- 1Department of Laboratory Medicine, The First Affiliated Hospital of Sun Yat-sen University, Guangzhou, China
- 2Department of Laboratory Medicine, The Second Affiliated Hospital of Guangzhou University of Chinese Medicine, Guangzhou, China
- 3Department of Laboratory Medicine, Guangdong Provincial Hospital of Chinese Medicine, Guangzhou, China
- 4Department of Laboratory Medicine, Ningxia Hospital of Ningxia Medical University, Yinchuan, China
- 5Public Health Research Institute Tuberculosis Center, New Jersey Medical School, Rutgers University, Newark, NJ, United States
- 6Department of Laboratory Medicine, Memorial Sloan Kettering Cancer Center, New York, NY, United States
- 7Department of Pathology and Laboratory Medicine, Weill Medical College of Cornell University, New York, NY, United States
Enterobacter cloacae has recently emerged as one of the most common carbapenem-resistant Enterobacteriaceae. The emergence and spread of metallo-β-lactamase-producing E. cloacae have posed an immediate threat globally. Here, we investigated the molecular characteristics of 84 carbapenem-resistant Enterobacter cloacae (CREL) collected from three tertiary hospitals in China between 2012 and 2016. Species identification and antimicrobial susceptibility testing were performed using a VITEK-2 system. Carbapenems, polymyxins B, and tigecycline were tested by broth microdilution method. The carbapenem in activation method (CIM) and cefoxitin three-dimensional test were used to detect carbapenemase and AmpC β-lactamase, respectively. Isolates were screened for β-lactam resistance genes by PCR, and expression of ompC and ompF was determined by qRT-PCR. Genetic relatedness was performed by pulsed-field gel electrophoresis (PFGE) and multilocus sequence typing (MLST), while selected isolates were subjected to whole-genome sequencing. Among the 84 CREL isolates, 50 (59.5%) were detected as carbapenemase producers. NDM-1 was the dominant carbapenemase (80.0%), followed by IMP-26 (8.0%) and IMP-4 (6.0%). Notably, we identified the first NDM-1 and IMP-1 co-producing E. cloacae, carrying plasmids of several incompatibility (Inc) groups, including IncHI2, IncHI2A, and IncN. Most isolates showed decreased expression of ompC and/or ompF, and contained a broad distribution of ESBLs and AmpC β-lactamases. These findings suggested that different molecular mechanisms, including carbapenemase, ESBL and/or AmpC plus loss of porins, have contributed to carbapenem resistance. The blaNDM−1-harboring plasmids contained highly conserved gene environment around blaNDM−1 (blaNDM−1-bleMBL-trpF-dsbD-cutA1-groES-groEL), which could be associated with the potential dissemination of blaNDM−1. IMP-type MBL was located within a variety of integrons and usually contained various gene cassettes encoding multidrug resistance. These isolates produced 54 different pulsotypes, and were classified into 42 STs by MLST. Nineteen blaNDM−1-positive E. cloacae isolates obtained from Ningxia had the same pulsotype (PFGE type 1), belonging to ST78 within clonal complex 74 (CC74). The plasmid-based replicon typing indicated that IncX3 plasmids mediated the dissemination of blaNDM−1 among these homologous strains. This is the first report on the outbreak of NDM-1-producing E. cloacae ST78 with contribution of IncX3 plasmids in Northwestern China. There's an immediate need to intensify surveillance attentively to prevent and control the further spread of NDM-1 in China.
Introduction
Enterobacter cloacae, an opportunistic pathogen ranking the third among all Enterobacteriaceae in healthcare-associated infections, may cause various nosocomial infections involving urinary tract, lower respiratory tract, skin and soft tissues, biliary tract, intravenous catheters, and central nervous system (Davin-Regli and Pagès, 2015). E. cloacae is intrinsically resistant to ampicillin, amoxicillin/clavulanic, cephamycin, and the 1st and 2nd generation cephalosporins owing to chromosomally encoded AmpC β-lactamase (Jacoby, 2009). Recently, severe comorbid conditions, extensive invasive procedures, and heavy exposure to antibiotics have involved in the global emergence of carbapenem-resistant E. cloacae (CREL). Carbapenems have increasingly been used as a common drug for the treatment of nosocomial infections. According to CHINET, one of the largest antimicrobial resistance surveillance networks in China, carbapenem resistance rates among E. cloacae were <1.0% in 2007. Whereas, in the year of 2017, the resistance rates to imipenem, meropenem, and ertapenem have rapidly increased to 6.9, 7.0, and 8.2%, respectively. Infections caused by CREL usually result in higher mortality rates, longer hospitalization and higher costs of treatment, which has posed an immediate threat to public health (Kelly et al., 2017).
Resistance to carbapenems is associated with several mechanisms. Carbapenemase, which is largely responsible for carbapenem resistance in Enterobacteriaceae, has been classified into three functional groups: class A (mostly KPC, GES), class B metallo-β-lactamase (MBL, mostly IMP, VIM and NDM) as well as class D OXA-type β-lactamase (mostly OXA-48-like) (Tzouvelekis et al., 2012). Genes encoding MBLs are most commonly identified in E. cloacae and often carried on mobile genetic elements such as plasmids and transposons. Integrons located on such mobile elements play a crucial role in the horizontal transfer of MBL genes between bacteria (Villa et al., 2014; Lee et al., 2017a). In China, carbapenemase production is largely attributed to MBLs such as VIM-1 (Yang et al., 2014), IMP-4 (Wang et al., 2017), IMP-8 (Yan et al., 2002), and NDM-1 (Dai et al., 2013), among which NDM-1 was even more worrisome for its sharp increase. Since the first case of carbapenem-resistant E. cloacae harboring NDM-1 was detected in Chongqing (Wang et al., 2017), NDM-1-producing E. cloacae have emerged in various regions across the country (Jia et al., 2018; Jin et al., 2018). Moreover, moderate- to high-level carbapenem resistance in most isolates was closely related to an additional mechanism of resistance, e.g., decreased porin permeability (Majewski et al., 2016). Alteration or loss of non-specific porins, coupled with ESBL and/or AmpC overexpression is considered as one of the main mechanisms of resistance (Wozniak et al., 2012).
However, there has been little epidemiological data on carbapenem-resistant E. cloacae (CREL) in certain regions in China, such as Southern (e.g., Guangdong) and Northwestern (e.g., Ningxia) China. To gain insights into the evolution of CREL isolates in these two regions, we conducted a molecular epidemiological study to describe the resistance mechanism to carbapenems, clonal relatedness, and the genetic environment of carbapenemases (NDM-1 or IMP) -encoding plasmids among CREL isolates.
Materials and Methods
Bacterial Strains and Characterization
From 2012 to 2016, a total of 84 non-duplicate strains from patients infected by CREL were collected from three tertiary hospitals including hospital A (First Affiliated Hospital of Sun Yat-sen University, Guangdong, China, 25 strains), hospital B (Guangdong Provincial Hospital of Chinese Medicine, Guangdong, China, 18 strains), and hospital C (Ningxia Hospital of Ningxia Medical University, Ningxia, China, 41 strains). Species identification and initial antibiotic susceptibility were analyzed by a VITEK-2 system (bioMerieux, France). Mueller-Hinton broth (Oxoid, UK) supplemented with calcium and magnesium (25.0 mg/L Ca2+ and 12.5 mg/L Mg2+) was used to test five antibiotics, including imipenem (ApexBio, USA), meropenem (ApexBio, USA), ertapenem (Menlunbio, China), polymyxin B (Sigma, USA), and tigecycline (ApexBio, USA). Minimum inhibitory concentrations (MICs) were performed according to the criteria of the Clinical and Laboratory Standards Institute (CLSI) (M100-S27). Isolates with an MIC of 4 μg/mL were considered resistant to polymyxin B. A susceptibility breakpoint of <2 μg/mL for tigecycline was interpreted in accordance with the European Committee on Antimicrobial Susceptibility Testing (EUCAST) guidelines. Carbapenemase production was assessed by the carbapenem in activation method (CIM) (Aguirre-Quiñonero et al., 2017), while AmpC β-lactamase production was examined by three-dimensional test. Strains used in quality control were Escherichia coli ATCC 25922 and Enterobacter cloacae ATCC 700323.
Genotype Analysis
Crude DNA extracts prepared by boiling method were used as template in polymerase chain reactions (PCR). Various β-lactam resistance genes were examined, including carbapenemase genes (blaKPC, blaNDM, blaIMP, blaVIM, and blaOXA−48), ESBL-related genes (blaTEM, blaCTX−M, and blaSHV) and AmpC-β-lactamase genes (blaEBC, blaMOX, blaCIT, and blaDHA). Moreover, mcr-1 colistin resistance gene and tetX tigecycline resistance gene were also determined by using primers as described previously (Pérez-Pérez and Hanson, 2002; Pagani et al., 2003; Biendo et al., 2008; Poirel et al., 2011; Liu et al., 2016). Positive PCR products were sequenced and analyzed by nucleotide homology comparison against GenBank database by BLAST (www.ncbi.nlm.nih.gov/blast/). Details about oligonucleotides and thermal conditions are presented in Supplementary Table 1.
Outer Membrane Protein Gene Expressions
Expression of genes encoding outer membrane proteins (OmpF and OmpC porins) was determined using real-time reverse transcription PCR. The total RNA was extracted using the Trizol method and reversely transcribed into cDNA using the PrimeScriptTM RT reagent Kit with gDNA Eraser (TAKARA, China). Real-time quantitative PCR was performed using SYBR® Green I assay with analysis of dissociation curve (2× SYBR Green q-PCR Master Mix, Biomake, USA) on Applied Biosystems ViiATM 7 Dx (Life Technologies, USA). Each experiment was performed in triplicate. The expression of porin-encoding genes, ompF and ompC, relative to rpoB was determined using specific primers and conditions described previously (Majewski et al., 2016). Relative expression was derived from the 2-Δ(ΔCT) formula, in which ΔCT represented the difference of cycle threshold (CT) between target genes and rpoB, while Δ(ΔCT) represented the difference of ΔCT between CREL strains and a standard strain, Enterobacter cloacae ATCC 700323.
Genetic Homologeity Analysis
All isolates were analyzed by PFGE to determine their genetic relatedness. In brief, the bacterial suspension mixed with equal volumes of low-melting-temperature agarose was cleaved with 1% sodium dodecyl sulfate and proteinase K (Sigma, USA) at 54°C overnight, and then digested with restriction endonuclease Xba I (Takara, Dalian, China) at 37°C for 8 h. DNA separation was performed in 0.5× TBE buffer using a pulsed-field electrophoresis system (CHEF MAPPER; Bio-Rad Laboratories, California, USA) under the following conditions: temperature 14°C; voltage 6.0 V/cm; switch angle, 120°; and switch ramp of 2.16–54.17 s for 18 h. Comparison of pulsotypes was performed with Bionumerics software version 6.6. Isolates were allocated into genetic similarity clusters using an 80% cut-off value. MLST was performed as described previously (https://pubmlst.org/ecloacae/). New alleles and sequence types were submitted to the MLST website and approved (https://pubmlst.org/ecloacae/). EnteroBase together with GrapeTree was used to analyze population evolutionary relationship (https://enterobase.readthedocs.io/en/latest/index.html).
Whole-Genome Sequencing
Six representative strains producing single NDM-1, six strains producing single IMP (according to clonal relatedness), one strain co-producing NDM-1 and IMP-1, and eight strains negative for all β-lactamase genes were selected for whole-genome sequencing. Genomic DNA was extracted using a MiniBEST Bacteria Genomic DNA Extraction Kit (TaKaRa, Dalian, China). DNA library was prepared using a QIAseq FX DNA Library Kit (Qiagen Inc., Valencia, CA) following the manufacturer's recommendations. The quantity and quality of the libraries were assessed with a Qubit dsDNA HS Assay Kit (Life technologies, USA) and LabChip GX Analyzer (Perkin Elmer; Waltham, MA). All barcoded libraries were pooled together in equimolar amounts and sequenced on NextSeq 500 platform (illumina Inc., San Diego, CA). Sequencing raw reads were processed for library adapter removal and filtering using FASTQ preprocessor Fastp v0.12.5 (Chen et al., 2018), followed by de novo assembly with SPAdes v3.13.0 (Bankevich et al., 2012) and annotating using Prokka annotation pipeline (Seemann, 2014). Antimicrobial resistance genes and plasmid-typing identification were mined using ABRicate program (https://github.com/tseemann/abricate, v0.8.2), and the contig containing carbapenemase genes, such as blaNDM and blaIMP, were extracted with an in-house Python script, followed by BLAST against NCBI sequence database. The sequence containing blaIMP of the variable regions of integrons were analyzed followed by the Integron Database (http://integrall.bio.ua.pt/). Genetic organization analysis was visualized using EasyFig v2.2.3 (Sullivan et al., 2011).
Statistical Analysis
All the statistical analyses were performed by SPSS 18.0 (IBM Corp., Armonk, USA). The chi-square test was applied to evaluate difference in antibiotic resistance between carbapenemase-positive and carbapenemase-negative subpopulations. Relative changes in gene expression were indicated with median and extremum. All the relevant data were analyzed by non-parametric rank sum test, and was considered statistically significant if P < 0.05.
Ethical Considerations
This study was approved by Institutional Review Board of Second Affiliated Hospital of Soochow University. The study was retrospective and patients were not identified during data collection. Informed consent was not needed for this study.
Result
Characteristics of Clinical CREL Isolates
Eighty-four clinical CREL isolates were found to be resistant to at least one carbapenem. These isolates were recovered from different sources: body fluids (n = 40), sputum (n = 19), urine (n = 8), wound secretion (n = 5), blood (n = 5), pus (n = 2), catheter (n = 2), cannula (n = 1), semen (n = 1), and tissue (n = 1). These CREL isolates were obtained from patients admitted to the hepatobiliary surgery (n = 25, 29.8%), intensive care unit (n = 17, 20.2%), general surgery (n = 11, 13.1%), neurosurgery (n = 7, 8.3%), pediatrics (n = 4, 4.8%), burns surgery (n = 5, 6.0%), respiratory department (n = 3, 3.6%), gastroenterological surgery (n = 2, 2.4%), cardiology department (n = 2, 2.4%), department of orthopedics (n = 2, 2.4%), nephrology department (n = 1, 1.2%), and other surgery wards (n = 5, 6.0%). Among 84 CREL isolates, 85.7% (72/84) of the isolates were classified as MDR as they were resistant to three or more classes of antibiotics. Seventy-two (85.7%) isolates were resistant to ertapenem, followed by 57 (67.9%) and 54 (64.3%) resistant to imipenem and meropenem, respectively. The strains showed high resistance rates to cefatriaxone (83.3%), ceftazidime (75.0%), cefepime (72.6%), and aztreonam (76.2%), while 43 (51.2%), 51 (60.7%), and 27 (32.1%) strains were resistant to fluoroquinolones, sulfanilamide, and aminoglycosides, respectively. In contrast, 94.0 and 86.9% were susceptible to amikacin and tigecycline. Thirteen E. cloacae isolates were resistant to polymyxin B and two isolates were suspected of heteroresistance. Two E. cloacae isolates (ECL-ZY07 and ECL-ZY14) with resistance to polymyxin B and tigecycline showed promising in-vitro activity against amikacin. Carbapenemase producers presented a dramatically higher resistance rate than negative ones for carbapenemase (P < 0.01). Descriptive statistics on antimicrobial susceptibility tests were presented in Tables 1, 2.
Phenotype and Genotype Analysis
Fifty-four isolates were considered positive in the CIM test according to Tijet et al. (2016), but only 50 (59.5%) were demonstrated as carbapenemase producers by PCR analysis. All KPC, NDM, VIM, or IMP producing isolates were unequivocally detected with the CIM test. Four isolates non- producing carbapenemase (one with blaTEM−1, blaSHV−12, blaCTX−M, and blaDHA−1, three hyperproducing AmpC) were positive by the test. The sensitivity and specificity of the detection assay compared with molecular methods were 100 and 89.5%. The blaNDM−1 gene was found the most prevalent carbapenemase gene (40/50), followed by blaIMP−26 (4/50), blaIMP−4 (3/50), blaIMP−1 (1/50), blaVIM−4 (1/50), and blaKPC−2 (1/50). We observed one E. cloacae isolate co-harboring blaNDM−1 and blaIMP−1, which resulted in strongly high MICs of all carbapenems (MICs = 256 μg/L). Remarkably, 97.6% (40/41) of the isolates obtained from Ningxia were MBL producers, but only 20.9% (9/43) produced MBL in Guangdong. No carbapenemase gene was detected in hospital B (Guangdong Provincial Hospital of Chinese Medicine, Guangdong, China). Moreover, 83.3% of the isolates continuously produced high AmpC β-lactamase, and 10.7% were pAmpC producers (eight with blaDHA−1, and one with blaLAP−2). All carried chromosomal-mediated ACT-type β-lactamase. ESBL producers were found in 36 (42.9%) isolates, among which 25 isolates overproduced AmpC. 19.0% (16/84), 15.5% (13/84), 26.2% (22/84), and 23.8% (20/84) of the isolates were detected as positive for blaTEM−30, blaTEM−1, blaCTX−M, and blaSHV−12, respectively. Meanwhile, 31.0% (26/84) of the isolates co-expressed carbapenemase and ESBL, and 7.1% (6/84) produced carbapenemase, ESBL and pAmpC simultaneously. None of the isolates were PCR-positive for blaOXA−48, mcr-1 or tetX.
Expression of ompC and ompF Genes
Relative expression of ompF gene fell within a range of 0.01–1.58, with a median value of 0.12, while expression of ompC gene ranged from 0.01 to 36.36, with a median value of 2.80. Thirty-two isolates lost or had lower expression of both major porins, while 52 isolates had decreased expression of one porin, especially for OmpF porin (50/52). Isolates with a combination of carbapenemase and porin loss showed extensively high carbapenem MICs. All 34 non-carbapenemase-producing isolates had lost at least one porin, among which 22 isolates had reduced expression of both major porins. Additionally, 7 isolates produced both ESBL and AmpC, and 24 isolates produced only one type of enzyme. The remaining 3 isolates were negative for ESBLs (TEM, SHV, or CTX-M) and AmpC. Porin loss together with AmpC overexpression typically resulted in insensitivity to one or two carbapenems. Compared with isolates non-susceptible to three carbapenems and isolates only non-susceptible to one or two carbapenems, the latter had significantly lower expression of ompC (P < 0.01), but there was no difference in ompF expression among two groups (P = 0.398). Further, lower expression of ompC was significantly associated with increasing MIC of imipenem (P < 0.01). All details about antibiotic susceptibilities and porin expression of CREL isolates were given in Table 3.
PFGE Analysis
Pulsed-field gel electrophoresis patterns of the 84 CREL isolates were identified into 54 pulsotypes (clusters 1–54), and of these, PFGE types 2, and 3 could be classified into 3 subtypes, while types 4, 5, and 7 were classified into 2 subtypes (Figure 1). PFGE revealed genetic diversity, whereas PFGE types 1, 2, 3, 4, 5, 6, and 7 were observed in 19, 6, 3, 2, 2, 2, and 2 different patients, respectively. Notably, all the 19 strains with a single dominant pulsotype (PFGE type 1) were isolated from patients in hepatobiliary surgery (17/19), intensive care unit (1/19), and vascular surgery (1/19) during a detection peak period (from Jan. 2016 to Sep. 2016) in hospital C. As shown in Figure 2, the strain ECL-NX38 which was isolated from a 78-year-old patient in hepatobiliary surgery on January 4, 2016 could be the first strain of these outbreak cases. Besides, three strains of type 3 were isolated from patients in two different wards in hospital C during 1-year period. All the six strains of PFGE type 2 and two strains of type 6 were distributed sporadically in different wards in hospital C. All details about clinical characteristics and PFGE patterns of 41 CREL isolates from hospital C was shown in Table 4. However, all pulsotypes were single isolates in hospital B. Two strains of PFGE type 4 were isolated from patients in ICU in hospital A within 1 year; two strains of type 5 were isolated from patients in two different wards within 2 months; while two strains of type 7 were isolated from patients in hepatobiliary surgery within 1 month (data not shown).
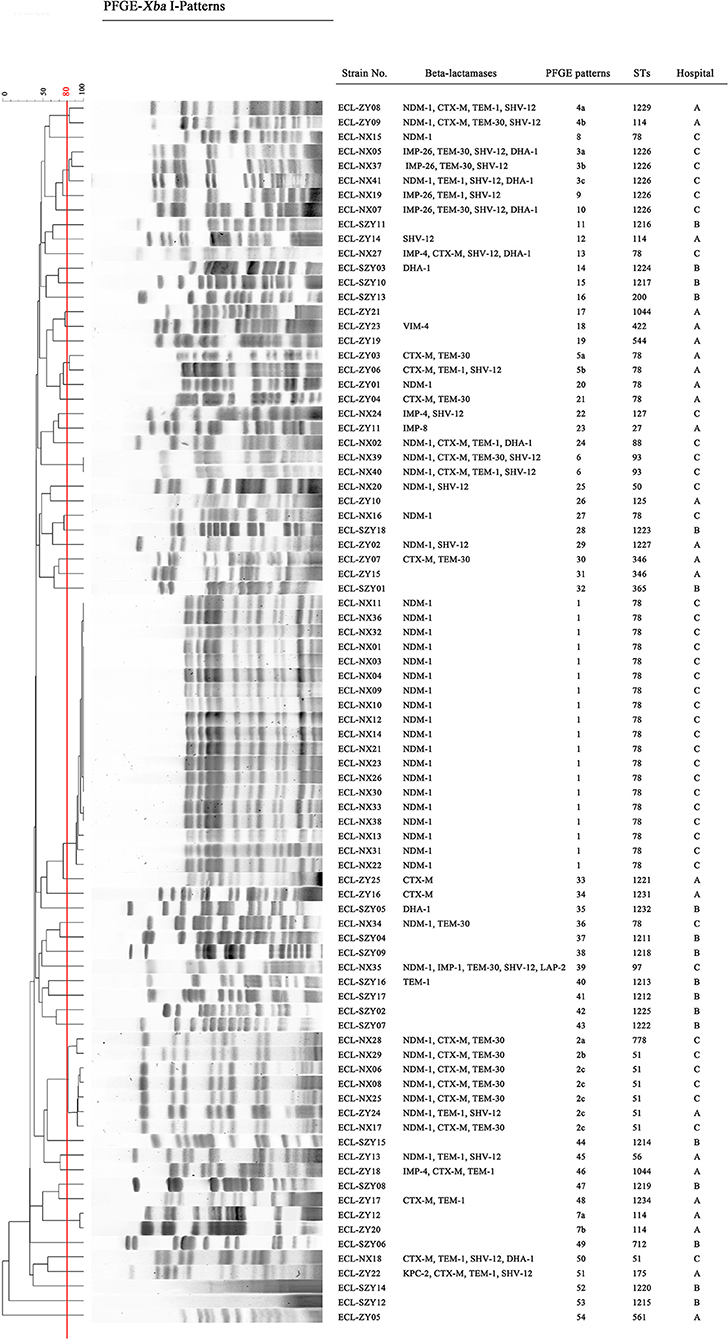
Figure 1. Dendrogram of the PFGE profiles of CREL isolates. The Dice coefficient was used to identify different types with a cutoff of 80.0% similarity. Hospital A, First Affiliated Hospital of Sun Yat-sen University, Guangdong, China; Hospital B, Guangdong Provincial Hospital of Chinese Medicine, Guangdong, China; Hospital C, Ningxia Hospital of Ningxia Medical University, Ningxia, China.
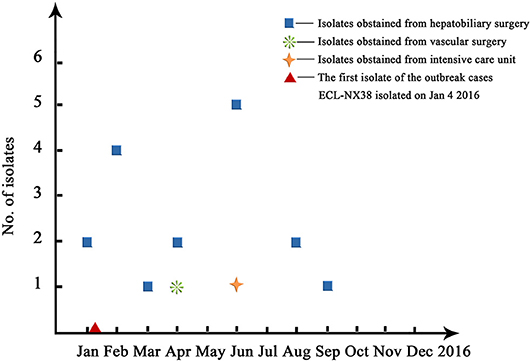
Figure 2. An overall time distribution of predominated ST78 clones in Hospital C in the year of 2016.
MLST Analysis
MLST distinguished 42 STs including 22 novel STs (ST1211-ST1229, ST1231, ST1232, and ST1234). The most prevalent ST was ST78 (n = 27, 32.1%), followed by ST51 (n = 7, 8.3%), ST1226 (n = 5, 6.0%), ST114 (n = 4, 4.8%), ST93 (n = 2, 2.4%), ST346 (n = 2, 2.4%), and ST1044 (n = 2, 2.4%). The remaining STs contained one isolate for each. MLST showed subtype diversity, which was relatively consistent with PFGE. As expected, PFGE was more discriminatory than MLST. Most isolates belonging to different subtypes of the same pulsotype had an identical ST. The only exceptions were one single-locus variant pairs (SLVs) (ST114 and ST1229) and five SLVs (ST51 and ST778), among which isolates of one ST were clustered into one of the pulsotypes of the other ST. Of the 42 STs, 33 (78.6%) were singletons, 6 (14.3%) had single SLVs and 3 (7.1%) clustered into a bigger group within CC114. The ST114-centered group encompassed 6 isolates of 3 STs including ST114 (n = 4), ST1229 (n = 1), and ST1231 (n = 1), and isolates were all obtained from hospital A. Twenty-eight isolates from hospital A and C belonged to ST78 (n = 27) and ST1221 (n = 1) within CC74, while eight isolates represented ST51 (n = 7) and ST50 (n = 1) within CC234. Other single SLVs consisted of ST1044 and ST1228, indicating direct clonal evolution between the SLVs. All of the STs were analyzed by Grapetree in order to reveal their relationships (Figure 3). The ST78 clones of eight PFGE pulsotypes exhibited various β-lactamase profiles. The most prevailing type harboring NDM-1 belonged to ST78 and carried plasmids of two or three Inc types, including IncFIB, IncX3, and IncX4 (Supplementary Table 2). Plasmid-based replicon typing revealed that the IncX3 type epidemic plasmid carrying blaNDM−1 caused an outbreak of E. cloacae. These dominated clones carried several other β-lactamase genes, including blaACT−5 and blaOXA−1. However, ST78 E.cloacae isolates were not always associated with NDM-1. Three ST78 strains from hospital A produced different ESBLs but not NDM-1, and one ST78 E.cloacae from hospital C produced IMP-4. Each of these comprised various Inc types of plasmids. Six ST51 isolates with highly similar pulsotypes produced NDM-1-type MBL, and four isolates belonged to ST1226 diversified into three pulsotypes, were frequently related to IMP-26. Due to the deficiency of clinic case and limited data, all these observations were still to be further validated.
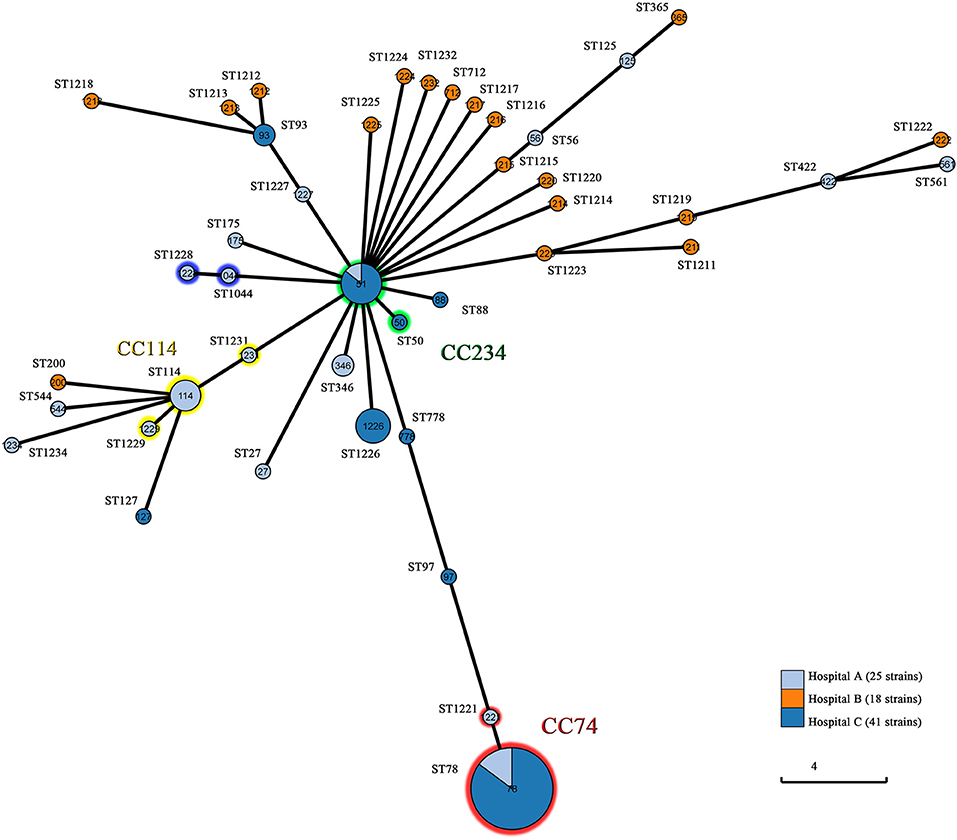
Figure 3. Population structure of the 84 CREL isolates by GrapeTree analysis. Each circle is a Node and each line is a branch. Node size is dependent on the number of strains within that node. Numbers in circles represent ST types. Branch length varies on the distance between nodes. Hospital A, First Affiliated Hospital of Sun Yat-sen University, Guangdong, China; Hospital B, Guangdong Provincial Hospital of Chinese Medicine, Guangdong, China; Hospital C, Ningxia Hospital of Ningxia Medical University, Ningxia, China.
Genetic Environments of NDM-1 or IMP Carrying Contigs
As shown in Figure 4, seven NDM-1-harboring plasmids had a common genetic structure, which was highly similar to some previous plasmids, such as pNDM-ECN49 (GenBank Accession No. KP765744). Compared with another epidemic plasmid pNDM-BJ01 in A. lwoffii (GenBank Accession No. JQ001791), however, a complete or partial IS5 supplanted the ISAba125 upstream of the blaNDM−1 gene. The blaNDM−1-like-carrying plasmids could be divided into two different types. Type A plasmids mainly differed by the insertion of a Tn3-like transposon downstream of groES. The Tn3-like transposon consisted of tnpA, tnpR, and two ORFs encoding hypothetical proteins, flanked by a pair of 38 bp inverted repeats (IR). The inverted repeat motifs of target site 6 bp at the boundaries of the Tn3-like element indicated insertion by transposition. However, IS91-like element, which probably transpose via a rolling-circle replication mechanism, was located downstream of groES among two plasmids of Type B.
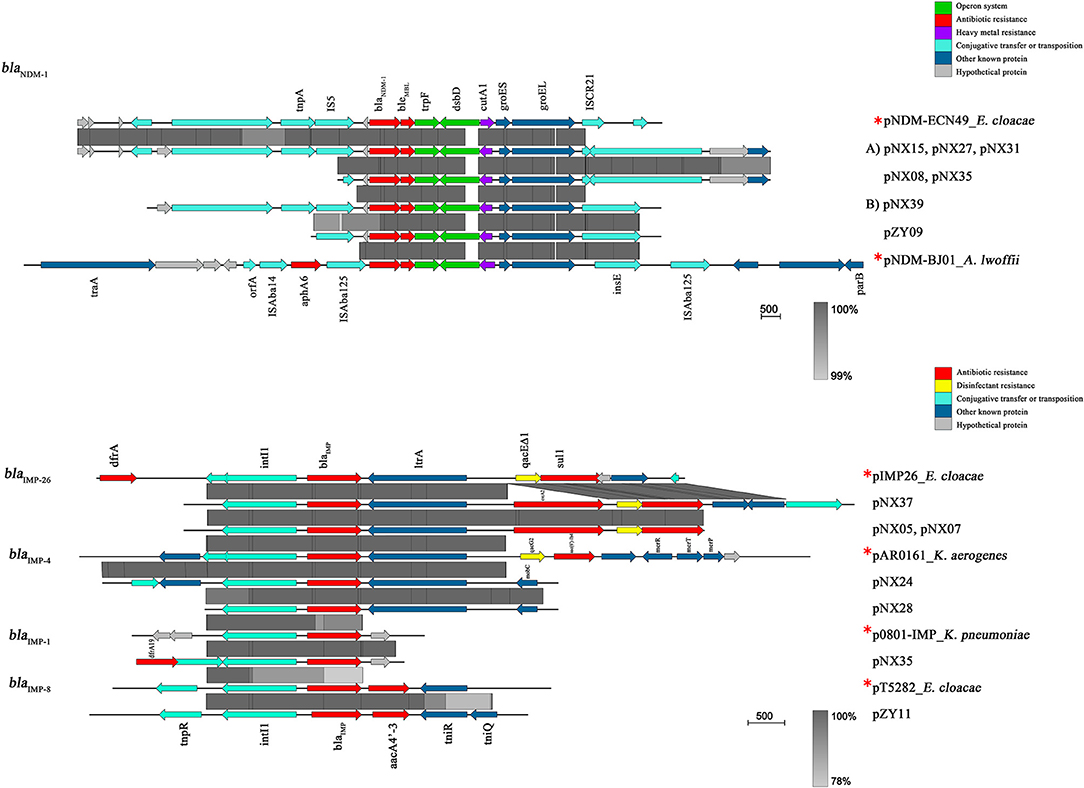
Figure 4. Linear comparison of the representative plasmid sequences carrying NDM-1 or IMP. *Reference plasmids were as following: pNDM-1-ECN49 in E. cloacae (GenBank Accession No. KP765744), pNDM-BJ01 in A. lwoffii (GenBank Accession No. JQ001791), pIMP26 in E. cloacae (GenBank Accession No. MH399264), pAR0161 in K. aerogenes (GenBank Accession No. MF344574), p0801-IMP in K. pneumoniae (GenBank Accession No. KT345947), and pT5282 in E. cloacae (GenBank Accession No. MF344574). Block arrows indicate confirmed or putative open reading frames (ORFs) and their orientations. Arrow size is proportional to the predicted ORF length. Regions of homology are marked by gray shading. The color code is as follows: red, antibiotic resistance genes; purple, heavy metal resistance genes; yellow, disinfectant-resistance genes; green, operon; cyan, genes associated with the transfer; dark blue, other known genes; hypothetical and unknown genes are represented by light-gray arrows.
All of the blaIMP genes were situated within class 1 integrons. The blaIMP−8 gene was identified in In655 containing blaIMP−8-aacA4′-3, which was previously detected in E. cloacae (GenBank Accession No. MF344574). Three genetic mutations in coding region of intl1 (A38978C, G38958C, and T38953C) were observed in the integron mediated IMP-8. The integron harboring blaIMP−26 contained the gene cassette array: blaIMP−26-ereA2–qacEΔ1/sul1, and was detected in 3 isolates. The integron had a structurally similar cassette to pIMP26 in E. cloacae (GenBank Accession No. MH399264), but the difference in ereA2 gene insertion resulted in erythromycin resistance. Integrons of blaIMP−4 gene had a similar genetic structure to pAR0161 (GenBank Accession No. MF344574), and 3′-CS qacEΔ1-sul1 were also observed downstream. However, we were unable to sequence the complete integron-related gene cassettes due to the limitations of short-read sequencing. The blaIMP−1 gene was determined in a shorter contig accompanied with a partial aacA4 gene downstream. The sequence alignment revealed that one synonymous mutation (T39C) were observed, compared with the widely reported sequence of blaIMP−1 gene (GenBank Accession No. MG287118). This resultant sequence was almost completely consistent with the partial published sequence in K. oxytoca p7121-IMP (GenBank Accession No. KX784502.1).
Discussion
E. cloacae is frequently implicated in serious healthcare-associated infections, with a high proportion of carbapenemase producers among carbapenem-resistant Enterobacteriaceae (Davin-Regli and Pagès, 2015). E. cloacae has been reported to acquire carbapenemases including KPC-2 (Andrade et al., 2018), NDM-1 (Torres-González et al., 2015), IMP-4 (Lee et al., 2017b), IMP-26 (Dai et al., 2013), IMP-8 (Pang et al., 2016), IMP-1 (Aoki et al., 2018), and VIM-4 (Sonnevend et al., 2017). Our study demonstrated the high prevalence of carbapenemase among carbapenem-resistant E. cloacae (CREL) in south and northwest of China (59.5%). MBLs, especially NDM-1 was, the dominant mechanism of resistance to carbapenems in E. cloacae in China. Most cases of infections or colonizations of NDM-1-producing bacteria originated in southern Asia, most commonly in India (Walsh and Toleman, 2011). The different prevalence of NDM-1 between countries might be explained by its high genetic transfer rate among unrelated bacterial species. Meanwhile, IMP-type MBL has been identified as the second common carbapenemase (10.7%). Compared to the worldwide distribution of IMP-4 (Leung et al., 2013; Lee et al., 2017b) and IMP-8 (Wang et al., 2015; Pang et al., 2016), IMP-26 and IMP-1 were also detected in our study. Although KPC or VIM-producing E. cloacae has been reported to spread rapidly in the last decade (Kanamori et al., 2017; Sonnevend et al., 2017; Daniels et al., 2018), the prevalence remained relatively low in our study. Moreover, the carriage rate of ESBL-related genes among CREL isolates was high (42.9%), though no dominant ESBL type was identified. Our study showed that 83.3% of the isolates continuously produced high AmpC β-lactamase. ACT-type inducible AmpC enzyme remained conservative. DHA-type, however which has become the most prevalent plasmid-mediated AmpC β-lactamase, was less frequently described in the literature with regard to E. cloacae. These additional β-lactamases might explain a slight increase of carbapenem resistance among CREL isolates (Souna et al., 2014). Of note, two MBLs, NDM-1 and IMP-1, were firstly identified to be co-expressed in E. cloacae, which was isolated from a patient after cardiac surgery in Ningxia. This XDR E. cloacae isolate co-harbored blaSHV−12, blaTEM−30, blaLAP−2, blaACT−5, qnrS1, aac(6')-IIc, aadA6, aph(3')-Ia, aph(6)-Id, catA2, sul1, and dfrA10. Resistance genes existed in multiple incompatibility groups, including IncN, IncHI2A, and IncHI2, which could further aggravate the dissemination under the selective pressure of antibiotics (Supplementary Table 2).
Besides, the absence or reduced expression of porins coupled with ESBL and/or AmpC overexpression, could lead to increased carbapenem MICs (Babouee Flury et al., 2016). In our study, most isolates had decreased membrane permeability caused by low expression of ompC or ompF, or both. Most isolates exhibited down-regulation of ompF, but up-regulation of ompC. These results were consistent with the previous reports and indicated that the decreased expression of ompF might lead to the potential role of OmpC-directed OM protein polarization (Majewski et al., 2016). Combinations of either ESBL or AmpC and porin loss played a pivotal role especially among carbapenemase-negative isolates. These isolates had lost at least one porin, among which 91.2% (31/34) of the ioslates produced different types of β-lactamase. Analysis of ompC expression showed a significant decrease among isolates only non-susceptible to imipenem. The decrease or loss of OmpC, a smaller pore size membrane porin compared to that of OmpF, might be correlated with the slightly increase in imipenem MIC (Lavigne et al., 2012). Hence, porin loss combined with ESBL and/or AmpC overexpression was one of the major mechanisms of drug resistance. Carbapenemase, ESBL and/or AmpC hyperproduction plus porin loss usually resulted in extensively high carbapenem MICs.
In this study, we observed significantly differential distribution and molecular characteristics of CREL isolates in two geological regions. In Ningxia, China, MBLs, especially NDM-1, was identified in the highest proportion, indicating a great potential risk of spread of drug-resistant strains in northwest of China. However, lower prevalence of carbapenemase producers has been described in Guangdong, China during our investigation, and that approximately half of isolates showed intermediate resistance to one or two carbapenems. This phenomenon might be mainly due to additional β-lactamases and impaired permeability. No carbapenemase producer was found in hospital B (Guangdong Provincial Hospital of Chinese Medicine, Guangdong, China). The possible interpretation was that carbapenems might be utilized less frequently in these hospitals. In most cases, patients with infections caused by CREL usually suffer from critical basic diseases, lower immune function, and all kinds of invasive operations, and they may be less inclined to TCM hospitals. Another explanation, probably different genetic events among E. cloacae isolates have occurred over time in TCM hospitals and carbapenemase genes was very rare or unique. Besides, about other mechanisms, especially high level of AmpC β-lactamases plus porin loss, and overexpression of efflux pumps, would play a more important part in carbapenem resistance among these E. cloacae isolates. Therefore, a variety of mechanisms could induce resistance in varying degrees in the process of drug resistance in E. cloacae.
The most susceptible antimicrobials to CREL isolates was amikacin (94.0%), followed by tigecycline (86.9%), and polymyxin B (82.1%). Amikacin, an aminoglycoside group antibiotic, has been restricted in recent years due to its side effects such as nephrotoxicity and ototoxicity (Wargo and Edwards, 2014). Polymyxin B and tigecycline are currently the most active therapeutic option for carbapenem-resistant Enterobacteriaceae including CREL (Rafailidis and Falagas, 2014). However, polymyxin B and tigecycline resistance has sporadically occurred in recent years (Kumar, 2016; Karaiskos et al., 2017). We found 13 isolates resistant to polymyxin B and 11 isolates non-susceptible to tigecycline, but mcr-1 and tetX were not detected. Resistance to polymyxins might be associated with alterations in the lipopolysaccharide structure and overexpression of efflux pumps (Lim et al., 2010). Besides, ribosome protection and excessive expression of efflux pumps might contribute to decreased susceptibility to tigecycline (Pournaras et al., 2016). Remarkably, polymyxin B heterogeneous subpopulations were detected in two isolates, indicating that more consideration should be taken to heterogeneously colistin resistant E. cloacae.
Our results suggested that NDM-1-harboring plasmids contained a highly conserved region around blaNDM−1 (blaNDM−1-bleMBL-trpF-dsbD-cutA1-groES-groEL), which might be involved in the further spread of blaNDM−1. The highly similar genetic structure was also obtained from various NDM-1-encoding plasmids, such as C. freudii from China (Yang et al., 2015) and K. pneumoniae from Australia (Wailan et al., 2016). More importantly, the region flanking blaNDM−1 was highly homologous to some Acinetobacter spp. isolated from China (Bogaerts et al., 2013). Recent studies proposed that the acquisition of blaNDM−1 in Enterobacteriaceae might be derived from Acinetobacter spp. via horizontal transmission of drug-resistant plasmids (Bogaerts et al., 2013). These findings suggested that the potential dissemination of acquired blaNDM−1 gene among different bacterial species had become an emerging global threat. IMP-type MBL was located within a variety of integrons. Similar to previous studies, IMP-producing E. cloacae presented a diverse genomic environment due to the transfer and rearrangement of plasmids and integrons in all probability (Aoki et al., 2018). Different genetic events might occur among E. cloacae isolates and other isolates, leading to the diversity of integrons in our study. The presence of various integrons associated with plasmids could facilitate horizontal or clonal transmission among bacteria of different genera and species through conjugation.
PFGE revealed that 84 CREL isolates possessed 54 pulsotypes. Nineteen NDM-1-producing E. cloacae isolates obtained from Ningxia had a single dominant pulsotype. These predominated clones have obtained fast transmission in hepatobiliary surgery, vascular surgery, and ICU during a peak period (from Jan. 2016 to Sep. 2016), which might be the evidence of intra-hospital clonal dissemination. The further examining revealed that all outbreak strains were ST78, belonging to clonal complex CC74, which was considered as the most versatile genetic lineages among the world (Izdebski et al., 2015). The uniform genetic background among NDM-1-producing E. cloacae with conjugative IncX3 plasmids showed horizontal transmission between epidemic isolates. These results suggested that IncX3-type plasmids might contribute significantly to the outbreak of blaNDM−1 within E. cloacae in China. Previous studies have reported that the IncX3-type plasmid was a serious threat, particularly because of the global spread of NDM-1-producing Enterobacteriaceae spp (Yang et al., 2015). The outbreak strains producing NDM-1 co-harbored multiple resistance genes, including blaACT−5, blaOXA−1, sul1, dfrA15b, mphA, catB3, arr3 as well as the qnrA1 and aac(6')Ib-cr genes encoding quinolone resistance. Studies have reported the emergency and spread of E. cloacae ST120, ST74, ST418, and ST88 producing NDM-1 in China (Liu et al., 2015; Jia et al., 2018; Jin et al., 2018). This implied that the blaNDM−1 gene might have been transmitted to multiple clones of E. cloacae in many parts of China. To the extent of our knowledge, this is the first identification of an outbreak of blaNDM−1 harboring E. cloacae ST78 isolates in China. Our study suggested that ST78 has become a successful hospital-related clone with a unique ability to accept plasmids for further dissemination in China. ST78 was one of the leading clonal lineages with increased epidemic potential, which might be associated with the spread of carbapenem resistance (Miyoshi-Akiyama et al., 2013). In summary, we reported that CREL isolates were still distributed popularly in Guangdong, China during our investigation. However, we emphasized the spread of NDM-1-producing E. cloacae ST78 with contribution of IncX3 plasmids in Ningxia, China. The outbreak has provided a new model for the dissemination of the blaNDM−1-haboring E. cloacae in China. Therefore, epidemiological surveillance of resistance is vital and should be performed routinely, especially in high-risk departments.
MLST distinguished 42 STs with ST78 (32.1%), ST51 (8.3%), ST1226 (6.0%), and ST114 (4.8%), and being the predominant STs. Previous studies revealed that ST66, ST78, ST108, and ST114 were the most prevalent and widespread E. cloacae STs (Izdebski et al., 2015). Our study showed that 36.9% (31/84) of the isolates belonged to the potentially high-risk international clones, with ST78 prevailing. We found that E. cloacae ST78 producing NDM-1 with a higher epidemic potential might be more prone to cause severe drug-resistant outbreaks. The ST78 clones demonstrated various β-lactamase profiles. Early studies also indicated a lack of strict correlation between β-lactamase profiles and ST78. The ST78 E. cloacae, originally identified in Japan, was a major international clone. The ST78 clones was widely distributed and independently obtained plasmids with β-lactamase genes, making specific pulsotypes more suitable for further spread (Miyoshi-Akiyama et al., 2013). In addition, seven ST51 isolates belonged to CC234, one of dominant clonal groups with high prevalence and wide distribution. Four ST114 isolates were diversified into multiple pulsotypes and caused some sporadic isolates. As the central genotype of CC114, ST114 has a wide global distribution formerly detected in Japan, France, Italy, Spain, Greece and Israel, mainly associated with blaCTX−M−15 (Izdebski et al., 2015). Moreover, we identified a novel ST type, ST1226 detected in 5 isolates with highly similar pulsotypes, and might be related to the carriage of blaIMP−26 in our study. Strikingly, we described in detail one E. cloacae ST97, which co-harbored blaNDM−1 and blaIMP−1 as well as multiple resistance genes.
Compared with carbapenem-resistant K. pneumoniae (CRKP), our study demonstrated that NDM-1-type MBL was the most commonly identified in E. cloacae. Recent surveillance data in China indicated that NDM was predominant in E. coli and E. cloacae, whereas KPC appeared to be most common in K. pneumoniae (Wang et al., 2018). Most previous studies revealed that the substantial burden of KPC-type carbapenemase mainly lied in North America, Latin America and Europe (Munoz-Price et al., 2013). However, NDM-1-mediated carbapenem resistance was widespread in Asia, such as India, Pakistan, and China (Nordmann et al., 2012). In contrast to the close connection between ST258/ST11 and blaKPC in K. pneumoniae (Liu et al., 2018), blaNDM−1 apparently has a higher association with IncX3-type plasmids regardless of ST type in E. cloacae. Diverse clones of NDM-1-carrying E. cloacae have been widely distributed in geography. Evidence showed that clonal expansion was the cause of most transmitted cases. Thus the rapid spread of NDM-1-producing E. cloacae has comprised one of the greatest challenges to global health.
This study had several limitations. Our collection may not represent the prevalence and evolution of carbapenem-resistant E. cloacae in China. Our study lacks a comprehensive analysis of clinical risk factors. We were unable to determine complete genetic structures owing to the limitation of short-read sequencing. Long-read sequencing techniques would provide more detailed analysis of integron structures and plasmid backbones.
In conclusion, our study revealed that carbapenemase, ESBL and/or AmpC overexpression combined with porin loss were the primary mechanisms responsible for carbapenem resistance. Moreover, we initially reported the nosocomial outbreak caused by NDM-1-producing E. cloacae ST78 in Northwest China. Therefore, it emphases a critical concern to monitor and control the further spread of NDM-1 in China.
Author Contributions
BH, LC, and Y-WT designed the study. YC, XY, KLa, MZ, XM, and YH carried out the experiments. CC, WJ, JZ, KLi, PG, and WZ analyzed the data. YC wrote the manuscript.
Funding
The study was supported in part by the National Natural Science Foundation of China (grants 81572058, 81672081, 81772249, 81871703), the US National Institutes of Health Cancer Center Support Grant (grant P30 CA008748), the Guangdong Natural Science Foundation (grant 2014A030313143), and the State Education Ministry Scientific Research Foundation for the Returned Overseas Chinese Scholars.
Conflict of Interest Statement
The authors declare that the research was conducted in the absence of any commercial or financial relationships that could be construed as a potential conflict of interest.
Acknowledgments
This thesis is supported by the China-America Carbapenem-Resistant Enterobacteriaceae Consortium (CACREC). We thank all the hospitals that provided carbapenem-resistant E. cloacae isolates, including First Affiliated Hospital of Sun Yat-sen University, Guangdong Provincial Hospital of Chinese Medicine, and Ningxia Hospital of Ningxia Medical University.
Supplementary Material
The Supplementary Material for this article can be found online at: https://www.frontiersin.org/articles/10.3389/fmicb.2019.01610/full#supplementary-material
References
Aguirre-Quiñonero, A., Cano, M. E., Gamal, D., Calvo, J., and Martínez-Martínez, L. (2017). Evaluation of the carbapenem inactivation method (CIM) for detecting carbapenemase activity in enterobacteria. Diagn. Microbiol. Infect. Dis. 88, 214–218. doi: 10.1016/j.diagmicrobio.2017.03.009
Andrade, L. N., Siqueira, T. E. S., Martinez, R., and Darini, A. L. C. (2018). Multidrug-resistant CTX-M-(15, 9, 2)- and KPC-2-producing Enterobacter hormaechei and Enterobacter asburiae isolates possessed a set of acquired heavy metal tolerance genes including a chromosomal sil operon (for acquired silver resistance). Front. Microbiol. 9:539. doi: 10.3389/fmicb.2018.00539
Aoki, K., Harada, S., Yahara, K., Ishii, Y., Motooka, D., Nakamura, S., et al. (2018). Molecular characterization of IMP-1-producing Enterobacter cloacae complex isolates in Tokyo. Antimicrob. Agents Chemother. 62:e02091-17. doi: 10.1128/AAC.02091-17
Babouee Flury, B., Ellington, M. J., Hopkins, K. L., Turton, J. F., Doumith, M., Loy, R., et al. (2016). Association of novel nonsynonymous single nucleotide polymorphisms in ampD with cephalosporin resistance and phylogenetic variations in ampC, ampR, ompF, and ompC in Enterobacter cloacae isolates that are highly resistant to carbapenems. Antimicrob. Agents Chemother. 60, 2383–2390. doi: 10.1128/AAC.02835-15
Bankevich, A., Nurk, S., Antipov, D., Gurevich, A. A., Dvorkin, M., Kulikov, A. S., et al. (2012). SPAdes: a new genome assembly algorithm and its applications to single-cell sequencing. J. Comput. Biol. 19, 455–477. doi: 10.1089/cmb.2012.0021
Biendo, M., Canarelli, B., Thomas, D., Rousseau, F., Hamdad, F., Adjide, C., et al. (2008). Successive emergence of extended-spectrum beta-lactamase-producing and carbapenemase-producing Enterobacter aerogenes isolates in a university hospital. J. Clin. Microbiol. 46, 1037–1044. doi: 10.1128/JCM.00197-07
Bogaerts, P., Huang, T. D., Rezende de Castro, R., Bouchahrouf, W., and Glupczynski, Y. (2013). Could Acinetobacter pittii act as an NDM-1 reservoir for Enterobacteriaceae? J. Antimicrob. Chemother. 68, 2414–2415. doi: 10.1093/jac/dkt201
Chen, S., Zhou, Y., Chen, Y., and Gu, J. (2018). fastp: an ultra-fast all-in-one FASTQ preprocessor. Bioinformatics 34, i884–i890. doi: 10.1093/bioinformatics/bty560
Dai, W., Sun, S., Yang, P., Huang, S., Zhang, X., and Zhang, L. (2013). Characterization of carbapenemases, extended spectrum beta-lactamases and molecular epidemiology of carbapenem-non-susceptible Enterobacter cloacae in a Chinese hospital in Chongqing. Infect. Genet. Evol. 14, 1–7. doi: 10.1016/j.meegid.2012.10.010
Daniels, J. B., Chen, L., Grooters, S. V., Mollenkopf, D. F., Mathys, D. A., Pancholi, P., et al. (2018). Enterobacter cloacae complex ST-171 isolates expressing KPC-4 carbapenemase recovered from canine patients in Ohio, USA. Antimicrob. Agents Chemother. 62:e01161-18. doi: 10.1128/AAC.01161-18
Davin-Regli, A., and Pagès, J. M. (2015). Enterobacter aerogenes and Enterobacter cloacae; versatile bacterial pathogens confronting antibiotic treatment. Front. Microbiol. 6:392. doi: 10.3389/fmicb.2015.00392
Izdebski, R., Baraniak, A., Herda, M., Fiett, J., Bonten, M. J., Carmeli, Y., et al. (2015). MLST reveals potentially high-risk international clones of Enterobacter cloacae. J. Antimicrob. Chemother. 70, 48–56. doi: 10.1093/jac/dku359
Jacoby, G. A. (2009). AmpC beta-lactamases. Clin. Microbiol. Rev. 22, 161–182. doi: 10.1128/CMR.00036-08
Jia, X., Dai, W., Ma, W., Yan, J., He, J., Li, S., et al. (2018). Carbapenem-resistant E. cloacae in Southwest China: molecular analysis of resistance and risk factors for infections caused by NDM-1-producers. Front Microbiol. 9:658. doi: 10.3389/fmicb.2018.00658
Jin, C., Zhang, J., Wang, Q., Chen, H., Wang, X., Zhang, Y., et al. (2018). Molecular characterization of carbapenem-resistant Enterobacter cloacae in 11 Chinese cities. Front. Microbiol. 9:1597. doi: 10.3389/fmicb.2018.01597
Kanamori, H., Parobek, C. M., Juliano, J. J., van Duin, D., Cairns, B. A., Weber, D. J., et al. (2017). A prolonged outbreak of KPC-3-producing Enterobacter cloacae and Klebsiella pneumoniae driven by multiple mechanisms of resistance transmission at a large academic burn center. Antimicrob. Agents Chemother. 61:e01516-16. doi: 10.1128/AAC.01516-16
Karaiskos, I., Souli, M., Galani, I., and Giamarellou, H. (2017). Colistin: still a lifesaver for the 21st century? Expert Opin. Drug Metab. Toxicol. 13, 59–71. doi: 10.1080/17425255.2017.1230200
Kelly, A. M., Mathema, B., and Larson, E. L. (2017). Carbapenem-resistant Enterobacteriaceae in the community: a scoping review. Int. J. Antimicrob. Agents 50, 127–134. doi: 10.1016/j.ijantimicag.2017.03.012
Kumar, M. (2016). Colistin and tigecycline resistance in carbapenem-resistant enterobacteriaceae: checkmate to our last line of defense. Infect. Control Hosp. Epidemiol. 37, 624–625. doi: 10.1017/ice.2016.31
Lavigne, J. P., Sotto, A., Nicolas-Chanoine, M. H., Bouziges, N., Bourg, G., Davin-Regli, A., et al. (2012). Membrane permeability, a pivotal function involved in antibiotic resistance and virulence in Enterobacter aerogenes clinical isolates. Clin. Microbiol. Infect. 18, 539–545. doi: 10.1111/j.1469-0691.2011.03607.x
Lee, J. H., Bae, I. K., Lee, C. H., and Jeong, S. (2017a). Molecular characteristics of first IMP-4-producing Enterobacter cloacae sequence type 74 and 194 in Korea. Front. Microbiol. 8:2343. doi: 10.3389/fmicb.2017.02343
Lee, J. Y., Hong, Y. K., Lee, H., and Ko, K. S. (2017b). High prevalence of non-clonal imipenem-nonsusceptible Enterobacter spp. isolates in Korea and their association with porin down-regulation. Diagn. Microbiol. Infect. Dis. 87, 53–59. doi: 10.1016/j.diagmicrobio.2016.10.004
Leung, G. H., Gray, T. J., Cheong, E. Y., Haertsch, P., and Gottlieb, T. (2013). Persistence of related bla-IMP-4 metallo-beta-lactamase producing Enterobacteriaceae from clinical and environmental specimens within a burns unit in Australia - a six-year retrospective study. Antimicrob. Resist. Infect. Control 2:35. doi: 10.1186/2047-2994-2-35
Lim, L. M., Ly, N., Anderson, D., Yang, J. C., Macander, L., Jarkowski, A., et al. (2010). Resurgence of colistin: a review of resistance, toxicity, pharmacodynamics, and dosing. Pharmacotherapy 30, 1279–1291. doi: 10.1592/phco.30.12.1279
Liu, C., Qin, S., Xu, H., Xu, L., Zhao, D., Liu, X., et al. (2015). New Delhi metallo-beta-lactamase 1(NDM-1), the dominant carbapenemase detected in carbapenem-resistant Enterobacter cloacae from Henan Province, China. PLoS ONE 10:e0135044. doi: 10.1371/journal.pone.0135044
Liu, L., Feng, Y., Tang, G., Lin, J., Huang, W., Qiao, F., et al. (2018). Carbapenem-resistant isolates of the Klebsiella pneumoniae complex in Western China: the common ST11 and the surprising hospital-specific types. Clin. Infect. Dis. 67(Suppl_2), S263–S265. doi: 10.1093/cid/ciy662
Liu, Y. Y., Wang, Y., Walsh, T. R., Yi, L. X., Zhang, R., Spencer, J., et al. (2016). Emergence of plasmid-mediated colistin resistance mechanism MCR-1 in animals and human beings in China: a microbiological and molecular biological study. Lancet Infect. Dis. 16, 161–168. doi: 10.1016/S1473-3099(15)00424-7
Majewski, P., Wieczorek, P., Ojdana, D., Sienko, A., Kowalczuk, O., Sacha, P., et al. (2016). Altered outer membrane transcriptome balance with AmpC overexpression in carbapenem-resistant Enterobacter cloacae. Front. Microbiol. 7:2054. doi: 10.3389/fmicb.2016.02054
Miyoshi-Akiyama, T., Hayakawa, K., Ohmagari, N., Shimojima, M., and Kirikae, T. (2013). Multilocus sequence typing (MLST) for characterization of Enterobacter cloacae. PLoS ONE 8:e66358. doi: 10.1371/journal.pone.0066358
Munoz-Price, L. S., Poirel, L., Bonomo, R. A., Schwaber, M. J., Daikos, G. L., Cormican, M., et al. (2013). Clinical epidemiology of the global expansion of Klebsiella pneumoniae carbapenemases. Lancet Infect. Dis. 13, 785–796. doi: 10.1016/S1473-3099(13)70190-7
Nordmann, P., Poirel, L., and Dortet, L. (2012). Rapid detection of carbapenemase-producing Enterobacteriaceae. Emerg. Infect. Dis. 18, 1503–1507. doi: 10.3201/eid1809.120355
Pagani, L., Dell'Amico, E., Migliavacca, R., D'Andrea, M. M., Giacobone, E., Amicosante, G., et al. (2003). Multiple CTX-M-type extended-spectrum beta-lactamases in nosocomial isolates of Enterobacteriaceae from a hospital in northern Italy. J. Clin. Microbiol. 41, 4264–4269. doi: 10.1128/JCM.41.9.4264-4269.2003
Pang, F., Jia, X. Q., Song, Z. Z., Li, Y. H., Wang, B., Zhao, Q. G., et al. (2016). Characteristics and management of Enterobacteriaceae harboring IMP-4 or IMP-8 carbapenemase in a tertiary hospital. Afr. Health Sci. 16, 153–161. doi: 10.4314/ahs.v16i1.21
Pérez-Pérez, F. J., and Hanson, N. D. (2002). Detection of plasmid-mediated AmpC beta-lactamase genes in clinical isolates by using multiplex PCR. J. Clin. Microbiol. 40, 2153–2162. doi: 10.1128/JCM.40.6.2153-2162.2002
Poirel, L., Walsh, T. R., Cuvillier, V., and Nordmann, P. (2011). Multiplex PCR for detection of acquired carbapenemase genes. Diagn. Microbiol. Infect. Dis. 70, 119–123. doi: 10.1016/j.diagmicrobio.2010.12.002
Pournaras, S., Koumaki, V., Spanakis, N., Gennimata, V., and Tsakris, A. (2016). Current perspectives on tigecycline resistance in Enterobacteriaceae: susceptibility testing issues and mechanisms of resistance. Int. J. Antimicrob. Agents 48, 11–18. doi: 10.1016/j.ijantimicag.2016.04.017
Rafailidis, P. I., and Falagas, M. E. (2014). Options for treating carbapenem-resistant Enterobacteriaceae. Curr. Opin. Infect. Dis. 27, 479–483. doi: 10.1097/QCO.0000000000000109
Seemann, T. (2014). Prokka: rapid prokaryotic genome annotation. Bioinformatics 30, 2068–2069. doi: 10.1093/bioinformatics/btu153
Sonnevend, Á., Yahfoufi, N., Ghazawi, A., Jamal, W., Rotimi, V., and Pál, T. (2017). Contribution of horizontal gene transfer to the emergence of VIM-4 carbapenemase producer Enterobacteriaceae in Kuwait. Infect. Drug. Resist. 10, 469–478. doi: 10.2147/IDR.S149321
Souna, D., Amir, A. S., Bekhoucha, S. N., Berrazeg, M., and Drissi, M. (2014). Molecular typing and characterization of TEM, SHV, CTX-M, and CMY-2 beta-lactamases in Enterobacter cloacae strains isolated in patients and their hospital environment in the west of Algeria. Med. Mal. Infect. 44, 146–152. doi: 10.1016/j.medmal.2014.01.008
Sullivan, M. J., Petty, N. K., and Beatson, S. A. (2011). Easyfig: a genome comparison visualizer. Bioinformatics 27, 1009–1010. doi: 10.1093/bioinformatics/btr039
Tijet, N., Patel, S. N., and Melano, R. G. (2016). Detection of carbapenemase activity in Enterobacteriaceae: comparison of the carbapenem inactivation method versus the Carba NP test. J. Antimicrob. Chemother. 71, 274–276. doi: 10.1093/jac/dkv283
Torres-González, P., Bobadilla-Del Valle, M., Tovar-Calderón, E., Leal-Vega, F., Hernández-Cruz, A., Martínez-Gamboa, A., et al. (2015). Outbreak caused by Enterobacteriaceae harboring NDM-1 metallo-beta-lactamase carried in an IncFII plasmid in a tertiary care hospital in Mexico City. Antimicrob. Agents Chemother. 59, 7080–7083. doi: 10.1128/AAC.00055-15
Tzouvelekis, L. S., Markogiannakis, A., Psichogiou, M., Tassios, P. T., and Daikos, G. L. (2012). Carbapenemases in Klebsiella pneumoniae and other Enterobacteriaceae: an evolving crisis of global dimensions. Clin. Microbiol. Rev. 25, 682–707. doi: 10.1128/CMR.05035-11
Villa, J., Viedma, E., Brañas, P., Orellana, M. A., Otero, J. R., and Chaves, F. (2014). Multiclonal spread of VIM-1-producing Enterobacter cloacae isolates associated with In624 and In488 integrons located in an IncHI2 plasmid. Int. J. Antimicrob. Agents 43, 451–455. doi: 10.1016/j.ijantimicag.2014.02.006
Wailan, A. M., Paterson, D. L., Kennedy, K., Ingram, P. R., Bursle, E., and Sidjabat, H. E. (2016). Genomic characteristics of NDM-producing Enterobacteriaceae isolates in Australia and their blaNDM genetic contexts. Antimicrob. Agents Chemother. 60, 136–141. doi: 10.1128/AAC.01243-15
Walsh, T. R., and Toleman, M. A. (2011). The new medical challenge: why NDM-1? Why Indian? Expert Rev. Anti Infect. Ther. 9, 137–141. doi: 10.1586/eri.10.159
Wang, J. T., Wu, U. I., Lauderdale, T. L., Chen, M. C., Li, S. Y., Hsu, L. Y., et al. (2015). Carbapenem-nonsusceptible Enterobacteriaceae in Taiwan. PLoS ONE 10:e0121668. doi: 10.1371/journal.pone.0121668
Wang, Q., Wang, X., Wang, J., Ouyang, P., Jin, C., Wang, R., et al. (2018). Phenotypic and genotypic characterization of carbapenem-resistant Enterobacteriaceae: data from a longitudinal large-scale CRE study in China (2012-2016). Clin. Infect. Dis. 67(Suppl_2), S196–S205. doi: 10.1093/cid/ciy660
Wang, Y., Lo, W. U., Lai, R. W., Tse, C. W., Lee, R. A., Luk, W. K., et al. (2017). IncN ST7 epidemic plasmid carrying blaIMP-4 in Enterobacteriaceae isolates with epidemiological links to multiple geographical areas in China. J. Antimicrob. Chemother. 72, 99–103. doi: 10.1093/jac/dkw353
Wargo, K. A., and Edwards, J. D. (2014). Aminoglycoside-induced nephrotoxicity. J. Pharm. Pract. 27, 573–577. doi: 10.1177/0897190014546836
Wozniak, A., Villagra, N. A., Undabarrena, A., Gallardo, N., Keller, N., Moraga, M., et al. (2012). Porin alterations present in non-carbapenemase-producing Enterobacteriaceae with high and intermediate levels of carbapenem resistance in Chile. J. Med. Microbiol. 61(Pt 9), 1270–1279. doi: 10.1099/jmm.0.045799-0
Yan, J. J., Ko, W. C., Chuang, C. L., and Wu, J. J. (2002). Metallo-beta-lactamase-producing Enterobacteriaceae isolates in a university hospital in Taiwan: prevalence of IMP-8 in Enterobacter cloacae and first identification of VIM-2 in Citrobacter freundii. J. Antimicrob. Chemother. 50, 503–511. doi: 10.1093/jac/dkf170
Yang, L., Wu, A. W., Su, D. H., Lin, Y. P., Chen, D. Q., and Qiu, Y. R. (2014). Resistome analysis of Enterobacter cloacae CY01, an extensively drug-resistant strain producing VIM-1 metallo-beta-lactamase from China. Antimicrob. Agents Chemother. 58, 6328–6330. doi: 10.1128/AAC.03060-14
Keywords: carbapenem-resistant, Enterobacter cloacae, outbreak investigation, NDM-1, IncX3 plasmids, ST78
Citation: Cai Y, Chen C, Zhao M, Yu X, Lan K, Liao K, Guo P, Zhang W, Ma X, He Y, Zeng J, Chen L, Jia W, Tang Y-W and Huang B (2019) High Prevalence of Metallo-β-Lactamase-Producing Enterobacter cloacae From Three Tertiary Hospitals in China. Front. Microbiol. 10:1610. doi: 10.3389/fmicb.2019.01610
Received: 01 December 2018; Accepted: 27 June 2019;
Published: 09 August 2019.
Edited by:
Benjamin Andrew Evans, University of East Anglia, United KingdomReviewed by:
Andres Felipe Opazo-Capurro, Universidad de Concepción, ChileWejdene Mansour, University of Sousse, Tunisia
Abdelaziz Touati, University of Béjaïa, Algeria
Copyright © 2019 Cai, Chen, Zhao, Yu, Lan, Liao, Guo, Zhang, Ma, He, Zeng, Chen, Jia, Tang and Huang. This is an open-access article distributed under the terms of the Creative Commons Attribution License (CC BY). The use, distribution or reproduction in other forums is permitted, provided the original author(s) and the copyright owner(s) are credited and that the original publication in this journal is cited, in accordance with accepted academic practice. No use, distribution or reproduction is permitted which does not comply with these terms.
*Correspondence: Wei Jia, MTM1MTkyOTkwOTBAMTI2LmNvbQ==; Yi-Wei Tang, dGFuZ3lAbXNrY2Mub3Jn; Bin Huang, aHVhbmdiM0BtYWlsLnN5c3UuZWR1LmNu
†These authors have contributed equally to this work