- 1Microbiology Graduation Program, Department of Zoology and Botany, São Paulo State University (UNESP), São Paulo, Brazil
- 2Center for Fundamental and Applied Microbiomics, Biodesign Institute, Arizona State University, Tempe, AZ, United States
- 3Facultad de Agronomía, Instituto de Investigaciones Fisiológicas y Ecológicas Vinculadas a la Agricultura (IFEVA-CONICET), Universidad de Buenos Aires, Buenos Aires, Argentina
- 4Center for Nuclear Energy in Agriculture (CENA), University of São Paulo (USP), Piracicaba, Brazil
The last decade was marked by efforts to define and identify the main cyanobacterial players in biological crusts around the world. However, not much is known about biocrusts in Brazil’s tropical savanna (cerrado), despite the existence of environments favorable to their development and ecological relevance. We examined the community composition of cyanobacteria in biocrusts from six sites distributed in the Southeast of the country using high throughput sequencing of 16S rRNA and phylogenetic placement in the wider context of biocrusts from deserts. Sequences ascribable to 22 genera of cyanobacteria were identified. Although a significant proportion of sequences did not match those of known cyanobacteria, several clades of Leptolyngbya and Porphyrosiphon were found to be the most abundant. We identified significant differences in dominance and overall composition among the cerrado sites, much larger than within-site variability. The composition of cerrado cyanobacterial communities was distinct from those known in biocrusts from North American deserts. Among several environmental drivers considered, the opposing trend of annual precipitation and mean annual temperature best explained the variability in community composition within Brazilian biocrusts. Their compositional uniqueness speaks of the need for dedicated efforts to study the ecophysiology of tropical savanna biocrust and their roles in ecosystem function for management and preservation.
Introduction
Biological soil crusts (BSCs or biocrusts) are microbial assemblages present at the top soil of several terrestrial ecosystems, especially in drylands. Biocrusts are typically composed of cyanobacteria (Garcia-Pichel et al., 2001) but sometimes also eukaryotic algae, lichens, or mosses (Bates et al., 2010b) as primary producers, accompanied by a variety of chemotrophic bacteria (da Rocha et al., 2015), archaea (Soule et al., 2009), and fungi (Bates et al., 2010a). Biocrusts are ecologically important biotic components of arid lands (see reviews by Eldridge and Greene, 1994; Belnap et al., 2016), but they can occur in a variety of climatic regions, colonizing places where sunlight reaches the soil surface or even temperate areas subject to disturbance (Gundlapally and Garcia-Pichel, 2006; Elbert et al., 2009; Pointing and Belnap, 2012). Cyanobacteria contribute to important soil functions within biocrusts, providing stability and protection against erosive forces (Belnap, 2003, 2005) and are also responsible for carbon and nitrogen fixation, enriching soils with macronutrients (Johnson et al., 2007) and micronutrients (Beraldi-Campesi et al., 2009). These contributions of cyanobacteria to the ecosystem highlight the importance of understanding their composition and function worldwide.
However, a majority of the studies on cyanobacteria have focused in arid or semiarid areas in North America (Garcia-Pichel et al., 2013; Couradeau et al., 2016; Fernandes et al., 2018), China (Zhang et al., 2016), Australia (Delgado-Baquerizo et al., 2018), Spain (Williams et al., 2016), and the Middle East (Abed et al., 2010; Hagemann et al., 2015; Nejidat et al., 2016). The bundle-forming morphogenus Microcoleus seems to be dominant worldwide, even in areas where studies only used microscopy or clone libraries, such as Chile (Baumann et al., 2018) and the hyperarid Atacama Desert (Patzelt et al., 2014). Arid land biocrusts are usually first colonized by Microcoleus species, which stabilize the soil and start fixing carbon (Garcia-Pichel and Wojciechowski, 2009), enriching the soil and allowing heterocytous nitrogen-fixing cyanobacteria, usually Scytonema sp. and Tolypothrix sp. to colonize. Other common cyanobacteria present in arid land crusts include species of Nostoc, Calothrix, Chroococidiopsis, Leptolyngbya, Phormidium and Schizothrix. Whenever compositional studies have been carried out in biocrust from other climates, apparently differentiated communities were encountered (Pushkareva et al., 2015; Muñoz-Martín et al., 2019).
In Brazil, cyanobacterial biocrusts are found in both arid and semiarid areas in the Northeast of the country, but also in the savanna biome called cerrado. This biome represents about 2 million km2 or 23% of the country’s land surface, surpassed only by the Amazonian forest (Ratter et al., 1997), but its natural areas (anthropized areas – urban and agricultural – and water bodies excluded) cover approximated 1.24 million km2 (Sano et al., 2007). Some of cerrado phytophysiognomies are more favorable to crust occurrence due to the absence of a developed canopy and these non-forest formations are estimated to cover some 0.8 million km2, corresponding to 68% of the total cerrado area, according to Sano et al. (2007). The cerrado climate is classified as semi-humid tropical and is one of the most humid savanna regions in the world but with a severe dry season during April-September. Average annual precipitation varies between 800 and 2,000 mm, and average annual temperatures between 18 and 28°C (Eiten, 1982). Preliminary analyses by microscopy revealed the possibility of finding species not previously reported for biocrusts. Considering the absence of prior biocrust work on the cerrado, we undertook a survey of six sites to study their cyanobacterial components. The results of such an approach allowed us to compare the biodiversity and composition of the cyanobacterial assemblages of crusts from different environments (savanna vs. desert). In addition, the influence of selected environmental factors on composition within cerrado biocrusts was also evaluated. Our study provides a necessary foundation for more detailed studies and a relevant source of information for management and restoration practices of disturbed areas (Giraldo-Silva et al., 2018), particularly in view of their compositional uniqueness, which makes extrapolation of knowledge obtained from desert biocrust uncertain.
Materials and Methods
Sampling
Biocrusts were sampled in six sites distributed in four preserved areas in the SE region of Brazil (Supplementary Figure S1): Furnas do Bom Jesus São Paulo State Park of (one site), Vassununga São Paulo State Park (one site), Serra do Cipó National Park (two sites: Cipó and Capão), and Serra da Canastra National Park (two sites: Canastra and Zagaia) (Table 1). Ten sequential equidistant samples were collected throughout a 200 m long transect with two parallel transects set at each site, encompassing a total of 120 samples for the study (10 samples/transect × two transects/site × six sites). Soil crusts were collected with a Petri dish (55 mm × 1 cm high) and transported to the laboratory where they were kept dry at −20°C until processing.
Library Preparation and Illumina Sequencing of the 16S rRNA Gene
Soil grains, rocks, and organic matter (plant roots and leaves) were manually removed, and 1 mg of the sampled biocrust was used for DNA extraction with MoBio Powersoil kit (Laboratories Inc., Carlsbad, CA, USA) according to the manufacturer’s instructions. The region V3-V4 of 16S rRNA gene (420 bp) was amplified with CYA 359 and 781a/b primers as described in Nübel et al. (1997), with an overhang Illumina adapter included in the primers. The PCR reaction contained 10 ng of eDNA, 0.2 μM of each primer, and 1x KAPA HiFi HotStart Ready Mix (KAPA Biosystems) to 25 μl of final volume. The PCR product from the samples 1–3, 4–6, and 7–10 of each transect were pooled and purified using AMPure XP purification kit (Beckman Coulter Inc., Brea, CA, USA). Afterwards, Illumina sequencing adapters and dual-index barcodes were added to the amplicon target using the Nextera XT Index Kit (Illumina, USA), according to the manufacturer’s instructions. The product was purified using AMPure XP purification kit, quantified with Qubit Fluorometric Quantitation (Thermofisher/Life Technology, USA). The samples were normalized and, then, pooled in an equimolar fashion. The preparation of the samples followed the Illumina guidelines for sequencing in a MiSeq platform (Illumina) available at Center of Nuclear Energy and Agriculture (ESALQ/USP) and using MiSeq Reagent kit v3. 2 × 300 cycle.
Data Analysis Pipeline
The 16S rRNA gene forward and reverse sequences were paired using PANDAseq (Masella et al., 2012) and a fastq file was generated. The “QIIME 1.9” [Quantitative Insights into Microbial Ecology (Caporaso et al., 2010)] was used for further analyses. The script “multiple_split_libraries_fasq.py” was run without quality filtering, as quality filtering was done before pairing using Trimmomatic (Bolger et al., 2014). Next, the script “pick_open_reference_otus.py”, clustered reads into 97% self-similar operational taxonomic units (OTUs) using SUMAClust (Schloss, 2016) and SortMeRNA (Kopylova et al., 2012) in combination with the Greengenes 13_8 database (DeSantis et al., 2006). A filter was applied to the OTU table and only the OTUs that appeared in at least two samples were considered in the analyses. The OTU table also was used to do a rarefaction curve using “Chao1” and “goods_coverage” methods, through the script “alpha_diversity.py”, also in “QIIME 1.9”. All sequence datasets are publicly available through NCBI under the project “Diversity and ecology of cyanobacteria of biological soil crusts in Brazilian Savannah” (NCBI identification number: SRP137259).
Operational Taxonomic Unit Taxonomic Assignment
OTUs were identified by comparison with taxonomic information provided by public databanks. OTUs that presented more than 199 reads were first identified using QIIME (based on SortMeRNA and the Green Genes 13_8 database – DeSantis et al., 2006) and then compared one by one with GenBank data (NCBI nr/nt) using the tool “Basic Local Alignment Search Tool” (Baumann et al., 1990) to refine the initial identification. Cyanobacterial OTUs’ taxonomic assignment at the genus and species level was further informed through phylogenetic placement in the cyanobacterial reference database, Cydrasil (v. rc1, https://github.com/FGPLab/cydrasil). The Cydrasil rc1 database contains 1,161 curated cyanobacterial 16S rRNA gene sequences that are at least 1,100 bp long and includes a phylogenetic tree generated using RAxML 8 (Berger and Stamatakis, 2011). Query cyanobacterial sequences were aligned to the reference alignment with PaPaRa (Berger and Stamatakis, 2011), placed into the reference tree using the RaxML8 Evolutionary Placement Algorithm (Berger and Stamatakis, 2011) without changing the tree topology, and visualized on the iTOL 3 server (Letunic and Bork, 2016). This procedure allowed to relate an OTU sequence to a well-curated specific phylogenetic group or clade in a database that is enriched in cyanobacterial sequences from biocrusts, and thus confirm (or correct) the initial taxonomic identification. In general, OTUs that were 95% similar to an identified sequence (following Yarza et al., 2014) and placed in a highly supported and well-defined clade composed of coherently identified sequences were considered pertaining to the same genus. OTUs that presented less than 94% of similarity to the closest sequence were not identified. OTUs related to a single genus name but distributed in different clades (as the cases of the polyphyletic genera Leptolyngbya and Microcoleus) were considered to be effective genus-level taxa and given provisional identifiers (e.g., Leptolyngbya - Clade I, Leptolyngbya - Clade II…). To facilitate the visualization of the OTUs distribution and the composition of the sites, plots were constructed using the packages “ggplot2,” “scales” (Wickham, 2018a,b) and “reshape2” (Wickham, 2017) written in R language (R Core Team, 2018).
Comparison of the Taxonomic Composition Between Regions
A meta-analysis of a set of 15 libraries of bacterial 16S rRNA gene sequences of biocrusts from North American deserts (Table 2; Velasco Ayuso et al., 2017: NCBI identification numbers PRJNA343817; Fernandes et al., 2018: NCBI identification number PRJNA394792) was used for comparisons. These libraries had been constructed using primers (515F and 806R primers - Caporaso et al., 2012), whereas those in this work used CYA 359 and 781a/b (Nübel et al., 1997). Because of this, the quality-controlled and paired sequence files were merged into a single FASTA file and imported together into the QIIME 22018.2 for analyses. Sequences were clustered at 97% similarity using closed reference OTU picking with VSEARCH (Rognes et al., 2016) with the Green Genes 13_8 database (DeSantis et al., 2006) providing the reference sequences. The resulting OTU table was filtered to only include cyanobacterial sequences. OTUs were then aligned using Mafft (Katoh and Standley, 2013) and a phylogenetic tree was generated using FastTree (Price et al., 2010). Community differences were assessed via permutational multivariate analysis of variance (PERMANOVA) performed on Bray-Curtis distance matrices of relative abundance derived from sequencing and used 9,999 permutations. PERMANOVAs were performed using the function “adonis2” in the <vegan> package (Dixon, 2003) run in “R” (R Core Team, 2018). The <vegan> function “betadispar” was used to test the variances (PERMDISP). A p of 0.05 was set as the significant threshold for all multivariate statistical analyses. Community composition was visualized with NMDS, using 25 restarts and 9,999 iterations.
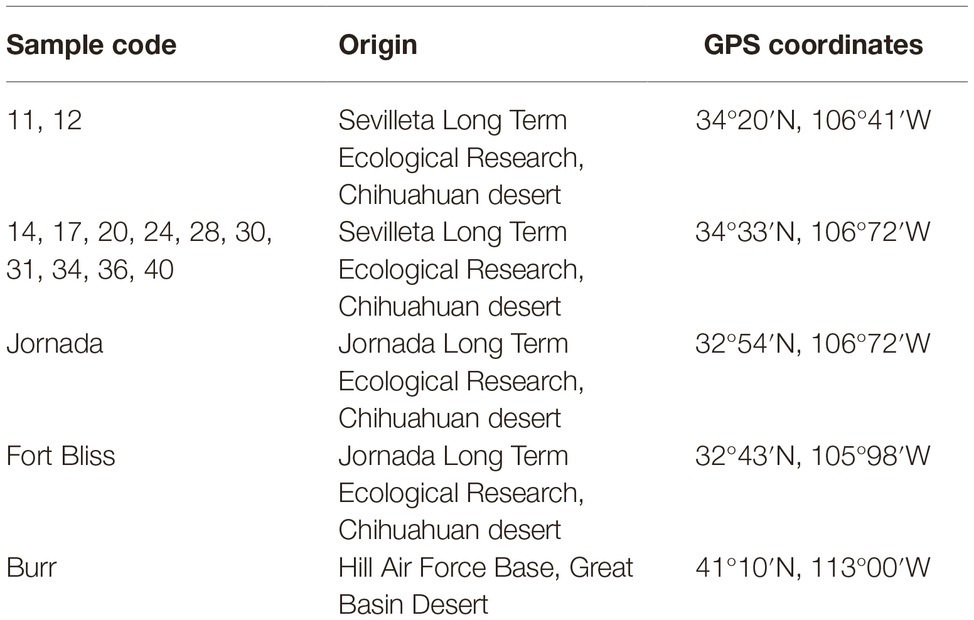
Table 2. Samples and sampling localities of biological crusts in United States of America deserts used for comparison.
Identification of Significant Environmental Parameters
Climatic data were obtained from the public database available at Center for Weather Forecasting and Climate Research (INPE/MCT; http://bancodedados.cptec.inpe.br/). Average annual precipitation (PRE), average annual high temperature (HT), average annual air humidity (AH), and altitude (ALT) were retrieved. Soil temperature (ST) and pH, which were measured in the field, completed the environmental dataset (Table 1). The packages “vegan” (Oksanen et al., 2016), “ggplot2” (Wickham, 2018b), and “gridBase” (Murrell, 2014), written in R language (R Core Team, 2016) were used to relate environmental data to the distribution and abundance of OTUs. After normalizing the OTU table, a redundancy analysis (RDA) was run as a constrained ordination method to search for possible spatial patterns in the cyanobacterial database.
Results
Cyanobacterial Diversity From cerrado Biocrusts
Altogether, we detected 14,465 cyanobacterial OTUs, of which the 600 most frequently represented comprised 70% of total reads. Rarefaction curves showed that most samples (except CA1, ZA3, and ZB1) reached a plateau, and therefore most of diversity was accessed (Supplementary Figure S2). The samples that did not reach the plateau were still included in the subsequent analyses because they were similar in community composition with other samples from the same localities that did.
With our taxonomic identification constrained at the genus level, a large portion of the cyanobacterial diversity remained unassigned (Canastra 34.5%, Capão 19.8%, Cipó 46.5%, Furnas 9.4%, Vassununga 26.9%, and Zagaia 35.9%). But a majority of the unassigned OTUs also failed to align to sequences found within the NCBI database. Even among publicly available environmental sequences, such OTUs did not align with greater than 95% sequence identity, speaking for the presence of a significant level of biodiversity novelty in our biocrusts. At this level of resolution, community composition was relatively homogeneous among samples from the same site (Figure 1). OTUs assignable to various clades of Leptolyngbya had the most reads. They were dominant in Furnas, Cipó, Capão, and Canastra. In some Capão samples, sequences allied to Pycnacronema were also abundant. The most compositionally divergent communities within our set were those from Zagaia and Vassununga, where biocrusts were dominated by sequences assignable to Porphyrosiphon notarisii Kützing ex Gomont.
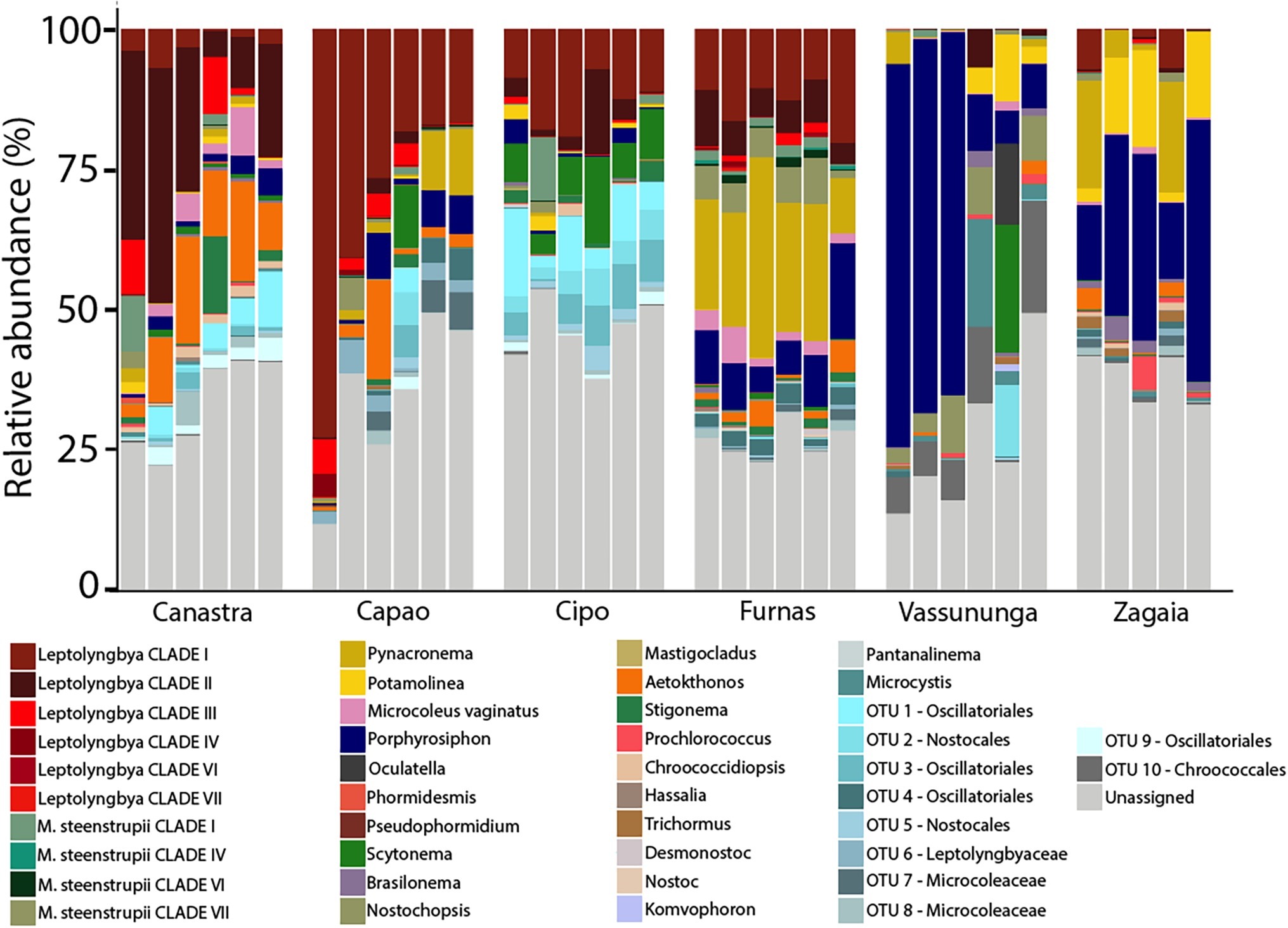
Figure 1. Relative abundance by proportion of 16S rRNA gene sequence reads showing assignable and unassignable to the genus-equivalent level in cerrado biocrusts, grouped by sampling locality.
On the basis of OTUs (i.e., independently of taxonomic assignments and including unassigned OTUs), communities also differed significantly in composition (Supplementary Figure S3) among localities but not within them (PERMANOVA, Pseudo-F = 5.6904, df = 5/30, p < 0.0001), each locality being statistically different from each other in pairwise comparisons (p < 0.05). Again here, Zagaia and Vassununga seemed to be the most divergent cerrado localities along a compositional continuum. These differences, however, were much less marked than those found between North American desert communities and those from the cerrado as a whole (Figure 2; PERMANOVA, Pseudo-F = 16.442, df = 1/50, p < 0.0001).
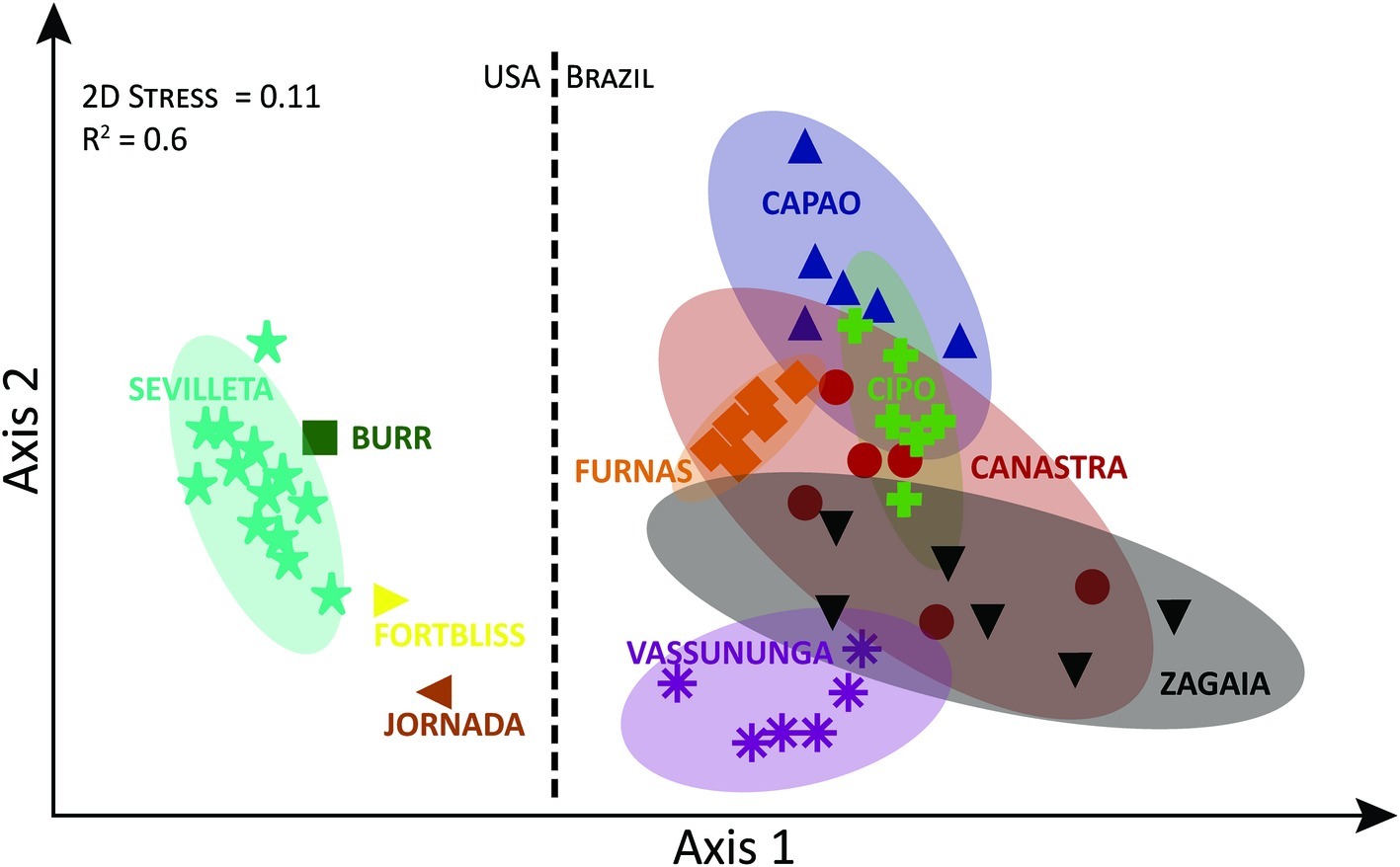
Figure 2. Non-metric Multi-Dimensional Scaling of biocrust cyanobacterial community composition at the OTU level, based on Bray-Curtis similarity including cerrado samples and literature-based data for North American biocrusts, for comparison. Ellipses correspond to 95% CI spaces. Data points and ellipses are color coded by locality.
Identity of Major Cyanobacterial Members in the cerrado Biocrusts
The detailed phylogenetic placement using Cydrasil revealed the occurrence of several important clades of OTUs, which we describe in more detail here. The placement of major cerrado biocrusts inhabitants within the cyanobacterial radiation is given in Figure 3. Details for the different taxa are in Supplementary Figures S4–S6.
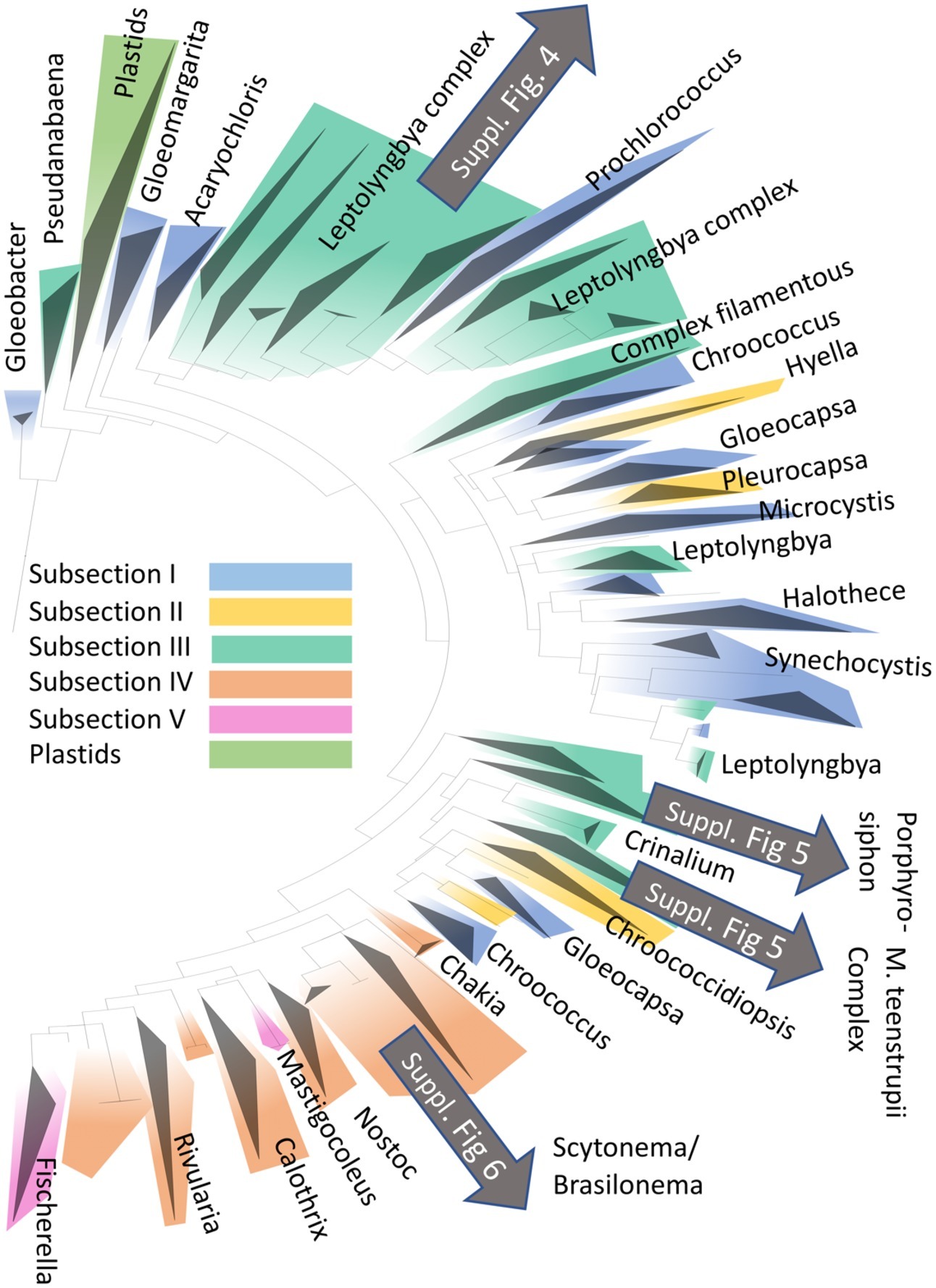
Figure 3. Placement of the more abundant cyanobacteria found in the present study (with greater number of 16S rRNA gene sequence reads; gray arrows) in the phylogenetic radiation of cyanobacteria based on the Cydrasil database. Clades have been colored according to Bergey’s Manual of Determinative Bacteriology’s major morphotypic subsections, and exemplary genera contained within selected clades have been used to label them. Detailed phylogenies of biocrusts OTUs can be found in Supplementary Figures S4–S6, as indicated.
Leptolyngbya Clade I fell within the poorly defined, polyphyletic complex of sequences belonging to thin filamentous cyanobacteria that are usually attributed to this morphogenus and was formed by OTUs related to the public sequence of Leptolyngbya frigida (Fritsch) Anagnostidis and Komárek. It was clearly polyphyletic to the clade containing the sequence for the generic type species (L. boryana Anagnostidis and Komárek), so it likely represents a new generic unit. A second clade in this complex, Leptolyngbya clade II, was composed of OTUs similar to the sequence of Leptolyngbya sp. 7FUR (MF109116), but they were also, as Leptolyngbya Clade I, polyphyletic to true Leptolyngbya. Finally, OTUs placed within Leptolyngbya Clade III gathered around the sequence of the type species and are likely Leptolyngbya sensu stricto. A tree for the “Leptolyngbya complex” with assignments can be found in the Supplementary Figure S4.
A set of abundant OTUs with high representation in some of our localities was affiliated with Porphyrosiphon notarisii as judged by close similarity with several new sequences derived from bona fide cultures obtained from Furnas biocrusts (now permanently added to the Cydrasil database). This clade of sequences is well separated phylogenetically from sequences of other large-celled members of the Phormidiaceae, indicating that they do indeed represent a differentiated generic entity, as the traditional taxonomy would predict (Supplementary Figure S5).
A second complex of biocrust cyanobacteria corresponds to the epithet “Microcoleus steenstrupii” which has been recognized as a supra-generic entity in need of re-evaluation, not only because its members are not related to M. vaginatus (Vaucher) Gomont ex Gomont (the type species for the genus Microcoleus, which falls within the Complex Filamentous clade of Figure 3), but also because it encompasses a variety of separated clades with apparently diverging ecological traits (Fernandes et al., 2018). Sensu lato, the complex also includes sequences aligned with cultured strains assigned to M. paludosus Gomont, and the recently described Pycnacronema savannensis Martins, Machado-de-Lima, and Branco isolated and described from Brazilian savanna biocrusts (Martins et al., 2019). All these cyanobacteria are morphologically indistinguishable from Phormidium, except for the fact that they often form trichome bundles. Many OTUs in our crusts clustered within this complex, and given the difficulty in systematics, we have maintained the epithet “Microcoleus steenstrupii complex” to refer to them, except for OTUs clearly affiliated with P. savannensis, which conformed a major component in some of our crusts (Supplementary Figure S5). Several new full sequences of P. savannensis from bona fide cultures were included in the database before analyses to ensure that this choice was correct. OTUs in this complex not affiliated with Pycnacronema were not very numerous in terms of total reads.
OTUs most similar to the sequences of Microcoleus vaginatus, the type species for the genus Microcoleus in the Oscillatoriaceae, and likely the most common terrestrial cyanobacterium globally (Garcia-Pichel, 2009), while not very common here, constituted another clear assignment.
Within the heterocytous cyanobacteria, many OTUs were phylogenetically cognate with members of Brasilonema sp. (Supplementary Figure S6), previously unreported from soil crusts. Among the three major heterocytous types found in North American arid land crusts (Yeager et al., 2007), only Scytonema found significant representation in the cerrado crusts.
Relationship Between Operational Taxonomic Units and Environmental Variables
Our RDA analyses (Figure 4) showed that the combined effect of all environmental variables considered (Supplementary Table S1) could explained 58% of the total variability in cyanobacterial community composition among Brazilian biocrusts. The two statistical axes represented in Figure 4 explained 40% of this variability. The opposing vectors of high annual temperature (HT) and precipitation (PRE)/air humidity (AH) explained the majority of the variation in community composition among locations, particularly separating Zagaia and Vassununga (related to hotter, drier climate) from the rest. The arid end of this continuum was determined significantly by the strong contributions of Porphyrosiphon, the opposite end by those of Leptolyngbya Clade I. Variability among Canastra, Cipó, Capão, and Furnas communities seemed to be driven by soil pH (Figure 4).
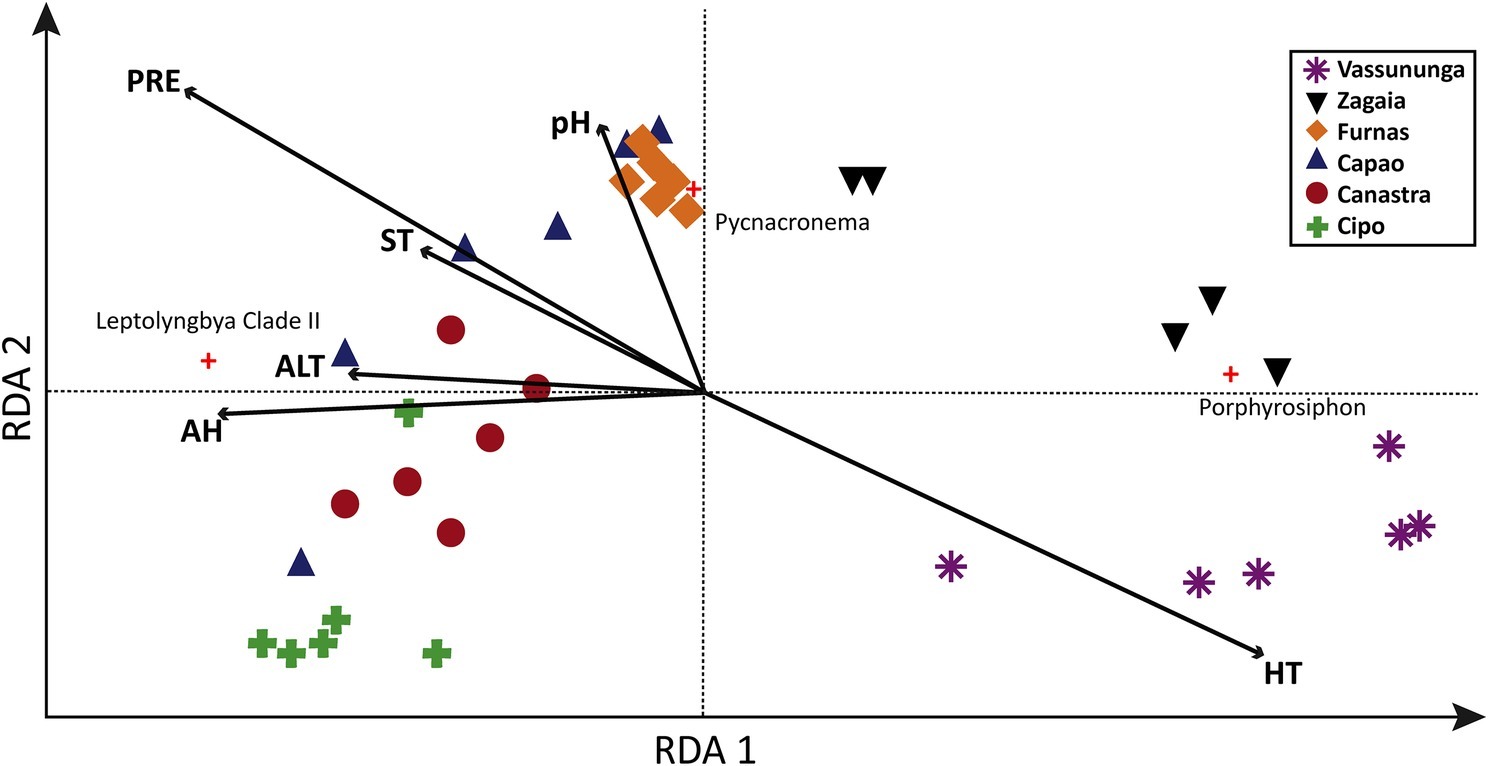
Figure 4. Redundancy analysis (RDA) relating climatic variables with cyanobacterial diversity in 36 sites from six localities in the Brazilian cerrado. ALT, altitude; AH, air humidity; PRE, average annual precipitation; ST, pH, soil temperature; HT, average annual maximum temperature.
Discussion
Microcoleus vaginatus, M. steenstrupii Petersen, M. sociatus West and West, Nostoc commune Vaucher ex Bornet and Flahault, Calothrix, Lyngbya, Oscillatoria, Phormidium, Scytonema, and Tolypothrix are considered the most representative and abundant taxa of cyanobacteria in biological soil crusts throughout the world (Büdel, 2003). Microcoleus vaginatus and the “Microcoleus steenstrupii complex” are dominant in biocrust from North American and other arid lands (Garcia-Pichel et al., 2001; Gundlapally and Garcia-Pichel, 2006; Büdel et al., 2009; Hagemann et al., 2015; Schulz et al., 2015; Dulić et al., 2017). Interestingly, many of those taxa were absent (or present only in low abundance) in the cerrado crust. Instead, Leptolyngbya and Porphyrosiphon were well represented and often dominant in these subtropical biocrusts. Among the heterocytous cyanobacteria, Nostoc sp. and Tolypothrix sp., typical of the colder climates in arid lands (Yeager et al., 2012; Zhou et al., 2016; Giraldo-Silva et al., 2019, submitted) had no relevance in the cerrado crusts, yielding to Scytonema and Brasilonema. Additionally, many of the cyanobacterial OTUs from this study did not have phylogenetically close representatives within common databases, resulting in a high proportion of unassigned diversity. This indicates that a significant unique component of biodiversity in the cerrado remains to be characterized. The notion of compositional idiosyncrasy of the cerrado biocrusts is supported strongly by their clear differentiation from arid land biocrusts in nMDS analyses. One cannot principally distinguish from these data if barriers to dispersal or differential selection by environmental conditions are responsible for the pattern. However, the fact that at least some taxa typical of arid land crusts are found at low population density in the cerrado would rather support the latter view.
Leptolyngbya has been commonly reported in BSCs but rarely appearing as the dominant genus in biodiversity studies (Kaštovská et al., 2005; Alwathnani and Johansen, 2011). It is distributed worldwide (Büdel et al., 2009; Hagemann et al., 2015; Schulz et al., 2015), from glacial areas in Svalbard (Norwegian archipelago – Kaštovská et al., 2005) to desert areas at mid-latitudes (Mojave Desert - Alwathnani and Johansen, 2011). However, it is unclear to which of its many polyphyletic clades each reported occurrence belongs. Likely, the “lumping” morphological approach to this genus conceals significant biological and phylogenetic diversity rather than support the notion that Leptolyngbya is a true generalist organism. Based on the results and analyses presented here, separating Leptolyngbya sequences into distinct phylogenetic clades may help solve this issue and allow the establishment of an ecologically meaningful systematic treatment.
The recognition of this distinct composition and the high frequency of Porphyrosiphon and Leptolyngbya clearly speak for the need of investigating these players at the physiological and ecological levels since they will be paramount in establishing a baseline for future understanding of the dynamics of the cerrado environment. On the assumption that emergent ecological properties of biocrusts depend on their species composition, it will be necessary to revise locally the paradigms of biocrust function and ecosystem services that have largely been derived from arid land biocrusts. Porphyrosiphon, an easily recognizable morphotype because of its bright red sheaths, e.g., has also been reported from biocrusts of the savanna ecosystems of Australia (Williams and Büdel, 2012) and Africa (Ullmann and Büdel, 2003), where it is considered widely dominant over other cyanobacteria. We could relate the presence of this organism in the field with sequences of cultivated isolates, which should enable an easier identification of its global biogeography in the future. Porphyrosiphon produces gelatinous sheaths that could bind soil particles playing a similar role to that of bundle-forming Microcoleus vaginatus and M. steenstrupii in arid land biocrusts (Péli et al., 2011).
OTUs that could not be assigned to genus level but with high number of reads, and for which a sufficiently similar sequence was found to public database entries, were classified at order rank (OTU1 to OTU10). This was preferred (instead of family rank) because familial divisions in Cyanobacteria are phylogenetically ill-defined or polyphyletic, and taxonomically unresolved. Most unassigned OTUs, however, represent sequences with low number of reads.
The importance of aridity (including temperature and rainfall) as determinant of variations in species composition within the cerrado; however, parallels what has been found in arid land and Mediterranean biocrusts (Garcia-Pichel et al., 2013; Muñoz-Martín et al., 2019). Although it is necessary to consider that the set of variables used in this work was rather restricted, in the present case, Leptolyngbya Clade-I abundance was positively correlated with the wetter, least hot sites, while Porphyrosiphon showed an opposite trend, explaining their apparent mutual exclusion as dominant forms. Porphyrosiphon notarisii was found to be also a dominant species in Australian Mulga Lands (Williams and Büdel, 2012), where it reportedly tolerated and recovered from drought exceptionally well. This success could be, at least in part, related to their copious sheath investments, which are thick and deeply colored with a red extracellular pigment known as gloeocapsin, likely serving a sunscreen role, and which probably decreases the albedo of the soil, increasing its temperature even further (Couradeau et al., 2016).
The biodiversity of cyanobacteria that exists in biocrusts from cerrado seems to be distinct from that of other well-known assemblages, such as those from deserts, and in many aspects quite unique. Dominant species in cerrado crusts are marginally present, or even absent, in arid lands evidencing the forcing by large-scale climatic patterns. At the same time, biocrusts from relatively close areas within the cerrado domain showed variations in composition that are attributed to local, geographically more restricted conditions. The interaction of the conditions on both scales determines the specific characteristics of the communities and may have implications for the ecological services that biocrust may be able to provide to the ecosystem in each locale.
Data Availability Statement
The datasets generated for this study can be found in the NCBI Bioproject database under the accession number PRJNA381019 (https://www.ncbi.nlm.nih.gov/bioproject/PRJNA381019).
Author Contributions
NM-L and VF contributed equally to this manuscript. NM-L, JR, and LB designed experiments and sampled the biocrusts. NM-L, JR and VF performed the laboratory research. NM-L, VF, FG-P and LB processed and analyzed the data. DR wrote and executed the codes for bioinformatics analysis. SV wrote the code for the environmental data analysis and executed it. NM-L, VF, FG-P and VF wrote the manuscript. All authors contributed to the discussion of data and finalization of the manuscript. All authors read and approved the final manuscript.
Funding
The São Paulo Research Foundation (FAPESP) supported this study by the research grant 2012/019468-0 to LB and the scholarships 14/06245-8 and 15/17709-8 to NM-L.
Conflict of Interest
The authors declare that the research was conducted in the absence of any commercial or financial relationships that could be construed as a potential conflict of interest.
Supplementary Material
The Supplementary Material for this article can be found online at: https://www.frontiersin.org/articles/10.3389/fmicb.2019.02798/full#supplementary-material
References
Abed, R. M., Al Kharusi, S., Schramm, A., and Robinson, M. D. (2010). Bacterial diversity, pigments and nitrogen fixation of biological desert crusts from the Sultanate of Oman. FEMS Microbiol. Ecol. 72, 418–428. doi: 10.1111/j.1574-6941.2010.00854.x
Alwathnani, H., and Johansen, J. R. (2011). Cyanobacteria in soils from a Mojave Desert ecosystem. Monogr. West. North Am. Nat. 5, 71–89. doi: 10.3398/042.005.0103
Bates, S. T., Garcia-Pichel, F., and Nash, T. H. III. (2010a). Fungal components of biological soil crusts: insights from culture-dependent and culture-independent studies. Bibliotheca Lichenologica 105, 197–210.
Bates, S. T., Nash, T. H., Sweat, K. G., and Garcia-Pichel, F. (2010b). Fungal communities of lichen-dominated biological soil crusts: diversity, relative microbial biomass, and their relationship to disturbance and crust cover. J. Arid Environ. 74, 1192–1199. doi: 10.1016/j.jaridenv.2010.05.033
Baumann, K., Jung, P., Samolov, E., Lehnert, L. W., Büdel, B., Karsten, U., et al. (2018). Biological soil crusts along a climatic gradient in Chile: richness and imprints of phototrophic microorganisms in phosphorus biogeochemical cycling. Soil Biol. Biochem. 127, 286–300. doi: 10.1016/j.soilbio.2018.09.035
Baumann, K., Jung, P., Samolov, E., Lehnert, L. W., Büdel, B., Karsten, U., et al. (1990). Basic local alignment search tool. Soil Biol. Biochem. 215, 286–300.
Belnap, J. (2003). The world at your feet: desert biological soil crusts. Front. Ecol. Environ. 1, 181–189. doi: 10.1890/1540-9295(2003)001[0181:TWAYFD]2.0.CO;2
Belnap, J. (2005). “Crusts: biological” in Encyclopedia of soils in the environment. ed. D. Hillel (London: Elsevier), 339–346.
Belnap, J., Weber, B., and Burkhard, B. (2016). “Biological soil crusts as an organizing principle in drylands” in Biological soil crusts: An organizing principle in drylands (Cham, Switzerland: Springer International Publishing).
Beraldi-Campesi, H., Hartnett, H. E., Anbar, A., Gordon, G. W., and Garcia-Pichel, F. (2009). Effect of biological soil crusts on soil elemental concentrations: implications for biogeochemistry and as traceable biosignatures of ancient life on land. Geobiology 7, 348–359. doi: 10.1111/j.1472-4669.2009.00204.x
Berger, S. A., and Stamatakis, A. (2011). Aligning short reads to reference alignments and trees. Bioinformatics 27, 2068–2075. doi: 10.1093/bioinformatics/btr320
Bolger, A. M., Lohse, M., and Usadel, B. (2014). Trimmomatic: a flexible trimmer for Illumina sequence data. Bioinformatics 30, 2114–2120. doi: 10.1093/bioinformatics/btu170
Büdel, B. (2003). “Synopsis: comparative biogeography and ecology of soil crust biota” in Biological soil crusts: structure, function, and management. eds. J. Belnap and O. L. Lange (Berlin-Heidelberg, Germany: Springer Verlag), 141–152.
Büdel, B., Darienko, T., Deutschewitz, K., Dojani, S., Friedl, T., Mohr, K. I., et al. (2009). Southern African biological soil crusts are ubiquitous and highly diverse in drylands, being restricted by rainfall frequency. Microb. Ecol. 57, 229–247. doi: 10.1007/s00248-008-9449-9
Caporaso, J. G., Kuczynski, J., Stombaugh, J., Bittinger, K., Bushman, F. D., Costello, E. K., et al. (2010). QIIME allows analysis of high-throughput community sequencing data intensity normalization improves color calling in SOLiD sequencing. Nat. Publ. Group 7, 335–336. doi: 10.1038/nmeth.f.303
Caporaso, J. G., Lauber, C. L., Walters, W. A., Berg-lyons, D., Huntley, J., Fierer, N., et al. (2012). Ultra-high-throughput microbial community analysis on the Illumina HiSeq and MiSeq platforms. ISME J. 6, 1621–1624. doi: 10.1038/ismej.2012.8
Couradeau, E., Karaoz, U., Lim, H. C., Nunes da Rocha, U., Northen, T., Brodie, E., et al. (2016). Bacteria increase arid-land soil surface temperature through the production of sunscreens. Nat. Commun. 7:10373. doi: 10.1038/ncomms10373
da Rocha, U. N., Cadillo-Quiroz, H., Karaoz, U., Rajeev, L., Klitgord, N., Dunn, S., et al. (2015). Isolation of a significant fraction of non-phototroph diversity from a desert biological soil crust. Front. Microbiol. 6, 1–14. doi: 10.3389/fmicb.2015.00277
Delgado-Baquerizo, M., Maestre, F. T., Eldridge, D. J., Bowker, M. A., Jeffries, T. C., and Singh, B. K. (2018). Biocrust-forming mosses mitigate the impact of aridity on soil microbial communities in drylands: observational evidence from three continents. New Phytol. 220, 824–835. doi: 10.1111/nph.15120
DeSantis, T. Z., Hugenholtz, P., Larsen, N., Rojas, M., Brodie, E. L., Keller, K., et al. (2006). Greengenes, a chimera-checked 16S rRNA gene database and workbench compatible with ARB. Appl. Environ. Microbiol. 72, 5069–5072. doi: 10.1128/AEM.03006-05
Dixon, P. (2003). VEGAN, a package of R functions for community ecology. J. Vegeta. Sci. 14, 927–930. doi: 10.1111/j.1654-1103.2003.tb02228.x
Dulić, T., Meriluoto, J., Palanački Malešević, T., Gajić, V., Važić, T., Tokodi, N., et al. (2017). Cyanobacterial diversity and toxicity of biocrusts from the Caspian lowland loess deposits, North Iran. Quat. Int. 429, 74–85. doi: 10.1016/j.quaint.2016.02.046
Eiten, G. (1982). “Brazilian ‘Savannas’” in Ecology of tropical savannas. Ecological studies. Vol. 42. eds. B. J. Huntley and B. H. Walker (Berlin-Heidelberg, Germany: Springer Verlag), 25–47.
Elbert, W., Weber, B., Andreae, M. O., and Poschl, U. (2009). Microbiotic crusts on soil, rock and plants: neglected major players in the global cycles of carbon and nitrogen? Biogeosci. Discus. 6, 6983–7015. doi: 10.5194/bgd-6-6983-2009
Eldridge, D. J., and Greene, R. S. B. (1994). Microbiotic soil crusts: a review of their roles in soil and ecological processes in the rangelands of Australia. Aust. J. Soil Res. 32, 389–415. doi: 10.1071/SR9940389
Fernandes, V. M. C., Machado de Lima, N. M., Roush, D., Rudgers, J., Collins, S. L., and Garcia-Pichel, F. (2018). Exposure to predicted precipitation patterns decreases population size and alters community structure of cyanobacteria in biological soil crusts from the Chihuahuan desert. Environ. Microbiol. 20, 259–269. doi: 10.1111/1462-2920.13983
Garcia-Pichel, F. (2009). “Cyanobacteria” in Encyclopedia of microbiology. ed. M. Schaechter (Oxford: Academic Press), 107–124.
Garcia-Pichel, F., López-Cortés, A., and Nübel, U. (2001). Phylogenetic and morphological diversity of cyanobacteria in soil desert crusts from the Colorado Plateau. Appl. Environ. Microbiol. 67, 1902–1910. doi: 10.1128/AEM.67.4.1902-1910.2001
Garcia-Pichel, F., Loza, V., Marusenko, Y., Mateo, P., and Potrafka, R. M. (2013). Temperature drives the continental-scale distribution of key microbes in topsoil communities. Science 340, 1574–1577. doi: 10.1126/science.1236404
Garcia-Pichel, F., and Wojciechowski, M. F. (2009). The evolution of a capacity to build supra-cellular ropes enabled filamentous cyanobacteria to colonize highly erodible substrates. PLoS One 4, 4–9. doi: 10.1371/journal.pone.0007801
Giraldo-Silva, A., Nelson, C., Barger, N. N., and Garcia-Pichel, F. (2018). Nursing biocrusts: isolation, cultivation, and fitness test of indigenous cyanobacteria. Restor. Ecol. 27, 793–803. doi: 10.1111/rec.12920
Gundlapally, S. R., and Garcia-Pichel, F. (2006). The community and phylogenetic diversity of biological soil crusts in the Colorado plateau studied by molecular fingerprinting and intensive cultivation. Microb. Ecol. 52, 345–357. doi: 10.1007/s00248-006-9011-6
Hagemann, M., Henneberg, M., Felde, V. J. M. N. L., Drahorad, S. L., Berkowicz, S. M., Felix-Henningsen, P., et al. (2015). Cyanobacterial diversity in biological soil crusts along a precipitation gradient, Northwest Negev Desert, Israel. Microb. Ecol. 70, 219–230. doi: 10.1007/s00248-014-0533-z
Johnson, S. L., Neuer, S., and Garcia-Pichel, F. (2007). Export of nitrogenous compounds due to incomplete cycling within biological soil crusts of arid lands. Environ. Microbiol. 9, 680–689. doi: 10.1111/j.1462-2920.2006.01187.x
Kaštovská, K., Elster, J., Stibal, M., and Šantrůčková, H. (2005). Microbial assemblages in soil microbial succession after glacial retreat in Svalbard (high Arctic). Microb. Ecol. 50:396. doi: 10.1007/s00248-005-0246-4
Katoh, K., and Standley, D. M. (2013). MAFFT multiple sequence alignment software version 7: improvements in performance and usability. Mol. Biol. Evol. 30, 772–780. doi: 10.1093/molbev/mst010
Kopylova, E., Noé, L., and Touzet, H. (2012). SortMeRNA: fast and accurate filtering of ribosomal RNAs in metatranscriptomic data. Bioinformatics 28, 3211–3217. doi: 10.1093/bioinformatics/bts611
Letunic, I., and Bork, P. (2016). Interactive tree of life (iTOL) v3: an online tool for the display and annotation of phylogenetic and other trees. 44, 242–245. doi: 10.1093/nar/gkw290
Martins, M. D., Machado-de-Lima, N. M., and Branco, L. H. Z. (2019). Polyphasic approach using multilocus analyses supports the establishment of the new aerophytic cyanobacterial genus Pycnacronema (Coleofasciculaceae, Oscillatoriales). J. Phycol. 55, 146–159. doi: 10.1111/jpy.12805
Masella, A. P., Bartram, A. K., Truszkowski, J. M., Brown, D. G., and Neufeld, J. D. (2012). PANDAseq: paired-end assembler for illumina sequences. BMC Bioinform. 13:31. doi: 10.1186/1471-2105-13-31
Muñoz-Martín, M., Becerra-Absalón, I., Perona, E., Fernández-Valbuena, L., Garcia-Pichel, F., and Mateo, P. (2019). Cyanobacterial biocrust diversity in Mediterranean ecosystems along a latitudinal and climatic gradient. New Phytol. 221, 123–141. doi: 10.1111/nph.15355
Murrell, P. (2014). gridBase: integration of base and grid graphics. Available at: https://cran.r-project.org/web/packages/gridBase/gridBase.pdf (Accessed April 2016).
Nejidat, A., Potrafka, R. M., and Zaady, E. (2016). Successional biocrust stages on dead shrub soil mounds after severe drought: effect of micro-geomorphology on microbial community structure and ecosystem recovery. Soil Biol. Biochem. 103, 213–220. doi: 10.1016/j.soilbio.2016.08.028
Nübel, U., Muyzer, G., Garcia-Pichel, F., and Muyzer, G. (1997). PCR primers to amplify 16S rRNA genes from cyanobacteria PCR primers to amplify 16S rRNA genes from cyanobacteria. Microbiology 63, 3327–3332.
Oksanen, J., Blanchet, F. G., Kindt, R., Legendre, P., Minchin, P. R., O’hara, R. B., et al. (2016). Vegan: community ecology package version 2.3–5. Available at: https://cran.r-project.org/web/packages/vegan/vegan.pdf (Accessed April 2016).
Patzelt, D. J., Hodač, L., Friedl, T., Pietrasiak, N., and Johansen, J. R. (2014). Biodiversity of soil cyanobacteria in the hyper-arid Atacama Desert, Chile. J. Phycol. 50, 698–710. doi: 10.1111/jpy.12196
Péli, E. R., Lei, N., Pócs, T., Laufer, Z., Porembski, S., and Tuba, Z. (2011). Ecophysiological responses of desiccation-tolerant cryptobiotic crusts. Cent. Eur. J. Biol. 6, 838–849. doi: 10.2478/s11535-011-0075-z
Pointing, S. B., and Belnap, J. (2012). Microbial colonization and controls in dryland systems. Nat. Rev. Microbiol. 10, 551–562. doi: 10.1038/nrmicro2831
Price, M. N., Dehal, P. S., and Arkin, A. P. (2010). FastTree 2--approximately maximum-likelihood trees for large alignments. PLoS One 5:e9490. doi: 10.1371/journal.pone.0009490
Pushkareva, E., Pessi, I., Wilmotte, A., and Elster, J. (2015). Cyanobacterial community composition in Arctic soil crusts at different stages of development. FEMS Microbiol. Ecol. 91:fiv143. doi: 10.1093/femsec/fiv143
R Core Team (2016). R: A language and environment for statistical computing. Vienna, Austria: R Foundation for Statistical Computing. Available at: https://www.R-project.org/
R Core Team (2018). R: A language and environment for statistical computing. Vienna, Austria: R Foundation for Statistical Computing. Available at: https://www.R-project.org/ (Accessed: December 2019).
Ratter, J. A., Ribeiro, J. F., and Bridgewater, S. (1997). The Brazilian Cerrado vegetation and threats to its biodiversity. Ann. Bot. 80, 223–230. doi: 10.1006/anbo.1997.0469
Rognes, T., Flouri, T., Nichols, B., Quince, C., and Mahé, F. (2016). VSEARCH: a versatile open source tool for metagenomics. PeerJ 4:e2409v1. doi: 10.7717/peerj.2584
Sano, E. S., Rosa, R., Brito, J. L. S., and Ferreira, L. G. (2007). “Mapeamento de cobertuta vegetal do Bioma Cerrado: estratégias e resultados” in Mapeamento de cobertuta vegetal do Bioma Cerrado: Estratégias e resultados (Distrito Federal, Brazil: Empraba Cerrados).
Schloss, P. D. (2016). Application of a database-independent approach to assess the quality of operational taxonomic unit picking methods. mSystems 1, e00027–e00116. doi: 10.1128/mSystems.00027-16
Schulz, K., Mikhailyuk, T., Dreßler, M., Leinweber, P., and Karsten, U. (2015). Biological soil crusts from coastal dunes at the Baltic Sea: cyanobacterial and algal biodiversity and related soil properties. Microb. Ecol. 71, 178–193. doi: 10.1007/s00248-015-0691-7
Soule, T., Anderson, I. J., Johnson, S. L., Bates, S. T., and Garcia-Pichel, F. (2009). Archaeal populations in biological soil crusts from arid lands in North America. Soil Biol. Biochem. 41, 2069–2074. doi: 10.1016/j.soilbio.2009.07.023
Ullmann, I., and Büdel, B. (2003). “Biological soil crusts of Africa” in Biological soil crusts: Structure, function, and management. eds. J. Belnap and O. L. Lange (Berlin Heidelberg: Springer-Verlag), 107–118.
Velasco Ayuso, S., Giraldo Silva, A., Nelson, C., Barger, N. N., and Garcia-Pichel, F. (2017). Microbial nursery production of high-quality biological soil crust biomass for restoration of degraded dryland soils. Appl. Environ. Microbiol. 83, e02179–e02116. doi: 10.1128/AEM.02179-16
Wickham, H. (2017). reshape2. Available at: https://cran.r-project.org/web/packages/reshape2/reshape2.pdf (Accessed January 2019).
Wickham, H. (2018a). Scales. Available at: https://cran.r-project.org/web/packages/scales/scales.pdf (Accessed January 2019).
Wickham, H. (2018b). ggplot2. Available at: https://cran.r-project.org/web/packages/ggplot2/ggplot2.pdf (Accessed January 2019).
Williams, W. J., and Büdel, B. (2012). Species diversity, biomass and long-term patterns of biological soil crusts with special focus on cyanobacteria of the Acacia aneura Mulga lands of Queensland, Australia. Algol. Stud. 140, 23–50. doi: 10.1127/1864-1318/2012/0059
Williams, L., Loewen-Schneider, K., Maier, S., and Büdel, B. (2016). Cyanobacterial diversity of western European biological soil crusts along a latitudinal gradient. FEMS Microbiol. Ecol. 92:fiw157. doi: 10.1093/femsec/fiw157
Yarza, P., Yilmaz, P., Pruesse, E., Glöckner, F. O., Ludwig, W., Schleifer, K. H., et al. (2014). Uniting the classification of cultured and uncultured bacteria and archaea using 16S rRNA gene sequences. Nat. Rev. Microbiol. 12, 635–645. doi: 10.1038/nrmicro3330
Yeager, C. M., Kornosky, J. L., Morgan, R. E., Cain, E. C., Garcia-Pichel, F., Housman, D. C., et al. (2007). Three distinct clades of cultured heterocystous cyanobacteria constitute the dominant N2-fixing members of biological soil crusts of the Colorado plateau, USA. FEMS Microbiol. Ecol. 60, 85–97. doi: 10.1111/j.1574-6941.2006.00265.x
Yeager, C. M., Kuske, C. R., Carney, T. D., Johnson, S. L., Ticknor, L. O., and Belnap, J. (2012). Response of biological soil crust diazotrophs to season, altered summer precipitation, and year-round increased temperature in an arid grassland of the Colorado plateau, USA. Front. Microbiol. 3:358. doi: 10.3389/fmicb.2012.00358
Zhang, B., Kong, W., Wu, N., and Zhang, Y. (2016). Bacterial diversity and community along the succession of biological soil crusts in the Gurbantunggut Desert, Northern China. J. Basic Microbiol. 56, 670–679. doi: 10.1002/jobm.201500751
Zhou, X., Smith, H., Silva, A. G., Belnap, J., and Garcia-Pichel, F. (2016). Differential responses of dinitrogen fixation, diazotrophic cyanobacteria and ammonia oxidation reveal a potential warming-induced imbalance of the N-cycle in biological soil crusts. PLoS One 11, 1–15. doi: 10.1371/journal.pone.0164932
Keywords: biocrust, cerrado savanna, cyanobacteria, Leptolyngbya, Porphyrosiphon, aridity
Citation: Machado-de-Lima NM, Fernandes VMC, Roush D, Velasco Ayuso S, Rigonato J, Garcia-Pichel F and Branco LHZ (2019) The Compositionally Distinct Cyanobacterial Biocrusts From Brazilian Savanna and Their Environmental Drivers of Community Diversity. Front. Microbiol. 10:2798. doi: 10.3389/fmicb.2019.02798
Edited by:
Steven D. Warren, United States Forest Service, United StatesReviewed by:
Lara Vimercati, University of Colorado Boulder, United StatesChris Yeager, Los Alamos National Laboratory (DOE), United States
Copyright © 2019 Machado-de-Lima, Fernandes, Roush, Velasco Ayuso, Rigonato, Garcia-Pichel and Branco. This is an open-access article distributed under the terms of the Creative Commons Attribution License (CC BY). The use, distribution or reproduction in other forums is permitted, provided the original author(s) and the copyright owner(s) are credited and that the original publication in this journal is cited, in accordance with accepted academic practice. No use, distribution or reproduction is permitted which does not comply with these terms.
*Correspondence: Ferran Garcia-Pichel, ZmVycmFuQGFzdS5lZHU=; Luis Henrique Zanini Branco, bHVpcy5icmFuY29AdW5lc3AuYnI=
†These authors have contributed equally to this work