could repress expR expression in a manner similar with plant signal. Data are expressed as average ± SEM for three replicates. Different letters indicate statistical significance as assessed by Duncan tests (P < 0.05).
Potentiated Interaction Between QsrR and expR Promoter by WSHM
In order to test whether QsrR regulate the expression of expR directly or indirectly, EMSA were performed. As shown in Figure 5, a retarded DNA was observed when His6-QsrR (3.3 μM) was added to the assay mixture, indicating that QsrR was able to bind directly with the promoter region of expR gene. There was no retardation in the binding specificity assay or the negative control. These findings indicate that the transcription of expR is directly regulated by QsrR. In the EMSA test that determined the effect of WSHM on the interaction between QsrR and expR promoter, no band was detected when WSHM was added into the reaction system (data not shown). This might result from the possibility that WSHM interfered with the experimental process of EMSA, such as electrophoresis or binding of DNA with nylon membrane. Therefore, the role of WSHM in regulating expR expression via QsrR was further investigated by a bacterial one-hybrid assay (see Supplementary Methods and Supplementary Figure S3 for detail). The negative control strains E. coli USO (pB1H1/pH3U3-R1) and USO (pB1H1/pH3U3-R4) did not express qsrR and grew poorly; meanwhile, the positive strains USO (pB1H1-qsrR/pH3U3-R1) and USO (pB1H1-qsrR/pH3U3-R4) expressed qsrR and grew much better than the negative controls on selective medium (NM+3-AT) (Figure 6C). The positive strains were able to grow on medium with 2 mM of 3-AT that confirmed the ability of QsrR to bind with the promoter region of the expR gene. The binding region of QsrR is closer to the start of SMc03900 gene rather than that of expR (region R4, Figure 6B). In addition, the growth of the positive strains was significantly enhanced when WSHM were added to the selective medium; tolerance to 3-AT also increased from 2 to 5 mM. Meanwhile, growth of the negative control strains (USO: pB1H1/pH3U3-R1 and USO: pB1H1/pH3U3-R4) was not enhanced by WSHM on NM medium containing either 3 or 5 mM of 3-AT, further confirming that WSHM do not serve as a source of nutrients for these strains. These results suggest that QsrR can bind with the promoter region of expR gene, while WSHM can significantly potentiate this interaction.
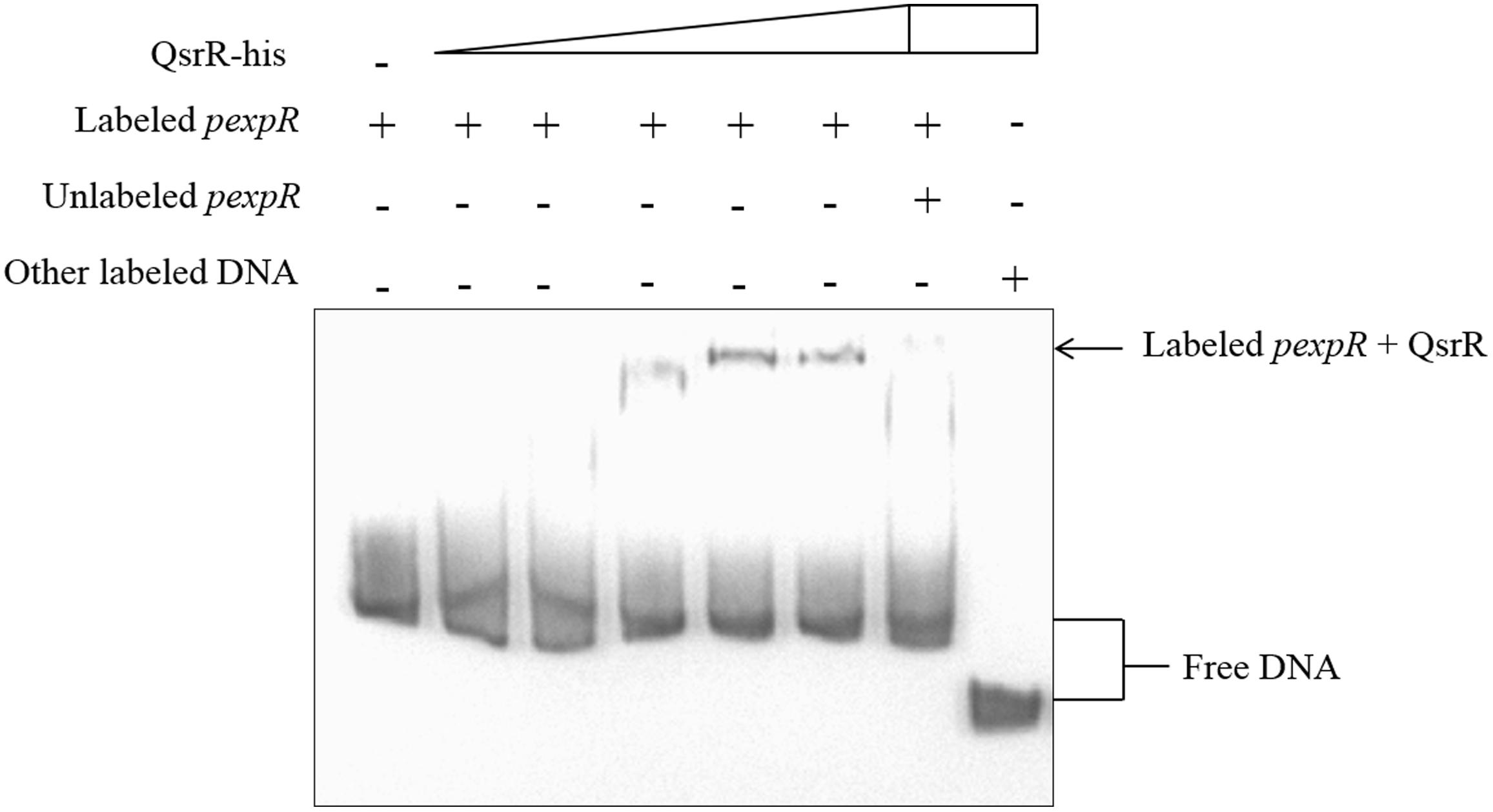
Figure 5. Electrophoretic mobility shift assays confirmed direct binding of QsrR to the expR promoter (pexpR). pexpR is a 286 bp DNA fragment from the translational start codon of gene expR. Each lane contained 0.005 nM of labeled pexpR. The labeled pexpR and a ∼100-fold excess of the unlabeled pexpR were used in competitive assays. Labeled non-specific DNA from Streptomyces avermitilis was used as negative control. The amount of His6-QsrR added in each lane were 0 μM, 0.92 Mm, 1.65 μM, 2.38 μM, 3.3 μM, 4.77 μM, 4.77 μM, and 4.77 μM, respectively.
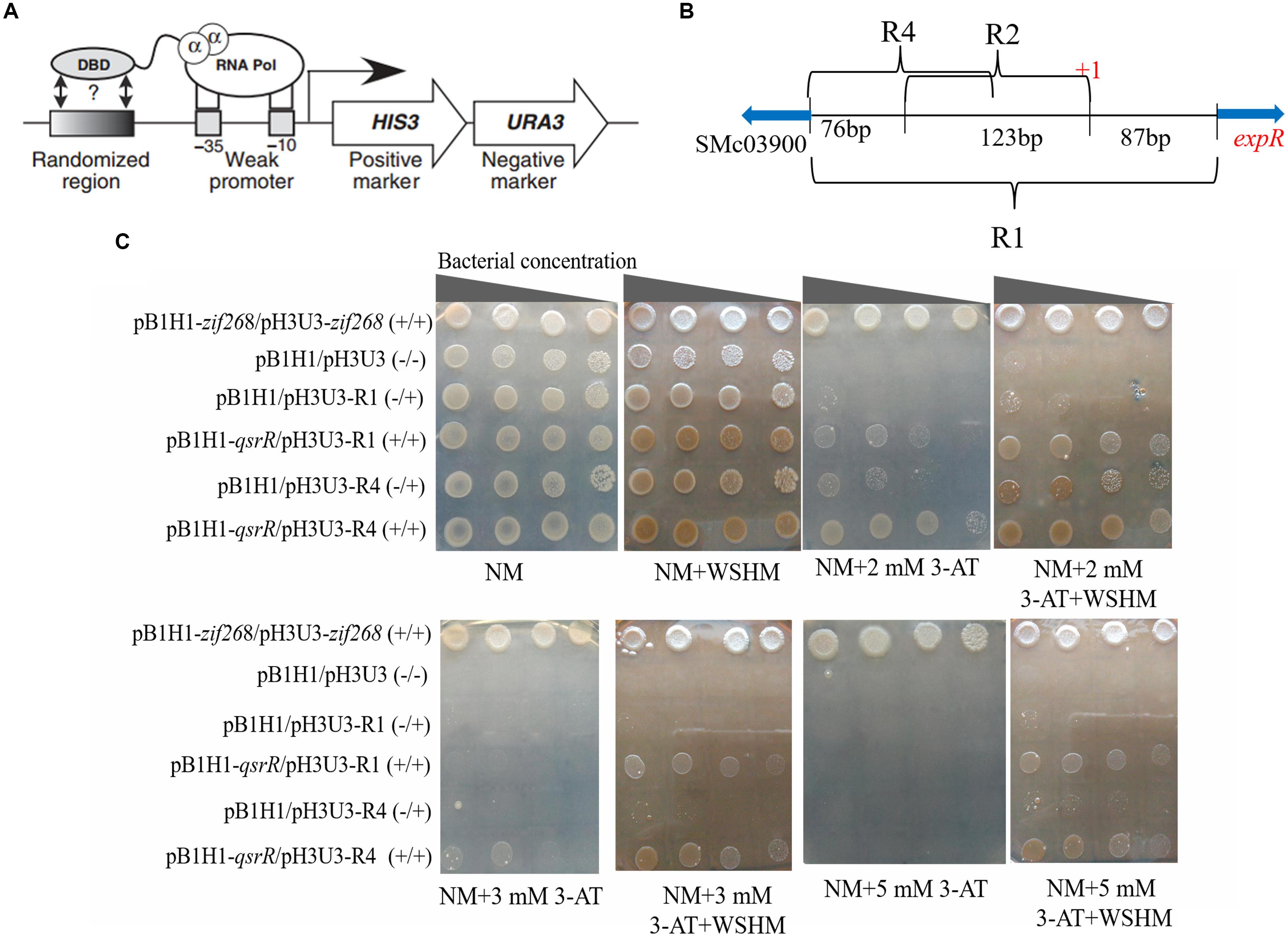
Figure 6. Bacterial-one-hybrid assays showing interaction between expR promoter (R1, R4) and QsrR which was potentiated by WSHM. (A) Schematic diagram of bacterial one-hybrid system. The HIS3/URA3 cistron is in the pH3U3 prey vector. The target DNA sequences were ligated to pH3U3. The binding of DNA-binding domain (DBD, ligated to pB1H1) with the target DNA sequence enables the transcription of HIS3 and URA3. The expression of HIS3 enabled E. coli USO grow on NM selective medium containing the desired concentration of 3-amino-triazole (3-AT), a competitive inhibitor of HIS3. The expression of URA3 prevented E. coli USO growth on medium containing 5- fluoroorotic acid (5-FOA). This figure was adopted from Meng et al. (2005). (B) Schematic diagram of the promoter region of gene expR which was cloned into pH3U3. “+1” in red was marked as the transcription start site of expR which was located in 87 bp upstream of ATG of expR (Charoenpanich et al., 2013). Regions R1, R2, and R4 were ligated to pH3U3 prey vector to detect the binding region of QsrR. Region R1 is the entire intergenic region between expR and SMc03900. Region R2 is the middle region of R1 which lost the 87bp right flank and the 76bp left flank of R1. Region R4 is the left flank of R1 (142 bp). The blue arrows indicate transcriptional direction of expR and SMc03900. Region R2 was excluded from further analyses because of the high level self-activation of (E. coli USO: pB1H1/pH3U3-R2) (see Materials and Methods for details). (C) The interaction of QsrR and pexpR was assayed by the growth of bacteria on selective medium (NM lacking histidine) supplemented with 2, 3, or 5 mM of 3-AT. The genetic information of test strains is labeled on the left. “+/–” indicated that qsrR gene was cloned into pB1H1 (+) or the plasmid was left empty (–), and similar information for pH3U3. Images were assembled and the marked lines were eliminated without modifying the observed data in Adobe Photoshop CS5.
Effects of WSHM on M. sativa Growth and Nodulation With S. meliloti 8530
Water-soluble humic materials treatment increased plant dry weight by 5.4% compared to the control (Table 1). The nitrogenase activity and plant dry weight of M. sativa inoculated with S. meliloti 8530 and 500 mg L-1 of WSHM were significantly increased by 56.4 and 90.36%, respectively, compared with plants inoculated only with S. meliloti 8530, while no statistically significant difference was detected in nodule number and nodule fresh weight (13.1 and 29.9%) between these two treatments. No significant difference was observed in nitrogenase activity, plant dry weight, nodule number, or nodule fresh weight between the M. sativa inoculated with S. melilotiΔqsrR +/- WSHM. Besides, the nitrogenase activity of plants inoculated with S. melilotiΔqsrR alone was significantly increased by 69.53% compared with plants inoculated with S. meliloti 8530.
Transmission electron microscopy was performed to tested whether the increased nitrogenase activity of M. sativa inoculated with S. meliloti 8530 by WSHM was due to increased bacteroid density in nodules. The TEM images revealed that the number and morphology of bacteroids in alfalfa nodules induced by S. meliloti 8530 were not impacted by WSHM treatment (Supplementary Figure S4). Thus, WSHM may have affected the nitrogenase activity of the root nodules by other mechanisms, such as regulating the expression of nitrogen fixation genes or improving the energy supply to the bacteroids.
Discussion
Quorum sensing regulates metabolically costly cooperative behaviors of bacteria depending on the environmental and physiological characteristics, such as production of exopolysaccharides, motility, and life-style switches related to symbiosis with eukaryotic hosts (Hense and Schuster, 2015; Calatrava-Morales et al., 2018). Many plant-associated bacteria communicate with the plant host through QS (González and Venturi, 2013; Schikora et al., 2016). The ExpR/Sin system is the sole QS system in S. meliloti 8530 (Krol and Becker, 2014) (Figure 1) that is involved in the regulation of metabolic and symbiotic procedures (Hoang et al., 2004; Gurich and Gonzalez, 2009). Our study regarding the effects of WSHM on the ExpR/Sin system in S. meliloti 8530 yielded several important findings.
SMc03890, renamed as qsrR, was shown to code a protein that directly represses expR gene transcription (Figures 4–6). As a versatile LuxR homolog regulator, ExpR directly or indirectly regulates the expression of at least 570 genes and plays a central role in the QS network in S. meliloti (Hoang et al., 2004; Gurich and Gonzalez, 2009; Charoenpanich et al., 2013). Thus, qsrR might have an essential function in different processes of S. meliloti. Previously, few studies on the regulation of the expR gene have been performed. One previous study showed that L-canavanine can repress expR expression as an arginine analog (Keshavan et al., 2005); meanwhile, Gao M. et al. (2015) reported that the RNA-binding protein Hfq regulates expR post-transcriptionally at higher population densities. Our study described QsrR as a novel repressor for expR transcription (Figure 4) and revealed the physical interaction between QsrR and the expR promoter by EMSA (Figure 5) and one-hybrid assays (Figure 6C). In addition, the increased nitrogenase activity in the nodules formed by S. melilotiΔqsrR compared with those formed by S. meliloti 8530 (Table 1) suggests that QsrR may also regulate the expression of other genes, including those involved in nitrogen fixation. The function of QsrR is worthy of further research due to the versatile role of ExpR in regulating the metabolism and nodulation of S. meliloti. For example, the specificity of DNA sequences that can bind with QsrR might be further studied in order to reveal details in the interaction between QsrR and expR promoter.
The regulation of QS system in S. meliloti by WSHM via repressing expR expression (Figures 2–4) was evidenced for the first time, although different biological effects of humic materials on plants (Jannin et al., 2012; Traversa et al., 2013; Canellas et al., 2015; Savy et al., 2017) and bacteria (Tikhonov et al., 2010) have been demonstrated. The results from Figures 4–6 demonstrated that QsrR may mediate the repression of expR expression by WSHM. It is possible that WSHM also repress sinR expression via additional mechanisms since QsrR cannot bind with the promoter region of sinR (Supplementary Figure S2). Moreover, our results suggest that WSHM function in a manner comparable to alfalfa seed exudates and may act as plant signal to repress expR gene expression in S. meliloti (Figure 4). Previous reports have demonstrated that plants are able to produce compounds that mimic or inhibit bacterial QS processes to promote their development of beneficial traits (Gao et al., 2003; Schikora et al., 2016; Nievas et al., 2017). WSHM might help plants to regulate bacterial QS and improve the symbiotic relationship between the host plant and bacteria. Compounds such as furan, pyrrole, benzpyrole, benzene rings, and esters have been identified in WSHM (Gao T.G. et al., 2015) and they may interfere with microbial QS systems (Gonzalez and Keshavan, 2006; Christensen et al., 2013). However, further investigation is needed to identify the compounds responsible for S. meliloti QS regulation in WSHM or alfalfa seed exudates.
In addition, the results suggest that the inactivation of rhizobia ExpR/Sin genes in nodules might be due to regulation by the plant host. Even though the QS system in S. meliloti has been reported to control cell functions essential for successful plant invasion, the ExpR/Sin genes were inactive in nodules due to an unknown mechanism (Gurich and Gonzalez, 2009). Alfalfa seed exudates and WSHM repressed the expression of expR in S. meliloti (Figure 4), suggesting that the inactivity of ExpR/Sin genes in nodules is a response of rhizobia to the host signal molecules. The repression of expR by host signal might due to the presence of L-canavanine in alfalfa seed exudates and L-canavanine could cause misfolding of the ExpR protein (Keshavan et al., 2005), while ExpR has been reported to possess the potential for self-regulation (Charoenpanich et al., 2013).
Water-soluble humic materials can stimulate S. meliloti growth by repressing QS. Although WSHM contain 52.18% C and 3.72% N (Gao et al., 2012) and humic acids could act as C or N source for bacteria growth (Tikhonov et al., 2010; Gao T.G. et al., 2015), only 0.09% of the increased biomass in WSHM treatment could be contributed to the C/N supply by WSHM in YM broth (Figure 2A). Thus, we conclude that WSHM increase S. meliloti 8530 growth mainly due to the regulatory effects on cell metabolism. The repression of the QS system in S. meliloti 8530 by WSHM could stimulate growth (Figures 2, 3), which supported the observation that QS restrains growth in S. meliloti, M. huakuii, R. leguminosarum, and Rhizobium sp. NGR234 (Wilkinson et al., 2002; He et al., 2003; Gao et al., 2006; Charoenpanich et al., 2015).
Water-soluble humic materials could regulate EPS synthesis, mainly decrease EPS II and MLG production, but do not affect EPS I production (Figures 2C,D). Amongst the three kinds of EPS produced by S. meliloti 8530 (Pérez-Mendoza et al., 2015), EPS I is the most efficient compound at initiating and maintaining infection threads (Jones, 2012). The probability of causing aberrant infection threads by EPS II, which is less efficient than EPS I at inducing infection thread formation, is 8–10 times higher than that of EPS I (Pellock et al., 2000). Furthermore, EPS synthesis in rhizobia is energy consuming; thus, EPS II is not required if EPS I is present (Zhan et al., 1989; Mithöfer, 2002). Even though MLG is involved in the attachment of S. meliloti to alfalfa roots, there was no significant difference in nodulation occupancy between a wild-type and a MLG synthesis deficient mutant (Pérez-Mendoza et al., 2015). Therefore, EPS II and MLG are not likely to be essential for efficient nodulation between S. meliloti and alfalfa. This notion is supported by the fact that the nodule occupancy of S. meliloti 1021 (ΔexpR) was 10–20% higher than that of S. meliloti 8530 (Charoenpanich et al., 2015). Thus, shutting down EPS II and MLG production with WSHM may promote symbiotic nitrogen fixation between S. meliloti and M. sativa. It is noteworthy that the ExpR/Sin QS system can regulate EPS II and MLG production (Mueller and González, 2010; Pérez-Mendoza et al., 2015), while EPS I biosynthesis becomes independent of QS in the absence of ExpR, and mutations to expR did not alter the amounts of EPS I produced (Glenn et al., 2007). This may be because MucR increases the production of EPS I independently of QS (Mueller and González, 2010). Thus, WSHM may regulate EPS production in S. meliloti 8530 through their regulation of the QS system.
Water-soluble humic materials improved symbiotic nitrogen fixation between M. sativa and S. meliloti (Table 1). Since WSHM did not affect the density of bacteroids in nodules (Supplementary Figure S4), the increase in nitrogenase activity of M. sativa following treatment with WSHM (Table 1) may be due to the enhanced expression of nifA gene via repressing expR expression. It has been reported that AHL-ExpR repress the expression of genes related to nitrogen fixation, such as fixTQK, which induce the expression of nifA (Hoang et al., 2004; Charoenpanich et al., 2013). In addition, the failed of significant increase in nodule number and nodule fresh weight of M. sativa by WSHM may result from the fact that nodule number and nodule weight are controlled by the plant host to ensure optimal growth (Mortier et al., 2012; Zipfel and Oldroyd, 2017). The promotion of nitrogen fixation between S. meliloti and M. sativa by WSHM offers an economical and efficient route for improving biological nitrogen fixation in agriculture. In a field experiment, WSHM treatment (500 mg/mL) on seeds (375 g WSHM per ha) increased alfalfa yield by 29% per year (unpublished data).
Conclusion
We identified QsrR as a direct repressor of expR (a gene central to the QS system in S. meliloti 8530) transcription. WSHM were proposed to repress expR expression by modulating the interaction between QsrR and the expR promoter, and ultimately decrease AHL, EPS II and MLG production. Consequently, WSHM increased the growth of S. meliloti, as well as symbiotic nitrogen fixation with M. sativa. In addition, both expR and sinR were down-regulated by WSHM with independent mechanisms. We hypothesize that QsrR may mediate the repression of expR expression by WSHM; however, the mechanism by which WSHM down regulate sinR expression requires further investigation.
Author Contributions
Y-YX, J-SY, CL, and H-LY conceived and designed the study. Y-YX, R-NW, and X-QQ performed the experiments. Y-YX and E-TW wrote the manuscript. Y-YX, and B-ZL participated in the preparation of water-soluble humic materials. H-LY and W-FC helped to design the experiments and drafted the manuscript.
Funding
This work was supported by the National Natural Science Foundation of China (Grant No. 31770541), the Special Fund for Agro-scientific Research in the Public Interest (Grant No. 201403048-2) and by the fund for Shanxi “1331 Project” Collaborative Innovation Center.
Conflict of Interest Statement
The authors declare that the research was conducted in the absence of any commercial or financial relationships that could be construed as a potential conflict of interest.
Acknowledgments
We thank Mengsheng Gao for providing S. melilotiΔsinI and ΔsinR mutant strains, Li Luo for providing strain S. meliloti 8530, Scot A. Wolfe and Zhi Chen for providing bacterial-one-hybrid system, and Jun Zhu for providing Agrobacterium tumefaciens KYC55 (pJZ372, pJZ384, and pJZ410) and R10 (pCF218). We would also like to thank Lijie Sun for the very helpful discussions.
Supplementary Material
The Supplementary Material for this article can be found online at: https://www.frontiersin.org/articles/10.3389/fmicb.2018.03194/full#supplementary-material
References
Bogino, P. C., Nievas, F. L., and Giordano, W. (2015). A review: quorum sensing in Bradyrhizobium. Appl. Soil Ecol. 94, 49–58. doi: 10.1016/j.apsoil.2015.04.016
Bourassa, D. V., Kannenberg, E. L., Sherrier, D. J., Buhr, R. J., and Carlson, R. W. (2017). The lipopolysaccharide lipid a long-chain fatty acid is important for Rhizobium leguminosarum growth and stress adaptation in free-living and nodule environments. Mol. Plant Microbe Interact. 30, 161–175. doi: 10.1094/MPMI-11-16-0230-R
Cai, T., Cai, W., Zhang, J., Zheng, H., Tsou, A. M., Xiao, L., et al. (2009). Host legume-exuded antimetabolites optimize the symbiotic rhizosphere. Mol. Microbiol. 73, 507–517. doi: 10.3390/genes9050263
Calatrava-Morales, N., McIntosh, M., and Soto, M. J. (2018). Regulation mediated by N-Acyl Homoserine Lactone quorum sensing signals in the Rhizobium-Legume symbiosis. Genes 9, 263. doi: 10.1111/j.1365-2958.2009.06790.x
Canellas, L. P., Olivares, F. L., Aguiar, N. O., Jones, D. L., Nebbioso, A., Mazzei, P., et al. (2015). Humic and fulvic acids as biostimulants in horticulture. Sci. Hortic. 196, 15–27. doi: 10.1016/j.scienta.2015.09.013
Chai, Y., Norman, T., Kolter, R., and Losick, R. (2010). An epigenetic switch governing daughter cell separation in Bacillus subtilis. Gene Dev. 24, 754–765. doi: 10.1101/gad.1915010
Charoenpanich, P., Meyer, S., Becker, A., and McIntosh, M. (2013). Temporal expression program of quorum sensing-based transcription regulation in Sinorhizobium meliloti. J. Bacteriol. 195, 3224–3236. doi: 10.1128/JB.00234-13
Charoenpanich, P., Soto, M. J., Becker, A., and McIntosh, M. (2015). Quorum sensing restrains growth and is rapidly inactivated during domestication of Sinorhizobium meliloti. Environ. Microbiol. Rep. 7, 373–382. doi: 10.1111/1758-2229.12262
Christensen, Q. H., Grove, T. L., Booker, S. J., and Greenberg, E. P. (2013). A high-throughput screen for quorum-sensing inhibitors that target acyl-homoserine lactone synthases. Proc. Natl. Acad. Sci. U.S.A. 110, 13815–13820. doi: 10.1073/pnas.1313098110
Dong, L., Yuan, Q., and Yuan, H. (2006). Changes of chemical properties of humic acids from crude and fungal transformed lignite. Fuel 85, 2402–2407. doi: 10.1016/j.fuel.2006.05.027
Gao, M., Tang, M., Guerich, L., Salas-Gonzalez, I., and Teplitski, M. (2015). Modulation of Sinorhizobium meliloti quorum sensing by Hfq-mediated post-transcriptional regulation of ExpR. Environ. Microbiol. Rep. 7, 148–154. doi: 10.1111/1758-2229.12235
Gao, M., Teplitski, M., Robinson, J. B., and Bauer, W. D. (2003). Production of substances by Medicago truncatula that Affect Bacterial Quorum Sensing. Mol. Plant Microbe Interact. 16, 827–834. doi: 10.1094/MPMI.2003.16.9.827
Gao, T. G., Jiang, F., Yang, J. S., Li, B. Z., and Yuan, H. L. (2012). Biodegradation of Leonardite by an alkali-producing bacterial community and characterization of the degraded products. Appl. Microbiol. Biotechnol. 93, 2581–2590. doi: 10.1007/s00253-011-3669-5
Gao, T. G., Xu, Y. Y., Jiang, F., Li, B. Z., Yang, J. S., Wang, E. T., et al. (2015). Nodulation characterization and proteomic profiling of Bradyrhizobium liaoningense CCBAU05525 in response to water-soluble humic materials. Sci. Rep. 5:10836. doi: 10.1038/srep10836
Gao, Y., Zhong, Z., Sun, K., Wang, H., and Zhu, J. (2006). The quorum-sensing system in a plant bacterium Mesorhizobium huakuii affects growth rate and symbiotic nodulation. Plant Soil 286, 53–60. doi: 10.1007/s11104-006-9025-3
Glenn, S. A., Gurich, N., Feeney, M. A., and Gonzalez, J. E. (2007). The ExpR/Sin quorum-sensing system controls succinoglycan production in Sinorhizobium meliloti. J. Bacteriol. 189, 7077–7088. doi: 10.1128/JB.00906-07
Gonzalez, J. E., and Keshavan, N. D. (2006). Messing with bacterial quorum sensing. Microbiol. Mol. Biol. Rev. 70, 859–875. doi: 10.1128/MMBR.00002-06
González, J. F., and Venturi, V. (2013). A novel widespread interkingdom signaling circuit. Trends Plant Sci. 18, 167–174. doi: 10.1016/j.tplants.2012.09.007
Gonzalez-Ortiz, G., Quarles Van, Ufford, H. C., Halkes, S. B., Cerda-Cuellar, M., Beukelman, C. J., et al. (2014). New properties of wheat bran: anti-biofilm activity and interference with bacteria quorum-sensing systems. Environ. Microbiol. 16, 1346–1353. doi: 10.1111/1462-2920.12441
Gurich, N., and Gonzalez, J. E. (2009). Role of quorum sensing in Sinorhizobium meliloti-Alfalfa Symbiosis. J. Bacteriol. 191, 4372–4382. doi: 10.1128/JB.00376-09
Hayes, M. H. B., and Wilson, W. S. (eds). (1997). Humic Substances, Peats and Sludges: Health and Environmental Aspects. Amsterdam: Elsevier.
He, X., Chang, W., Pierce, D. L., Seib, L. O., Wagner, J., and Fuqua, C. (2003). Quorum sensing in Rhizobium sp. Strain NGR234 regulates conjugal transfer (tra) gene expression and influences growth rate. J. Bacteriol. 185, 809–822. doi: 10.1128/JB.185.3.809-822.2003
Hense, B. A., and Schuster, M. (2015). Core principles of bacterial autoinducer systems. Microbiol. Mol. Biol. Rev. 79, 153–169. doi: 10.1128/MMBR.00024-14
Hoang, H. H., Becker, A., and Gonzalez, J. E. (2004). The LuxR homolog ExpR, in combination with the Sin quorum sensing system, plays a central role in Sinorhizobium meliloti gene expression. J. Bacteriol. 186, 5460–5472. doi: 10.1128/JB.186.16.5460-5472.2004
Jannin, L., Arkoun, M., Ourry, A., Laîné, P., Goux, D., Garnica, M., et al. (2012). Microarray analysis of humic acid effects on Brassica napus growth: involvement of N. C and S metabolisms. Plant Soil 359, 297–319. doi: 10.1007/s11104-012-1191-x
Jiang, F., Li, Z., Lv, Z., Gao, T., Yang, J., Qin, Z., et al. (2013). The biosolubilization of lignite by Bacillus sp. Y7 and characterization of the soluble products. Fuel 103, 639–645. doi: 10.1016/j.fuel.2012.08.030
Jones, K. M. (2012). Increased production of the exopolysaccharide succinoglycan enhances Sinorhizobium meliloti 1021 symbiosis with the host plant Medicago truncatula. J. Bacteriol. 194, 4322–4331. doi: 10.1128/JB.00751-12
Kalia, V. C. (2013). Quorum sensing inhibitors: an overview. Biotechnol. Adv. 31, 224–245. doi: 10.1016/j.biotechadv.2012.10.004
Keshavan, N. D., Chowdhary, P. K., Haines, D. C., and Gonzalez, J. E. (2005). L-Canavanine made by Medicago sativa interferes with quorum sensing in Sinorhizobium meliloti. J. Bacteriol. 187, 8427–8436. doi: 10.1128/JB.187.24.8427-8436.2005
Koul, S., Prakash, J., Mishra, A., and Kalia, V. C. (2016). Potential Emergence of Multi-quorum Sensing Inhibitor Resistant (MQSIR) Bacteria. Indian J. Microbiol. 56, 1–18. doi: 10.1007/s12088-015-0558-0
Krol, E., and Becker, A. (2014). Rhizobial homologs of the fatty acid transporter FadL facilitate perception of long-chain acyl-homoserine lactone signals. Proc. Natl. Acad. Sci. U.S.A. 111, 10702–10707. doi: 10.1073/pnas.1404929111
Luo, S., Sun, D., Zhu, J., Chen, Z., Wen, Y., and Li, J. (2014). An extracytoplasmic function sigma factor, σ25, differentially regulates avermectin and oligomycin biosynthesis in Streptomyces avermitilis. Appl. Microbiol. Biotechnol. 98, 7097–7112. doi: 10.1007/s00253-014-5759-7
Meng, X., Brodsky, M. H., and Wolfe, S. A. (2005). A bacterial one-hybrid system for determining the DNA-binding specificity of transcription factors. Nat. Biotechnol. 23, 988–994. doi: 10.1038/nbt1120
Mithöfer, A. (2002). Suppression of plant defence in rhizobia– legume symbiosis. Trends Plant Sci. 7, 440–444. doi: 10.1016/S1360-1385(02)02336-1
Mortier, V., Holsters, M., and Goormachtig, S. (2012). Never too many? How legumes control nodule numbers. Plant Cell Environ. 35, 245–258. doi: 10.1111/j.1365-3040.2011.02406.x
Mueller, K., and González, J. E. (2010). Complex regulation of symbiotic functions is coordinated by MucR and quorum sensing in Sinorhizobium meliloti. J. Bacteriol. 193, 485–496. doi: 10.1128/JB.01129-10
Nebbioso, A., and Piccolo, A. (2012). Advances in humeomics: enhanced structural identification of humic molecules after size fractionation of a soil humic acid. Anal. Chim. Acta 720, 77–90. doi: 10.1016/j.aca.2012.01.027
Nievas, F., Vilchez, L., Giordano, W., and Bogino, P. (2017). Arachis hypogaea L. produces mimic and inhibitory quorum sensing like molecules. Antonie van Leeuwenhoek 110, 891–902. doi: 10.1007/s10482-017-0862-2
Pellock, B. J., Cheng, H.-P., and Walker, G. C. (2000). Alfalfa root nodule invasion efficiency is dependent on Sinorhizobium meliloti polysaccharides. J. Bacteriol. 182, 4310–4318. doi: 10.1128/JB.182.15.4310-4318.2000
Pellock, B. J., Teplitski, M., Boinay, R. P., Bauer, W. D., and Walker, G. C. (2002). A LuxR homolog controls production of symbiotically active extracellular polysaccharide II by Sinorhizobium meliloti. J. Bacteriol. 184, 5067–5076. doi: 10.1128/JB.184.18.5067-5076.2002
Pérez-Montaño, F., Guasch-Vidal, B., González-Barroso, S., López-Baena, F. J., Cubo, T., Ollero, F. J., et al. (2011). Nodulation-gene-inducing flavonoids increase overall production of autoinducers and expression of N-acyl homoserine lactone synthesis genes in rhizobia. Res. Microbiol. 162, 715–723. doi: 10.1016/j.resmic.2011.05.002
Pérez-Montaño, F., Jimenez-Guerrero, I., Del Cerro, P., Baena-Ropero, I., Lopez-Baena, F. J., Ollero, F. J., et al. (2014). The symbiotic biofilm of Sinorhizobium fredii SMH12, ncessary for successful colonization and symbiosis of Glycine max cv Osumi, is regulated by quorum sensing systems and inducing flavonoids via NodD1. PLoS One 9:e105901. doi: 10.1371/journal.pone.0105901
Pérez-Mendoza, D., Rodríguez-Carvajal, M. Á., Romero-Jiménez, L., Farias, G. d. A., Lloret, J., Gallegos, M. T., et al. (2015). Novel mixed-linkage β-glucan activated by c-di-GMP in Sinorhizobium meliloti. Proc. Natl. Acad. Sci. U.S.A. 112, E757–E765. doi: 10.1073/pnas.1421748112
Savy, D., Canellas, L., Vinci, G., Cozzolino, V., and Piccolo, A. (2017). Humic-Like water-soluble lignins from giant reed (Arundo donax L.) display hormone-like activity on plant growth. J. Plant Growth Regul. 36, 995–1001. doi: 10.1007/s00344-017-9696-4
Schikora, A., Schenk, S. T., and Hartmann, A. (2016). Beneficial effects of bacteria-plant communication based on quorum sensing molecules of the N-acyl homoserine lactone group. Plant Mol. Biol. 90, 605–612. doi: 10.1007/s11103-016-0457-8
Staehelin, C., Forsberg, L. S., D’Haeze, W., Gao, M. Y., Carlson, R. W., Xie, Z. P., et al. (2006). Exo-oligosaccharides of Rhizobium sp. Strain NGR234 are required for symbiosis with various legumes. J. Bacteriol. 188, 6168–6178. doi: 10.1128/JB.00365-06
Suganuma, N., Sonoda, N., Nakane, C., Hayashi, K., Hayashi, T., Tamaoki, M., et al. (1998). Bacteroids isolated from ineffective nodules of Pisum sativum mutant E135 (syml3) lack nitrogenase activity but contain the two protein components of nitrogenase. Plant Cell Physiol. 39, 1093–1098. doi: 10.1093/oxfordjournals.pcp.a029307
Tikhonov, V., Yakushev, A., Zavgorodnyaya, Y. A., Byzov, B., and Demin, V. (2010). Effects of humic acids on the growth of bacteria. Eurasian Soil Sci. 43, 305–313. doi: 10.1134/S1064229310030087
Traversa, A., Loffredo, E., Gattullo, C. E., Palazzo, A. J., Bashore, T. L., and Senesi, N. (2013). Comparative evaluation of compost humic acids and their effects on the germination of switchgrass (Panicum vigatum L.). J. Soils Sediments 14, 432–440. doi: 10.1007/s11368-013-0653-y
Wilkinson, A., Danino, V., Wisniewski-Dye, F., Lithgow, J. K., and Downie, J. A. (2002). N-Acyl-homoserine lactone inhibition of rhizobial growth is mediated by two quorum-sensing genes that regulate plasmid transfer. J. Bacteriol. 184, 4510–4519. doi: 10.1128/JB.184.16.4510-4519.2002
Zhan, H., Levery, S. B., Lee, C. C., and Leigh, J. A. (1989). A second exopolysaccharide of Rhizobium meliloti strain SU47 that can function in root nodule invasion. Proc. Natl. Acad. Sci. U.S.A. 86, 3055–3059. doi: 10.1073/pnas.86.9.3055
Zheng, H., Zhong, Z., Lai, X., Chen, W. X., Li, S., and Zhu, J. (2006). A LuxR/LuxI-type quorum-sensing system in a plant bacterium, Mesorhizobium tianshanense, controls symbiotic nodulation. J. Bacteriol. 188, 1943–1949. doi: 10.1128/JB.188.5.1943-1949.2006
Zhu, J., Chai, Y., Zhong, Z., Li, S., and Winans, S. C. (2003). Agrobacterium bioassay strain for ultrasensitive detection of N-acylhomoserine lactone-type quorum-sensing molecules: detection of autoinducers in Mesorhizobium huakuii. Appl. Environ. Microbiol. 69, 6949–6953. doi: 10.1128/AEM.69.11.6949-6953.2003
Keywords: Sinorhizobium meliloti, quorum sensing, humic materials, ExpR regulator, bacterial communication
Citation: Xu Y-Y, Yang J-S, Liu C, Wang E-T, Wang R-N, Qiu X-Q, Li B-Z, Chen W-F and Yuan H-L (2018) Water-Soluble Humic Materials Regulate Quorum Sensing in Sinorhizobium meliloti Through a Novel Repressor of expR. Front. Microbiol. 9:3194. doi: 10.3389/fmicb.2018.03194
Received: 03 September 2018; Accepted: 10 December 2018;
Published: 21 December 2018.
Edited by:
Hon-Ming Lam, The Chinese University of Hong Kong, ChinaReviewed by:
Muthu Venkateshwaran, University of Wisconsin–Platteville, United StatesYangrong Cao, Huazhong Agricultural University, China
Copyright © 2018 Xu, Yang, Liu, Wang, Wang, Qiu, Li, Chen and Yuan. This is an open-access article distributed under the terms of the Creative Commons Attribution License (CC BY). The use, distribution or reproduction in other forums is permitted, provided the original author(s) and the copyright owner(s) are credited and that the original publication in this journal is cited, in accordance with accepted academic practice. No use, distribution or reproduction is permitted which does not comply with these terms.
*Correspondence: Hong-Li Yuan, aGx5dWFuQGNhdS5lZHUuY24=; eXVhbmFlbW9pbEBjYXUuZWR1LmNu