- 1College of Food Science and Engineering, Northwest A&F University, Yangling, China
- 2Shenzhen Key Laboratory for Food Biological Safety Control, Food Safety and Technology Research Centre, The Hong Kong PolyU Shenzhen Research Institute, Shenzhen, China
- 3State Key Laboratory of Chirosciences, Department of Applied Biology and Chemical Technology, The Hong Kong Polytechnic University, Kowloon, Hong Kong
- 4College of Veterinary Medicine, Yangzhou University, Yangzhou, China
This study aims to investigate and compare the complete nucleotide sequences of the multidrug resistance plasmids pVb0267 and pVb0499, which were recovered from foodborne Vibrio parahaemolyticus isolates, and analyze the genetic environment of blaCTX–M–55 to provide insight into the dissemination mechanisms of this resistance element. Analysis of the sequences of plasmids pVb0267 (166,467 bp) and pVb0499 (192,739 bp) revealed that the backbones of these two plasmids exhibited a high degree of similarity with pR148, a recognized type 1a IncC plasmid recovered from Aeromonas hydrophila (99% identity). The resistance genes, found in both plasmids, included qacH, aadB, arr2, blaOXA–10, aadA1, sul1, tet(A), and blaCTX–M–55, which were mostly arranged in a specific region designated ARI-A. Plasmid pVb0499 was found to possess a larger size of ARI-A than pVb0267, which lacked a mer determination region, a qnr A segment, an aacC3 gene and several mobility-encoding genes. Comparative analysis of resistance island (RI) of these plasmids and others revealed the potential evolution route of these RI sequences. In conclusion, plasmids harboring the blaCTX–M–55 gene has been recovered in Vibrio parahaemolyticus strains of food origin. It is alarming to find that IncC plasmids harboring resistance islands are disseminating in aquatic bacterial strains. The continuous evolution of resistance genes in conjugative plasmid in aquatic bacteria could be due to bacterial adaption to aquaculture environment, where antibiotics were increasingly used.
Introduction
Vibrio parahaemolyticus is a gram-negative, halophilic, mesophilic, rod-shaped human pathogen that naturally occurs in the marine or estuarine environment (Lüdeke et al., 2015). Although V. parahaemolyticus is frequently isolated from a variety of seafood, such as shrimp, oyster, and fish, most isolates are non-pathogenic to human (Nishibuchi and Kaper, 1985). However strains carrying the tdh and trh genes can cause acute human gastroenteritis with the symptoms of headache, abdominal pain, and diarrhea, and in rare cases, wound infection and septicemia (Nishibuchi and Kaper, 1985; Zhang and Austin, 2005; Broberg et al., 2011). Seafood has gained enormous popularity leading to high demand, which has resulted in steady expansion of the Asian aquaculture industry. As a major vehicle of transmission of foodborne bacteria, increasing consumption of seafood could also lead to increase in foodborne illnesses in human. V. parahaemolyticus has become one of the most common causative agents of foodborne diseases in China in recent years, with the majority of infection cases being linked to consumption of contaminated seafood (Chen et al., 2010; Peng et al., 2010).
With the development and expansion of aquaculture practices, the health of aquatic animal has been under constant challenge (Bondad-Reantaso et al., 2005). To prevent and treat bacterial infections, a broad range of antibiotics such as oxytetracycline, tetracycline, quinolones, sulphonamides, and trimethoprim has been permitted for usage in the Asian aquaculture industry (Yano et al., 2014). The extensive use of antibiotics in aquaculture has resulted the emergence of antibiotic resistant strains in the environment. Besides, an increasing trend of resistance to front line antibiotics such as ciprofloxacin and ceftriaxone in V. parahaemolyticus has been reported in China and other parts of the world. Different β-lactamase-encoding genes such as blaCMY–2, blaPER–1, and blaVEB–2, which mediate cephalosporin resistance, have been detectable in V. parahaemolyticus (Li et al., 2015a, 2015b; Briet et al., 2018). Nevertheless, genes in the CTX-M family, commonly harbored by cephalosporin resistant bacteria (Naseer and Sundsfjord, 2011), remain rarely detectable in V. parahaemolyticus or other Vibrio spp. (Zheng et al., 2018). The blaCTX–M–55 gene belongs to the CTX-M-1 group and differs from blaCTX–M–15 by a single amino acid substitution of Val-77-Ala. The blaCTX–M–55 gene was first detected in clinical strains of E. coli and K. pneumoniae isolated in Thailand in 2005 (Kiratisin et al., 2007), and was subsequently found in Salmonella spp. in China, the United States, Korea, and Switzerland (Sjölund-Karlsson et al., 2011; Dahmen et al., 2013). A major epidemiological feature of Extended Spectrum Beta-Lactamases (ESBL)-producing strains is that the blaCTX–M–55 gene has emerged as a dominant resistance genotype among these strains. The prevalence rate of clinical isolates containing blaCTX–M–55 has exceeded those carrying blaCTX–M–15 in China, becoming the second most common resistance genotype among ESBL-producing strains (Zhang et al., 2014). Detection of blaCTX–M–55 is an alarming signal that depicts successful transmission of this important ESBL gene from Enterobacteriaceae to marine organisms. Continuous surveillance of this evolution process is therefore warranted.
Generally, Vibrio spp. are known to be susceptible to most clinically used antibiotics (Shaw et al., 2014; Letchumanan et al., 2015a). It is noted that most of the antibiotic resistance determinants are located in the plasmid, which is the most important mediator that facilitate the transfer of antibiotic resistant genes (Okamoto et al., 2009; Letchumanan et al., 2015b). IncC group plasmids have been identified in various species, indicating that such plasmids have been disseminated to, and accommodated by a broad range of bacterial hosts since they were first described among multidrug resistant Aeromonas hydrophila causing disease in cultured fish in the 1970s (Fricke et al., 2009; Sekizuka et al., 2011; Harmer and Hall, 2015; Li et al., 2015a). Moreover, their role in dissemination of ESBL and carbapenemase genes has attracted attention of researchers (Fricke et al., 2009; Sekizuka et al., 2011; Li et al., 2015a). IncA and IncC plasmids were grouped as the “A-C complex” at the beginning due to structural similarities and the strong entry exclusion (Hedges, 1974). Subsequently the term IncA/C has been used frequently to describe these two kinds of plasmid (Couturier et al., 1988; Harmer and Hall, 2015). Recently IncA and IncC plasmids have been proven to be compatible in the same bacterial strain and exhibited separate evolutionary routes and significant nucleotide divergence between their backbones (Ambrose et al., 2018). Thus, IncA/C is not used together anymore to describe plasmids. Analysis of the complete sequences of IncC plasmids to date has revealed that they are mostly large, ranging in size from 110 to 200 kb, self-mobilizable and confer resistance to a broad range of antibiotics. Upon acquisition by enterobacteria in both human and animals, IncC plasmids have apparently rapidly evolved and a number of lineages have been identified subsequently, with each carrying unique resistance genes (Harmer and Hall, 2015). In this work, we described the isolation and characterization of CTX-M-55 producing V. parahaemolyticus strains of food origin and depicted the evolution of IncC types of plasmids that harbored blaCTX–M–55.
Materials and Methods
Bacterial Isolation and Identification
The V. parahaemolyticus strains tested in this work were isolated from shrimp samples collected from a free market and two supermarkets located in the Nanshan district of Shenzhen, China, during the period August to October 2015. Samples were processed as previously described (Pinto et al., 2011). Briefly, 20 g of shrimp samples was homogenized with 50 mL of sterile saline. One milliliter saline homogenate was then added to 9 mL alkaline peptone water (APW) for enrichment of the strains at 37°C overnight. After incubation, the enrichment broths were streaked onto thiosulfate-citrate-bile salts-sucrose (TCBS) agar plates and incubated at 37°C for 18–24 h. At least three typical colonies of V. parahaemolyticus were isolated from each plate and subjected to identification by multiplex PCR assays and DNA sequencing (Kim et al., 2015). The genetic identity of the isolates was further confirmed by MALDI-TOF (Bruker).
Antimicrobial Susceptibility Tests and Conjugation Assay
The V. parahaemolyticus isolates were subjected to antimicrobial susceptibility tests, using the standard agar dilution method described by the Clinical and Laboratory Standards Institute (CLSI) (Jorgensen, 2015). Escherichia coli strain ATCC 25922 was included as the quality control strain. Interpretation of results was according to the latest CLSI breakpoints. The minimal inhibitory concentrations (MICs)of eleven antibiotics were tested on the V. parahaemolyticus isolates: ceftriaxone, cefotaxime, amoxocillin-clavulanic acid, ampicillin, tetracycline, amikacin, gentamicin, ciprofloxacin, nalidixic acid, chloramphenicol, and sulfamethoxazole-trimethoprim.
Conjugation by filter mating was performed to test the transferability of mobile resistance elements between the cephalosporin-resistant V. parahaemolyticus isolates and the azide-resistant E. coli strain J53. Both the donor and recipient strains were cultured to exponential phase in LB broth, 300 μL of each culture were collected and mixed together. The mixture was spotted on a filter membrane that was placed on an LB agar plate, and then incubated for mating at 37°C for 12–18 h. Bacteria were washed from filter membrane and spread onto an Eosin methylene blue (EMB) agar plate containing 2 mg/L of cefotaxime to select transconjugants, as only E. coli strains could grow in EMB, while V. parahaemolyticus strains could not. The species of transconjugants was confirmed by MALDI-TOF. The MICs for the transconjugants were also determined according to the aforementioned method.
Molecular Detection of β-Lactamase Genes
The cephalosporin-resistant isolates and the corresponding transconjugants were screened for carriage of known β-lactamase genes using a previously described multiplex PCR assay (Dallenne et al., 2010). PCR was carried out in 20 μL of reaction mixture which contained DNA template (2 μL), 1 × PCR buffer (TaKaRa, Japan), 200 mM of each deoxynucleotide triphosphate, a variable concentration of specific-group primers (Supplementary Table S3) and 1 U of Taq polymerase (TaKaRa, Japan). Amplification was performed with the following conditions: initial denaturation at 94°C for 10 min; 30 cycles of 94°C for 40 s, 60°C for 40 s and 72°C for 1 min; and a final elongation step at 72°C for 7 min. For the carbapenemase gene multiplex PCR assays, the annealing temperature was optimal at 55°C for amplification of blaVIM, blaIMP, and blaKPC genes, and optimal at 57°C for amplification of blaGES and blaOXA–48 genes. All PCR products were visualized by using 1.5% agarose gel and sequenced for further confirmation.
Molecular Typing by PFGE and Southern Blot Analysis
To evaluate the genetic relationship of the isolates, pulsed-field gel electrophoresis (PFGE) was performed. Specifically, XbaI-digested genomic DNA was prepared according to the manufacturer’s instructions and restricted fragments were separated on a CHEF MAPPER system (Bio-Rad, United States) for 18 h at 14°C with run conditions of 6 V/cm and switch times from 6.76 to 35.38 s. The results of PFGE were interpreted as recommended by Tenover et al. (1995). To estimate the size and location of blaCTX–M–55 in the parental strain and the transconjugant, genomic DNA was digested with S1-nuclease (TaKaRa, Japan), followed by pulsed-field gel electrophoresis (S1-PFGE) as described above. The DNA fragments were then transferred to a positive-charged nylon membrane. Southern hybridization was carried out in accordance to the manufacturer’s instructions for digoxigenin (DIG)-High Prime DNA labeling, using a digoxigenin-labeled blaCTX–M–55 specific probe. Finally, the DNA fragments were detected using an NBT/BCIP color detection kit (Roche, Germany) (Hui et al., 2007). The XbaI-digested DNA of Salmonella enterica serotype Braenderup H9812 was electrophoresed as the size marker.
S1 nuclease-PFGE and Southern blotting were performed to estimate the size of plasmids in the parental strains and transconjugants. Plasmid DNA electrophoresis, followed by transfer to nylon membrane, were performed according to standard methods (Li et al., 2015a). Southern blot hybridization and probe detection were carried out in accordance with the manufacturer’s instructions of the digoxigenin (DIG)-High Prime DNA labeling and detection starter kit II (Roche Diagnostics), using a digoxigenin-labeled blaCTX–M–55 probe.
Plasmid Analysis
Plasmids of the blaCTX–M–55-positive transconjugants were extracted using the Plasmid Extraction Kit (Qiagen, Hilden, Germany). The plasmids recovered from transconjugants were sequenced by the Illumina NextSeq 500 platform and the MinION sequencing platform. The method used to perform MinION sequencing was reported previously; Unicycler was used to perform hybrid assembly (Wick et al., 2017; Li et al., 2018). The completed plasmid sequence was confirmed by PCR and then annotated with the RAST tool and the NCBI Prokaryotic Genome Annotation Pipeline. BLAST tools were used for the comparative analysis of plasmid sequence and ARI-A sequences.
Nucleotide Sequence Accession Number
The complete nucleotide sequences of pVb0267 and pVb0499 have been submitted to GenBank and were assigned the accession numbers of MF627444 and MF627445, respectively.
Results
Phenotypic and Genotypic Characteristics of Cephalosporin Resistance in V. parahaemolyticus
A total of 116 V. parahaemolyticus strains were isolated from 48 out of the 80 shrimp samples tested. The antimicrobial susceptibility test results revealed that 21 of these 116 V. parahaemolyticus strains were resistant to all β-lactam antibiotics tested, including ceftriaxone, cefotaxime, ampicillin and amoxicillin-clavulanic acid (Supplementary Table S1). Carriage of β-lactamase genes in these 21 strains was determined, with results showing that the majority of these strains carried the blaVEB–1 and blaCMY–2 genes. These two resistance genes have previously been recovered from strains of the Vibrio species and were not included for further analysis in this study (Li et al., 2015a, 2016). Two V. parahaemolyticus strains, Vb0267 and Vb0499, were found to carry blaCTX–M–55, which is commonly present in Enterobacteriaceae but has never been reported in Vibrio species. We therefore focused on deciphering the genetic basis of the evolution of blaCTX–M–55 in V. parahaemolyticus. Conjugation experiments performed on these two strains showed that they could transfer the cephalosporin-resistance phenotype to strain J53 (Table 1). S1-PFGE and Southern hybridization of both parental strains and transconjugants revealed that the blaCTX–M–55 gene was harbored by plasmids with sizes of ca. 160 and 190 kb, respectively, in the two strains (Figure 1). PFGE fingerprint analysis revealed that Vb0267 and Vb0499 exhibited distinct PFGE profiles (Supplementary Figure S1). Multilocus sequence typing (MLST) analysis showed that both strains belong to unknown genetic types.
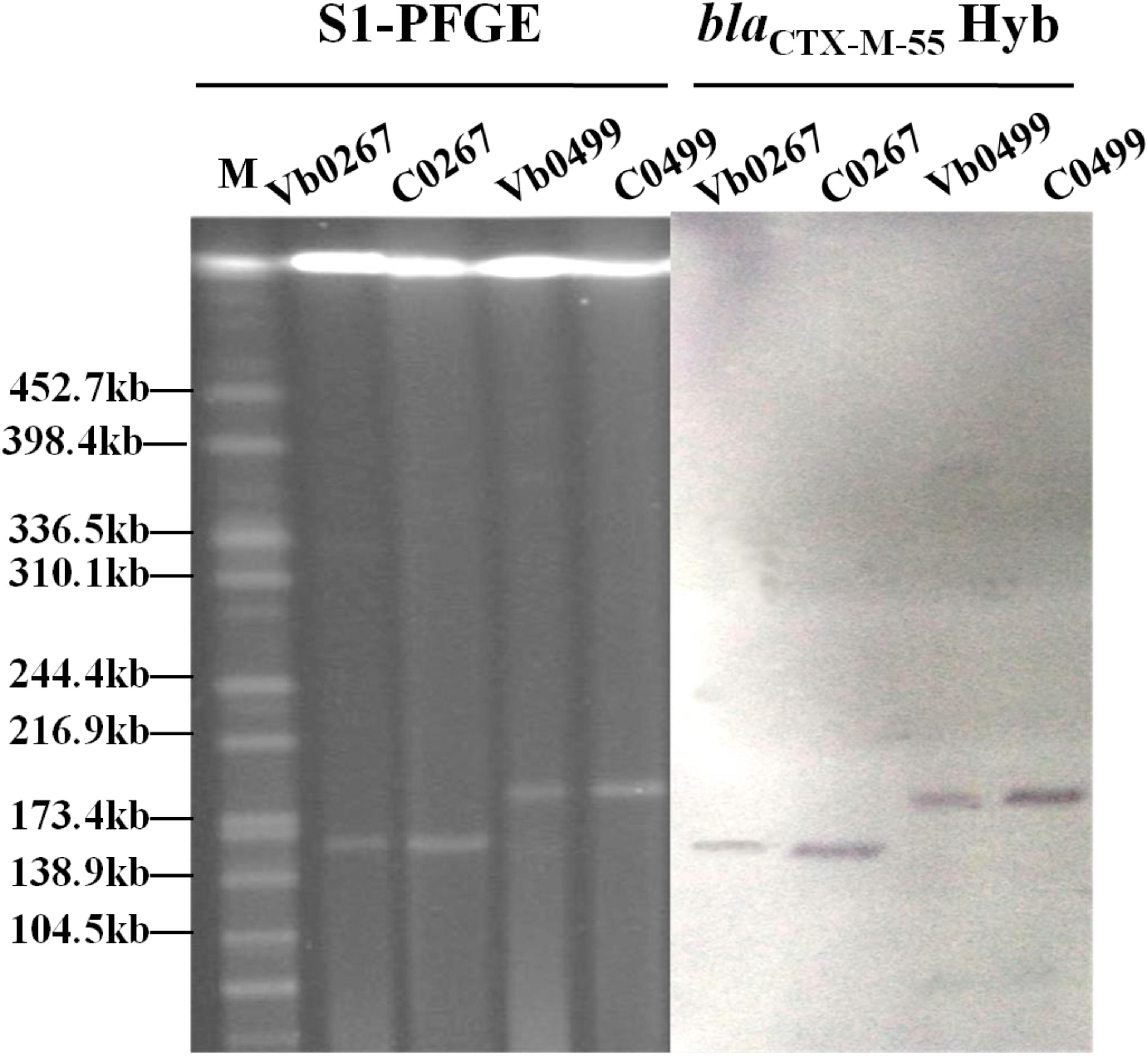
Figure 1. S1-PFGE and Southern hybridization analysis of carriage of blaCTX–M–55 -bearing plasmids in two V. parahaemolyticus strains (Vb0267 and Vb0499) and the corresponding transconjugants (C0267 and C0499). Hyb stands for Southern Hybridization.
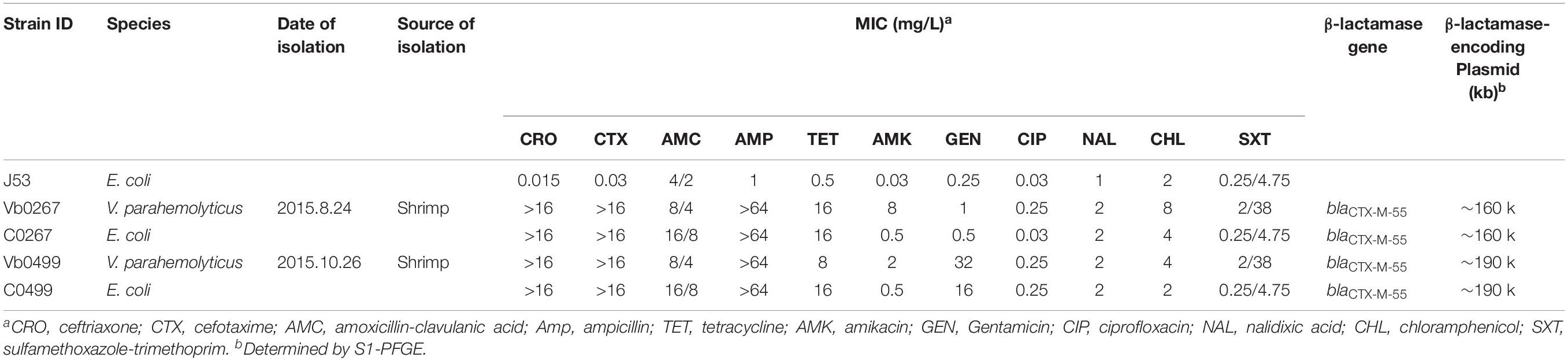
Table 1. MICs of different antibiotics among Vibrio parahaemolyticus strains tested in this study and the correspondingtransconjugants.
General Features of the blaCTX–M–55 -Bearing Plasmids pVb0267 and pVb0499
To investigate the genetic features of the two blaCTX–M–55-bearing plasmids, the complete sequences of these plasmids were obtained using the Illumina and Nanopore sequencing platforms, and were designated as pVb0267 (MF627444) and pVb0499 (MF627445), respectively. The complete nucleotide sequence of plasmid pVb0267, recovered from strain Vb0267, was 166,467 bp in length, with a mean G+C content of 51.9%, and was found to comprise 183 predicted coding sequences (CDSs). The complete nucleotide sequence of the plasmid pVb0499 was found to be 192,739 bp in size, with an average G+C content of 52.6%, and comprise a total of 213 CDSs. Complete sequence comparison showed that these two plasmids differed mainly by the genetic content of the multidrug-resistant (MDR) region (Figure 2). Sequences outside this region exhibited 100% identity between the two plasmids. Besides, BLAST analysis revealed that the backbones of pVb0267 and pVb0499, after removing the mobile elements and the MDR regions, shared an extremely high degree of genetic similarity (query coverage 98% and identity 99%) with a typical type 1a IncC plasmid, namely pR148 (JX141473), which was previously recovered from a Aeromonas hydrophila strain in Thailand (Del Castillo et al., 2013). Consistently, based on the presence or absence of orf1832/orf1847, rhs (Rearrangement hotspots)1/rhs2, i1 and i2, which were the key features that distinguish between type 1 and type 2 IncC plasmids (Harmer and Hall, 2015), these two plasmids, pVb0267 and pVb0499, were assigned as type 1a IncC plasmid. pVb0267 and pVb0499, together with the type 1a IncC reference plasmid pR148, were included in subsequent genomic comparison analysis (Figure 2).
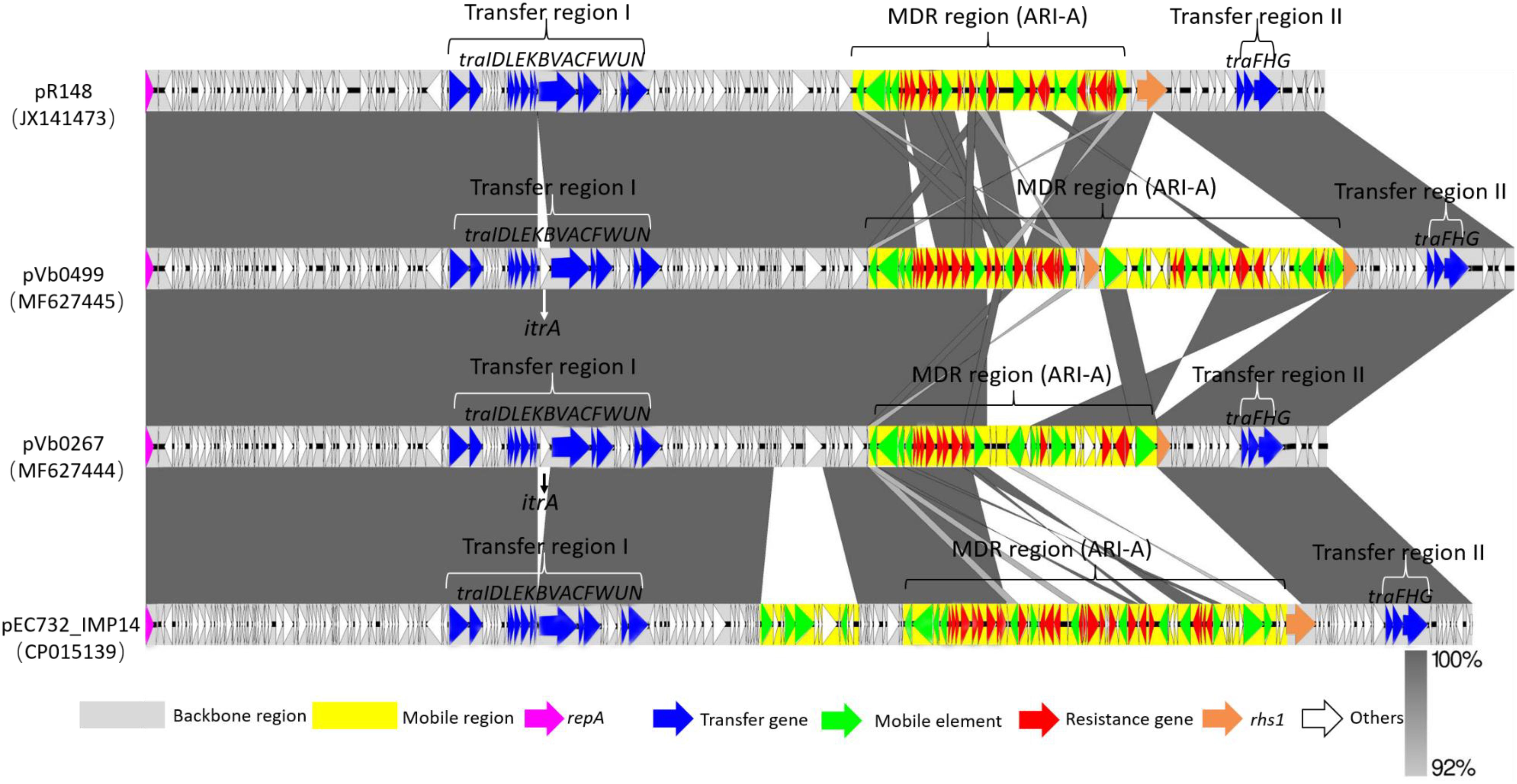
Figure 2. Sequence alignment of type 1a IncC plasmids. Horizontal arrows indicate the location, size and orientation of ORFs. Genes are colored based on their functional classification. Homologous segments generated by a BLASTn comparison are shown as gray blocks that are connected across plasmids. The GenBank accession numbers for each plasmid are as follows: JX141473 (pR148), MF627445 (pVb0499), MF627444 (pVb0267), and CP015139 (pEC732_IMP14).
The linear sequence comparison revealed two major differences in the backbones of pVb0267, pVb0499, and pR148. First, the itrA gene, encoding a Group II intron reverse maturase, was inserted at a site downstream of traA in pVb0267 and pVb0499, but not pR148. It should be noted that itrA is usually located in a group II intron sequence and barely exists alone (Rodríguez-Martínez et al., 2012). The acquisition mechanism of itrA by pVb0267 and pVb0499 was not clear. Second, only the rhs1 gene of pR148 was intact among these three plasmids. A fragment of N-terminus of the rhs1 gene was found deleted in pVb0267, whereas a Tn5403-mediated transposon was inserted at a site within the rhs1 gene in pVb0499. These features were not observable in pR148 (Supplementary Figure S2).
Except for the slight genetic differences in the backbones of these three plasmids, the master type 1a IncC plasmid backbone genes (Zhang et al., 2014) involved in replication (repA), partitioning and stability (parAB, parM, and stbA) and DNA metabolism (ssb–bet–exo, ter–kfrA, int–yacC, nuc, uvrD, and pri), as well as conjugative transfer (tra), were shared by the three plasmids (Supplementary Figure S2). The genes involved in plasmid conjugative transfer (tra) were separated into two regions (transfer region I and transfer region II) by an MDR region in these three plasmids. The transfer region I, upstream of MDR, was a 30 kb region containing 13 genes (traIDLEKBVACFWUN). The remaining three tra genes (traFHG) were located within another 6 kb region, namely transfer region II (Figure 2).
Comparison of the MDR Region in Type 1a IncC Plasmids
Various previous studies have reported that the modular structure of each plasmid was discriminated as type 1a IncC backbone, with insertion of one or more separate accessory modules. These accessory modules were further dissected as resistance islands including the ISEcp1-blaCMY unit, ARI-B (with the sul2 gene), and ARI-A (with a class 1 integron) resistance islands as well as the blaKPC–2 -bearing region, with one or more of these resistance islands inserted at various sites in the backbone of each plasmid (Harmer and Hall, 2014, 2015). Additionally, there appears to be a strong tendency for resistance genes to be incorporated into the rhs1 gene (Harmer and Hall, 2015). In the case of pVb0267 and pVb0499, ARI-A was detected in these two type 1a IncC plasmids. Moreover, some resistance genes were found inserted into the rhs1 gene (Supplementary Table S2).
The structure of the ARI-A region in a typical type 1a IncC plasmid pRMH760 was previously determined and described as being flanked by IS4321/ IS5075 (Sekizuka et al., 2011; Wasyl et al., 2015). A recent study reveals that the ARI-A region in the majority of sequences of type 1a IncC plasmids are located in the same site as that in pRMH760, namely 1711 bp upstream of the rhs1 gene. Hence the extremities of this region are highly conserved (Harmer and Hall, 2015). Based on the presence of IS4321/ IS5075, ARI-A islands were identified in pVb0267 and pVb0499. The subsequent ARI-A sequence analysis by BLAST indicated that a plasmid known as pEC732_IMP14 (CP015139), recovered from Escherichia coli, exhibited the highest degree of genetic similarity (query coverage 49% and identity 99%) with the ARI-A sequence of pVb0499. Complete ARI-A sequence comparison between pVb0267, pVb0499, pR148, and pEC732_IMP14 was then performed (Figure 3).
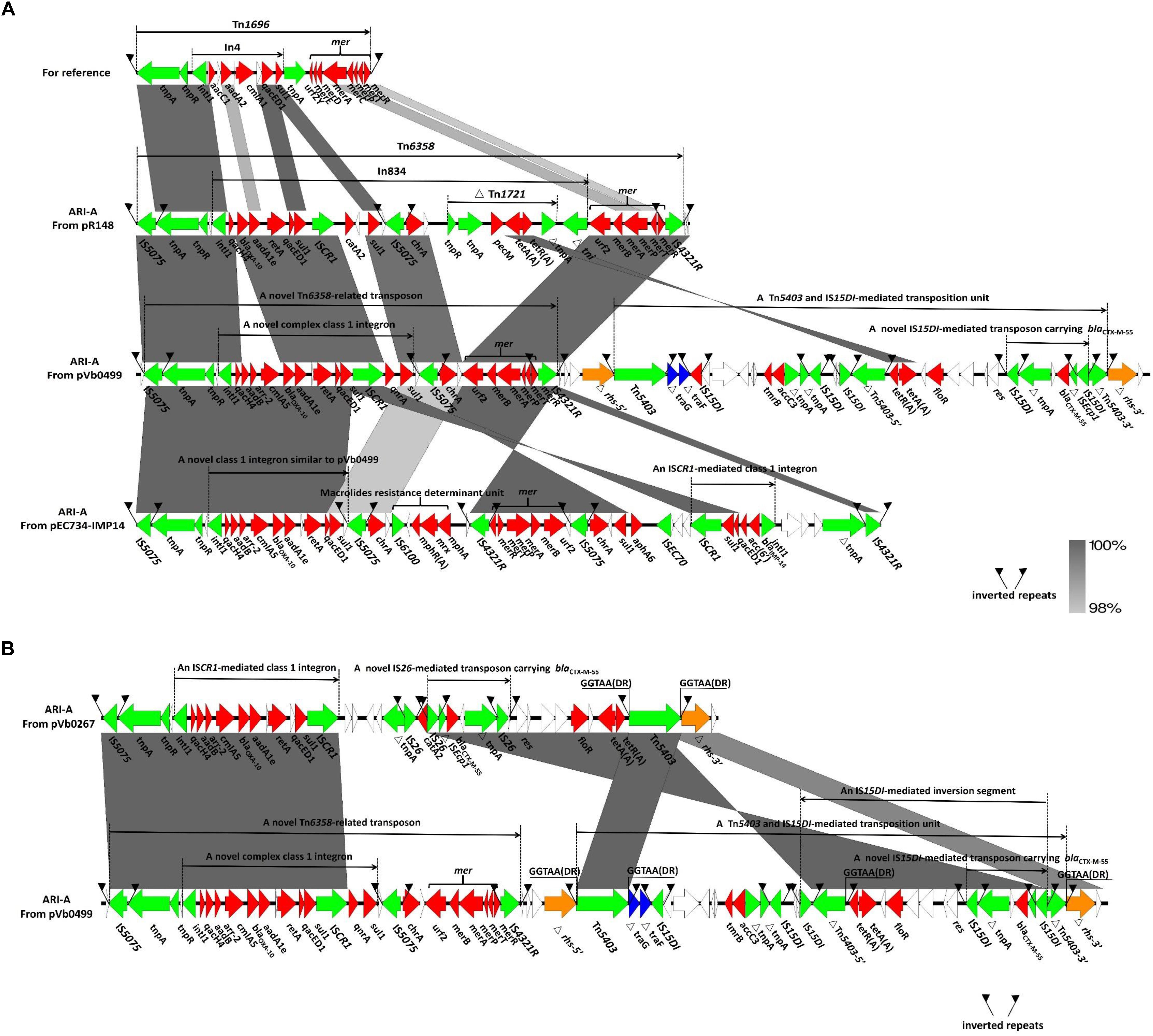
Figure 3. Genetic features of ARI-A islands. (A) Comparison of ARI-A islands between pR148, pVb0499, and pEC734-IMP14. Tn1696 are prototype mobile elements used as a reference for sequence comparison, with an accession number of U12338. (B) Comparison of ARI-A islands between pVb0267 and pVb0499. Direct repeats (DRs) were produced by independent insertion of Tn5403. Genes are denoted by arrows. Genes, mobile elements and other features are colored based on their functional classification. Shading denotes regions of homology (nucleotide identity > 90%).
Comparative analysis of the ARI-A sequences revealed that all the complex transposon units within the ARI-A region of pVb0267, pVb0499, pR148, and pEC732_IMP14 were derivatives of Tn1696, a transposon belonging to the Tn21 subgroup of the Tn3 family (Harmer et al., 2014). Tn1696 was generated from insertion of class 1 integron In4 at a site within the resolution (res) site of a core structure IRL (inverted repeat left)-tnpA (transposase)-tnpR (resolvase)-res-mer (mercury resistance locus)-IRR (inverted repeat right) (Figure 3A) (Partridge et al., 2001). The four Tn1696 derivatives identified in this study differed from the typical Tn1696 primarily by insertion of a distinct integron or integron-associated regions (Figure 3A). Specifically, the Tn1696 derivative in pR148 was designated as Tn6358, in which a complex class 1 integron (In834) harboring two intrinsic resistance regions (the cassette array qacH4-blaOXA–10-aadA1e and catA2) and two additional integrated resistance regions [the chrA region and a truncated Tn1721 carrying the class A tetracycline module tetA(A)-tetR(A) (Kim et al., 2008)], was inserted into the res gene (Allmeier et al., 1992). Compared to In834, a novel complex class 1 integron was observed in pVb0499 (Figure 3A). In the cassette array (qacH4-blaOXA–10-aadA1e), three additional resistance genes (aadB, arr-2, and cmlA5) were found to be located between qacH4 and blaOXA–10, resulting in a new cassette array (qacH4-aadB-arr-2-cmlA5-blaOXA–10-aadA1e). Besides, the catA2 region was replaced by the qnrA gene, which is responsible for quinolones resistance; this novel complex class 1 integron lacked the truncated Tn1721 carrying tetA(A)-tetR(A).
In the ARI-A of pEC732_IMP14, a macrolide resistance determinant unit (IS6100-mphR(A)-mrx-mph(A)) was found embedded at the upstream region of the mer module. Moreover, a class 1 integron harboring the cassette array (blaIMP–14-acc (6′)-qacED1) was found to be located near the right end of ARI-A. However, the qnrA gene was not found in pEC732_IMP14. It should be noted that the orientation of the chrA and mer segments was opposite to each other in pEC732_IMP14 and pVb0499, presumably due to homologous recombination events that occurred in ARI-A. Compared to the ARI-A in pVb0499, the one in pVb0267 has a 11940-bp deletion that spans a region from ISCR1 to IS4321R, a recognized right end region of ARI-A (Figure 3B). In other words, the ARI-A in pVb0267 has lost the qnrA gene, the chrA region, the mer module and IS4321R. The deletion events such as those occurred in pVb0267 were also observed in other type 1 IncC plasmids, which were usually associated with insertion of mobile elements such as IS26 (Lin et al., 1984; Partridge et al., 2011; Letchumanan et al., 2015a).
Besides being located in ARI-A, some resistance genes such as blaCTX–M–55 were also found inserted in the rhs1 gene of both pVb0267 and pVb0499. An rhs gene was first identified as the site that promoted recombination in Escherichia coli (Lin et al., 1984). Replacement of part of the C-terminus of rhs is one of the key features that distinguish between type 1 and type 2 IncC plasmids (Harmer and Hall, 2014). In particular, detailed analysis of the rhs1 region in pVb0267 showed that a deletion of the rhs-5′ region occurred in pVb0267 due to the insertion of mobile elements like Tn5403 and IS26, which also led to the absence of the right end of ARI-A in pVb0267 as described above (Figure 3B). In the case of pVb0499, a novel composite transposon, comprising two complex transposon Tn5403 at both ends, was found in the rhs1 gene, spliting rhs1 into two parts, namely △rhs-5′ and△rhs-3′ (Figure 3B). Analysis of the novel composite transposon revealed that the two Tn5403 elements were flanked by two typical paired 50 bp inverted repeats (IRL and IRR), which were in turn flanked by two 5 bp direct repeats (GGTAA) (DRs; target site duplication signals for transposition), suggesting that this composite transposon was acquired by the pVb0499 backbone via transposition event (Figure 3B). Remarkably, a 18,607 bp highly conserved sequence segment flanked by two copies of IS26 or IS15DI (variant of IS26) was found within the rhs1 region in pVb0267 and pVb0499. Nevertheless, the orientation of the conserved segment was opposite to each other between pVb0267 and pVb0499. Due to the inversion which occurred in pVb0499, the Tn5403 at the right end of the composite transposon was spilted into two parts, namely △Tn5403-5′ and △Tn5403-3′, which might in turn lead to the loss of ability to transpose independently (Figure 3B). Altogether, these evidences suggest that intramolecular homologous recombination mediated by IS26/IS15DI occurred during the process of evolution of the MDR region of plasmids pVb0267 and pVb0499.
Comparison of the Genetic Contexts of blaCTX–M–55 in Vb0267, Vb0499 and Other Gram-Negative Isolates
To obtain a deeper understanding of the transmission and dissemination of blaCTX–M–55 gene in different genetic environments, nine blaCTX–M–55-positive isolates of Enterobacteriaceae identified in recent years, together with Vb0267 and Vb0499, were included in a genomic comparison of the flanking region of blaCTX–M–55. Among these isolates, four were Escherichia coli, four were Klebsiella pneumoniae and one Salmonella enterica. The result of comparison is presented in Figure 4. Generally, all the 11 isolates harbored ISEcp1 in the region upstream of blaCTX–M–55 (in five cases, ISEcp1 was truncated by IS26, and in one case, it was truncated by IS1294), whereas orf477Δ was detected downstream of the blaCTX–M–55 gene from all the strains. The 11 blaCTX–M–55-positive isolates were further divided into five different groups according to the genetic contexts of blaCTX–M–55 gene, [Group I (4 isolates), Group II (3 isolates), Group III (1 isolate), Group IV (1 isolate), and Group V (2 isolate)]. Group I (ISEcp1-blaCTX–M–55-orf477Δ) was the most common. The arrangement of Group II (IS26-ISEcp1Δ-blaCTX–M–55-orf477Δ) and Group III (IS1294-ISEcp1Δ-blaCTX–M–55-orf477Δ) was similar with Group I, although ISEcp1 was disrupted by IS26 in Group II and by IS1294 in Group III. Group IV (ISEcp1-blaCTX–M–55-orf477Δ-tnpAΔ-IS2) and Group V (IS26-ISEcp1-blaCTX–M–55-orf477Δ-tnpAΔ-IS26) both harbored tnpA downstream of orf477Δ, while the tnpA gene was truncated by IS2 in Group IV and by IS26 in Group V.
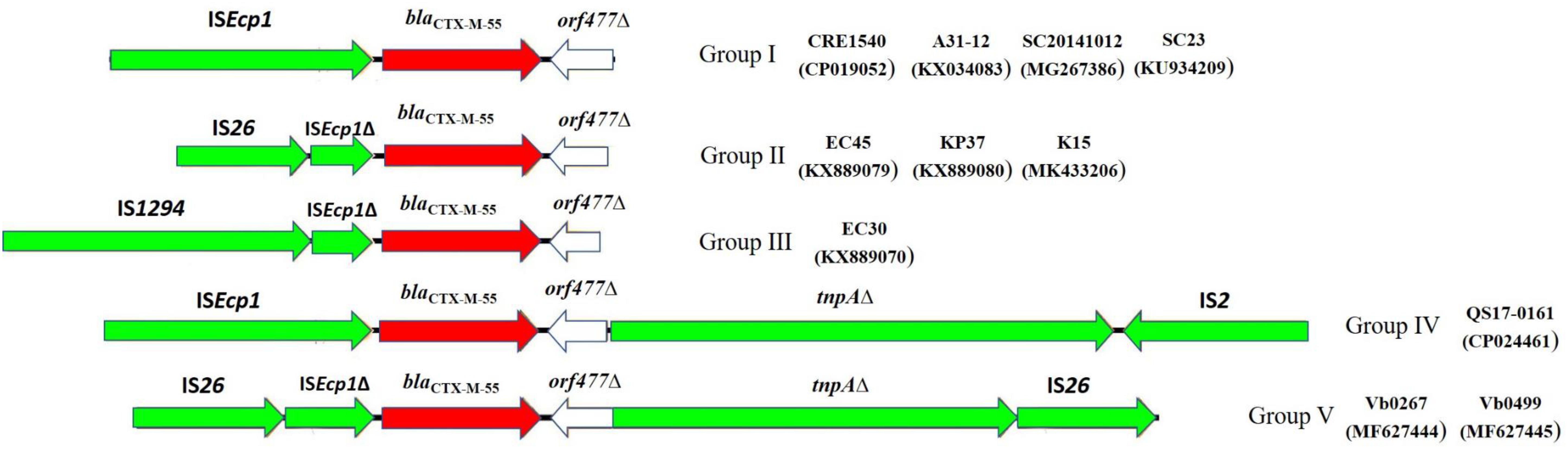
Figure 4. The surrounding regions of blaCTX–M–55 gene in this study. Group I (ISEcp1-blaCTX–M–55-orf477Δ) was found in isolates (A31-12, CRE1540, SC20141012, SC23); Group II (IS26-ISEcp1Δ-blaCTX–M–55-orf477Δ) was found in isolates (EC45, KP37,K15); Group III (IS1294-ISEcp1Δ-blaCTX–M–55-orf477Δ) was found in isolate (EC30); Group IV (ISEcp1-blaCTX–M–55-orf477Δ-tnpAΔ-IS2) was found in isolate (QS17-0161); Group V (IS26-ISEcp1-blaCTX–M–55-orf477Δ -tnpAΔ-IS26) was found in isolates (Vb0267, Vb0499).
DISCUSSION
The continuous and extensive use of antibiotics in the aquaculture industry facilitates the development of various resistant isolates and the dissemination of resistance genes within the bacterial population in the environment (Tendencia and Peña, 2002; Rebouças et al., 2011). All the antibiotics used in this study are recommended in the treatment of infections caused by strains of the Vibrio sp., including tetracycline, cefotaxime, amikacin, gentamicin, and trimethoprim-sulfamethoxazole, some of which, tetracycline and chloramphenicol in particular, are also widely used in aquaculture industry (Shaw et al., 2014; Letchumanan et al., 2015b). In this study, 82.8% of the V. parahaemolyticus isolates recovered from shrimp samples exhibited resistance to ampicillin. Our results are in agreement with other studies that reported resistance to ampicillin among the V. parahaemolyticus isolates recovered from seafood samples (Letchumanan et al., 2015b; Li et al., 2015b). Due to the extensive use of first generation antibiotics including ampicillin, the efficacy of ampicillin for Vibrio treatment has decreased (Letchumanan et al., 2015b).
Resistance to the third generation cephalosporins was observed in our V. parahaemolyticus isolates, with 18% of the test isolates being resistant to cefotaxime and 19% being resistant to ceftriaxone. This rate is much lower than to that reported in other studies on the resistance to third generation cephalosporin among V. parahaemolyticus isolates, with the rate of resistance to cefotaxime typically in the range of 73–80% (Sahilah et al., 2014; Letchumanan et al., 2015b). The discrepancies regarding the resistance phenotype to third generation cephalosporin could possibly due to the difference in test methodology or geographical variation.
pVb0267 and pVb0499 are the first two sequenced blaCTX–M–55-bearing type 1a IncC plasmids recovered from non-clinical isolates in China. Analysis of their structures provides new insight into the plasticity of the genetic context of ARI-A and the role of IS26/IS15DI in mediating homologous recombination events that occurred in rhs region, as well as mobilization of the blaCTX–M–55-containing transposon in pVb0267 and pVb0499. In the rhs resistance region of pVb0267 and pVb0499, a highly conservative fragment harboring blaCTX–M–55 was observed (Figure 3B). The complex fragment is composed of the ISEcp1 element, the blaCTX–M–55 gene, a orf477 truncated element (orf477△) and a truncated tnpA gene. Interestingly, the cassette array was flanked by two IS26 or IS15DI elements in both pVb0267 and pVb0499. Coincidentally, this arrangement is a representative composite transposon structure, suggesting that this cassette array has the potential to undergo horizontal transfer (Kidwell, 2005). However, sequence analysis did not reveal the presence of any distinct direct repeats (DRs). This finding is intriguing. Likewise, the underlying mechanism by which blaCTX–M–55 was acquired by pVb0267 and pVb0499 remains to be determined. To test a possible mechanism of formation of this apparent composite transposon, further sequence alignment by BLASTn against the transposon in pVb0267 was performed. Two similar sequences were found. One was from pA31-12 (KX034083) and the other was from pKPN1481-1 (CP020848). All of the three segments were found to share a highly conservative region (99% identity) of 1819 bp in size, and contain an ISEcp1 element, the blaCTX–M–55 gene and the orf477△ element (Figure 5). Taken together, we hypothesize that at the initial stage of evolution, a copy of IS26 and an ISEcp1-mediated transposon carrying blaCTX–M–55 and orf477△ was inserted into a tnpA gene, resulting in formation of a similar structure found in pKPN1481-1. Another IS26 was embedded in the ISEcp1, which eventually formed in the composite transposon observed in pVb0267. However, the absence of direct repeats may result from subsequent IS26-mediated adjacent deletions or a conservative IS26-mediated cointegrate formation event (Harmer et al., 2014). It is necessary to further assess the mobility of IS26 or its variant (Figure 5).
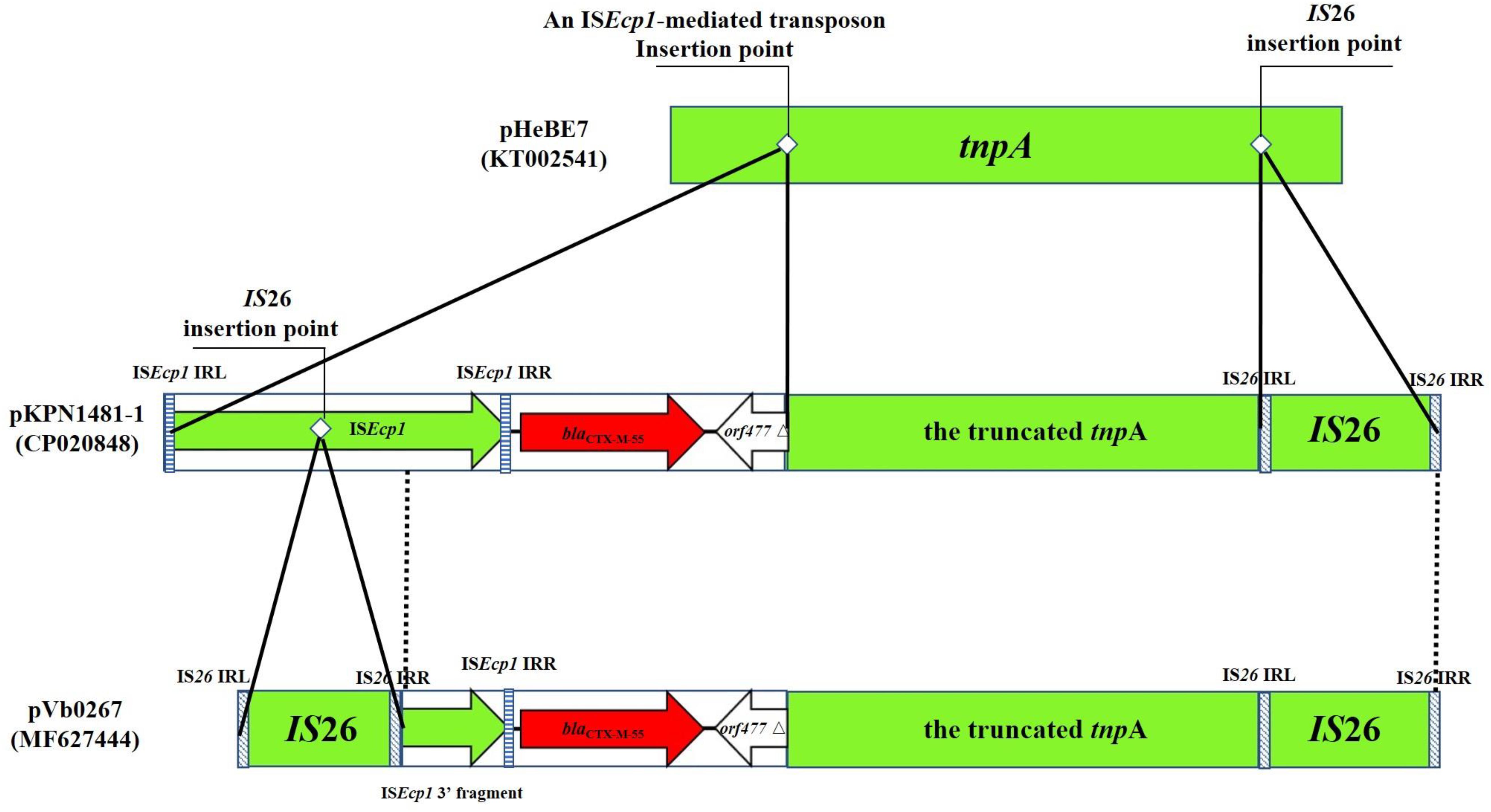
Figure 5. Proposed mechanism of formation of a novel IS26-mediated transposon carrying the blaCTX–M–55 gene. The transposon and insertion sequence (IS) are indicated by rectangles. Genes harbored in an ISEcp1-mediated transposon are denoted by arrows. Dotted vertical lines indicate the region of homology between pKPN1481-1 and pVb0267. Striped rectangles indicate the IRs of IS26 and ISEcp1, respectively. The insertion point of IS26 and an ISEcp1-mediated transposon are indicated by white rhombi. pHeBE7 and pKPN1481-1 are references for sequence comparison, with accession numbers being KT002541 and CP020848, respectively.
More importantly, the blaCTX–M family extended-spectrum β-lactamase-encoding genes have been widely disseminated among cephalosporin resistant clinical strains of Enterobacteriaceae such as those of Escherichia coli and K. pneumoniae (Zhang et al., 2014). In contrast, resistance toward cephalosporins in foodborne pathogenic Vibrio spp. remains relatively rare (Li et al., 2015a). To our knowledge, this is the first report of recovery of blaCTX–M–55 from Vibrio spp. These findings indicated that cephalosporin resistance mediated by the blaCTX–M and other related resistance determinants in Vibrio parahaemolyticus may become a major concern not only due to its growing clinical relevance as a key foodborne pathogen, but also because Vibrio parahaemolyticus may serve as a blaCTX–M–55 reservoir from which the resistance element may be readily transferred to other pathogenic organisms. This view has been confirmed by the finding that pVb0267 and pVb0499 could be successfully transferred to the J53 recipient strain in the present study. If no effective measures are taken, these evolutionary characteristics of ESBL-producing foodborne strains of Vibrio spp. may complicate future management of antibiotic resistance in China. Further investigations are required to confirm if these plasmids are responsible for causing an increasing incidence of carriage of blaCTX–M–55 or other resistance elements in strains of the Vibrio spp. in China and other countries. This study also indicated that the increasing evolution of conjugative plasmids carrying different antimicrobial resistance gene could be attributed to bacterial adaption to aquaculture environment with increasing amounts of antibiotics.
Data Availability
The datasets generated for this study can be found in Genbank, MF627444 and MF627445.
Author Contributions
All authors listed have made a substantial, direct and intellectual contribution to the work, and approved it for publication.
Funding
This work was supported by the Collaborative Research Fund of Hong Kong Research Grant Council (C5026-16G) and the Health and Medical Research Fund (14130402 and 15141322).
Conflict of Interest Statement
The authors declare that the research was conducted in the absence of any commercial or financial relationships that could be construed as a potential conflict of interest.
Supplementary Material
The Supplementary Material for this article can be found online at: https://www.frontiersin.org/articles/10.3389/fmicb.2019.01338/full#supplementary-material
References
Allmeier, H., Cresnar, B., Greck, M., and Schmitt, R. (1992). Complete nucleotide sequence of Tn1721: gene organization and a novel gene product with features of a chemotaxis protein. Gene 111, 11–20. doi: 10.1016/0378-1119(92)90597-i
Ambrose, S. J., Harmer, C. J., and Hall, R. M. (2018). Compatibility and entry exclusion of IncA and IncC plasmids revisited: IncA and IncC plasmids are compatible. Plasmid 9:7. doi: 10.1016/j.plasmid.2018.02.002
Bondad-Reantaso, M. G., Subasinghe, R. P., Arthur, J. R., Ogawa, K., and Chinabut, S. (2005). Disease and health management in Asian aquaculture. Vet. Parasitol. 132, 249–272. doi: 10.1016/j.vetpar.2005.07.005
Briet, A., Helsens, N., Delannoy, S., Debuiche, S., Brisabois, A., Midelet, G., et al. (2018). NDM-1-producing Vibrio parahaemolyticus isolated from imported seafood. J. Antimicrob. Chemother. 73, 2578–2579. doi: 10.1093/jac/dky200
Broberg, C. A., Calder, T. J., and Orth, K. (2011). Vibrio parahaemolyticus cell biology and pathogenicity determinants. Microb. Infect. 13, 992–1001. doi: 10.1016/j.micinf.2011.06.013
Chen, Y., Guo, Y., Wang, Z., Liu, X., Liu, H., Dai, Y., et al. (2010). [Foodborne disease outbreaks in 2006 report of the National Foodborne Disease Surveillance Network. China]. J. Hygiene Res. 39, 331–334.
Couturier, M., Bex, F., Bergquist, P. L., and Maas, W. K. (1988). Identification and classification of bacterial plasmids. Microbiol. Rev. 52, 375–395.
Dahmen, S., Madec, J. Y., and Haenni, M. (2013). F2:A-:B- plasmid carrying the extended-spectrum β-lactamase bla(CTX-M-55/57) gene in Proteus mirabilis isolated from a primate. Int. J. Antimicrob. Agents 41, 594–595. doi: 10.1016/j.ijantimicag.2013.02.004
Dallenne, C., Costa, A. D., Decré, D., Favier, C., and Arlet, G. (2010). Development of a set of multiplex PCR assays for the detection of genes encoding important β-lactamases in Enterobacteriaceae. J. Antimicrob. Chemother. 65, 490–495. doi: 10.1093/jac/dkp498
Del Castillo, C. S., Hikima, J., Jang, H. B., Nho, S. W., Jung, T. S., Wongtavatchai, J., et al. (2013). Comparative sequence analysis of a multidrug-resistant plasmid from Aeromonas hydrophila. Antimicrob. Agents Chemother. 57, 120–129. doi: 10.1128/AAC.01239-12
Fricke, W. F., Welch, T. J., Mcdermott, P. F., Mammel, M. K., Leclerc, J. E., White, D. G., et al. (2009). Comparative genomics of the IncA/C multidrug resistance plasmid family. J. Bacteriol. 191, 4750–4757. doi: 10.1128/JB.00189-09
Harmer, C. J., and Hall, R. M. (2014). pRMH760, a precursor of A/C2 plasmids carrying blaCMY and blaNDM genes. Microb. Drug Resist. 20, 416–423. doi: 10.1089/mdr.2014.0012
Harmer, C. J., and Hall, R. M. (2015). The A to Z of A/C plasmids. Plasmid 80, 63–82. doi: 10.1016/j.plasmid.2015.04.003
Harmer, C. J., Moran, R. A., and Hall, R. M. (2014). Movement of IS26-associated antibiotic resistance genes occurs via a translocatable unit that includes a single IS26 and preferentially inserts adjacent to another IS26. Mbio 5, 01801–1814. doi: 10.1128/mBio.01801-14
Hedges, R. W. (1974). R factors from providence. J. General Microbiol. 81, 171–181. doi: 10.1099/00221287-81-1-171
Hui, W., Ping, G., Hongli, S., He, W., Qiwen, Y., Minjun, C., et al. (2007). Molecular epidemiology of clinical isolates of carbapenem-resistant Acinetobacter spp. from Chinese hospitals. Antimicrob. Agents Chemother. 51:4022. doi: 10.1128/aac.01259-06
Jorgensen, J. H. (2015). Methods for Antimicrobial Dilution and Disk Susceptibility Testing of Infrequently Isolated or Fastidious Bacteria; Approved Guideline. Wayne, PA: Clinical and Laboratory Standards Institute.
Kim, H. J., Ryu, J. O., Lee, S. Y., Kim, E. S., and Kim, H. Y. (2015). Multiplex PCR for detection of the Vibrio genus and five pathogenic Vibrio species with primer sets designed using comparative genomics. BMC Microbiol. 15:239. doi: 10.1186/s12866-015-0577-3
Kim, M. J., Hirono, I., Kurokawa, K., Maki, T., Hawke, J., Kondo, H., et al. (2008). Complete DNA sequence and analysis of the transferable multiple-drug resistance plasmids (R Plasmids) from Photobacterium damselae subsp. piscicida isolates collected in Japan and the United States. Antimicrob. Agents Chemother. 52, 606–611. doi: 10.1128/aac.01216-07
Kiratisin, P., Apisarnthanarak, A., Saifon, P., Laesripa, C., Kitphati, R., and Mundy, L. M. (2007). The emergence of a novel ceftazidime-resistant CTX-M extended-spectrum β-lactamase. CTX-M-55, in both community-onset and hospital-acquired infections in Thailand⋆. Diagn. Microbiol. Infect. Dis. 58, 349–355. doi: 10.1016/j.diagmicrobio.2007.02.005
Letchumanan, V., Pusparajah, P., Tan, T. H., Yin, W. F., Lee, L. H., and Chan, K. G. (2015a). Occurrence and antibiotic resistance of Vibrio parahaemolyticusfrom shellfish in selangor, Malaysia. Front. Microbiol. 6:1417. doi: 10.3389/fmicb.2015.01417
Letchumanan, V., Yin, W. F., Lee, L. H., and Chan, K. G. (2015b). Prevalence and antimicrobial susceptibility of Vibrio parahaemolyticus isolated from retail shrimps in Malaysia. Front. Microbiol. 6:33. doi: 10.3389/fmicb.2015.00033
Li, R., Lin, D., Chen, K., Wong, M. H., and Chen, S. (2015a). First detection of AmpC β-lactamase bla(CMY-2) on a conjugative IncA/C plasmid in a Vibrio parahaemolyticus isolate of food origin. Antimicrob. Agents Chemother. 59, 4106–4111. doi: 10.1128/AAC.05008-14
Li, R., Wong, M. H. Y., Zhou, Y., Chan, E. W., and Chen, S. (2015b). Complete nucleotide sequence of a conjugative plasmid carrying blaPER-1. Antimicrob. Agents Chemother. 59, 3582–3584. doi: 10.1128/aac.00518-15
Li, R., Xie, M., Dong, N., Lin, D., Yang, X., Wong, M. H. Y., et al. (2018). Efficient generation of complete sequences of MDR-encoding plasmids by rapid assembly of MinION barcoding sequencing data. Gigascience 7, 1–9. doi: 10.1093/gigascience/gix132
Li, R., Ye, L., Zheng, Z., Chan, E. W., and Chen, S. (2016). Genetic Characterization of a blaVEB-2-carrying plasmid in Vibrio parahaemolyticus. Antimicrob. Agents Chemother. 60, 6965–6968. doi: 10.1128/AAC.01749-16
Lin, R. J., Capage, M., and Hill, C. W. (1984). A repetitive DNA sequence, rhs, responsible for duplications within the Escherichia coli K-12 chromosome. J. Mol. Biol. 177, 1–18. doi: 10.1016/0022-2836(84)90054-8
Lüdeke, C. H., Gonzalez-Escalona, N., Fischer, M., and Jones, J. L. (2015). Examination of clinical and environmental Vibrio parahaemolyticus isolates by multi-locus sequence typing (MLST) and multiple-locus variable-number tandem-repeat analysis (MLVA). Front. Microbiol. 6:564. doi: 10.3389/fmicb.2015.00564
Naseer, U., and Sundsfjord, A. (2011). The CTX-M conundrum: dissemination of plasmids and Escherichia coli clones. Microb. Drug Resist. 17, 83–97. doi: 10.1089/mdr.2010.0132
Nishibuchi, M., and Kaper, J. B. (1985). Thermostable direct hemolysin gene of Vibrio parahaemolyticus. J. Bacteriol. 162, 558–564.
Okamoto, A. S., Andreatti Filho, R. L., Rocha, T. S., Menconi, A., and Mariettogonçalves, G. A. (2009). Detection and transfer of antimicrobial resistance gene integron in Salmonella Enteritidis derived from avian material. Revista Brasileira De Ciência Avícola 11, 195–201. doi: 10.1590/s1516-635x2009000300009
Partridge, S., Brown, H., Stokes, H., and Hall, R. (2001). Transposons Tn1696 and Tn21 and their integrons In4 and In2 have independent origins. Antimicrob. Agents Chemother. 45:1263. doi: 10.1128/aac.45.4.1263-1270.2001
Partridge, S. R., Zong, Z., and Iredell, J. R. (2011). Recombination in IS26 and Tn2 in the evolution of multiresistance regions carrying blaCTX-M-15 on conjugative IncF plasmids from Escherichia coli. Antimicrob. Agents Chemother. 55, 4971–4978. doi: 10.1128/AAC.00025-11
Peng, F. M., Jiang, D. Y., and Hong-Hai, R. (2010). Pathogenic investigation on a food poisoning induced by Vibrio Parahaemolyticus. Prevent. Med. Tribune. 16, 746–747.
Pinto, A. D., Terio, V., Novello, L., and Tantillo, G. (2011). Comparison between thiosulphate-citrate-bile salt sucrose (TCBS) agar and CHROMagar Vibrio for isolating Vibrio parahaemolyticus. Food Control 22, 124–127. doi: 10.1016/j.foodcont.2010.06.013
Rebouças, R. H., de Sousa, O. V., Lima, A. S., Vasconcelos, F. R., de Carvalho, P. B., and Vieira, R. H. (2011). Antimicrobial resistance profile of Vibrio species isolated from marine shrimp farming environments (Litopenaeus vannamei) at Ceará. Brazil. Environ. Res. 111, 21–24. doi: 10.1016/j.envres.2010.09.012
Rodríguez-Martínez, J. M., Nordmann, P., and Poirel, L. (2012). Group IIC intron with an unusual target of integration in Enterobacter cloacae. J. Bacteriol. 194, 150–160. doi: 10.1128/JB.05786-11
Sahilah, A. M., Laila, R. A. S., Sallehuddin, H. M., Osman, H., Aminah, A., and Azuhairi, A. A. (2014). Antibiotic resistance and molecular typing among cockle (Anadara granosa) strains of Vibrio parahaemolyticus by polymerase chain reaction (PCR)-based analysis. World J. Microbiol. Biotechnol. 30, 649–659. doi: 10.1007/s11274-013-1494-y
Sekizuka, T., Matsui, M., Yamane, K., Takeuchi, F., Ohnishi, M., Hishinuma, A., et al. (2011). Complete sequencing of the blaNDM-1-positive IncA/C plasmid from Escherichia coli ST38 isolate suggests a possible origin from plant pathogens. PLoS One 6:e25334. doi: 10.1371/journal.pone.0025334.
Shaw, K. S., Goldstein, R. E. R., He, X., Jacobs, J. M., Crump, B. C., and Sapkota, A. R. (2014). Antimicrobial susceptibility of Vibrio vulnificus and Vibrio parahaemolyticus recovered from recreational and commercial areas of chesapeake bay and maryland coastal bays. PLoS One 9:e89616. doi: 10.1371/journal.pone.0089616
Sjölund-Karlsson, M., Howie, R., Krueger, A., Rickert, R., Pecic, G., Lupoli, K., et al. (2011). CTX-M–producing non-typhi Salmonella spp. isolated from humans, United States. Emerg. Infect. Dis. 17, 97–99. doi: 10.3201/eid1701.100511
Tendencia, E. A., and Peña, L. D. D. (2002). Level and percentage recovery of resistance to oxytetracycline and oxolinic acid of bacteria from shrimp ponds. Aquaculture 213, 1–13. doi: 10.1016/s0044-8486(02)00017-0
Tenover, F. C., Arbeit, R. D., Goering, R. V., Mickelsen, P. A., Murray, B. E., Persing, D. H., et al. (1995). Interpreting chromosomal DNA restriction patterns produced by pulsed-field gel electrophoresis: criteria for bacterial strain typing. J. Clin. Microbiol. 33, 2233–2239.
Wasyl, D., Kern-Zdanowicz, I., Domaå Ska-Blicharz, K., Zajä, C. M., and Hoszowski, A. (2015). High-level fluoroquinolone resistant Salmonella enterica serovar Kentucky ST198 epidemic clone with IncA/C conjugative plasmid carrying bla(CTX-M-25) gene. Vet. Microbiol. 175, 85–91. doi: 10.1016/j.vetmic.2014.10.014
Wick, R. R., Judd, L. M., Gorrie, C. L., and Holt, K. E. (2017). Unicycler: resolving bacterial genome assemblies from short and long sequencing reads. PLoS Computat. Biol. 13:e1005595. doi: 10.1371/journal.pcbi.1005595
Yano, Y., Hamano, K., Satomi, M., Tsutsui, I., Ban, M., and Aue-Umneoy, D. (2014). Prevalence and antimicrobial susceptibility of Vibrio species related to food safety isolated from shrimp cultured at inland ponds in Thailand. Food Control 38, 30–36. doi: 10.1016/j.foodcont.2013.09.019
Zhang, J., Zheng, B., Zhao, L., Wei, Z., Ji, J., Li, L., et al. (2014). Nationwide high prevalence of CTX-M and an increase of CTX-M-55 in Escherichia coli isolated from patients with community-onset infections in Chinese county hospitals. BMC Infect. Dis. 14:659. doi: 10.1186/s12879-014-0659-0
Zhang, X. H., and Austin, B. (2005). Haemolysins in Vibrio species. J. Appl. Microbiol. 98, 1011–1019. doi: 10.1111/j.1365-2672.2005.02583.x
Keywords: blaCTX-M-55, V. parahaemolyticus, conjugative plasmid, cephalosporin resistance, IncC plasmid
Citation: Zheng Z, Li R, Ye L, Wai-chi Chan E, Xia X and Chen S (2019) Genetic Characterization of blaCTX–M–55 -Bearing Plasmids Harbored by Food-Borne Cephalosporin-Resistant Vibrio parahaemolyticus Strains in China. Front. Microbiol. 10:1338. doi: 10.3389/fmicb.2019.01338
Received: 14 February 2019; Accepted: 29 May 2019;
Published: 18 June 2019.
Edited by:
Swapan Banerjee, Health Canada, CanadaReviewed by:
Learn-Han Lee, Monash University Malaysia, MalaysiaMasaki Shintani, Shizuoka University, Japan
Copyright © 2019 Zheng, Li, Ye, Wai-chi Chan, Xia and Chen. This is an open-access article distributed under the terms of the Creative Commons Attribution License (CC BY). The use, distribution or reproduction in other forums is permitted, provided the original author(s) and the copyright owner(s) are credited and that the original publication in this journal is cited, in accordance with accepted academic practice. No use, distribution or reproduction is permitted which does not comply with these terms.
*Correspondence: Xiaodong Xia, foodscixiaodong@yahoo.com; Sheng Chen, sheng.chen@polyu.edu.hk