- 1Research Institute of Tropical Forestry, Chinese Academy of Forestry, Guangzhou, China
- 2Key Laboratory of Vegetation Restoration and Management of Degraded Ecosystems, South China Botanical Garden, Chinese Academy of Sciences, Guangzhou, China
- 3Research Institute of Subtropical Forestry, Chinese Academy of Forestry, Hangzhou, China
Numerous reforestation projects have been conducted to improve soil fertility in degraded forests, often causing alterations to the soil microbial communities. However, it remains unclear whether microbial functional groups are affected and how these groups correlate with an increase in the nutrient contents during reforestation. We investigated the abundance and composition of free-living nitrogen-fixing microorganisms (diazotrophs) by quantifying and sequencing the marker gene nifH in bulk soils from five reforestation approaches, including legumes and non-legumes, in subtropical China. The relationships between diazotrophic community attributes and soil nitrogen (N) content [NO3−, NH4+, and microbial biomass N (MBN)] were examined under various approaches. Abundance of diazotrophs was highest in the native tree plantation (Schima spp. and Michelia macclurei) and Acacia mangium monoculture (AM), and lowest in the Pinus massoniana monoculture. The diazotrophic abundance correlated positively with soil organic matter and water content while there was a negative correlation to pH. The composition of diazotrophic community differed significantly among the five reforestation approaches examined and was closely correlated with variations in soil pH, NH4+ and water content. Diazotrophic community composition was closely related to soil NH4+ content, whereas abundance was not. The AM contained higher NH4+, NO3− and MBN contents than the other reforestation approaches, which may be associated with the indicator species of diazotrophs (Actinobacteria, Proteobacteria, and Firmicutes). However, there were more indicator species of Proteobacteria in the mixed Acacia plantation (Acacia mangium and Acacia crassicarpa) than in AM, which might have contributed to the remarkedly lower N content compared to AM. Overall, the soil N content under reforestation appeared to be more related to the composition of diazotroph community than to the abundance of diazotrophs.
Introduction
Nitrogen (N) availability in forest soils plays a significant role in regulating plant growth, microbial activity and the interactions between plant and soil microorganisms (Kaye and Hart, 1997; Dijkstra et al., 2008). To maintain biodiversity and function of forest ecosystem, an adequate N input is needed. Unlike agricultural lands that receive N from fertilization, forests usually gain N from natural inputs, such as atmospheric N deposition, organic N decomposition and biological N fixation (BNF) (Mirza et al., 2014). The latter is the dominant form of N input in forest ecosystems, accounting for as much as 97% (Galloway et al., 2008). A recent study demonstrated different effects of N enrichment on soil organisms, carbon (C), and N mineralization with and without plantation in a semi-arid grassland (Chen et al., 2018). Therefore, vegetation induced indirect N inputs may have considerable effects on the functioning of soil ecosystem.
Biological N fixation is driven by a highly diverse group of microorganisms (Dixon and Kahn, 2004). All N-fixing microorganisms harbor the same functional gene nifH, which encodes one sub-unit of the nitrogenase enzyme and is widely used as a marker for studying the abundance and composition of N-fixers in various environments. Mirza et al. (2014) investigated the nifH gene and found that forest-to-pasture conversion in the Amazon rainforest altered the diazotrophic composition rather than their abundance. In Alaskan boreal soil, Penton et al. (2016) used nifH sequencing data to determine that the N-fixer composition in a permafrost thaw gradient varied with the depth of the water table. Previous studies mainly focused on free-living N-fixers (diazotrophs), although symbiotic N-fixers are conventionally recognized as the main drivers of BNF (Gutschick, 1981). Therefore, it is imperative that the features and determinants of free-living diazotrophs in different environments are evaluated to determine the responses of soil N to environmental changes and the affecting factors.
The decline in soil fertility caused by forest degradation is a severe ecological problem globally. In forest ecosystems, the soil N content is sensitive to deforestation and soil erosion (Atucha et al., 2013). Deforestation can decrease soil organic N by reducing litter input and root exudates. Moreover, large amounts of plant biomass N cannot return to soil after deforestation but is emitted into atmosphere as N oxides when wood is burned as fuel (Silva et al., 2011). Reforestation is important to control soil degradation and improve soil fertility (Albert, 2015). The effects of reforestation on soil N generally vary with the plant species (Yuan et al., 2012; Wang et al., 2013). To gain N quickly, leguminous species are introduced to degraded land. Wang et al. (2010) compared the soil properties and N transformations between N-fixing and non-N-fixing plantations, and found that N-fixing plants were more effective in recovering N cycling processes in degraded subtropical forests. Franco and Faria (1997) discussed the importance of leguminous trees in land reclamation and sustainability of the tropical systems reporting that these trees increased the soil N stock by 0.19 Mg ha−1 year−1 in tropical forests, which promoted successful revegetation of degraded land. However, whether the increased soil N supply after planting legumes correlates with changes in the diazotrophic community abundance and composition remains unclear. Some researchers argue that planting local dominant species is more important in recovering the ecosystem’s function (Mcnamara et al., 2006; Hayden et al., 2010; Lu et al., 2017), while others demonstrated that the natural recovery of vegetation without human disturbance was an effective approach for soil nutrient sequestration (Zheng et al., 2004). However, limited studies have associated the re-establishment of soil nutrient content with alterations in specific microbial functional groups during reforestation.
The composition and abundance of the diazotrophic community in soil could be correlated with several factors, including soil N, C and phosphorus contents, soil texture, pH, soil temperature and moisture (Limmer and Drake, 1996, 1998; Burgmann et al., 2005; Hsu and Buckley, 2009). Moreover, the correlations between diazotrophic community and environmental factors often differ among vegetation types. Soil physicochemical properties could be modified by specific plant species through litter-fall, root phenes, and exudates containing different nutrients, which subsequently influence the microenvironment of the diazotrophic community (Saleem et al., 2018). The different N requirements of specific plant species may be an underlying driver of the diazotrophic community. The specific abundance and composition of diazotrophic community and their associations with soil N availability under different tree species are largely unknown. This limits our ability to evaluate the effectiveness of reforestation using various tree species.
In this study, we investigated the responses of the diazotrophic community in five reforestation approaches in subtropical China. One approach was natural revegetation (NR), and the other four were artificial plantations of different tree species, including a non-native legume monoculture of Acacia mangium (AM), non-native mixed legumes (ML; Acacia mangium and Acacia crassicarpa), native dominant species (NP; Schima spp. and Michelia macclurei) and native Pinus massoniana monoculture (PM). The abundance and composition of diazotrophs in growing season were determined using real-time PCR and amplicon sequencing targeting nifH. Correlations between soil physicochemical properties with abundance and composition of diazotrophic community were examined. Our objectives were to (1) clarify the abundance and composition of the diazotrophic community under the five reforestation approaches and their correlations with soil N content, and (2) analyze the predominant soil properties that relates to the abundance and composition of the diazotrophs under reforestation.
Materials and Methods
Study Site
This study was conducted at the Heshan National Field Research Station of Forest Ecosystem (112°50′E, 22°34′N), Guangdong Province, South China. This region has a subtropical monsoon climate, with mean annual precipitation of 1,668 mm and temperature of 22.5°C. The soil is classified as laterite (Oxisol in the USDA soil taxonomy), developed from sandstone. The original forest type is evergreen broadleaf with a large area severely disturbed owing to anthropogenic activities and deforestation, leading to soil erosion and degradation. Five reforestation approaches have been conducted in the degraded region since 2005. One approach was NR, and the other four were artificial plantations of different tree species, including a non-native legume monoculture of Acacia mangium (AM), non-native mixed legumes (ML; Acacia mangium and Acacia crassicarpa), native dominant species (NP; Schima spp. and Michelia macclurei) and native Pinus massoniana monoculture (PM). The NR was dominated by Miscanthus sinensis and Blechnum orientale, and a few shrubs, such as Rhodomyrtus tomentosa, Melastoma candidum, Gardenia jasminoides and Ilex asprella. The main understory shrubs were Limea rotundifolia, Aporosa dioica, Cinnamomum burmannii and Psychotria rubra in PM; Carallia brachiate, Syzygium levinei, and Syzygium cumini in AM; Ilex asprella, Rhodomyrtus tomentosa, and Dicranopteris pedata in ML; and Psychotria rubra, Aporosa dioica and Litsea glutinosa in NP. Each reforestation approach had three replicated plots, randomly distributed in the experimental area. The area of each plot is 1 ha, and the total area of the experimental region is 50 ha. Trees were planted with a spacing of 2 m × 3 m in the four plantations, and tree density was ∼1,650 ha−1.
Soil Sampling and Physicochemical Analysis
Soil sampling was conducted between trees to avoid the collection of rhizosphere soils. Two sampling sites were randomly selected in each plot during the growing season (August 2016), and three subsamples were collected at each site from the top layer (0–10 cm) using an anger (Φ 5 cm) and mixed thoroughly as one sample. Thus, two composite samples were collected in each plot, and a total of six composite samples were generated in each reforestation approach. These served as six replicates. Soil samples were sieved through a 2-mm mesh after removing litter, roots, and stones, and immediately taken to the laboratory in an ice box. Samples were divided into two: one was used for soil physicochemical analyses and stored at 4°C, while the other was used for the microbial analyses and stored at −20°C. Soil samples were analyzed within 2 weeks.
Soil pH was determined in a soil:water (1:2.5) suspension using a pH meter (Denver Instrument UB-7 pH/ev Meter, United States). The soil water content (SWC) was determined using the oven drying method. Soil organic matter (SOM), NH4+ and NO3− concentrations were measured using K2Cr2O7 oxidation, indophenol blue colorimetry and copperized cadmium reduction methods, respectively (Liu et al., 1996). Soil dissolved organic C (DOC) was determined from the 0.5 M K2SO4 extraction using a total organic C analysis instrument (TOC-VCSH, Shimadzu, Japan). Soil microbial biomass C (MBC) and microbial biomass N (MBN) were measured using the fumigation method (Vance et al., 1987). Briefly, 10 g of fresh soil was weighed into a glass beaker and fumigated with chloroform in a vacuum glass dryer for 24 h in dark. Then, the fumigated soil was extracted with 0.5 M K2SO4, and the extracted liquid from the un-fumigated soils was used for the DOC measurement. MBC and MBN were calculated as the difference in the C and N concentration, respectively, between fumigated and non-fumigated samples, divided by 0.45 and 0.54, respectively.
DNA Extraction and nifH Gene Quantification
Soil total DNA extraction and purification were performed using the HiPure Soil DNA Mini Kit (Magen, Guangzhou, China) with 0.3 g fresh soil. DNA solution was quantified using a NanoDrop 2000 spectrophotometer (Thermo Fisher Scientific Inc., United States), and the DNA solution was stored at −20°C for further analyses.
The nifH gene abundance was measured using absolute real-time PCR method on an ABI 7500 thermocycler system (Applied Biosystems, Foster City, CA, United States). The absolute real-time PCR correlates the PCR signal to input copy numbers using a calibration curve, and neither comparisons nor references are needed (Pfaffl, 2004). The primers nifHF (AAAGGYGGWATCGGYAARTCCACCAC) and nifHR (TTGTTSGCSGCRTACATSGCCATCAT) were used during real-time PCR (Rösch et al., 2002). A 96-well plate was used, with each well contained 12.5 μl of SYBR Premix Ex Taq (TaKaRa Biotechnology, Japan), 1 μl of each primer (10 mmol/L) and 2 μl of DNA template (1–10 ng). The following three-step amplification protocol was used for quantification: 1 cycle at 95°C for 30 s, and 40 cycles of 5 s at 95°C, 34 s at 55°C and 1 min at 72°C. A standard curve was generated from a 10-fold serial dilution (103–108 copies per μl) of plasmids extracted from clones containing the target functional gene fragment. The number of gene copies was directly calculated from the extracted plasmid DNA concentration, and was presented as functional gene abundance. The PCR efficiency and correlation coefficients (R2) for standard curves were 90.12% and 0.999, respectively.
NifH Gene Sequencing and Bioinformatics Analyses
The composition and diversity of diazotrophic community were detected using amplicon sequencing. Primers nifHF/nifHR tagged with multiplex identifier sequences were used in amplification of nifH sequences (Poly et al., 2001) with the Illumina Nextera adapter A on nifHF and adapter B on nifHR. Briefly, the PCR reaction was performed in 96-well plates (Axygen, United States) on a Bio-Rad S1000 thermal cycler (Bio-Rad Laboratory, Hercules, CA, United States), with 50-μl volumes in each well, which included 25 μl of 2× Premix Taq (TaKaRa Biotechnology, Japan), 2 μl of each primer (10 mM), 5 μl of DNA template (60 ng) and 16 μl of RNase free Ultra-Pure water. The amplification was performed under the following conditions: 5 min at 94°C; 30 cycles of 30 s at 95°C, 30 s at 55°C and 30 s at 72°C; followed by 10 min at 72°C. Each soil sample had three amplification replicates, and the PCR products from the three replicates were mixed. Then, the PCR products were pooled and purified using a PCR Purification Kit (Axygen Bio, United States) and diluted to a concentration of 10 ng ml−1 before sequencing. Finally, the paired-end sequencing of nifH amplicons was carried out using the Illumina HiSeq sequencer at Genepioneer Biotechnology Co., Ltd. (Nanjing, China).
Paired-end reads were merged with FLASH, and low quality sequences were discarded using QIIME (Caporaso et al., 2010). Chimeric composite sequences were filtered using Usearch (Edgar, 2010). Chimera-free nifH sequences were grouped into operational taxonomic units (OTUs) based on a threshold 97% identity level. The taxonomic classification of Chimera-free sequences was determined using the proGenomes database1. All nifH sequences obtained in this study have been deposited in GenBank under accession no. PRJNA486256.
To increase the taxonomic resolution of the nifH sequences (Lema et al., 2012; Mirza et al., 2014), the sequences were translated into amino acid sequences and then aligned on the functional gene pipeline (Fungene) within the Ribosomal Data Project (Cole et al., 2009). The aligned protein sequences were used for the clustering of operational protein units (OPUs) at 99%, 97%, 95%, 90%, and 80% similarity levels. A phylogenetic tree was constructed using OPUs containing more than five sequences on PHYML 3.0 with the maximum likelihood method (Guindon et al., 2005). A representative nifH sequence for each OPU was selected based on the relative abundance and was used for the taxonomic identification.
Composition and Diversity of the Diazotrophic Community
Shannon-Wiener index of the diazotrophic community was calculated for samples with the OTU table to analyze the species diversity. Non-metric multidimensional scaling (NMDS) based on the Bray–Curtis similarity was used to investigate the patterns of diazotrophic community structure. Statistical significance of the differences among five reforestation approaches was tested using the PerMANOVA method with 999 permutations (Anderson, 2001). The OTU tables of nifH abundance and presence/absence matrix were applied when conducting NMDS. Vegetation variables were converted to dummy binary variables before conducting the structural equation model (SEM) analysis, namely, PM = 1, ML = 2, NR = 3, NP = 4, and AM = 5. The SEM analysis was conducted to clarify how vegetation type could, directly and indirectly, affect diazotroph composition by using AMOS 21.0 (SPSS Inc., Chicago, IL, United States). Goodness of the model fits were examined by chi-square test (p > 0.05), goodness fit index (GFI > 0.95), comparative fit index (CFI > 0.95) and root mean square errors of approximation (RMSEA < 0.05) (Schermelleh-Engel et al., 2003). The model containing three soil properties (NH4+, SWC, and pH) showed the best fit, with p = 0.54, GFI = 0.96, CFI = 1, and RMSEA = 0. We therefore only reported the results of this model. Venn diagrams were drawn to show the unique and common OTUs between samples (Supplementary Figure S1). To identify the indicator species in each reforestation approach, the indicator values of the diazotrophic species were calculated under each approach as the product of the relative abundance and relative frequency of occurrence (Dufrene and Legendre, 1997). As a consequence, a greater indicator value was returned when more individuals of a species were found under a single reforestation approach, and the species occurred in more sampling sites under the same reforestation approaches (Supplementary Table S1). The statistical significance of the indicator value was assessed using a 999-site randomization procedure (Edgington, 1995). Briefly, in each randomization, samples were randomly redistributed and an indicator value was returned. A 95% confidence range was obtained by calculating the 2.5 and 97.5 percentiles of the randomly generated indicator values. If the tested indicator value was outside of the 95% confidence range, then the tested value was significant. All analyses were performed on R 3.3.2 (R Core Team, 2013) using the packages “Vegan” (Oksanen et al., 2013) and “labdsv” (Roberts, 2013).
Statistical Analyses
The normality (Kolmogorov–Smirnov test) and homogeneity of variances (Levène test) for variables were determined before statistical analyses. One-way analyses of variance with Tukey’s multiple comparisons were used to test the differences of soil physicochemical properties and diazotrophic community attributes among the five reforestation approaches. Soil physicochemical variables and the NH4+:NO3− ratio were subjected to principal component analyses to characterize and explain the soil nutrient conditions of different reforestation approaches. Spearman correlations between soil physicochemical variables and nifH abundance were calculated.
The OTU table of indicator species and soil physicochemical properties were subjected to canonical correspondence analysis (CCA) to detect the determining factors of the indicator species. The best CCA model was selected based on the Akaike’s Information Criterion value. The significance of the selected CCA model and the soil properties included in the model were tested by 999 permutations (Legendre and Legendre, 2012). All analyses were performed on R 3.3.2 (R Core Team, 2013) with the package “Vegan” (Oksanen et al., 2013). The significance level for the analyses was set as p < 0.05.
Results
Soil Physicochemical Properties
In the five reforestation approaches, the soil properties varied significantly except for soil pH, NO3−, MBN content and MBC:MBN ratio (Figure 1 and Supplementary Figure S2B). The SWC was significantly higher in NP and AM, and soil MBC and MBN was greater in AM than in PM, ML, and NR (Figures 1G,H). The soil NH4+content and the NH4+:NO3− ratio was significantly higher in NR and AM (Figure 1C and Supplementary Figure S2A). However, the soil DOC was considerably lower in NR than in the other reforestation approaches (p < 0.05, Figure 1E).
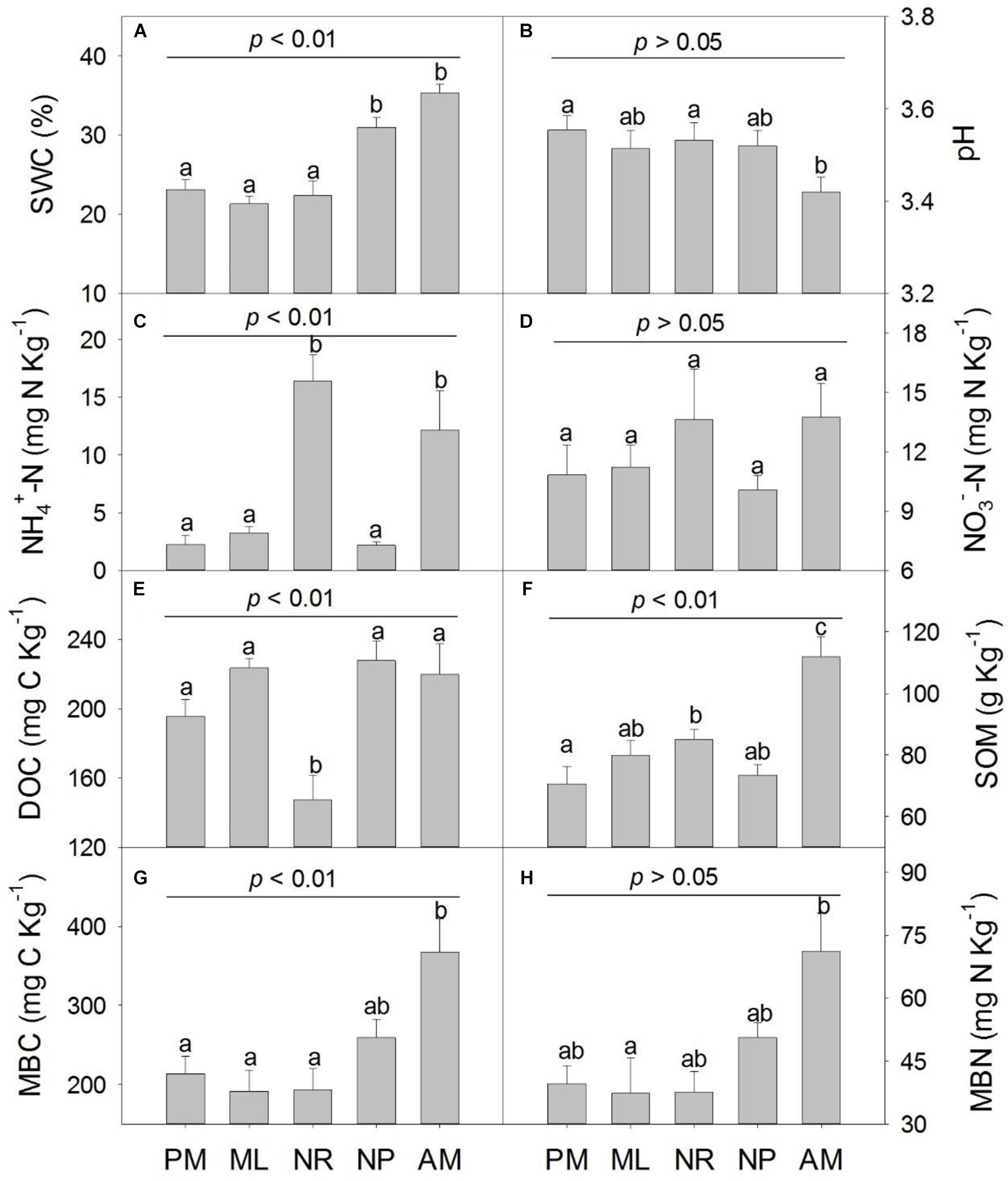
Figure 1. Soil physicochemical properties in five reforestation approaches in subtropical China. (A) soil water content (SWC), (B) soil pH, (C) soil NH4+ content, (D) soil NO3− content, (E) soil dissolved organic carbon (DOC), (F) soil organic matter (SOM), (G) soil microbial biomass carbon (MBC) and (H) soil microbial biomass nitrogen (MBN). The five reforestation approaches were: Pinus massoniana monoculture (PM), mixed Acacia crassicarpa and Acacia mangium (ML), natural revegetation (NR), native tree plantation (NP), and Acacia mangium monoculture (AM). Error bars represent the standard errors of the mean (n = 6). The upper p-value in each chart represent the overall difference in the variation among five approaches; different lowercase letters indicate the significant differences of multiple comparisons at p ≤ 0.05.
Principal component analysis showed that 65.7% of the total variance of soil properties in the five approaches could be explained by the first two principle components (PC1 and PC2) (Supplementary Figure S3). AM had higher SWC, NO3−, SOM, MBC, and MBN contents whereas NH4+ content and NH4+:NO3− ratio were higher in NR. PM, ML, and NP had lower SWC, MBC, MBN, SOM, NO3−, NH4+, and NH4+:NO3− ratio while higher DOC and pH (Supplementary Figure S3).
Abundance and Composition of the Diazotrophic Community
The nifH gene was most abundant in NP, followed by AM, NR, and ML (Figure 2A). PM had the lowest abundance of nifH compared to the other reforestation approaches, whereas the greatest Shannon-Wiener index for nifH was observed in PM (p > 0.05, Figure 2B). The total content of soil DNA was significantly higher in NP and AM than in PM (Figure 2C). The composition of diazotrophic communities differed significantly among the approaches, as indicated by the statistical significance of both the OTU abundance (F4,20 = 2.24, p = 0.001, Figure 3A) and OTU absence/presence (F4,20 = 2.19, p = 0.001, Supplementary Figure S4).
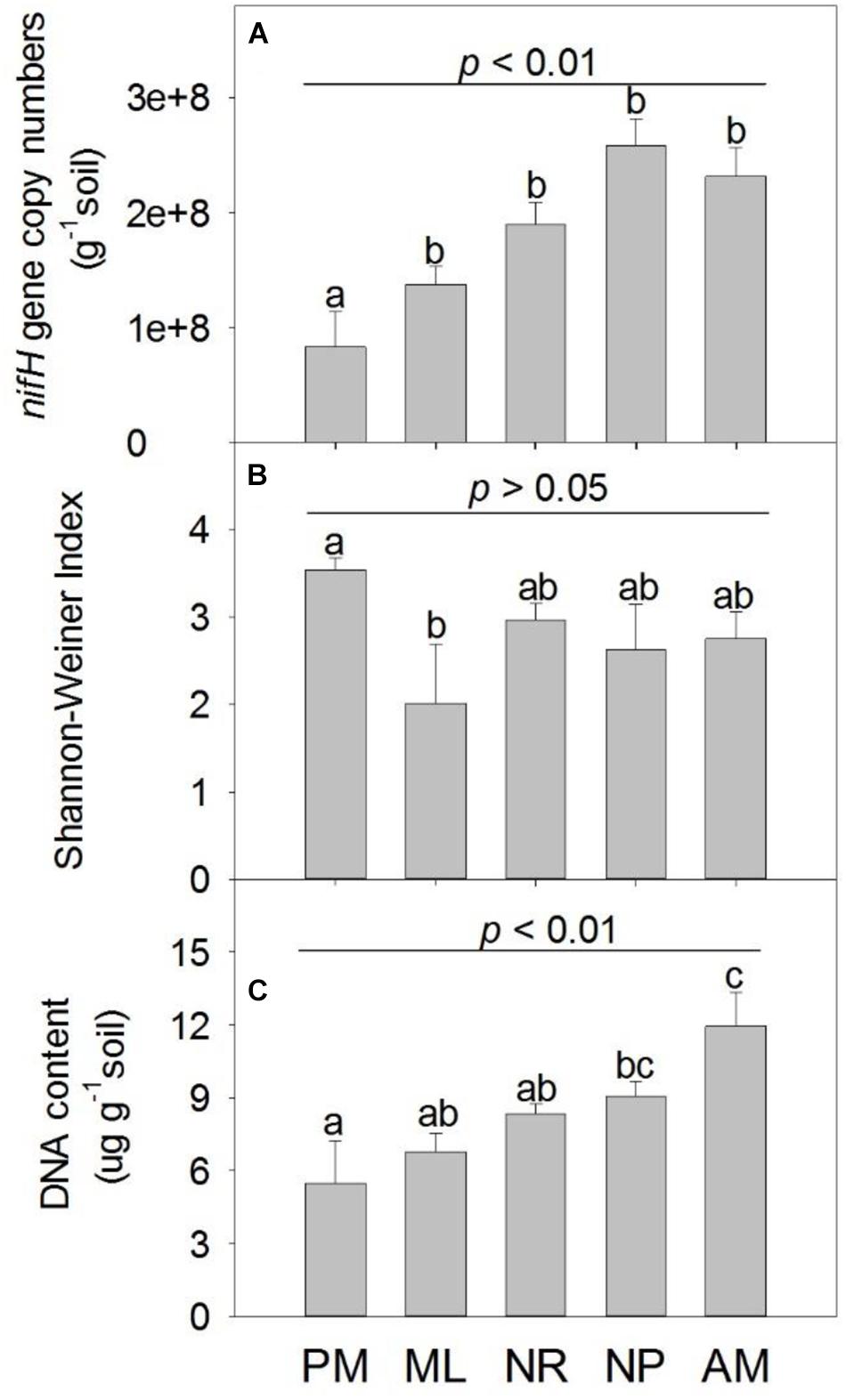
Figure 2. The (A) nifH gene copy numbers, (B) Shannon-Wiener Index of nifH and (C) soil total DNA content under five reforestation approaches in subtropical China. The five approaches were: Pinus massoniana monoculture (PM), mixed Acacia crassicarpa and Acacia mangium (ML), natural revegetation (NR), native tree plantation (NP), and Acacia mangium monoculture (AM). Error bars represent the standard errors of the mean (n = 6). The upper p-value in each chart represent the overall difference in the variation among five approaches; different lowercase letters indicate the significant differences of multiple comparisons at p ≤ 0.05.
The indicator species, which contributed to the differences in community composition, were identified by an indicator species analysis. PM had 31 indicator species that were dominated by the orders Rhizobiales and Clostridiales, such as Bradyrhizobium, Pleomorphomonas, and Ruminiclostridium. Ten indicator species were observed in the ML, and these species were mainly distributed in the orders Rhodospirillales (Azospirillum lipoferum), Rhizobiales (Azorhizobium doebereinerae) and Holophagales (Holophaga foetida). The NR was characterized by 13 indicator species, including Oceanospirillales, Rhodobacterales, and Corynebacteriales. NP and AM had 7 and 10 indicator species, respectively (Supplementary Table S1).
Associations of Diazotrophic Community Abundance and Composition With Soil Properties
The Spearman correlation, SEM and CCA were used to assess the associations between diazotrophic communities and soil physicochemical properties. Overall, nifH abundance and total soil DNA content were positively correlated with SWC and SOM (p < 0.05, Table 1), and negatively correlated with soil pH (p < 0.05, Table 1). Additionally, the total soil DNA content showed positive correlations to MBC and MBN (p < 0.05, Table 1). SEM analysis revealed that diazotrophic community composition based on the nifH gene was significantly correlated with NH4+ and SWC (Figure 3B). A 39–45% variation in nifH community composition among the reforestation approaches could be explained by the variations in vegetation type, soil NH4+, SWC, and pH. Results of CCA revealed that indicator nifH species among the approaches were mainly correlated with differences in soil pH, SWC, and NH4+ content (p < 0.05, Figure 4).
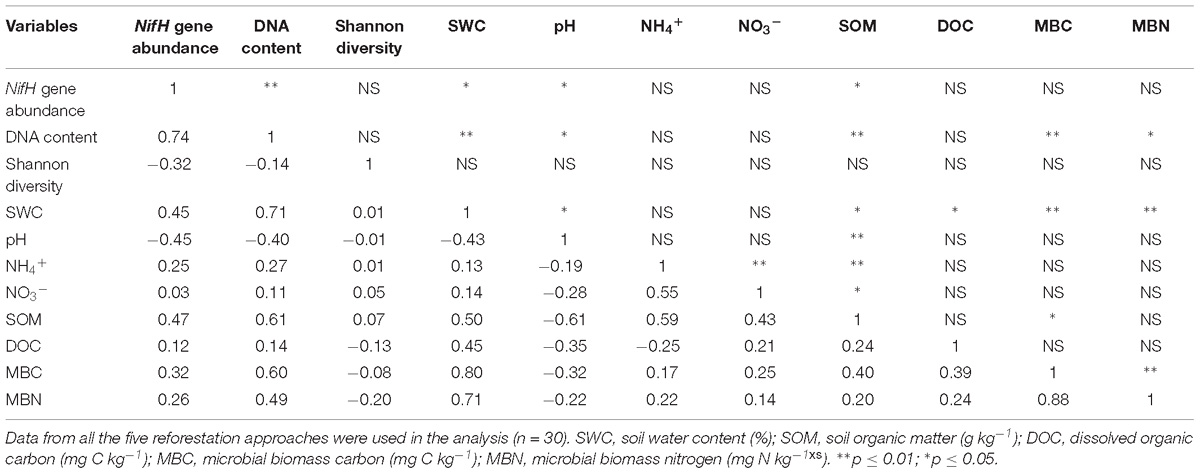
Table 1. Pearson correlation (r) between microbial attributes (DNA content, nifH abundance and Shannon-Wiener diversity) and soil physicochemical properties (SWC, pH, NH4+, NO3−, SOM, DOC, MBC, and MBN).
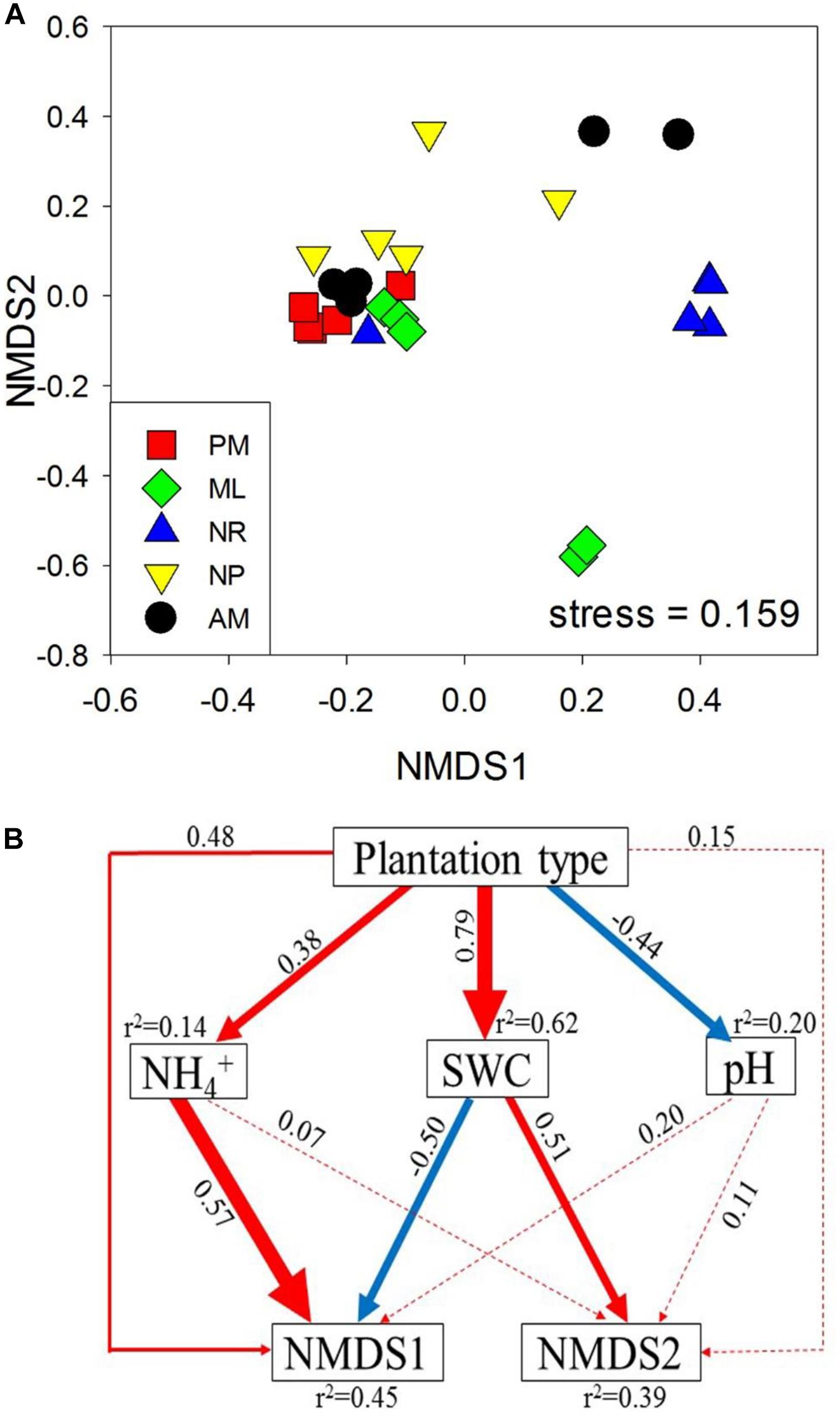
Figure 3. (A) Bray–Curtis similarity based nonmetric multidimensional scaling (NMDS) of the diazotrophic communities, determined by nifH amplicon sequencing, and (B) a structural equation model (SEM) analysis showing the potentially direct and indirect correlations between the diazotrophic community, represented by the two axes of the NMDS from (A), and soil properties. Red and blue arrows indicate the positive and negative relationships, respectively. Solid and dashed arrows indicate significant and non-significant correlations, respectively, and the widths of the arrows indicate the significance levels of the correlation coefficients. Numbers adjacent to arrows are path coefficients, and the r2 values represent the explained variation of the target variables. The five approaches were: Pinus massoniana monoculture (PM), mixed Acacia crassicarpa and Acacia mangium (ML), natural revegetation (NR), native tree plantation (NP), and Acacia mangium monoculture (AM).
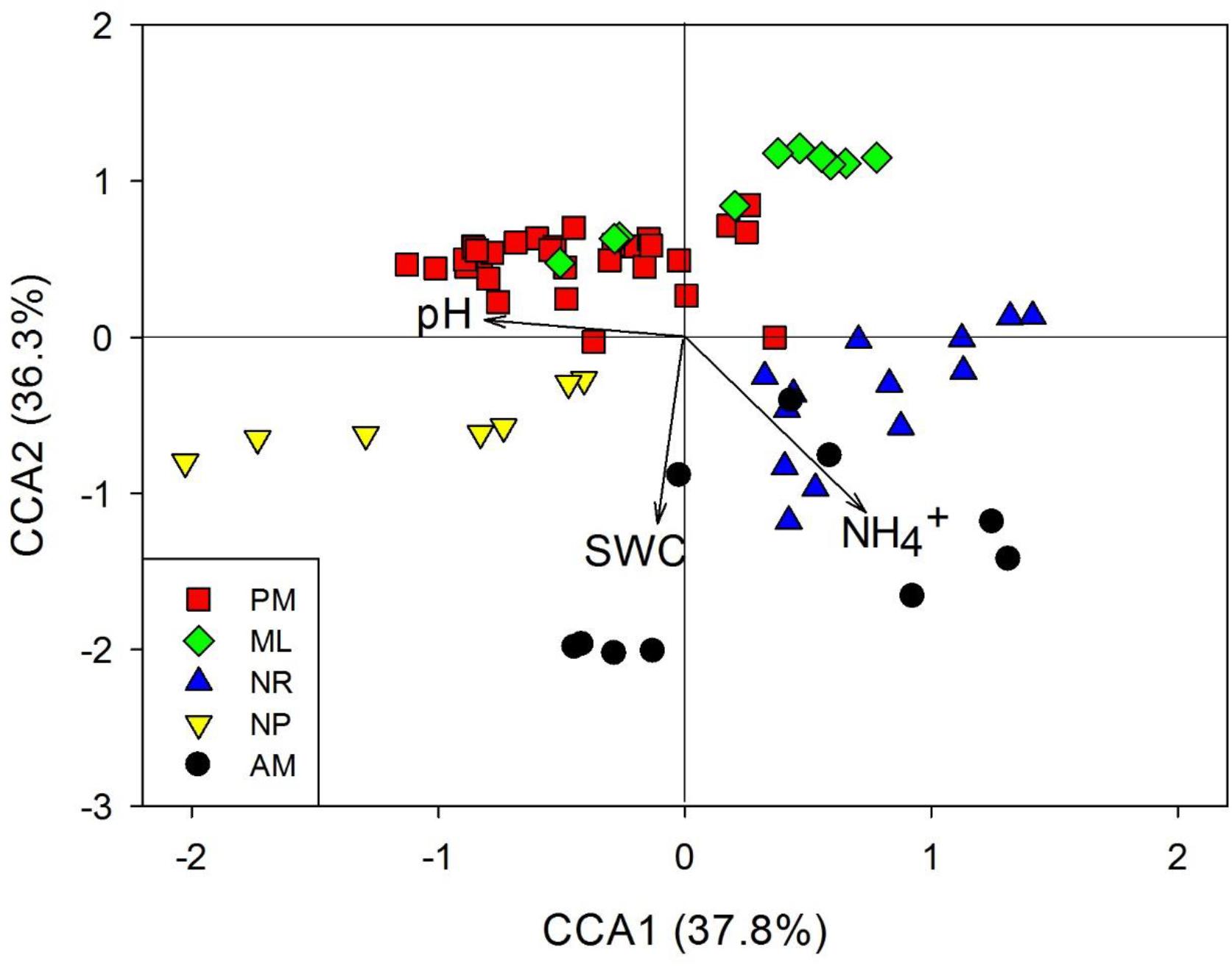
Figure 4. Canonical correspondence analysis (CCA) of the indicator nifH species composition among five reforestation approaches. The statistically significant environmental variables are presented as arrows. The five approaches were: Pinus massoniana monoculture (PM), mixed Acacia crassicarpa and Acacia mangium (ML), natural revegetation (NR), native tree plantation (NP), and Acacia mangium monoculture (AM).
OPU Analyses
The nifH sequences were translated into amino acid sequences and then aligned on the functional gene pipeline (Fungene) within the Ribosomal Data Project to cluster the OPUs. The OPU identification and phylogenetic clustering of the translated nifH sequences were used to investigate the responses of the diazotrophic community to different reforestation approaches at the protein level. A total of 150 OPUs were identified based on the 90% similarity cutoff for translated amino acid sequences. These OPUs contained more translated amino acid sequences (80%) than those identified based on other similarity cutoffs, such as 99%, 97%, 95%, and 80%. Consequently, we only focused on the OPUs from the 90% similarity cutoff. A total of 27 OPUs containing more than five sequences each were used to construct the phylogenetic tree (Figure 5). Among the 27 OPUs, more than 50% of the sequences were clustered into OPUs belonging to Archaea (OPU2, OPU85, OPU98, OPU102, and OPU109), followed by the OPUs related to Bacteroidetes (15%), Proteobacteria (11%), and Firmicutes (9%). Two OPUs containing 14% of the sequences (OPU10 and OPU78) were similar to the sequences of uncultured microorganisms. The distributions of these OPUs varied substantially among the five reforestation approaches. For example, OPUs assigned to Archaea (OPU2, OPU85, and OPU98), Bacteroidetes (OPU37), and δ-Proteobacteria (OPU19, OPU92, and OPU137) were more commonly found in NR (83%, 73%, and 96% of the total sequences in Archaea, Bacteroidetes, and δ-Proteobacteria were from NR). Three OPUs (OPU27, OPU128, and OPU141; most closely related to α- and γ-Proteobacteria) were more commonly found in NP (100% and 70% of the total sequences in α- and γ-Proteobacteria were from NP). OPU33 and OPU140 (affiliated with Firmicutes) were only detected in AM, and OPU96 only presented in ML. Five OPUs (OPU30, OPU102, OPU109, OPU122, and OPU130; most closely related to Bacteroidetes, Archaea, and Proteobacteria) were more commonly found in PM.
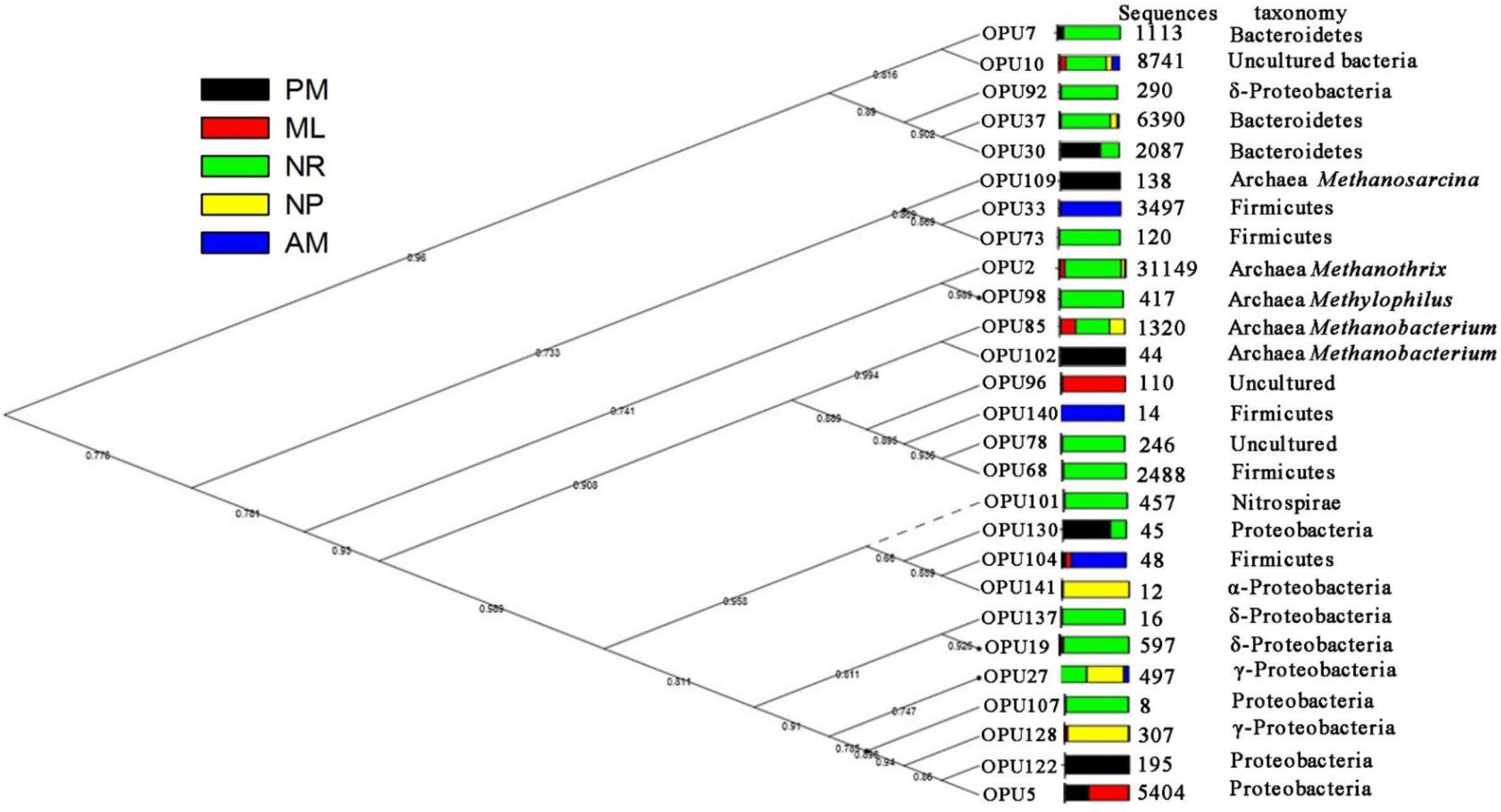
Figure 5. Maximum likelihood phylogenetic tree of translated amino acid sequences of the nifH gene in five reforestation approaches: black, Pinus massoniana monoculture plantation (PM); red, mixed Acacia crassicarpa and Acacia mangium (ML); green, natural revegetation (NR); yellow, native tree plantation (NP); and blue, Acacia mangium monoculture plantation (AM). Only the operational protein units (OPU) containing more than five sequences are displayed. The bootstrap support values are presented on the respective branches, and the dashed branches represent bootstrap support values less than 70%. The number of sequences and the taxonomic affiliations of the OPUs are indicated on the right.
Discussion
Diazotrophic Community Abundance and Relating Soil Properties
We investigated the abundance and composition of the diazotrophic community by quantifying and sequencing the nifH gene in bulk soil to compare the responses of free-living N-fixers to different reforestation approaches and to study their relation to soil N availability. Previous studies, focused on the 16S rRNA gene or phospholipid fatty acid (PLFA), implied significant shifts in the microbial community composition, and diversity in response to changes in vegetation during forest restoration (Banning et al., 2011; Song et al., 2015; Strickland et al., 2017). However, responses of the functional microorganisms involved in nutrient cycling have received little attention. The diazotrophic microorganisms are primary drivers of soil BNF that may be significantly correlated with diazotrophic community attributes, including abundance, composition, and activity (Bowers et al., 2008; Reed et al., 2010; Pogoreutz et al., 2017). In this study, both the abundance and composition of the diazotrophic community varied significantly among the reforestation approaches, which may indicate variation in the BNF rate. In the diazotrophic community, the gene nifH encodes a subunit of nitrogenase that is necessary for the catalysis of microbial N2-fixation. Thus, the nifH abundance might be related to BNF capacity (Newton, 2013). Overall, the average abundance of nifH in the reforestation approaches evaluated was higher than in other natural forest soils (Hayden et al., 2010; Mirza et al., 2014). This is possibly related to the limitation in soil N at the early stages of forest recovery (Kamijo et al., 2002; Zhan et al., 2009). In our study, soil inorganic N contents correlated with the nifH community composition but not with nifH abundance, suggesting that the composition of the diazotrophic community may be more critical than abundance in affecting soil N availability.
The positive correlation between nifH abundance and SOM indicated a covariation between diazotrophic abundance and the organic C content. A greater organic C content could support more diazotrophs, possibly because the BNF conducted by diazotrophs is energy consuming (Klucas, 1991). In addition to SOM, greater SWC may indirectly lead to a greater availability of organic C substrate to diazotrophs by enhancing the diffusive transport of organic soluble substrates and the mobility of microorganisms (Borken and Matzner, 2009; Ding et al., 2015). In line with this, in our study nifH abundance correlated positively with SWC. The greater nifH abundance in NP compared to the other reforestation approaches may have been related to the greatest SWC in the NP. Soils in the AM had the highest SOM and SWC and lowest pH. However, the nifH gene was not the most abundan in the AM, which may result from the effects of other soil properties, including phosphorus content (Orchard et al., 2009), soil texture and aggregate size (Poly et al., 2001).
Diazotrophic Community Composition and Its Correlation With Soil N Content
The SWC and NH4+ contributed most to the differences in soil environments among reforestation approaches, correlating significantly with the diazotrophic community composition and indicator species. The AM and NR had particularly higher NH4+ content compared to the other reforestation approaches, with correlations between the indicator species and the high content of NH4+. The indicator species compositions in the PM and ML were mainly associated with the low SWC. In addition, OPU2, OPU85, and OPU98 affiliated with Methanothrix, Methylophilus, and Methanobacterium, respectively, were common in NR. Although the coupling of methanogenesis and BNF processes remained unclear, the ability of methanogenic archaea to fix N2, and the interlinkage between C and N cycles have been well studied (Belay et al., 1984; Murray and Zinder, 1984). Our results suggest that archaeal biological N2 fixation was important and potentially associated with C cycling in NR. The AM had higher inorganic N (NH4+ and NO3−) and MBN than other reforestation approaches, suggesting that high soil inorganic N content could have driven microbial N immobilization in AM but not in NR. Possibly, the low soil organic C content in NR resulted in less microbial growth and microbial N immobilization. However, under ML, the diazotrophic community composition changed substantially, and the soil N content decreased markedly when compared to AM. The majority of indicator species in the PM were Rhizobiales. The dominance of Rhizobiales in PM may relate to the low quality of organic C resources owing to recalcitrant litter (Zhang et al., 2008; Kirschbaum, 2013; Wang et al., 2018). Planting some dominant native species during the initial forest restoration may be helpful in the functional and structural recovery of degraded forest ecosystems (Lu et al., 2017). In this study, the NP was dominated by versatile diazotrophic groups, such as Paraburkholderia, Candidatus Koribacter, and Desulfospira, which could help the plant resist diseases (Rahman et al., 2018), and tolerate soil acidification and infertility (Kai et al., 1997) during the early stages of restoration.
Conclusion
Our study demonstrated that the abundance and composition of the diazotrophic community varied among different reforestation approaches implemented in subtropical China. The variations of diazotrophic community were closely associated with soil pH, SWC, NH4+, and SOM. However, soil NH4+ was significantly correlated with the composition of diazotrophic community and not the abundance, suggesting that the composition may have a greater effect on soil N availability. This study suggested that determining the diazotrophic community composition during the early stages of reforestation can help us choose effective pioneer species for promoting soil N supply and to understand the mechanisms involved in microbial communities.
Author Contributions
JC and WS conceived the research. JC performed the experiments and analyzed the data. JC, WS, HX, YL, and TL wrote and edited the manuscript.
Funding
This study was conducted with financial support from the Central Public-interest Scientific Institution Basal Research Fund (CAFYBB2018SZ003) and National Natural Science Foundation of China (31425005 and 31670628).
Conflict of Interest Statement
The authors declare that the research was conducted in the absence of any commercial or financial relationships that could be construed as a potential conflict of interest.
Acknowledgments
We thank Mr. Shengxing Fu and Dr. Taotao Li for their help and assistance in sample collection. We also thank Afiya John Ph.D., an English native speaker, for her kind assistance in editing this manuscript.
Supplementary Material
The Supplementary Material for this article can be found online at: https://www.frontiersin.org/articles/10.3389/fmicb.2019.00508/full#supplementary-material
Footnotes
References
Albert, K. M. (2015). Role of revegetation in restoring fertility of degraded mined soils in Ghana: a review. Int. J. Biodiv. Conserv. 7, 57–80. doi: 10.5897/IJBC2014.0775
Anderson, M. J. (2001). A new method for a non-parametric multivariate analysis of variance. Austral Ecol. 26, 32–46.
Atucha, A., Merwin, I. A., Brown, M. G., Gardiazabal, F., Mena, F., Adriazola, C., et al. (2013). Soil erosion, runoff and nutrient losses in an avocado (Persea americana Mill) hillside orchard under different groundcover management systems. Plant Soil 368, 393–406. doi: 10.1007/s11104-012-1520-0
Banning, N. C., Gleeson, D. B., Grigg, A. H., Grant, C. D., Andersen, G. L., Brodie, E. L., et al. (2011). Soil microbial community successional patterns during forest ecosystem restoration. Appl. Environ. Microbiol. 77:6158. doi: 10.1128/AEM.00764-11
Belay, N., Sparling, R., and Daniels, L. (1984). Dinitrogen fixation by a thermophilic methanogenic bacterium. Nature 312, 286–288. doi: 10.1038/312286a0
Borken, W., and Matzner, E. (2009). Reappraisal of drying and wetting effects on C and N mineralization and fluxes in soils. Global Change Biol. 15, 808–824. doi: 10.1111/j.1365-2486.2008.01681.x
Bowers, T. H., Reid, N. M., and Lloyd-Jones, G. (2008). Composition of nifh in a wastewater treatment system reliant on N(2) fixation. Appl. Microbiol. Biotechnol. 79, 811–818. doi: 10.1007/s00253-008-1486-2
Burgmann, H., Meier, S., Bunge, M., Widmer, F., and Zeyer, J. (2005). Effects of model root exudates on structure and activity of a soil diazotroph community. Environ. Microbiol. 7, 1711–1724. doi: 10.1111/j.1462-2920.2005.00818.x
Caporaso, J. G., Kuczynski, J., Stombaugh, J., Bittinger, K., Bushman, F. D., Costello, E. K., et al. (2010). QIIME allows analysis of highthroughput community sequencing data. Nat. Methods 7, 335–336. doi: 10.1038/nmeth.f.303
Chen, D., Xing, W., Lan, Z., Saleem, M., Wu, Y., Hu, S., et al. (2018). Direct and indirect effects of nitrogen enrichment on soil organisms and carbon and nitrogen mineralization in a semi-arid grassland. Funct. Ecol. 33, 175–187. doi: 10.1111/1365-2435.13226
Cole, J. R., Wang, Q., Cardenas, E., Fish, J., Chai, B., Farris, R. J., et al. (2009). The ribosomal database project: improved alignments and new tools for rRNA analysis. Nucleic Acids Res. 37, D141–D145. doi: 10.1093/nar/gkn879
R Core Team (2013). R: A Language and Environment for Statistical Computing. Vienna: R Foundation for Statistical Computing.
Dijkstra, F. A., Pendall, E., Mosier, A. R., King, J. Y., Milchunas, D. G., and Morgan, J. A. (2008). Long-term enhancement of N availability and plant growth under elevated CO2 in a semi-arid grassland. Funct. Ecol. 22, 975–982. doi: 10.1111/j.1365-2435.2008.01398.x
Ding, K., Zhong, L., Xin, X. P., Xu, Z. H., Kang, X. M., Liu, W. J., et al. (2015). Effect of grazing on the abundance of functional genes associated with N cycling in three types of grassland in Inner Mongolia. J. Soils Sediments 15, 683–693. doi: 10.1007/s11368-014-1016-z
Dixon, R., and Kahn, D. (2004). Genetic regulation of biological nitrogen fixation. Nat. Rev. Microbiol. 2, 621–631. doi: 10.1038/nrmicro954
Dufrene, M., and Legendre, P. (1997). Species assemblages and indicator species: the need for a flexible asymmetrical approach. Ecol. Monogr. 67, 345–366. doi: 10.2307/2963459
Edgar, R. C. (2010). Search and clustering orders of magnitude faster than BLAST. Bioinformatics 26:2460. doi: 10.1093/bioinformatics/btq461
Franco, A. A., and Faria, S. M. D. (1997). The contribution of N2-fixing tree legumes to land reclamation and sustainability in the tropics. Soil Biol. Biochem. 29, 897–903. doi: 10.1016/S0038-0717(96)00229-5
Galloway, J. N., Townsend, A. R., Erisman, J. W., Bekunda, M., Cai, Z., Freney, J. R., et al. (2008). Transformation of the nitrogen cycle: recent trends. Questions, and potential solutions. Science 320, 889–892. doi: 10.1126/science.1136674
Guindon, S., Lethiec, F., Duroux, P., and Gascuel, O. (2005). PHYML Online-a web server for fast maximum likelihood-based phylogenetic inference. Nucleic Acids Res. 33, W557–W559. doi: 10.1093/nar/gki352
Gutschick, V. P. (1981). Evolved strategies in nitrogen acquisition in plants. Am. Nat. 118, 607–637. doi: 10.1086/283858
Hayden, H. L., Drake, J., Imhof, M., Oxley, A. P. A., Norng, S., and Mele, P. M. (2010). The abundance of nitrogen cycle genes amoA and nifH depends on land-uses and soil types in South-Eastern Australia. Soil Biol. Biochem. 42, 1774–1783. doi: 10.1016/j.soilbio.2010.06.015
Hsu, S. F., and Buckley, D. H. (2009). Evidence for the functional significance of diazotroph community structure in soil. ISME J. 3, 124–136. doi: 10.1038/ismej.2008.82
Kai, F., Liesack, W., and Tindall, B. J. (1997). Desulfospira joergensenii, gen. nov., sp. nov., a new Sulfate-reducing Bacterium Isolated from Marine Surface Sediment. Syst. Appl. Microbiol. 20, 201–208. doi: 10.1016/S0723-2020(97)80066-5
Kamijo, T., Kitayama, K., Sugawara, A., Urushimichi, S., and Sasai, K. (2002). Primary succession of the warm-temperate broad-leaved forest on a volcanic island, Miyake-jima, Japan. Folia Geobot. 37, 71–91. doi: 10.1007/BF02803192
Kaye, J. P., and Hart, S. C. (1997). Competition for nitrogen between plants and soil microorganisms. Trends Ecol. Evol. 12, 139–143. doi: 10.1016/S0169-5347(97)01001-X
Kirschbaum, M. U. F. (2013). Seasonal variations in the availability of labile substrate confound the temperature dependence of organic matter decomposition. Soil Biol. Biochem. 57, 568–576. doi: 10.1016/j.soilbio.2012.10.012
Klucas, R. V. (1991). “Associative nitrogen fixation in plants,” in Biology and Biochemistry of Nitrogen Fixation, eds M. J. Dilworth and A. R. Glenn (Amsterdam: Elsevier), 187–198.
Lema, K. A., Willis, B. L., and Bourne, D. G. (2012). Corals form specific associations with diazotrophic bacteria. Appl. Environ. Microbiol. 78, 3136–3144. doi: 10.1128/AEM.07800-11
Limmer, C., and Drake, H. L. (1996). Non-symbiotic N2-fixation in acidic and pH-neutral forest soils: aerobic and anaerobic differentials. Soil Biol. Biochem. 28, 177–183. doi: 10.1016/0038-0717(95)00118-2
Limmer, C., and Drake, H. L. (1998). Effects of carbon, nitrogen, and electron acceptor availability on anaerobic N2-fixation in a beech forest soil. Soil Biol. Biochem. 30, 153–158. doi: 10.1016/S0038-0717(97)00099-0
Liu, G. S., Jiang, N. H., Zhang, L. D., and Liu, Z. L. (1996). Soil Physical and Chemical Analysis and Description of Soil Profiles. Beijing: China Standards Press.
Lu, Y., Ranjitkar, S., Harrison, R. D., Xu, J., Ou, X., Ma, X., et al. (2017). Selection of native tree species for subtropical forest restoration in Southwest China. PLoS One 12:e0170418. doi: 10.1371/journal.pone.0170418
Mcnamara, S., Tinh, D. V., Erskine, P. D., Lamb, D., Yates, D., and Brown, S. (2006). Rehabilitating degraded forest land in central Vietnam with mixed native species plantings. Forest Ecol. Manag. 233, 358–365. doi: 10.1016/j.foreco.2006.05.033
Mirza, B. S., Potisap, C., Nüsslein, K., Bohannan, B. J. M., and Rodrigues, J. L. M. (2014). Response of free-living nitrogen-Fixing microorganisms to land use change in the Amazon Rainforest. Appl. Environ. Microbiol. 80:281. doi: 10.1128/AEM.02362-13
Murray, P. A., and Zinder, S. H. (1984). Nitrogen fixation by a methanogenic archaebacterium. Nature 312, 284–286. doi: 10.1038/312284a0
Newton, P. C. D. (2013). A reduced fraction of plant N derived from atmospheric N (%Ndfa) and reduced rhizobial nifh gene numbers indicate a lower capacity for nitrogen fixation in nodules of white clover exposed to long-term CO2 enrichment. Biogeosciences 10, 8269–8281. doi: 10.5194/bg-10-8269-2013
Oksanen, J., Guillaume, B. F., Kindt, R., Legendre, P., Minchin, P. R., O’Hara, R. B., et al. (2013). Vegan: Community Ecology Package. R Package Version 2.0–7.
Orchard, E. D., Webb, E. A., and Dyhrman, S. T. (2009). Molecular analysis of the phosphorus starvation response in Trichodesmium spp. Environ. Microbiol. 11, 2400–2411. doi: 10.1111/j.1462-2920.2009.01968.x
Penton, C. R., Yang, C., Wu, L., Wang, Q., Zhang, J., Liu, F., et al. (2016). NifH-harboring bacterial community composition across an Alaskan permafrost thaw gradient. Front. Microbiol. 7:1894. doi: 10.3389/fmicb.2016.01894
Pfaffl, M. W. (2004). “Quantification strategies in real-time PCR,” in A–Z of Quantitative PCR, ed. S. A. Bustin (LaJolla: International University Line), 89–113.
Pogoreutz, C., Rädecker, N., Cárdenas, A., Gärdes, A., and Voolstra, C. R. (2017). Nitrogen fixation aligns with nifh abundance and expression in two coral trophic functional groups. Front. Microbiol. 8:1187. doi: 10.3389/fmicb.2017.01187
Poly, F., Monrozier, L. J., and Bally, R. (2001). Improvement in the rflp procedure for studying the diversity of nifH genes in communities of nitrogen fixers in soil. Res. Microbiol. 152, 95–103. doi: 10.1016/S0923-2508(00)01172-4
Rahman, M., Sabir, A. A., Mukta, J. A., Khan, M., Mohiuddin, M., Miah, M. G., et al. (2018). Plant probiotic bacteria Bacillus and Paraburkholderia improve growth, yield and content of antioxidants in strawberry fruit. Sci. Rep. 8:2504. doi: 10.1038/s41598-018-20235-1
Reed, S. C., Townsend, A. R., and Nemergut, C. D. R. (2010). Microbial community shifts influence patterns in tropical forest nitrogen fixation. Oecologia 164, 521–531. doi: 10.1007/s00442-010-1649-6
Roberts, D. (2013). Labdsv: Ordination and Multivariate Analysis for Ecology. R Package Version 1.6-1. Vienna: R Foundation for Statistical Computing.
Rösch, C., Mergel, A., and Bothe, H. (2002). Biodiversity of denitrifying and dinitrogen-fixing bacteria in an acid forest soil. Appl. Environ. Microbiol. 68, 3818–3829. doi: 10.1128/AEM.68.8.3818-3829.2002
Saleem, M., Law, A. D., Sahib, M. R., Pervaiz, Z. H., and Zhang, Q. (2018). Impact of root system architecture on rhizosphere and root microbiome. Rhizosphere 6, 47–51. doi: 10.1016/j.rhisph.2018.02.003
Schermelleh-Engel, K., Moosbrugger, H., and Müller, H. (2003). Evaluating the fit of structural equation models: tests of significance and descriptive goodness-of-fit measures. MPR Online 8, 23–74.
Silva, J. M. N., Carreiras, J. M. B., Rosa, I., and Pereira, J. M. C. (2011). Greenhouse gas emissions from shifting cultivation in the tropics, including uncertainty and sensitivity analysis. J. Geophys. Res. Atmos. 116:D20304. doi: 10.1029/2011JD016056
Song, P., Ren, H., Jia, Q., Guo, J., Zhang, N., and Ma, K. (2015). Effects of historical logging on soil microbial communities in a subtropical forest in southern China. Plant Soil 397, 115–126. doi: 10.1007/s11104-015-2553-y
Strickland, M. S. Jr., Callaham, M. A., Gardiner, E. S., Stanturf, J. A., Leff, J. W., Fierer, N., et al. (2017). Response of soil microbial community composition and function to a bottomland forest restoration intensity gradient. Appl. Soil Ecol. 119, 317–326. doi: 10.1016/j.apsoil.2017.07.008
Vance, E. D., Brookes, P. C., and Jenkinson, D. S. (1987). An extraction method for measuring soil microbial biomass-C. Soil Biol. Biochem. 19, 703–707. doi: 10.1016/0038-0717(87)90052-6
Wang, F. M., Li, Z. A., Xia, H. P., Bi, Z., Li, N. Y., Jin, L., et al. (2010). Effects of nitrogen-fixing and non-nitrogen-fixing tree species on soil properties and nitrogen transformation during forest restoration in southern China. Soil Sci. Plant Nutr. 56, 297–306. doi: 10.1111/j.1747-0765.2010.00454.x
Wang, H., Liu, S., Wang, J., You, Y., Yang, Y., Shi, Z., et al. (2018). Mixed-species plantation with Pinus massoniana and Castanopsis hystrix accelerates C loss in recalcitrant coniferous litter but slows C loss in labile broadleaf litter in southern China. Forest Ecol. Manag. 422, 207–213. doi: 10.1016/j.foreco.2018.04.024
Wang, Y., Ouyang, Z. Y., Zheng, H., Zeng, J., Chen, F. L., and Zhang, K. (2013). Effects of different forest restoration approaches on the soil quality in red soil region of Southern China. Chin. J. Appl. Ecol. 24:1335.
Yuan, J., Ouyang, Z., Zheng, H., and Xu, W. (2012). Effects of different grassland restoration approaches on soil properties in the southeastern Horqin sandy land, northern China. Appl. Soil Ecol. 61, 34–39. doi: 10.1016/j.apsoil.2012.04.003
Zhan, S. X., Chen, F. S., Xiao-Fei, H. U., and Lu, G. (2009). Soil nitrogen and phosphorus availability in forest ecosystems at different stages of succession in the central subtropical region. Acta Ecol. Sin. 29,4673–4680.
Zhang, P., Tiana, X., Song, F., Ren, L., and Jiang, P. (2008). Effect of litter quality on its decomposition in broadleaf and coniferous forest. Eur. J. Soil Biol. 44, 392–399. doi: 10.1016/j.ejsobi.2008.09.001
Keywords: nitrogen-fixation, forest restoration, subtropical forest, legume, nifH gene
Citation: Chen J, Shen W, Xu H, Li Y and Luo T (2019) The Composition of Nitrogen-Fixing Microorganisms Correlates With Soil Nitrogen Content During Reforestation: A Comparison Between Legume and Non-legume Plantations. Front. Microbiol. 10:508. doi: 10.3389/fmicb.2019.00508
Received: 14 September 2018; Accepted: 27 February 2019;
Published: 14 March 2019.
Edited by:
Hongchen Jiang, China University of Geosciences Wuhan, ChinaReviewed by:
Petri Penttinen, Zhejiang Agriculture and Forestry University, ChinaMuhammad Saleem, Alabama State University, United States
Copyright © 2019 Chen, Shen, Xu, Li and Luo. This is an open-access article distributed under the terms of the Creative Commons Attribution License (CC BY). The use, distribution or reproduction in other forums is permitted, provided the original author(s) and the copyright owner(s) are credited and that the original publication in this journal is cited, in accordance with accepted academic practice. No use, distribution or reproduction is permitted which does not comply with these terms.
*Correspondence: Jie Chen, Y2hlbmppZWNhZkBob3RtYWlsLmNvbQ==