- 1Natural Drug Research Laboratory, Department of Biotechnology, School of Biosciences, Periyar University, Salem, India
- 2Biomedical Zebrafish Laboratory, Department of Medical Biotechnology, Faculty of Allied Health Sciences, Chettinad Academy of Research and Education, Chennai, India
- 3Marine Biotechnology and Ecological Genomics Laboratory, Department of Biotechnology, School of Biosciences, Periyar University, Salem, India
Fungal metabolites are considered to be most efficient tools to overcome the issues related to insecticide resistance and environmental pollution. The present study focus on the evaluation of the mosquito larvicidal efficacy of metabolites of seven indigenous fungal isolates (Penicillium sp. Aspergillus niger, A. flavus, A. parasiticus, Rhizopus sp. Mucor sp. and Aspergillus sp.) on the larvae of Aedes aegypti and Culex quinquefasciatus under the laboratory condition. The preliminary screening of the isolate, Penicillium sp. showed better larvicidal effect when compared to other fungi. The fungus was grown on Potato Dextrose Broth (PDB) in the laboratory (at 25∘C) and maintained in the relative humidity (at 76 ± 4% for 15 days). Larvicidal potency of mycelial ethyl acetate extract (MEAE) of Penicillium sp. was performed against 1st to 4th instars larvae of Ae. aegypti and Cx. quinquefasciatus using four different concentrations (100, 200, 300, and 500 μg/ml) that showed better larval mortality values (μg/ml) of LC50 = 6.554, 5.487, 6.874, 6.892, and the LC90 = 11.486, 10.366, 12.879, 13.865 for Ae. aegypti and LC50 = 7.000, 13.943, 18.129, 25.212 and the LC90 = 12.541, 23.761, 30.923, 41.696 for Cx. quinquefasciatus. Exposure of metabolite to larvae resulted in behavior changes i.e., excitation, up and down with aggressive movement, anal papillae biting behavior. Further, the larvae treated with Penicillium sp. metabolite exhibited significant reduction in the levels of acetylcholinesterase. The 4th instar mosquito larvae treated with the 500 μg/ml mycelia extract showed severe histological damages. During the antibacterial analysis of Penicillium sp.- mycelium the maximum growth inhibition zone was recorded in Shigella dysenteriae (31.2 mm) and Klebsiella pneumoniae (31.1 mm) followed by others. In addition, to check the toxicity of Penicillium sp. MEAE against embryos of Zebrafish, a model system, using different concentrations of metabolites (1.0, 0.5, 0.125 mg/ml, 30, 3.0, and 0.5 μg/ml) and life-stage parameters were observed at 124 hpf. Furthermore, the Fourier Transformed Infrared and GCMS spectrum analysis of mycelium reflected several chemical compounds. The outcome of the study clearly shows that Penicillium sp. metabolites could serve as an ideal eco-friendly, single-step and inexpensive source for the control of Ae. aegypti and Cx. quinquefasciatus larvae.
Introduction
Globally, mosquitoes are one of most life threatening insects, as they are major vectors for the cause of several diseases (Ghosh et al., 2013; Benelli et al., 2016; Benelli and Romano, 2017; Ward and Benelli, 2017). The dengue vector, Aedes aegypti, distributed in tropical and sub-tropical regions of the world has the potential to transmit several viruses that cause Zika, dengue, chickungunya and yellow fever (Reegan et al., 2015; Benelli et al., 2017a,b). Another mosquito, Culex quinquefasciatus (Say) belonging to the family, Culicidae is predominantly found in the tropical and temperate regions (Bernhard et al., 2003). This mosquito could transmit the Lymphatic filariasis to humans and which also plays an important role in the transmission of West Nile Virus (Tsai and Mitchell, 1989; Reuben et al., 1994).
Approximately, 390 million people are now at risk of the dengue fever (WHO, 2011). Another (Brady et al., 2012) study proved the prevalence of dengue and they estimate that 3.9 billion peoples in 128 countries are at risk of infection with dengue virus. Wuchereria bancrofti, the lymphatic dwelling parasite infected about 90 million people worldwide (Kovendan et al., 2009). 40% of the filarial cases occur in India which results in an annual economic loss of about 720 crores (Hotez et al., 2004).
For the management of mosquitoes and houseflies we mainly depend on the application of synthetic and commercial chemicals viz; organochlorines, organophosphates, carbamates, Dichloro Diphenyl Trichloroethane’s (DDT) and others, especially to control their increased resistance and to arrest the severe side effects (Wood, 2003; Harzsch and Hafner, 2006; Benelli, 2015a,b; Naqqash et al., 2016). Moreover, the cost of synthetic pyrethroids are very high and they cause environmental and food safety issues besides they affect non-target organisms (Mittal and Subbarao, 2003; Pates and Curtis, 2005; Nathan et al., 2006; Benelli, 2015b). More intensive researches have been recently carried out to control mosquito vectors in an holistic way (Singh et al., 2006). Hence, the scientists from across the world, have been searching for an alternative method for vector control (Vyas et al., 2007). The utilization of natural products or metabolites from microbial resources is a possible alternative way, which is environmentally safe, biodegradable, low cost larvicidal agent for vector control (Killeen et al., 2002; Scholte et al., 2005). Fungal based products have been reported as highly toxic to mosquitoes, with less side effects to non-target organisms (Kirschbaum, 1985). Generally, microbial insecticides are considered as alternative to chemical insecticides, because of their selective toxicity, decomposability, and eco-friendly nature (Misato, 1983; Shaalan et al., 2005). Mycelial extract of various fungi have been reported for their larvicidal, cellulolytic and cytotoxic activity (Valentin Bhimba et al., 2011; Zhang et al., 2013; Fahd, 2018). The most commonly known fungal species which possess significant larvicidal activity are; Penicillium sp. Aspergillus sp. Fusarium sp. Podospora sp. Mucor sp. Cladosporium sp. and Stoloniferum sp. (Dennis et al., 2013).
The increasing prevalence of antibacterial resistance among microbes against commercial antibiotics has become a major health issue globally. Although various new antibiotics were developed during recent decades but none of them found effective on multidrug-resistant bacteria (Mohanty et al., 2012). Therefore, an intensive search is needed to treat Gram-positive and negative human pathogens. Fungi are being considered as best sources of bioactive metabolites (Schulz et al., 2002; Strobel, 2003) as they could possess significant antimicrobial agents (Chomcheon et al., 2005; Li et al., 2005; Phongpaichit et al., 2007; Weber et al., 2007).
The zebrafish (Danio rerio) is a well-established vertebrate model organism for testing the toxicity of metabolites or nanoparticles toxicant (Deng et al., 2009). The characteristic features like maximum fecundity, controlled and visible embryological phase or internal organs and reactive to toxicants have been the advantages of zebrafish embryos as to use it as model organism in the field of toxicology and pharmacology (Hallare et al., 2006; Chandrasekar et al., 2011; López-Romero et al., 2012; Xu et al., 2014; Yang et al., 2014; Schmidt et al., 2015). Interestingly, the genetic composition of zebra fish is similar to human and approximately 84% of gene associated with human diseases (Howe et al., 2013; Truong et al., 2014). In view of the above, the present study was designed to isolate and identify the potent indigenous soil fungi to evaluate their metabolites larvicidal and histopathological effects on mosquitoes.
Materials and Methods
Chemicals and Reagents
The necessary chemical/reagents like Potato Dextrose Agar (PDA), PDB, Muller Hinton Agar (MHA), Nutrient broth (NB), Lactophenol cotton blue stain, acetylthiocholine iodide (AChEI), fast blue B, Sodium dodecyl sulfate (SDS), 5,5-dithiobis-2-nitrobenzoic acid (DTNB), and 10% Dimethyl sulfoxide (DMSO) were purchased from Hi-Media, Mumbai, India. The deionized water was used throughout the bioassay. All the glasswares were washed with diluted nitric acid (HNO3), followed by distilled water and dried in hot air oven.
Collection of Soil Sample
The soil samples were collected from the Karumandurai hills, Salem District Tamil Nadu, India (Latitude 78°20′E and Longitude 11°45′N). Soil samples (approximately 100 g) were aseptically collected (about 5–7 cm depth) using sterile polyethylene bags and brought to the laboratory. Then, it was stored in refrigerator at 4°C until further use.
Isolation of Fungi by Serial Dilution Method
Fungal isolation was done on PDA medium enriched with antibiotic (Chloramphenicol 1 mg/ml) to arrest the bacterial growth. The culture medium was poured on petri plates and allowed to solidify (Apinis, 1963). Serial dilution of the soil sample (up to 10−6 dilution) was carried out using sterile distilled water. To enhance the fungal isolation, sterile pipette was used, 30% of dilution was taken and introduced into the surface of the agar medium and uniformly spread out using sterile L-rod. All the PDA plates were incubated (for 3 days) at room temperature (27 ± 1.5°C) for fungal growth. The growth of various fungal colonies were observed on each plate and they were then isolated separately by the modified plating method of Durowade et al. (2007).
Point Inoculation Method
The isolated fungi were purified by adopting point inoculating method on plates containing PDA medium and each fungus was purified by repeating the step. Pure form of the isolated fungi was confirmed by microscopic examination of the culture (at 40× magnification) through light microscope. Further, the fungal cultures were sub-cultured on PDA slants incubated for a period of 5–7 days and the cultures were stored at 4°C (Gilman, 1957).
Macroscopic and Microscopic Characteristics
On the basis of colony morphology and microscopic characteristics, the fungal cultures were stained using a Lacto phenol cotton blue (LCB) stain (Madavasamy and Panneerselvam, 2012). The stained cultures were observed under microscope and the isolated fungi identified using staining method and also checked with available fungal identification manual (Gilman and Abbott, 1957). The following morphological characteristics were taken for identification i.e., colony growth (length and width), occurrence of aerial mycelium, colony color, presence of wrinkles and furrows and pigment production etc. (Abutaha et al., 2015).
Mycelial Metabolite Preparation
The mycelial mats of the isolated fungi were inoculated into PDB, and incubated for 3 weeks under dark condition. Once the maximal growth was obtained, the fungal mycelial mat was harvested by filtration. The fungal mycelium (10 g) was extracted with 100 ml of ethyl acetate in static condition for 5 days. The mixture was filtered through muslin cloth and the extraction steps were repeated for 3 times. The concentrated mixture was centrifuged at 12,000 rpm for 5 min to remove the unwanted debris. Then, the mixture was transferred to a round bottom flask and dried under rotary evaporator at (40°C), and stored at −20°C until further use (Belofsky et al., 2000; Josphat et al., 2011).
Collection, Maintenance and Larvicidal Activity of Mosquitoes
The test mosquito larvae were collected from agricultural fields, Karuppur Panchayat, Salem and the larvae were identified as per the mosquitoes key manual (Rueda, 2004; Tyagi et al., 2012). Then, they were maintained in the Natural Drug Research Laboratory (NDRL) under the temperature of 25 ± 2°C, relative humidity at 75 ± 2% and photoperiod of 14:10 (L/D). The mycelial extract was prepared in defined concentrations (100, 200, 300, and 500 μg/ml) and tested for larvicidal activity against targeted mosquitoes (Ragavendran et al., 2017b). Mortality and survival rate was recorded after 24 h of the exposure. During the experimental period, no food was provided to the larvae. All the experiments were performed in thrice to validate results. All test containers were tightly covered with mosquito net and kept at room temperature (avoiding exposure of sunlight) and the dead larvae were counted (WHO, 2005).
Dose-Response Bioassay
For dose response bioassay, Ae. aegypti and Cx. quinquefasciatus larvae were taken in beakers containing sterile deionized water. Then, various concentrations of metabolites were prepared using 100 ml water. The mycelial metabolite was dissolved in 10% DMSO for prepared at 1 mg/ml concentration (stock solution 5 ml) and bioassays experiment was performed using different concentrations (100, 200, 300, and 500 μg/ml) of metabolites from mycelium extract (Chandrasekar et al., 2011). The negative control of each experiment (treated with DMSO-distilled water) was tested for three times. The mortality (using Abbott’s formula) and survival rate was determined after 24 h of the exposure (Abbott, 1925). All the test containers were kept at room temperature without any disturbances. The LC50 and LC90 values of exposed larvae were calculated using probit analysis (Finney, 1971).
Behavioral Studies in Metabolites Against Ae. aegypti and Cx. quinquefasciatus Larvae
In larvicidal bioassay, the larvae were observed carefully for behavioral changes i.e., wriggling speed, horizontal and vertical movements and self-biting behaviors. The larval behavior symptoms were recorded and photographed with Nikon D-SLR Digital Camera (Nikon Inc., Japan). Similarly, control larvae were also observed and compared with treated larvae (Warikoo and Kumar, 2013).
Preparation of Whole Body Homogenates
The Penicillium sp. extract treated 4th instar larvae were washed with sterile distilled water, and dried with the help of blotting tissue paper. Then, it was homogenized in eppendorf tubes using a mortar and pestle by adding l ml of ice-cold sodium phosphate buffer (20 mM, pH 7.0) for esterases activity. The whole body homogenates were centrifuged (8000 rpm; 4°C) for 15 min, and the clear supernatants were used to perform the enzyme assay.
Acetylcholinesterase Assay
For this assay, the larval homogenate supernatants were measured by spectrophotometer (at 412 nm) by following the modified method of Ellman et al. (1961) and the acetylthiocholine iodide was served as substrate (Ikezawa and Taguchi, 1981). Acetylcholinesterase activity inhibition was calculated by the formula:
Acontrol and A sample = the absorbance of the control sample.
Morphology and Histopathology Study
The 4th instar larvae of the test mosquitoes were treated with 500 μg/ml of Penicillium sp. mycelia metabolites. The morphological and behavioral changes of the treated larvae were identified using Labomed microscope (at 40×). The dead larvae were mounted on a microscopic slide with Hoyer’s medium and observed under the microscope (Kamalakannan et al., 2014). The control and treated larvae were fixed with 10% formalin, dried out using ethyl alcohol series, cleaned by xylene and fixed by using paraplast and taken section (7 μm). The sectioned larvae and control samples were stained with eosin and hematoxylin, respectively by adopting standard staining protocols (Kaewnang-O et al., 2011; Feldman and Wolfe, 2014). The mid-guts of control and treated larvae were visualized through microscope (Labomed) and photographed.
Antibacterial Activity Test
Collection and Maintenance of Broth Culture
Two Gram-positive (Staphylococcus aureus MTCC 737 and Enterococcus faecalis) and five Gram-negative (Escherchia coli MTCC 443, Salmonella typhi MTCC 3224, Shigella boydii MTCC 11947, Shigella dysentriae and Klebsiella pneumoniae MTCC 4030) were procured from the Microbial Type Culture Collection (MTCC), Institute of Microbial Technology, Chandigarh, India. The bacteria cultures were maintained in NA slants (at 4°C) and served as stock cultures. The selected bacteria were inoculated into MHB and incubated at 37°C and 190 rpm for 10–14 h. The turbidity of the resulting suspension was diluted in MHB and matched with 1 McFarland turbidity standard. The resultant level of turbidity was diluted with MHB and is equivalent to approximately 3.0 × 10−8 CFU/mL (0.5 MacFarland standards).
Agar Well Diffusion Method
The antibacterial activity of MEAE of Penicillium sp. was evaluated by agar diffusion method (Thornberry, 1950). Approximately, 25 ml of MHA medium was poured in the sterilized Petri dishes. The selected bacterial cultures were grown in MHB for 24 h. The broth culture of each bacterium (100 μl) was uniformly spread on the each plate, using sterile cotton swabs. Under aseptic condition, wells (6 mm) were made using sterilized stainless steel cork borer. The Penicillium sp. MEAE and negative control (solvent only) was dissolved in 10% aqueous DMSO, obtained different concentrations (50, 75, and 100 μg/ml) and they were loaded on marked wells under aseptic conditions with the help of micropipette, then the plates were incubated at 37°C for 24 h. The antibiotic Chloramphenicol (4 μg/ml) was served as positive control. The growth inhibition zone was measured using a ruler and maintained the values in millimeter (mm) as mean ± SD (Prabhu et al., 2010).
Fish Maintenance and Breeding
The fishes were maintained at the temperature of 27 ± 2°C with a 14:10 h of light and dark (photoperiod cycle) in 100 L tanks as per the OECD guidelines as well as the modified protocols of Unai Vicario-Pares et al. (2014). The fish embryos were obtained for spawning the adults Danio in the breeding tanks with the sex ratio of 3:5 (3 male:5 female) and the spawning was induced in the morning and after 2 h, embryos were collected and washed using embryo medium. The collected embryos (with help of Pasteur pipettes) and fertilized viable eggs were selected using a stereoscopic microscope (Nikon smz800, Kanagawa, Japan).
Acute Toxicity Test of Penicillium sp. on Zebra Fish
The acute toxicity test of Penicillium sp. on zebrafish was performed by following the modified FET test (OECD, 2006, 2013). Fertilized embryos were treated with different concentrations of mycelia extracts (1.0, 0.5, 0.125 mg/ml, 30, 3.0, and 0.5 μg/ml), prepared from the stock solution (1 mg/L), for 4– 124 hpf. The control fish (with solvents) were also maintained in an embryonic medium with 0.1% DMSO and additional sets of control (without solvent and toxicant) was taken. Then, the embryos were incubated at a temperature of 26.0°C ± 1.0°C with 14:10 (Light:Dark) cycle and monitored at respective time intervals (24, 48, 72, and 96 h). During the treatments, the heartbeat, hatching rate, percentage of survival and body length of fish were noticed using an inverted microscope (Nikon TE2000-U) (Manjunatha et al., 2018). Each test was done three times for obtaining accurate results.
Fourier Transformed Infrared Spectroscopy (FTIR)
The dried powdered MEAE of Penicillium sp. was subjected to FTIR spectrometer analysis through scanning it in the range 400 to 4000 cm−1 at a resolution of 4 cm−1. These measurements were performed on a Bruker Optics (Germany-made) Tensor 27 model in the diffuse reflectance mode operated at a resolution of 0.4 cm−1 in KBr pellets. In brief, 500 mg of sample was mixed with 300 mg of potassium bromide (KBr) powder and pelletized (3 mm dm). The pellets were analyzed through FTIR spectroscopy measurement (Vivek et al., 2012).
Gas Chromatography–Mass Spectroscopy (GC–MS)
The GC–MS spectrum of Penicillium sp. MEAE was performed in electron ionization (EI) mode on a GC–MS, PerkinElmer, Turbo mass gold, GC model 680 (Boesch, Huenenberg, Switzerland) system. The details of instrumental working condition and sample loading procedure was done as explained by Ragavendran and Natarajan (2015). The bioactive components of the mycelia extract interpreted based on the obtained mass spectra, which were then compared with the data already available in the National Institute of Standards and Technology (NIST) libraries (Devi and Singh, 2013).
Statistical Analysis
The LC50, LC90% confidence limits of upper and lower confidence limits, and chi-square values of mortality data were calculated using Probit analysis and the Statistical Package of Social Sciences IBM 20.0 software (SPSS Inc., Chicago, IL, United States). All the obtained results were considered to be statistically significant, at the larval of P < 0.05. Acetylcholinesterase inhibition activity measurements were made in triplicates and the ±SD by Graph Pad Prism 5.0 software program.
Results
Isolation of Fungi
Totally 7 different fungi were isolated from the soil samples by serial dilution method and the isolated fungi were purified using a point inoculation technique. The fungi were preliminarily identified by using the microscopic (40×), morphological characters and soil fungal identification key manual (Gilman and Abbott, 1957) and the salient features of all the isolates have been depicted in Table 1. Based on the above results (microscopy and morphological features), the isolated fungi have been identified as: Penicillium sp. A. niger, A. flavus, A. parasiticus, Rhizopus sp. Mucor sp. and Aspergillus sp. Then, the Penicillium sp. was sub-cultured in the PDA media, for preparation of pure culture and used for further study.
Preliminary Larvicidal Activity
The mycelia extract of the isolated fungi were screened preliminarily and secondary metabolites were tested for larvicidal activity against 1st to 4th instar Ae. aegypti and Cx. quinquefasciatus larvae. The fungal MEAE of Penicillium sp. exhibited superior toxicity against Ae. aegypti 3rd instar larvae with LC50 value of 6.874 and LC90 = 12.879 μg/ml after 24 h treatment, followed by A. niger (LC50 = 39.293 and LC90 = 87.65 μg/ml), A. flavus (LC50 = 46.381 and LC90 = 110.791 μg/ml), A. parasiticus (LC50= 50.228 and LC90 = 147.023 μg/ml), Rhizopus sp. (LC50= 56.062 and LC90 = 210.908 μg/ml), Mucor sp. (LC50 = 108.363 and LC90 = 464.026 μg/ml), Aspergillus sp. (LC50 = 238.273 and LC90 = 962.642 μg/ml). The isolated fungal mycelia metabolites treated larvae exhibited mortality rate of following order: Aspergillus sp. > Mucor sp. > Rhizopus sp. > A. parasites > A. flavus > A. niger > Penicillum sp. The Penicillium sp. only revealed higher larvicidal activity when compared with the other fungi and hence it was selected for further larvicidal tests.
Larvicidal Activity of Potential Fungus
The larvicidal effect of fungal MEAE prepared from Penicillium sp. has shown excellent activity against 1–4th instar larvae of the Ae. aegypti (Table 2) and the larval mortality was noticed after 24 h and the control did not show nil mortality. The metabolite treated 1, 2 and 4th instar larvae (of Ae. aegypti) revealed high effect at the maximum concentration (500 μg/ml), and the mortality started within 8 h of exposure. More than 50% larval death occurred within the 12 h. Similar effect was observed against Cx. quinquefasciatus at a dose of 500 μg/ml. Similarly, 2nd instar larvae also affected at 300 μg/ml concentration where mortality occurred 100% after 24 h post-treatment. The lowest concentration of metabolite arrested the larval growth and development. Overall, we obtained the Penicillium sp. mycelia metabolite was exhibited the results in dose and time dependent death rate.
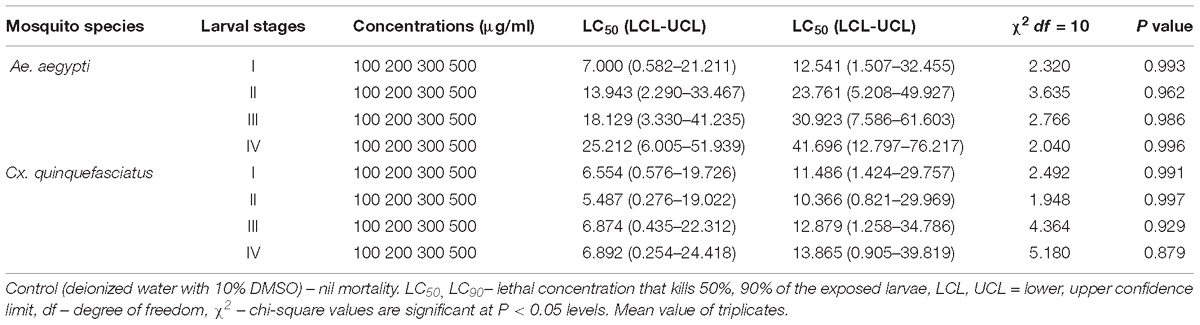
Table 2. Larvicidal activity of MEAE of Penicillium sp. against Ae. aegypti and Cx. quinquefasciatus.
Neurobehavioral Toxicity
The Penicillum sp. mycelia metabolites have exerted neurobehavioral toxicity on 4th instar larvae of the tested mosquitoes (Figures 1a,c). The control larvae have shown normal behaviors and after 30 min exposure of extract, the larvae have exhibited energetic and restless movement with an increased exposure periods i.e., unnatural restlessness and excitation, movements was altered, forceful self bite of anal papillae and mouth parts leading to the development of circle-shaped structures (Figures 1b,d). It was noticed that these continuous behaviors and orientation symptoms of treated larvae and larvae became more irritated and noticed their up and down wriggling movements and several larvae exhibited vibrating movements and paralytic symptoms. Morphological changes were noticed in the treated larvae with damaged anal papillae area and the cuticle layers (Figure 2).
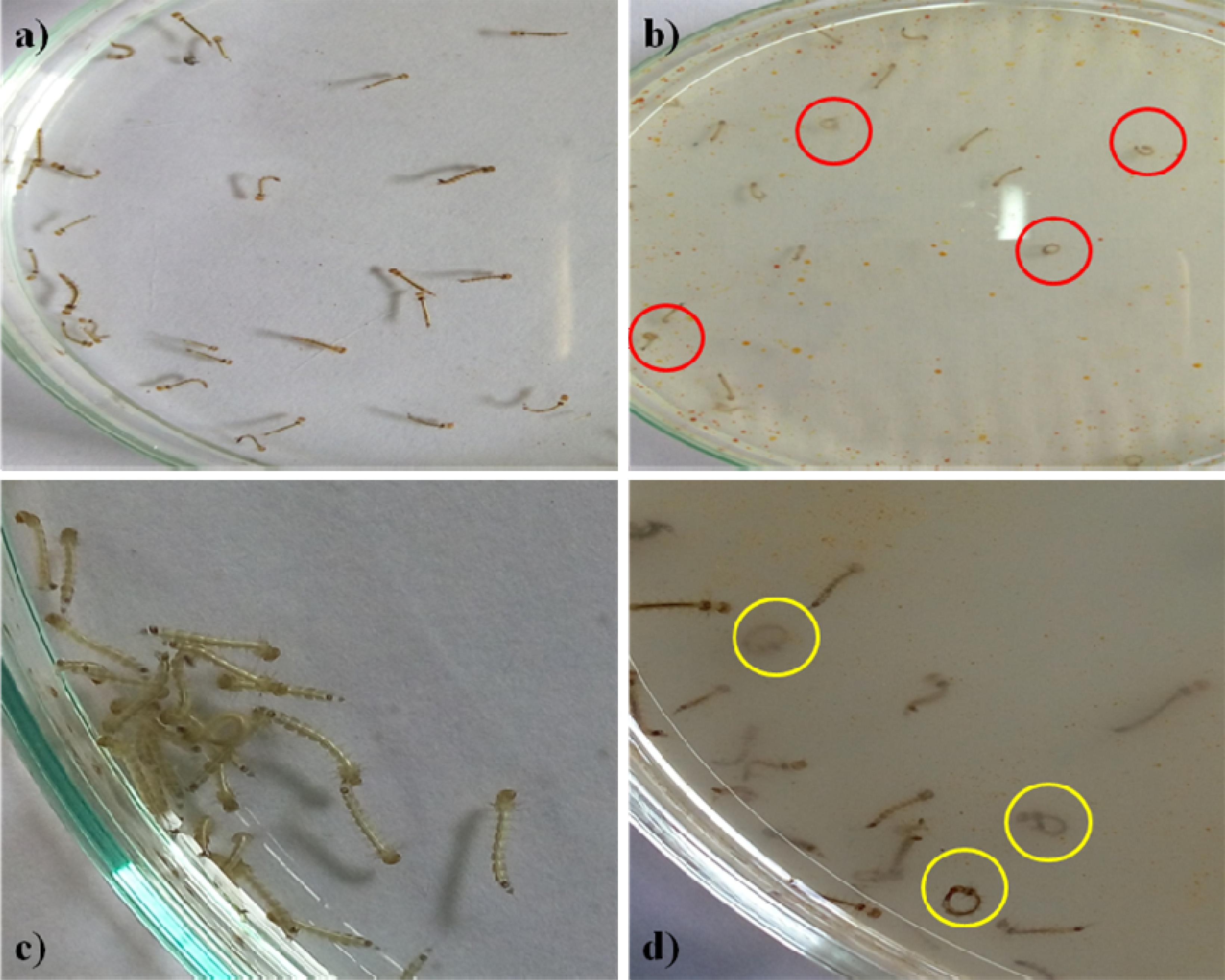
Figure 1. Fourth instar larvae (a). Control Aedes aegypti (b). Treated larvae (500 μg/ml) (c). Control Culex quinquefasciatus (d). Treated with Penicilluim sp. metabolite (500 μg/ml). The red and yellow circle indicates the aggressive anal gill biting behavior.
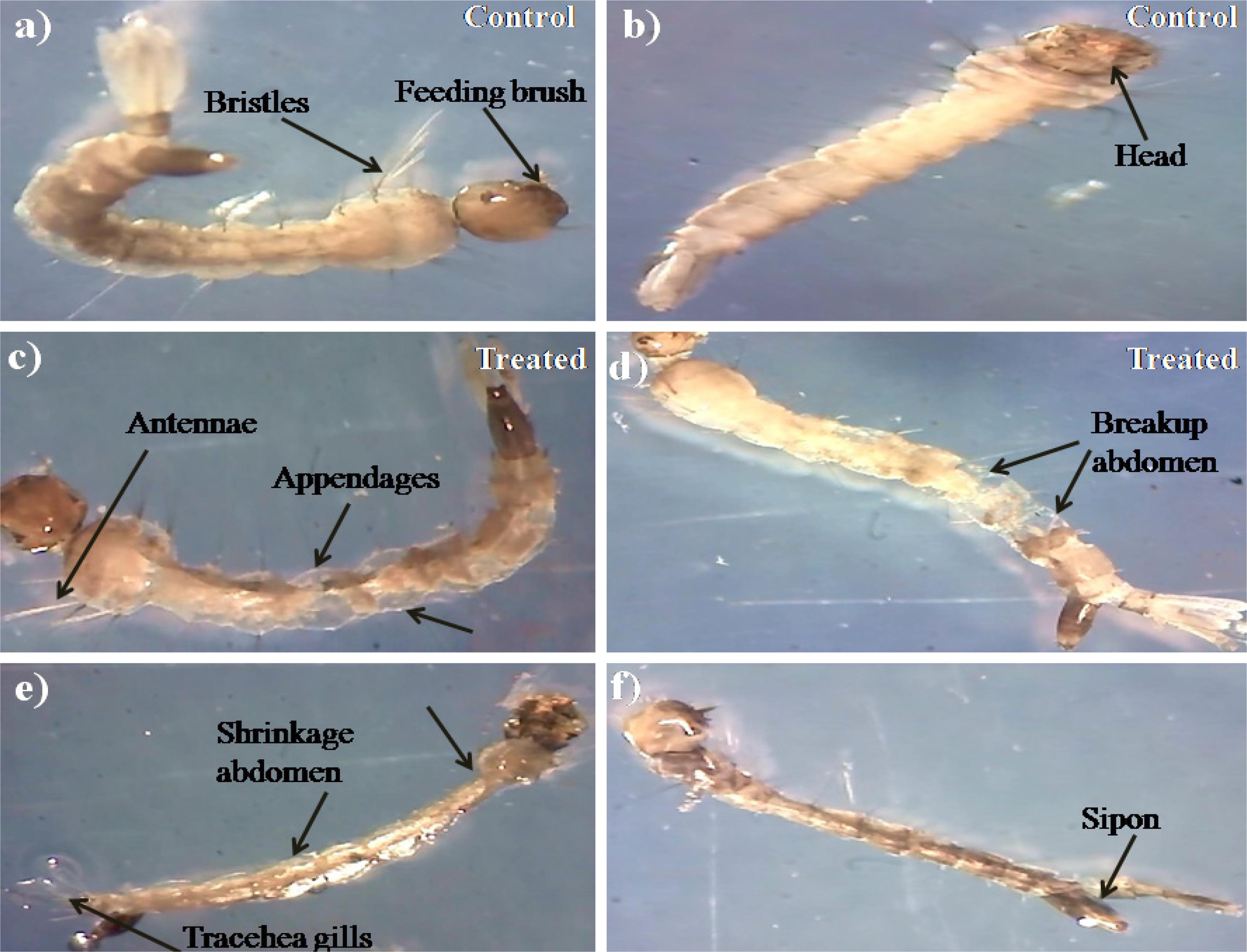
Figure 2. Stereo microscopic view of 4th instar (a,b) control larvae of Ae. aegypti and Cx. quinquefasciatus of midgut, thorax, and anal gill parts. (c–f) Ae. aegypti and Cx. quinquefasciatus treated with Penicillium sp. mycelium extract at 500 μg/ml concentration and extract treatment induced toxic effects on many regions of the body (including thorax, abdomen, anal gills, (black arrows) indicates loss of external hairs, crumbled epithelial layer of the outer cuticle and shrinkage of the larvae).
Acetylcholinesterase Inhibition Test of 4th Instar Larvae
Presently, the Penicillium sp. metabolites exposed to 4th instar larvae of Ae. aegypti caused reduced AchE level (9.35 ± 0.2 to 7.3 ± 0.7 μM/min/mg larval protein; F4 = 311.93; P < 0.05). Similar trend also observed in Cx. quinquefasciatus larval (11.01 ± 0.4 to 8.1 ± 0.4 μM/min/mg larval protein; F4 = 186.26; P < 0.05) (Figure 3). At the concentration of 100 μg/ml metabolites, the AchE activity was notably inhibited and the maximum inhibition was observed at 500 μg/ml. The untreated larva has shown only enzyme. The enzyme inhibition activity results clearly confirmed the dose dependent approach of metabolites. A post hoc Tukey’s HSD test showed significant difference in the inhibition rates based on the concentration of metabolite. A maximum inhibition was observed in Ae. aegypti larvae than the Cx. quinquefasciatus.
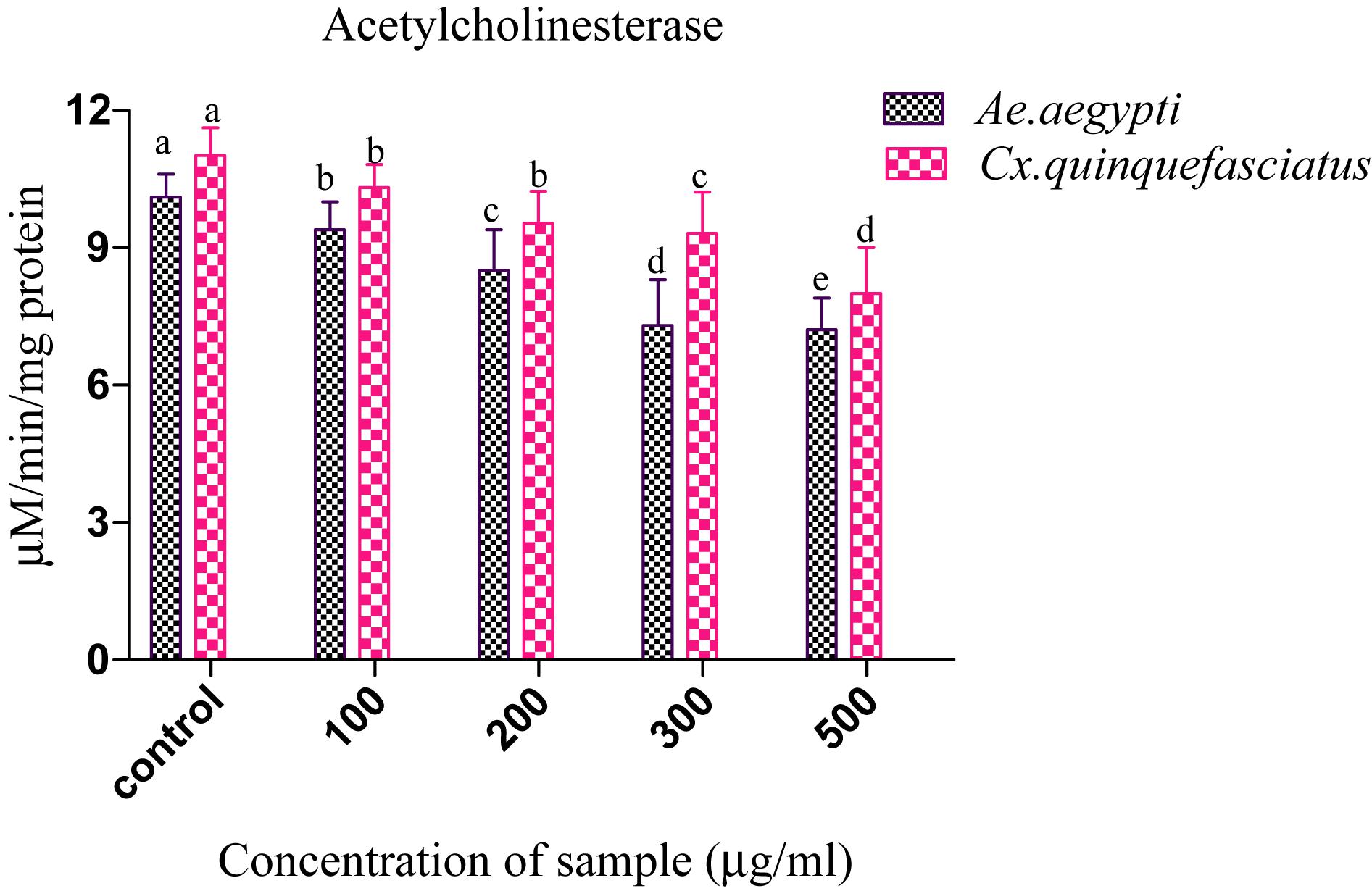
Figure 3. Acetylcholinesterase (AchE) inhibition of Ae. aegypti and Cx. quinquefasciatus after treatment with Penicillium sp. Statistical values followed by the same letter are not significantly differences according to Tukey’s HSD test at P < 0.05 (one way ANOVA).
Histopathological Study
During histopathological study, Ae. aegypti treated larvae showed histological alterations in the digestive tract, midgut, cortex with hyperplasia of gut epithelial cells, collapsed brush border, broken membranes and also observed cytoplasmic masses (Figure 4b). On the other hand the, Cx. quinquefasciatus larvae showed complete disintegration of abdominal region especially the mid-gut and caeca, and the epithelial layer was disorganized with most of the cells disappeared (Figure 4d). Both the untreated larvae exhibited the midgut epithelium with a single layer of digestive cells exhibiting well developed brush border, cell membrane and cytoplasm regions (Figures 4a–c).
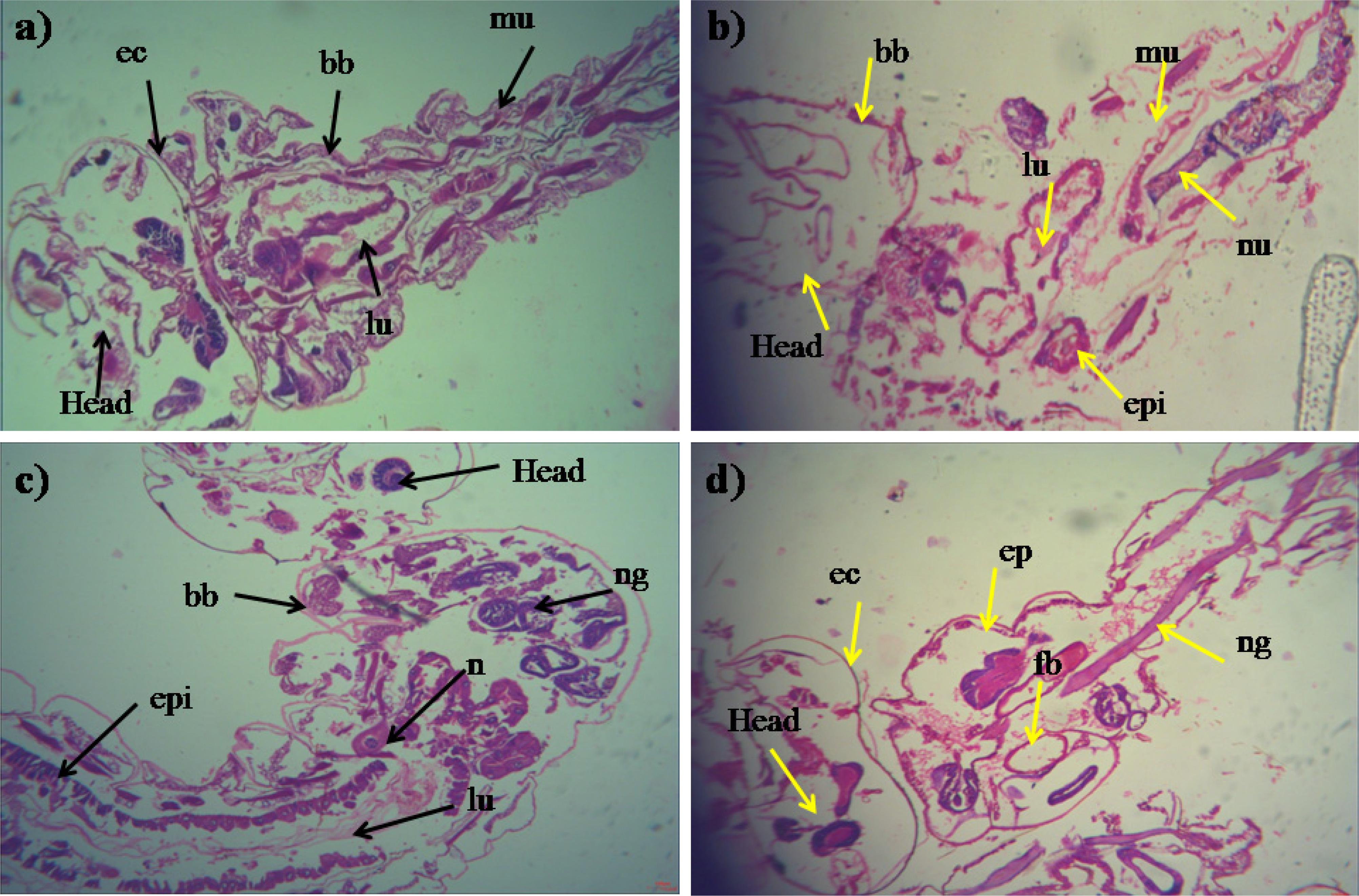
Figure 4. Histopathology profiles of treated 4th instar larvae of Ae. aegypti and Cx. quinquefasciatus: (a–c) Control, (b–d) treated with the Penicillium sp. MEAE (500 μg/ml), Black arrow represents control larvae structures parts, yellow arrow indicates the treated gut epithelium (epi) and muscles (mu), a nerve ganglion (ng), intact neuropile (nu), damaged gastric (enteric) caeca (gc), brush border (bb), peritrophic matrix (pm) and gut lumen (lu). Magnification is 200×, respectively.
Antibacterial Activity
Presently, antibacterial activity of Penicillium sp. MEAE was tested against 7 Gram-positive and negative bacterial pathogens with 3 different concentrations (50, 75, and 100 μg/ml) of metabolites and the results are presented in Supplementary Figure 1 and Table 3. The maximum inhibitory zone was caused by Shigella dysenteriae (31.2 mm), followed by K. pneumoniae (31.1 mm) and S. boydii (30.2 mm). No zone formation was observed in the DMSO (Negative control). The antibacterial efficacy of fungal is mainly depends on the dose of the mycelia metabolites that are more specific to Gram negative bacteria than Gram-positive organisms.
Toxicity Assay of Zebrafish
The mycelia extract treated embryos have shown the body length with slight inhibition in the higher concentration of mycelia extract when compared to the untreated groups (Supplementary Figures 2C,D). The hatching out of the zebrafish embryos was noticed as 98.43% after 72 h in the control group. The embryo hatching rate was completely decreased at 1 mg/ml concentration (as compared to control and 0.1% DMSO groups) (Supplementary Figure 2A). While, the embryos exposed to MEAE mycelia extract and the metabolites could not induce any deformities (or) an increase in the pericardial area. But, the heart beat rate was notably reduced in the larvae of all exposure groups than control (Supplementary Figure 2B), The maximum concentration (1 mg/ml) of metabolites exerted slight malformation of embryos pericardial, yolk sac edema and also spine deformation occurred (Supplementary Figures 3A,B).
FTIR and GC–MS Analysis of Penicillium sp. Metabolites
Fourier Transformed Infrared spectrum of the Penicillium sp. MEAE revealed the presence of various absorption peaks. The notable peak was observed at 3431.31 cm−1 that may be attributed to the O–H stretching of aromatic compounds. The intense peak at 2922.59 cm−1 could be assigned to the C–H stretching of alkenes. The medium peak at 1631.41 cm−1 could be attributed to the N–H bending of the primary amines. The peak at 1383.68 cm−1 could be corresponds to the C–H bending of alkyls, respectively. The peak at 1323.89 cm−1 region of spectrum corresponds to the C–N stretching arising from strong aromatic amine groups. A medium peak at 1105.01 cm−1 corresponds to C–N stretching aliphatic amines. The absorption band appearing in 1028.84 cm−1 denote the presence of bending = C–H vibrations due to carboxylic acids. The obtained bands at 872.63 and 619.03 cm−1 corresponds to the N–H wagging and C = C–H bending of primary amines and alkyl halides, respectively (Supplementary Figure 4 and Table 4). The GC–MS analysis of the Penicillium sp. MEAE showed several compounds, based on the peak values and with the aid of NIST library. The identified compounds structure, molecular formula and molecular weight of the identified compounds are presented in Supplementary Figure 5 and Table 5.
Discussion
Isolation of Fungi
Fungi are highly complex group having variety of species with diverse metabolites that could kill mosquitoes. Fungi and actinomycetes are the greatest source for the production of diverse secondary metabolites (Vijayan and Balaraman, 1991). They act as a better source of powerful agents, which could be used toward the bio-control of pests and parasites (Rose, 1979). Generally, the number of fungi present in natural sources are very high when compared to the other microbes. They are being isolated from various sources by using different methods. Most of the fungal species are identified based on their morphological appearance (Rekha et al., 2015). Presently, seven different fungal species were isolated from soil viz, Penicillium sp. A. niger, A. flavus, A. parasiticus, Rhizopus sp. Mucor sp. and Aspergillus sp. Earlier, Kostadinova et al. (2009) have reported that the isolation and identification of fungi from the soils and their most frequently obtained forms belonged to the genera; Penicillium, Aspergillus, Cladosporium, Alternaria, Geomyces, and Lecanicillium. Manivannan and Kathiresan (2007) have reported the isolation of P. fellutanum from the rhizosphere the regions. Interestingly, the present findings are in agreement the results of Kumari et al. (2005) who have isolated 46 fungi (including Aspergillus and Penicillium) from 40 different soil samples (Gugnani and Shrivastav, 1972; Mini et al., 2012).
Larvicidal Activity
Microbe based control agents offer an alternative to chemical pest/insect control, because it can be more selective than chemical insecticides (Abdel-Baky and Abdel-Salam, 2003). The different types of biolarvicidal agents have been produced by several researchers with effective LC50 and LC90 values against many diseases causing mosquito-borne vectors (Kamaraj et al., 2009). The fungus metabolite has created highest mortalities in mosquito populations particularly in Culex sp. (Axtell et al., 1982) and Anopheles sp. (Kerwin and Washino, 1986). McCray et al. (1973) have proved that the indigenous soil fungus might successfully infect and kill the larvae of test mosquitoes with 100% mortality. Recently, several researchers have focused on identifying more potential mosquitocidal agents from biological resources toward the control of disease-transmitting mosquito vectors. Presently, the mycelial extracts of 7 different soil fungi were tested for larvicidal activity against the Ae. aegypti and Cx. quinquefasciatus larvae. The MEAE of Penicillium sp. was found to be more toxic than the other fungi. Presently, Penicillium sp. was found be more effective on all larval stages of Cx. quinquefasciatus. Presently, high LC50 and LC90 values against Ae. aegypti was found in least doses of metabolites when compared to the earlier report on Penicillium daleae mycelium extract (Ragavendran et al., 2017b). Earlier, Ragavendran et al. (2017a) reported that the Beauveria bassiana MEAE exerted larvicidal effect on 1st to 4th instar larvae of tested mosquitoes. Also, Govindarajan et al. (2005) have reported the mycelial extracts from various fungi i.e., Aspergillus flavus, A. parasiticus, Penicillium falcicum, Fusarium vasinfectum and Trichoderma viride have more toxicity caused against Cx. quinquefaciatus. Earlier, Cooper and Sweeney (1986) and Seif and Shaarawi (2003) reported that the Tolypocladium cylindrosporum and Culicinomyces clavisporus have shown toxic effect on larvae of selected mosquitoes. Further, Thomas and Read (2007) also investigated the fungal derived products that were found to be toxic against the tested mosquitoes and they found only lower toxicity against brine shrimp organisms. Balaraman et al. (1979) investigated the larval bio-effect of Metarhizium anisopliae, Beauveria tenella and Fusarium oxysporum. The outcome of present investigation, found that high potential utility of fungal metabolites acted as a complement for the existing larval control measures. This approach is being supported by Singh and Prakash (2012) who have demonstrated that the fungal metabolites could serve as a new strategy for filariasis and dengue control. The metabolites of L. giganteum showed a significant effect against Cx. quinquefasciatus and Ae. aegypti (Scholte et al., 2005).
Behavioral Studies
Shaalan et al. (2005) reported that secondary metabolites from fungus had shown the growth inhibitory effects on the various developmental stages of different mosquitoes i.e., delays in larval development, extended pupal durations, molting inhibition, morphological abnormalities, and mortality rate especially in molting and melanization processes. Presently, it was noticed that the Penicillium sp. MEAE-treated larvae displayed an unusual movement, excitation, horizontal and vertical aggressive movements. Similar kind of result was earlier obtained by Choochote et al. (2005). Chaithong et al. (2006) have reported the Pepper longum ethanolic extract treated Ae. aegypti larvae exhibited morphological alterations such as anal papillae shrinkage and cuticle dysfunction. Earlier, Kumar et al. (2010) who have studied the peppercorns extract treated Aedes sp. larvae that revealed deformation, vigorous self anal biting and shrinkage of external membranes. Earlier, Becker et al. (2010) studied the role of anal papillae in electrolyte balance regulation, which is necessary for the development of the life functions. Perumalsamy et al. (2010) have proved that the structural malformation of anal papillae may be intrinsically connected with the death of the treated larvae.
Acetylcholinesterase Assay
The organophosphorus and carbamate are the major cluster which served as target to the acetylcholinesterase (AChE) involved in the synaptic communication of nerve impulse in living beings (Yu et al., 2001). Microbial/plant secondary metabolites have been reported to decreases the AchE activity. The dose dependent response of α-carboxylesterase activity was noticed in the larvae of Choristoneura rosaceana, exposed to neem oil (Smirle et al., 1996). Likewise, Ortego et al. (1999) reported Limnoid has the potential as larvicide (from Scutellaria alpine) and found Leptinotarsa decemlineata esterase activity was significantly reduced in the coleopteran larvae. The present study showed that Penicillium sp. metabolite inhibits the AchE enzyme in the dengue and filarial vectors. The inhibition of AChE enzymes by mycelia extract was dose dependent and direct connections with the insect toxicity and which leads to eventual death of the organism. Likewise, Jukic et al. (2007) and Lopez-Hernandez et al. (2009) have studied the inhibitory property of AChE activity of monoterpenoids against various pests.
Histopathological Profiles of 4th Instar Larvae
The histopathological analysis of the treated larvae exhibited several alterations namely shrinkage of abdominal region, and damages to the midgut, muscles, and epithelial layers. Abinaya et al. (2018) have studied the Bacillus licheniformis exopolysaccharide treatment on 4th instar larvae of An. stephensi and Ae. aegypti and found different histological changes such as the gastric caeca, muscles shrinkage, damaged and disorganized nerve cord ganglia. Earlier, Farida et al. (2018) studied the B. bassiana metabolites toxicity on 4th instar larvae of Cx. pipiens and observed many histological changes and malformation in the treated larval body and tissues, especially in the adipose cells, cuticles and midgut. And, Ragavendran et al. (2017b) have reported that the midgut cells of tested mosquitoes (4th-instar larvae) had swelling in the gut lumen, reduced intercellular contents and degeneration of nuclei, after treated with P. daleae mycelium extract.
Antibacterial Activity
Natural products play an essential role in the discovery and development of drugs for the treatment of human diseases and microbial environment is an important source of novel bioactive agents (Newman et al., 2003). Since, the discovery of penicillin, the micromycetes have been famous as producers of antibiotics and other secondary metabolites with biological activity (Rancic et al., 2006). Penicillium sp have been proved as being abundant producers of many bioactive secondary metabolites/mycotoxins (Nicoletti et al., 2014) like citrinin and penicillic acid has shown potent antimicrobial activity (Kang et al., 2007; Yu et al., 2010). Presently, Penicillium sp. mycelia extract was screened for antibacterial activity against human pathogens. The mycelia metabolites exhibited significant antibacterial activity against all tested pathogenic bacteria particularly, K. pneumoniae and Salmonella dysentriae had maximum antibacterial activity. Gram-negative bacteria possess an outer membrane and a unique periplasmic space (Duffy and Power, 2001) whereas the Gram-positive bacteria are found to be more susceptible because they have only an outer peptidoglycan layer which is not an efficient permeability barrier (Scherrer and Gerhardt, 1971). The increased permeability of the membrane by the insertion of metabolites or free fatty acids can allow internal contents to leak from the cell, which can cause growth inhibition or even death (Wang and Johnson, 1992; Boyaval et al., 1995; Shin et al., 2007). Moreover, Devi et al. (2012) found a maximum antibacterial activity against Vibrio cholerae, E. coli and S. aureus by Penicillium chrysogenum bioactive metabolites. Kaur et al. (2014) isolated antimicrobial compound 6-[1,2-dimethyl-6-(2-methyl-allyloxy)-hexyl]-3- (2-methoxy-phenyl)-chromen-4-one from Penicillium sp that showed effective growth inhibition against S. aureus, E. coli, S. epidermidis and Salmonella typhimurium. Interestingly, Zhuo et al. (2012) reported the Penicillium commune bioassay guided fractionated compound xanthocillin X that showed significant antibacterial activity against S. aureus and E. coli. Specian et al. (2012) isolated the Diaporthe helianthi strain and its metabolites was tested against several human pathogenic bacteria. Silva et al. (2011) reported that the secondary metabolites of A. niger, Curvularia pallescens, Guignardia bidwelii, Paecilomyces variotii and Mycelia sterilia showed antibacterial activity against S. aureus, B. subtilis, E. faecalis, M. luteus, E. coli and Pseudomonas aeruginosa. Awad et al. (2018) have reported that the soil derived fungus, Trichoderma viridae mycelia metabolites exhibited significant antibacterial effects on Bacillus subtilis, E. coli and Pseudomonas fluorescens. Danagoudar et al. (2017) also studied the ethyl acetate extract of Penicillium citrinum that was tested against the human pathogenic bacteria and Cx. quinquefasciatus larvae.
Zebrafish Toxicity Assay
Danio rerio, zebrafish is an important in vitro aquatic model test organism being widely used in eco-toxicology fields. The acute aquatic toxicity tests are required for the assessment of biocides and plant based products (Seiler et al., 2014). Presently, the zebra fish embryos body length, hatching rates; heart beat count and survival percentage of D. rerio was significantly decreased. Rajaofera et al. (2017) isolated Bacillus atrophaeus substances that are non-toxic against D. rerio embryo. Further, Cao et al. (2016) have also reported the morphological and physiological changes in the cyhalofop-butyl treated zebrafish, that showed reduced hatching rate of embryos, delayed spontaneous movement, decreased heartbeat, and the reduced body length of larvae (at higher concentrations). On the other hand, Abutaha et al. (2015) isolated the Cochliobolus spicifer endophytic fungal extract that was tested for larvicidal as well as zebrafish embryo toxicity but its metabolites did not induce any toxicity symptoms on embryo.
FTIR Analysis
Presently, the FTIR spectrum of the mycelia extract of Penicillium sp. reflects the presence of various functional groups such as phenolic, alkenes, carboxylic acids and amine groups. Devi and Singh (2013) have studied the FT-IR spectral characteristics of Colletotrichum gloeosporioides fungus that revealed the functional groups of phenolic group, carboxylic acids (C–H group), aliphatic amines (C–N stretch), alkenes (C–H stretch), primary or secondary amines, and carboxy groups (C–C, stretch). Earlier, Ragavendran and Natarajan (2015) also reported the active functional group from FTIR spectrum of Aspergillus terreus mycelia extract at the peak value of 1023.59 cm−1 corresponding to C–O and C = N, which indicated the presence of carboxylic acid and amine groups. In addition to this, Abutaha et al. (2015) reported the C. spicifer fungi extract that revealed different functional groups such as carbonyl, methyl, phenol, amide and amines, respectively.
GCMS Analysis
The GC–MS analysis of the Penicillium sp. mycelial extract showed the presence of 1-Octadecene (5.10%) and 9-Octadecenoic acid (12.33%) which may responsible for mosquitocidal properties. Recently, Jeyasankar and Chinnamani (2018) reported that the Solonum pseudocapsicum extract revealed many compounds viz; n-Hexadecanoic acid, 9-Hexadecenoic acid and 9,12-Octadecadienoic acid (Z,Z)-, methyl ester that were responsible for larvicidal and pupicidal properties. Senthilkumar et al. (2014) have reported the insecticidal activity of Phomopsis sp because of the presence of Dodecanoic acid ethylester and Phthalic acid, octyl 2-pentyl ester with better insecticidal property. The main chemical constituents were identified by using GC–MS viz: Phenol, 2, 4-bis (1,1 dimethylethyl), 1-Hexadecene, 1-Hexadecanol, Hexadecanoic acid, octadecanoic acid methyl ester and 1-Nonadecene (Devi and Singh, 2013). On the other hand, Stanly Pradeep et al. (2015) have reported the soil fungus Fusarium moniliforme derived compounds (2-(4-((3E, 5E)-14-aminotetradeca-3,5-dienyloxy)butyl)-1,2,3,4-tetrahydroisoquinolin-4-ol) that exhibited potent mosquito larvicidal activity against 3–4th instar larvae of Ae. aegypti and An. stephensi, respectively. Ealier, Elsa Lycias Joel and Valentin Bhimba (2013) through GCMS analysis of marine fungus Meyerozyma guilliermondii found mainly secondary metabolites viz: Isohexyl neopentyl ester, Tridecane, Pentadecane, Isobutyl undecyl ester, 1-Nonadecanol, and 9-Octadecenoic acid. Lorenzen et al. (1996) reported the isolation of 11-hydroxy-4-methyl-2,4,6-dodecatrienoic acid from Mucor sp. which showed nematocidal activity.
Conclusion
In conclusion, totally seven indigenous fungal species (Penicillium sp. A. niger, A. flavus, A. parasiticus, Rhizopus sp. Mucor sp. and Aspergillus sp.) were isolated from soils. Among them, only the Penicillium sp. showed the better larvicidal, histopathological and antibacterial potential. The fungal MEAE of Penicillium sp. expressed good toxicity against Ae. aegypti 3rd instar larvae with LC50 value of 6.874 and LC90 12.879 μg/ml, after 24 h treatment. After 30 min exposure of metabolites, the behavioral symptoms of treated larvae showed excitation, up and down, horizontal and vertical movements with violent self anal biting features. Morphological changes were observed in the treated larvae as noticed in damaged anal papillae area and the cuticle layers. The Ae. aegypti and Cx. quinquefasciatus 4th instar larval mortality was directly linked to the inhibition of acetylcholinesterase activities. Histological study of metabolites showed in the digestive tract, midgut, cortex, hyperplasia of gut epithelial cells, brush border collapsed broken membranes and also observed cytoplasm masses. The antibacterial activities of three different concentrations of mycelia metabolites (50, 75, and 100 μL) with seven microorganisms were tested. The maximum zone of growth inhibition was recorded in S. boydii (30.2 mm), S. dysenteriae (31.2 mm) and K. pneumoniae (31.1 mm). Furthermore, the toxicity assay of zebrafish embryo was done using the Penicillium sp. mycelia extract after 124 hpf hatched embryo. The treated embryos showed slightly decreased the body length at higher concentration of mycelia extract as compared to untreated groups. The hatching out of the zebrafish embryos was noticed as 98.43% after 72 h in the control group. FTIR spectrum of the mycelia extract of Penicillium sp. revealed notable peak at 3431.31 cm−1 that could be attributed to the O–H stretching of aromatic compounds. GCMS analysis revealed that the fungus Penicillium sp. produced more number of bioactive compounds against tested larvae. The outcome of the results opens a new avenue for Penicillium sp. based novel metabolites that could form potential bio-insecticidal agents to control the vector-borne diseases in future.
Author Contributions
CR and DN designed the research plan and drafted the manuscript. CR, CK, JP, and VM performed the experimental works and data compilation. CR, CK, PP, and GB coordinated the work and discussed the results. CR, VM, and JP performed embryo toxicity test and data compilation. All authors read and approved the final manuscript.
Conflict of Interest Statement
The authors declare that the research was conducted in the absence of any commercial or financial relationships that could be construed as a potential conflict of interest.
Acknowledgments
CR thank the Council of Scientific and Industrial Research (CSIR), Government of India, New Delhi for the award of Senior Research Fellowship [09/810 (0024)/2016-EMR-I, 30/03/2017]. The authors thank the Department of Science and Technology, Government of India, for extending the DST-FIST grant facility to the Department of Biotechnology, Periyar University and to M/S. TUV SUD South Asia Pvt. Ltd., Vellore, Tamilnadu, India for GC–MS analysis.
Supplementary Material
The Supplementary Material for this article can be found online at: https://www.frontiersin.org/articles/10.3389/fmicb.2019.00427/full#supplementary-material
References
Abbott, W. S. (1925). A method of computing the effectiveness of an insecticide. J. Econ. Entomol. 18, 265–267. doi: 10.1093/jee/18.2.265a
Abdel-Baky, N. F., and Abdel-Salam, A. H. (2003). Natural incidence of Cladosporium sp. as a bio-control agent against whiteflies and aphids in Egypt. J. Appl. Entomol. 127, 228–235. doi: 10.1046/j.1439-0418.2003.00662.x
Abinaya, M., Vaseeharan, B., Divya, M., Vijayakumar, S., Govindarajan, M., Alharbi, N. S., et al. (2018). Structural characterization of Bacillus licheniformis Dahb1 exopolysaccharide—antimicrobial potential and larvicidal activity on malaria and Zika virus mosquito vectors. Environ. Sci. Pollut. Res. Int. 25, 18604–18619. doi: 10.1007/s11356-018-2002-6
Abutaha, N., Mashaly, A. M., Al-Mekhlafi, F. A., Farooq, M., Al-shami, M., and Wadaan, M. A. (2015). Larvicidal activity of endophytic fungal extract of Cochliobolus spicifer (Pleosporales: Pleosporaceae) on Aedes caspius and Culex pipiens (Diptera: Culicidae). Appl. Entomol. Zool. 50, 405–414. doi: 10.1007/s13355-015-0347-6
Anjana, S., and Thoppil, J. E. (2013). Chemical composition of the essential oils of four Pogostemon sp. and their larvicidal activity against Aedes albopictus Skuse (Diptera: Culicidae). Int. J. Environ. Biol. 3, 26–31.
Apinis, A. E. (1963). Occurence of thermophilous microfungi in certain Alluvial soils Near Nottigham. Verlag Von J. Cramer. 5, 57–78.
Arora, S., Kumar, G., and Meena, S. (2017). Gas chromatography-mass spectroscopy analysis of root of an economically important plant, Cenchrus ciliaris l. From thar Desert, Rajasthan (India). Asian J. Pharm. Clin. Res. 10, 64–69. doi: 10.22159/ajpcr.2017.v10i9.19259
Awad, N. E., Kassem, H. A., Hamed, M. A., El- Feky, A. M., Mohamed, A. A., Mahmoud, E. K., et al. (2018). Isolation and characterization of the bioactive metabolites from the soil derived fungus Trichoderma viride. Mycology 9, 70–80. doi: 10.1080/21501203.2017.1423126
Axtell, R. C., Jaronski, S. T., and Merriam, T. L. (1982). Efficacy of the mosquito fungal pathogen, Lagenidium giganteum (Oomycetes: Lagenidiales). Proc. Calif. Mosq. Vector Control Assoc. 50, 41–42.
Balajee, S. A., Weaver, M., Imhof, A., Gribskov, J., and Marr, K. A. (2004). Aspergillus fumigatus variant with decreased susceptibility to multiple antifungals. Antimicrob. Agents Chemother. 48, 1197–1203. doi: 10.1128/AAC.48.4.1197-1203.2004
Balaraman, K., Rao, U. B., and Rajagopalan, P. K. (1979). Isolation of Metarrhizium anisopliae, Beauveria tenella and Fusarium oxysporum (Deuteromycetes) and their pathogenicity to Culex fatigans and Anopheles stephensi. Ind. J. Med. Res. 70, 718–722.
Becker, N., Petric, D., Zqomba, M., Boase, C., Madon, M., Dahl, C., et al. (2010). Mosquitoes and Their Control, 2nd Edn. New York, NY: Springer, 409–599. doi: 10.1007/978-3-540-92874-4
Belofsky, G. N., Anguera, M., Jensen, P. R., Fenical, W., and Köck, M. (2000). Oxepinamides A-C and Fumiquinazolines H-I: bioactive metabolites from a marine isolate of a fungus of the genus Acremonium. Chemistry 6, 1355–1360. doi: 10.1002/(SICI)1521-3765(20000417)6:8<1355::AID-CHEM1355>3.0.CO;2-S
Benelli, G. (2015a). Plant-borne ovicides in the fight against mosquito vectors of medical and veterinary importance: a systematic review. Parasitol. Res. 114, 3201–3212. doi: 10.1007/s00436-015-4656-z
Benelli, G. (2015b). Research in mosquito control: current challenges for a brighter future. Parasitol. Res. 114, 2801–2805. doi: 10.1007/s00436-015-4586-9
Benelli, G., Iacono, A. L., Canale, A., and Mehlhorn, H. (2016). Mosquito vectors and the spread of cancer: an overlooked connection? Parasitol Res. 115, 2131–2137. doi: 10.1007/s00436-016-5037-y
Benelli, G., Pavela, R., Maggi, F., Petrelli, R., and Nicoletti, M. (2017a). Commentary: making green pesticides greener? The potential of plant products for nanosynthesis and pest control. J. Cluster Sci. 28, 3–10. doi: 10.1007/s10876-016-1131-7
Benelli, G., Rajeswary, M., and Govindarajan, M. (2017b). Towards green oviposition deterrents? Effectiveness of Syzygium lanceolatum (Myrtaceae) essential oil against six mosquito vectors and impact on four aquatic biological control agents. Environ. Sci. Pollut. Res. 25, 10218–10227. doi: 10.1007/s11356-016-8146-3
Benelli, G., and Romano, D. (2017). Mosquito vectors of Zika virus. Entomol. Gener. 36, 309–318. doi: 10.1127/entomologia/2017/0496
Bernhard, L., Bernhard, P., and Magnussen, P. (2003). Management of patients with lymphoedema caused by filariasis in North-eastern Tanzania: alternative approaches. Physiotherapy 89, 743–749. doi: 10.1016/S0031-9406(05)60500-7
Bouaziz, F., Koubaa, M., Chaabene, M., Barba, F. J., Ghorbel, R. E., and Chaabouni, S. E. (2017). High throughput screening for bioactive volatile compounds and polyphenols from Almond (Prunus amygdalus) Gum: assessment of their antioxidant and antibacterial activities. J. Food Process Preserve. 41, 1–10. doi: 10.1111/jfpp.12996
Boyaval, P., Corre, C., Dupuis, C., and Roussel, E. (1995). Effects of free fatty acids on propionic acid bacteria. Lait 75, 17–29. doi: 10.1051/lait:199512
Brady, O. J., Gething, P. W., Bhatt, S., Messina, J. P., Brownstein, J. S., Hoen, A. G., et al. (2012). Refining the global spatial limits of dengue virus transmission by evidence-based consensus. PLoS Negl. Trop Dis. 6:e1760. doi: 10.1371/journal.pntd.0001760
Cao, F., Liu, X., Wang, C., Zheng, M., Li, X., and Qiu, L. (2016). Acute and short-term developmental toxicity of cyhalofop-butyl to zebrafish (Danio rerio). Environ. Sci. Pollut. Res. 23, 10080–10089. doi: 10.1007/s11356-016-6236-x
Chaithong, U., Choochote, W., Kamsuk, K., Jitpakdi, A., Tippawangkosol, P., Chaiyasit, D., et al. (2006). Larvicidal effect of pepper plants on Aedes aegypti (L.) (Diptera: Culicidae). J. Vector Ecol. 31, 138–143. doi: 10.3376/1081-1710(2006)31[138:LEOPPO]2.0.CO;2
Chandrasekar, G., Arner, A., Kitambi, S. S., Dahlman-Wright, K., and Lendahl, M. A. (2011). Developmental toxicity of the environmental pollutant 4-nonylphenol in zebrafish. Neurotoxicol. Teratol. 33, 752–764. doi: 10.1016/j.ntt.2011.09.009
Chomcheon, P., Wiyakrutta, S., Sriubolmas, N., Ngamrojanavanich, N., Isarangkul, D., and Kittakoop, P. (2005). 3-Nitropropionic acid (3-NPA), a potent antimycobacterial agent from endophytic fungi: is 3-NPA in some plants produced by endophytes. J. Nat. Prod. 68,1103–1105.
Choochote, W., Chaiyasit, D., Kanjanapothi, D., Rattanachanpichai, E., Jitpakdi, A., Tuetun, B., et al. (2005). Chemical composition and antimosquito potential of rhizome extract and volatile oil derived from Curcuma aromatica against Aedes aegypti (Diptera: Culicidae). J. Vector Ecol. 30, 302–309. doi: 10.1021/np050036a
Cooper, R. D., and Sweeney, A. W. (1986). Laboratory studies on the recycling potential of the mosquito pathogenic fungus Culicinomyces clavisporus. J. Invert Pathol. 48, 152–158. doi: 10.1016/0022-2011(86)90117-5
Danagoudar, A., Joshi, C. G., Nivya, M. T., Manjunath, H. M., Poyya, J., and Sunil Kumar, R. (2017). Antimicrobial and larvicidal potential of endophytic fungi isolated from Tragia involucrata Linn. Ann. Plant Sci. 6, 1494–1498. doi: 10.21746/aps.2017.01.002
Deng, J., Yu, L., Liu, C., Yu, K., Shi, X., Yeung, L. W., et al. (2009). Hexabromocyclododecane-induced developmental toxicity and apoptosis in Zebrafish embryos. Aquat. Toxicol. 93, 29–36. doi: 10.1016/j.aquatox.2009.03.001
Dennis, K. C., Omondi Ouma, R. B., Kiplagat Cheplogoi, P., and Ouma Omolo, J. (2013). Larvicidal activity of extracellular secondary metabolites from a Stereum species Hill ex Pers. (JO5289) against the dengue fever mosquito, Aedes aegypti (Linn) (Diptera: Culicidae). African J. Chem. 01, 1–8.
Devi, N. N., and Singh, M. S. (2013). GC-MS Analysis of metabolites from endophytic fungus Colletotrichum gloeosporioides isolated from Phlogacanthus thyrsiflorus Nees. Int. J. Pharm. Sci. 23, 392–395.
Devi, P., Rodrigues, C., Naik, C. G., and D’Souza, L. (2012). Isolation and characterization of antibacterial compound from a mangrove-endophytic fungus, Penicillium chrysogenum. Ind. J. Microbiol. 52, 617–623. doi: 10.1007/s12088-012-0277-8
Duffy, C. F., and Power, R. F. (2001). Antioxidant and antimicrobial properties of some Chinese plant extracts. Int. J. Antimicob. Agents 17, 527–529. doi: 10.1016/S0924-8579(01)00326-0
Durowade, K. A., Kolawole, O. M., and Uddin, R. O. II (2007). Isolation of ascomycetous fungi from a tertiary institution campus soil. J. Appl. Sci. Environ. Manag. 11, 57–61.
Ellman, G. L., Courtney, K., Andres, V., and Featherstone, R. M. (1961). A new and rapid colorimetric determination of acetylcholinesterase activity. Biochem. Pharmacol. 7, 88–95. doi: 10.1016/0006-2952(61)90145-9
Fahd, A. A. (2018). Larvicidal, ovicidal activities and histopathological alterations induced by Carum copticum (Apiaceae) extract against Culex pipiens (Diptera: Culicidae). Saudi J. Biol. Sci. 25, 52–56. doi: 10.1016/j.sjbs.2017.02.010
Farida, B., Sonia, H., Hakima, M. K., Fatma, B., and Fatma, H. (2018). Histological changes in the larvae of the domestic mosquito Culex pipiens treated with the entomopathogenic fungus Beauveria bassiana. Sci. Res. Essays 13, 1–10. doi: 10.5897/SRE2017.6544
Feldman, A. T., and Wolfe, D. (2014). “Tissue processing and hematoxylin and eosin staining,” in Histopathology, ed. A. Burt (New York, NY: Humana Press), 31–43.
Ghosh, A., Mukherjee, S., Dutta, T., Roy, S., and Mondal, F. B. (2013). Community perceptions regarding mosquito borne diseases in some selected localitities of Bankura, a Peri-Urban area of West Bengal, India. J. Mosquito Res. 3, 54–57. doi: 10.5376/jmr.2013.03.0007
Gilman, J. C., and Abbott, E. V. (1957). A Manual of Soil Fungi, Spondylocladium Australe. Ames: Iowa State College Press, 342.
Govindarajan, M., Jebanesan, A., and Reetha, D. (2005). Larvicidal effect of extracellular secondary metabolites of different fungi against the mosquito, Culex quinquefasciatus Say. Trop Biomed. 22, 1–3.
Gugnani, H. C., and Shrivastav, J. B. (1972). Occurrence of pathogenic fungi in soil in India. Ind. J. Med. Res. 60, 40–47.
Hallare, A., Nagel, K., Köhler, H. R., and Triebskorn, R. (2006). Comparative embryotoxicity and proteotoxicity of three carrier solvents to zebrafish (Danio rerio) embryos. Ecotoxicol. Environ. Saf. 63, 378–388. doi: 10.1016/j.ecoenv.2005.07.006
Harzsch, S., and Hafner, G. (2006). Evolution of eye development in arthropods: phylogenetic aspects. Arthropod Struct. Dev. 35, 319–340. doi: 10.1016/j.asd.2006.08.009
Hemalatha, P., Elumalai, D., Janaki, A., Babu, M., Velu, K., Velayutham, K., et al. (2015). Larvicidal activity of Lantana camara aculeata against three important mosquito species. J. Entomol. Zool. Stud. 3, 174–181.
Hotez, P. J., Remme, H. J. F., Buss, P., Alleyne, G., Morel, C., and Breman, J. G. (2004). Combating tropical infectious diseases: report of the disease control priorities in developing countries project. Clin. Infect. Dis. 38, 871–878. doi: 10.1086/382077
Howe, K., Clark, M. D., Torroja, C. F., Torrance, J., Berthelot, C., Muffato, M., et al. (2013). The zebrafish reference genome sequence and its relationship to the human genome. Nature 496:498. doi: 10.1038/nature12111
Ikezawa, H., and Taguchi, R. (1981). Phosphatidylinositol-specific phospholipase C from Bacillus cereus and Bacillus thuringiensis. Methods Enzymol. 71, 731–741. doi: 10.1016/0076-6879(81)71086-3
Inui, I., Takeda, Y., and Iizuka, H. (1965). Taxonomical studies of the nus Rhizopus. J. Gen. Appl. Microbiol. 11(Suppl.), 1–121. doi: 10.2323/jgam.11.Supplement_1
Jasim, H., Hussein, A. O., Hameed, I. H., and Kareem, M. A. (2015). Characterization of alkaloid constitution and evaluation of antimicrobial activity of Solanum nigrum using gas chromatography mass spectrometry (GC-MS). J. Pharm. Phytother. 7, 56–72.
Jayaseelan, C., Gandhi, P. R., Rajasree, S. R. R., Suman, T. Y., and Mary, R. R. (2018). Toxicity studies of nanofabricated palladium against filariasis and malaria vectors. Environ. Sci. Pollut. Res. 25, 324–332. doi: 10.1007/s11356-017-0428-x
Jeyasankar, A., and Chinnamani, T. (2018). Larvicidal and pupicidal activities of Solonum pseudocapsicum fruits compounds against Aedes aegypti, Anopheles stephensi and Culex quinquefasciatus (Diptera: Culicidae). J. Infect. Dis. Med. Microbiol. 2, 11–16.
Joel, E. L., and Valentin Bhimba, B. (2013). Evaluation of secondary metabolites from mangrove associated fungi Meyerozyma guilliermondii. Alex. J. Med. 49, 189–194. doi: 10.1016/j.ajme.2013.04.003
Josphat, C. M., Dittrich, B., Schueffler, A., and Laatsch, H. (2011). Larvicidal activity of metabolites from the endophytic Podospora sp. against the malaria vector Anopheles gambiae. Parasitol. Res. 108, 561–566. doi: 10.1007/s00436-010-2098-1
Jukic, M., Politeo, O., Maksimovic, M., and Milos, M. (2007). In vitro acetylcholinesterase inhibitory properties of thymol, carvacrol and their derivatives thymoquinone and thymohydroquinone. Phytother. Res. 21, 259–261. doi: 10.1002/ptr.2063
Kaewnang-O, E., Ngampongsai, A., Subhadhirasakul, S., and Srichana, T. (2011). Toxicity of fixed oil and crude extract from sa-dao-thiam, Azadirachta excelsa (Jack) seed kernel to Aedes aegypti (L.). Sci. Technol. 33, 43–49.
Kamalakannan, S., Gobinath, C., and Ananth, S. (2014). Synthesis and characterization of fungus mediated silver nanoparticle for toxicity on filarial vector, Culex quinquefasciatus. Int. J. Pharm. Sci. Rev. Res. 24, 124–132.
Kamaraj, C., Bagavan, A., Rahuman, A. A., Zahir, A. A., Elango, G., and Pandiyan, G. (2009). Larvicidal potential of medicinal plant extracts against Anopheles subpictus Grassi and Culex tritaeniorhynchus Giles (Diptera: Culicidae). Parasitol. Res. 104, 1163–1171. doi: 10.1007/s00436-008-1306-8
Kang, S. W., Park, C. H., Hong, S. I., and Kim, S. W. (2007). Production of penicillic acids by Aspergillus sclerotiorum CGF. Bioresour. Technol. 98, 191–197. doi: 10.1016/j.biortech.2005.11.033
Kaur, H., Arora, D. S., and Sharma, V. (2014). Isolation, purification, and characterization of antimicrobial compound 6-[1,2-dimethyl-6-(2-methyl-allyloxy)-hexyl]-3- (2-methoxy-phenyl)-chromen-4-one from Penicillium sp. HT-28. Appl. Biochem. Biotechnol. 173, 1963–1976. doi: 10.1007/s12010-014-0979-y
Kerwin, J. L., and Washino, R. K. (1986). Ground and aerial application of the sexual and asexual stages of Lagenidium giganteum (Oomycetes: Lagenidiales) for mosquito control. J. Am. Mosq. Contr. Assoc. 2, 182–189.
Killeen, G. F., Fillinger, U., Kiche, I., Gouagna, L. C., and Knols, B. G. (2002). Eradication of Anopheles gambiae from Brazil: lessons for malaria control in Africa? Lancet Infect. Dis. 2, 618–627. doi: 10.1016/S1473-3099(02)00397-3
Kirschbaum, J. B. (1985). Potential implication of genetic engineering and other biotechnologies to insect control. Annu. Rev. Entomol. 30, 51–70. doi: 10.1146/annurev.en.30.010185.000411
Klich, M. A. (2002). Identification of Common Aspergillus Species. Netherland: Fungal Biodiversity Centre.
Klich, M. A., and Pitt, J. I. (1986). “The theory and practice of distinguishing species of the Aspergillus flavus group,” in Advances in Penicillium and Aspergillus systematics, ed. R. A. Samson (Boston, MA: Springer), 211–220.
Klich, M. A., and Pitt, J. I. (1988). A Laboratory Guide to Common Aspergillus Species and Their Teleomorphs. North Ryde, N.S.W: CSIRO, Div. of Food Processing.
Kostadinova, N., Krumova, E., Tosi, S., Pashova, and Angelova, M. (2009). Isolation and identification of filamentous fungi from island Livingston, Antarctica. Biotech. Biotechnol. Equip. 23(Suppl. 1), 267–270. doi: 10.1080/13102818.2009.10818416
Kovendan, K., Murugan, K., Thiyagarajan, P., Naresh Kumar, A. D., and Asaikkutti, A. (2009). Impact of climate change on the filarial vector, Culex quinquefasciatus Say (Diptera: Culicidae). Int. Congress Glob. Warm. Biodivers. Insects 62–69.
Kumar, S., Warikoo, R., and Wahab, N. (2010). Larvicidal potential of ethanolic extracts of dried fruits of three species of peppercorns against different instars of an Indian strain of dengue fever mosquito, Aedes aegypti L. (Diptera: Culicidae). Parasitol. Res. 107, 901–907. doi: 10.1007/s00436-010-1948-1
Kumari, G. R., Mahrora, S., and Rao, P. S. (2005). Prevalence of non-keratinophilic fungi in the soil. Ind. J. Med. Microbiol. 23, 144–145. doi: 10.4103/0255-0857.16063
Li, Y., Song, Y. C., Liu, J. Y., Ma, Y. M., and Tan, R. X. (2005). Anti-Helicobacter pylori substances from endophytic fungal cultures. World J. Microbiol. Biotech. 21, 553–558. doi: 10.1007/s11274-004-3273-2
Lopez-Hernandez, G. Y., Thinschmidt, J. S., Zheng, G., Zhang, Z., Crooks, A. P., Dwoskin, L. P., et al. (2009). Selective inhibition of acetylcholine-evoked responses of alpha 7 neuronal nicotinic acetylcholine receptors by novel tris- and tetrakisazaaromatic quaternary ammonium antagonists. Mol. Pharm. 76, 652–666. doi: 10.1124/mol.109.056176
López-Romero, F., Zúñiga, G., and Martínez-Jerónimo, F. (2012). Asymmetric patterns in the cranial skeleton of zebrafish (Danio rerio) exposed to sodium pentachlorophenate at different embryonic developmental stages. Ecotoxicol. Environ. Saf. 84, 25–31. doi: 10.1016/j.ecoenv.2012.06.008
Lorenzen, K., Anke, T., and Sterner, O. (1996). 11-hydroxy- 4-methyl-2,4,6-dodecatrienoic acid from fermentations of a Mucor species. Phytochem 43, 791–792. doi: 10.1016/0031-9422(96)00365-2
Madavasamy, S., and Panneerselvam, A. (2012). Isolation, identification of fungi from Avicinnia marina Muthupet mangroves Thiruvarur Dt. Asian J. Plant Sci. Res. 2, 452–459.
Manivannan, S., and Kathiresan, K. (2007). Alkaline protease production by Penicillium fellutanum isolated from mangrove sediment. Int. J. Biol. Chem. 1, 98–103. doi: 10.3923/ijbc.2007.98.103
Manjunatha, B., Park, S. H., Kim, K., Kundapur, R. R., and Lee, S. J. (2018). In vivo toxicity evaluation of pristine graphene in developing Zebrafish (Danio rerio) embryos. Environ. Sci. Pollut. Res. Int. 25, 12821–12829. doi: 10.1007/s11356-018-1420-9
Marella, A., Tanwar, O. P., Saha, R., Ali, M. R., Srivastava, S., Akhter, M., et al. (2013). Quinoline: a versatile heterocyclic. Saudi Pharm. J. 21, 1–12. doi: 10.1016/j.jsps.2012.03.002
McClenny, N. (2005). Laboratory detection and identification of Aspergillus species by microscopic observation and culture: the traditional approach. Med. Mycol. 43(Suppl. 1), 125–128. doi: 10.1080/13693780500052222
McCray, E. M., Womeldorf, D. J., Husbands, R. C., and Eliason, D. A. (1973). Laboratory observations and field tests with Lagenidium against California mosquitoes. Proc. Pap. Annu. Conf. Calif. Mosq. Control Assoc. Inc. 41, 123–128.
Mini, K. D., Mathew, J., Mini, S. S., and Paul, K. (2012). Keratinophilic fungal diversity of soil from Ernakulam and Thrissur districts-Kerala. Eur. J. Exp. Biol. 2, 1261–1264.
Misato, T. (1983). Recent status and future aspects of agricultural antibiotics. Nat. Prod. 2, 241–246. doi: 10.1016/B978-0-08-029223-6.50041-0
Mittal, P. K., and Subbarao, S. K. (2003). Prospect of using herbal product in the control of mosquito vectors. ICMR Bull. 33, 1–10.
Mohanty, S., Mishra, S., Jena, P., Jacob, B., Sarkar, B., and Sonawane, A. (2012). An investigation on the antibacterial, cytotoxic, and antibiofilm efficacy of starch-stabilized silver nanoparticles. Nanomed 8, 916–924. doi: 10.1016/j.nano.2011.11.007
Moustafa, M. F., Alamri, S. A., Taha, T. H., and Alrumman, S. A. (2013). In vitro antifungal activity of Argemone ochroleuca Sweet latex against some pathogenic fungi. Afr. J. Biotech. 12, 1132–1137.
Mushtaq, A., Rasool, N., Riaz, M., Tareen, R. B., Zubair, M., Rashid, U., et al. (2013). Antioxidant, Antimicrobial studies and characterisation of essential oil, fixed oil of Clematis graveolens by GC-MS. Oxid. Commun. 4, 11067–11078.
Nakalembe, I., and Kabasa, J. D. (2012). Anti-microbial activity and biochemical constituents of two edible and medicinal mushrooms of Mid-Western, Uganda. Res J. Pharmacol. 6, 4–11. doi: 10.3923/rjpharm.2012.4.11
Naqqash, M. N., Gökçe, A., Bakhsh, A., and Salim, M. (2016). Insecticide resistance and its molecular basis in urban insect pests. Parasitol. Res. 115, 1363–1373. doi: 10.1007/s00436-015-4898-9
Nathan, S. S., Kalaivani, K., and Sehoon, K. (2006). Effects of Dysoxylum malabaricum Bedd.(Meliaceae) extract on the malarial vector Anopheles stephensi Liston (Diptera: Culicidae). Biores. Technol. 97, 2077–2083. doi: 10.1016/j.biortech.2005.09.034
Newman, D. J., Cragg, G. M., and Snader, K. M. (2003). Natural products as source of new drugs over the period 1981–2002. J. Nat. Prod. 66, 1022–1037. doi: 10.1021/np030096l
Nicoletti, R., Fiorentino, A., and Scognamiglio, M. (2014). Endophytism of Penicillium species in woody plants. Open Mycol. J. 8, 1–26. doi: 10.2174/1874437001408010001
Nqoro, X., Tobeka, N., and Aderibigbe, B. A. (2017). Quinoline-based hybrid compounds with antimalarial activity. Molecules 22, 2268. doi: 10.3390/molecules22122268
OECD (2006). Fish Embryo Toxicity (FET) Test. Draft OECD Guideline for the Testing of Chemicals. Available at: http://www.oecd.org/dataoecd/39/59/36817070.pdf
OECD (2013). Guidelines for the Testing of Chemicals, Test No. 236: Fish Embryo Acute Toxicity (FET) Test. Paris: OECD Publishing.
Ortego, F., Opez-Olguín, J. L., Ruiz, M., and Castañera, P. (1999). Effects of toxic and deterrent terpenoids on digestive protease and detoxification enzyme activities of Colorado potato beetle larvae. Pestic. Biochem. Physiol. 63, 76–84. doi: 10.1006/pest.1998.2386
Osama, H. E. S., Mohsen, M. S., Shash, A. S. M., and Hamed, S. R. (2015). Isolation, structure elucidation and biological activity of Di- (2-ethylhexyl) phthalate produced by Penicillium janthinellum62. Inter. J. Chem. Tech. Res. 8, 51–56.
Paritala, V., Chiruvella, K. K., Thammineni, C., Ghanta, R. G., and Mohammed, A. (2015). Phytochemicals and antimicrobial potentials of mahogany family. Revista Brasileira de Farmacognosia 25, 61–83. doi: 10.1111/jphp.13005
Pates, H., and Curtis, C. (2005). Mosquito behavior and vector control. Annu. Rev. Entomol. 50, 53–70. doi: 10.1146/annurev.ento.50.071803.130439
Perumalsamy, H., Chang, K. S., Park, C., and Ahn, Y. J. (2010). Larvicidal activity of Asarum heterotropoides root constituents against insecticide-susceptible and-resistant Culex pipiens pallens and Aedes aegypti and Ochlerotatus togoi. J. Agric. Food Chem. 58, 10001–10006. doi: 10.1021/jf102193k
Phongpaichit, S., Nikom, J., Rungjindamai, N., Sakayaroj, J., Hutadilok-Towatana, N., Rukachaisirikul, V., et al. (2007). Biological activities of extracts from endophytic fungi isolated from Garcinia plants. FEMS Immunol. Med. Microbiol. 51, 517–525. doi: 10.1111/j.1574-695X.2007.00331.x
Piatak, D. M., and Reimann, K. A. (1970). Isolation of 1-octacosanol from Euphorbia corollata. Phytochemistry 9, 2585–2586. doi: 10.1016/S0031-9422(00)85784-2
Piscopo, E., Diurno, M. V., Cataldi, M. T., Scala, G., and Aliberti, F. (1984). Biological activity of 4-hydroxyisophthalic acid derivatives. Hydrazones with antimicrobial activity. Bollettino Della Societa Italiana Di Biologia Sperimentale 60, 1169–1175.
Pitt, J. I. (1975). “Xerophilic fungi and the spoilage of foods of plant origin,” in Water Relations of Foods, ed. R. B. Duckworth (London: Academic Press), 273–307.
Pitt, J. I. (1988). A Laboratory Guide to Common Penicillium Species (2nd Edn). North Ryde, NSW: CSIRO Division of Food Processing.
Prabhu, N., Raj, D. T., Yamuna, G. K., Ayisha, S. S., Puspha, J., and Innocent, D. (2010). Synthesis of silver phyto nanoparticles and their antibacterial efficacy. Dig. J. Nanomater. Biostruct. 5, 185–189.
Pradeep, F. S., Palaniswamy, M., Ravi, S., Thangamani, A., and Pradeep, B. V. (2015). Larvicidal activity of a novel isoquinoline type pigment from Fusarium moniliforme KUMBF1201 against Aedes aegypti and Anopheles stephensi. Process Biochem. 50, 1479–1486. doi: 10.1016/j.procbio.2015.05.022
Ragavendran, C., Dubey, N. K., and Natarajan, D. (2017a). Beauveria bassiana (Clavicipitaceae): a potent fungal agent for controlling mosquito vectors of Anopheles stephensi, Culex quinquefasciatus and Aedes aegypti (Diptera: Culicidae). RSC Adv. 7:3838. doi: 10.1039/C6RA25859J
Ragavendran, C., Mariappan, T., and Natarajan, D. (2017b). Larvicidal, histopathological efficacy of Penicillium daleae against larvae of Culex quinquefasciatus and Aedes aegypti plus biotoxicity on Artemia nauplii a non-target aquatic organism. Front. Pharmacol. 8:773. doi: 10.3389/fphar.2017.00773
Ragavendran, C., and Natarajan, D. (2015). Insecticidal potency of Aspergillus terreus against larvae and pupae of three mosquito species Anopheles stephensi, Culex quinquefasciatus, and Aedes aegypti. Environ. Sci. Pollut. Res. 22, 17224–17237. doi: 10.1007/s11356-015-4961-1
Rajaofera, M. J. N., Jin, P. F., Fan, Y. M., Sun, Q. Q., Huang, W. K., Wang, W. B., et al. (2017). Antifungal activity of the bioactive substance from Bacillus atrophaeus strain HAB-5 and its toxicity assessment on Danio rerio. Pestic. Biochem. Physiol. 147, 153–161. doi: 10.1016/j.pestbp.2017.06.006
Rancic, A., Sokovic, M., Karioti, A., Vukojevic, J., and Skaltsa, H. (2006). Isolation and structural elucidation of two secondary metabolites from the filamentous fungus Penicillium ochrochloron with antimicrobial activity. Environ. Toxicol. Pharmacol. 22, 80–84. doi: 10.1016/j.etap.2005.12.003
Raper, K. B., and Fennell, D. I. (1965). The Genus Aspergillus. Baltimore, MD: Williams and Wilkins Company.
Raper, K. B., and Thom, C. (1949). A Manual of the Penicillia. Baltimore, MD: Williams and Wilkins Co.
Rawani, A., Ray, A. S., Ghosh, A., Sakar, M., and Chandra, G. (2017). Larvicidal activity of phytosteroid compounds from leaf extract of Solanum nigrum against Culex vishnui group and Anopheles subpictus. BMC Res. Notes 10:135. doi: 10.1186/s13104-017-2460-9
Reegan, A. D., Rajiv Gandhi, M., Paulraj, M. G., and Ignacimuthu, S. (2015). Ovicidal and oviposition deterrent activities of medicinal plant extracts against Aedes aegypti L. and Culex quinquefasciatus Say Mosquitoes (Diptera: Culicidae). Osong Public Health Res. Perspect. 6, 64–69. doi: 10.1016/j.phrp.2014.08.009
Rekha, B. C., Krishnaveni, G., and Rajendran, P. (2015). Prevalence of Trichophyton species from selected soils of Chennai, India. Asian J. Multidiscip. Stud. 3, 126–130.
Reuben, R., Tewari, S. C., Hiriyan, J., and Akiyama, J. (1994). Illustrated keys to species of Culex associated with Japanese encephalitis in Southeast Asia (Diptera: Culicidae). Mosq. Syst. 26, 75–96.
Rose, A. H. (1979). Economic Microbiology. Vol.(3). Secondary products of metabolism. Academic Press. London.Sweeney, A. W. 1975b. Austr. J. Zool. 23, 59–64.
Rudrapal, M., and Chetia, D. (2010). Synthesis and antibacterial activity evaluation of some novel 7-chloro-4- aminoquinoline derivatives. Int. J. ChemTech Res. 2, 1606–1611.
Rueda, L. M. (2004). Pictorial Keys for the Identification of Mosquitoes (Diptera: Culicidae) Associated with Dengue Virus Transmission. Washington DC: Walter Reed Army Inst of Research.
Samson, R. A., and Pitt, J. I. (1990). Modern Concepts in Penicillium and Aspergillus Classification. New York, NY: Plenum Press. doi: 10.1007/978-1-4899-3579-3
Sanabria-Ríos, D. J., Rivera-Torres, Y., Maldonado-Domínguez, G., Domínguez, I., Ríos, C., Díaz, D., et al. (2014). Antibacterial activity of 2-alkynoic fatty acids against multidrug-resistant bacteria. Chem. Phys. Lipids 178, 84–91. doi: 10.1016/j.chemphyslip.2013.12.006
Scherrer, R., and Gerhardt, P. (1971). Molecular sieving by the Bacillus megaterium cell wall and protoplast. J. Bacteriol. 107, 718–735.
Schipper, M. A. A. (1978). On certain species of Mucor with a key to all accepted species. Stud. Mycol. 17, 475–491.
Schipper, M. A. A. (1984). A revision of the genus Rhizopus. Stud. Mycol. 25, 1–34. doi: 10.1371/journal.pone.0027507
Schmidt, H. R., Radić, Z., Taylor, P., and Fradinger, E. A. (2015). Quaternary and tertiary aldoxime antidotes for organophosphate exposure in a zebrafish model system. Toxicol. Appl. Pharmacol. 284, 197–203. doi: 10.1016/j.taap.2015.02.011
Scholte, E. J., Nghabi, K., Kihonda, J., Takken, W., Paaijmans, K., Abdulla, S., et al. (2005). An entomopathogenic fungus for control of adult African malaria mosquitoes. Science 308, 1641–1642. doi: 10.1126/science.1108639
Schulz, B., Boyle, C., Draeger, S., Römmert, A. K., and Krohn, K. (2002). Endophytic fungi: a source of novel biologically active secondary metabolites. Mycol. Res. 106, 996–1004. doi: 10.1017/S0953756202006342
Seif, A. I., and Shaarawi, F. A. (2003). Preliminary field trials with Culicinomyces clavosporus against some Egyptian mosquitoes in selected habitats. J. Egypt. Soc. Parasitol. 33, 291–304.
Seiler, T. B., Best, N., Fernqvist, M. M., Hercht, H., Smith, K. E., Braunbeck, T., et al. (2014). PAH toxicity at aqueous solubility in the fish embryo test with Danio rerio using passive dosing. Chemosphere 112, 77–84. doi: 10.1016/j.chemosphere.2014.02.064
Selvamangai, G., and Bhaskar, A. (2012). Analysis of phytocomponents in the methanolic extract of Eupatorium triplinerve by GC-MS method. Int. J. Drug Dev. Res. 5, 384–391.
Senthilkumar, N., Murugesan, S., Babu, D. S., and Rajeshkannan, C. (2014). GC-MS analysis of the extract of endophytic fungus, Phomopsis sp. isolated from tropical tree species of India, Tectona grandis L. Int J. Innov. Res. Sci. Eng. Tech. 3, 10176–10179.
Shaalan, E. A., Canyon, D., Younes, M. W., Abdel-Wahab, H., and Mansour, A. H. (2005). A review of botanical phytochemicals with mosquitocidal potential. Environ. Int. 31, 1149–1166. doi: 10.1016/j.envint.2005.03.003
Shin, S. Y., Bajpai, V. K., Kim, H. R., and Kang, S. C. (2007). Antibacterial activity of eicosapentaenoic acid (EPA) against food borne and food spoilage microorganisms. LWT 40, 1515–1519. doi: 10.1016/j.lwt.2006.12.005
Siddiquee, S., Cheong, B. E., Taslima, K., Kausar, H., and Hasan, M. M. (2012). Separation and identification of volatile compounds from liquid cultures of Trichoderma harzianum by GC-MS using three different capillary columns. J. Chromatogr. Sci. 50, 358–367. doi: 10.1093/chromsci/bms012
Silva, M. R. O., Almeida, A. C., Arruda, F. V. F., and Gusmao, N. (2011). “Endophytic fungi from Brazilian mangrove plant Laguncularia racemosa (L.) Gaertn. (Combretaceae): their antimicrobial potential,” in Science Against Microbial Pathogens: Communicating Current Research and Technological Advances, ed. A. Mendez-Vilas (Badajoz: Formatex), 1260–1266.
Silva, V. D. C. B., Ribeiro Neto, J. A., Alves, S. N., and Lima, L. A. (2015). Larvicidal activity of oils, fatty acids, and methyl esters from ripe and unripe fruit of Solanum lycocarpum (Solanaceae) against the vector Culex quinquefasciatus (Diptera: Culicidae). Revista da Sociedade Brasileira de Medicina Tropical. 48, 610–613. doi: 10.1590/0037-8682-0049-2015
Sina, I., Zaharah, and Mohd Sabri, M. S. (2015). Larvicidal activities of extract flower Averrhoa bilimbi L. Towards important species mosquito, Anopheles barbirostris (Diptera: Culicidae). Int. J. Zool. Res. 12, 25–31. doi: 10.3923/ijzr.2016.25.31
Singh, G., and Prakash, S. (2012). Lethal effects of Aspergillus niger against mosquitoes vector of filaria, malaria, and dengue: a liquid mycoadulticide. ScientificWorldJournal 2012, 1–5. doi: 10.1100/2012/603984
Singh, R. K., Dhiman, R. C., and Mittal, P. K. (2006). Mosquito larvicidal properties of Momordica charantia Linn (family: Cucurbitaceae). J. Vect. Borne Dis. 43, 88–91.
Smirle, M. J., Lowery, T., and Zurowski, C. L. (1996). Influence of neem oil on detoxification enzyme activity in the obliquebanded leafroller, Choristoneura rosaceana. Pestic. Biochem. Physiol. 56, 220–230. doi: 10.1006/pest.1996.0075
Song, Y., Cheon, S. A., Lee, K. E., Lee, S. Y., Lee, B. K., Oh, D. B., et al. (2008). Role of the RAM network in cell polarity and hyphal morphogenesis in Candida albicans. Mol. Bio. Cell 19, 5456–5477. doi: 10.1091/mbc.E08-03-0272
Specian, V., Sarragiotto, M. H., Pamphile, J. A., and Clemente, E. (2012). Chemical characterization of bioactive compounds from the endophytic fungus Diaporthe helianthi isolated from Luehea divaricata. Brazilian J. Microbiol. 43, 1174–1182.
Strobel, G. A. (2003). Endophytes as sources of bioactive products. Microb. Infect. 5, 535–544. doi: 10.1016/S1286-4579(03)00073-X
Thomas, M. B., and Read, A. F. (2007). Can fungal biopesticides control malaria? Nat. Rev. Microbiol. 5, 377–383. doi: 10.1038/nrmicro1638
Thornberry, H. H. (1950). A paper-disk plate method for the quantitative evaluation of fungicides and bactericides. Phytopathology 40,419–429.
Truong, L., Reif, D. M., St Mary, L., Geier, M. C., Truong, H. D., and Tanguay, R. L. (2014). Multidimensional in vivo hazard assessment using zebrafish. Toxicol. Sci. 137, 212–233. doi: 10.1093/toxsci/kft235
Tsai, T. F., and Mitchell, C. J. (1989). “St. Louis encephalitis,” in The Arboviruses: Epidemiology and Ecology, ed. T. P. Monath (Boca Florida: CRC Press), 431–458.
Tyagi, B. K., Munirathinam, A., Krishnamooerthy, R., and Venkatesh, A. (2012). A Field Based Handbook of Identification Key to Mosquitoes of Public Health Importance in India. (Madurai: Centre for Research in Medical Entomology), 1–62
Valentin Bhimba, B., Franco, D. A. D., Jose, G. M., Mathew, J. M., and Joel, E. L. (2011). Characterization of cytotoxic compound from mangrove derived fungi Irpex hydnoides VB4. Asian Pac. J. Trop. Biomed. 1, 223–226. doi: 10.1016/S2221-1691(11)60031-2
Vicario-Pares, U., Castañaga, L., Lacave, J. M., Miriam, O., Reip, P., Berhanu, D., et al. (2014). Comparative toxicity of metal oxide nanoparticles (CuO, ZnO and TiO2) to developing zebrafish embryos. J. Nanopart. Res. 16:2550. doi: 10.1007/s11051-014-2550-8
Vijayan, V., and Balaraman, K. (1991). Metabolites of fungi & actinomycetes active against mosquito larvae. Ind J. Med. Res. 93, 115–117.
Vivek, R., Thangam, R., Muthuchelian, K., Gunasekaran, P., Kaveri, K., and Kannan, S. (2012). Green biosynthesis of silver nanoparticles from Annona squamosa leaf extract and its in vitro cytotoxic effect on MCF-7 cells. Process Biochem. 47, 2405–2410. doi: 10.1016/j.procbio.2012.09.025
Vyas, N., Dua, K. K., and Prakash, S. (2007). Efficacy of Lagenidium giganteum metabolites on mosquito larvae with reference to nontarget organisms. Parasitol. Res. 101, 385–390. doi: 10.1007/s00436-007-0496-9
Wang, L. L., and Johnson, E. A. (1992). Inhibition of Listeria monocytogenes by fatty acids and monoglycerides. Appl. Environ. Microbiol. 58, 624–629.
Ward, M., and Benelli, G. (2017). Avian and simian malaria: do they have a cancer connection? Parasitol. Res. 116, 839–845. doi: 10.1007/s00436-016-5352-3
Warikoo, R., and Kumar, S. (2013). Impact of Argemone mexicana extracts on the cidal, morphological and behavioral response of dengue vector, Aedes aegypti L. (Diptera: Culicidae). Parasitol. Res. 112, 3477–3484. doi: 10.1007/s00436-013-3528-7
Weber, R. W., Kappe, R., Paululat, T., Mösker, E., and Anke, H. (2007). Anti-candida metabolites from endophytic fungi. Phytochemistry 68, 886–892. doi: 10.1016/j.phytochem.2006.12.017
Wood, A. (2003). Compendium of Pesticide Common Names: Pseticides. Available at: http://www.alanwood.net/pesticides/class_pesticides.html
Xu, T., Zhao, J., Hu, P., Dong, Z., Li, J., Zhang, H., et al. (2014). Pentachlorophenol exposure causes Warburg-like effects in zebrafish embryos at gastrulation stage. Toxicol. Appl. Pharmacol. 277, 183–191. doi: 10.1016/j.taap.2014.03.004
Yang, Y., Ma, H., Zhou, J., Liu, J., and Liu, W. (2014). Joint toxicity of permethrin and cypermethrin at sublethal concentrations to the embryo-larval zebrafish. Chemosphere 96, 146–154. doi: 10.1016/j.chemosphere.2013.10.014
Yu, H., Zhang, L., Li, L., Zheng, C., Guo, L., Li, W., et al. (2010). Recent developments and future prospects of antimicrobial metabolites produced by endophytes. Microbiol. Res. 165, 437–449. doi: 10.1016/j.micres.2009.11.009
Yu, Q., Holloway, H. W., Flippen-Anderson, J. L., Hoffman, B., Brossi, A., and Greig, N. H. (2001). Methyl analogues of the experimental Alzheimer drug phenserine: synthesis and structure/activity relationships for acetyl- and butyrylcholinesterase inhibitory action. J. Med. Chem. 44,4062–4067.
Zhang, Y., Mu, J., Feng, Y., Li, H., and Dong, X. (2013). Biological and chemical diversity of cytotoxin-producing symbiotic marine fungi in intertidal zone of Dalian. Chin. Sci. Bull. 58, 2290–2297. doi: 10.1007/s11434-012-5442-2
Keywords: larvicidal, acetylcholinesterase, histopathology, antibacterial, zebrafish embryo, FTIR, GCMS
Citation: Ragavendran C, Manigandan V, Kamaraj C, Balasubramani G, Prakash JS, Perumal P and Natarajan D (2019) Larvicidal, Histopathological, Antibacterial Activity of Indigenous Fungus Penicillium sp. Against Aedes aegypti L and Culex quinquefasciatus (Say) (Diptera: Culicidae) and Its Acetylcholinesterase Inhibition and Toxicity Assessment of Zebrafish (Danio rerio). Front. Microbiol. 10:427. doi: 10.3389/fmicb.2019.00427
Received: 11 September 2018; Accepted: 19 February 2019;
Published: 18 March 2019.
Edited by:
Carlos Augusto Fernandes Oliveira, University of São Paulo, BrazilReviewed by:
Ram Prasad, Amity University, IndiaAmir Ismail, Bahauddin Zakariya University, Pakistan
Copyright © 2019 Ragavendran, Manigandan, Kamaraj, Balasubramani, Prakash, Perumal and Natarajan. This is an open-access article distributed under the terms of the Creative Commons Attribution License (CC BY). The use, distribution or reproduction in other forums is permitted, provided the original author(s) and the copyright owner(s) are credited and that the original publication in this journal is cited, in accordance with accepted academic practice. No use, distribution or reproduction is permitted which does not comply with these terms.
*Correspondence: Devarajan Natarajan, bWRuYXRhcmFqQHJlZGlmZm1haWwuY29t; bmF0YXJhanB1QGdtYWlsLmNvbQ==