- 1Christian Doppler Laboratory for Monitoring of Microbial Contaminants, Department of Farm Animal and Public Health in Veterinary Medicine, Institute for Milk Hygiene, Milk Technology and Food Science, University of Veterinary Medicine, Vienna, Austria
- 2Department of Farm Animal and Public Health in Veterinary Medicine, Institute of Milk Hygiene, Milk Technology and Food Science, University of Veterinary Medicine, Vienna, Austria
Many bacteria enter the viable but non-culturable (VBNC) state to maximize resources and increase their tolerance to harmful conditions to cope with environmental stress, which has been described for a plethora of important human and foodborne pathogens. VBNC pathogens can potentially present a serious risk to human health as they are invisible to routine microbiological culture-based methods. Of high importance is the increased tolerance to antibiotics or disinfectant measures while in the VBNC state. The greatest remaining challenge for such investigations is the lack of an appropriate, cost-effective multi-species screening method due to experimental constraints. In this study, we investigated if de novo ATP production of cells in the VBNC state is a suitable indicator for overall cell viability that can be utilized to determine the minimum ATP inhibitory concentration (MAIC) of antibiotics and other antimicrobials. To validate this approach, heat-stress time-kill experiments were performed with both culturable and VBNC cells. We developed a comprehensive experimental setup and demonstrated the applicability of this VBNC–MIC assay for testing the tolerance of 12 strains of 4 important bacterial species (Escherichia coli, Bacillus cereus, Pseudomonas aeruginosa, and Listeria monocytogenes) in the VBNC state to eight important antimicrobials including four different antibiotics. We confirmed that bacteria in the VBNC state were resistant to all tested antibiotics (ampicillin, imipenem, ciprofloxacin, and gentamicin) and additionally insensitive to disinfectants (benzalkonium chloride and trioctylmethylammonium chloride) and preservatives (bronopol and sodium azide). These data emphasize the need for further research regarding the characteristics of bacterial pathogens in the VBNC state and present the advantages and high-throughput capabilities of ATP determinations to investigate tolerance of VBNC pathogens to antimicrobials. The presented method should be helpful in order to identify appropriate countermeasures, treatments, or disinfectants when confronted with bacterial pathogens in the VBNC state.
Introduction
Bacteria are exposed to environments with constantly changing conditions. To cope with these environmental stresses, many bacteria enter a viable but non-culturable (VBNC) state that results in higher tolerances to harmful conditions (Oliver, 2010; Zhang et al., 2015; Lee and Bae, 2017). The VBNC state was already discovered in 1982 by Xu et al. but has been a controversial issue and along the years it was accepted as a survival strategy of bacteria and recently has become an important part in microbial research (Pinto et al., 2015; Ayrapetyan et al., 2018). The cost of this transition is that VBNC cells lose their ability to grow on routine media, although they retain intact membranes, undamaged genetic material, and metabolic activity and can potentially revert to the active state (Oliver, 2005; Lin et al., 2017). VBNC cells form during exposure to unfavorable environmental conditions such as incompatible temperatures, pH, and starvation or by exposure to noxious chemicals (Li et al., 2014; Orruño et al., 2017). Food preservatives, cleaners, disinfectants, or chlorine are examples of substances inducing the VBNC state (Rowan, 2004; Zhang et al., 2015). However, research has shown that large populations of VBNC cells also exist in unstressed environments, which appear to be a stochastic physiological transformation to protect a subpopulation of cells from unexpected stresses (Ayrapetyan et al., 2015; Gonçalves and de Carvalho, 2016). It has also been demonstrated that VBNC bacteria are able to resist a wide range of unfavorable conditions including starvation, temperature shifts, high or low pH and salinity, oxidative stress, cleaners, antimicrobial compounds, and antibiotics (Nowakowska and Oliver, 2013; Li et al., 2014; Zhao et al., 2017; Ayrapetyan et al., 2018; Robben et al., 2018). In addition, the accumulation of several non-toxic stresses has been shown to switch pathogens into the VBNC state (Overney et al., 2017). The VBNC state of pathogens therefore has relevance to human and animal health.
Emerging bacterial resistance to antibiotics has become a global environmental problem. In contrast to culturable cells, where antibiotic resistance involves specific resistance genes, resistance of VBNC cells is essentially believed to result from a non-specific stress reaction that slows metabolic activity and thereby minimizes the consequences of otherwise unimpaired antibiotic binding (Lewis, 2006; Lin et al., 2017). Research has also shown that efflux genes are upregulated in VBNC bacteria and this contributes actively to antibiotic defense (Harms et al., 2016; Pu et al., 2016; Sikri et al., 2018).
Antimicrobials are included in the range of substances that have been reported to induce the VBNC state. For example, foods treated with antimicrobials have been found generally to harbor a higher number of VBNC cells (Anvarian et al., 2016; Zhao et al., 2017). Nevertheless, published research regarding the persistence of VBNC bacteria in the presence of antimicrobials is limited. Nowakowska and Oliver (2013) showed that VBNC cells of Vibrio vulnificus are protected against a wide variety of stresses by resuscitating the VBNC cells following stress removal. However, from over 100 species known to enter the VBNC state, only a small number of pathogens have been successfully resuscitated (Ayrapetyan et al., 2018). To analyze VBNC bacteria that are not able to resuscitate, Lin et al. (2017) used flow cytometry to analyze the antibiotic resistance of a non-resuscitable VBNC Escherichia coli strain. In contrast, in culturable bacteria, antimicrobial susceptibility and resistance are generally measured by the minimum inhibitory concentration (MIC), which is the lowest concentration of an antimicrobial that prevents visible growth of a microorganism (Andrews, 2001; Li et al., 2017). Consequently, there is a need for a cost- and time-effective analysis method to investigate in more detail the tolerance of VBNC cells to antimicrobials, which is neither dependent upon bacterial resuscitation nor culture. Since all VBNC bacteria have in common the need to remain metabolically active, we selected ATP generation of VBNC cells as an indicator for vitality during and after experimental stress exposure. After confirmation of the VBNC state by investigating metabolic activity and membrane integrity, we therefore exposed VBNC pathogens to serial dilutions of antibiotics, disinfectants, and preservatives, comparable to MIC testing for culturable cells, and determined the VBNC–MIC by investigating the de novo ATP generation of those cells during stress exposure and compared the outcome to culturable cells.
This approach permits us to investigate the VBNC state of pathogens, such as Listeria monocytogenes, that have been shown to resuscitate only under very specific conditions, without the need for novel technology. With this approach we established a protocol to determine the MIC of antibiotics and antimicrobials on VBNC cells and thereby their susceptibilities, by using the BacTiter-GloTM Microbial Cell Viability Assay.
Materials and Methods
Bacterial Strains and Culture Conditions
This study employed four strains of L. monocytogenes: 3253 (sequence type-ST121) (Schmitz-Esser et al., 2015), EGDe (ST35), QC1 (ST403), and ScottA (ST290); three strains of E. coli: BW25113, JW5503, and ATCC25922; three strains of Pseudomonas aeruginosa: ATCC10145, K71, and K73; and two strains of Bacillus cereus: ATCC11778 and KSS. Bacterial strains are part of the collection of bacterial strains at the Institute of Milk Hygiene, Milk Technology and Food Science, University of Veterinary Medicine, Vienna, Austria.
Overnight cultures were prepared by selecting a single colony of the respective strain and growing it in a 9 ml of fresh brain heart infusion (BHI) broth supplemented with yeast extract in a shaking incubator. L. monocytogenes, E. coli, and B. cereus strains were incubated at 37°C while Pseudomonas strains were incubated at 30°C.
Minimum Inhibitory Concentration (MIC) Assessment of Culturable Cells
Minimum inhibitory concentrations of the respective antimicrobial compounds were calculated by applying the serial twofold dilution microtiter plate method in BHI medium. In order to create a constant cell status for each experiment, 1 ml aliquots of the respective overnight cultures were transferred into 9 ml of fresh BHI medium and incubated until it reached an OD600 of 0.6. This ensured that cells were in a logarithmic growth phase. Subsequently, each well, which contained a serially diluted antimicrobial (dilution 1:2), was inoculated with 5 × 105 CFU/ml of the respective bacterial cells. After bacterial inoculation, the 96-well microtiter plates (Corning B.V. Life Sciences, Amsterdam, Netherlands) were measured at 610 nm wavelength in a TECAN F100 microplate reader (Tecan Austria GmbH, Groeding, Austria) to monitor any possible interference by the compounds at the given wavelength. The microtiter plates were then incubated for 24 h at 30°C for Pseudomonas strains and at 37°C for all other strains. Afterward bacterial growth was assessed by measuring absorbance at 610 nm. The MIC was defined as the lowest concentration of the antimicrobial where no bacterial growth could be measured after 24 h. Results are displayed as mean MIC, including upper and lower limits, of at least three experiments performed on different days.
Induction and Confirmation of the VBNC State
Induction and confirmation of the VBNC state was performed according to Robben et al. (2018). One milliliter of early log phase bacteria (OD600: 0.6) was centrifuged for 5 min at 8,000 ×g. The pellet was resuspended in 1 ml of the respective treatment conditions (Table 1) and incubated for 1 h at room temperature (RT) (Robben et al., 2018). After incubation, samples were centrifuged for 5 min at 8,000 ×g and pellets washed with 1 ml of 1× phosphate buffer solution (PBS). Cells were resuspended in 1 ml BHI medium and maintained at their respective incubation temperature.
To confirm VBNC induction, culturability and ATP production were tested directly and 24 h after resuspending cells in fresh BHI medium (Figure 1). Corresponding to Robben et al. (2018) we set the threshold of 105 RLU for de novo ATP production within 24 h, which is an empirical value derived from the previous publication, to make sure that sufficient cells entered the VBNC cells with regard to further experiments. Testing culturability after 24 h also served as a control in order to exclude possible regrowth of remaining culturable cells in the respective samples. Culturability testing was performed by plating 10 μl of the culture onto a tryptone soya agar (TSA) plate and overnight incubation at 37°C.
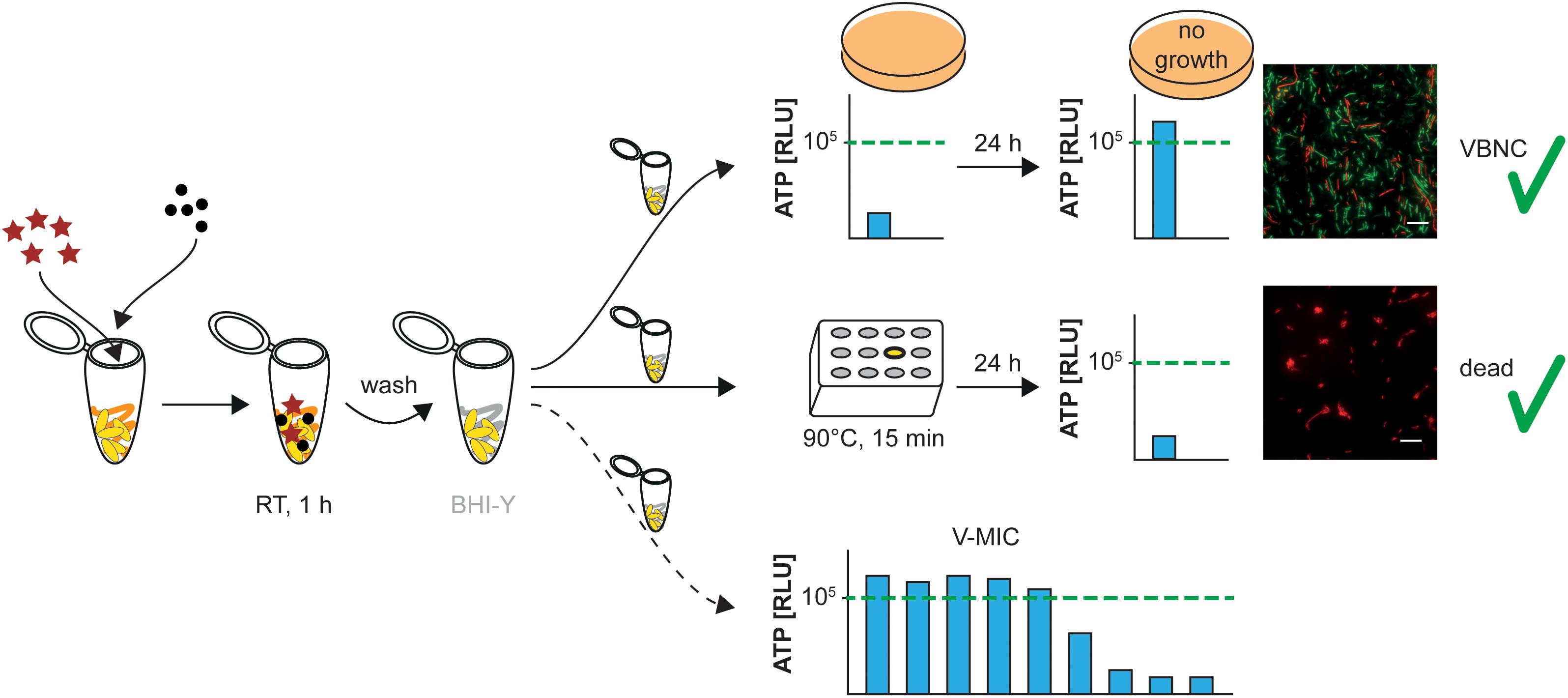
Figure 1. Workflow of VBNC–MIC assay. VBNC state is induced by a specific combination of detergent (stars) and salt (dots); after induction, cells are washed and resuspended in fresh BHI medium; positive control: untreated VBNC cells confirmed by ATP measurements and BacLight viability assay; negative control: VBNC cells killed at 90°C for 15 min; MAIC of antimicrobials is determined by measuring de novo ATP production of VBNC cells exposed to the respective antimicrobial, viability marker: 105 RLU threshold. Scale bar: 50 μm.
Metabolic Activity of Non-culturable Bacteria
To investigate the metabolic activity of non-culturable cells, ATP was quantified using the BacTiter-GloTM Microbial Cell Viability Assay (Promega, Madison, WI, United States). Therefore, 100 μl of bacterial culture was mixed with 100 μl luciferase reagent and incubated for 10 min at RT in the dark. Luminescence was recorded in relative light units (RLUs) using the Tecan F100 microplate reader (Männedorf, Switzerland). BHI medium was used as negative control.
Establishing the Experimental Setup to Determine the Minimum ATP Inhibitory Concentration (MAIC)
To determine the minimum ATP inhibitory concentration (MAIC), respective VBNC induction conditions for each bacterial species had to be determined by preceding screening (Robben et al., 2018). In principle, the respective bacterial strains (L. monocytogenes EGDe, ScottA, QC1, and MFPT3; Escherichia coli BW, ATCC, and JW; B. cereus KSS and ATCC; Pseudomonas aeruginosa ATCC, K71, and K73) were exposed to different combinations of non-ionic detergents and inorganic salts for 1 h (Table 1). After incubation, a successful induction of the VBNC state was determined as follows. Cells were confirmed to have entered the VBNC state if they: (i) lost their ability to grow on non-selective agar plates, (ii) could produce de novo ATP over a level of 105 RLU within 24 h without regaining their culturability, but (iii) could be killed by incubation at 90°C for 15 min (Figure 1).
In addition to the preceding screening, the optimal induction conditions for each strain and the subsequent incubation conditions for optimal de novo ATP generation had to be evaluated for each strain individually. For this purpose, three different cell concentrations of each strain (OD600 of 0.2, 0.4, and 0.6) as well as three different incubation temperatures (RT, 30, and 37°C) were tested. To exclude ATP production by regrown cells or resuscitation, 10 μl of each individual sample was plated onto TSA plates. All tests were done in triplicates. Conditions leading to the maximum de novo ATP production were used for further experiments.
Temperature-Dependent Time-Kill Experiment to Evaluate de novo ATP Production as Viability Indicator
After setting the optimal framework conditions, a series of temperature-dependent time-kill experiments were conducted to evaluate de novo ATP production as an indicator of overall bactericidal effect of a defined stress. In these experiments, the CFU/ml of a L. monocytogenes EGDe culture was measured over a period of 180 min when exposed to three different incubation temperatures (50, 60, and 70°C). The plate count results of the culturable cells were compared to the de novo ATP production of VBNC cells after 24 h incubation in BHI medium. This was done in triplicates.
Assessment of the Minimum ATP Inhibitory Concentration of VBNC Cells (VBNC–MIC)
Before determination of the MAIC, the general induction procedure was performed as described above. After induction, the cells were pelleted via centrifugation (5 min, 8,000 ×g) and washed once with PBS to remove the induction solution, before resuspending the bacterial pellet in fresh BHI medium (final concentration: ∼5 × 108 cells/ml). In order to make sure that the cells are really in the VBNC state, a part of the VBNC suspension was aliquoted into a separate vial and subsequently heated at 90°C for 15 min to kill all cells reliably to serve as a (negative) control. Subsequently, 10 μl of each cell suspension was plated onto TSA plates, to check for remaining culturable cells or resuscitation, and the initial ATP concentration in each suspension was measured to determine the background level released from suspended cells (Figure 1).
Again, to determine the MAICs of the respective antimicrobial compounds, the serial twofold dilution was applied in a 96-well microtiter plate with BHI medium. Subsequently a 100 μl aliquot of the VBNC cell suspension was added to the microtiter plate to give the final desired concentration. The suspensions were then incubated at 37°C for 24 h, after which the ATP concentration was determined. The MAIC was identified by the threshold whereby de novo ATP production with increasing antimicrobial concentration dropped below 105 RLU.
LIVE/DEADTM BacLightTM Bacterial Viability Fluorescence Microscopy
To investigate if cell membranes of non-culturable bacteria remained intact after exposure to the respective antimicrobial treatment, we used the LIVE/DEADTM BacLightTM bacterial viability kit (Molecular Probes, Eugene, OR, United States). Therefore, 100 μl of the bacterial sample was removed from the respective wells, centrifuged at 8,000 ×g for 5 min, washed with PBS twice, and diluted 1:100. Then 1.5 μl of SYTO 9 dye and 1.5 μl of propidium iodide were added to the sample, followed by vortexing and 15 min incubation in the dark. After incubation, cells were trapped with a 0.2-μm polycarbonate membrane filter (Sterlitech, Kent, WA, United States), which were subsequently placed between a slide and a coverslip and used for fluorescence microscopy. Viable cells with intact membranes will appear green by fluorescent SYTO 9, which permeates intact or damaged plasma membrane, while propidium iodide can only permeate damaged and disrupted plasma membranes and competes with SYTO 9 for DNA-binding sites. Therefore, cells with intact membranes show green fluorescence while cells with damaged membranes appear with red fluorescence. Those were considered to be dead. Pictures were taken with the Zeiss Observer Z1 inverted widefield microscope and ZEN 2012 (blue edition) software (Oberkochen, Germany).
Results
Setup to Determine the Minimum ATP Inhibitory Concentration
To determine the MAIC, VBNC induction conditions for each bacterial species (L. monocytogenes EGDe, ScottA, QC1, and MFPT3; Escherichia coli BW, ATCC, and JW; B. cereus KSS and ATCC; and Pseudomonas aeruginosa ATCC, K71, and K73) had to be evaluated by a preceding screening, as described by Robben et al. (2018). The VBNC state was confirmed if cells lost the ability to grow on non-selective agar plates, could produce de novo ATP over a level of 105 RLU within 24 h without regaining their culturability, and could be killed by incubation at 90°C for 15 min (Figure 1).
As a result of the preceding screening, a non-ionic detergent–salt combination was determined for each of the 12 bacterial strains, which led to stable VBNC induction (Table 1). Nevertheless, as induction of the VBNC state is not a 100% reliable process the respective VBNC confirmation and controls, comparison of BacLight viability assay, and ATP measurements of VBNC and dead bacteria, as described in Figure 1, were performed for each corresponding experiment.
For clarity purposes, only exemplary results for one representative strain per bacterial species are depicted in Figure 2, due to the fact that no differences between the respective strains were found (data not shown).
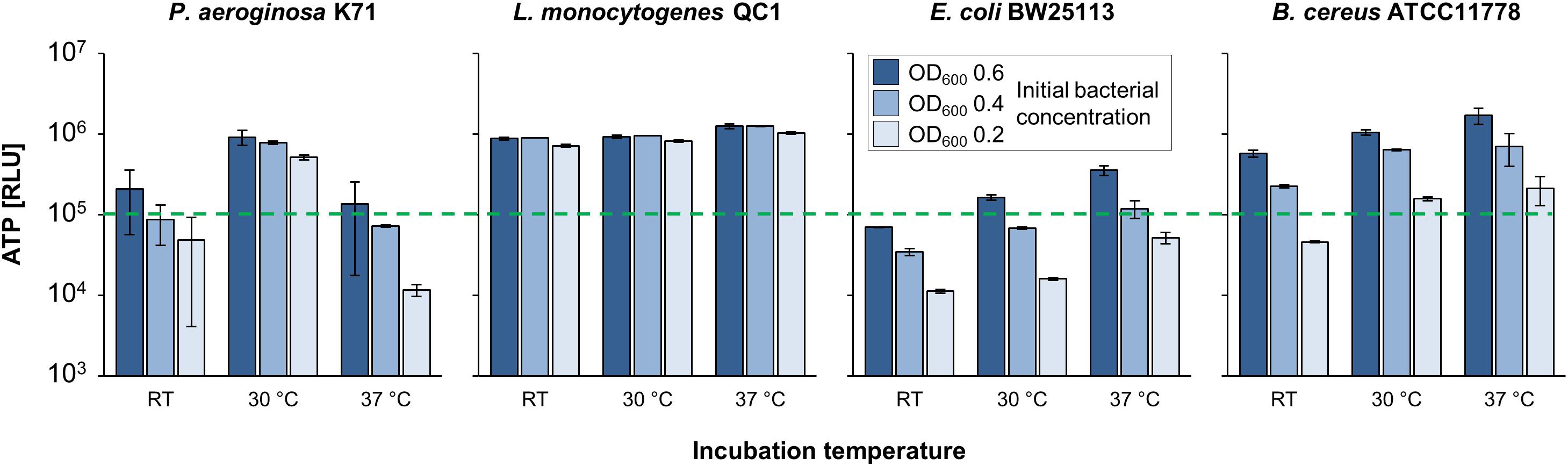
Figure 2. VBNC incubation setup for optimum framework conditions. De novo ATP production [relative light units (RLU)] of VBNC cells was monitored at room temperature (RT), 30, and 37°C at three different optical densities (OD600: 0.2, 0.4, and 0.6) with different bacterial strains.
The highest de novo ATP production after 24 h incubation in BHI was found to be at the respective optimal growth temperature and at the highest cell density of each strain (Figure 2). Based on these results, from this point forward incubation of the culture after VBNC induction was performed at 37°C for E. coli, L. monocytogenes, and B. cereus. For P. aeruginosa strains, the incubation temperature was adapted to their optimal temperature of 30°C, as it resulted in higher ATP production compared to incubation at RT or 37°C. Cultures were used with an optical density of 0.6 (Figure 2).
Loss of de novo ATP Production of Cells in the VBNC State Is a Suitable Indicator of Killing Efficiency
After setting the optimal framework conditions, temperature-dependent time-kill experiments were conducted to evaluate de novo ATP production as an indicator of the overall bactericidal effect of a defined stress. The plate count results after 24 h of incubation are presented as blue bars in Figure 3. The identical experimental approach was used with L. monocytogenes cells in the VBNC state with a subsequent measurement of de novo ATP production after 24 h incubation in BHI medium indicated as green bars.
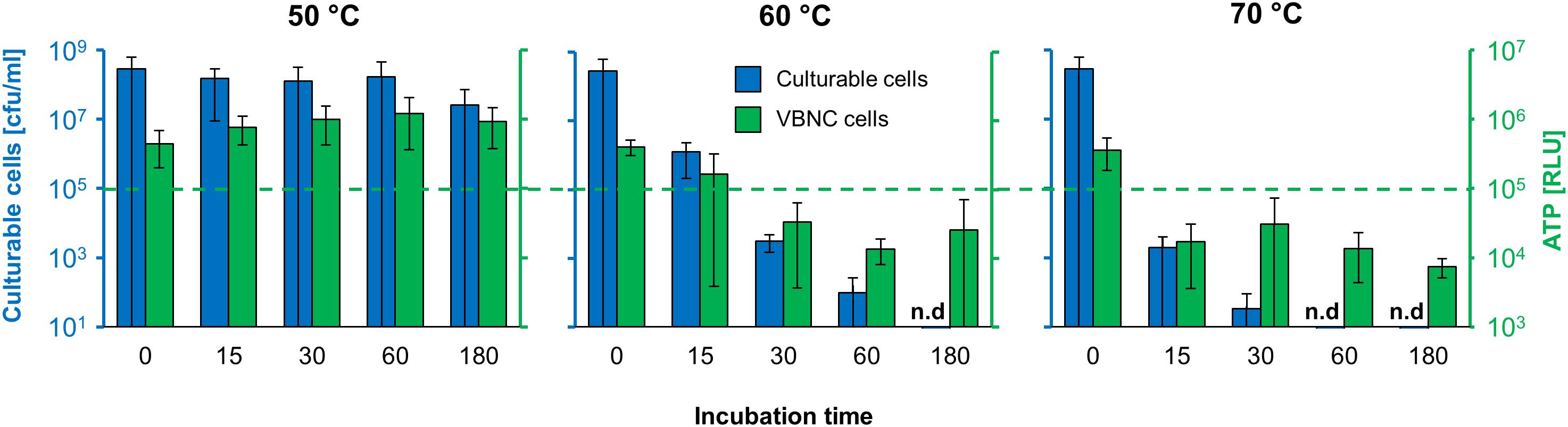
Figure 3. Evaluation of the VBNC–MIC assay by temperature-dependent time-kill curves. De novo ATP production (green bars) of VBNC L. monocytogenes was determined and compared to plate count (blue bars) of culturable L. monocytogenes after incubation at 50, 60, and 70°C over a period of 180 min; n.d., not detectable.
After incubation at 50°C, no significant reduction in the CFU/ml was found, demonstrating the ability of L. monocytogenes to withstand this stress condition for at least 60 min. Similar results were also obtained for cells in the VBNC state, where no significant impact of the incubation temperature on subsequent de novo ATP production was found.
In the case of 60°C incubation, there was a clear time dependency in terms of reduction of living cells for both the culturable cells as well as for the VBNC cells. In the case of the culturable cells, a two log reduction was found after 15 min, while after 30, 60, and 180 min a four-log reduction (indicating disinfection efficiency) was found. In the case of the VBNC cells, de novo ATP production was still clearly above the 105 RLU threshold after 15 min, indicating that the VBNC cells were still intact, while after 30, 60, and 180 min the number was below this threshold. Similar results were also obtained at 70°C, where both the culturable cells and the VBNC cells were reduced to a non-detectable level after merely 15 min of incubation. Overall, there was good agreement between the results for L. monocytogenes EGDe in either the culturable or VBNC state at each of the different incubation temperatures.
Validation of the VBNC–MIC Assay
While the results of the time-kill heat stress experiment indicate that this method could be used for such semi-quantitative analysis, the focus of this study was to use it as a qualitative Yes/No screening method comparable to classic microbiological MIC testing.
Therefore, an initial MIC screening of the disinfectant benzalkonium chloride (BK) and antibiotic ampicillin (Amp) on L. monocytogenes EGDe in the “normal” culturable state as well as the VBNC state was performed (VBNC–MIC assay). In the case of the VBNC cells, de novo ATP production in the presence of the respective stress was measured after 24 h of incubation and the cellular status was determined with the BacLight viability assay (Figure 4).
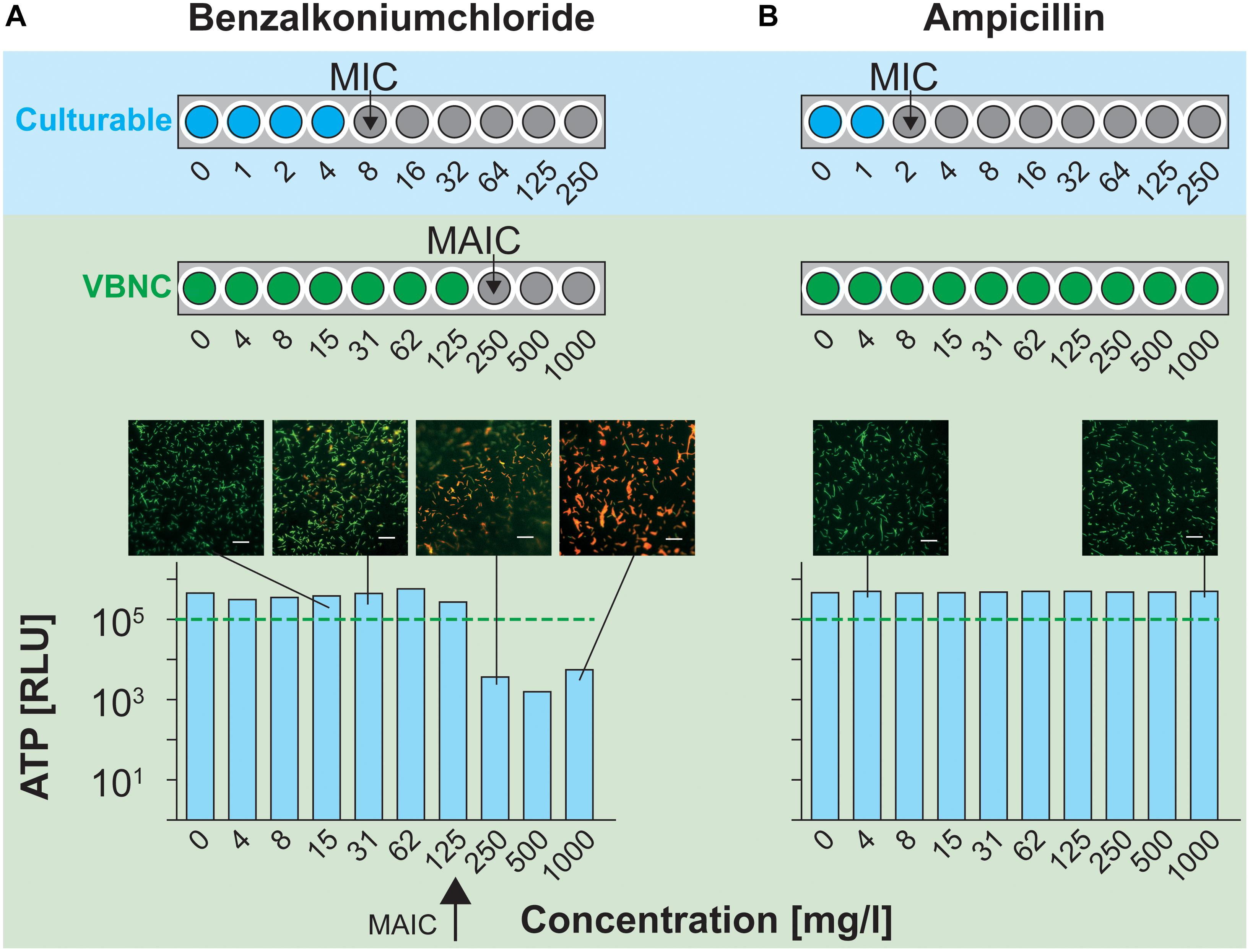
Figure 4. MIC determination for culturable and MAIC determination for VBNC L. monocytogenes. (A) MIC of benzalkonium chloride (BK) (blue dots) was assessed by the standard broth dilution procedure (MIC assay) and plate count method. MAIC (green dots) of BK for VBNC L. monocytogenes was determined by de novo ATP measurements (VBNC–MIC assay). The BacLight viability assay was used to validate the VBNC–MIC assay. (B) MIC and MAIC assessment of Amp. Scale bar: 50 μm.
In the case of BK, the MIC for culturable cells was 8 mg/l, while in the VBNC state a MAIC of 250 mg/l was calculated. The values determined by the ATP measurements correlated well with the cellular status determined with the BacLight viability assay. At BK concentrations where de novo ATP production exceeded 105 RLU, the majority of the cells appeared bright green, indicating intact cell membranes and overall cell viability (Figure 4A). At the respective MAIC of 250 mg/l, cells switched to appear predominantly red instead of green, indicating damaged cell membranes and thus non-viable cells.
In the case of Amp, the amount of de novo ATP production by L. monocytogenes in the VBNC state did not drop below the threshold of 105 RLU for any concentration within the experimental approach (maximum tested concentration of 1,000 mg/l). In contrast, the MIC for culturable cells was 1 mg/l. These results were confirmed with the BacLight assay, where VBNC cells appeared bright green, even at 1,000 mg/l (Figure 4B).
Resistance of VBNC Cells to Antibiotics
To evaluate results obtained, we performed an extended screening of the 12 bacterial strains and determined the respective MIC and MAIC of four antibiotics (Amp, imipenem, ciprofloxacin, and gentamicin). As there were no significant differences between the different strains of the respective bacterial species, for clarity purposes only exemplary results for one representative strain per bacterial species are depicted in Table 2, and the results for each of the 12 strains are compared in Supplementary Table S1.
Results clearly show that VBNC pathogens were resistant to all the antibiotics used, while culturable cells completely lost their culturability during overnight incubation in the presence of Amp, imipenem, ciprofloxacin, and gentamicin.
Tolerance of VBNC Cells to Disinfectants and Preservatives
The MIC and MAIC were also determined for two quaternary ammonium compounds (QACs) [BK and trioctylmethylammonium (TOMA) chloride] and two preservatives (bronopol and sodium azide). As there were no significant differences between the different strains of the respective bacterial species only exemplary results for one representative strain per bacterial species are depicted in Table 3, and the results for each of the 12 strains are listed in Supplementary Table S1.
Indeed, VBNC pathogens were much more tolerant to BK and TOMA chloride as well as to the preservatives bronopol and sodium azide.
Taking L. monocytogenes as a representative example, it is up to 220 times more tolerant to QACs compared to its culturable form. While the averaged MIC for culturable cells was 6.9 mg/l, VBNC L. monocytogenes survived until a mean concentration of 381.3 mg/l. Similar results were obtained for TOMA chloride. In addition, the MIC and MAIC of the preservatives bronopol and sodium azide were tested. While the mean MIC concentration of bronopol on culturable L. monocytogenes was 59.5 mg/l, VBNC cells were only inactivated using a concentration exceeding 700 mg/l. Moreover, they were resistant to sodium azide, the second preservative tested. Related results were obtained for E. coli, B. cereus, and P. aeruginosa, where VBNC cells were distinctly more tolerant than culturable cells. Further information regarding differences within each species detailed test results and statistics can be taken from Supplementary Table S1.
Discussion
The VBNC state is a unique survival strategy adopted by many bacteria in response to adverse environmental conditions. However, the VBNC state is a major problem when it involves pathogens, where they can evade culture-based standard detection methods and tolerate higher antibiotic and disinfectant concentrations (Ramamurthy et al., 2014). It is therefore essential to understand the characteristics of cells in the VBNC state, which unfortunately has been very difficult to investigate experimentally until now.
Therefore, the aim of this study was to develop a cost-effective, time-saving, high-throughput method for determining the tolerance of VBNC bacteria to antimicrobials based on de novo ATP production. ATP measurements have been successfully and routinely performed for the detection or confirmation of viable bacterial cells, including those in the VBNC state, for more than a decade (Lindback et al., 2010). ATP measurements have been successfully and routinely performed for the detection or confirmation of viable bacterial cells, including those in the VBNC state, for more than a decade. However, to the best of our knowledge, de novo ATP production has never been utilized as an indicator of effective bactericidal concentrations.
Establishment of the VBNC–MIC Assay for High-Throughput MAIC Determinations
In order to develop this novel VBNC–MIC screening approach, we induced the VBNC state in 12 different bacterial strains through a combinational effect of non-ionic detergents and salts. For the VBNC–MIC assay to work, it is essential that the cells in the VBNC state are still metabolically active to produce enough ATP de novo within 24 h, which permits reliably differentiation of VBNC cells from dead bacteria. We found that the optimum framework conditions were an initial cell density of ∼1 × 108 CFU/ml (OD600 of 0.6) with a subsequent 24 h incubation at the respective optimal growth temperature of each strain.
The overall reliability of the VBNC–MIC approach was confirmed by a series of time-kill experiments, where the effect of three different incubation temperatures (50, 60, and 70°C) within 3 h on L. monocytogenes EGDe in the culturable as well as in the VBNC state were compared. While Nowakowska and Oliver (2013) reported a higher tolerance of V. vulnificus to 50°C stress, we suspected that yet higher stress would eventually inactivate VBNC cells. Indeed, our results show that the course of the time-kill curves of VBNC bacteria under temperature stress was very similar to those of culturable cells. Although the use of RLUs as a quantitative measure is in this case not completely applicable, we could see semi-quantitative correlations to the course of culturable cells that confirmed that this method is reliable to answer binary-based questions. As confirmation, we double-checked the outcome with BacLight fluorescence staining and obtained corresponding results, which validated 105 RLU as a reliable cut-off to distinguish between viable and dead cells. Consequently, the new VBNC–MIC approach can be used in a straightforward way to compare the tolerance of cells in the VBNC state to those in the culturable state to a defined stress. While the results of the time-kill heat stress experiments indicate that this method could be used for a semi-quantitative analysis, the focus of this study was to use it as a qualitative Yes/No screening method comparable to classic microbiological MIC testing.
With this VBNC–MIC approach, the susceptibility of four different L. monocytogenes strains, three E. coli strains, two B. cereus strains, and three P. aeruginosa strains in the exponential phase of growth and in the VBNC state, to antibiotics and antimicrobials, was tested by MAIC determination. This variety of strains and species, with a range of susceptibilities to antimicrobials in the culturable state, was used to analyze different responses of their VBNC counterparts to antimicrobial stress.
VBNC–MIC Assay Reveals That VBNC Pathogens Withstand Antimicrobials
While previous studies were either limited to resuscitation of V. vulnificus or utilized more complex experimental setups using RT-qPCR or fluorescence-activated cell sorting (FACS), the results obtained in this study using the VBNC–MIC assay corroborate previous results and demonstrate the complete ineffectiveness of antibiotics to bacterial cells in the VBNC state (Lleò et al., 2003; Oliver, 2010; Nowakowska and Oliver, 2013; Lin et al., 2017). We could show that when in the VBNC state, each of 12 investigated bacterial strains became resistant to Amp, imipenem, gentamicin, and ciprofloxacin, which represent three major antibiotic classes. Additionally, the MIC for the culturable bacteria is in agreement with clinical breakpoints for bacteria, according to the European Committee on Antimicrobial Susceptibility Testing (EUCAST). Hereby, Amp represents the beta-lactam antibiotics and targets cell wall synthesis by inhibiting the enzyme transpeptidase (Bamford et al., 2017). Imipenem is also a beta-lactam but unlike Amp, it is a member of the carbapenem class and remains stable in the presence of beta-lactamase. Imipenem has been shown to activate peptidoglycan genes in P. aeruginosa and may contribute to VBNC induction, as increased thickness of the cell wall is characteristic for some VBNC pathogens (Davies et al., 2006; Oliver, 2010; Pasquaroli et al., 2014). Ciprofloxacin, a synthetic fluoroquinolone, inhibits cell division by inhibiting DNA gyrase and is also used for VBNC detection by direct viable counting (Ramamurthy et al., 2014). Gentamicin, an aminoglycoside that irreversibly binds to the 16S rRNA component of the 30S subunit of the bacterial ribosome and inhibits protein synthesis, was shown not only to be ineffective against VBNC pathogens but could also induce the VBNC state in culturable P. aeruginosa (Kohanski et al., 2010; Lee and Bae, 2018). Research shows that VBNC pathogens are protected from antibiotics by their lower metabolic activity, growth arrest, and higher efflux rates (Lin et al., 2017; Sikri et al., 2018). These findings are consistent with our results.
In addition to the antibiotics, this study also compared the susceptibility of bacteria in the VBNC state to a QAC (BK; often used in cleaning solutions and disinfectants) and an ionic liquid (TOMA chloride, a promising new class of disinfectant) (Abbaszadegan et al., 2015; Mester et al., 2016; Gundolf et al., 2018). QACs have strong bactericidal activity, depending upon their structure and length of the alkyl side chain (Ferreira et al., 2011). QACs disrupt cell walls and result in leakage of the cytoplasm, therefore exemplifying a completely different antimicrobial approach than antibiotics (Bragg et al., 2014). The results of this study show that, in the VBNC state, bacterial cells were up to 300 times more tolerant to BK and TOMA chloride compared with culturable equivalents. Possible explanations for this observed tolerance could be increased membrane thickness, reduced membrane fluidity, or increased efflux pump activity, which have all been reported for the VBNC state and each of which would reduce the effectiveness of QACs or ionic liquids (Mester et al., 2016). However, in marked contrast, Rowe et al. (1998) reported that VBNC Campylobacter jejuni was more sensitive to BK than culturable C. jejuni. As only limited research has been conducted so far on this topic, it is not clear if these differences are a result of different induction procedures, or characteristic of the antimicrobials or bacterial strains used.
Similar to BK and TOMA chloride, 12 strains in the VBNC state demonstrated higher tolerance to the preservatives bronopol (a formaldehyde releaser) and sodium azide (known to inhibit microbial growth and oxidation of manganese, iron, and sulfur by cytochrome c oxidase) compared to respective culturable cells (Wang and Levin, 2009; Kireche et al., 2011; Cabrol et al., 2017). Again, the results obtained with the VBNC–MIC approach in this study are in good agreement with previous studies that demonstrated elevated resistance of bacterial cells in the VBNC state to antibiotic treatments using different strains and methods (Nowakowska and Oliver, 2013).
This observed tolerance of bacterial cells in the VBNC state is a serious concern for human health. Cleaning and disinfection strategies are currently adapted to culturable bacteria and in light of this study are not now seen to be sufficient as a proper intervention against VBNC pathogens. From our point of view, these results have significant implications for microbiologists in all fields, especially in the context of detection, intervention, and chemotherapy when confronted with VBNC pathogens. The results show that the VBNC–MIC approach is suitable for high-throughput susceptibility screening of bacterial cells in the VBNC state. This information can be used for further research and to provide a better understanding of the underlying mechanisms regarding VBNC resistance and tolerance.
Data Availability
All datasets generated for this study are included in the manuscript and/or the Supplementary Files.
Author Contributions
CR, PM, AW, BS, and PR conceived and designed the experiments. CR and PM performed the experiments. CR, PM, AW, and DS analyzed the data and wrote the manuscript. BS provided the bacterial strains.
Funding
This work was supported by the Christian Doppler Research Association, the Austrian Federal Ministry for Digital, Business and Enterprise, and the National Foundation of Research, Technology and Development.
Conflict of Interest Statement
The authors declare that the research was conducted in the absence of any commercial or financial relationships that could be construed as a potential conflict of interest.
Acknowledgments
We thank Martin Bobal and the VetCore – Facility for Research for methodological support and for providing great images and helpful discussions.
Supplementary Material
The Supplementary Material for this article can be found online at: https://www.frontiersin.org/articles/10.3389/fmicb.2019.01365/full#supplementary-material
References
Abbaszadegan, A., Nabavizadeh, M., Gholami, A., Aleyasin, Z. S., Dorostkar, S., Saliminasab, M., et al. (2015). Positively charged imidazolium-based ionic liquid-protected silver nanoparticles: a promising disinfectant in root canal treatment. Int. Endodon. J. 48, 790–800. doi: 10.1111/iej.12377
Andrews, J. M. (2001). Determination of minimum inhibitory concentrations. J. Antimicrob. Chemother. 48(Suppl. 1), 5–16.
Anvarian, A. H. P., Smith, M. P., and Overton, T. W. (2016). The effects of orange juice clarification on the physiology of Escherichia coli; growth-based and flow cytometric analysis. Int. J. Food Microbiol. 219, 38–43. doi: 10.1016/j.ijfoodmicro.2015.11.016
Ayrapetyan, M., Williams, T., and Oliver, J. D. (2018). Relationship between the viable but nonculturable state and antibiotic persister cells. J. Bacteriol. 200, e249–e218. doi: 10.1128/JB.00249-218
Ayrapetyan, M., Williams, T. C., Baxter, R., and Oliver, J. D. (2015). Viable but nonculturable and persister cells coexist stochastically and are induced by human serum. Infect. Immun. 83, 4194–4203. doi: 10.1128/IAI.00404-415
Bamford, R. A., Smith, A., Metz, J., Glover, G., Titball, R. W., and Pagliara, S. (2017). Investigating the physiology of viable but non-culturable bacteria by microfluidics and time-lapse microscopy. BMC Biol. 15:121. doi: 10.1186/s12915-017-0465-464
Bragg, R., Jansen, A., Coetzee, M., van der Westhuizen, W., and Boucher, C. (2014). Bacterial resistance to Quaternary Ammonium Compounds (QAC) disinfectants. Adv. Exp. Med. Biol. 808, 1–13. doi: 10.1007/978-81-322-1774-9_1
Cabrol, L., Quéméneur, M., and Misson, B. (2017). Inhibitory effects of sodium azide on microbial growth in experimental resuspension of marine sediment. J. Microbiol. Methods 133, 62–65. doi: 10.1016/j.mimet.2016.12.021
Davies, J., Spiegelman, G. B., and Yim, G. (2006). The world of subinhibitory antibiotic concentrations. Curr. Opin. Microbiol. 9, 445–453. doi: 10.1016/j.mib.2006.08.006
Ferreira, C., Pereira, A. M., Pereira, M. C., Melo, L. F., and Simões, M. (2011). Physiological changes induced by the quaternary ammonium compound benzyldimethyldodecylammonium chloride on Pseudomonas fluorescens. J. Antimicrob. Chemother. 66, 1036–1043. doi: 10.1093/jac/dkr028
Gonçalves, F. D., and de Carvalho, C. C. (2016). Phenotypic modifications in Staphylococcus aureus cells exposed to high concentrations of vancomycin and teicoplanin. Front. Microbiol. 7:13. doi: 10.3389/fmicb.2016.00013
Gundolf, T., Rauch, B., Kalb, R., Rossmanith, P., and Mester, P. (2018). Influence of bacterial lipopolysaccharide modifications on the efficacy of antimicrobial ionic liquids. J. Mol. Liquids 271, 220–227. doi: 10.1016/j.molliq.2018.08.134
Harms, A., Maisonneuve, E., and Gerdes, K. (2016). Mechanisms of bacterial persistence during stress and antibiotic exposure. Science 354:aaf4268. doi: 10.1126/science.aaf4268
Kireche, M., Peiffer, J.-L., Antonios, D., Fabre, I., Giménez-Arnau, E., Pallardy, M., et al. (2011). Evidence for chemical and cellular reactivities of the formaldehyde releaser bronopol, independent of formaldehyde release. Chem. Res. Toxicol. 24, 2115–2128. doi: 10.1021/tx2002542
Kohanski, M. A., Dwyer, D. J., and Collins, J. J. (2010). How antibiotics kill bacteria: from targets to networks. Nat. Rev. Microbiol. 8, 423–435. doi: 10.1038/nrmicro2333
Lee, S., and Bae, S. (2017). Molecular viability testing of viable but non-culturable bacteria induced by antibiotic exposure. Microbiol. Biotechnol. 11, 1008–1016. doi: 10.1111/1751-7915.13039
Lee, S., and Bae, S. (2018). Molecular viability testing of viable but non-culturable bacteria induced by antibiotic exposure. Microbiol. Biotechnol. 11, 1008–1016. doi: 10.1111/1751-7915.13039
Lewis, K. (2006). Persister cells, dormancy and infectious disease. Nat. Rev. Microbiol. 5, 48–56. doi: 10.1038/nrmicro1557
Li, J., Xie, S., Ahmed, S., Wang, F., Gu, Y., Zhang, C., et al. (2017). Antimicrobial activity and resistance: influencing factors. Front. Pharmacol. 8:364. doi: 10.3389/fphar.2017.00364
Li, L., Mendis, N., Trigui, H., Oliver, J. D., and Faucher, S. P. (2014). The importance of the viable but non-culturable state in human bacterial pathogens. Front. Microbiol. 5:258. doi: 10.3389/fmicb.2014.00258
Lin, H., Ye, C., Chen, S., Zhang, S., and Yu, X. (2017). Viable but non-culturable E. coli induced by low level chlorination have higher persistence to antibiotics than their culturable counterparts. Environ. Poll. 230, 242–249. doi: 10.1016/j.envpol.2017.06.047
Lindback, T., Rottenberg, M. E., Roche, S. M., and Rorvik, L. M. (2010). The ability to enter into an avirulent viable but non-culturable (VBNC) form is widespread among Listeria monocytogenes isolates from salmon, patients and environment. Vet. Res. 41:8. doi: 10.1051/vetres/2009056
Lleò, M. M., Bonato, B., Signoretto, C., and Canepari, P. (2003). Vancomycin resistance is maintained in enterococci in the viable but nonculturable state and after division is resumed. Antimicrobial. Agents Chemother. 47, 1154–1156. doi: 10.1128/AAC.47.3.1154-1156.2003
Mester, P., Jehle, A. K., Leeb, C., Kalb, R., Grunert, T., and Rossmanith, P. (2016). FTIR metabolomic fingerprint reveals different modes of action exerted by active pharmaceutical ingredient based ionic liquids (API-ILs) on Salmonella typhimurium. RSC Adv. 6, 32220–32227. doi: 10.1039/C5RA24970H
Nowakowska, J., and Oliver, J. D. (2013). Resistance to environmental stresses by Vibrio vulnificus in the viable but nonculturable state. FEMS Microbiol. Ecol. 84, 213–222. doi: 10.1111/1574-6941.12052
Oliver, J. D. (2010). Recent findings on the viable but nonculturable state in pathogenic bacteria. FEMS Microbiol. Rev. 34, 415–425. doi: 10.1111/j.1574-6976.2009.00200.x
Orruño, M., Kaberdin, V. R., and Arana, I. (2017). Survival strategies of Escherichia coli and Vibrio spp.: contribution of the viable but nonculturable phenotype to their stress-resistance and persistence in adverse environments. World J. Microbiol. Biotechnol. 33:45. doi: 10.1007/s11274-017-2218-2215
Overney, A., Jacques-André-Coquin, J., Ng, P., Carpentier, B., Guillier, L., and Firmesse, O. (2017). Impact of environmental factors on the culturability and viability of Listeria monocytogenes under conditions encountered in food processing plants. Int. J. Food Microbiol. 244, 74–81. doi: 10.1016/j.ijfoodmicro.2016.12.012
Pasquaroli, S., Citterio, B., Cesare, A. D., Amiri, M., Manti, A., Vuotto, C., et al. (2014). Role of daptomycin in the induction and persistence of the viable but non-culturable state of Staphylococcus aureus biofilms. Pathogens 3, 759–768. doi: 10.3390/pathogens3030759
Pinto, D., Santos, M. A., and Chambel, L. (2015). Thirty years of viable but nonculturable state research: unsolved molecular mechanisms. Critic. Rev. Microbiol. 41, 61–76. doi: 10.3109/1040841X.2013.794127
Pu, Y., Zhao, Z., Li, Y., Zou, J., Ma, Q., Zhao, Y., et al. (2016). Enhanced efflux activity facilitates drug tolerance in dormant bacterial cells. Mol. cell 62, 284–294. doi: 10.1016/j.molcel.2016.03.035
Ramamurthy, T., Ghosh, A., Pazhani, G. P., and Shinoda, S. (2014). Current perspectives on viable but non-culturable (VBNC) pathogenic bacteria. Front. Public Health 2:103. doi: 10.3389/fpubh.2014.00103
Robben, C., Fister, S., Witte, A. K., Schoder, D., Rossmanith, P., and Mester, P. (2018). Induction of the viable but non-culturable state in bacterial pathogens by household cleaners and inorganic salts. Sci. Rep. 8:15132. doi: 10.1038/s41598-018-33595-33595
Rowan, N. J. (2004). Viable but non-culturable forms of food and waterborne bacteria: quo vadis? Trends Food Sci. Technol. 15, 462–467. doi: 10.1016/j.tifs.2004.02.009
Rowe, M. T., Dunstall, G., Kirk, R., Loughney, C. F., Cooke, J. L., and Brown, S. R. (1998). Development of an image system for the study of viable but non-culturable forms of Campylobacter jejuni and its use to determine their resistance to disinfectants. Food Microbiol. 15, 491–498. doi: 10.1006/fmic.1997.0178
Schmitz-Esser, S., Müller, A., Stessl, B., and Wagner, M. (2015). Genomes of sequence type 121 Listeria monocytogenes strains harbor highly conserved plasmids and prophages. Front. Microbiol. 6:380. doi: 10.3389/fmicb.2015.00380
Sikri, K., Duggal, P., Kumar, C., Batra, S. D., Vashist, A., Bhaskar, A., et al. (2018). Multifaceted remodeling by vitamin C boosts sensitivity of Mycobacterium tuberculosis subpopulations to combination treatment by anti-tubercular drugs. Redox Biol.gy 15, 452–466. doi: 10.1016/j.redox.2017.12.020
Wang, J. D., and Levin, P. A. (2009). Metabolism, cell growth and the bacterial cell cycle. Nat. Rev. Microbiol. 7, 822–827. doi: 10.1038/nrmicro2202
Zhang, S., Ye, C., Lin, H., Lv, L., and Yu, X. (2015). UV disinfection induces a vbnc state in Escherichia coli and Pseudomonas aeruginosa. Environ. Sci. Technol. 49, 1721–1728. doi: 10.1021/es505211e
Keywords: viable but non-culturable (VBNC), antibiotic resistance, food safety, antimicrobial tolerance, minimum inhibitory concentration, metabolic activity
Citation: Robben C, Witte AK, Schoder D, Stessl B, Rossmanith P and Mester P (2019) A Fast and Easy ATP-Based Approach Enables MIC Testing for Non-resuscitating VBNC Pathogens. Front. Microbiol. 10:1365. doi: 10.3389/fmicb.2019.01365
Received: 05 April 2019; Accepted: 31 May 2019;
Published: 14 June 2019.
Edited by:
Satoru Suzuki, Ehime University, JapanReviewed by:
Hiroyuki Yamamoto, Japan Agency for Marine-Earth Science and Technology, JapanSunil D. Saroj, Symbiosis International (Deemed University), India
Copyright © 2019 Robben, Witte, Schoder, Stessl, Rossmanith and Mester. This is an open-access article distributed under the terms of the Creative Commons Attribution License (CC BY). The use, distribution or reproduction in other forums is permitted, provided the original author(s) and the copyright owner(s) are credited and that the original publication in this journal is cited, in accordance with accepted academic practice. No use, distribution or reproduction is permitted which does not comply with these terms.
*Correspondence: Patrick Mester, UGF0cmljay1qdWxpYW4ubWVzdGVyQHZldG1lZHVuaS5hYy5hdA==
†Present address: Anna Kristina Witte, HTK Hygiene Technologie Kompetenzzentrum GmbH, Bamberg, Germany