- 1IHU Méditerranée Infection, Marseille, France
- 2Aix-Marseille Université, IRD, MEPHI, IHU Méditerranée Infection, Marseille, France
- 3Département de Bactériologie-Virologie, Centre Hospitalier Universitaire de Montpellier, Montpellier, France
Background: Planctomycetes bacteria are known to be difficult to isolate, we hypothesized this may be due to missing iron compounds known to be important for other bacteria. We tested the growth-enhancement effect of complementing two standard media with Escherichia coli culture filtrate on two cultured strains of Gemmata spp. Also, the acquisition of iron by Gemmata spp. was evaluated by measuring various molecules involved in iron metabolism.
Materials and Methods: Gemmata obscuriglobus and Gemmata massiliana were cultured in Caulobacter and Staley’s medium supplemented or not with E. coli culture filtrate, likely containing siderophores and extracellular ferrireductases. We performed iron metabolism studies with FeSO4, FeCl3 and deferoxamine in the cultures with the E. coli filtrate and the controls.
Results and Discussion: The numbers of G. obscuriglobus and G. massiliana colonies on Caulobacter medium or Staley’s medium supplemented with E. coli culture filtrate were significantly higher than those on the standard medium (p < 0.0001). Agar plate assays revealed that the Gemmata colonies near E. coli colonies were larger than the more distant colonies, suggesting the diffusion of unknown growth promoting molecules. The inclusion of 10–4 to 10–3 M FeSO4 resulted in rapid Gemmata spp. growth (4–5 days compared with 8–9 days for the controls), suggesting that both species can utilize FeSO4 to boost their growth. In contrast, deferoxamine slowed down and prevented Gemmata spp. growth. Further studies revealed that the complementation of Caulobacter medium with E. coli culture filtrate and 10–4 M FeSO4 exerted a significant growth-enhancement effect compared with that obtained with Caulobacter medium supplemented with E. coli culture filtrate alone (p < 0.0122). Moreover, the intracellular iron concentrations in G. obscuriglobus and G. massiliana cultures in iron-depleted broth supplemented with the E. coli filtrate were 0.63 ± 0.16 and 0.78 ± 0.12 μmol/L, respectively, whereas concentrations of 1.72 ± 0.13 and 1.56± 0.11 μmol/L were found in the G. obscuriglobus and G. massiliana cultures grown in broth supplemented with the E. coli filtrate and FeSO4. The data reported here indicated that both E. coli culture filtrate and FeSO4 act as growth factors for Gemmata spp. via a potentiation mechanism.
Background
Bacteria of the genus Gemmata belong to the superphylum Planctomycetes–Verrucomicrobia–Chlamydia (PVC) and the phylum Planctomycetes (Wagner and Horn, 2006). Similarly to other members of Planctomycetes, Gemmata bacteria constitute one of the phylogenetically distinct major groups with increasing relevance to research in microbial ecology, molecular evolution, cell biology, and most recently, clinical microbiology (Fuerst, 2004; Drancourt et al., 2014; Aghnatios and Drancourt, 2016; van Niftrik and Devos, 2017). Indeed, some biologists now claim that Gemmata bacteria are nucleus−bearing prokaryotes but are considered evolutionary intermediates in the transition from prokaryote to eukaryote due to their amazingly complex cellular architectures that are typical of eukaryotes, such as those associated with cytosolic compartmentalization (Santarella-Mellwig et al., 2013; Sagulenko et al., 2014), sterol synthesis (Pearson et al., 2003; Gudde et al., 2019) and endocytosis-like macromolecular uptake (Lonhienne et al., 2010; Boedeker et al., 2017). These species form a remarkable gram-negative-staining group of bacteria that exhibit characteristic bud production, and a division process independent of FtsZ via budding-mediated polar fission, which is different from that of ordinary bacteria, where FtsZ is the main molecule involved in cell division (Fuerst, 2004; Bernander and Ettema, 2010). Both G. obscuriglobus and G. massiliana are slow-growing, fastidious organisms, and G. obscuriglobus exhibits a 13-h doubling time (Lee et al., 2009). Gemmata bacteria require highly specific culture media and long incubation times (Schlesner, 1994; Winkelmann and Harder, 2009; Lage and Bondoso, 2012; Mishek et al., 2018). We recently found some Gemmata-like sequences in blood collected from two patients with febrile aplastic neutropenia and leukemia, although we failed to isolate any Planctomycetes from these blood samples (Drancourt et al., 2014). Accordingly, conventional automated microbial detection of blood culture systems is not appropriate for the detection of these type of bacteria (undetected) and is less sensitive than the culture of mock-infected blood on Caulobacter agar (Christen et al., 2018). Nevertheless, the resistance of these bacteria to most of the routinely used antibiotics (Cayrou et al., 2010a; Godinho et al., 2019) and their recently demonstrated association with humans (Cayrou et al., 2013; Drancourt et al., 2014) support the potential behavior of Gemmata organisms as opportunistic pathogens, and this hypothesis warrants further investigations (Aghnatios and Drancourt, 2016).
The culture-based isolation of microbial pathogens remains the gold standard in diagnostic microbiological laboratories, but it has been reported that the lack of complex factors/conditions in these laboratories contributes to the inability to isolate some fastidious bacterial species. Accordingly, the provision of environmental and nutritional conditions similar to those existing in the natural habitat where yet-uncultured/refractory bacteria can be detected might be an option for their potential isolation and culture (Kaeberlein et al., 2002; Vartoukian et al., 2010). Some yet uncultured planctomycetes, such as Planctomycetes bekefii, possess stalks encrusted with iron oxide deposits (Schmidt et al., 1981), but the associated mechanism (active oxidation or passive deposition) has not been determined, and these findings suggest an important role for iron in these organisms. Our preliminary genome analysis of Gemmata obscuriglobus [UQM 2246 (GenBank: NZ_ABGO00000000.1)] and G. massiliana [GenBank: CBXA000000000.1], which are the only cultured representatives of the Planctomycetes genus Gemmata that have been formally described (Franzmann and Skerman, 1984; Aghnatios et al., 2015) using the Rapid Annotation Subsystem Technology server (Meyer et al., 2008), revealed that these bacteria do not contain molecules involved in the iron acquisition pathway, which might partially explain their notable fastidiousness when grown on culture media. We thus hypothesized that supplementation of the standard culture media (Caulobacter and Stanley) for Gemmata spp. with Escherichia coli culture filtrate and iron (“ecological” medium) could enhance their growth and isolation in clinical microbiology laboratories.
Materials and Methods
Bacterial Strains
Gemmata obscuriglobus DSM 5831T and G. massiliana DSM 26013T (CSUR P189T) were obtained from the Collection de Souches de l’Unité des Rickettsies (Marseille, France) and the German Collection of Microorganisms and Cell Cultures (Braunschweig, Germany). Both species were subcultured on Caulobacter medium DSMZ 595 or Staley’s maintenance medium DSMZ 629 prepared as described on the website1 The bacteria were grown through aerobic incubation on these solid media at 30°C for 7 to 14 days. The colonies was identified by matrix-assisted laser desorption/ionization time-of-flight mass spectrometry (MALDI-TOF-MS) analysis as previously described (Cayrou et al., 2010b).
Escherichia coli Culture Filtrate Preparation
Escherichia coli strain CIP 7624 (Collection de l’Institut Pasteur, Paris, France) was initially cultured on blood agar (BioMérieux, Marcy-l’Étoile, France) for 24 h at 37°C and identified by MALDI-TOF-MS as previously described (Seng et al., 2009). The bacterial cell counts were calibrated to 1012 colony forming units (CFUs)/mL using Kovas slide 10 (Hycor Biomedical, Germany) and microscopic examination. One milliliter of this suspension was then subcultured in 75-cm2 culture flasks containing 49 mL of autoclaved GLD medium (1 g of glucose, 1.4 g of peptone, 0.3 g of NaCl, 20 mL of Hutner’s salt (DSMZ 590), 10 mL of Staley vitamins (DSMZ 600, added after filter-sterilized) and 970 mL of distilled water) and incubated aerobically with shaking at 250 rpm for 2 days at 30°C to elicit the release of E. coli siderophores in a low-iron environment (Miethke and Marahiel, 2007). Sonication was performed to increase the release of E-coli siderophores, as previously described (Kwon and Jewett, 2015). Briefly, the cells were transferred to 1.5-mL microtubes and sonicated in a water bath sonicator (Bransonic® Ultrasonic Cleaner Model 5510R-MT, Branson Ultrasonic Corporation) at ∼20°C, a frequency of 20 kHz and an amplitude of 50% for 1 × 2 h. Subsequently, the sonication broth was filtered through a 0.2 μm filter (Sigma-Aldrich, Saint-Quentin-Fallavier, France) to obtain the E. coli filtrate named solution A. Solution B was prepared in the same manner as solution A with the exception that the GLD medium was supplemented with 10–4 M ferrous sulfate heptahydrate (Sigma-Aldrich) and the culture was incubated for 3 days and then filtered. Solution B was prepared with the aim of inducing the production of extracellular iron reductase by E. coli in an iron-rich environment. As a negative control, autoclaved noninoculated GLD medium was manipulated under the same conditions as the inoculated culture flasks. Finally, 10 μL of solution A, solution B and the control GLD medium were seeded in blood, Staley’s and Caulobacter solid agar to ensure sterility.
Culture of Gemmata spp. on Caulobacter and Staley’s Liquid Media With E. coli Filtrate
Gemmata obscuriglobus and G. massiliana were cultured independently in five replicates in a final volume of Caulobacter liquid medium of 15 mL. In detail, five tubes contained 9 mL of Caulobacter liquid medium supplemented with 5 mL of E. coli filtrate (2.5 mL of solution A + 2.5 mL of solution B), and five tubes contained 9 mL of Caulobacter liquid medium supplemented with 5 mL of GLD medium (negative controls). Each tube (five test tubes and five control tubes) was inoculated with 1 mL of 3.102 CFUs/mL suspended in sterile distilled water (Bio-Rad Laboratories, Hercules, CA, United States). Moreover, two test tubes and two control tubes were inoculated with 1 mL of sterile distilled water (noninoculated tubes) and manipulated in parallel to the negative control tubes. The preparations were then incubated at 30°C in an aerobic atmosphere for 7 days. At days 1, 2, 3, 4, and 7 postinoculation, each tube was shaken, and 1 mL of the broth was removed to obtain serial dilutions of 1, 1/10, 1/100, 1/1000, and 1/10000 in sterile distilled water for culture-based microbial enumerations. The CFUs were enumerated on 100-mm Petri dishes containing Caulobacter solid agar, and the colonies were counted using scanning software (ImageJ, Interscience, Saint-Nom-la-Bretèche, France). The means and standard errors were calculated at each time point (five replicates, n = 5). All experiments were reproduced independently with G. obscuriglobus in Staley’s liquid medium, and these were performed in parallel to those conducted with G. massiliana.
Culture of Gemmata spp. Under Iron-Repleted and Iron-Depleted Conditions in the Presence or Absence of E. coli Culture Filtrate, FeSO4, FeCl3 and Deferoxamine
Five experiments with Caulobacter liquid medium (Gemmata spp. grown in standard iron-free medium compared with Staley’s medium, which contains FeSO4) were performed independently. The iron metabolism in assay tubes containing Caulobacter liquid medium in the presence of E. coli culture filtrate under iron-repleted, iron-depleted and control conditions was studied. Ferrous iron heptahydrate (FeSO4●7H2O, Sigma Aldrich), ferric chloride (FeCl3, Sigma Aldrich) and deferoxamine mesylate (Desferal®, Novartis, Rueil-Malmaison, France) were used to probe iron assimilation. Each of these components was added to a final concentration of 10–4 M in a final volume of 15 mL (appropriately low concentrations of E. coli filtrate (5%), FeSO4 (0.2 M), FeCl3 (0.2 M) and deferoxamine (100 mg/mL), as determined by serial dilution of 0.2 M to 10–4 M; see Table 1 for the oxidation-reduction potential (ORP) and pH at 25°C obtained with all initial solutions used). In detail, the first tube contained 10–4 M FeSO4, the second tube contained 10–4 M FeCl3, the third tube contained 10–4 M deferoxamine, the fourth tube contained 10–4 M FeSO4 + 10–4 M deferoxamine, the fifth tube contained 10–4 M FeCl3 + 10–4 M deferoxamine dissolved in Caulobacter liquid medium, and the last tube contained only Caulobacter liquid medium. In parallel, six other tubes contained 9 mL of Caulobacter liquid medium supplemented with 5 mL of E. coli culture filtrate (2.5 mL of solution A + 2.5 mL of solution B), and each of these components was added to a final concentration of 10–4 M in a final volume of 15 mL, as described above. Subsequently, the 12 tubes were inoculated with 1 mL of 3 × 102 CFUs/mL suspended in Caulobacter liquid medium and incubated aerobically at 30°C for 7 days. One noninoculated (negative control) tube for each of the 12 tubes was manipulated in parallel. At days 1, 2, 3, 4, and 7 postinoculation, each tube was shaken, and 1 mL was removed to obtain serial dilutions of 1, 1/10, 1/100, 1/1000, and 1/10000 in distilled sterile water for culture-based CFU enumerations on Caulobacter solid agar. In addition, daily measurements of the ORP and pH at 25°C (accumet® AE150, Fisher Scientific) of each liquid medium were performed in parallel. Moreover, 2 × 50 μL of each liquid medium was adsorbed on blotting paper and deposited on solid medium in parallel to observe the growth time around the blotting paper. Furthermore, for each tube, 100 mm Petri dishes containing solid agar were prepared in parallel to monitor the growth on solid media (colony features, color and growth time in the presence or absence of E. coli filtrate), and these contained all the above-mentioned components at the same final concentrations. The Petri dishes prepared to contain E. coli filtrate were supplemented with 500 μL of solution A and 500 μL of solution B and dried at room temperature for 30 min in a laminar flow cabinet. The noninoculated (negative control) tubes and Petri dishes were manipulated in parallel. The bacteria were then counted using scanning software. G. massiliana and G. obscuriglobus were cultured independently in the same manner. The amount of intracellular iron was quantified after incubation for 1 and 7 days. Ten microliters of each liquid culture were inoculated on Caulobacter solid medium and Caulobacter solid medium complemented with each component as described above to monitor the bacterial features, survival and contamination. After incubation for 7 days, the liquid medium was centrifuged at 1.1 g for 5 min, and the pellet was washed three times with 10–4 M deferoxamine. The concentration of iron was measured using a colorimetric ferrozine method as previously described (Riemer et al., 2004). Briefly, 200 μL of 50 mM NaOH, 200 μL of 10 mM HCl and 200 μL of iron-releasing solution were added to the specimens, and the mixtures were incubated for 2 h at 60°C. All the solutions were then filtered through a 0.2-μm filter, and the iron concentration in a 350 μL aliquot was measured using an Iron 2 Cobas kit (Cobas, Meylan, France).
Gemmata spp. Growth on Petri Dishes in the Presence of a Panel of Molecules Involved in Iron Metabolism Absorbed in Sterile Blotting Paper
Live E. coli (soaked in sterile blotting paper, used as a helper strain) was cultured in close proximity to G. obscuriglobus and G. massiliana to assess its ability to promote the growth of these Gemmata bacteria. The growth of Gemmata spp. in the presence of E. coli filtrate (prepared in simple Caulobacter liquid medium) was then assessed through plate assays as previously described by D’Onofrio et al. (2010). Briefly, 2 × 50 μL of each solution containing various molecules involved in iron metabolism, namely, FeSO4, FeCl3, FeSO4 and deferoxamine at concentrations of 0.2, 10–1, 10–2, 10–3, and 10–4 M, with and without E. coli filtrate was triturated and adsorbed on blotting paper to study the influence of these components on Gemmata growth in Caulobacter medium through plate assays.
Results and Discussion
To the best of our knowledge, no member of Planctomycetes has been isolated from clinical samples, even though Planctomycetes bacteria have recently been detected in aplastic patients by PCR (Drancourt et al., 2014). This study aimed to develop an optimal medium for the culture and recovery of fastidious Gemmata bacteria in our laboratory using an “ecological” medium. Hence, this study was performed from a translational perspective for environmental/clinical microbiologists, and the results should not be translated to mechanistic studies conducted in clinical microbiology laboratories aiming to describe the iron metabolism of fastidious Gemmata.
Thus, we reasoned that the enhancement in the growth of Gemmata obscuriglobus and Gemmata massiliana obtained by supplementation with filtrates of E. coli cultures and iron at low concentrations (5% filtrates and 10–4 M FeSO4) reduce the doubling time of these fastidious bacteria potentially via a potentiation mechanism. Indeed, our observations revealed that although the noninoculated (negative) controls remained sterile throughout the experiments, the number of G. obscuriglobus colonies on Caulobacter medium supplemented with E. coli filtrate (126 ± 13 colonies on day 1 and 787 ± 38 colonies on day 7) was significantly higher than that on the standard medium (62 ± 10 colonies on day 1 and 261 ± 27 colonies on day 7) (p < 0.0001). Similarly, the number of G. obscuriglobus colonies on Staley’s medium supplemented with E. coli filtrate (75 ± 11 colonies on day 1 and 247 ± 20 colonies on day 7) was significantly higher than that on the standard medium (32 ± 6 colonies on day 1 and 82 ± 18 colonies on day 7) (p < 0.0001) (Figure 1). For G. massiliana, the number of colonies on the medium supplemented with E. coli filtrate (Caulobacter medium, 170 ± 29 colonies on day 1 and 694 ± 35 colonies on day 7; Staley medium, 74 ± 12 colonies on day 1 and 246 ± 21 colonies on day 7) was significantly higher than that on the standard medium (Caulobacter medium, 89 ± 11 colonies on day 1 and 329 ± 37 colonies on day 7, p < 0.0001; Staley medium, 54 ± 8 colonies on day 1 and 148 ± 17 colonies on day 7, p < 0.0001) (Figure 2). Altogether, a significantly higher number of Gemmata spp. colonies was obtained after enrichment of the reference culture medium with E. coli filtrate (p < 0.0001). Surprisingly, the growth of Gemmata spp. on Caulobacter medium supplemented with E. coli filtrate was improved compared with that on Staley’s medium supplemented with E. coli culture filtrate (Figures 1, 2), even though Staley’s medium contains more components such as Staley’s vitamins (see medium DSZM 600) and Hunter’s salts (see medium DSZM 590), which includes 99 mg/L FeSO4. These observations are consistent with the fact that many planctomycetes grow better in nutrient-poor (oligotrophic) medium (Staley, 1973; Schlesner, 1994). In addition, not all Staley vitamins are needed for optimal growth, as noted in a previous study (Mishek et al., 2018). To better understand the mechanism associated with the improvement in growth obtained with the addition of E. coli culture filtrate, iron-free Caulobacter medium (which contains less nutrients than Staley’s medium) was retained as the baseline for further study on iron acquisition by Gemmata spp. Indeed, this study was suggested to us by the marked diversity of Planctomycetes lineages, including Gemmata-Isosphaera, Planctomyces, Phycisphaerae, Pirellula-Rhodopirellula-Blastopirellula and the “OM190” lineage, detected in iron-hydroxide deposits in association with other bacteria that synthetize bacterioferritin, which captures and stores ferric iron. The high diversity of Planctomycetes in these microbial-rich environments contrasts with the restricted diversity of Planctomycetes in some other environments, which suggests the existence of an iron-based cooperation between ordinary bacteria such as Proteobacteria (E. coli live in the human gut in association with Gemmata spp., Cayrou et al., 2013) and members of Planctomycetes (van Niftrik and Jetten, 2012; Storesund and Øvreås, 2013). Consistent with this hypothesis agar plate assays revealed that the Gemmata colonies near E. coli colonies are larger than those farther from E. coli colonies, which suggests the diffusion of unknown molecules that serve as potential growth factors for Gemmata spp. (Figure 3). In addition, the impregnation of FeSO4 at concentrations ranging from 10–4 to 10–3 M in blotting paper or solid agar plates resulted in rapid Gemmata spp. growth around the nitrocellulose
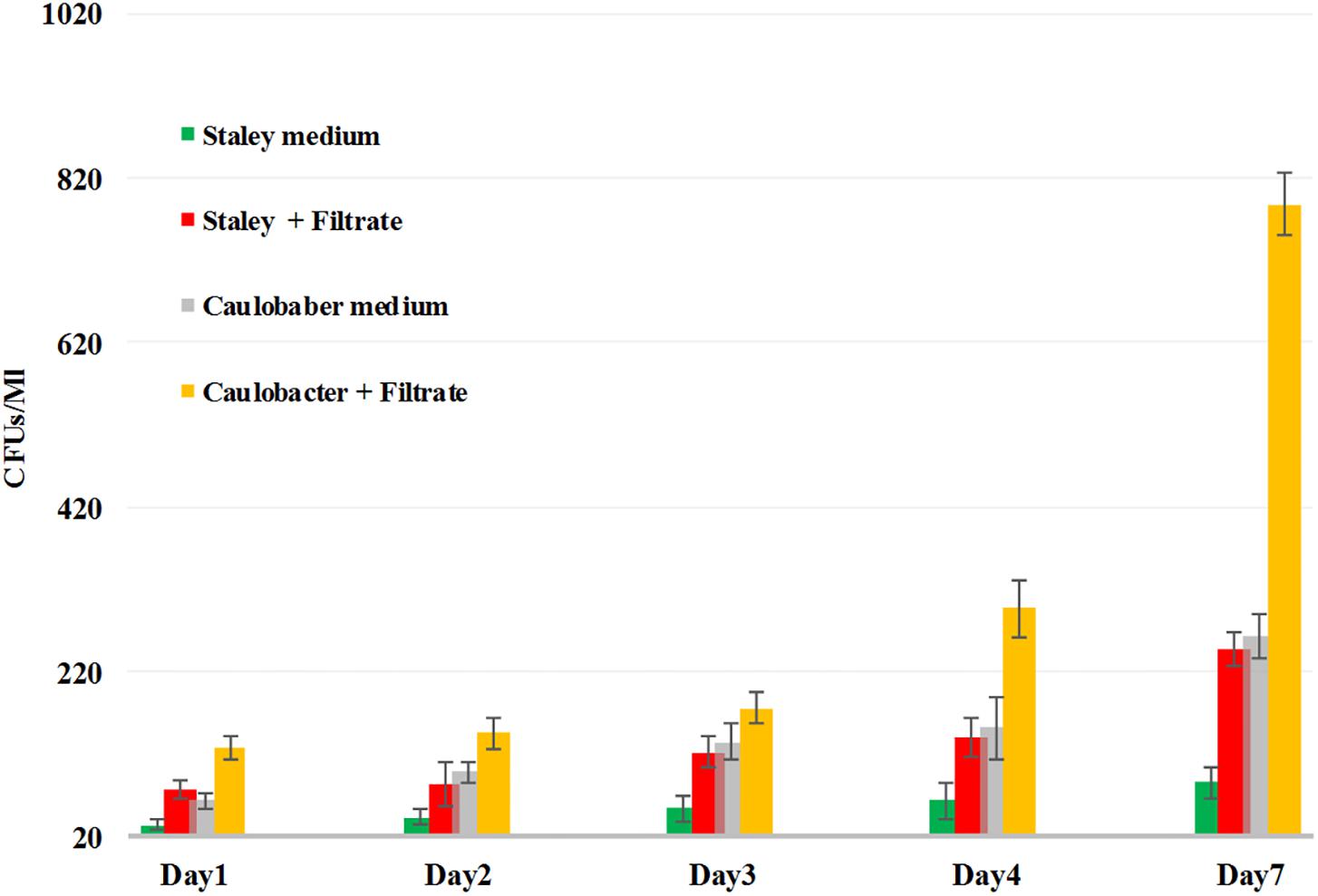
Figure 1. G. obscuriglobus growth in standard Caulobacter medium (gray bar), standard Staley medium (green bar), Caulobacter medium supplemented with E. coli filtrate (yellow bar) and Staley medium supplemented with E. coli filtrate (red bar). The number of G. obscuriglobus colonies per milliliter (Y axis) on solid agar medium was monitored over a 7-day period (X axis).
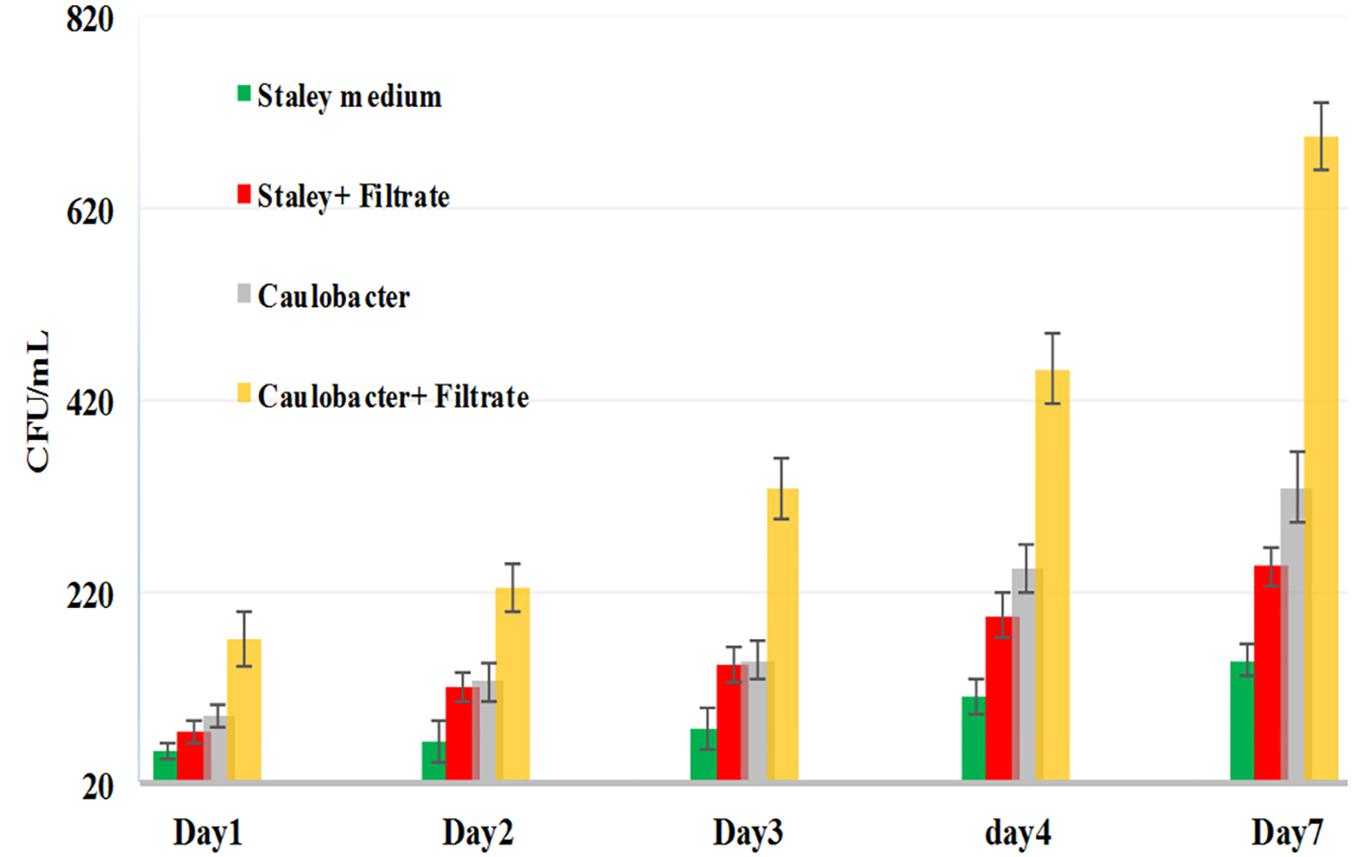
Figure 2. G. massiliana growth in standard Caulobacter medium (gray bar), standard Staley medium (green bar), Caulobacter medium supplemented with E. coli filtrate (yellow bar) and Staley medium supplemented with E. coli filtrate (red bar). The number of G. massiliana colonies per milliliter (Y axis) on solid agar medium was monitored over a 7-day period (X axis).
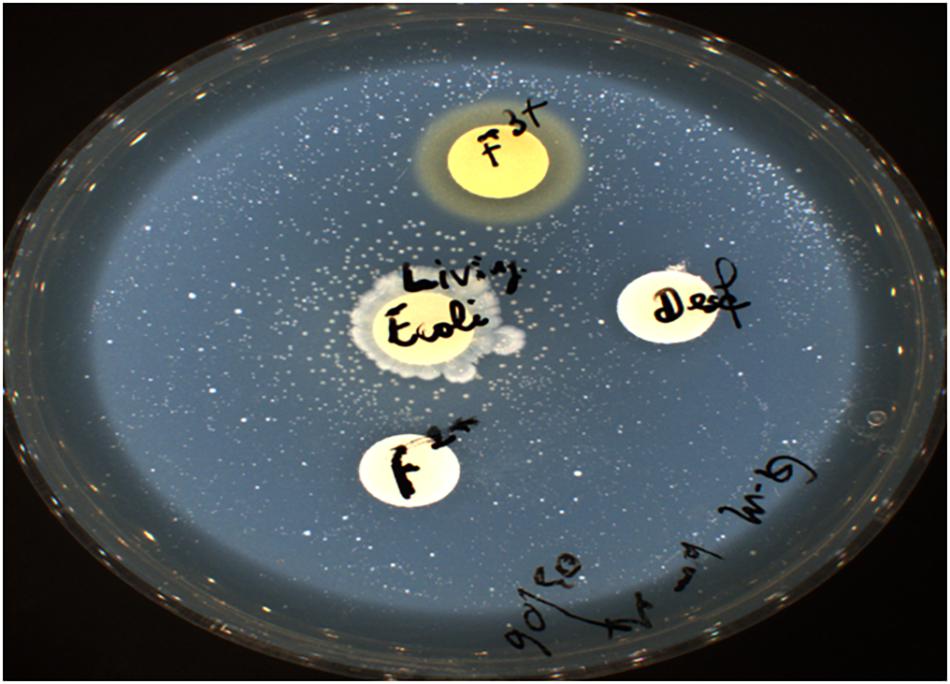
Figure 3. Live E. coli promotes the growth of Gemmata massiliana. Ferric and ferrous iron at 0.2 M are toxic to Gemmata, and 10–4 M deferoxamine prevents bacterial growth.
disks, which was detected on days 4 and 5 (Figure 4A), whereas small colonies did not begin to appear until days 8 and 9 in more distant areas of the disk (Figure 4B). This effect was observed with both Gemmata massiliana and Gemmata obscuriglobus, even though a more dramatic effect was obtained with Gemmata massiliana. This finding suggests that both species can use iron under aerobic conditions. FeSO4 at a concentration ranging from 10–4 to 10–3 M promotes greater Gemmata spp. growth than FeCl3 at the same concentration; however, 0.2 to 10–1 M FeCl3 and 0.2 to 10–1 M iron is toxic for both species. The finding that deferoxamine slows down and prevents the growth of Gemmata spp. suggests that iron improves Gemmata spp. growth, as indicated in Figures 5, 6.
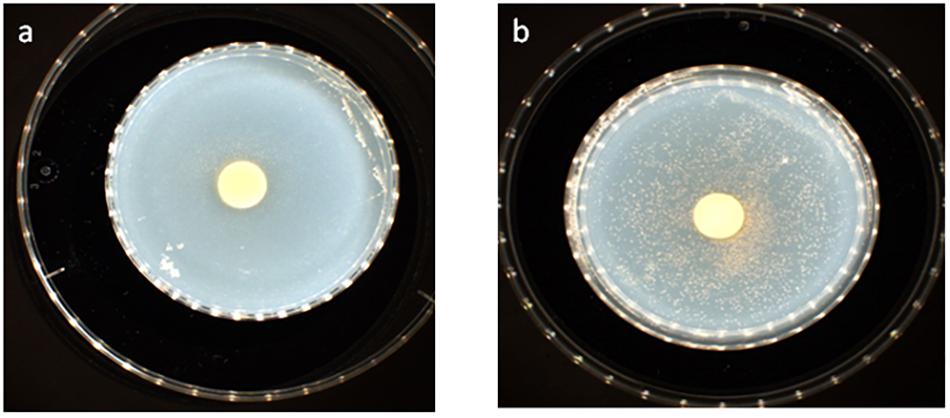
Figure 4. Gemmata massiliana showed improved growth in blotting paper impregnated with 10–3 M FeSO4 (50 mL) nitrocellulose disks at day 4 (a), whereas small colonies did not begin to appear until days 8 and 9 in more distant areas in the disks (b).
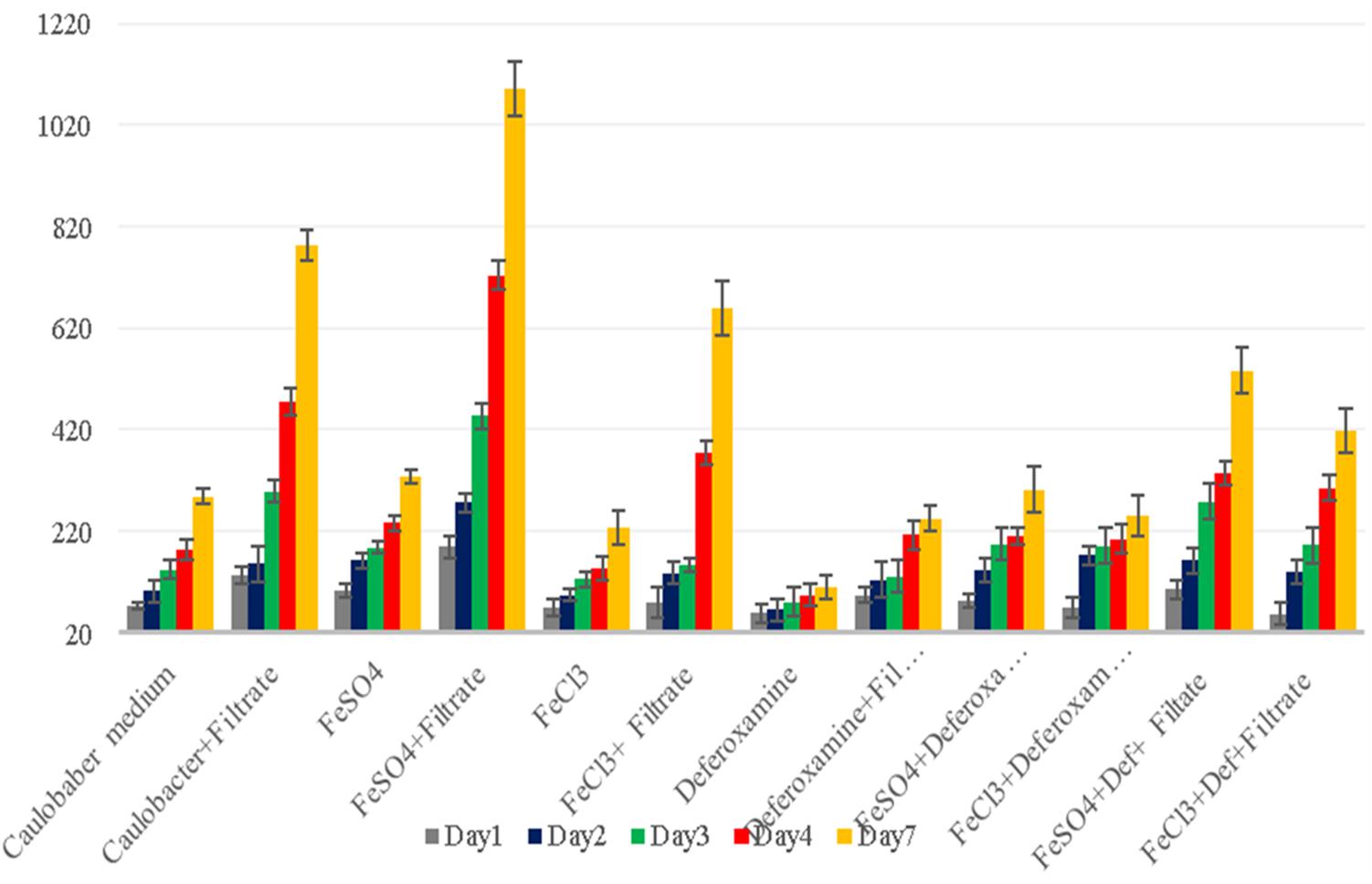
Figure 5. G. obscuriglobus growth in standard Caulobacter medium supplemented with E. coli filtrate, FeSO4, FeCl3, and deferoxamine and control medium. The number of G. obscuriglobus colonies per milliliter (Y axis) on solid agar medium was monitored over a 7-day period (X axis).
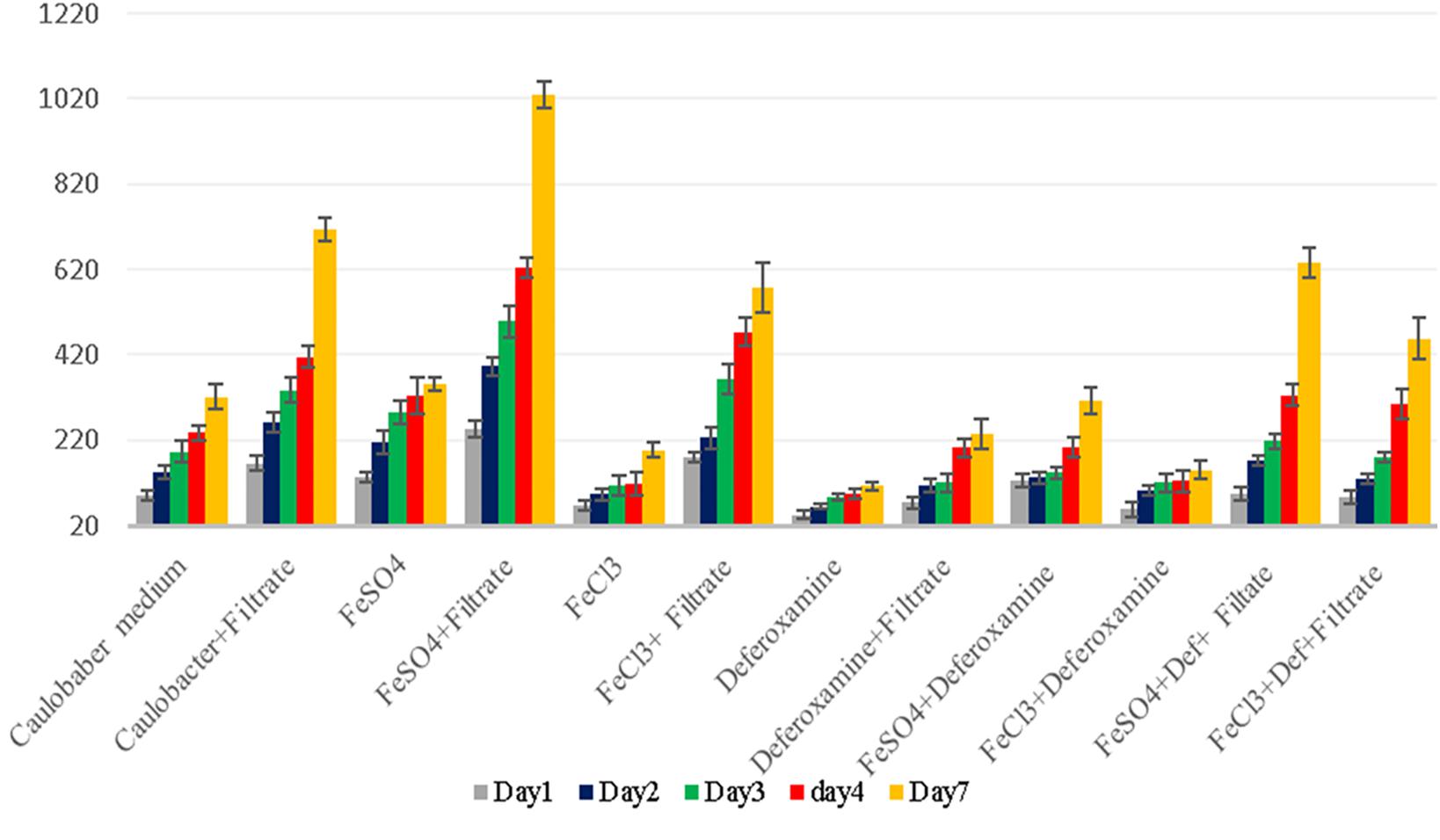
Figure 6. G. massiliana growth in standard Caulobacter medium supplemented with E. coli filtrate, FeSO4, FeCl3, and deferoxamine and control medium. The number of G. massiliana colonies per milliliter (Y axis) on solid agar medium was monitored over a 7-day period (X axis).
Iron is a trace metal involved in many crucial biological processes as components of metalloproteins and serves as a cofactor or structural element for enzymes needed for bacterial survival and growth (Schalk et al., 2011). Iron found in soil, sediments and, more rarely, ocean water (Andrews et al., 2003) is extracted from the environment and transported into a bacterial cell by siderophores, which are repressed in an iron-rich environment. Additionally, environmental ferric iron must be reduced into ferrous iron by extracellular bacterial reductase for assimilation by bacteria (Vartivarian and Cowart, 1999; Guan et al., 2001; Miethke and Marahiel, 2007; D’Onofrio et al., 2010). The ferric uptake regulator protein controls iron acquisition through the ferrous iron-mediated repression of iron-regulated promoters because an excess of intracellular iron induces the production of reactive oxygen species via the Fenton reaction (Escolar et al., 1999). Therefore, several bacteria lacking siderophores depend on other bacteria to provide them with iron (Reeves et al., 1983; Posey and Gherardini, 2000; D’Onofrio et al., 2010), which partly explains the fastidiousness of these bacteria when grown on a synthetic medium (D’Onofrio et al., 2010). Accordingly, our observations revealed that the complementation of Caulobacter medium with E. coli culture filtrate and 10–4 M FeSO4 exerted a high growth-enhancement effect (G. obscuriglobus, 189 ± 22 colonies on day 1 and 1,091 ± 53 colonies on day 7; G. massiliana, 248 ± 19 colonies on day 1 and 1,029 ± 32 colonies on day 7) compared with that obtained with Caulobacter medium supplemented with E. coli filtrate alone (G. obscuriglobus, 134 ± 17 colonies on day 1 and 783 ± 31 colonies on day 7, p < 0.0016; G. massiliana, 166 ± 18 colonies on day 1 and 713 ± 27 colonies on day 7, p < 0.0122) (Figures 5, 6). The intracellular iron concentrations in G. obscuriglobus and G. massiliana cultured in an iron-depleted broth supplemented with E. coli filtrate were 0.63 ± 0.16 μmol/L and 0.78 ± 0.12 μmol/L, respectively, whereas concentrations of 1.72 ± 0.13 and 1.56 ± 0.11 μmol/L were found in G. obscuriglobus and G. massiliana grown in broth supplemented with E. coli filtrate and FeSO4. Under the other culture conditions, the iron concentrations in G. obscuriglobus and G. massiliana were 0.66 ± 0.17 and 0.52 ± 0.14 μmol/L, respectively. Hence, the addition of E. coli culture filtrate was found to act as a growth-promoting factor, and this finding raises questions regarding the nature of unknown growth-promoting factors in E. coli culture filtrate that improve the iron metabolism in microbial communities (D’Onofrio et al., 2010). In contrast, some siderophores produced by certain bacteria, such as deferoxamine by Streptomyces, could slow down and inhibit the growth of Gemmata and lead to the inability to isolate these bacteria via chelating iron. As indicated in Figures 5, 6, E. coli culture filtrate might contain siderophores that have higher affinity for iron than deferoxamine, which suggests that E. coli siderophores are able to shift the balance between deferoxamine and iron and make iron more available for cell growth. Additionally, our experiments revealed the aerobic oxidation of ferrous iron (the color of the Caulobacter liquid medium turned from light yellow to a color similar to that of iron rust after the addition of 7.5 μL of iron (to obtain a concentration of 0.2 M) at neutral pH (7.24), and it is possible that E. coli filtrate contains certain molecules, such as the ferrireductase enzyme, that can reduce ferric iron to promote iron uptake, as shown in Figure 7. The pH and ORP measured for all the media over the 7-day experiment ranged from 7 to 6, which suggests that the predominant form of iron is ferric iron (Supplementary Figures S1, S2). Both species can adapt to various culture conditions, including iron-replete and iron-depleted conditions, and regulate the pH under neutral conditions.
The analysis of the features of the colonies on solid Caulobacter agar complemented or not completed with E. coli filtrate (500 μL of solution A and 500 μL of solution B), FeSO4, FeCl3 and deferoxamine showed that the colonies grown on iron-enriched Caulobacter broth were bigger and redder in color than the colonies grown under the other culture conditions, which were small and pale pink in color. Although this phenomenon was observed with both G. obscuriglobus and G. massiliana, the effect on G. obscuriglobus was more dramatic, and the growth times to achieve visible colony formation in the presence of E. coli filtrate and FeSO4 (5–7 days for G. obscuriglobus and 6–7 days for G. massiliana) were shorter than those in media supplemented by FeSO4 or FeCl3 without E. coli filtrate (8–9 days for G. massiliana). Additionally, the bacteria showed moderate growth on Caulobacter solid agar after preincubation in broth containing deferoxamine (Figures 5, 6). Moreover, a slight growth-enhancement effect was observed in medium supplemented with E. coli filtrate and 10–4 M FeCl3, which might suggest that the presence of Cl slowed Gemmata growth compared with the presence of sulfate in FeSO4 because planctomycetes possess many sulfatases.
These data suggest that in the environment, as well as in human microbiota, Gemmata organisms might rely on neighboring bacteria to obtain the required amount of ferrous iron. In contrast, axenic media limit the ability of Gemmata bacteria to acquire iron because ferrous iron oxidizes into ferric iron at pH higher than 5, which results in a very low amount of available ferrous iron in axenic media. However, the growth-enhancing effect of filtrate and iron supplementation on the two species might only be explained by a potentiation mechanism. These results are encouraging, but further studies are needed to identify the potential growth factors secreted by E. coli via their purification and freeze-drying and to thus define approaches for enriching planctomycetes culture media.
In conclusion, our results indicate that not only ferrous iron but also E. coli culture filtrate, as a source of unknown growth factors that promote the rapid growth of Gemmata species, enhance Gemmata growth and can thus be used to improve the empirical culture media for Planctomycetes, as illustrated for Gemmata species in this study. This strategy involving the design of specific culture media helped improve the culture of fastidious bacteria and allows researchers to design specialized media from an empirical medium. Similarly, future investigation of the nutrients required by Gemmata organisms might aid the design of new culture media for their recovery from both environmental samples and host microbiota (Drancourt et al., 2014).
Data Availability Statement
The raw data supporting the conclusions of this manuscript will be made available by the authors, without undue reservation, to any qualified researcher.
Author Contributions
OK and RA performed the experiments and drafted the manuscript. SG and MD interpreted the data and drafted the manuscript.
Funding
This work was supported by the French Government under the Investissements d’Avenir (Investments for the Future) program managed by the Agence Nationale de la Recherche (ANR, fr: National Agency for Research) (reference: Méditerranée Infection 10-IAHU-03). This work was supported by Région Provence Alpes Côte d’Azur and the European fund FEDER PA 0000319 IHUBIOTK.
Conflict of Interest
The authors declare that the research was conducted in the absence of any commercial or financial relationships that could be construed as a potential conflict of interest.
Acknowledgments
We acknowledge Saber Khelaifia, Safiatou Fall, and Marion Bonnet for the technical help provided. OK benefits from a Ph.D. grant provided by IHU Méditerranée Infection, Marseille, France. We acknowledge the contribution provided by Magdalen LARDIERE, who reviewed the English language of the manuscript.
Supplementary Material
The Supplementary Material for this article can be found online at: https://www.frontiersin.org/articles/10.3389/fmicb.2019.02552/full#supplementary-material
FIGURE S1 | Daily oxidoreduction potential (ORP) of Gemmata obscuriglobus. The Y axis represents the ORP value, and the X axis represents the day of measurement.
FIGURE S2 | Daily oxidoreduction potential (ORP) of Gemmata massiliana. The Y axis represents the ORP value, and the X axis shows the day of measurement.
Footnotes
References
Aghnatios, R., Cayrou, C., Garibal, M., Robert, C., Azza, S., Raoult, D., et al. (2015). Draft genome of Gemmata massiliana sp. nov, a water-borne Planctomycetes species exhibiting two variants. Stand. Genomic Sci. 10:120. doi: 10.1186/s40793-015-0103-0
Aghnatios, R., and Drancourt, M. (2016). Gemmata species: Planctomycetes of medical interest. Future Microbiol. 11, 659–667. doi: 10.2217/fmb-2015-11
Andrews, S. C., Robinson, A. K., and Rodríguez-Quiñones, F. (2003). Bacterial iron homeostasis. FEMS Microbiol. Rev. 27, 215–237. doi: 10.1016/s0168-6445(03)00055-x
Bernander, R., and Ettema, T. J. (2010). FtsZ-less cell division in archaea and bacteria. Curr. Opin. Microbiol. 13, 747–752. doi: 10.1016/j.mib.2010.10.005
Boedeker, C., Schüler, M., Reintjes, G., Jeske, O., van Teeseling, M. C. F., Jogler, M., et al. (2017). Determining the bacterial cell biology of Planctomycetes. Nat. Commun. 8:14853. doi: 10.1038/ncomms14853
Cayrou, C., Raoult, D., and Drancourt, M. (2010a). Broad-spectrum antibiotic resistance of Planctomycetes organisms determined by Etest. J. Antimicrob. Chemother. 65, 2119–2122. doi: 10.1093/jac/dkq290
Cayrou, C., Raoult, D., and Drancourt, M. (2010b). Matrix-assisted laser desorption/ionization time-of-flight mass spectrometry for the identification of environmental organisms: the Planctomycetes paradigm. Environ. Microbiol. Rep. 2, 752–760. doi: 10.1111/j.1758-2229.2010.00176.x
Cayrou, C., Sambe, B., Armougom, F., Raoult, D., and Drancourt, M. (2013). Molecular diversity of the Planctomycetes in the human gut microbiota in France and Senegal. APMIS 121, 1082–1090. doi: 10.1111/apm.12087
Christen, J.-R., Edmond, E., and Drancourt, M. (2018). Methods for detecting Gemmata spp. bacteremia in the microbiology laboratory. BMC Res. Notes 11:11. doi: 10.1186/s13104-017-3119-2
D’Onofrio, A., Crawford, J. M., Stewart, E. J., Witt, K., Gavrish, E., Epstein, S., et al. (2010). Siderophores from neighboring organisms promote the growth of uncultured bacteria. Chem. Biol. 17, 254–264. doi: 10.1016/j.chembiol.2010.02.010
Drancourt, M., Prebet, T., Aghnatios, R., Edouard, S., Cayrou, C., Henry, M., et al. (2014). Planctomycetes DNA in febrile aplastic patients with leukemia, rash, diarrhea, and micronodular pneumonia: FIG 1. J. Clin. Microbiol. 52, 3453–3455. doi: 10.1128/JCM.01207-14
Escolar, L., Pérez-Martín, J., and de Lorenzo, V. (1999). Opening the iron box: transcriptional metalloregulation by the Fur protein. J. Bacteriol. 181, 6223–6229.
Franzmann, P. D., and Skerman, V. B. D. (1984). Gemmata obscuriglobus, a new genus and species of the budding bacteria. Antonie Van Leeuwenhoek 50, 261–268. doi: 10.1007/BF02342136
Fuerst, J. A. (2004). Planctomycetes: a phylum of emerging interest for microbial evolution and ecology. WFCC Newslett. 38, 1–11.
Godinho, O., Calisto, R., Øvreås, L., Quinteira, S., and Lage, O. M. (2019). Antibiotic susceptibility of marine Planctomycetes. Antonie Van Leeuwenhoek 112, 1273–1280. doi: 10.1007/s10482-019-01259-7
Guan, L. L., Kanoh, K., and Kamino, K. (2001). Effect of exogenous siderophores on iron uptake activity of marine bacteria under iron-limited conditions. Appl. Environ. Microbiol. 67, 1710–1717. doi: 10.1128/aem.67.4.1710-1717.2001
Gudde, L. R., Hulce, M., Largen, A. H., and Franke, J. D. (2019). Sterol synthesis is essential for viability in the Planctomycete bacterium Gemmata obscuriglobus. FEMS Microbiol. Lett. 366:fnz019. doi: 10.1093/femsle/fnz019
Kaeberlein, T., Lewis, K., and Epstein, S. S. (2002). Isolating “uncultivable” microorganisms in pure culture in a simulated natural environment. Science 296, 1127–1129. doi: 10.1126/science.1070633
Kwon, Y.-C., and Jewett, M. C. (2015). High-throughput preparation methods of crude extract for robust cell-free protein synthesis. Sci. Rep. 5:8663. doi: 10.1038/srep08663
Lage, O. M., and Bondoso, J. (2012). Bringing Planctomycetes into pure culture. Front. Microbiol. 3:405. doi: 10.3389/fmicb.2012.00405
Lee, K.-C., Webb, R. I., and Fuerst, J. A. (2009). The cell cycle of the planctomycete Gemmata obscuriglobus with respect to cell compartmentalization. BMC Cell Biol. 10:4. doi: 10.1186/1471-2121-10-4
Lonhienne, T. G., Sagulenko, E., Webb, R. I., Lee, K.-C., Franke, J., Devos, D. P., et al. (2010). Endocytosis-like protein uptake in the bacterium Gemmata obscuriglobus. Proc. Natl. Acad. Sci. U.S.A. 107, 12883–12888. doi: 10.1073/pnas.1001085107
Meyer, F., Paarmann, D., D’Souza, M., Olson, R., Glass, E., Kubal, M., et al. (2008). The metagenomics RAST server – a public resource for the automatic phylogenetic and functional analysis of metagenomes. BMC Bioinformatics 9:386. doi: 10.1186/1471-2105-9-386
Miethke, M., and Marahiel, M. A. (2007). Siderophore-based iron acquisition and pathogen control. Microbiol. Mol. Biol. Rev. 71, 413–451. doi: 10.1128/mmbr.00012-07
Mishek, H. P., Stock, S. A., Florick, J. D. E., Blomberg, W. R., and Franke, J. D. (2018). Development of a chemically-defined minimal medium for studies on growth and protein uptake of Gemmata obscuriglobus. J. Microbiol. Methods 145, 40–46. doi: 10.1016/j.mimet.2017.12.010
Pearson, A., Budin, M., and Brocks, J. J. (2003). Phylogenetic and biochemical evidence for sterol synthesis in the bacterium Gemmata obscuriglobus. Proc. Natl. Acad. Sci. U.S.A. 100, 15352–15357. doi: 10.1073/pnas.2536559100
Posey, J. E., and Gherardini, F. C. (2000). Lack of a role for iron in the Lyme disease pathogen. Science 5471, 1651–1653. doi: 10.1126/science.288.5471.1651
Reeves, M. W., Pine, L., Neilands, J. B., and Balows, A. (1983). Absence of siderophore activity in Legionella species grown in iron-deficient media. J. Bacteriol. 154, 324–329.
Riemer, J., Hoepken, H. H., Czerwinska, H., Robinson, S. R., and Dringen, R. (2004). Colorimetric ferrozine-based assay for the quantitation of iron in cultured cells. Anal. Biochem. 331, 370–375. doi: 10.1016/j.ab.2004.03.049
Sagulenko, E., Morgan, G. P., Webb, R. I., Yee, B., Lee, K.-C., and Fuerst, J. A. (2014). Structural studies of Planctomycete Gemmata obscuriglobus support cell compartmentalisation in a bacterium. PLoS One 9:e91344. doi: 10.1371/journal.pone.0091344
Santarella-Mellwig, R., Pruggnaller, S., Roos, N., Mattaj, I. W., and Devos, D. P. (2013). Three-dimensional reconstruction of bacteria with a complex endomembrane system. PLoS Biol. 11:e1001565. doi: 10.1371/journal.pbio.1001565
Schalk, I. J., Hannauer, M., and Braud, A. (2011). New roles for bacterial siderophores in metal transport and tolerance. Environ. Microbiol. 13, 2844–2854. doi: 10.1111/j.1462-2920.2011.02556.x
Schlesner, H. (1994). The development of media suitable for the microorganisms morphologically resembling Planctomyces spp., pirellula spp., and other Planctomycetales from various aquatic habitats using dilute media. Syst. Appl. Microbiol. 17, 135–145. doi: 10.1016/S0723-2020(11)80042-1
Schmidt, J. M., Sharp, W. P., and Starr, M. P. (1981). Manganese and iron encrustations and other features of Planctomyces crassus Hortobágyi 1965, morphotype Ib of the Blastocaulis-Planctomyces group of budding and appendaged bacteria, examined by electron microscopy and X-ray micro-analysis. Curr. Microbiol. 5, 241–246. doi: 10.1007/bf01571155
Seng, P., Drancourt, M., Gouriet, F., La Scola, B., Fournier, P., Rolain, J. M., et al. (2009). Ongoing revolution in bacteriology: routine identification of bacteria by matrix-assisted laser desorption ionization time-of-flight mass spectrometry. Clin. Infect. Dis. 49, 543–551. doi: 10.1086/600885
Staley, J. T. (1973). Budding bacteria of the pasteuria – blastobacter group. Can. J. Microbiol. 19, 609–614. doi: 10.1139/m73-100
Storesund, J. E., and Øvreås, L. (2013). Diversity of Planctomycetes in iron-hydroxide 498 deposits from the Arctic Mid Ocean Ridge (AMOR) and description of Bythopirellula 499 goksoyri gen. nov., sp. nov., a novel Planctomycete from deep sea iron-hydroxide 500 deposits. Antonie Van Leeuwenhoek 104, 569–584. doi: 10.1007/s10482-013-0019-x
van Niftrik, L., and Devos, D. P. (2017). Planctomycetes-verrucomicrobia-chlamydiae bacterial superphylum: new model organisms for evolutionary cell biology. Front. Microbiol. 8:1458. doi: 10.3389/fmicb.2017.01458
van Niftrik, L., and Jetten, M. S. (2012). Anaerobic ammonium-oxidizing bacteria: unique microorganisms with exceptional properties. Microbiol. Mol. Biol. Rev. 76, 585–596. doi: 10.1128/MMBR.05025-11
Vartivarian, S. E., and Cowart, R. E. (1999). Extracellular iron reductases: identification of a new class of enzymes by siderophore-producing microorganisms. Arch. Biochem. Biophys. 364, 75–82. doi: 10.1006/abbi.1999.1109
Vartoukian, S. R., Palmer, R. M., and Wade, W. G. (2010). Strategies for culture of ‘unculturable’ bacteria: culturing the unculturable. FEMS Microbiol. Lett. 309, 1–7. doi: 10.1111/j.1574-6968.2010.02000.x
Wagner, M., and Horn, M. (2006). The Planctomycetes, Verrucomicrobia, Chlamydiae and sister phyla comprise a superphylum with biotechnological and medical relevance. Curr. Opin. Biotechnol. 17, 241–249. doi: 10.1016/j.copbio.2006.05.005
Keywords: Planctomycetes, Gemmata obscuriglobus, Gemmata massiliana, E. coli culture filtrate, iron, culture
Citation: Kaboré OD, Aghnatios R, Godreuil S and Drancourt M (2019) Escherichia coli Culture Filtrate Enhances the Growth of Gemmata spp. Front. Microbiol. 10:2552. doi: 10.3389/fmicb.2019.02552
Received: 01 April 2019; Accepted: 22 October 2019;
Published: 06 November 2019.
Edited by:
Yuji Morita, Meiji Pharmaceutical University, JapanReviewed by:
John A. Fuerst, The University of Queensland, AustraliaKaren Trchounian, Yerevan State University, Armenia
Copyright © 2019 Kaboré, Aghnatios, Godreuil and Drancourt. This is an open-access article distributed under the terms of the Creative Commons Attribution License (CC BY). The use, distribution or reproduction in other forums is permitted, provided the original author(s) and the copyright owner(s) are credited and that the original publication in this journal is cited, in accordance with accepted academic practice. No use, distribution or reproduction is permitted which does not comply with these terms.
*Correspondence: Michel DrancourtbWljaGVsLmRyYW5jb3VydEB1bml2LWFtdS5mcg==