- 1CNRS, Inserm, CHU Lille, U1019 – UMR 8204 – CIIL – Centre d’Infection et d’Immunité de Lille, Institut Pasteur de Lille, Université de Lille, Lille, France
- 2Délégation à la Recherche Clinique et à l’innovation, Groupement des Hôpitaux de l’Institut Catholique de Lille, Lille, France
- 3ISA-YNCREA Hauts-de-France, Lille, France
- 4CNRS, ISEN, UMR 8520 – IEMN, Université de Lille, Lille, France
- 5Laboratoire Ecologie et Biodiversité, Faculté de Gestion Economie et Sciences, Institut Catholique de Lille, Lille, France
- 6Service d’Anatomie et de Cytologie Pathologiques, Groupement des Hôpitaux de l’Institut Catholique de Lille, Lille, France
- 7Gènes Diffusion, Douai, France
- 8PEGASE-Biosciences, Institut Pasteur de Lille, Lille, France
- 9Ifremer, Laboratoire Ecosystèmes Microbiens et Molécules Marines pour les Biotechnologies, Nantes, France
- 10Centre for Sustainable Aquatic Ecosystems, College of Science, Health, Engineering and Education, Murdoch University, Perth, WA, Australia
- 11Laboratory for Food Safety, French Agency for Food, Environmental and Occupational Health and Safety (ANSES), Boulogne-sur-mer, France
Cryptosporidium, a zoonotic pathogen, is able to infect a wide range of hosts including wild and domestic animals, and humans. Although it is well known that some parasites are both fish pathogens and recognized agents of zoonosis with a public health impact, little information is available concerning the prevalence of Cryptosporidium in wild aquatic environments. To evaluate the prevalence of Cryptosporidium spp. in commercially important edible marine fish in different European seas (English channel, North sea, Bay of Biscay, Celtic sea and Mediterranean sea), 1,853 specimens were collected as part of two surveys. Nested PCR followed by sequence analysis at the 18S rRNA gene locus was used to identify Cryptosporidium spp. The overall prevalence of Cryptosporidium spp. in sampled fish reached 2.3% (35 out of 1,508) in a first campaign and 3.2% (11 out of 345) in a second campaign. Sequence and phylogenetic analysis of positive samples identified Cryptosporidium parvum (n = 10) and seven genotypes which exhibited between 7.3 and 10.1% genetic distance from C. molnari, with the exception of one genotype which exhibited only 0.5–0.7% genetic distance from C. molnari. Among 31 analyzed fish species, 11 (35.5%) were identified as potential hosts for Cryptosporidium. A higher prevalence of Cryptosporidium spp. was observed in larger fish, in fish collected during the spring-summer period, and in those caught in the North East Atlantic. Pollachius virens (saithe) was the most frequently Cryptosporidium positive species. In fish infected by other parasites, the risk of being Cryptosporidium positive increased 10-fold (OR: 9.95, CI: 2.32–40.01.04, P = 0.0002). Four gp60 subtypes were detected among the C. parvum positive samples: IIaA13G1R1, IIaA15G2R1, IIaA17G2R1, and IIaA18G3R1. These C. parvum subtypes have been previously detected in terrestrial mammals and may constitute an additional source of infection for other animals and in particular for humans. Microscopical examination of histological sections confirmed the presence of round bodies suggestive of the development of C. parvum within digestive glands. We report herein the first epidemiological and molecular data concerning the detection of Cryptosporidium in edible marine fish in European seas surrounding France broadening its host range and uncovering potential novel infection routes.
Introduction
The world fish production increased to 171 million tons in 2016 (compared for instance to a production of 156 million tons in 2012) with European fisheries and aquaculture representing overall 6.4 million tons. Interestingly, within the European Union, around 80% of fish consumed is wild caught with an annual fish consumption of 18.1 kg per capita (FAO, 2018).
Considering that protozoan and metazoan parasites can infest edible fish worldwide, the problem of human health risks due to wild fish ingestion is an important issue. Some of these parasites are both fish pathogens and recognized zoonotic pathogens with public health impacts but uncertainty exists about the zoonotic potential of some fish pathogens. The most important fish species in the fish industries from many countries can be infected with parasites. Furthermore, some fish parasites can affect the appearance, touch, odor, texture, temperature and taste (organoleptic properties) of fish products, negatively impacting the fish industry economy (Agence Française de Sécurité Sanitaire des Aliments [ANSES], 2010).
Even in developed countries, where sanitary infrastructures are usually good, food- or water-borne parasitic infections are frequent (Omarova et al., 2018). Indeed, the persistence of water- or foodborne outbreaks and the occurrence of infections due to emerging or re-emerging pathogens are favored by different factors related to changes in consumer life styles such as: increased consumption of fresh products, exotic food or raw or lightly cooked meat and increasing global demand for protein of animal origin, and home-meal replacement (Collins, 1997; Broglia and Kapel, 2011).
Cryptosporidium is a waterborne and foodborne protozoan parasite responsible for more than 8 million cases of foodborne illness annually (Ryan et al., 2018). The parasite is a cause of severe diarrhea mainly in immunocompromised people and young children but also in a wide range of vertebrates including fish, amphibians, reptiles, birds, and mammals (Ryan et al., 2014). To date, it has been genetically characterized in more than 25 species of both marine and freshwater fish. Three species of Cryptosporidium are recognized in fish: Cryptosporidium molnari (Alvarez-Pellitero and Sitjà-Bobadilla, 2002; Palenzuela et al., 2010), C. scophthalmi (Alvarez-Pellitero et al., 2004) and C. huwi (previously known as piscine genotype I) (Ryan et al., 2015). Additionally, other Cryptosporidium species identified in other groups of vertebrates such as C. parvum, C. hominis, C. scrofarum and C. xiaoi, have also been detected in fish. Furthermore, fifteen Cryptosporidium fish genotypes, and one Cryptosporidium rat III-like genotype, have been reported (Ryan et al., 2014; Yang et al., 2015, 2016; Couso-Pérez et al., 2018).
In fish hosts, Cryptosporidium is found either in the stomach or intestine. Additionally, it has been described that the parasite can cause pathological effects in fish (Alvarez-Pellitero and Sitjà-Bobadilla, 2002; Alvarez-Pellitero et al., 2004) as well as an increase in the mortality rate, mainly in juvenile fish (Murphy et al., 2009).
The majority of studies on piscine Cryptosporidium have been carried out on aquarium or farmed fish but scarce data is available concerning the molecular identification of Cryptosporidium genotypes and species in wild marine fish and, in particular, in edible fish. Actually, only two studies have been carried on in Australia and Papua New Guinea on wild marine fish (Reid et al., 2010; Koinari et al., 2013). To our knowledge, no updated information on Cryptosporidium infection in marine fish is available in France. In a previous study from our group, the overall prevalence of Cryptosporidium spp. in freshwater fish sampled from Lake Geneva (France) reached 37% (Certad et al., 2015).
Thus, the main goal of our study was to evaluate the prevalence of Cryptosporidium species and genotypes in selected edible fish species sampled in defined marine geographic areas surrounding France (European fishing waters). Considering that many factors related to the host and to the environment can influence host-parasites relationships (Sitjà-Bobadilla et al., 2005), we also aimed to determine the influence of host and environmental factors on Cryptosporidium prevalence in fish.
Materials and Methods
Fish Sampling
Epidemiological studies were conducted during two surveys: For the first survey, commercially important fish species were collected between 2011 and 2014 through research cruises belonging to the French Ifremer (Institut Français de Recherche pour l’Exploitation de la Mer) in different European seas as follows: the English channel, the North sea, the Bay of Biscay, the Celtic sea and the Mediterranean sea, or through purchases from wholesalers or retailers for commercial catches, and for farmed fish in the case of salmon and sea bass. Fish retailers were chosen by their representativeness of fishing areas in order to complement marine fish species according to range and origin. For the second survey, fish sampling was performed at Boulogne-Sur-Mer, the first France’s fishermen’s port, between 2014 and 2015 targeting the four most frequently caught fish species at this port: saithe, mackerel, herring, and whiting. Sampling surveys allowed catching fresh ungutted fish.
Available data about fishing area, fishing vessel, fishing date and kind of storage were recorded. Fish specimens of the selected species were sampled at various times and areas on the basis of fishery season. Fishes were analyzed immediately after been caught in the case of specimens collected through research cruises or after fish landing when obtained from retailers. When fishes were obtained from retailers, it was not always possible to pinpoint the exact location of the fishing area within the North East Atlantic. The weight, size, sex, and sexual maturity were determined for each fish. The presence of ecto and endoparasites other than Cryptosporidium was detected by visual examination of the body of the fish before and after evisceration. In order to classify fish according to weight and size, five groups were defined ranging from 1-smallest fishes to 5-largest fishes (Supplementary Figure S1) using a hierarchical cluster analysis with the R stats package. Fish were dissected, scrapings of the gastrointestinal epithelia were performed for each animal, the cells were preserved in RCL2® (Alphelys, Plaisir, France) and stored at -20°C as previously described (Certad et al., 2015). Sections of the stomach and bowel were fixed in 10% buffered formalin for histological analysis. Ifremer research cruises are carried out with the French Oceanographic Fleet and are under the supervision of the French Ministry of Education and Research. A Steering Committee evaluates and approves the entire scientific campaign program before implementation. The study was performed in accordance with the EU directive 2010/63/EU and followed all the guidelines of the deontology charter of Ifremer’s research.
DNA Extraction
Genomic DNA extraction was performed from scrapings of the gastric and intestinal epithelia on 96-well plates, using the NucleoSpinTM Kit (Macherey-Nagel, GmbH & Co KG, Germany) according to the manufacturer as previously described (Certad et al., 2015). DNA was eluted in 100 μl of elution buffer.
Nested PCR
Fish samples were tested for identification of Cryptosporidium at the 18S rRNA gene locus as previously described (Certad et al., 2015). Nested PCR was performed using a MJ Research PTC-200 Thermal Cycler (Marshall Scientific, Waltham, MA, United States). Secondary PCR products were visualized on a 2% agarose gel stained with Ethidium Bromide fluoresce under ultraviolet light. Although nested PCR may not accurately represent the genetic diversity originally present in the sample, this technique is often appropriate to obtain sufficient DNA copies, considering that environmental samples, and in particular gastric or intestinal tissues from fishes or feces from wildlife, frequently contain low amounts of oocysts and high levels of PCR inhibitors (Paparini et al., 2017).
DNA Sequencing and Analysis
To identify Cryptosporidium species or genotypes, positive secondary nested PCR products were purified using the NucleoFast® 96 PCR kit (Macherey Nagel, GmbH & Co KG, Germany). Purified PCR products were sequenced directly in both directions, using the secondary PCR primers (Genoscreen, Pasteur Institute of Lille, France). Obtained nucleotide sequences were aligned using the BioEdit v7.0.1 package, and compared with available DNA sequences of Cryptosporidium in GenBank data base using the NCBI BLAST basic local alignment search tool1. Subtyping of C. parvum was based on sequence analysis of the 60 kDa glycoprotein (gp60) gene as previously reported (Gatei et al., 2007). The amplified DNA fragments were purified, sequenced, and analyzed as described above. All of the nucleotide sequences identified in this study were deposited in GenBank under the accession numbers MK236538-MK236548.
Phylogenetic Analysis
The 18S rRNA nucleotide sequences from the present study were aligned with Cryptosporidium sequences retrieved from GenBank (Benson et al., 2004). Sequences were aligned with MUSCLE (Elwakil and Hewedi, 2010) and the most suitable nucleotide substitutions model was selected using jModelTest2 (Darriba et al., 2012). Maximum Likelihood (ML) and Distance trees were constructed using MEGA version 7 using the Kimura 2-parameter model and gaps/missing data treatment set to complete deletion (Kumar et al., 2016). Bootstrap support was based on 1000 replications. Bayesian phylogenetic reconstructions were produced from alignments using MrBayes (Ronquist et al., 2012), with the HK85 substitution model, a MCMC length of 1,100,000, burn-in of 10,000, and subsampling every 200 iterations.
Histological Analysis
Paraffin-embedded tissues were cut to a thickness of 5 μm and stained with hematoxylin and eosin (H & E). A Leica DMRB microscope equipped with a Leica digital camera connected to an Imaging Research MCID analysis system (MCID Software, Cambridge, United Kingdom) was used for observation of the histological sections.
Statistical Analysis
Fisher’s exact test was used to analyze the relationship between different categorical variables. A logistic regression model was used to calculate odds ratios (OR) with Cryptosporidium presence as the main outcome. Multiple correspondence analysis (MCA), a data reduction technique similar to factor or principal component analysis, was applied to identify potential risk factors for Cryptosporidium infection in fishes. This descriptive statistical technique is valuable to analyze data and confirm associations or similarities between quantitative or qualitative variables; then, these variables are categorized without a probabilistic distribution defined a priori (Greenacre and Blasius, 2006). MCA facilitates the examination of different variables simultaneously; the results are represented by a graphic, and a point represents each category of every variable, and the distance from one point to another and from the center represents the relationship among the categorical variables. Risk factor variables included in the analysis were: localization of the fishing area, campaigns, seasonality, taxonomical position (order) of fish, size and weight grouping. The general significance level was set at a P-value below 0.05. All analyses were performed using Vassarstats software and packages stats from the R statistical computing program.
Results
In total, 1,508 fishes were collected in the first survey: 765 onboard Ifremer research vessels and 743 from fish retailers, representing 31 different fish species. The molecular analysis of fish digestive tissues allowed the identification of Cryptosporidium spp. in 35 out of 1,508 fish, representing a prevalence of 2.3% (Table 1). Table 1 shows the distribution of positive Cryptosporidium samples according to different surveys. The fish sex had no statistically significant effect on the presence of the parasite (P = 0.88). Concerning the distribution of Cryptosporidium according to fish areas, 28 (81%) positive cases were found in specimens sampled inland from wholesalers or retailers compared to 7 (19%) in specimens sampled during research campaigns. This difference in distribution was statistically significant, with the risk of Cryptosporidium detection four times higher in the first group (OR: 4, CI: 1.64–8.69, P = 0.0001) (Table 2). No specimens from aquaculture were found positive for the parasite. Concerning seasonality, 29 (83%) Cryptosporidium positive cases were found in fish collected during the spring-summer period, compared to 6 (17%) during the fall-winter period, with the risk of Cryptosporidium presence 4-fold higher in the first group. This difference in distribution was statistically significant (OR: 4.14, CI: 1.71–10.04, P = 0.0007) (Table 2). Overall, 952 (63%) fishes out of 1,508 were infected by different parasites. The most frequently detected parasites were nematodes of the Anisakidae family in 56% of fishes. Other parasites were also found such as trematodes, cestodes, microsporidia, and copepods. In fish infected with other parasites, the risk of simultaneous presence of Cryptosporidium increased 10-fold (OR: 9.95, CI: 2.32–40.01.04, P = 0.0002) (Table 2).
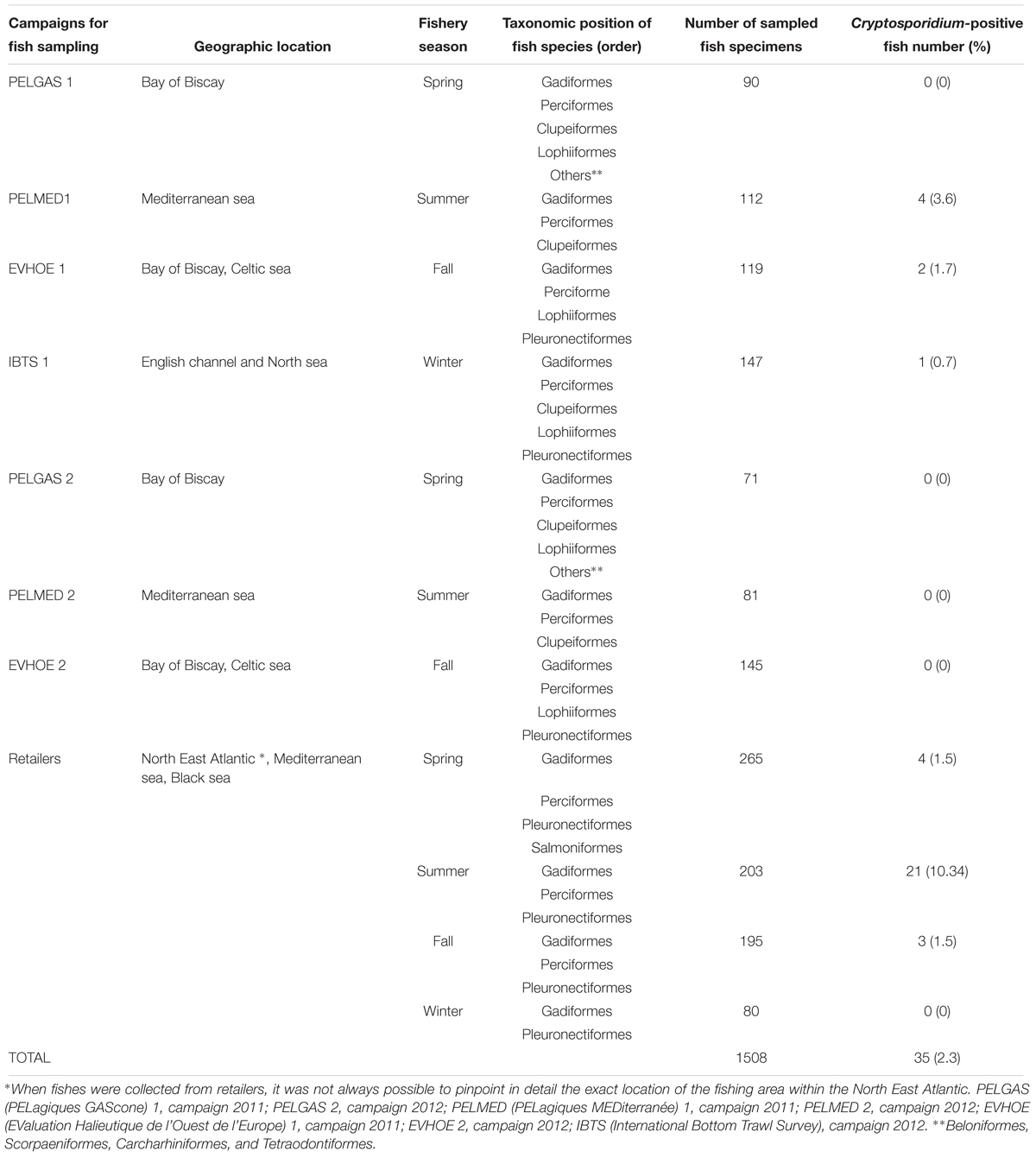
Table 1. Description of campaigns for fish sampling: geographic location, seasonality, taxonomic position of fish species, and prevalence of Cryptosporidium (First survey).
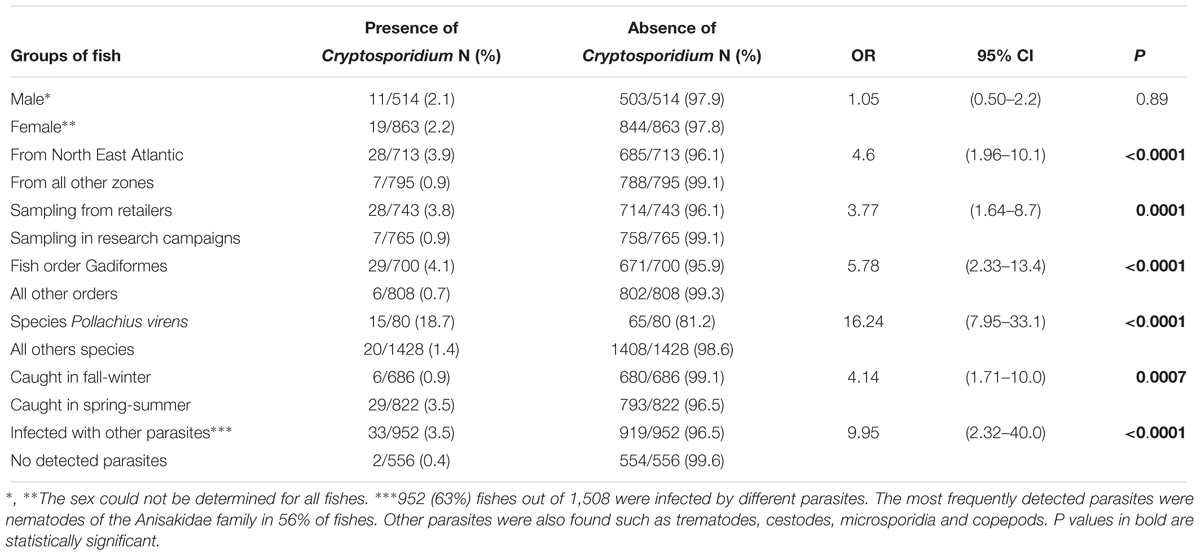
Table 2. Prevalence of Cryptosporidium in fishes sampled during the national survey according to host and environmental factors.
Overall, out of 31 analyzed fish species, 11 (35.5%) were recognized as potential new hosts for Cryptosporidium spp. with Pollachius virens, Scomber japonicus and Molva dypterygia the species with the highest prevalence (Table 3). In particular, the prevalence in Pollachius virens reached 19% and the risk of detection of Cryptosporidium in this host, increased 16-fold when compared with the other fish species (OR: 16.24, CI: 7.95–33.18, P < 0.0001) (Table 2).
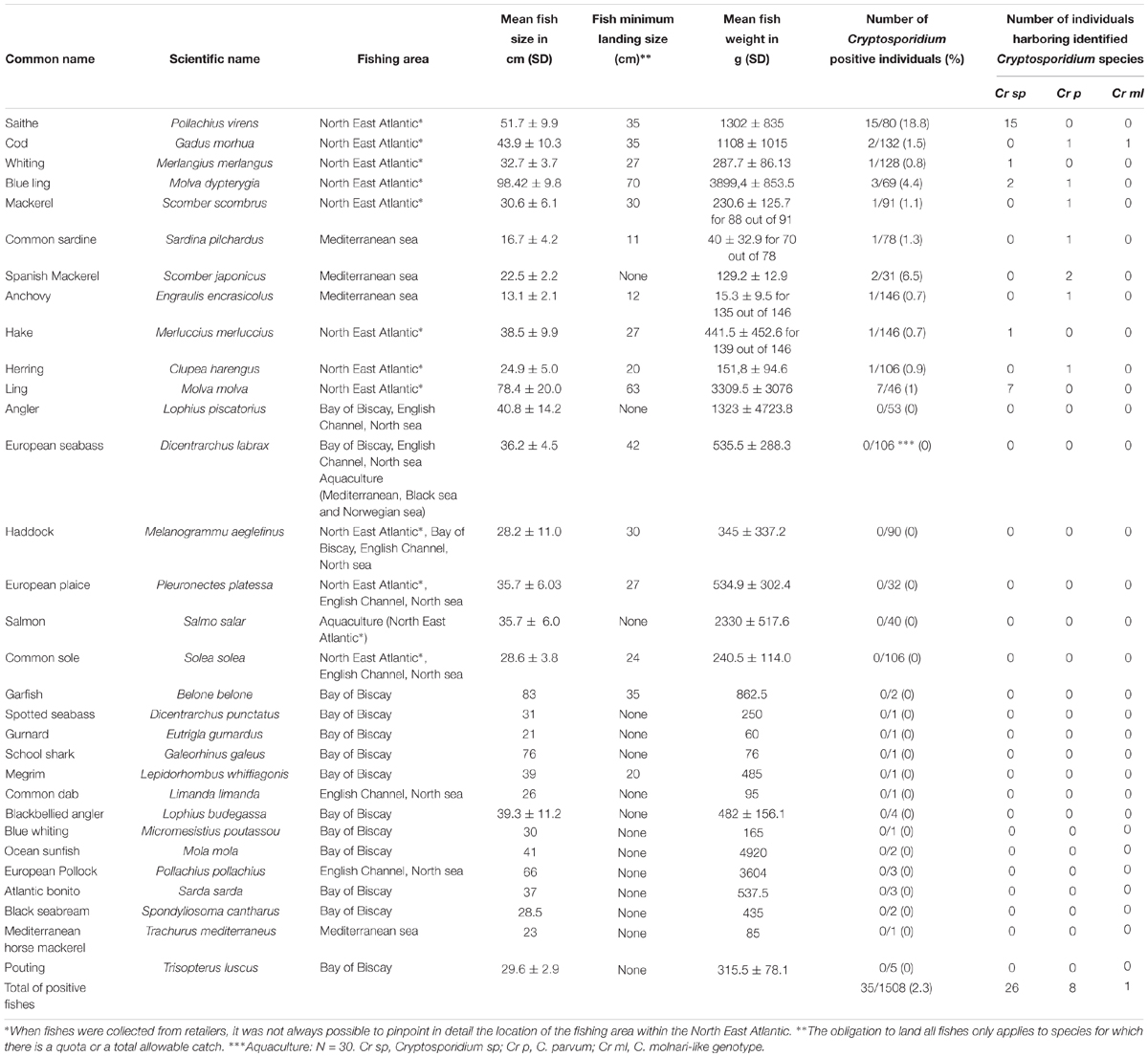
Table 3. Cryptosporidium distribution in wild marine fishes identified at the 18S rRNA gene locus (national survey).
A higher prevalence of Cryptosporidium was recorded in larger fishes according to weight and size grouping, with a tendency to decrease in smaller fishes.
The MCA (Figure 1) shows the coordinates of each variable on the two dimensions which explains the largest percentage of the variance in the data. Variables which are closest to each other on the scatterplot and far from the center of the plot are the most closely related. Even if these variables are not grouped as perfect clusters, most Cryptosporidium positive fishes are in the zone called cluster 1. In general, this cluster contained larger fishes mainly of size and weight groupings 4 and 5, from the Gadiformes order, caught in the North East Atlantic.
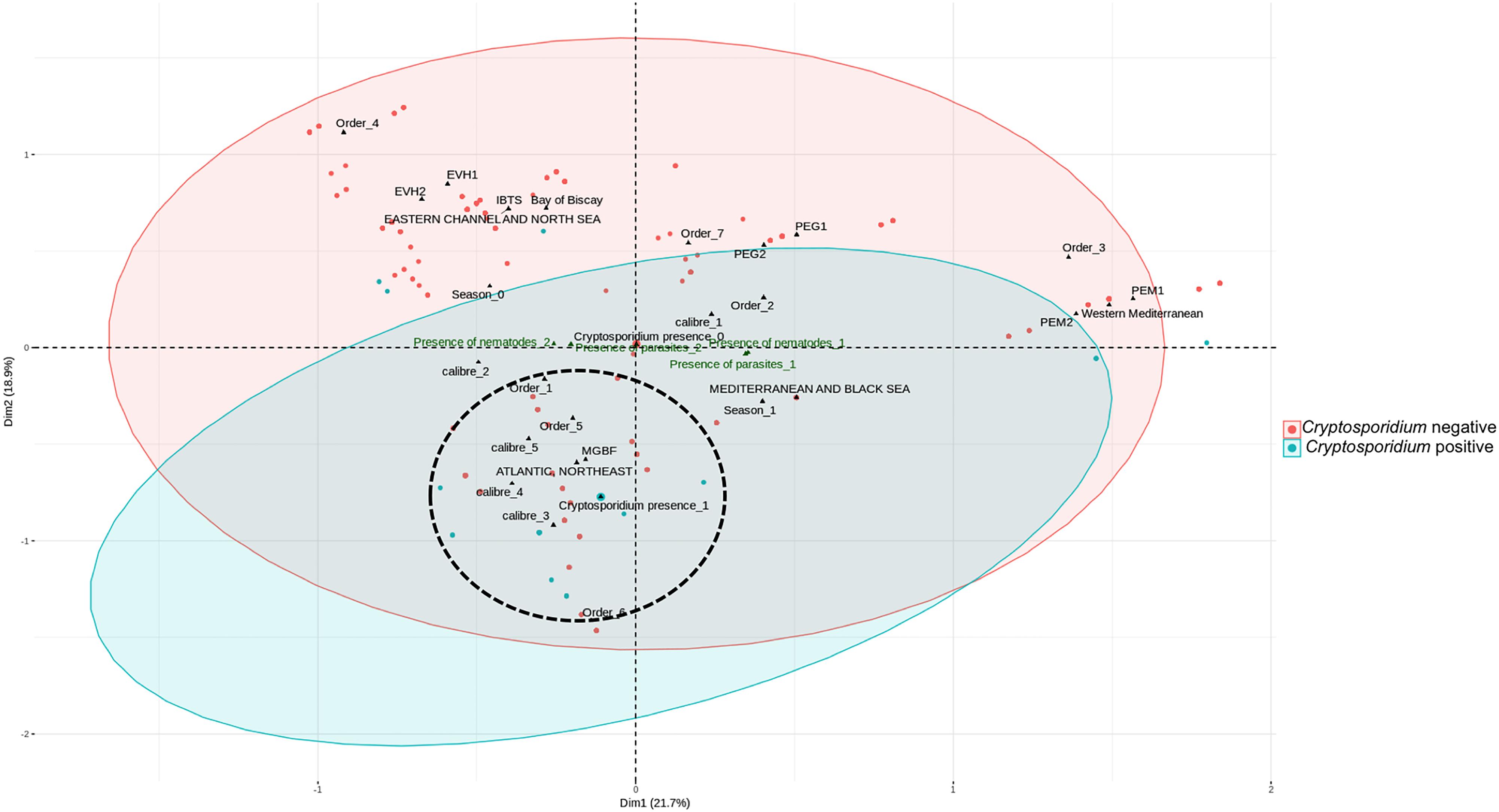
Figure 1. Multiple correspondence analysis. The cluster analysis explained 41% of the total variation. Variables which are closest to each other and distant from the center on the scatterplot are the most likely related. Even if these variables are not grouped as perfect clusters, most Cryptosporidium positive fishes are in the zone encircled (dot lines) that we called cluster 1. In general, this cluster contained larger fishes mainly of size and weight groupings (calibers) 4 and 5, from the order Gadiformes, caught in the North East Atlantic. PELGAS (PELagiques GAScone) 1, campaign 2011; PELGAS 2, campaign 2012; PELMED (PELagiques MEDiterranée) 1, campaign 2011; PELMED 2, campaign 2012; EVHOE (EValuation Halieutique de l’Ouest de l’Europe) 1, campaign 2011; EVHOE 2, campaign 2012; IBTS (International Bottom Trawl Survey), campaign 2012; MGBF, Retailers. Season 0, fall/winter; Season 1, spring/summer. Order 1, Gadiformes; Order 2, Perciformes; Order 3, Clupeiformes; Order 4, Lophiiformes; Order 5, Pleuronectiformes; Order 6, Salmoniformes; Order 7, others. Fishes were classified according to weight and size and five groups were defined ranging from 1-smallest fishes to 5-largest fishes (Supplementary Figure S1) using a hierarchical cluster analysis with the R stats package.
Sequence and phylogenetic analysis (Figure 2) at the 18S rRNA gene locus identified one species of Cryptosporidium and seven genotypes, distributed as follows: 8 (22%) C. parvum, 16 (45.7%) belonged to a novel genotype (#Cryptofish1) that exhibited 7.3–8.5% genetic distance from C. molnari (HM243547- HM243550), 6 (17.1%) belonged to another genotype (#Cryptofish 2) that exhibited 8.5–9.5% genetic distance from C. molnari, 2 (5.7%) belonged to a new genotype (#Cryptofish 3) which exhibited 8.9–10.1% genetic distance from C. molnari, single isolates (#Cryptofish 4 and # Cryptofish 5) (5.7%) which exhibited 8.2–9.5% and 7.6–8.8% genetic distance from C. molnari, respectively, and one (2.9%) (#Cryptofish 6) which exhibited 0–0.5% genetic distance from C. molnari-like isolates (HQ585890 and KR610356) and 0.5–0.7% genetic distance from C. molnari. Estimates of evolutionary divergence between sequences are shown in Supplementary Figure S2.
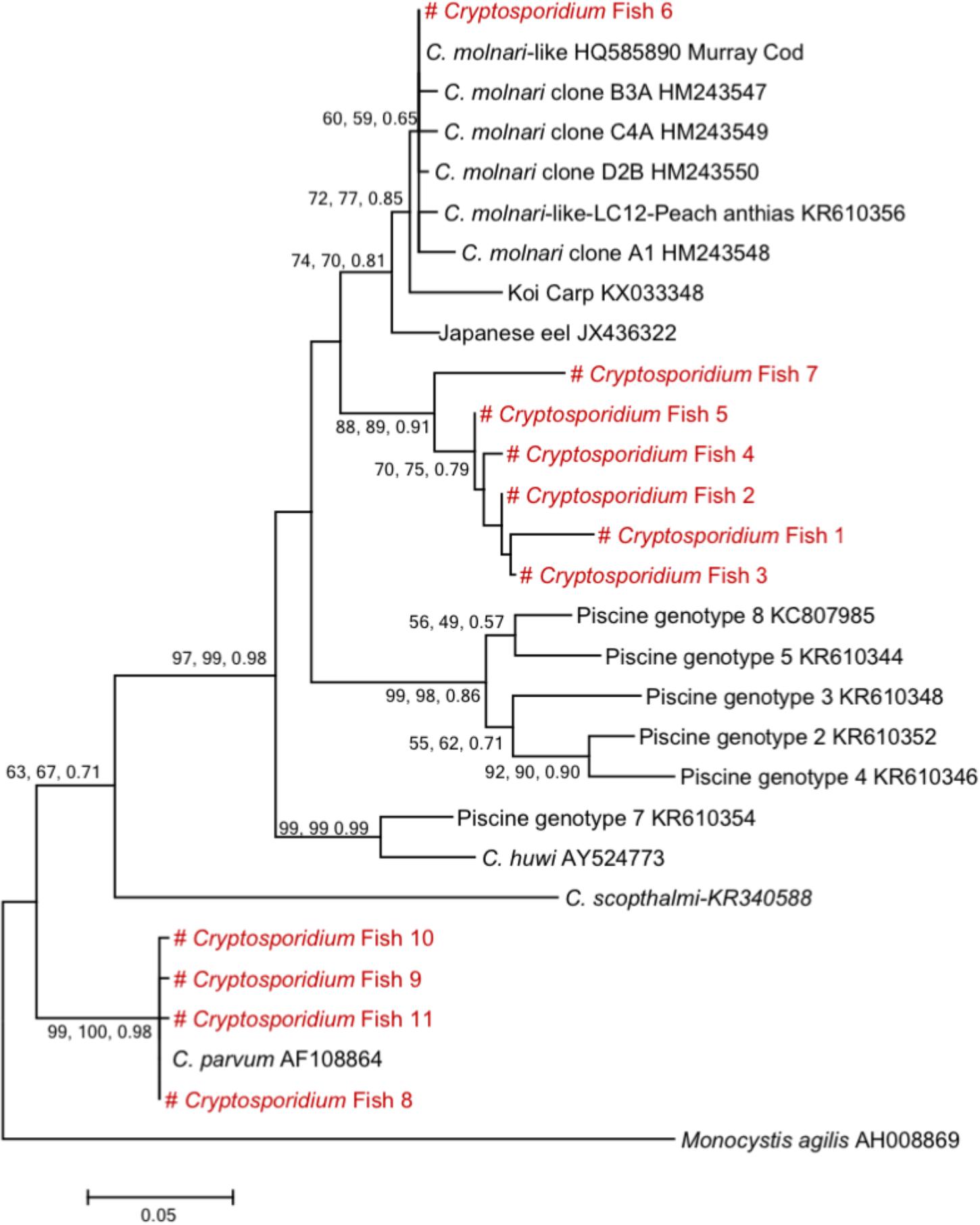
Figure 2. Phylogenetic tree showing the evolutional relationships of Cryptosporidium piscine isolates inferred by ML analysis of 18S rRNA gene sequences. Percentage support (>50%) from 1,000 pseudoreplicates from ML and distance analyses and posterior probabilities from Bayesian analysis are indicated at the left of the supported node. Red texts correspond to the sequences from this study.
The distribution of Cryptosporidium species and genotypes according to the species of fish identified as hosts is shown in Figure 3. Molecular analysis of DNA extracted from each fish stomach or intestine, followed by nested 18S rRNA PCR and sequencing allowed the identification of C. parvum in the intestine of 7 fish and in the stomach of one fish, while the C. molnari-like genotype (#Cryptofish 6) was found in the stomach of one fish. The various novel Cryptosporidium genotypes were detected in the stomach of 20 (76.9%) fish, in the intestine of one (3.8%) fish and in both the intestine and stomach of 5 (19.2%) fish. For the latter case, the same genotypes were found in both organs for each fish. In Figure 4A, the distribution of species/genotypes according to anatomical location is shown. Four gp60 subtypes were identified among the C. parvum positive samples: IIaA13G1R1, IIaA15G2R1, IIaA17G2R1, and IIaA18G3R1.
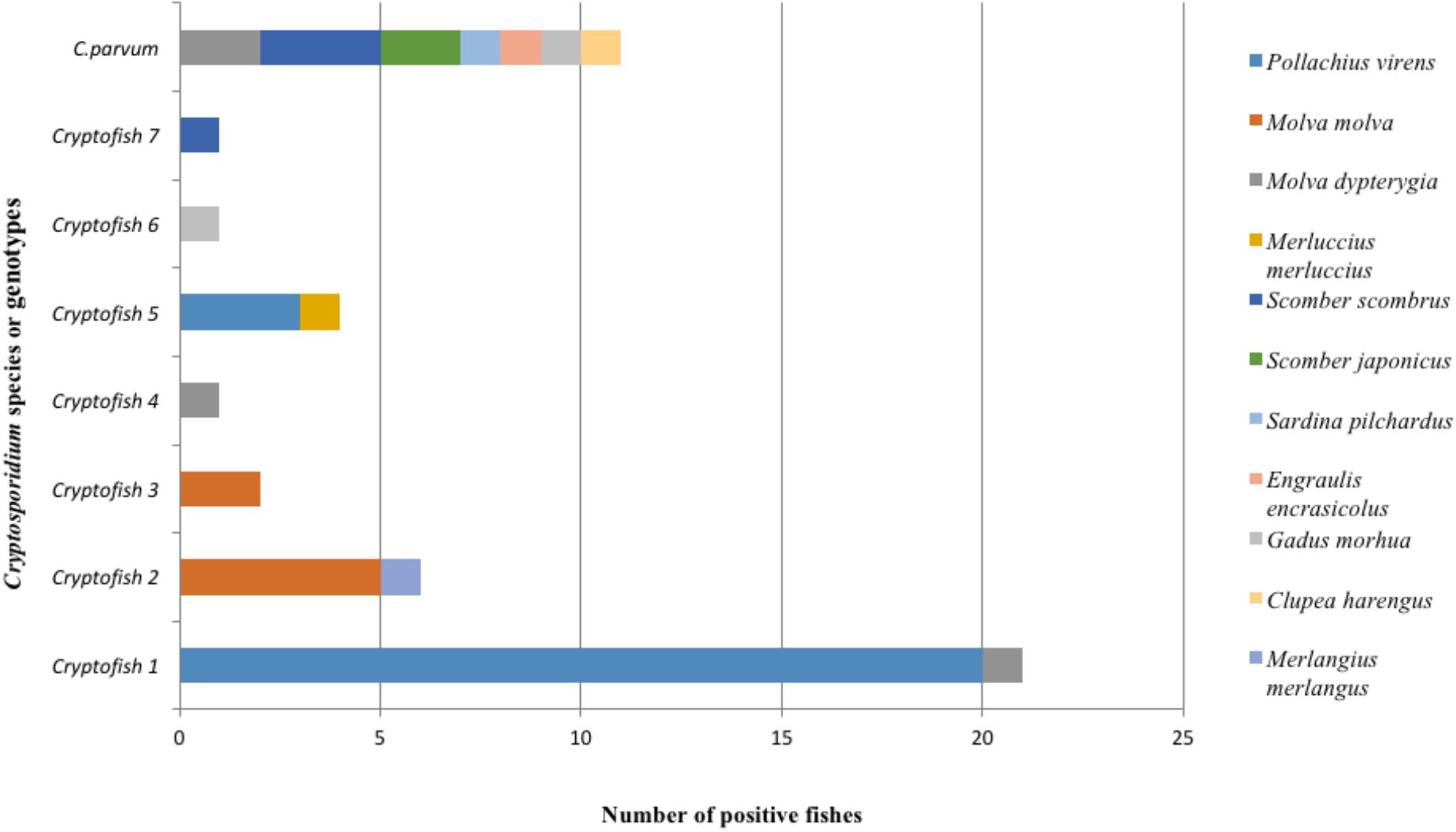
Figure 3. Global distribution of different types of Cryptosporidium sequences identified at the 18S rRNA gene locus according to fish species found as hosts in both surveys (n = 46). Eleven new species of fish were identified as potential hosts for Cryptosporidium. Cryptofish 1 was the most frequently identified novel genotype. Cryptosporidium genotypes had less host diversity when compared to C. parvum which was found in seven different fish species.
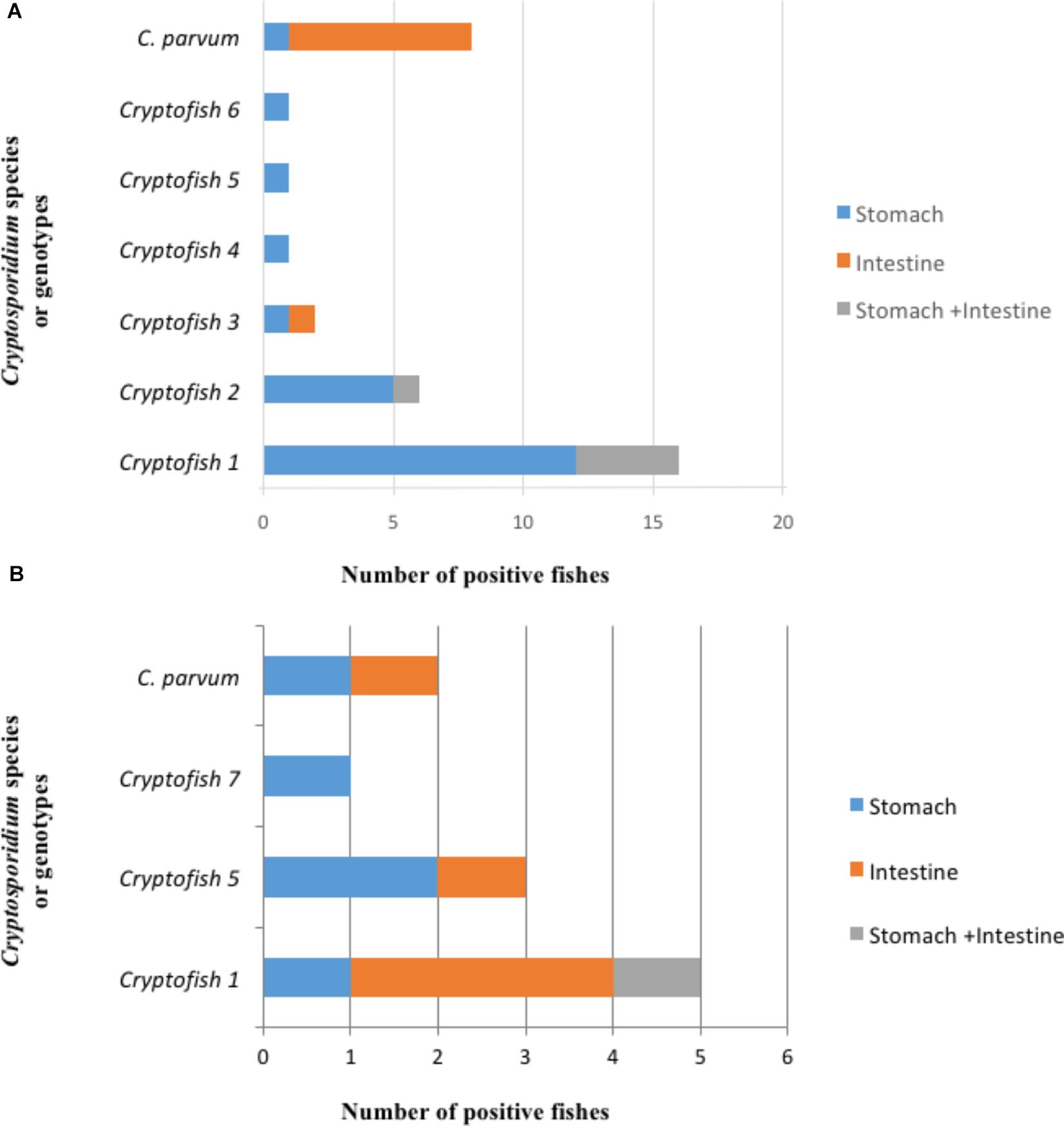
Figure 4. Distribution of different types of Cryptosporidium sequences identified at the 18S rRNA gene locus according to the anatomical location. (A) First survey (n = 35). C. parvum was identified in the intestine of 7 fishes and in the stomach of one fish, while the C. molnari-like genotype (#Cryptofish 6) was found in the stomach of one fish. The various novel Cryptosporidium genotypes were detected in the stomach of 20 fish, in the intestine of one fish and in both the intestine and stomach of 5 fish. (B) Second survey (n = 11). C. parvum was identified in the bowel of one fish and the stomach of one fish. The 3 novel Cryptosporidium genotypes were identified simultaneously in the stomach and bowel of one fish, in the stomach only of four fishes, and in the intestine only of four fishes.
After examination of histological sections from the digestive tract, the presence of Cryptosporidium-like bodies within the cells of the intestinal epithelium and in apical position was detected in one C. parvum-positive fish (Figure 5). The presence of parasites could not be studied in all positive fishes due to considerable lysis of tissues.
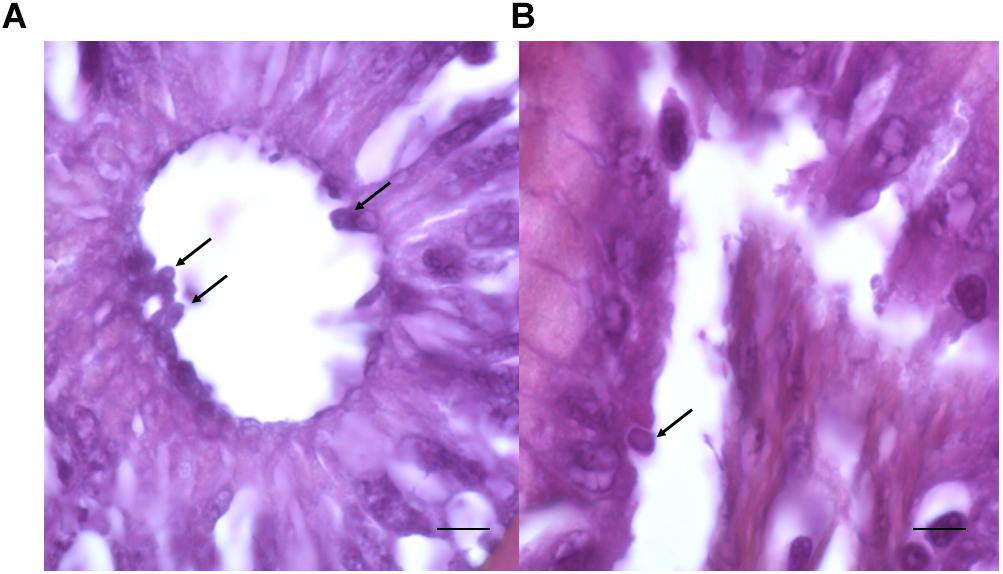
Figure 5. Stained sections of the intestinal tract of fishes. (A,B) Presence of round bodies suggestive of the developmental stages of C. parvum observed in the apical position (arrows) within the intestinal epithelial cells. Bars = 15 μm (A) and 5 μm (B).
Concerning the second survey, in total, 345 fishes were collected in this regional survey, from fishermen at Boulogne-Sur-Mer. Cryptosporidium exhibited a prevalence of 3.2% (11/345) in edible fish. The parasite was only detected in Pollachius virens and Scomber scombrus, with Pollachius virens the species with the highest prevalence (Table 4), confirming data collected from the national survey. After genotyping, C. parvum was identified in two fishes. Additionally, genotype # Cryptofish 1 was identified in six isolates, genotype # Cryptofish 5 in three and another novel genotype #Cryptofish 7 which exhibited 9.1–10.4% genetic distance from C. molnari was identified in one isolate (Figure 2). Distribution of the different types of Cryptosporidium sequences identified in their corresponding host species is shown in Figure 3. Unfortunately, C. parvum samples from this survey were unsuccessfully subtyped by sequence analysis of the gp60.
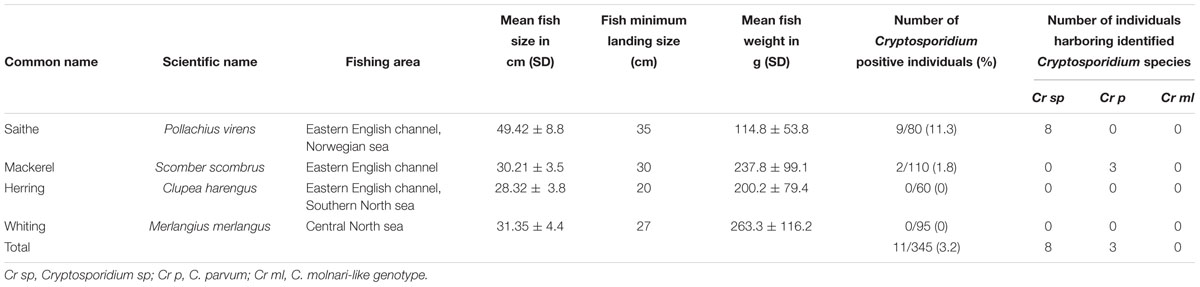
Table 4. Cryptosporidium distribution in wild marine fishes identified at the 18S rRNA gene locus (regional survey).
The selective extraction of DNA from the organs allowed the identification of C. parvum in the bowel of one fish and the stomach of one fish. The various novel Cryptosporidium genotypes were identified simultaneously in the stomach and bowel of one fish, in the stomach only of four fishes, and in the intestine only of four fishes. In Figure 4B, the distribution of species/genotypes according to anatomical location is shown.
Discussion
This is the first epidemiological and molecular data on the presence of Cryptosporidium in edible marine fishes in European waters. Overall, the prevalence of Cryptosporidium spp. in sampled fish reached 2.3% in the first campaign and 3.2% in the second campaign. A lower Cryptosporidium prevalence in marine fish was found in Western Australia (0.8%) (Reid et al., 2010) and in Papua New Guinea 1.4% (Koinari et al., 2013).
A higher prevalence of Cryptosporidium was found in fishes from retailers. As explained before, fish retailers were chosen to complement marine fish species, as the fishing areas covered by them were the same as the research campaigns. One hypothesis to explain the higher prevalence in retail fishes would be that thanks to retailers the collection of all Pollachius virens specimens sampled for this study was possible. This fish species had the highest Cryptosporidium prevalence contributing to an increase in the overall prevalence of specimens sampled inland. Pollachius virens may be more susceptible to this parasite for unknown reasons, further studies need to be done to clarify this aspect. It is not likely that holding the fish prior to processing or the handling itself affected parasite abundance since multiple measures to avoid cross contamination between specimens were taken as a precaution in each experimental step, as described in the materials and methods section.
Previously, other studies described a high prevalence of Cryptosporidium spp. in marine fish, but mainly in juveniles. In cultured marine fish, the prevalence of C. molnari in Dicentrarchus labrax and Sparus aurata was 50 and 95% in hatcheries and 58 and 65% in the ongrowing systems, respectively (Sitjà-Bobadilla et al., 2005). Prevalence rates of up to 100% for C. scophthalmi were also reported in juvenile turbot (Psetta maxima) (Alvarez-Pellitero et al., 2004). Interestingly, in the present study Cryptosporidium was not detected in fishes sampled from aquaculture. The absence of parasite species in fishes from aquaculture has been attributed to the strengthened immunity of properly raised fish in aquaculture systems (Mladineo and Poljak, 2014). In addition, farmed fishes are usually fed with fish controlled food. This practice may likely decrease the risk for parasite transmission. In mammals, including calves, pigs and humans, it has been reported that Cryptosporidium infections are usually more frequent in neonates and young individuals and less prevalent in adults (Ryan et al., 2014). Due to our sampling method, according to size and weight (and sexual maturity when this parameter could be determined) all the analyzed fishes including those from aquaculture were adults (Tables 3, 4).
In addition, the highest prevalence of Cryptosporidium spp. was observed in larger fishes according to the weight and size groupings. A previous study reported a Cryptosporidium prevalence of 5% in adult farmed rainbow trout (Oncorhynchus mykiss), but a higher Cryptosporidium prevalence of 14% was observed in younger animals (Couso-Pérez et al., 2018). As juvenile fish were not analyzed in the present study, the Cryptosporidium prevalence may be underestimated. However, the higher prevalence in larger fish in the present study could be due to parasite accumulation during the lifetime of the fish, as it has been reported for other parasites such as Anisakis (Valero et al., 2000), and longer exposure to contaminated water (Couso-Pérez et al., 2018) or more consumption of prey potentially carrying parasites.
Interestingly, 35.5% of selected sampled species were positive for Cryptosporidium, and eleven new species of fish were identified as potential hosts for Cryptosporidium (Tables 3, 4) increasing the host range for this waterborne parasite. Among these fish hosts the most commonly Cryptosporidium positive were: Pollachius virens (saithe), Gadus morhua (cod) and Molva dypterygia (blue ling) belonging to the order Gadiformes and Scomber scombrus (mackerel) belonging to the order Perciformes. As already mentioned above, Pollachius virens was the species in which the parasite was most frequently detected in both national and regional campaigns, with a 16-fold higher risk of being Cryptosporidium positive. Interestingly, in these hosts, one type of sequence (#Cryptofish1) was recorded in the majority of animals either in the national or the regional campaign. The behavior of these fish species could influence parasite transmission. As gadid fishes are social and gregarious (Anders et al., 2017), parasite transmission could occur during cohabitation in dense groupings. This type of transmission was reported in a population of reared fishes experimentally infected with C. molnari (Sitjà-Bobadilla and Alvarez-Pellitero, 2003). Moreover, as the fish species included in our survey were predators, Cryptosporidium could be transmitted to other fishes through the food chain. For example, Mendez-Hermida (Méndez-Hermida et al., 2007) reported the potential role of the live microcrustacean Artemia salina as a Cryptosporidium vehicle for piscine fishes. As fishes in the present study were sampled mainly off shore, contamination through coastal water would appear to be less significant. However, in some fish species, particularly those caught in the Mediterranean sea, and in the case of intertidal fish (moving in and out of the seashore), this kind of transmission cannot be excluded.
One species of Cryptosporidium (C. parvum) and seven genotypes were detected in the present report. The 18S rRNA gene sequences of all C. parvum isolates identified in the present study in either the national or the regional campaign were 99–100% identical to those of C. parvum GenBank reference sequences. The genotypes, however, exhibited substantial genetic distances from C. molnari (7.3–10.1%), with the exception of one genotype (#Cryptofish 6) which exhibited 0.5–0.7% genetic distance from C. molnari and was 100% identical to C. molnari-like genotypes from Peach anthias (Pseudanthias dispar) (KR610356) and Murray Cod (HQ585890). This “C. molnari-like genotype” has been previously described (Zanguee et al., 2010), and more recently has also been characterized at the actin locus (Yang et al., 2015), where it exhibited 7.3–8.7% genetic distance from C. molnari and is therefore likely to be a novel species. Similarly, the large genetic distances of genotypes #1–5 and #7 from C. molnari also indicate that they are representative of novel species, even if further analysis at the actin locus is required for confirmation. The high diversity of Cryptosporidium species and genotypes identified in fish in the present and in other studies indicate a long-term association of Cryptosporidium to their fish hosts. In addition, when considering the spectrum of fish hosts, C. parvum seems to have a broad host range (Figure 3).
Seasonality of Cryptosporidium spp distribution was observed in the present study, with maximal prevalence occurring in spring and summer. Consistently, a seasonal distribution of C. molnari was also described in farmed gilthead seabream in Spain, with maximal parasite intensity and prevalence during the same seasons (Sitjà-Bobadilla et al., 2005). Variations in the intensity of parasitic infections linked to seasonality have been described in marine ecosystems (Wilson et al., 2001) and seasonal changes may influence the physiology of the host including the immune function or the intensity of feeding. This correlation has been also reported in natural infections of different fishes (Sitjà-Bobadilla et al., 2005) and in other Cryptosporidium infected non-piscine hosts including humans (Jagai et al., 2009; Wells et al., 2015), however, it is difficult to know if the seasonality found in the present study could be attributed to the infection or more likely to different fish species caught at each season.
The identification of C. parvum among edible fish hosts is of public health significance, as this species is the most common source of zoonotic infections (Ryan et al., 2014). Previously, studies in Papua New Guinea, Australia and Spain also described the detection of C. parvum in fishes (Reid et al., 2010; Koinari et al., 2013; Certad et al., 2015; Couso-Pérez et al., 2018). The presence of C. parvum in fish samples, and in particular the IIa subtype (IIaA13G1R1, IIaA15G2R1, IIaA17G2R1, and IIaA18G3R1) is maybe related to water contamination by animal and human wastes. Indeed, the zoonotic C. parvum IIa subtype family has mainly been reported in humans and calves in Europe, North America, and Australia (Xiao, 2010; Follet et al., 2011). Subtypes IIaA17G2R1 and IIaA15G2R1 were identified in North Atlantic fishes and are the same subtypes that were previously identified in the Geneva lake, France (Certad et al., 2015).
Cryptosporidium parvum subtypes IIaA18G3R1 and IIaA15G2R1 have been detected in foals in Brazil (Inácio et al., 2017) and are also common subtypes in both humans and cattle worldwide including Australia (Zahedi et al., 2016a). The subtype IIaA13G1R1 has been identified in lambs, goats, and wild boars in Spain (Díaz et al., 2018).
In a previous study from our group, the presence of C. molnari was detected by nested 18S rRNA PCR and sequencing in freshwater European perch (Perca fluviatilis) filets (Certad et al., 2015). We suggested that filet contamination with the parasite could occur after handling the fish. Although there is no evidence of transmission of Cryptosporidium from fish hosts to mammals (Certad et al., 2010), the presence of the parasite in filets highlighted the risk of Cryptosporidium infection to humans, either during the preparation process of fish or when consuming uncooked or undercooked fish carrying zoonotic species of this parasite.
The findings of the present study are important from a public health point of view considering that some of these fish species are hosts for C. parvum including pilchards, anchovies, and herrings which are commonly eaten after only a slight preparation (for example salted or marinated), and even without gutting (Sánchez-Monsalvez et al., 2005; Mladineo and Poljak, 2014).
In addition, it was reported that fishermen were at risk of cryptosporidiosis after fishing and consuming captured fish (Roberts et al., 2007) and that C. parvum oocysts could be transferred to persons handling blue crabs (Graczyk et al., 2007). Moreover, immunosuppressed patients are also at risk of Cryptosporidium infection, either by consumption of raw or undercooked fish or by contact with fish during preparation and handling (McOliver et al., 2009).
Since C. parvum is a zoonotic species, fish carrying this species are a potential source of infection for other animals and in particular for humans, and may also contribute to the contamination of the aquatic ecosystem. Nevertheless, it is not still clear if C. parvum can cause a true infection and multiply in fish hosts. The analysis of digestive histological sections from a C. parvum-positive marine fish however, allowed the identification of intracellular round bodies in apical position suggestive of C. parvum developmental stages on epithelial cells. No signal was detected when immunofluorescence using an anti-Cryptosporidium antibody (Crypto Cel immunofluorescence test, Cellabs, Brookvale, New South Wales, and Australia) was performed to confirm the presence of the parasite in fish tissues. This failure was probably due to the fact that formalin progressively cross-links proteins of the parasite, particularly after long time of formalin fixation (Barugahare et al., 2011). Further studies have to be done to confirm this aspect.
In conclusion, this study provides the first epidemiological data regarding the presence of Cryptosporidium in marine edible fish in European waters. New fish species were identified as hosts for this parasite. In addition, the influence of host factors such as the species, the weight and size groupings and environmental factors such as geographical localization or seasonality were shown to have an impact on parasite infection. Finally, the detection of zoonotic Cryptosporidium in fish suggests that the parasite may represent a sentinel for environmental contamination. Since wildlife can potentially contribute to Cryptosporidium contamination of water systems, the identification of the sources/carriers of zoonotic strains is needed for accurate risk assessment (Zahedi et al., 2016b). In addition, as the consumption of raw or thermally inadequately treated fishery products represents novel trends in human eating habits, strategic research in the field is extremely important. Further studies are required to confirm the species status of the seven novel genotypes identified.
Author Contributions
GC, JF, KG, EF, VV-B, MG, CA-D, and EV conceived and designed the experiments. NG, OH-G, SB-V, YS, BD, and CC performed the experiments. GC, JF, NG, GE, UR, and EV analyzed the data. GE, VV-B, MG, and CA-D contributed to reagents, materials, and analysis tools. GC and UR wrote the manuscript.
Funding
This work was supported by the French National Research Agency (Grant No. ANR 2010 ALIA 004-01), the Conseil Regional Hauts-de-France (Concerted Research Actions of Regional Initiative, ARCir 13 ABC FISH No. 13003283), and the regional competitiveness center, AQUIMER (Boulogne s/mer, France).
Conflict of Interest Statement
The authors declare that the research was conducted in the absence of any commercial or financial relationships that could be construed as a potential conflict of interest.
Acknowledgments
We dedicate this article to the memory of Eduardo Dei-Cas. We wish to thank the Ifremer scientific campaigns (PELGAS, PELMED, EVOHE, and IBTS), Dr. Slapeta for kindly providing us with the DNA from C. molnari-like genotype, and the Fish-Parasites Network.
Supplementary Material
The Supplementary Material for this article can be found online at: https://www.frontiersin.org/articles/10.3389/fmicb.2019.01037/full#supplementary-material
FIGURE S1 | Hierarchical cluster analysis was used to define variables (R stats package). Five groups were defined ranging from 1-smallest fishes to 5-largest fishes.
FIGURE S2 | The number of base substitutions per site from between sequences are shown. Analyses were conducted using the Tamura 3-parameter model. The analysis involved 29 nucleotide sequences. All positions with less than 95% site coverage were eliminated. That is, fewer than 5% alignment gaps, missing data, and ambiguous bases were allowed at any position. There were a total of 371 positions in the final dataset. Evolutionary analyses were conducted in MEGA6.
Footnotes
References
Agence Française de Sécurité Sanitaire des Aliments [ANSES] (2010). Consommation des Poissons, Mollusques et Crustacés: Aspects Nutritionnels et Sanitaires Pour L’Homme (RAPPORT). Maisons-Alfort Cedex: ANSES.
Alvarez-Pellitero, P., Quiroga, M. I., Sitjà-Bobadilla, A., Redondo, M. J., Palenzuela, O., Padrós, F., et al. (2004). Cryptosporidium n. sp. (Apicomplexa: Cryptosporidiidae) from cultured turbot Scophthalmus maximus. light and electron microscope description and histopathological study. Dis. Aquat. Organ. 62, 133–145. doi: 10.3354/dao062133
Alvarez-Pellitero, P., and Sitjà-Bobadilla, A. (2002). Cryptosporidium molnari n. sp. (Apicomplexa: Cryptosporidiidae) infecting two marine fish species, Sparus aurata L. and Dicentrarchus labrax L. Int. J. Parasitol. 32, 1007–1021.
Anders, N., Fernö, A., Humborstad, O.-B., Løkkeborg, S., Rieucau, G., and Utne-Palm, A. C. (2017). Size-dependent social attraction and repulsion explains the decision of Atlantic cod Gadus morhua to enter baited pots. J. Fish Biol. 91, 1569–1581. doi: 10.1111/jfb.13453
Barugahare, R., Dennis, M. M., Becker, J. A., and Slapeta, J. (2011). Detection of Cryptosporidium molnari oocysts from fish by fluorescent-antibody staining assays for Cryptosporidium spp. affecting humans. Appl. Environ. Microbiol. 77, 1878–1880. doi: 10.1128/AEM.02691-10
Benson, D. A., Karsch-Mizrachi, I., Lipman, D. J., Ostell, J., and Wheeler, D. L. (2004). GenBank. Nucleic Acids Res. 33, D34–D38. doi: 10.1093/nar/gki063
Broglia, A., and Kapel, C. (2011). Changing dietary habits in a changing world: emerging drivers for the transmission of foodborne parasitic zoonoses. Vet. Parasitol. 182, 2–13. doi: 10.1016/j.vetpar.2011.07.011
Certad, G., Creusy, C., Guyot, K., Mouray, A., Chassat, T., Delaire, B., et al. (2010). Fulminant cryptosporidiosis associated with digestive adenocarcinoma in SCID mice infected with Cryptosporidium parvum TUM1 strain. Int. J. Parasitol. 40, 1469–1475. doi: 10.1016/j.ijpara.2010.07.007
Certad, G., Dupouy-Camet, J., Gantois, N., Hammouma-Ghelboun, O., Pottier, M., Guyot, K., et al. (2015). Identification of Cryptosporidium species in fish from Lake Geneva (Lac Léman) in France. PLoS One 10:e0133047. doi: 10.1371/journal.pone.0133047
Collins, J. E. (1997). Impact of changing consumer lifestyles on the emergence/reemergence of foodborne pathogens. Emerg. Infect. Dis. 3, 471–479. doi: 10.3201/eid0304.970409
Couso-Pérez, S., Ares-Mazás, E., and Gómez-Couso, H. (2018). Identification of a novel piscine Cryptosporidium genotype and Cryptosporidium parvum in cultured rainbow trout (Oncorhynchus mykiss). Parasitol. Res. 9, 2987–2996. doi: 10.1007/s00436-018-5995-3
Darriba, D., Taboada, G. L., Doallo, R., and Posada, D. (2012). jModelTest 2: more models, new heuristics and parallel computing. Nat. Methods 9:772. doi: 10.1038/nmeth.2109
Díaz, P., Navarro, E., Prieto, A., Pérez-Creo, A., Viña, M., Díaz-Cao, J. M., et al. (2018). Cryptosporidium species in post-weaned and adult sheep and goats from N.W. Spain: Public and animal health significance. Vet. Parasitol. 254, 1–5. doi: 10.1016/j.vetpar.2018.02.040
Elwakil, H. S., and Hewedi, I. H. (2010). Pathogenic potential of blastocystis hominis in laboratory mice. Parasitol. Res. 107, 685–689. doi: 10.1007/s00436-010-1922-y
FAO (2018). The State of World Fisheries and Aquaculture 2018 - Meeting the sustainable development goals. Rome: FAO.
Follet, J., Guyot, K., Leruste, H., Follet-Dumoulin, A., Hammouma-Ghelboun, O., Certad, G., et al. (2011). Cryptosporidium infection in a veal calf cohort in France: molecular characterization of species in a longitudinal study. Vet. Res. 42:116. doi: 10.1186/1297-9716-42-116
Gatei, W., Das, P., Dutta, P., Sen, A., Cama, V., Lal, A. A., et al. (2007). Multilocus sequence typing and genetic structure of Cryptosporidium hominis from children in Kolkata, India. Infect. Genet. Evol. 7, 197–205. doi: 10.1016/j.meegid.2006.08.006
Graczyk, T. K., McOliver, C., Silbergeld, E. K., Tamang, L., and Roberts, J. D. (2007). Risk of handling as a route of exposure to infectious waterborne Cryptosporidium parvum oocysts via Atlantic blue crabs (Callinectes sapidus). Appl. Environ. Microbiol. 73, 4069–4070. doi: 10.1128/AEM.00166-07
Greenacre, M., and Blasius, J. (2006). Multiple Correspondence Analysis and Related Methods, 1st Edn. Boca Raton: Chapman and Hall/CRC.
Inácio, S. V., Zucatto, A. S., de Aquino, M. C. C., Oliveira, B. C. M., Bresciani, K. D. S., Widmer, G., et al. (2017). First description of Cryptosporidium hominis GP60 genotype IkA20G1 and Cryptosporidium parvum GP60 genotypes IIaA18G3R1 and IIaA15G2R1 in foals in Brazil. Vet. Parasitol. 233, 45–51. doi: 10.1016/j.vetpar.2016.11.021
Jagai, J. S., Castronovo, D. A., Monchak, J., and Naumova, E. N. (2009). Seasonality of cryptosporidiosis: a meta-analysis approach. Environ. Res. 109, 465–478. doi: 10.1016/j.envres.2009.02.008
Koinari, M., Karl, S., Ng-Hublin, J., Lymbery, A. J., and Ryan, U. M. (2013). Identification of novel and zoonotic Cryptosporidium species in fish from Papua New Guinea. Vet. Parasitol. 198, 1–9. doi: 10.1016/j.vetpar.2013.08.031
Kumar, S., Stecher, G., and Tamura, K. (2016). MEGA7: molecular evolutionary genetics analysis version 7.0 for bigger datasets. Mol. Biol. Evol. 33, 1870–1874. doi: 10.1093/molbev/msw054
McOliver, C. C., Lemerman, H. B., Silbergeld, E. K., Moore, R. D., and Graczyk, T. K. (2009). Risks of recreational exposure to waterborne pathogens among persons with HIV/AIDS in Baltimore, Maryland. Am. J. Public Health 99, 1116–1122. doi: 10.2105/AJPH.2008.151654
Méndez-Hermida, F., Gómez-Couso, H., and Ares-Mazás, E. (2007). Possible involvement of Artemia as live diet in the transmission of cryptosporidiosis in cultured fish. Parasitol. Res. 101, 823–827. doi: 10.1007/s00436-007-0543-6
Mladineo, I., and Poljak, V. (2014). Ecology and genetic structure of zoonotic Anisakis spp. from adriatic commercial fish species. Appl. Environ. Microbiol. 80, 1281–1290. doi: 10.1128/AEM.03561-13
Murphy, B. G., Bradway, D., Walsh, T., Sanders, G. E., and Snekvik, K. (2009). Gastric cryptosporidiosis in freshwater angelfish (Pterophyllum scalare). J. Vet. Diagn. Invest. 21, 722–727. doi: 10.1177/104063870902100523
Omarova, A., Tussupova, K., Berndtsson, R., Kalishev, M., and Sharapatova, K. (2018). Protozoan parasites in drinking water: A system approach for improved water, sanitation and hygiene in developing countries. Int. J. Environ. Res. Public Health 15:495. doi: 10.3390/ijerph15030495
Palenzuela, O., Alvarez-Pellitero, P., and Sitjá-Bobadilla, A. (2010). Molecular characterization of Cryptosporidium molnari reveals a distinct piscine clade. Appl. Environ. Microbiol. 76, 7646–7649. doi: 10.1128/AEM.01335-10
Paparini, A., Yang, R., Chen, L., Tong, K., Gibson-Kueh, S., Lymbery, A., et al. (2017). Cryptosporidium in fish: Alternative sequencing approaches and analyses at multiple loci to resolve mixed infections. Parasitology 144, 1–10. doi: 10.1017/S0031182017001214
Reid, A., Lymbery, A., Ng, J., Tweedle, S., and Ryan, U. (2010). Identification of novel and zoonotic Cryptosporidium species in marine fish. Vet. Parasitol. 168, 190–195. doi: 10.1016/j.vetpar.2009.11.015
Roberts, J. D., Silbergeld, E. K., and Graczyk, T. (2007). A probabilistic risk assessment of Cryptosporidium exposure among Baltimore urban anglers. J. Toxicol. Environ. Health. A 70, 1568–1576. doi: 10.1080/15287390701384791
Ronquist, F., Teslenko, M., van der Mark, P., Ayres, D. L., Darling, A., Höhna, S., et al. (2012). MrBayes 3.2: efficient Bayesian phylogenetic inference and model choice across a large model space. Syst. Biol. 61, 539–542. doi: 10.1093/sysbio/sys029
Ryan, U., Fayer, R., and Xiao, L. (2014). Cryptosporidium species in humans and animals: current understanding and research needs. Parasitology 141, 1667–1685. doi: 10.1017/S0031182014001085
Ryan, U., Hijjawi, N., and Xiao, L. (2018). Foodborne cryptosporidiosis. Int. J. Parasitol. 48, 1–12. doi: 10.1016/j.ijpara.2017.09.004
Ryan, U., Paparini, A., Tong, K., Yang, R., Gibson-Kueh, S., O’Hara, A., et al. (2015). Cryptosporidium huwi n. sp. (Apicomplexa: Eimeriidae) from the guppy (Poecilia reticulata). Exp. Parasitol. 150, 31–35. doi: 10.1016/j.exppara.2015.01.009
Sánchez-Monsalvez, I., De Armas-Serra, C., Martínez, J., Dorado, M., Sánchez, A., and Rodríguez-Caabeiro, F. (2005). A new procedure for marinating fresh anchovies and ensuring the rapid destruction of Anisakis larvae. J. Food Prot. 68, 1066–1072. doi: 10.4315/0362-028X-68.5.1066
Sitjà-Bobadilla, A., and Alvarez-Pellitero, P. (2003). Experimental transmission of Cryptosporidium molnari (Apicomplexa: Coccidia) to gilthead sea bream (Sparus aurata L.) and European sea bass (Dicentrarchus labrax L.). Parasitol. Res. 91, 209–214. doi: 10.1007/s00436-003-0945-z
Sitjà-Bobadilla, A., Padrós, F., Aguilera, C., and Alvarez-Pellitero, P. (2005). Epidemiology of Cryptosporidium molnari in Spanish gilthead sea bream (Sparus aurata L.) and European sea bass (Dicentrarchus labrax L.) cultures: from hatchery to market size. Appl. Environ. Microbiol. 71, 131–139. doi: 10.1128/AEM.71.1.131-139.2005
Valero, A., Martin-Sánchez, J., Reyes-Muelas, E., and Adroher, F. J. (2000). Larval anisakids parasitizing the blue whiting, Micromesistius poutassou, from Motril bay in the Mediterranean region of southern Spain. J. Helminthol. 74, 361–364. doi: 10.1017/S0022149X00000536
Wells, B., Shaw, H., Hotchkiss, E., Gilray, J., Ayton, R., Green, J., et al. (2015). Prevalence, species identification and genotyping Cryptosporidium from livestock and deer in a catchment in the Cairngorms with a history of a contaminated public water supply. Paras. Vect. 8:66. doi: 10.1186/s13071-015-0684-x
Wilson, K., Bjørnstad, O. N., Dobson, A. P., Merler, S., Poglayen, G., Randolf, S. E., et al. (2001). “Heterogeneities in macroparasite infections: patterns and processes,” in The Ecology of Wildlife Diseases, eds P. J. Hudson, A. Rizzoli, B. T. Grenfell, H. Heesterbeek, and A. P. Dobson (Oxford: Oxford University Press), 6–44.
Xiao, L. (2010). Molecular epidemiology of cryptosporidiosis: an update. Exp. Parasitol. 124, 80–89. doi: 10.1016/j.exppara.2009.03.018
Yang, R., Dorrestein, G. M., and Ryan, U. (2016). Molecular characterisation of a disseminated Cryptosporidium infection in a Koi carp (Cyprinus carpio). Vet. Parasitol. 226, 53–56. doi: 10.1016/j.vetpar.2016.06.027
Yang, R., Palermo, C., Chen, L., Edwards, A., Paparini, A., Tong, K., et al. (2015). Genetic diversity of Cryptosporidium in fish at the 18S and actin loci and high levels of mixed infections. Vet. Parasitol. 214, 255–263. doi: 10.1016/j.vetpar.2015.10.013
Zahedi, A., Monis, P., Aucote, S., King, B., Paparini, A., Jian, F., et al. (2016a). Zoonotic Cryptosporidium species in animals inhabiting sydney water catchments. PLoS One 11:e0168169. doi: 10.1371/journal.pone.0168169
Zahedi, A., Paparini, A., Jian, F., Robertson, I., and Ryan, U. (2016b). Public health significance of zoonotic Cryptosporidium species in wildlife: Critical insights into better drinking water management. Int. J. Parasitol. Paras. Wildl. 5, 88–109. doi: 10.1016/j.ijppaw.2015.12.001
Keywords: Cryptosporidium, edible marine fish, 18S rRNA gene, gp60, molecular epidemiology, phylogeny, novel genotypes, European seas
Citation: Certad G, Follet J, Gantois N, Hammouma-Ghelboun O, Guyot K, Benamrouz-Vanneste S, Fréalle E, Seesao Y, Delaire B, Creusy C, Even G, Verrez-Bagnis V, Ryan U, Gay M, Aliouat-Denis C and Viscogliosi E (2019) Prevalence, Molecular Identification, and Risk Factors for Cryptosporidium Infection in Edible Marine Fish: A Survey Across Sea Areas Surrounding France. Front. Microbiol. 10:1037. doi: 10.3389/fmicb.2019.01037
Received: 14 December 2018; Accepted: 24 April 2019;
Published: 15 May 2019.
Edited by:
Julio Parra-Flores, University of the Bío-Bío, ChileReviewed by:
Rebecca Gast, Woods Hole Oceanographic Institution, United StatesMario Santoro, Istituto Zooprofilattico Sperimentale del Mezzogiorno, Italy
Jose Guadalupe Rendon-Maldonado, Autonomous University of Sinaloa, Mexico
Copyright © 2019 Certad, Follet, Gantois, Hammouma-Ghelboun, Guyot, Benamrouz-Vanneste, Fréalle, Seesao, Delaire, Creusy, Even, Verrez-Bagnis, Ryan, Gay, Aliouat-Denis and Viscogliosi. This is an open-access article distributed under the terms of the Creative Commons Attribution License (CC BY). The use, distribution or reproduction in other forums is permitted, provided the original author(s) and the copyright owner(s) are credited and that the original publication in this journal is cited, in accordance with accepted academic practice. No use, distribution or reproduction is permitted which does not comply with these terms.
*Correspondence: Gabriela Certad, Z2FicmllbGEuY2VydGFkQHBhc3RldXItbGlsbGUuZnI=
†These authors have contributed equally to this work