- 1Department of Genomic and Applied Microbiology and Göttingen Genomics Laboratory, Institute of Microbiology and Genetics, Georg-August University, Göttingen, Germany
- 2Department of General Microbiology, Institute of Microbiology and Genetics, Georg-August University, Göttingen, Germany
Soil represents a significant reservoir of antibiotic resistance genes (ARGs), which can potentially spread across distinct ecosystems and be acquired by pathogens threatening human as well as animal health. Currently, information on the identity and diversity of these genes, enabling anticipation of possible future resistance development in clinical environments and the livestock sector, is lacking. In this study, we applied functional metagenomics to discover novel sulfonamide as well as tetracycline resistance genes in soils derived from forest and grassland. Screening of soil metagenomic libraries revealed a total of eight so far unknown ARGs. The recovered genes originate from phylogenetically diverse soil bacteria (e.g., Actinobacteria, Chloroflexi, or Proteobacteria) and encode proteins with a minimum identity of 46% to other antibiotic resistance determinants. In particular forest soil ecosystems have so far been neglected in studies focusing on antibiotic resistance. Here, we detected for the first time non-mobile dihydropteroate synthase (DHPS) genes conferring resistance to sulfonamides in forest soil with no history of exposure to these synthetic drugs. In total, three sulfonamide resistant DHPSs, differing in taxonomic origin, were discovered in beech or pine forest soil. This indicates that sulfonamide resistance naturally occurs in forest-resident soil bacterial communities. Besides forest soil-derived sulfonamide resistance proteins, we also identified a DHPS affiliated to Chloroflexi in grassland soil. This enzyme and the other recovered DHPSs confer reduced susceptibility toward sulfamethazine, which is widely used in food animal production. With respect to tetracycline resistance, four efflux proteins affiliated to the major facilitator superfamily (MFS) were identified. Noteworthy, one of these proteins also conferred reduced susceptibility toward lincomycin.
Introduction
Pathogenic bacteria resistant to multiple classes of antibiotics pose risks to public health and are considered as one of the major global challenges within the 21st century. Some of the antibiotic resistance genes (ARGs) carried by these bacteria have been traced to soil origins (Forsberg et al., 2012) and can potentially spread via e.g., groundwater or wildlife (Davies and Davies, 2010). Nevertheless, in contrast to clinical pathogens, bacterial communities inhabiting complex environments such as soil have been rarely considered within studies focusing on antibiotic resistance (Walsh, 2013b). To assess risks of environmental resistomes and develop strategies to tackle antibiotic resistance, an improved knowledge on the ecology of resistance determinants including their origins, diversity and underlying resistance mechanisms is urgently required (Allen et al., 2009; Wang et al., 2017).
Among Earth’s microbial habitats, soil harbors the highest diversity of prokaryotes including numerous multi-resistant bacteria (Delmont et al., 2011; Walsh and Duffy, 2013; Nesme and Simonet, 2015). The synthesis of antibiotics likely evolved in this habitat and promoted the development of different antimicrobial compound-specific resistance mechanisms (D’Costa et al., 2007; Walsh, 2013a). Previously unknown soil-derived ARGs were recovered from both, pristine and intensively managed sites, by function-based screening of metagenomic libraries (Allen et al., 2009; Perron et al., 2015; Lau et al., 2017). In contrast to sequence-based metagenomic library screening, this culture-independent approach is not based on conserved DNA regions and therefore allows the identification of entirely novel target genes (Nacke and Daniel, 2014; Cheng et al., 2017). For instance, a so far unknown peptide-associated macrolide resistance mechanism was uncovered by coupling function-based metagenomic library screening and high-resolution proteomics analysis (Lau et al., 2017). Besides dependence on conserved DNA regions, the fact that various resistance genes show high levels of similarity to genes encoding other cellular functions (Martínez, 2008; Perron et al., 2015) represents another limitation of sequence-based resistome analysis. An illustrative example are efflux pumps of the resistance-nodulation-division (RND) superfamily, which can confer antibiotic resistance, but can also transport proteins involved in cell division and nodulation, or both (Piddock, 2006; Perron et al., 2015).
In this study, we used function-based metagenomic library screening to identify so far unknown tetracycline and sulfonamide resistance genes in forest and grassland soil. Due to an excellent therapeutic index, few side effects, oral administration and low cost, tetracyclines belong to the most widely used classes of broad spectrum antibiotics in clinic (Thaker et al., 2010; Wang et al., 2017). After more than 60 years of excessive tetracycline usage, tetracycline resistance became one of the most abundant antibiotic resistances among clinical and commensal microbes (Wang et al., 2017). Another class of antibiotics, sulfonamides, is also commonly prescribed to people suffering from infections (Landers et al., 2012).
ARGs present in forests and grasslands, belonging to the most abundant terrestrial ecosystems worldwide, might become clinically relevant as they can potentially spread via lateral gene transfer. Here, we report the identification of four novel tetracycline and four previously unknown sulfonamide resistance genes derived from these ecosystems. Most of the proteins encoded by the novel ARGs showed low identity to already known antibiotic resistance determinants.
Materials and Methods
Site Description, Soil Sampling, and Metagenomic Library Construction
Soil samples were derived from forest and grassland sites of the German Biodiversity Exploratories Schorfheide-Chorin and Schwäbische Alb (Fischer et al., 2010). The land use intensity index (LUI) (Blüthgen et al., 2012) was calculated for all grassland sites. To account for interannual variation in management practices, the LUI was calculated from 2006 to 2008 (sampling year) (Table 1). LUI allows separate analysis of the intensity of grazing (calculated by considering numbers of grazing cattle, horses, or sheep, and duration of grazing with respect to each site), the mowing frequency, and the intensity of fertilization. Forest plots were dominated by European beech (Fagus sylvatica) or Scots pine (Pinus sylvestris) (Table 1).
The collection of the samples was performed previously as described by Nacke et al. (2011a). Descriptions of the soil characteristics are provided in Table 2. Total microbial community DNA was isolated from collected soil by employing the PowerSoil DNA isolation kit (MoBio Laboratories, Carlsbad, CA, United States) and metagenomic libraries, named AEG2, AEG3, and SEG8 were generated as described by Nacke et al. (2011b). The metagenomic libraries AEW9, SEG6, SEW2, and SEW5 were previously constructed (Nacke et al., 2011b). Names of constructed metagenomic libraries refer to the designation of the samples from which the libraries were derived.
Antibiotic Resistance Screening and Sequence Analysis
The function-based screening was based on the ability of metagenomic library-bearing Escherichia coli clones to form colonies on LB agar medium containing 50 mg/L kanamycin, which selects for the screening vector pCR-XL-TOPO (Thermo Fisher Scientific, Braunschweig, Germany), and 5 mg/L tetracycline or 250 mg/L sulfamethoxazole. Colonies formed after incubation for 1–3 days at 37°C under aerobic conditions were picked for further study.
The recombinant plasmids derived from positive clones were sequenced by Microsynth Seqlab (Göttingen, Germany) using Sanger sequencing technology. All plasmid inserts were taxonomically classified using the software KAIJU (Menzel et al., 2016). An initial prediction of ORFs located on the inserts was performed by employing the ORF finder tool provided by the National Center for Biotechnology Information (NCBI) and the Artemis program (Rutherford et al., 2000; Wheeler et al., 2003). The results were verified and improved manually by e.g., GC frame plot and ribosome-binding site analysis. Subsequently, blast (Altschul et al., 1990) search against the NCBI non-redundant protein sequence database was performed. In addition, Resfams (Gibson et al., 2015), a recently generated database of protein families and associated profile hidden Markov models, representing all major ARG classes, was used for sequence comparisons. Blast searches against the ACLAME database (Leplae et al., 2010) version 0.4 and the Gypsy database (Llorens et al., 2011) release 2.0 were performed to identify mobile genetic elements. Moreover, the IS finder (database from 2018-09-11) (Siguier et al., 2006) was employed for identification of bacterial insertion sequences.
A neighbor-joining phylogenetic tree was constructed in MEGA (version 7.0) (Kumar et al., 2016) based on a ClustalW (Thompson et al., 1994) alignment of dihydropteroate synthase (DHPS) sequences. A total number of 1,000 bootstrap samplings were carried out to test the tree topology. Branches corresponding to partitions reproduced in less than 50% bootstrap replicates were collapsed. The evolutionary distances were computed using the number of differences method.
Subcloning of ORFs Potentially Encoding Antibiotic Resistance
To verify if candidate ORFs encode antibiotic resistance, they were subcloned into vector pCR4-TOPO (Thermo Fisher Scientific) and subsequently introduced into E. coli TOP10. Two insert sequences (corresponding plasmids, pLAEG3_tet01 and pLSEG6_tet01) encoded proteins with similarity to members of the TetR family of regulators. In this case, the gene encoding the regulator as well as the potential ARG were subcloned together. In a first step, PCR was performed for amplification of candidate ORFs (including sequences potentially comprising promoters) from plasmid DNA. PCR primers are listed in Table 3. The PCR reaction mixture (50 μl) contained 10 μl 5-fold Phusion GC buffer, 200 μM of each of the four deoxynucleoside triphosphates, 5% DMSO, 0.2 μM of each primer, 1 U of Phusion HF DNA polymerase (Thermo Fisher Scientific), and approximately 20 ng of plasmid DNA. The following thermal cycling scheme was used: initial denaturation at 98°C for 1 min, 20 cycles of denaturation at 98°C for 1 min, annealing for 45 s (annealing temperatures, see Table 3), and extension at 72°C for 30 s per kb, followed by a final extension period at 72°C for 5 min. PCR products were purified using the QIAquick PCR purification kit (Qiagen, Hilden, Germany) according to the instructions of the manufacturer. Subsequently, a deoxyadenosine was added to the 3′ termini of the DNA as described by Nacke et al. (2011b) to facilitate cloning by the TA method. The DNA was then purified using the QIAquick PCR purification kit (Qiagen) and inserted into vector pCR4-TOPO (Thermo Fisher Scientific) as described by the manufacturer. Transformation of resulting vectors into E. coli TOP10 chemically competent cells was performed according to the protocol of the manufacturer.
Antibiotic Susceptibility Analysis
Antibiotic susceptibility assays were conducted by using the 2-fold serial microtiter broth dilution method by considering the Clinical and Laboratory Standards Institute (CLSI) guidelines document M100-S24 (2014) and the MICs were recorded after 20 h of incubation at 37°C. The antibiotics cefotaxime, chloramphenicol, erythromycin, gentamicin, lincomycin, rifampicin, sulfadiazine, sulfamethoxazole, sulfamethazine, sulfisoxazole, tetracycline, and tylosin were considered. All assays were performed in duplicate. In addition, the susceptibility to different sulfonamides was further analyzed by spotting serial dilutions of cultures with starting OD600 of 0.5 onto Iso-Sensitest agar (Thermo Fisher Scientific) supplemented with sulfamethoxazole, sulfamethazine, sulfisoxazole or sulfadiazine. E. coli TOP10 carrying vector pCR4-TOPO (Thermo Fisher Scientific) was used as control.
Accession Numbers
The insert sequences of the plasmids carried by metagenomic library clones showing decreased susceptibility to sulfamethoxazole or tetracycline have been submitted to GenBank under accession numbers MK159018 to MK159025.
Results and Discussion
In order to discover so far unknown ARGs in environmental resistomes, soil metagenomic libraries were subjected to function-based screening. As sequence information is not required before screening, this is the only strategy that bears the potential to discover entirely novel ARGs (Simon and Daniel, 2009). In addition, it is selective for full-length genes and functional gene products. The soil used for construction of metagenomic libraries was derived from forest and grassland varying in land use history. Fertilized and non-fertilized grassland sites as well as pristine and age class forest sites, harboring different dominant tree species, were considered (Table 1). This enabled the identification of ARGs in soils from hardly as well as intensively managed ecosystems.
Metagenomic libraries contained approximately 39,800–559,000 clones (Table 4). The quality of the libraries was controlled by determining the average insert sizes and the percentage of insert-bearing E. coli clones. The average insert sizes of metagenomic DNA-containing plasmids ranged from 2.6 to 6.0 kb and the frequency of clones carrying plasmid inserts was at least 73% (Table 4).
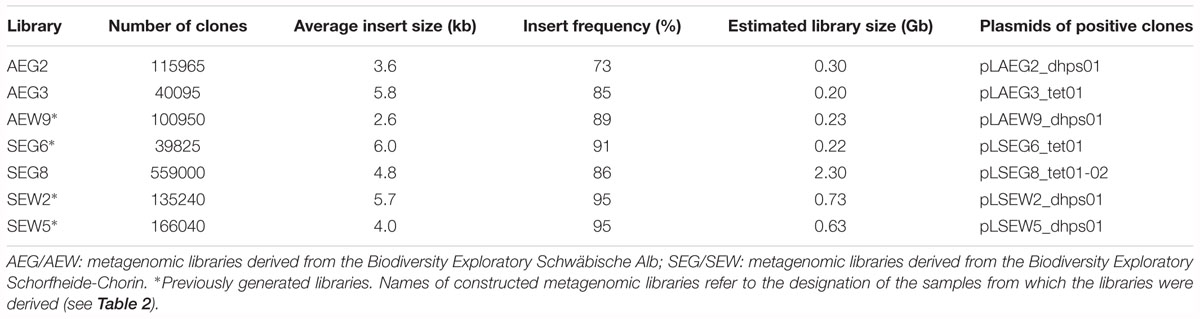
Table 4. Characterization of soil metagenomic libraries and designation of plasmids harbored by positive clones.
Novel ARGs Derived From Phylogenetically Divergent Soil Bacteria
The soil-derived metagenomic libraries were screened for resistance against tetracycline and sulfamethoxazole using selective agar medium. We recovered eight positive E. coli clones, harboring plasmids listed in Table 4, from functional screens. The entire inserts of these plasmids were sequenced and taxonomically classified, which revealed in all cases a bacterial origin (Supplementary Table S1). Some of the insert sequences are affiliated to Gram-negative bacterial phyla including Bacteroidetes and Proteobacteria whereas others belong to Actinobacteria (Supplementary Table S1). Noteworthy, one of the insert sequences was affiliated to the poorly characterized candidate phylum Zixibacteria.
Forsberg et al. (2014) reported that bacterial phyla, which were abundant in soil samples as determined by 16S rRNA gene sequencing, were also well-represented among taxa inferred from antibiotic resistance-conferring metagenomic library inserts derived from the same samples. Previously, we detected Proteobacteria, Actinobacteria, Bacteroidetes, and Chloroflexi among the dominant phyla in soils of our study sites via pyrosequencing of 16S rRNA genes (Kaiser et al., 2016). These phyla were also covered by the antibiotic resistance-conferring inserts described in this study (see Supplementary Table S1). Despite their high-GC content and predicted transcriptional incompatibilities with E. coli, also Actinobacteria were represented with respect to inserts of positive clones reported here and by Forsberg et al. (2014). The taxonomic origins of our resistance-conferring inserts show that the metagenomic library host E. coli allows identification of ARGs carried by phylogenetically divergent soil bacteria.
Forest Soil Not Exposed to Synthetic Drugs Harbors Sulfonamide-Resistant DHPSs
Sulfonamides are synthetic antimicrobial compounds targeting the folic acid pathway enzyme DHPS. Although all forest sites analyzed in this study exhibit no history of exposure to these synthetic compounds, three genes, AEW9_dhps01, SEW2_dhps01, and SEW5_dhps01, conferring sulfonamide resistance, were recovered from beech or pine forest soil (Tables 1, 5 and Figure 1). Furthermore, with respect to both forest sites (SEW2 and SEW5) located in the Schorfheide-Chorin exploratory (Northeastern Germany), as well as the forest site (AEW9) located in the Schwäbische Alb exploratory (Southwestern Germany), to our knowledge soils were not exposed to chemicals that resemble sulfonamides in their molecular structure. Especially, in case of the site AEW9 it is unlikely that such chemicals were spread, as this site belongs to an unmanaged beech forest. Resistance to sulfonamides is commonly mediated by the mobile DHPS-encoding genes sul1, sul2, or sul3 (Sköld, 2000; Perreten and Boerlin, 2003), which have been detected in various environments such as shrimp ponds, swine farm wastewater and manured soil (Phuong Hoa et al., 2008; Wang et al., 2014), but also in clinical isolates (Grape et al., 2003). To our knowledge, we report here for the first time the presence of functional non-mobile sulfonamide-resistant DHPSs in forest soil ecosystems. The deduced gene products of AEW9_dhps01, SEW2_dhps01, and SEW5_dhps01 showed only 46 to 58% amino acid sequence identities to the closest known DHPSs over the full length proteins (Table 5). Furthermore, AEW9_dhps01 harbors the alternative start codon GTG (all other detected dhps genes harbored the start codon ATG).
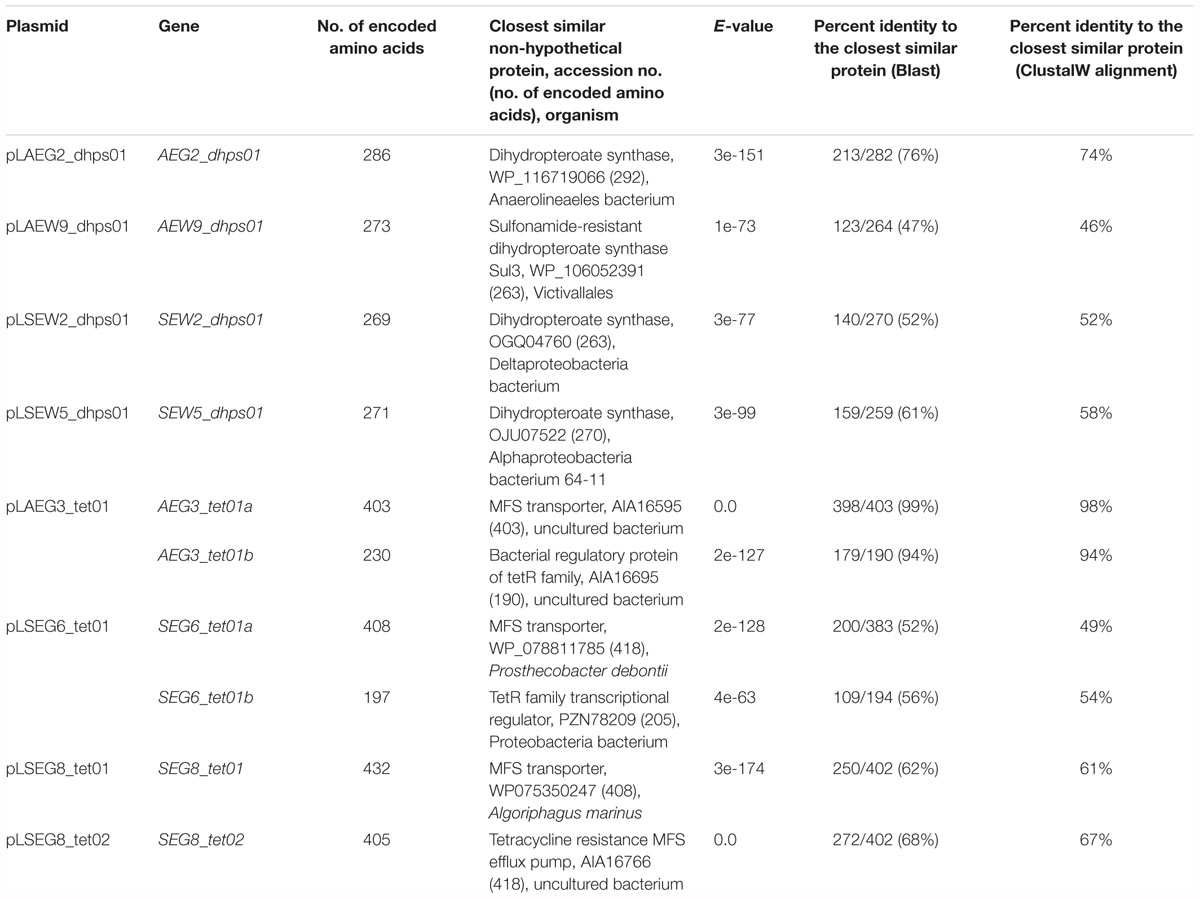
Table 5. Proteins encoded by genes associated with antibiotic resistance and their observed sequence identities.
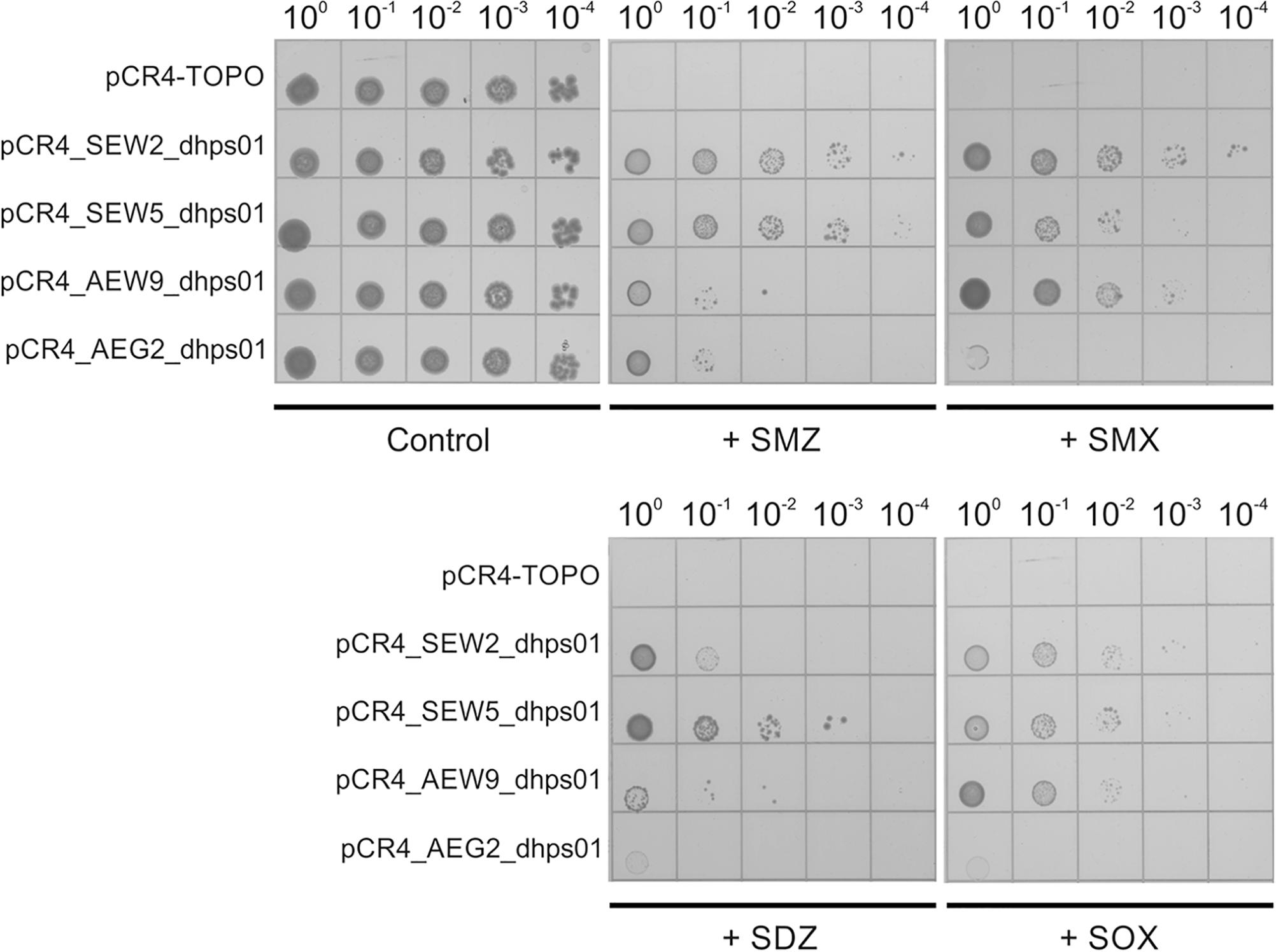
Figure 1. Resistance against sulfonamide antibiotics mediated by SEW2_dhps01, SEW5_dhps01, AEW9_dhps01, and AEG2_dhps01. Five microliters of serially diluted E. coli TOP10 cultures with starting OD600 of 0.5 were spotted onto Iso-Sensitest agar plates supplemented with 1000 mg/L sulfamethazine (+ SMZ), 250 mg/L sulfamethoxazole (+ SMX), 250 mg/L sulfadiazine (+ SDZ) or 500 mg/L sulfisoxazole (+ SOX). Iso-Sensitest agar plates with no sulfonamide added (control) were also included. E. coli TOP10 cultures carrying the cloning vector pCR4-TOPO, pCR4_SEW2_dhps01, pCR4_SEW5_dhps01, pCR4_AEW9_dhps01 or pCR4_AEG2_dhps01 were considered.
Phylogenetic analysis revealed that SEW2_DHPS01 exhibits homology with a putative DHPS affiliated to Deltaproteobacteria (Figure 2). Nevertheless, it has so far not been analyzed if this putative enzyme represents a functional DHPS, which can confer resistance to sulfonamides. In contrast to SEW2_DHPS01, AEW9_DHPS01 showed low identity (46%) to a DHPS with confirmed sulfonamide resistance, but branched separately from this enzyme affiliated to the Lentisphaerae (family Victivallales), in a phylogenetic tree (Figure 2). The remaining sulfonamide resistance-conferring enzyme identified in forest soil, SEW5_DHPS01, was most similar (58% identity) to a DHPS from Alphaproteobacteria. Strikingly, no mobile genetic elements were predicted with respect to the inserts comprising AEW9_dhps01, SEW2_dhps01, and SEW5_dhps01. This indicates that different bacterial phyla colonizing forest soil ecosystems harbor DHPSs, which are naturally insensitive to the inhibitory effects of sulfonamides. Furthermore, our results show that forest soil-derived DHPSs can provide high-level resistance in E. coli TOP10 (Figures 1, 3) and therefore potentially also in clinically relevant Enterobacteriaceae. As sulfonamides are used to treat gastrointestinal or urinary infections in human and belong to the most commonly sold and administered veterinary antibiotics (De Briyne et al., 2014; Santman-Berends et al., 2014), mobilization and spread of so far unknown genes conferring resistance to these synthetic compounds would have severe consequences, especially for the animal sector. In particular, SEW2_DHPS01 and SEW5_DHPS01 exhibited high-level resistance toward sulfamethazine (Figure 1), which is widely used in food animal production (Lau et al., 2017).
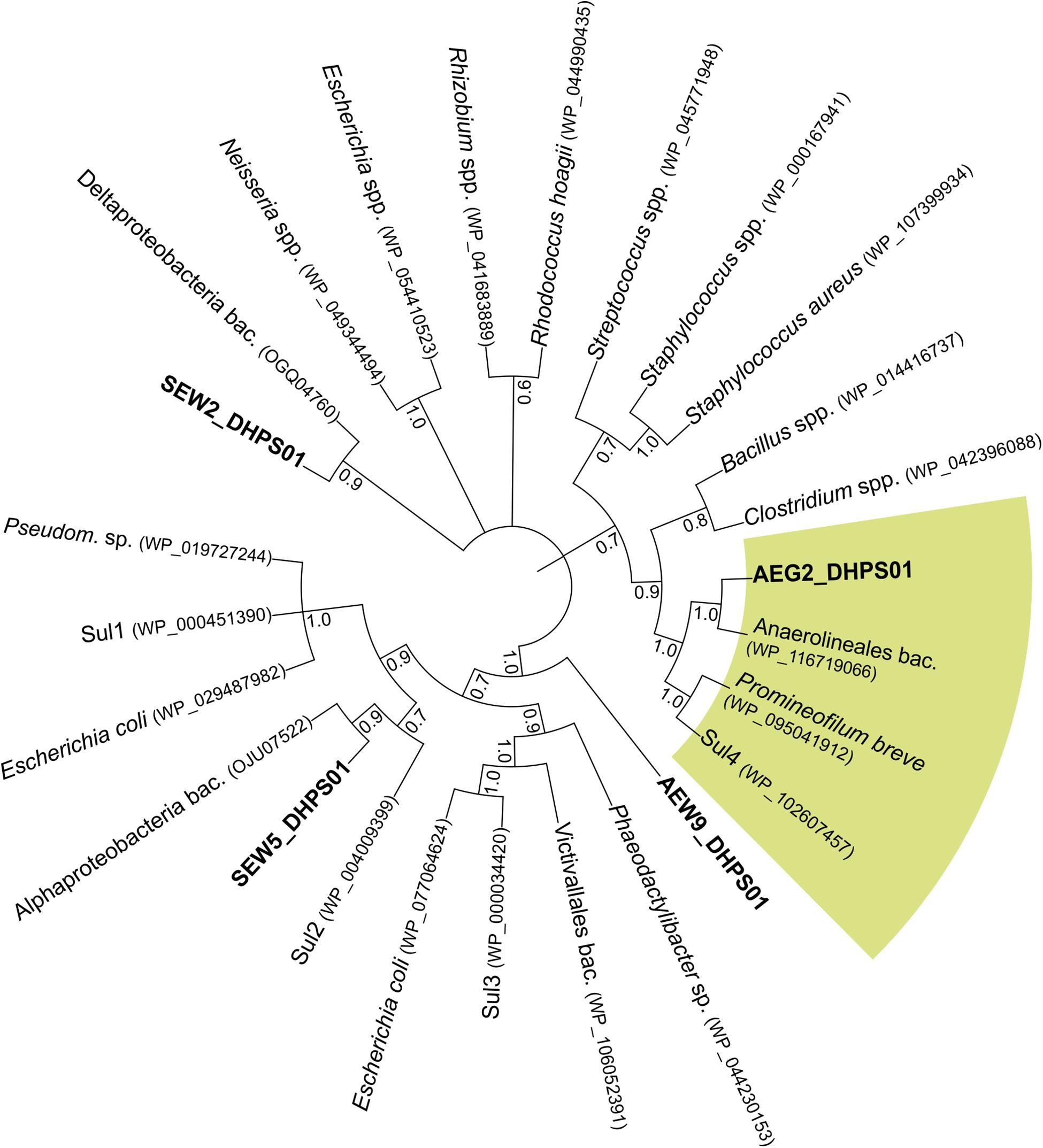
Figure 2. Neighbor-joining phylogenetic tree based on amino acid sequences of SEW2_DHPS01, SEW5_DHPS01, AEW9_DHPS01, AEG2_DHPS01 and other bacterial DHPSs. Besides DHPSs identified in this study, their closest related reference database entries, the mobile sulfonamide-resistant Sul1, Sul2, Sul3 and Sul4, and further DHPSs were considered. Bootstrap values based on 1,000 replicates are shown at the branching points. Branches are annotated with the identified taxon name and accession number in parentheses. The green segment indicates DHPSs belonging to Chloroflexi. Pseudom., Pseudomonas; bac., bacterium.
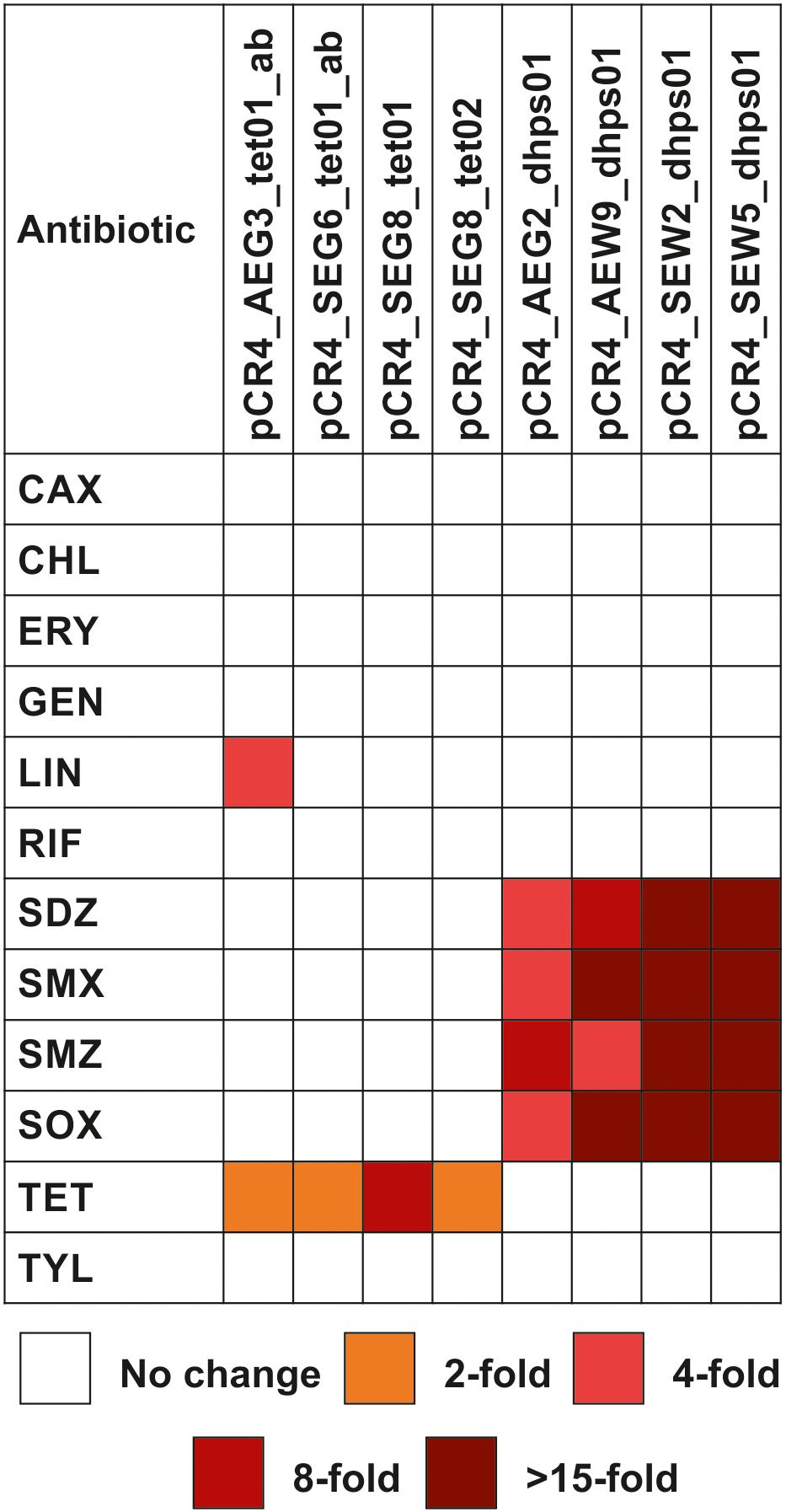
Figure 3. Antibiotic susceptibility profiles of E. coli TOP10 carrying soil-derived genes involved in antibiotic resistance. The genes were subcloned into plasmid vector pCR4-TOPO. MICs of antibiotics were determined using the broth microdilution method and are presented as fold increase relative to those for E. coli TOP10 carrying the cloning vector pCR4-TOPO. CAX, cefotaxime; CHL, chloramphenicol; ERY, erythromycin; GEN, gentamicin; LIN, lincomycin; RIF, rifampicin; SDZ, sulfadiazine; SMX, sulfamethoxazole; SMZ, sulfamethazine; SOX, sulfisoxazole; TET, tetracycline; TYL, tylosin.
Discovery of a Grassland Soil-Derived DHPS Affiliated to Chloroflexi
Recently, a fourth mobile sulfonamide resistance gene (sul4), encoding a DHPS phylogenetically related to representatives of the phylum Chloroflexi, has been discovered in polluted Indian river sediment (Razavi et al., 2017). This gene is flanked by an ISCR element, which is known to be involved in horizontal gene transfer (Razavi et al., 2017). In this study, we identified an enzyme (AEG2_DHPS01) with reduced susceptibility toward sulfonamides (Figure 1 and Table 6), showing similarity to DHPSs from Chloroflexi, in a fertilized grassland soil. AEG2_DHPS01 shares 76% sequence identity with a DHPS from a member of the Anaerolineae (Table 5) and clusters with different Chloroflexi DHPSs including Sul4 in a phylogenetic tree (Figure 2).
As sul4 is flanked by a partial folK ORF, it might have been decontextualized from a set of chromosomal genes involved in folate synthesis (Razavi et al., 2017). Nevertheless, Razavi et al. (2017) pointed out that further investigations on Chloroflexi could provide additional hints about the original host of sul4 and how it has been decontextualized. With respect to the insert carrying AEG2_dhps01, no genes potentially involved in folate synthesis were identified. Instead, AEG2_dhps01 is flanked by an ORF encoding a putative gene product with low similarity (23% identity) to a primosomal protein N′ (replication factor Y) – superfamily 2 helicase from an Anaerolineae bacterium (Supplementary Table S2). It is possible that this gene product can contribute to horizontal gene transfer between Chloroflexi and other bacterial taxa as helicases play a major role in replication, recombination, and repair of nucleic acid substrates (Flechsig et al., 2011; Byrd and Raney, 2012). Besides the potential helicase gene, AEG2_dhps01 is flanked by an ORF encoding a gene product with similarity to a hypothetical protein of an Anaerolineales bacterium.
Taxonomic analysis of the complete insert carrying AEG2_dhps01 confirmed that its original host belongs to the Chloroflexi (Supplementary Table S1). Thus, besides Sul4, AEG2_DHPS01 represents the so far only identified DHPS showing reduced susceptibility toward sulfonamides (Table 6), which is affiliated to the Chloroflexi. In order to analyze if sulfonamide resistance is a common characteristic of Chloroflexi, isolates belonging to this phylum should be analyzed with respect to susceptibility toward synthetic drugs in future surveys. Apart from sulfonamides, no decreased susceptibility toward other tested antibiotics was detected with respect to E. coli TOP10 carrying the subcloned dhps genes (Figure 3 and Table 6).
An Efflux Protein Conferring Reduced Tetracycline and Lincomycin Susceptibility
We identified four plasmids, pLAEG3_tet01, pLSEG6_tet01, pLSEG8_tet01, and pLSEG8_tet02, conferring efflux-mediated tetracycline resistance. All of these plasmids encode gene products with similarity to major facilitator superfamily (MFS) efflux proteins (Table 5). MFS efflux systems are widely distributed in both Gram-positive and Gram-negative bacteria (Sun et al., 2014). Accordingly, Wang et al. (2017) reported that 21 out of 24 tetracycline resistance genes, identified by functional metagenomics in Chinese soils, were affiliated to the MFS. The proteins encoded by these 21 genes showed identities ≥78% to the closest related reference database entries (Wang et al., 2017). In contrast, three out of four MFS representatives identified in this study shared ≤67% identity with their closest related proteins (Table 5). Besides an MFS representative, two insert sequences (corresponding plasmids, pLAEG3_tet01 and pLSEG6_tet01) encoded proteins with similarity to members of the TetR family of regulators (Table 5). These regulators are associated with antibiotic resistance and are known to control expression of MFS members (Cuthbertson and Nodwell, 2013). Noteworthy, the insert of plasmid pLtetSEG8_02 encodes a protein with similarity to an endonuclease (Supplementary Table S2), which might contribute to horizontal gene transfer.
McGarvey et al. (2012) identified a tetracycline-resistant metagenomic library clone, harboring a MFS representative, with reduced susceptibility toward rifampicin. Here, no resistance toward rifampicin was detected with respect to recombinant MFS producing E. coli clones (Figure 3). Nevertheless, the tetracycline-resistant clone carrying plasmid pCR4_AEG3_tet01ab showed reduced susceptibility toward lincomycin (Figure 3 and Table 6). The gene product AEG3_Tet01a encoded by this plasmid shows 98% identity to a soil-derived MFS from an uncultured bacterium (Table 5), which confers resistance to chloramphenicol. So far, it has not been analyzed if this chloramphenicol resistance mediating MFS identified by Forsberg et al. (2014) also encodes lincomycin resistance.
Conclusion
Our findings highlight the vast potential of functional metagenomics for the discovery of so far unknown antibiotic resistance determinants in environmental resistomes. We recovered several soil-derived target genes and proteins with low similarity to reference database entries from hardly as well as intensively managed forest and grassland, indicating that the resistance reservoir of the uncultured microbial majority is far from being extensively explored. As we detected here for the first time non-mobile DHPSs conferring resistance to sulfonamides in forest soil with no history of exposure to these synthetic drugs, it is possible that this characteristic naturally occurs in complex bacterial communities. Most of the detected antibiotic resistance determinants were not flanked by potential mobile genetic elements. Nevertheless, the recent finding of a fourth mobile sulfonamide resistance gene indicates ongoing forces that introduce, mobilize and maintain antibiotic resistance determinants in bacterial communities (Razavi et al., 2017). Considering, that several ARGs reported here conferred high-level resistance to non-pathogenic E. coli, it can be assumed that this could also be the case with respect to clinically relevant Enterobacteriaceae. In order to predict the emergence of antibiotic resistance, an extensive knowledge on environmental resistomes will be required, which might also direct the design of novel antibiotics that are less susceptible to resistance.
Data Availability
The datasets generated for this study can be found in GenBank, MK159018 to MK159025.
Author Contributions
HN designed the study. IW, AK, NA, DK, SB, FF, and HN carried out field and laboratory work. IW and HN prepared and analyzed the data. All authors interpreted the results and wrote the manuscript.
Funding
This work was funded by the DFG Priority Program 1374 “Infrastructure-Biodiversity-Exploratories” (NA 848/2-1) and the Open Access Publication Funds of the Göttingen University.
Conflict of Interest Statement
The authors declare that the research was conducted in the absence of any commercial or financial relationships that could be construed as a potential conflict of interest.
Acknowledgments
We thank the managers of the three Exploratories, Kirsten Reichel-Jung, Iris Steitz, Sandra Weithmann, Florian Straub, Katrin Lorenzen, Juliane Vogt, Miriam Teuscher and all former managers for their work in maintaining the plot and project infrastructure; Christiane Fischer for giving support through the central office, Andreas Ostrowski for managing the central data base, and Markus Fischer, Eduard Linsenmair, Dominik Hessenmöller, Daniel Prati, Ingo Schöning, François Buscot, Ernst-Detlef Schulze, Wolfgang W. Weisser, and the late Elisabeth Kalko for their role in setting up the Biodiversity Exploratories project. Field work permits were issued by the responsible state environmental offices of Baden-Württemberg, Thüringen, and Brandenburg. We thank Melissa Kocatuerk who contributed to the performance of the MIC assays.
Supplementary Material
The Supplementary Material for this article can be found online at: https://www.frontiersin.org/articles/10.3389/fmicb.2019.00460/full#supplementary-material
References
Allen, H. K., Moe, L. A., Rodbumrer, J., Gaarder, A., and Handelsman, J. (2009). Functional metagenomics reveals diverse beta-lactamases in a remote Alaskan soil. ISME J. 3, 243–251. doi: 10.1038/ismej.2008.86
Altschul, S. F., Gish, W., Miller, W., Myers, E. W., and Lipman, D. J. (1990). Basic local alignment search tool. J. Mol. Biol. 215, 403–410. doi: 10.1016/S0022-2836(05)80360-2
Blüthgen, N., Dormann, C. F., Prati, D., Klaus, V. H., Kleinebecker, T., Hölzel, N., et al. (2012). A quantitative index of land-use intensity in grasslands: integrating mowing, grazing and fertilization. Basic Appl. Ecol. 13, 207–220. doi: 10.1016/j.baae.2012.04.001
Byrd, A. K., and Raney, K. D. (2012). Superfamily 2 helicases. Front. Biosci. 17:2070–2088. doi: 10.2741/4038
Cheng, J., Romantsov, T., Engel, K., Doxey, A. C., Rose, D. R., Neufeld, J. D., et al. (2017). Functional metagenomics reveals novel β-galactosidases not predictable from gene sequences. PLoS One 12:e0172545. doi: 10.1371/journal.pone.0172545
Cuthbertson, L., and Nodwell, J. R. (2013). The TetR family of regulators. Microbiol. Mol. Biol. Rev. 77, 440–475. doi: 10.1128/MMBR.00018-13
Davies, J., and Davies, D. (2010). Origins and evolution of antibiotic resistance. Microbiol. Mol. Biol. Rev. 74, 417–433. doi: 10.1128/MMBR.00016-10
D’Costa, V. M., Griffiths, E., and Wright, G. D. (2007). Expanding the soil antibiotic resistome: exploring environmental diversity. Curr. Opin. Microbiol. 10, 481–489. doi: 10.1016/j.mib.2007.08.009
De Briyne, N., Atkinson, J., Pokludová, L., and Borriello, S. P. (2014). Antibiotics used most commonly to treat animals in Europe. Vet. Rec. 175:325. doi: 10.1136/vr.102462
Delmont, T. O., Robe, P., Cecillon, S., Clark, I. M., Constancias, F., Simonet, P., et al. (2011). Accessing the soil metagenome for studies of microbial diversity. Appl. Environ. Microbiol. 77, 1315–1324. doi: 10.1128/AEM.01526-10
Fischer, M., Bossdorf, O., Gockel, S., Hänsel, F., Hemp, A., Hessenmöller, D., et al. (2010). Implementing large-scale and long-term functional biodiversity research: the biodiversity exploratories. Basic Appl. Ecol. 11, 473–485. doi: 10.1016/j.baae.2010.07.009
Flechsig, H., Popp, D., and Mikhailov, A. S. (2011). In silico investigation of conformational motions in superfamily 2 helicase proteins. PLoS One 6:e21809. doi: 10.1371/journal.pone.0021809
Forsberg, K. J., Patel, S., Gibson, M. K., Lauber, C. L., Knight, R., Fierer, N., et al. (2014). Bacterial phylogeny structures soil resistomes across habitats. Nature 509, 612–616. doi: 10.1038/nature13377
Forsberg, K. J., Reyes, A., Wang, B., Selleck, E. M., Sommer, M. O., and Dantas, G. (2012). The shared antibiotic resistome of soil bacteria and human pathogens. Science 337, 1107–1111. doi: 10.1126/science.1220761
Gibson, M. K., Forsberg, K. J., and Dantas, G. (2015). Improved annotation of antibiotic resistance determinants reveals microbial resistomes cluster by ecology. ISME J. 9, 207–216. doi: 10.1038/ismej.2014.106
Grape, M., Sundström, L., and Kronvall, G. (2003). Sulphonamide resistance gene sul3 found in Escherichia coli isolates from human sources. J. Antimicrob. Chemother. 52, 1022–1024. doi: 10.1093/jac/dkg473
Kaiser, K., Wemheuer, B., Korolkow, V., Wemheuer, F., Nacke, H., Schöning, I., et al. (2016). Driving forces of soil bacterial community structure, diversity, and function in temperate grasslands and forests. Sci. Rep. 6:33696. doi: 10.1038/srep33696
Kumar, S., Stecher, G., and Tamura, K. (2016). MEGA7: molecular evolutionary genetics analysis version 7.0 for bigger datasets. Mol. Biol. Evol. 33, 1870–1874. doi: 10.1093/molbev/msw054
Landers, T. F., Cohen, B., Wittum, T. E., and Larson, E. L. (2012). A review of antibiotic use in food animals: perspective, policy and potential. Public Health Rep. 127, 4–22. doi: 10.1177/003335491212700103
Lau, C. H., van Engelen, K., Gordon, S., Renaud, J., and Topp, E. (2017). Novel novel antibiotic resistance determinants from agricultural soil exposed to antibiotics widely used in human medicine and animal farming. Appl. Environ. Microbiol. doi: 10.1128/AEM.00989-17 [Epub ahead of print].
Leplae, R., Lima-Mendez, G., and Toussaint, A. (2010). ACLAME: a classification of mobile genetic elements, update 2010. Nucleic Acids Res. 38, D57–D61. doi: 10.1093/nar/gkp938
Llorens, C., Futami, R., Covelli, L., Domínguez-Escribá, L., Viu, J. M., Tamarit, D., et al. (2011). The gypsy database (GyDB) of mobile genetic elements: release 2.0. Nucleic Acids Res. 39, D70–D74. doi: 10.1093/nar/gkq1061
Martínez, J. L. (2008). Antibiotics and antibiotic resistance genes in natural environments. Science 321, 365–367. doi: 10.1126/science.1159483
McGarvey, K. M., Queitsch, K., and Fields, S. (2012). Wide variation in antibiotic resistance proteins identified by functional metagenomic screening of a soil DNA library. Appl. Environ. Microbiol. 78, 1708–1714. doi: 10.1128/AEM.06759-11
Menzel, P., Ng, K. L., and Krogh, A. (2016). Fast and sensitive taxonomic classification for metagenomics with Kaiju. Nat. Commun. 7:11257. doi: 10.1038/ncomms11257
Nacke, H., and Daniel, R. (2014). “Approaches in metagenome research: progress and challenges,” in Encyclopedia of Metagenomics, ed. K. Nelson (New York, NY: Springer).
Nacke, H., Thürmer, A., Wollherr, A., Will, C., Hodac, L., Herold, N., et al. (2011a). Pyrosequencing-based assessment of bacterial community structure along different management types in German forest and grassland soils. PLoS One 6:e17000. doi: 10.1371/journal.pone.0017000
Nacke, H., Will, C., Herzog, S., Nowka, B., Engelhaupt, M., and Daniel, R. (2011b). Identification of novel lipolytic genes and gene families by screening of metagenomic libraries derived from soil samples of the German Biodiversity Exploratories. FEMS Microbiol. Ecol. 78, 188–201. doi: 10.1111/j.1574-6941.2011.01088.x
Nesme, J., and Simonet, P. (2015). The soil resistome: a critical review on antibiotic resistance origins, ecology and dissemination potential in telluric bacteria. Environ. Microbiol. 17, 913–930. doi: 10.1111/1462-2920.12631
Perreten, V., and Boerlin, P. (2003). A new sulfonamide resistance gene (sul3) in Escherichia coli is widespread in the pig population of Switzerland. Antimicrob. Agents Chemother. 47, 1169–1172. doi: 10.1128/AAC.47.3.1169-1172.2003
Perron, G. G., Whyte, L., Turnbaugh, P. J., Goordial, J., Hanage, W. P., Dantas, G., et al. (2015). Functional characterization of bacteria isolated from ancient arctic soil exposes diverse resistance mechanisms to modern antibiotics. PLoS One 10:e0069533. doi: 10.1371/journal.pone.0069533
Phuong Hoa, P. T., Nonaka, L., Hung Viet, P., and Suzuki, S. (2008). Detection of the sul1, sul2, and sul3 genes in sulfonamide-resistant bacteria from wastewater and shrimp ponds of north Vietnam. Sci. Total Environ. 405, 377–384. doi: 10.1016/j.scitotenv.2008.06.023
Piddock, L. J. (2006). Multidrug-resistance efflux pumps - not just for resistance. Nat. Rev. Microbiol. 4, 629–636. doi: 10.1038/nrmicro1464
Razavi, M., Marathe, N. P., Gillings, M. R., Flach, C. F., Kristiansson, E., and Joakim Larsson, D. G. (2017). Discovery of the fourth mobile sulfonamide resistance gene. Microbiome 5:160. doi: 10.1186/s40168-017-0379-y
Rutherford, K., Parkhill, J., Crook, J., Horsnell, T., Rice, P., Rajandream, M. A., et al. (2000). Artemis: sequence visualization and annotation. Bioinformatics 16, 944–945. doi: 10.1093/bioinformatics/16.10.944
Santman-Berends, I., Luttikholt, S., Van den Brom, R., Van Schaik, G., Gonggrijp, M., Hage, H., et al. (2014). Estimation of the use of antibiotics in the small ruminant industry in The Netherlands in 2011 and 2012. PLoS One 9:e105052. doi: 10.1371/journal.pone.0105052
Siguier, P., Perochon, J., Lestrade, L., Mahillon, J., and Chandler, M. (2006). ISfinder: the reference centre for bacterial insertion sequences. Nucleic Acids Res. 34, D32–D36. doi: 10.1093/nar/gkj014
Simon, C., and Daniel, R. (2009). Achievements and new knowledge unraveled by metagenomic approaches. Appl. Microbiol. Biotechnol. 85, 265–276. doi: 10.1007/s00253-009-2233-z
Sköld, O. (2000). Sulfonamide resistance: mechanisms and trends. Drug Resistance Updates 3, 155–160. doi: 10.1054/drup.2000.0146
Sun, J., Deng, Z., and Yan, A. (2014). Bacterial multidrug efflux pumps: mechanisms, physiology and pharmacological exploitations. Biochem. Biophys. Res. Commun. 453, 254–267. doi: 10.1016/j.bbrc.2014.05.090
Thaker, M., Spanogiannopoulos, P., and Wright, G. D. (2010). The tetracycline resistome. Cell. Mol. Life Sci. 67, 419–431. doi: 10.1007/s00018-009-0172-6
Thompson, J., Higgins, D., and Gibson, T. (1994). CLUSTAL W: improving the sensitivity of progressive multiple sequence alignment through sequence weighting, position-specific gap penalties and weight matrix choice. Nucleic Acids Res. 22, 4673–4680. doi: 10.1093/nar/22.22.4673
Walsh, F. (2013a). Investigating antibiotic resistance in non-clinical environments. Front. Microbiol. 4:19. doi: 10.3389/fmicb.2013.00019
Walsh, F. (2013b). The multiple roles of antibiotics and antibiotic resistance in nature. Front. Microbiol. 4:255. doi: 10.3389/fmicb.2013.00255
Walsh, F., and Duffy, B. (2013). The culturable soil antibiotic resistome: a community of multi-drug resistant bacteria. PLoS One 8:e65567. doi: 10.1371/journal.pone.0065567
Wang, N., Yang, X., Jiao, S., Zhang, J., Ye, B., and Gao, S. (2014). Sulfonamide-resistant bacteria and their resistance genes in soils fertilized with manures from Jiangsu Province, Southeastern China. PLoS One 9:e112626. doi: 10.1371/journal.pone.0112626
Wang, S., Gao, X., Gao, Y., Li, Y., Cao, M., Xi, Z., et al. (2017). Tetracycline resistance genes identified from distinct soil environments in china by functional metagenomics. Front. Microbiol. 8:1406. doi: 10.3389/fmicb.2017.01406
Keywords: soil metagenome, functional metagenomics, antibiotic resistance, dihydropteroate synthase, tetracycline resistance, sulfonamide resistance
Citation: Willms IM, Kamran A, Aßmann NF, Krone D, Bolz SH, Fiedler F and Nacke H (2019) Discovery of Novel Antibiotic Resistance Determinants in Forest and Grassland Soil Metagenomes. Front. Microbiol. 10:460. doi: 10.3389/fmicb.2019.00460
Received: 08 December 2018; Accepted: 21 February 2019;
Published: 07 March 2019.
Edited by:
Ziad Daoud, University of Balamand, LebanonReviewed by:
Atte Von Wright, University of Eastern Finland, FinlandGhassan M. Matar, American University of Beirut, Lebanon
Copyright © 2019 Willms, Kamran, Aßmann, Krone, Bolz, Fiedler and Nacke. This is an open-access article distributed under the terms of the Creative Commons Attribution License (CC BY). The use, distribution or reproduction in other forums is permitted, provided the original author(s) and the copyright owner(s) are credited and that the original publication in this journal is cited, in accordance with accepted academic practice. No use, distribution or reproduction is permitted which does not comply with these terms.
*Correspondence: Heiko Nacke, aG5hY2tlQGd3ZGcuZGU=