- 1Department of Soil Ecology, UFZ – Helmholtz Centre for Environmental Research, Halle/Saale, Germany
- 2German Centre for Integrative Biodiversity Research (iDiv) Halle-Jena-Leipzig, Leipzig, Germany
Climate change models predict more frequent and prolonged drought events in Central Europe, which will exert extraordinary pressure on agroecosystems. One of the consequences is drought-related nutrient limitations for crops negatively affecting agricultural productivity. These effects can be mitigated by beneficial plant growth promoting rhizobacteria. In this study, we investigated the potential of cultivable bacterial species for phosphate solubilization in the rhizosphere of winter wheat at two relevant growth stages - stem elongation and grain filling stages. Rhizosphere samples were collected in the Global Change Experimental Facility in Central Germany, which comprises plots with conventional and organic farming systems under ambient and future climate. Phosphate-solubilizing bacteria were selectively isolated on Pikovskaya medium, phylogenetically classified by 16S rRNA sequencing, and tested for in vitro mineral phosphate solubilization and drought tolerance using plate assays. The culture isolates were dominated by members of the genera Phyllobacterium, Pseudomonas and Streptomyces. Cultivation-derived species richness and abundance of dominant taxa, especially within the genera Phyllobacterium and Pseudomonas, as well as composition of Pseudomonas species were affected by wheat growth stage. Pseudomonas was found to be more abundant at stem elongation than at grain filling, while for Phyllobacterium the opposite pattern was observed. The abundance of Streptomyces isolates remained stable throughout the studied growth stages. The temporal shifts in the cultivable fraction of the community along with considerable P solubilization potentials of Phyllobacterium and Pseudomonas species suggest functional redundancy between and among genera at different wheat growth stages. Phosphate-solubilizing Phyllobacterium species were assigned to Phyllobacterium ifriqiyense and Phyllobacterium sophorae. It is the first time that phosphate solubilization potential is described for these species. Since Phyllobacterium species showed the highest drought tolerance along all isolates, they may play an increasingly important role in phosphate solubilization in a future dryer climate.
Introduction
Winter wheat (Triticum aestivum L.) is the most commonly grown crop in Germany accounting for 54% of the total agricultural area in 2017 (Schmeling, 2017). At the same time wheat is the second most produced crop worldwide after maize (Statista, 2018). The production of winter wheat depends on frequent precipitation and sufficient nutrient supply (Fischer, 2003). Its cultivation is hence restricted to appropriate regions and is commonly supported by sufficient fertilization. Besides nitrogen and potassium, phosphorus (P) has to be applied, since the plant-available P in the rooted topsoil often represents a growth limiting factor. However, P fertilization does not necessarily increase P availability. Up to 90% of fertilized mineral P is quickly immobilized by reactive cations, such as Al3+ or Fe3+ in acidic or Ca2+ in calcareous or neutral soils (Oehl et al., 2002). At a global scale, models suggest an increase in P input into agricultural systems of 51–86% by the year 2050 (Mogollón et al., 2018) to maintain productivity. In parallel, the source for P fertilizer – natural rock phosphate – is expected to deplete in 50–100 years (Cordell et al., 2009). Regarding the application of organic fertilizers phosphorus is bound into complex compounds and first has to be released before plant uptake.
Processes that release P from mineral and organic compounds in the soil are mainly driven by microorganisms. Therefore, to counteract P limitation, plants enrich a variety of beneficial microbes in their rhizospheres by secreting nourishing, carbon-containing rhizodeposits. Besides mycorrhizal fungi, plant growth promoting rhizobacteria (PGPR) can enhance plant P uptake. PGPR thereby stimulate root growth, release P from immobilized inorganic and organic P pools in the soil, and transport solved P compounds to the plant’s roots (Artursson et al., 2006). However, these mutual interactions between the plant and its rhizosphere community are highly dynamic and related to the plant growth stage as well as to environmental conditions.
Seasonal shifts in rhizosphere microbiomes related to plant growth stages are well documented for canola and grasses (Dunfield and Germida, 2003; Wolsing and Priemé, 2004; Francioli et al., 2018). The changes are mainly caused by variations in the quantity and quality of carbon-derived rhizodeposits serving as energy sources for soil microbes (reviewed in Bais et al., 2006). These rhizodeposits are predominantly released into the rhizosphere when plants are actively growing (Badri and Vivanco, 2009). Thus, flowering plants (Aulakh et al., 2001; Lucas García et al., 2001) mainly secrete root exudates during stem elongation, booting and flowering stages, and much less during fruit development and ripening stages. Malhi et al. (2006) also found stem elongation and tillering to be the stages with maximum nutrient uptake of cereals. Investigations of the wheat rhizobiome often focus on the stages of tillering and flowering, but also on grain filling (predominantly in field experiments, e.g., Juhnke et al., 1987; Creus et al., 2004; Naiman et al., 2009; Ilyas and Bano, 2010) or are independent of certain growth stages throughout the first weeks of wheat development (predominantly in pot experiments, e.g., Kasim et al., 2013; Timmusk et al., 2014). Clearly, more information on the interrelationships between plant growth stages and the PGPR are needed.
Besides the seasonal dynamics, microbial communities in agricultural soils have to cope with frequent disturbances. Agricultural management practices such as fertilization, tillage, pesticide application and crop rotation including brown fallow periods cause dramatic changes of C supply patterns within the soil rooting zone. It was shown that tillage (Frey et al., 1999; Kladivko, 2001) and crop rotation (Tiemann et al., 2015) lead to a decrease in fungal biomass (Smith and Read, 2010) and diversity (Verbruggen et al., 2010). A recent study of Banerjee et al. (2019) also demonstrated that land use intensification reduces fungal network complexity in conventional and even in no tillage land use systems. In contrary, bacterial biomass was nearly unaffected (Elliott et al., 1988), leading to establishment of a primarily bacteria-based food web in managed agroecosystems (Hendrix et al., 1986; de Vries and Shade, 2013). Therefore, we focused on the bacterial rather than the fungal community in this study.
The high adaptation potential of soil bacteria to changing conditions is related to their high growth and mutation rates, as well as rapid recombination by lateral gene transfer (Badri and Vivanco, 2009). Moreover, to maintain crucial soil processes after disturbances and under changing conditions, the huge bacterial diversity allows quick and diverse reordering of the active community (Kennedy, 1999). According to Sheik et al. (2011), stress conditions such as the combination of warming and drought effects lead to less diverse microbial populations. In contrast, functions that have to be provided for plants by microbes may be even more essential under stress conditions and a more complex population structure may arise, since more complex co-occurrence networks improve complementarity and efficiency (Tardy et al., 2014; Karimi et al., 2017). Thus, soil bacterial communities play a key role in stress resistance and recovery after disturbances of agroecosystems (Shade et al., 2012).
Most field studies on beneficial wheat rhizosphere microbes and their plant growth promoting properties have been performed in arid and semiarid regions of India (Rana et al., 2011; Jog et al., 2014; Kumar et al., 2014; Singh and Lal, 2016; Verma et al., 2016), Pakistan (Ilyas and Bano, 2010; Naveed et al., 2014) and Argentina (Creus et al., 2004; Fischer et al., 2007). As these regions are strongly limited in water supply and therefore in the amount of available nutrients, which are solved in the soil water, the potential of PGPR to support wheat growth under drought conditions by different functional properties is of high interest. In contrast, studies in temperate zones with moderate precipitation are underrepresented, although climate change already causes obvious negative effects on agroecosystem productivity (Ciais et al., 2005; Trnka et al., 2014). For instance in 2018 East and Middle Germany experienced a severe drought period, which led to premature ripening and an early harvest of crops (BMEL, 2018). Drought along with nutrient limitation caused yield losses will likely increase in future as climate change models predict altered precipitation patterns with extended drought periods combined with higher temperatures in the vegetation period (Petersen and Weigel, 2015). Therefore, the role of soil bacteria and their potentials for tolerance and resilience of agroecosystems in the temperate zones is of increasing importance.
To understand the role of the rhizosphere bacterial community in providing P to wheat plants over the growing season, it is indispensable to identify P-solubilizing key species and their functionality in dependency on wheat growth stage while simultaneously considering the impacts of the two most important global change drivers, agricultural management and climate (Sala et al., 2000). In order to address this issue, we investigated P-solubilizing rhizosphere bacteria at two relevant wheat growth stages, namely stem elongation (vegetative biomass production) and grain filling stage (generative biomass production). Wheat samples were collected in the Global Change Experimental Facility (GCEF, Schädler et al., 2019). The design of the experimental field platform allows the comparison of two different farming systems (conventional vs. organic agriculture) exposed to either ambient climate, at present, or future expected climate scenario with warming and increased summer drought in the latter. Within this experimental setup we used a cultivation-dependent approach based on Pikovskaya medium (Pikovskaya, 1948) to isolate exclusively for P-solubilizing bacteria. We decided for tri-calcium phosphate (TCP) as insoluble phosphate source in the neutral Pikovskaya media since the pH in the plots of the GCEF is mainly neutral and reactive anion Ca2+ is predominantly binding mineral P in these soils forming stable complexes (Oehl et al., 2002, Bashan et al., 2013). Solubility of TCP increases with decreasing pH. Acidification by production and release of organic acids is thus one possibility to release phosphate from insoluble TCP source and is therefore an indicator for the potential of phosphate solubilization in vitro (Bashan et al., 2013). We compared the in vitro P solubilization potential and the drought tolerance of the predominantly isolated P-solubilizing taxa at the stem elongation stage (vegetative biomass production) with those present at the grain filling stage (generative biomass production) in different land use systems and under different climatic conditions. We aimed to distinguish the responses in terms of structural resistance and/or functional redundancy between and among cultivable P-solubilizing bacterial taxa. Under changing conditions, identical taxa and functions would suggest adaptation and resistance. In contrast, shifts in the cultivable fraction of the community would either reflect changes in functionality or reveal functional redundancy among P-solubilizing bacterial species.
We hypothesized that (i) the abundances of the predominant P-solubilizing taxa in the wheat rhizosphere vary between stages of wheat development, displaying a higher abundance, diversity and activity of P-solubilizing bacteria at the stem elongation stage due to higher resource demand by plants than at the grain filling stage (Malhi et al., 2006). Furthermore, we hypothesized that (ii) increased drought decreases diversity but induces targeted structural changes within the bacterial community, in a way that P solubilization is maintained. Finally, due to a lower availability of nutrients, we expected (iii) a lower abundance, but higher activity potentials of P-solubilizing bacteria in the organic farming system compared to the conventional farming system.
Materials and Methods
Experimental Platform
The Global Change Experimental Facility (GCEF) (see http://www.ufz.de/index.php?en=40038 for general information and visualizations) was established in 2013 to investigate climate change effects on managed terrestrial ecosystems (Schädler et al., 2019). The GCEF is located at the field research station of the Helmholtz-Centre for Environmental Research (UFZ) in Bad Lauchstädt, Germany (51°23′35″N 11°52′55″E, 118 m a.s.l.). The site is characterized by a temperate climate with an average temperature of 9.7°C (1993–2013) and a mean annual precipitation of 525 mm (1993–2013). The soil type is a humus- and nutrient-rich Haplic Chernozem (Altermann et al., 2005). Besides three grassland management types, a conventional and an organic farming system are part of the GCEF design. In total, the facility includes 50 large plots (24 × 16 m) that allow realistic field management. The 50 plots are arranged in ten blocks, whereby each block comprises all five land use types that are randomly distributed (Supplementary Figure S1). Five blocks are subjected to ambient climatic conditions and the other five to future climatic conditions, resulting in a split-plot experimental design (Supplementary Figure S1). Ambient climate treatment refers to the actual and non-manipulated climate in terms of precipitation and temperature at the field site. According to the projected climate change within the next 50 years for Central Germany, the future climate treatment of the GCEF consists in increasing the mean daily temperature and shifting the annual precipitation pattern. Temperature is increased passively by roofing the plots during the night. Precipitation is modulated based on the actual weather conditions, with a 20% decrease of precipitation during summer (Jun-Aug) and a 10% increase of precipitation in spring (March–May) and autumn (September–November). In this study we focused on the conventional farming (CF) and organic farming (OF) systems. The CF includes a typical regional crop rotation consisting of a sequence of winter rape, winter wheat and winter barley. In two out of 3 years, the crop cycle for OF includes winter wheat and winter barley just as for the CF. In the first and fourth year of this bipartite crop sequence, the nitrogen-fixating legumes alfalfa and white clover are included in the crop cycle, respectively. Herbicides, fungicides, insecticides and plant growth regulators are applied as usual in CF practice (Schädler et al., 2019). For OF only mechanical weed control and a restricted use of pesticides are allowed. Whereas N, P, and K in CF is applied in form of mineral fertilizers, N fertilization in OF is done by the inclusion of legumes in the crop rotation and further fertilization is restricted to the application of rock phosphate (P-Ca-Mg) and patent kali (K-Mg-S) in the first year of the crop cycle.
Sampling and Characterization of Soil Chemical Parameters
Rhizosphere from root systems of winter wheat was collected in organic (OF) and conventional farming (CF) plots of the GCEF exposed either to ambient (A) or simulated future (F) climate conditions. From each of these plots of the GCEF (20 plots) – CF-A (5 plots), CF-F (5 plots), OF-A (5 plots) and OF-F (5 plots) – three plants were taken with root system finalizing in 15 samples per treatment. To cover relevant growth stages for vegetative and generative biomass production over the growing season, sampling campaigns took place at the 26th of May 2015 (BBCH growth stages 37–39, representing stem elongation phase) and at the 8th of July 2015 (BBCH growth stages 75–77, representing the grain filling stage), respectively. Growth stages were identified according to BBCH-scale (“Biologische Bundesanstalt, Bundessortenamt und CHemische Industrie”). Wheat plants were carefully removed from the soil, their root systems cut and shaken to discard unattached soil, and transferred in plastic bags on ice to the laboratory. Subsequently, rhizosphere soil was collected by gently, manually loosening the root-attached soil to avoid damages to the roots. To measure soil chemical properties, bulk soil samples were collected in parallel to rhizosphere samples. Six soil cores per plot were taken to a depth of 15 cm, pooled, sieved to 2 mm and manually cleaned from organic material. The obtained rhizosphere and bulk soil were stored frozen until usage.
Soil chemical properties of the bulk soil samples were analyzed as follows: gravimetric water content of fresh soil was quantified with a halogen moisture analysator (Mettler Toledo, Gießen, Deutschland). pH was measured with an electrode after shaking the soil for 1 h in 0.01 M CaCl2 (1:2.5 w/v). Amounts of total carbon and nitrogen content were determined from air-dried soil using an elemental analyzer (Elementar Vario EL III, Elementar, Hanau, Germany). For analysis of mineral nitrogen 5 g of fresh soil were suspended in 20 ml of 1 M KCl solution and measured per flow injection analysis (FlAstar 5000, Foss GmbH, Rellingen, Germany). Extraction of labile phosphorus in the soil was performed with double lactate solution (1:50 w/v) at pH 3.6 and determined using the colorimetrical molybdenum blue method (Murphy and Riley, 1962).
Selective Isolation and Phylogenetic Classification of Phosphate Solubilizing Bacteria From Wheat Rhizosphere
For cultivation and isolation of bacteria from wheat rhizosphere, 0.5 g of fresh rhizosphere soil was suspended in 50 ml of distilled water and stirred for 5 min to break up soil particles and bring bacteria in suspension. Subsequently, the samples were sonicated for 10 s and stirred for another 2 min. Mineral tri-calcium phosphate solubilizing bacteria were isolated from a 1:200 (v/v) dilution of this rhizosphere soil suspension by plating 50 μl of it in triplicate on Pikovskaya agar (Pikovskaya, 1948), supplemented with the fungicide cycloheximide (10 mg/ml). After 2 weeks of incubation at 25°C, colony forming units (CFU) were counted. Bacterial strains produce and release organic acids to solubilize P from insoluble tri-calcium phosphate source, therefore, forming a clear zone in the otherwise turbid agar around the colony - from heron referred to as halo. Up to 25 colonies (Supplementary Table S4) with the largest halos were collected for each sample from the Pikovskaya plates and transferred to yeast malt extract (YME) agar plates. To identify and store these isolates, polyethylene glycol (PEG) and glycerol stocks were prepared and frozen. Cells in the PEG stock were destroyed mechanically with glass beads by vortexing to release their DNA into the liquid. Polymerase chain reaction (PCR) was performed on the DNA obtained from the PEG stocks with the primers 27f (10 μM – 5′-AGAGTTTGATCMTGGCTCAG-3′: Lane, 1991) and 1492r (10 μM – 5′-GGTTACCTTGTTACGACTT-3′: Lane, 1991) and Promega Green (Promega, Madison, WI, United States). Partial 16S rRNA sequencing was performed with the primer BAC 341f primer (10 μM – 5′-CCTACGGGAGGCAGCAG-3′: Muyzer et al., 1993) using Big Dye Termination Mix (GeneCust Europe, Dudelange, Luxemburg). Quality control of the sequences was done manually using Sequencher 5.4.5. Quality-checked sequences were compared with type strain reference sequences of the National Center of Biotechnology Information (NCBI), and subsequently clustered according to a level of 99.5% identity. Representative sequences of each cluster were aligned using neighbor-joining algorithms of the MAFFT server (Katoh and Standley, 2013). Phylogenetic trees were constructed and visualized along with the trait and abundance data using Evolview (Zhang H. et al., 2012). All 16S rRNA gene sequences were deposited in the NCBI database with continuous accession numbers from MK637853 to MK638668 (Supplementary Table S1).
Estimating the Functional Properties of Isolated Bacteria
Pure cultures of the dominant P-solubilizing taxa were characterized by functional tests using agar plate bioassays. For each isolate, organic acid production to solubilize tri-calcium phosphate was quantitatively and qualitatively determined on Pikovskaya agar (Pikovskaya, 1948) by the formation of a clear zone in the otherwise turbid agar around the colony. Drought tolerance of the isolates was determined by comparing their growth on YME to surface polyethylene glycol 6000 (PEG) infiltrated YME. Thus, in this study drought tolerance potential of the strains was not related to the formation of dormant spores, but to their potential to maintain metabolisms and growth under water limiting conditions. To simulate severe drought stress, PEG concentration was set to 500 g/L, which corresponds to an osmotic potential level of around −1.1 MPa (Verslues et al., 2006).
For both bioassays, the strains were grown to the end of the exponential growth phase in liquid YME and 1 μl of each liquid pre-culture was applied on the test media in triplicates. P solubilization was assessed after 14 and drought tolerance after 3 days of incubation in the dark at 25°C. Phosphate solubilization was quantified as P release derived from phosphate concentration per area of plate and valued using two complementary indices. On the one hand, P release was calculated based on the whole area including colony plus halo, representing the total amount of P released by the colony (Phosphate Solubilization Index 1, PSI 1). Since primarily the outer cells of a colony determine the distance between colony rim and halo, we further calculated P release (Phosphate Solubilization Index 2, PSI 2) based solely on the halo around the colony (halo diameter = twice the distance from colony rim to the end of the halo), representing cell-specific, rather than colony-specific P solubilization potential. Drought stress tolerance was quantified based on the percentage differences of colony diameters on PEG-YME agar, compared to control YME agar. For statistical analysis only data from bacterial strains growing on the functional media were used.
Statistical Analyses
All statistical analyses were performed in the open source program R version 3.4.0 (2017-04-21), GNU project (R Core Team, 2017). An analysis of variance (ANOVA) was run to evaluate, if the abundance and traits of P-solubilizing bacteria were influenced by climate, land use, wheat growth stage, or interaction of these factors. The ANOVA was followed by a multiple comparison analysis with Tukey HSD to identify respective differences in the means of groups. To test for differences in phosphate solubilization potentials and drought tolerances between dominant bacterial taxa, the factor taxa was added to the formula resulting in a four factorial ANOVA. Significance levels were defined according to ***p < 0.001, **p < 0.01, and *p < 0.05.
To test for homogeneity of multivariate dispersion of Phyllobacterium, Pseudomonas and Streptomyces phylogenetic clusters among and between test factors climate, land use and wheat growth stage, betadisper function (R, vegan package) was run based on a dissimilarity matrix using relative abundance (Bray Curtis) and incidence data (Jaccard). Biodiversity indices, i.e., species richness and Shannon-Weaver-index were calculated to test for impacts of climate, land use and wheat growth stage on structure and composition of cultured Phyllobacterium, Pseudomonas and Streptomyces species. ANOVA was applied to test for differences in cluster dispersion regarding structure and composition of Phyllobacterium, Pseudomonas and Streptomyces species between and among test factors.
Results
Soil Chemical Parameters
Soil chemical properties of the studied samples are summarized in Supplementary Table S2. Since our study was performed only 2 years after establishment of the GCEF, basic soil parameters did not differ between treatments and showed comparable values of pH (6.7), total organic carbon (1.9%) and total nitrogen (0.16%). Available P and mineral N were higher in CF than in OF, but the difference was only significant for mineral N (Supplementary Table S2). The soil moisture differed between the ambient and future plots at the days of sampling and showed average values of 10.6 and 15.9% at stem elongation stage in May and at grain filling stage in July, respectively (Supplementary Table S2).
Effects of Wheat Growth Stage, Land Use and Climate on P-Solubilizing Rhizosphere Bacteria
Colony counts on Pikovskaya agar indicated higher numbers of P-solubilizing rhizobacteria at the stage of grain filling in July compared to the stage of stem elongation in May (p < 0.001). At both growth stages more bacteria were detected in CF than in OF (May: p = 0.008, July: p = 0.03) and under ambient climate than under future climate at grain filling stage in July (p = 0.02) (Supplementary Figure S2).
In total 410 bacterial isolates were obtained from samples collected at the stage of stem elongation and another 407 strains from samples at the grain filling stage (Supplementary Table S4). Within the isolates collected from the May samples at stem elongation stage, the dominant taxa (relative abundance ≥2%) belonged to the genera Pseudomonas (150 isolates = 37%), Streptomyces (81 isolates = 20%), Phyllobacterium (37 isolates = 9%), Rhizobium (20 isolates = 5%), Mesorhizobium (16 isolates = 4%), Bacillus (12 isolates = 3%) and Agrobacterium (10 isolates = 2%), while in the collection from July samples at the grain filling stage Phyllobacterium (147 isolates = 36%), Streptomyces (116 isolates = 29%), Rhizobium (24 isolates = 6%), Agrobacterium (20 isolates = 5%), Pseudomonas (17 isolates = 4%), Mesorhizobium (16 isolates = 4%), Ensifer (10 isolates = 2%) and Bacillus (9 isolates = 2%) (Supplementary Table S5) were the dominant genera. Thus, along both wheat growth stages, the taxa belonging to the genera Phyllobacterium, Pseudomonas and Streptomyces were found to dominate the bacterial collections, jointly accounting for 65% and 69% of the total isolates at stem elongation in May and grain filling stage in July, respectively. However, between the two plant growth stages, shifts in abundances of these dominant taxa were observed (Figures 1A,B and Supplementary Table S5). At the stage of stem elongation in May, Pseudomonas were predominant with 150 isolates accounting for more than one third of the bacterial isolates, whereas Phyllobacterium species only made up a small fraction with 37 isolates (9%). Conversely, at the grain filling stage in July, a much higher abundance of P-solubilizing Phyllobacterium species (147 isolates = 36%) than Pseudomonas species (17 isolates = 4%) was detected. Contrary to these, the abundance of Streptomyces isolates was less affected by the wheat growth stage, when 81 isolates (20%) and 116 isolates (29%) were obtained from stem elongation stage and grain filling stage, respectively.
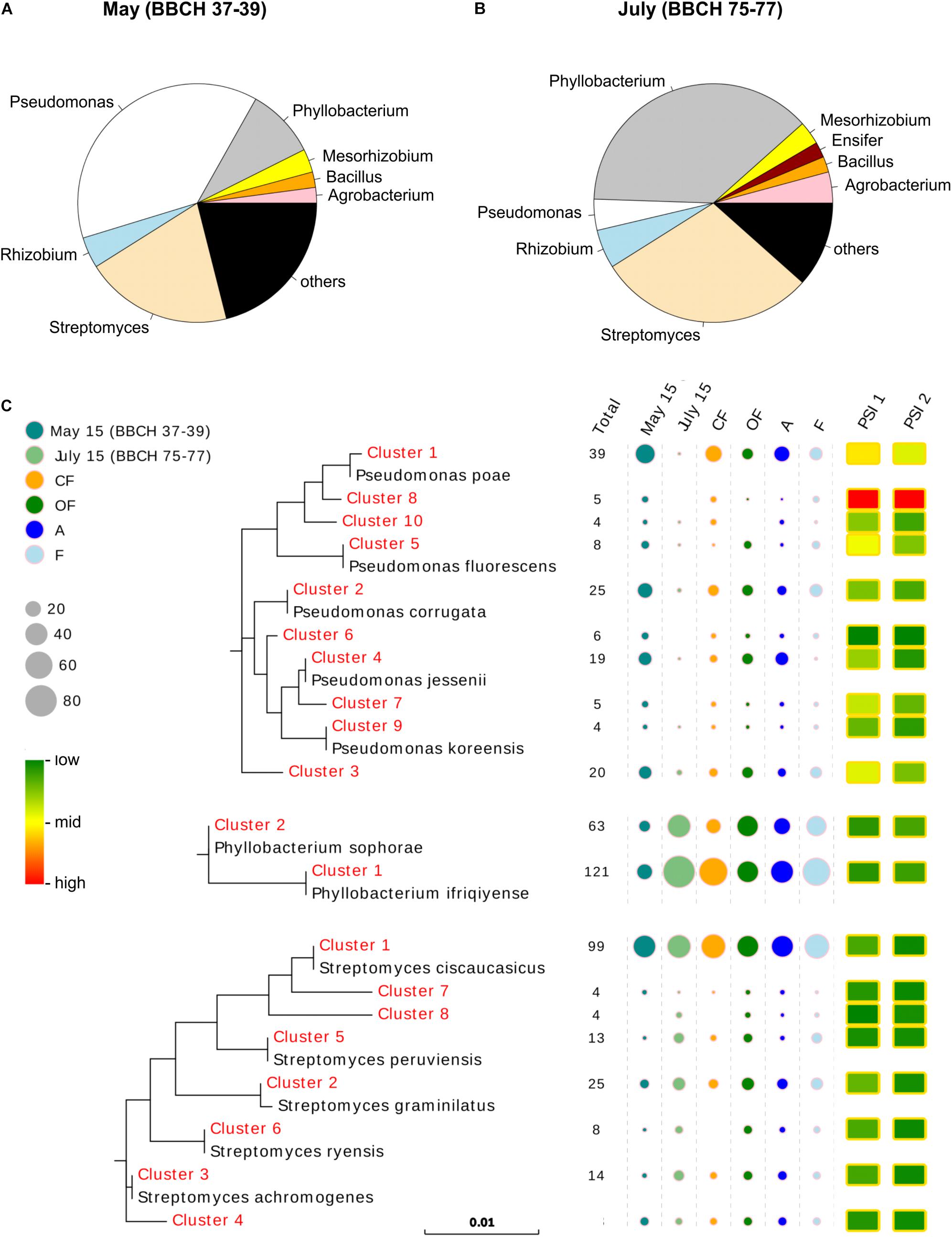
Figure 1. Identity, abundance and activity levels of cultivable mineral phosphate-solubilizing bacteria in the wheat rhizospheres. Taxa have been summarized on genus level and the most dominant ones, with a relative abundance ≥2% within the collection, are shown in the pie charts at (A) stem elongation stage in May 2015 and (B) grain filling stage in July 2015. The predominantly isolated taxa of the genus Phyllobacterium, Pseudomonas and Streptomyces were clustered (identity level of 99.5%) and (C) phylogenetic trees were created for dominant clusters (number of isolates >3) with reference sequences from NCBI. Information on abundance of the dominant clusters within treatment levels of growth stage (stem elongation in May, grain filling in July), climate (A-ambient, F-future) and land use (CF-conventional farming, OF-organic farming) are given as circles, whereas circle size is related to the number of isolates. Moreover, the average phosphate solubilizing potentials for each bacterial 16S rRNA gene cluster were calculated for PSI 1 and PSI 2 and differences in expression levels are indicated using a heatmap.
Comparable abundances along the two growth stages were also found for the less abundant taxa of the genus Agrobacterium, Bacillus and Rhizobium, while the genus Ensifer was predominantly detected in July (Figure 1B and Supplementary Table S5). Some of the less abundant genera also showed indications for land use and climate-specific patterns. Agrobacterium and Buttiauxiella were more frequently detected in CF than in OF, whereby Agrobacterium spp. were mainly isolated from ambient climate plots, while Buttiauxiella spp. were exclusively detected in future climate plots (Supplementary Table S5). A comparable enrichment under future climatic conditions was also observed for Bacillus, and species were more often isolated from OF than from CF (Supplementary Table S5).
To assess species identity and trait distribution within the dominant genera, we clustered the Phyllobacterium, Pseudomonas and Streptomyces isolates according to their 16S rRNA gene sequences at a similarity level of 99.5%. Phylogenetic trees were constructed with representative sequences of clusters comprising more than three isolates (Figure 1C), resulting in two 16S rRNA gene clusters for Phyllobacterium, ten for Pseudomonas and eight for Streptomyces. The clusters of Phyllobacterium species were related to Phyllobacterium ifriqiyense (cluster 1) and Phyllobacterium sophorae (cluster 2). Besides the strong impact of growth stage and the fact that more isolates were assigned to cluster one than to cluster two, the abundances of the Phyllobacterium clusters showed divergent patterns between the two farming systems. Cluster one was more abundant in CF than in OF, while cluster two showed a contrary trend (Figure 1C and Supplementary Table S6). Phylogenetic cluster distributions of the taxa within the genus Pseudomonas and Streptomyces were more heterogeneous. Pseudomonas clusters grouped with Pseudomonas poae (clusters 1, 8, and 10), Pseudomonas corrugate (cluster 2), Pseudomonas jessenii (cluster 4 and 7), Pseudomonas fluorescens (cluster 5), and Pseudomonas koreensis (cluster 9) (Figure 1C). Streptomyces cluster 1 contained the highest number of isolates and grouped with clusters 7 and 8, and reference sequence Streptomyces ciscaucasicus (Figure 1C). The other less abundant Streptomyces clusters grouped with reference sequences of Streptomyces graminilatus (cluster 2), Streptomyces achromogenes (clusters 3 and 4), Streptomyces peruviensis (cluster 5), and Streptomyces ryensis (cluster 6) (Figure 1C). Effects of climate and land use treatments on Streptomyces clusters could not be detected, but for Pseudomonas cluster 1. Representatives of this cluster were approximately twice as often isolated from CF and plots with ambient climatic conditions compared to OF and plots with future climatic conditions (Supplementary Table S6).
Dispersion patterns for Phyllobacterium, Pseudomonas and Streptomyces clusters significantly changed over the growing season (p = 0.001) (Figure 2A). At the genus level, compositional shifts were observed among the taxa of the genus Pseudomonas (p = 0.006) and Phyllobacterium (p = 0.04), but not for Streptomyces (Supplementary Figure S3). At cluster level, a shift in the cultivable fraction of the community was detected only for clusters of genus Pseudomonas (p = 0.02). Species richness (Figure 2B) and Shannon-Weaver diversity index (Figure 2C) were found to be higher at stem elongation stage of May samples compared to grain filling stage of July samples with p = 0.02 and p = 0.04, respectively. Effects of climate or land use on dispersion patterns and diversity indices could not be observed.
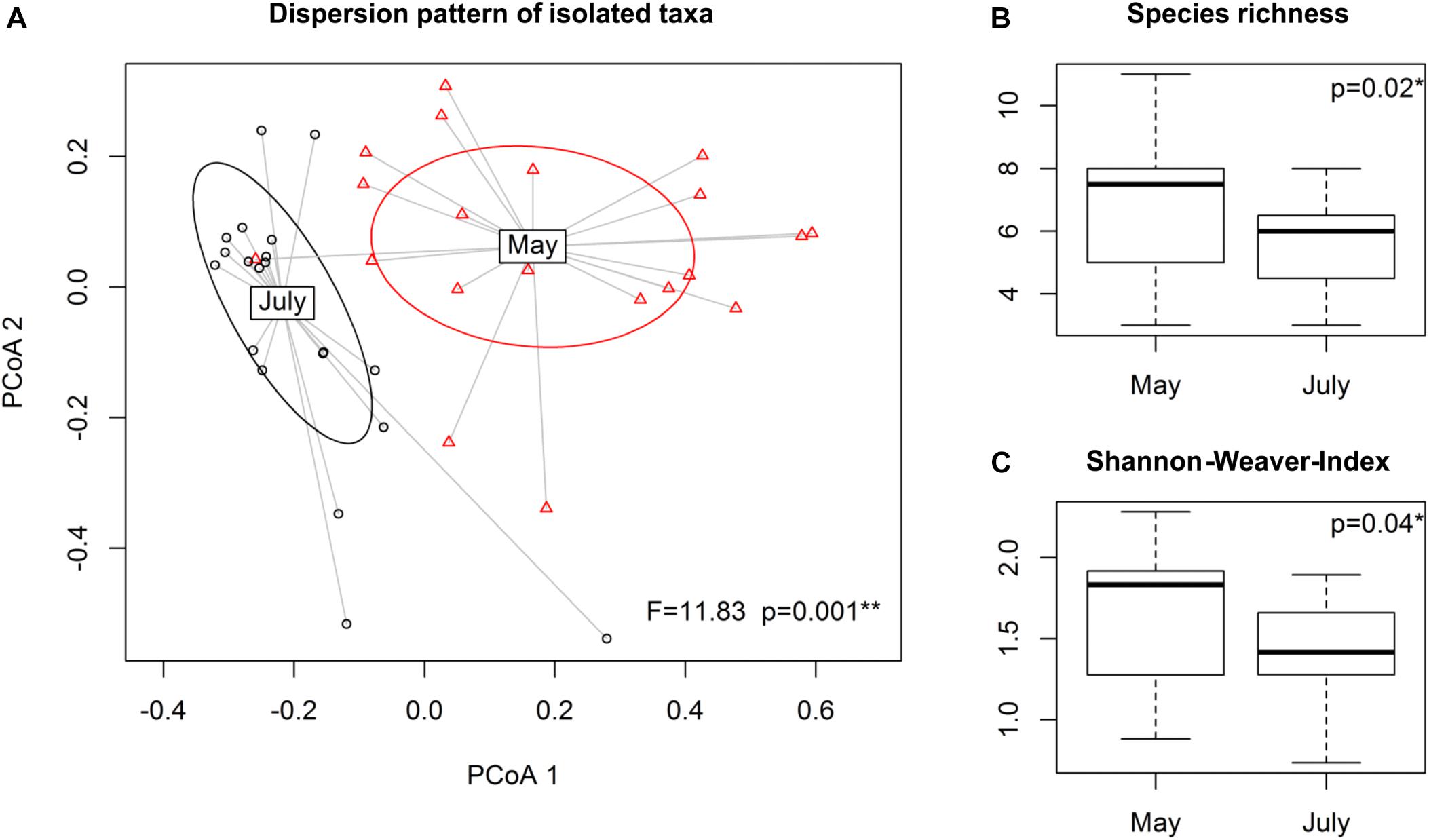
Figure 2. Effect of time on composition of isolated rhizobacterial taxa among and within the dominating genera Phyllobacterium, Pseudomonas and Streptomyces. Running betadisper function based on Bray-Curtis dissimilarity (A) dispersion patterns of the isolated taxa are presented in an ordination plot. Diversity indices (B) species richness and (C) Shannon-Weaver-Index were calculated, followed by ANOVA, testing for differences in isolated species composition of the three dominant genera between the two sampling points, May (stem elongation stage, BBCH 37-39) and July (grain filling stage, BBCH 75-77) 2015, respectively.
P Solubilization Potentials of Key Taxa
Phosphate-solubilizing potentials of dominant taxa within the genera Phyllobacterium, Pseudomonas, and Streptomyces isolated from the wheat rhizospheres at the stem elongation stage in May, as well as at the grain filling stage in July are presented for PSI 1 and PSI 2 in Figures 3A,B, respectively. Among the taxa of the three genera, Pseudomonas species expressed highest P solubilization potentials irrespective of employed indices, PSI 1 (Figure 3A) and PSI 2 (Figure 3B), and at the two growth stages studied. Phyllobacterium species showed approximately half of the P solubilization potential of the Pseudomonas species at both growth stages independently of the used P solubilization index. In contrast, the assessment of the P solubilization potentials of Streptomyces species depended on the index used. Excluding the colony size for the assessment of P solubilization, as done in PSI 2, clearly revealed significantly lower P solubilization potentials of Streptomyces species compared to taxa of the two other genera (p < 0.001) (Figure 3B).
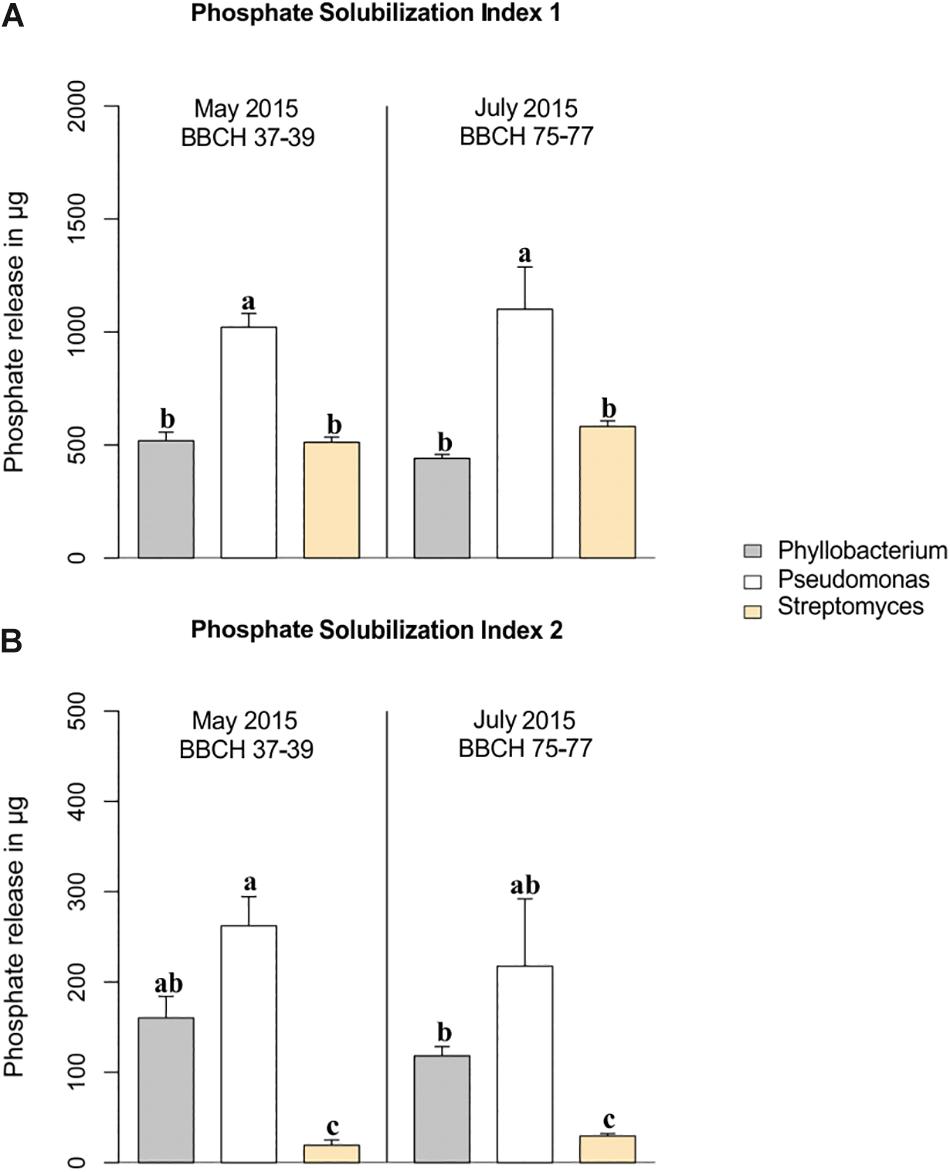
Figure 3. Phosphate-solubilizing potentials of the three dominant genera. Comparison of the two indices, (A) PSI 1 and (B) PSI 2 for Phyllobacterium, Pseudomonas und Streptomyces isolates at stem elongation in May and at grain filling in July 2015 sampled from wheat rhizosphere. Standard errors are given in the bars. Significant differences were calculated for the both indices separately and are marked by different letters (ANOVA and Tukey HSD Test).
Regarding the effect of studied impact factors within the three dominant genera, neither plant growth stage (Figure 3), nor climate or land use affected the P solubilization potential of Phyllobacterium and Streptomyces. However, interaction effects of climate and land use were found for Pseudomonas species, which showed higher P solubilization potentials under future climatic conditions compared to ambient climatic conditions in CF (p < 0.001 for PSI 2) (Supplementary Figure S4). Furthermore, under future climatic conditions, P solubilization potentials of Pseudomonas species were significantly higher in CF compared to OF (p < 0.001 for both indices, Supplementary Figure S4).
The phylogenetic cluster-specific, mean P solubilization potentials of the genera Phyllobacterium and Streptomyces revealed consistent P solubilization potentials in either employed index (Figure 1C and Supplementary Table S6). In contrast to that, the average P solubilization potentials of the individual clusters assigned to the genus Pseudomonas showed a huge variance (Figure 1C and Supplementary Table S6). While, Pseudomonas cluster 6 showed a low P solubilization potential, the isolates assigned to cluster 8 expressed the highest average P solubilization potential among all clusters of all three dominant genera. Even more important, the most abundant Pseudomonas cluster (cluster 1, represented by 39 isolates = 23% of all Pseudomonas isolates) showed a high average P solubilization potential which exceeded mean P solubilization of all genera and clusters, besides the aforementioned Pseudomonas cluster 8 (Figure 1C and Supplementary Table S6).
Drought Tolerance of Key Taxa
Drought stress tolerance was quantified as relative difference in colony size on PEG medium compared to control medium. Thus, higher drought tolerance was reflected by higher maintenance of colony size (maximum 100%) and vice versa. Pseudomonas species (35.1% at stem elongation in May and 35.7% at grain filling stage in July) and Streptomyces species (43.3% in May and 46.7% in July) were less resistant to drought compared to species assigned to the genus Phyllobacterium (54.1% in May and 63.6% in July) (Table 1). Although the low number of isolates at stem elongation in May samples prevents from drawing significant conclusions, species drought tolerance of genus Phyllobacterium was improved in the course of the growing season (Table 1). Total drought tolerance of taxa belonging to the genera Pseudomonas and Streptomyces did not change throughout the observed growth stages, but show different behavior under ambient and future climatic conditions. Under ambient climate an increase and under future climate conditions a decrease of drought tolerance could be observed for Pseudomonas species, while for Streptomyces species the opposite pattern showed up (Table 1). Land use did not impact key genera’s drought tolerance, but the climate treatment affected average drought tolerance of the genus Streptomyces. Strains isolated from plots with future climatic conditions showed higher drought tolerance (52.4%) as compared to those isolated from plots with ambient climate (38.6%) (p = 0.01). This trend was also observed at the cluster level, even though it was not significant due to the low number of isolates per cluster (Supplementary Figure S5). In contrast to streptomycetes, climate treatment showed no effect on the drought tolerance of taxa within the genera Phyllobacterium and Pseudomonas.
At the cluster level, a marginal higher drought tolerance of the species isolated from plots with future climatic conditions, compared to those isolated from ambient plots was observed for Phyllobacterium cluster 2 (Supplementary Figure S5). Within the genus Pseudomonas, we observed no increase in drought tolerance of species from future climate plots except for the low abundant cluster 10 and, to a lower extent, for cluster 3 and cluster 8 (Supplementary Figure S5). Overall, species of both Phyllobacterium clusters exhibit a significantly higher drought tolerance as compared to those of the predominant clusters of Pseudomonas (clusters 1 to 4) to and Streptomyces species (clusters 1 to 4) (Supplementary Figure S5 and Supplementary Table S6).
Discussion
In this study we have shown that members of the genera Phyllobacterium, Pseudomonas and Streptomyces are predominant within the cultivable fraction of the P-solubilizing community in the wheat rhizosphere along both growth stages studied, namely vegetative biomass production (BBCH 37–39, stem elongation) and generative biomass production stages (BBCH 75–77, grain filling). All taxa within the three dominant genera showed high resilience against differences in studied agricultural management practices and climatic conditions, but structure shifted with wheat growth stages. This shift was mainly driven by changes among P-solubilizing taxa of the genera Pseudomonas and Phyllobacterium, as well as on single taxa level within the genus Pseudomonas. The considerable in vitro P solubilization potential of both genera Pseudomonas and Phyllobacterium pleads for functional redundancy upon structural changes within the cultivable fraction of the P-solubilizing community. While the genera Pseudomonas and Streptomyces comprise well-known P solubilizers, Phyllobacterium species have rarely been associated with P solubilization. Considering their medium-borne P solubilization potential in combination with an accentuated tolerance of actively growing colonies to drought conditions, Phyllobacterium species may play an important role in P supply for plants under drought.
Wheat Growth Stage and Farming System Drive Abundance and Identity of Phosphate-Solubilizing Rhizobacteria
The abundance of cultivable P-solubilizing rhizobacteria (PSR), mirrored by CFU per gram dry soil, was higher at the grain filling stage of wheat in July than at the stem elongation stage in May and contradicts our assumptions made in the first hypothesis. This discrepancy might be related to higher soil moisture at the day of sampling in July, since increased water availability supports microbial growth and enhances metabolic activities (Williams and Rice, 2007). Due to the realistic scenario-based approach of the GCEF, the soil moisture is mainly dependent on the actual weather. However, the impact of the climate treatment was clearly evident for the annual soil moisture dynamics of 2015 (Schädler et al., 2019) and led to significantly reduced winter wheat yields under future climatic conditions compared to ambient ones (Supplementary Table S3).
According to our third hypothesis, the conventional farming (CF) showed higher PSR numbers than the organic farming (OF), which is likely related to the higher nutrient availability in CF. Physicochemical soil parameters are well known as important drivers of microbial biomass (Widmer et al., 2006) as well as of the community structure and composition (Cleveland and Liptzin, 2007; Lauber et al., 2008; Francioli et al., 2016). Nevertheless, we consistently identified Phyllobacterium, Pseudomonas and Streptomyces species as the predominant cultivable PSR in both farming systems. In general, plants recruit beneficial PGPR from the surrounding soil to their rhizospheres (Badri and Vivanco, 2009) by plant specific rhizodeposition patterns (Paterson et al., 2007). This selection effect may mask environmental impacts on the community structure and composition in the rhizosphere and, thus, explain the low differences in the identity of predominant PSR isolated across both farming systems.
Phosphate-Solubilizing Potential First Time Reported for P. ifriqiyense and P. sophorae
Multiple studies report Streptomyces (Molla et al., 1984; Jog et al., 2014) and Pseudomonas species to be strong P solubilizers (e.g., Richardson et al., 2009; Marasco et al., 2012; Sharma et al., 2013) and successful colonizers of the wheat rhizosphere (Mohammadi, 2012; Jog et al., 2014). Conversely, members of the genus Phyllobacterium, taxonomically classified to the order Rhizobiales, are hardly described for their P solubilization potential, but rather for their biocontrol activities (Lambert et al., 1990; Aisyah et al., 2017) or the capacity to fix nitrogen (Rojas et al., 2001). Our study now provides evidence for the potential of two Phyllobacterium species, namely P. ifriqiyense and P. sophorae, to in vitro solubilize P from its bound mineral form in the rhizosphere of winter wheat in the temperate climatic zone. The two strains have first been isolated from root nodules of Astragalus algerianus and Lathyrus numidicus in South Tunisia - P. ifriqiyense - (Mantelin et al., 2006), and of Sophora flavescens in China - P. sophorae - (Jiao et al., 2015). Only two further previous studies indicate the potential of Phyllobacterium myrsinacearum for P solubilization in subtropical soils (Chen et al., 2006) and in metal-polluted soils (Ma et al., 2013). Genome shotgun sequencing of Phyllobacterium sp. (National Center of Biotechnology Information, NCBI) revealed quinoprotein glucose dehydrogenase coding genes. These genes are involved in gluconic acid production and, thus, suggest a potential for mineral phosphate solubilization (Rodríguez and Fraga, 1999). This coding gene has also been indicated for Pseudomonas species (Miller et al., 2010) and Streptomyces species (Jog et al., 2014) and may, therefore, serve as indicator for P solubilization potential of Phyllobacteria in vivo. Therefore, the contribution of Phyllobacterium species to phosphate solubilization in soils and rhizospheres might be still underestimated.
Complementarity Between Phyllobacterium and Pseudomonas at Different Wheat Growth Stages
Abundances of P-solubilizing Phyllobacterium and Pseudomonas isolates were complementary between the two wheat growth stages. Since an isolation-based approach was used, this complementarity mirrors different proportions of active microorganisms. Blagodatskaya and Kuzyakov (2013) suggested that more than 95% of the total microbial biomass are inactive at a given time point. Accordingly, active growth and dormancy phases of rhizosphere species are dependent on nutrient levels, interspecific competition as well as on the plant growth stage and its related rhizodeposition (Blagodatskaya and Kuzyakov, 2013). Plant nutrient uptake and root exudation were reported to be high at stem elongation stage and considerably lower at grain filling (Aulakh et al., 2001; Malhi et al., 2006). Of note, Pseudomonas species were predominantly isolated from the wheat rhizosphere at the stage of stem elongation in May. Members of this genus are well characterized for their rapid growth, high chemotactic activity and motility toward root exudates (Scher et al., 1988; Walsh et al., 2001), as well as the production of secondary metabolites (Haas and Defago, 2005) and secretion of siderophores (Kloepper et al., 1980), to metabolize rhizodeposits and outcompete other microorganisms (Paulsen et al., 2005). Indeed, root colonization of active Phyllobacterium species may be inhibited by pseudomonads competing for root exudates at the stage of high biomass production in May. Even though Phyllobacterium species are described to live in a large variety of habitats and to “communicate” with plant tissues, the group is also known to be non-pathogenic (Mantelin et al., 2006). When plants reach their mature state, the release of root exudates and competition between microorganisms in the rhizosphere decreases drastically (Hamlen et al., 1972), likely explaining the decline in abundance of Pseudomonas and the enrichment of Phyllobacterium species at the grain filling stage. Antagonistic effects have not been tested within this study, but may provide important information on colonization success in plant rhizospheres at different growth stages.
Resistance and Functional Redundancy in Terms of P Solubilization Between and Among Dominant Phosphate Solubilizing Genera
Besides abundance shifts, the cluster dispersion patterns of the cultivated dominant taxa varied significantly between the two time points with increased species richness at the stage of stem elongation in May samples. This partly supports our first hypothesis, since the diversity decrease in July is in line with reduced carbon and nutrient availabilities (Hamlen et al., 1972). The shifts within the cultivable fraction of the PSR community were based on two different responses: changes in the dominant PSR taxa, which are mainly driven by shifts within the genera Phyllobacterium and Pseudomonas, as well as altered species composition of Pseudomonas species. Changes in the structure and identity of Pseudomonas species at different wheat growth stages indicate that upon a change in the environmental conditions, the culture derived Pseudomonas community is dynamic. Concurrent changes in community structure and composition according to plants growth stages are widely observed in ecology (Dunfield and Germida, 2003; Wolsing and Priemé, 2004; Francioli et al., 2018). As we found moderate to high average P solubilization potentials of taxa classified to the genera Pseudomonas and Phyllobacterium at the two growth stages studied, the observed shifts plead for functional redundancy (Allison and Martiny, 2008) between and among genera at different wheat growth stages.
Besides the effect of different growth stages over the growing season, an effect of farming system was found. Increased P solubilization potentials for Pseudomonas isolates from CF, compared to OF, were detected under future climatic conditions. The mechanisms behind this observation cannot be revealed within this study, but we assume soil nutrient availability to play a role in the activity of PSR under drought. Interestingly, this finding is, however, supported by a previous study performed in grasslands, where we found higher P solubilization potentials of Pseudomonas species under low soil moisture conditions (Breitkreuz, unpublished). Within this study, we found comparable P solubilization potentials expressed by the predominant taxa along both time points, which is in contrast to our assumptions made in hypothesis one that P solubilization potential is higher at the stem elongation stage. Higher activities of Pseudomonas species in CF under future climate contradicts hypothesis three expecting higher P solubilization potential in OF than in CF.
The abundances of isolated Streptomyces species, but also the structure and composition within the genus were only slightly affected by the wheat growth stage and not at all by the climate or land use treatments. Despite their high abundance, P solubilization potential expressed according to PSI 2 were significantly less pronounced compared to those of the isolated Phyllobacterium and Pseudomonas species. However, Battini et al. (2017) recently emphasized the capability of Streptomyces species to facilitate P uptake into fungal hyphae in root free compartments, which is subsequently transported via the mycorrhizosphere to maize plants. In fact, Tarkka et al. (in preparation) isolated phosphate solubilizing bacteria from bulk soils of conventional and organic farming plots of the GCEF and identified Streptomyces species among the most abundant phosphate solubilizes. Their lower phosphate solubilizing potentials may be caused by slower development rates and delayed activity expression, compared to the mainly fast growing Phyllobacterium and Pseudomonas species (Garrity et al., 2007). The slow growth of streptomycetes is related to the formation of complex structures, e.g., branching hyphal filaments and spores (Chater et al., 2010). Dormant spores in the soil are beneficial to endure severe drought events waiting for more favorable conditions (Viaene et al., 2016). In line with this, recently published studies observed accumulation of actinobacteria in drought-treated soils and rhizospheres of different plants (Bouskill et al., 2016; Taketani et al., 2017).
For estimating the strain-specific P solubilization potentials, two complementary indices were calculated. PSI 1, which is based on the total colony and halo size, is directly related to the total amount of released P, but the values are strongly biased by the respective colony size. Consequently, identical distances between halo and colony rim along with larger colony sizes may overrate the activity potential since bigger colonies have higher area values and vice versa. The same problem occurs when using the commonly published ratios, halo to colony size (e.g., Nguyen et al., 1992; Kumar and Narula, 1999; Pérez et al., 2007). However, the diffusion expanse of organic acids in the surrounding of bacterial colonies to solubilize bound P is mainly determined by the outermost cells of the colony. PSI 2 remedies the strong impact of the colony size that, in our case for Streptomyces species, may impact appraisal of the P solubilization potential. We thus suggest to focus on approaches based on diffusion expanse (i.e., the distance between halo and colony) for estimation of phosphate solubilizing potential when using plate bioassays.
Drought Tolerance of P-Solubilizing Bacteria
The tolerance of the predominant taxa against severe drought stress was quantified in this study by water deficit bioassays with polyethylene glycol 6000 (PEG). Using this approach, we focused on whether isolated strains remained physiologically active under low moisture conditions. In this respect, evaluating growth on nutrient agar with PEG may provide information on active PSR under drought conditions.
As assumed in our second hypothesis, we consistently observed a (by trend) increased drought tolerance of the dominant taxa isolated at stage of grain filling from July as compared to those at stem elongation from May. This indicates enhanced adaptive bacterial responses against dehydration stress in summer. While we detected no land use effect on drought tolerance, we found a higher drought tolerance for Streptomyces species, when isolated from plots under future climatic conditions, compared to those from ambient climate plots. Overall, we observed the highest drought tolerance for Phyllobacterium species, followed by Streptomyces and consecutively Pseudomonas species. Soil microorganisms tolerate water stress in a variable extent (Manzoni et al., 2012). As a most common mitigation strategy, bacteria as well as eukaryotes accumulate low molecular weight compounds, so called osmolytes, once water potentials decrease (Kempf and Bremer, 1998). These compounds do not interfere with cellular functions, but stabilize cellular structures and prevent desiccation. Their production and accumulation in the abundant clusters observed within this study is established, for Phyllobacterium (Fujihara, 2008; Sagot et al., 2010; Zaprasis et al., 2015), but also for Pseudomonas species, when isolated from arid or semi-arid regions of India (Sandhya et al., 2009). However, Lennon et al. (2012) performed a study in (MI, United States, humid region) and found with decreasing water potentials that respiration of Pseudomonas species drastically dropped, which supports the findings for Pseudomonas in our study.
Field Application Potential of P-Solubilizing Bacteria
Besides rhizosphere competence, the trait combination of high P solubilization potential and drought tolerance is a prerequisite for field applications. Inoculation with microbial species of the genera Bacillus and Paenibacillus (Zhang J. et al., 2012; Kasim et al., 2013; Kumar et al., 2014; Timmusk et al., 2014), as well as Azospirillum and Pseudomonas (Creus et al., 2004; Naiman et al., 2009; Ilyas and Bano, 2010; Kasim et al., 2013) and Streptomyces (Jog et al., 2014) was found to increase wheat yields in field and pot experiments. In particular, Pseudomonas species are well investigated, as the genus comprises species with promising plant growth promoting properties (Burr et al., 1978; Naiman et al., 2009; Rajkumar et al., 2010). In contrast, prior to this study little was known about P-solubilizing Phyllobacterium species, or their drought tolerance in wheat rhizosphere. They do act as PGPR e.g., in canola plants (Bertrand et al., 2001), but underlying mechanisms remain unclear. Model studies with Arabidopsis thaliana and Phyllobacterium brassicacearum indicated higher drought tolerance (Bresson et al., 2014). In the same context, Kechid et al. (2013) observed a delay in reproduction and lowered transpiration rate of Arabidopsis thaliana upon inoculation with Phyllobacterium brassicacearum accompanied by stimulated lateral root and root hair growth. Within this study we could prove in vitro potential for P-solubilization of two taxa in the genus Phyllobacterium, which has to be further justified in vivo under different biotic and abiotic conditions. To check applicability of these species as phosphate delivering biofertilizers, the timing of inoculation and bacterial level has to be defined in pretests to ensure survival of the inoculated strains, but also to keep viability of the seeds. Bashan (1986) found inoculation at early growth stages of wheat with 105–106 colony forming units per ml to be optimal for Azospirillum and Pseudomonas strains to increase productivity. The success of colonization depends on different factors and is favored by high mobility of the bacteria along the growing root, rapid multiplication and the ability to colonize the rhizoplane and inner root tissues (Höflich et al., 1995). Therefore, the colonization potential for different plant root systems, the effect of different soil parameters and antagonistic effects within the native microbial community has to be quantified in inoculation experiments under natural (non-sterile) and controlled (sterile) conditions (Gaskins et al., 1985; Höflich et al., 1995). Finally, the strains have to be tested for their plant growth promoting activities exploiting various sources of insoluble P in the soil, besides tri-calcium phosphate also other insoluble inorganic and organic phosphate sources (Bashan et al., 2013), and their drought stress tolerance under natural conditions in pot and field experiments. Nevertheless, the non-pathogenic status and high plant-interaction potential of Phyllobacterium species, along with the considerable P solubilization potential in vitro and high drought tolerance revealed in this study, make this group attractive for possible future application as plant growth promoting inoculants for wheat production.
Conclusion
Our findings suggest that the bacterial taxa involved in the provision of P in the rhizosphere of wheat plants depend on plant’s growth stage and only minor on farming system and climate under in vitro conditions. This observation clearly pleads for more studies at defined and yield-determining stages of plant growth under in vitro and in vivo conditions. We found that Pseudomonas species were dominant at the stem elongation stage, i.e., the peak of vegetative biomass production, while Phyllobacterium species dominated at the grain filling stage (generative stage). This is the first time that the two isolated Phyllobacterium species are reported for their high P solubilization potential. Drought tolerance potentials of the three dominant genera were found to be increased at the second growth stage studied in July compared to the first one in May indicating an adaptation to drought over time and a higher tolerance in summer months. Phyllobacterium species expressed highest drought tolerance potential among isolated dominant phosphate solubilizing rhizobacteria. Therefore, further work should concentrate on functionality and abundance of this genus in rhizospheres of plants. Especially in the context of climate change related summer drought, the observed trait combination of Phyllobacterium species in this study may be of particular importance to adapt agriculture to dryer conditions in the future.
Data Availability Statement
The raw data supporting the conclusions of this manuscript will be made available by the authors, without undue reservation, to any qualified researcher.
Author Contributions
All authors conceived and designed the experiments, obtained funding, interpreted the results, and contributed to revisions and approved submission of the manuscript. TR and CB performed the field experiments. CB performed the laboratory works and data analysis. MT, TR, and CB wrote the manuscript with input from FB.
Conflict of Interest
The authors declare that the research was conducted in the absence of any commercial or financial relationships that could be construed as a potential conflict of interest.
Funding
The work has been done in the frame of a scholarship funded by the “Deutsche Bundesstiftung Umwelt” (DBU) with the topic “Einfluss pflanzenwachstumsfördender Rhizobakterien auf die Stressresistenz der Kulturpflanze Weizen (Triticum aestivum L.)” (AZ:20015/391).
Acknowledgments
We appreciate the Helmholtz Association, the Federal Ministry of Education and Research, the State Ministry of Science and Economy of Saxony-Anhalt and the State Ministry for Higher Education, Research and the Arts Saxony, to fund the Global Change Experimental Facility (GCEF) project. We want to thank the staff of the Bad Lauchstädt Experimental Research Station (especially Ines Merbach and Konrad Kirsch) and Martin Schädler for their work in maintaining the plots and infrastructures of the GCEF, as well as Harald Auge, Stefan Klotz, and Martin Schädler for their role in setting up the GCEF. Special thanks go to Julia Siebert and Tom Künne, and all helpers participated in soil and rhizosphere sampling campaigns in 2015. We further want to thank Kerstin Hommel, Beatrix Schnabel, and Laura Herzig for their support in lab works. Finally we thank the Ph.D. Advisory Committee of this project: Prof. Dr. Nico Eisenhauer and Dr. Petra Högy for their input and fruitful discussions on data analysis and interpretation.
Supplementary Material
The Supplementary Material for this article can be found online at: https://www.frontiersin.org/articles/10.3389/fmicb.2019.03109/full#supplementary-material
References
Aisyah, S. N., Sulastri, S., Retmi, R., Yani, R., Syafriani, E., Syukriani, L., et al. (2017). Suppression of colletotrichum gloeosporioides by indigenous Phyllobacterium and its compatibility with rhizobacteria. Asian J. Plant Pathol. 11, 139–147. doi: 10.3923/ajppaj.2017.139.147
Allison, S. D., and Martiny, J. B. H. (2008). Resistance, resilience, and redundancy in microbial communities. Proc. Natl. Acad. Sci. U.S.A. 105, 11512–11519. doi: 10.1073/pnas.0801925105
Altermann, M., Rinklebe, J., Merbach, I., Körschens, M., Langer, U., and Hofmann, B. (2005). Chernozem-soil of the year 2005. J. Plant Nutr. Soil Sci. 168, 725–740. doi: 10.1002/jpln.200521814
Artursson, V., Finlay, R. D., and Jansson, J. K. (2006). Interactions between arbuscular mycorrhizal fungi and bacteria and their potential for stimulating plant growth. Environ. Microbiol. 8, 1–10. doi: 10.1111/j.1462-2920.2005.00942.x
Aulakh, M. S., Wassmann, R., Bueno, C., Kreuzwieser, J., and Rennenberg, H. (2001). Characterization of root exudates at different growth stages of ten rice (Oryza sativa L.) cultivars. Plant Biol. 3, 139–148. doi: 10.1055/s-2001-12905
Badri, D. V., and Vivanco, J. M. (2009). Regulation and function of root exudates. Plant Cell Environ. 32, 666–681. doi: 10.1111/j.1365-3040.2008.01926.x
Bais, H. P., Weir, T. L., Perry, L. G., Gilroy, S., and Vivanco, J. M. (2006). The role of root exudates in rhizosphere interactions with plants and other organisms. Annu. Rev. Plant Biol. 57, 233–266. doi: 10.1146/annurev.arplant.57.032905.105159
Banerjee, S., Walder, F., Büchi, L., Meyer, M., Held, A. Y., Gattinger, A., et al. (2019). Agricultural intensification reduces microbial network complexity and the abundance of keystone taxa in roots. ISME J. 13, 1722–1736. doi: 10.1038/s41396-019-0383-2
Bashan, Y. (1986). Significance of timing and level of inoculation with rhizosphere bacteria on wheat plants. Soil Biol. Biochem. 18, 297–301. doi: 10.1016/0038-0717(86)90064-7
Bashan, Y., Kamnev, A. A., and de-Bashan, L. E. (2013). Tricalcium phosphate is inappropriate as a universal selection factor for isolating and testing phosphate-solubilizing bacteria that enhance plant growth: a proposal for an alternative procedure. Biol. Fertil. Soils 49, 465–479. doi: 10.1007/s00374-012-0737-7
Battini, F., Gronlund, M., Agnolucci, M., Giovannetti, M., and Jakobsen, I. (2017). Facilitation of phosphorus uptake in maize plants by mycorrhizosphere bacteria. Sci. Rep. 7:4686. doi: 10.1038/s41598-017-04959-0
Bertrand, H., Nalin, R., Bally, R., and Cleyet-Marel, J.-C. (2001). Isolation and identification of the most efficient plant growth-promoting bacteria associated with canola (Brassica napus). Biol. Fertil. Soils 33, 152–156. doi: 10.1007/s003740000305
Blagodatskaya, E., and Kuzyakov, Y. (2013). Active microorganisms in soil: critical review of estimation criteria and approaches. Soil Biol. Biochem. 67, 192–211. doi: 10.1016/j.soilbio.2013.08.024
Bouskill, N. J., Wood, T. E., and Baran, R. (2016). Belowground response to drought in a tropical forest soil. I. changes in microbial functional potential and metabolism. Front. Microbiol. 7:525. doi: 10.3389/fmicb.2016.00525
Bresson, J., Vasseur, F., Dauzat, M., Labadie, M., Varoquaux, F., Touraine, B., et al. (2014). Interact to survive: Phyllobacterium brassicacearum improves Arabidopsis tolerance to severe water deficit and growth recovery. PLoS One 9:e107607. doi: 10.1371/journal.pone.0107607
Burr, T., Schroth, M., and Suslow, T. (1978). Increased potato yields by treatment of seed pieces with specific strains of Pseudomonas fluorescens and P. putida. Phytopathology 68, 1377–1383.
Chater, K. F., Biro, S., Lee, K. J., Palmer, T., and Schrempf, H. (2010). The complex extracellular biology of Streptomyces. FEMS Microbiol. Rev. 34, 171–198.
Chen, Y. P., Rekha, P. D., Arun, A. B., Shen, F. T., Lai, W. A., and Young, C. C. (2006). Phosphate solubilizing bacteria from subtropical soil and their tricalcium phosphate solubilizing abilities. Appl. Soil Ecol. 34, 33–41. doi: 10.1016/j.apsoil.2005.12.002
Ciais, P., Reichstein, M., and Viovy, N. (2005). Europe-wide reduction in primary productivity caused by the heat and drought in 2003. Nature 437:529. doi: 10.1038/nature03972
Cleveland, C. C., and Liptzin, D. (2007). C: N: p stoichiometry in soil: is there a “Redfield ratio” for the microbial biomass? Biogeochemistry 85, 235–252. doi: 10.1007/s10533-007-9132-0
Cordell, D., Drangert, J. O., and White, S. (2009). The story of phosphorus: global food security and food for thought. Glob. Environ. Change 19, 292–305. doi: 10.1016/j.gloenvcha.2008.10.009
Creus, C. M., Sueldo, R. J., and Barassi, C. A. (2004). Water relations and yield in Azospirillum-inoculated wheat exposed to drought in the field. Can. J. Botany 82, 273–281. doi: 10.1139/b03-119
de Vries, F. T., and Shade, A. (2013). Controls on soil microbial community stability under climate change. Front. Microbiol. 4:265. doi: 10.3389/fmicb.2013.00265
Dunfield, K. E., and Germida, J. J. (2003). Seasonal changes in the rhizosphere microbial communities associated with field-grown genetically modified canola (Brassica napus). Appl. Environ. Microbiol. 69, 7310–7318. doi: 10.1128/aem.69.12.7310-7318.2003
Elliott, E. T., Hunt, H. W., and Walter, D. E. (1988). Detrital foodweb interactions in North American grassland ecosystems. Agric. Ecosyst. Environm. 24, 41–56. doi: 10.1016/0167-8809(88)90055-2
Fischer, C. (2003). Geographie Infothek: Weizen. Klettverlag, Leipzig. Available at: https://www.klett.de/alias/1010150 (accessed February 28, 2019).
Fischer, S. E., Fischer, S. I., Magris, S., and Mori, G. B. (2007). Isolation and characterization of bacteria from the rhizosphere of wheat. World J. Microbiol. Biotechnol. 23, 895–903.
Francioli, D., Schulz, E., Buscot, F., and Reitz, T. (2018). Dynamics of soil bacterial communities over a vegetation season relate to both soil nutrient status and plant growth phenology. Microb. Ecol. 75, 216–227. doi: 10.1007/s00248-017-1012-0
Francioli, D., Schulz, E., Purahong, W., Buscot, F., and Reitz, T. (2016). Reinoculation elucidates mechanisms of bacterial community assembly in soil and reveals undetected microbes. Biol. Fertil. Soils 52, 1073–1083. doi: 10.1007/s00374-016-1141-5
Frey, S. D., Elliott, E. T., and Paustian, K. (1999). Bacterial and fungal abundance and biomass in conventional and no-tillage agroecosystems along two climatic gradients. Soil Biol. Biochem. 31, 573–585. doi: 10.1016/s0038-0717(98)00161-8
Fujihara, S. (2008). Biogenic amines in rhizobia and legume root nodules. Microb. Environ. 24, 1–13. doi: 10.1264/jsme2.me08557
Garrity, G., Brenner, D. J., Krieg, N. R., and Staley, J. R. (2007). Bergey’s Manual® of Systematic Bacteriology: Volume 2: The Proteobacteria, Part B: The Gammaproteobacteria. Berlin: Springer.
Gaskins, M. H., Albrecht, S. L., and Hubbell, D. H. (1985). Rhizosphere bacteria and their use to increase plant productivity: a review. Agric. Ecosyst. Environ. 12, 99–116. doi: 10.1016/0167-8809(85)90071-4
Haas, D., and Defago, G. (2005). Biological control of soil-borne pathogens by fluorescent pseudomonads. Nat. Rev. Microbiol. 3, 307–319. doi: 10.1038/nrmicro1129
Hamlen, R. A., Lukezic, F. L., and Bloom, J. R. (1972). Influence of age and stage of development on the neutral carbohydrate components in root exudates from alfalfa plants grown in a gnotobiotic environment. Can. J. Plant Sci. 52, 633–642. doi: 10.4141/cjps72-097
Hendrix, P. F., Parmelee, R. W., Crossley, D. A. Jr., Coleman, D. C., Odum, E. P., and Groffman, P. M. (1986). Detritus food webs in conventional and no-tillage agroecosystems. Bioscience 36, 374–380. doi: 10.2307/1310259
Höflich, G., Wiehe, W., and Hecht-Buchholz, C. (1995). Rhizosphere colonization of different crops with growth promoting Pseudomonas and Rhizobium bacteria. Microbiol. Res. 150, 139–147. doi: 10.1016/s0944-5013(11)80048-0
Ilyas, N., and Bano, A. (2010). Azospirillum strains isolated from roots and rhizosphere soil of wheat (Triticum aestivum L.) grown under different soil moisture conditions. Biol. Fertil. Soil 46, 393–406. doi: 10.1007/s00374-009-0438-z
Jiao, Y. S., Yan, H., Ji, Z. J., Liu, Y. H., Sui, X. H., Zhang, X. X., et al. (2015). Phyllobacterium sophorae sp. nov., a symbiotic bacterium isolated from root nodules of Sophora flavescens. Int. J. Syst. Evol. Microbiol. 65, 399–406. doi: 10.1099/ijs.0.067017-0
Jog, R., Pandya, M., Nareshkumar, G., and Rajkumar, S. (2014). Mechanism of phosphate solubilization and antifungal activity of Streptomyces spp. isolated from wheat roots and rhizosphere and their application in improving plant growth. Microbiology 160, 778–788. doi: 10.1099/mic.0.074146-0
Juhnke, M. E., Mathre, D. E., and Sands, D. C. (1987). Identification and characterization of Rhizosphere-competent bacteria of wheat. Appl. Environ. Microbiol. 53, 2793–2799.
Karimi, B., Maron, P. A., Chemidlin-Prevost Boure, N., Bernard, N., Gilbert, D., and Ranjard, L. (2017). Microbial diversity and ecological networks as indicators of environmental quality. Environ. Chem. Lett. 15, 265–281. doi: 10.1007/s10311-017-0614-6
Kasim, W. A., Osman, M. E., Omar, M. N., Abd El-Daim, I. A., Bejai, S., and Meijer, J. (2013). Control of drought stress in wheat using plant-growth-promoting bacteria. J. Plant Growth Regul. 32, 122–130. doi: 10.1007/s00344-012-9283-7
Katoh, K., and Standley, D. M. (2013). MAFFT multiple sequence alignment software version 7: improvements in performance and usability. Mol. Biol. Evol. 30, 772–780. doi: 10.1093/molbev/mst010
Kechid, M., Desbrosses, G., Rokhsi, W., Varoquaux, F., Djekoun, A., and Touraine, B. (2013). The NRT2. 5 and NRT2. 6 genes are involved in growth promotion of Arabidopsis by the plant growth-promoting rhizobacterium (PGPR) strain Phyllobacterium brassicacearum STM196. New Phytol. 198, 514–524. doi: 10.1111/nph.12158
Kempf, B., and Bremer, E. (1998). Uptake and synthesis of compatible solutes as microbial stress responses to high-osmolality environments. Arch. Microbiol. 170, 319–330. doi: 10.1007/s002030050649
Kennedy, A. C. (1999). Bacterial diversity in agroecosystems. Agric. Ecosyst. Environ. 74, 65–76. doi: 10.1016/b978-0-444-50019-9.50007-8
Kladivko, E. J. (2001). Tillage systems and soil ecology. Soil Tillage Res. 61, 61–76. doi: 10.1016/s0167-1987(01)00179-9
Kloepper, J. W., Leong, J., Teintze, M., and Schroth, M. N. (1980). Pseudomonas siderophores - a mechanism explaining disease-suppressive soils. Curr. Microbiol. 4, 317–320. doi: 10.1007/bf02602840
Kumar, A., Maurya, B. R., and Raghuwanshi, R. (2014). Isolation and characterization of PGPR and their effect on growth, yield and nutrient content in wheat (Triticum aestivum L.). Biocataly. Agric. Biotechnol. 3, 121–128. doi: 10.1016/j.bcab.2014.08.003
Kumar, V., and Narula, N. (1999). Solubilization of inorganic phosphates and growth emergence of wheat as affected by Azotobacter chroococcum mutants. Biol. Ferti. Soils 28, 301–305. doi: 10.1007/s003740050497
Lambert, B., Joos, H., Dierickx, S., Vantomme, R., Swings, J., Kersters, K., et al. (1990). Identification and plant interaction of a Phyllobacterium sp., a predominant rhizobacterium of young sugar beet plants. Appl. Environ. Microbiol. 56, 1093–1102.
Lane, D. J. (1991). “16S/23S rRNA sequencing,” in Nucleic Acid Techniques in Bacterial Systematics, eds E. Stackebrandt and M. Goodfellow (New York, NY: John Wiley and Sons), 115–175.
Lauber, C. L., Strickland, M. S., Bradford, M. A., and Fierer, N. (2008). The influence of soil properties on the structure of bacterial and fungal communities across land-use types. Soil Biol. Biochem. 40, 2407–2415. doi: 10.1016/j.soilbio.2008.05.021
Lennon, J. T., Aanderud, Z. T., Lehmkuhl, B. K., and Schoolmaster, D. R. (2012). Mapping the niche space of soil microorganisms using taxonomy and traits. Ecology 93, 1867–1879. doi: 10.1890/11-1745.1
Lucas García, J. A., Barbas, C., Probanza, A., Barrientos, M. L., and Gutierrez Mañero, F. J. (2001). Low molecular weight organic acids and fatty acids in root exudates of two Lupinus cultivars at flowering and fruiting stages. Phytochem. Anal. 12, 305–311. doi: 10.1002/pca.596
Ma, Y., Rajkumar, M., Luo, Y., and Freitas, H. (2013). Phytoextraction of heavy metal polluted soils using sedum plumbizincicola inoculated with metal mobilizing Phyllobacterium myrsinacearum RC6b. Chemosphere 93, 1386–1392. doi: 10.1016/j.chemosphere.2013.06.077
Malhi, S. S., Johnston, A. M., Schoenau, J. J., Wang, Z. L., and Vera, C. L. (2006). Seasonal biomass accumulation and nutrient uptake of wheat, barley and oat on a Black Chernozem Soil in Saskatchewan. Can. J. Plant Sci. 86, 1005–1014. doi: 10.4141/p05-116
Mantelin, S., Saux, M. F., Zakhia, F., Bena, G., Bonneau, S., Jeder, H., et al. (2006). Emended description of the genus Phyllobacterium and description of four novel species associated with plant roots: Phyllobacterium bourgognense sp. nov., Phyllobacterium ifriqiyense sp. nov., Phyllobacterium leguminum sp. nov. and Phyllobacterium brassicacearum sp. nov. Int. J. Syst. Evol. Microbiol. 56, 827–839. doi: 10.1099/ijs.0.63911-0
Manzoni, S., Schimel, J. P., and Porporato, A. (2012). Responses of soil microbial communities to water stress: results from a meta-analysis. Ecology 93, 930–938. doi: 10.1890/11-0026.1
Marasco, R., Rolli, E., and Ettoumi, B. (2012). A drought resistance-promoting microbiome is selected by root system under desert farming. PLoS One 7:e48479. doi: 10.1371/journal.pone.0048479
Miller, S. H., Browne, P., Prigent-Combaret, C., Combes-Meynet, E., Morrissey, J. P., and O’Gara, F. (2010). Biochemical and genomic comparison of inorganic phosphate solubilization in Pseudomonas species. Environ. Microbiol. Rep. 2, 403–411. doi: 10.1111/j.1758-2229.2009.00105.x
Mogollón, J. M., Beusen, A. H. W., Van Grinsven, H. J. M., Westhoek, H., and Bouwman, A. F. (2018). Future agricultural phosphorus demand according to the shared socioeconomic pathways. Glob. Environ. Change 50, 149–163. doi: 10.1016/j.gloenvcha.2018.03.007
Mohammadi, K. (2012). Phosphorus solubilizing bacteria: occurrence, mechanisms and their role in crop production. Resour. Environ. 2, 80–85.
Molla, M. A. Z., Chowdhury, A. A., Islam, A., and Hoque, S. (1984). Microbial mineralization of organic phosphate in soil. Plant Soil 78, 393–399. doi: 10.1007/bf02450372
Murphy, J., and Riley, J. P. (1962). A modified single solution method for the determination of phosphate in natural waters. Analy. Chim. Acta 27, 31–36. doi: 10.1016/s0003-2670(00)88444-5
Muyzer, G., de Waal, E. C., and Uitterlinden, A. G. (1993). Profiling of complex microbial populations by denaturing gradient gel electrophoresis analysis of polymerase chain reaction-amplified genes coding for 16S rRNA. Appl. Environ. Microbiol. 59, 695–700.
Naiman, A. D., Latrónico, A., and García de Salamone, I. E. (2009). Inoculation of wheat with Azospirillum brasilense and Pseudomonas fluorescens: impact on the production and culturable rhizosphere microflora. Eur. J. Soil Bio. 45, 44–51. doi: 10.1016/j.ejsobi.2008.11.001
Naveed, M., Hussain, M. B., Zahir, Z. A., Mitter, B., and Sessitsch, A. (2014). Drought stress amelioration in wheat through inoculation with Burkholderia phytofirmans strain PsJN. Plant Growth Regul. 73, 121–131. doi: 10.1007/s10725-013-9874-8
Nguyen, C., Yan, W., Le Tacon, F., and Lapeyrie, F. (1992). Genetic variability of phosphate solubilizing activity by monocaryotic and dicaryotic mycelia of the ectomycorrhizal fungus Laccaria bicolor (Maire) PD Orton. Plant Soil 143, 193–199. doi: 10.1007/bf00007873
Oehl, F., Oberson, A., Tagmann, H. U., Besson, J. M., Dubois, D., Mäder, P., et al. (2002). Phosphorus budget and phosphorus availability in soils under organic and conventional farming. Nutr. Cycl. Agroecosyst. 62, 25–35.
Paterson, E., Gebbing, T., Abel, C., Sim, A., and Telfer, G. (2007). Rhizodeposition shapes rhizosphere microbial community structure in organic soil. New Phytol. 173, 600–610. doi: 10.1111/j.1469-8137.2006.01931.x
Paulsen, I. T., Press, C. M., and Ravel, J. (2005). Complete genome sequence of the plant commensal Pseudomonas fluorescens Pf-5. Nat. Biotechnol. 23:873.
Pérez, E., Sulbarán, M., Ball, M. M., and Yarzábal, L. A. (2007). Isolation and characterization of mineral phosphate-solubilizing bacteria naturally colonizing a limonitic crust in the south-eastern Venezuelan region. Soil Biol. Biochem. 39, 2905–2914. doi: 10.1016/j.soilbio.2007.06.017
Petersen, U., and Weigel, H.-J. (2015). Klimaresilienz Durch Agrobiodiversität?. Thünen-Report 25. Waldsieversdorf: Thünen.
Pikovskaya, R. I. (1948). Mobilization of phosphorus in soil connection with the vital activity of some microbial species. Microbiologiya 17, 362–370.
R Core Team (2017). R: A Language and Environment for Statistical Computing. Vienna: R Foundation for Statistical Computing.
Rajkumar, M., Ae, N., Prasad, M. N., and Freitas, H. (2010). Potential of siderophore-producing bacteria for improving heavy metal phytoextraction. Trends Biotechnol. 28, 142–149. doi: 10.1016/j.tibtech.2009.12.002
Rana, A., Saharan, B., Joshi, M., Prasanna, R., Kumar, K., and Nain, L. (2011). Identification of multi-trait PGPR isolates and evaluating their potential as inoculants for wheat. Ann. Microbiol. 61, 893–900. doi: 10.1007/s13213-011-0211-z
Richardson, A. E., Barea, J.-M., McNeill, A. M., and Prigent-Combaret, C. (2009). Acquisition of phosphorus and nitrogen in the rhizosphere and plant growth promotion by microorganisms. Plant Soil 321, 305–339. doi: 10.1007/s11104-009-9895-2
Rodríguez, H., and Fraga, R. (1999). Phosphate solubilizing bacteria and their role in plant growth promotion. Biotechnol. Adv. 17, 319–339. doi: 10.1016/s0734-9750(99)00014-2
Rojas, A., Holguin, G., Glick, B. R., and Bashan, Y. (2001). Synergism between Phyllobacterium sp. (N2-fixer) and Bacillus licheniformis (P-solubilizer), both from a semiarid mangrove rhizosphere. FEMS Microbiol. Ecol. 35, 181–187. doi: 10.1016/s0168-6496(01)00091-5
Sagot, B., Gaysinski, M., Mehiri, M., Guigonis, J.-M., Le Rudulier, D., and Alloing, G. (2010). Osmotically induced synthesis of the dipeptide N-acetylglutaminylglutamine amide is mediated by a new pathway conserved among bacteria. Proc. Natl. Acad. Sci. U.S.A. 107, 12652–12657. doi: 10.1073/pnas.1003063107
Sala, O. E., Chapin, F. S., Armesto, J. J., Berlow, E., Bloomfield, J., Dirzo, R., et al. (2000). Global biodiversity scenarios for the year 2100. Science 287, 1770–1774. doi: 10.1126/science.287.5459.1770
Sandhya, V., Grover, M., Reddy, G., and Venkateswarlu, B. (2009). Alleviation of drought stress effects in sunflower seedlings by the exopolysaccharides producing Pseudomonas putida strain GAP-P45. Biol. Fertil. Soils 46, 17–26. doi: 10.1007/s00374-009-0401-z
Schädler, M., Buscot, F., Klotz, S., Reitz, T., Durka, W., Bumberger, J., et al. (2019). Investigating the consequences of climate change under different land-use regimes - a novel experimental infrastructure. Ecosphere 10:e02635. doi: 10.1002/ecs2.2635
Scher, F. M., Kloepper, J. W., Singleton, C., Zaleska, I., and Laliberte, M. (1988). Colonization of sybean roots by Pseudomonas and Serratia species: relationship to bacterial motility, chemotaxis, and generation time. Phytopathology 78, 1055–1059.
Schmeling, D. (2017). Auf 54% der Getreideanbaufläche steht 2017 Winterweizen. Available at: https://www.destatis.de/DE/PresseService/Presse/Pressemitteilungen/2017/05/PD17_163_412pdf.pdf?__blob=publicationFile (accessed March 18, 2019).
Shade, A., Peter, H., Allison, S. D., Baho, D. L., Berga, M., Bürgmann, H., et al. (2012). Fundamentals of microbial community resistance and resilience. Front. Microbiol. 3:417. doi: 10.3389/fmicb.2012.00417
Sharma, S. B., Sayyed, R. Z., Trivedi, M. H., and Gobi, T. A. (2013). Phosphate solubilizing microbes: sustainable approach for managing phosphorus deficiency in agricultural soils. Springerplus 2:587. doi: 10.1186/2193-1801-2-587
Sheik, C. S., Beasley, W. H., Elshahed, M. S., Zhou, X., Luo, Y., and Krumholz, L. R. (2011). Effect of warming and drought on grassland microbial communities. ISME J. 5, 1692–1700. doi: 10.1038/ismej.2011.32
Singh, Y., and Lal, N. (2016). Isolation and Characterization of PGPR from Wheat (Triticum aestivum) Rhizosphere and Their Plant Growth Promoting Traits in Vitro. Front. Microbiol. 6:198.
Taketani, R. G., Lançoni, M. D., Kavamura, V. N., Durrer, A., Andreote, F. D., and Melo, I. S. (2017). Dry season constrains bacterial phylogenetic diversity in a semi-arid rhizosphere system. Microb. Ecol. 73, 153–161. doi: 10.1007/s00248-016-0835-4
Tardy, V., Mathieu, O., Lévêque, J., Terrat, S., Chabbi, A., Lemanceau, P., et al. (2014). Stability of soil microbial structure and activity depends on microbial diversity. Environ. Microbiol. Rep. 6, 173–183. doi: 10.1111/1758-2229.12126
Tiemann, L. K., Grandy, A. S., Atkinson, E. E., Marin-Spiotta, E., and McDaniel, M. D. (2015). Crop rotational diversity enhances belowground communities and functions in an agroecosystem. Ecology Lett. 18, 761–771. doi: 10.1111/ele.12453
Timmusk, S., Abd El-Daim, I. A., and Copolovici, L. (2014). Drought-tolerance of wheat improved by rhizosphere bacteria from harsh environments: enhanced biomass production and reduced emissions of stress volatiles. PLoS One 9:e96086. doi: 10.1371/journal.pone.0096086
Trnka, M., Rötter, R. P., Ruiz-Ramos, M., Kersebaum, K. C., Olesen, J. E., Žalud, Z., et al. (2014). Adverse weather conditions for European wheat production will become more frequent with climate change. Nat. Clim. Change 4:637. doi: 10.1038/nclimate2242
Verbruggen, E., Röling, W. F., Gamper, H. A., Kowalchuk, G. A., Verhoef, H. A., and van der Heijden, M. G. (2010). Positive effects of organic farming on below-ground mutualists: large-scale comparison of mycorrhizal fungal communities in agricultural soils. New Phytol. 186, 968–979. doi: 10.1111/j.1469-8137.2010.03230.x
Verma, P., Yadav, A. N., Khannam, K. S., Kumar, S., Saxena, A. K., and Suman, A. (2016). Molecular diversity and multifarious plant growth promoting attributes of Bacilli associated with wheat (Triticum aestivum L.) rhizosphere from six diverse agro-ecological zones of India. J. Basic Microbiol. 56, 44–58. doi: 10.1002/jobm.201500459
Verslues, P. E., Agarwal, M., Katiyar-Agarwal, S., Zhu, J., and Zhu, J.-K. (2006). Methods and concepts in quantifying resistance to drought, salt and freezing, abiotic stresses that affect plant water status. Plant J. 45, 523–539. doi: 10.1111/j.1365-313x.2005.02593.x
Viaene, T., Langendries, S., Beirinckx, S., Maes, M., and Goormachtig, S. (2016). Streptomyces as a plant’s best friend? FEMS Microbiol. Ecol. 92:fiw119. doi: 10.1093/femsec/fiw119
Walsh, U. F., Morrissey, J. P., and O’Gara, F. (2001). Pseudomonas for biocontrol of phytopathogens: from functional genomics to commercial exploitation. Curr. Opin. Biotechnol. 12, 289–295. doi: 10.1016/s0958-1669(00)00212-3
Widmer, F., Rasche, F., Hartmann, M., and Fliessbach, A. (2006). Community structures and substrate utilization of bacteria in soils from organic and conventional farming systems of the DOK long-term field experiment. Appl. Soil Ecol. 33, 294–307. doi: 10.1016/j.apsoil.2005.09.007
Williams, M. A., and Rice, C. W. (2007). Seven years of enhanced water availability influences the physiological, structural, and functional attributes of a soil microbial community. Appl. Soil Ecol. 35, 535–545. doi: 10.1016/j.apsoil.2006.09.014
Wolsing, M., and Priemé, A. (2004). Observation of high seasonal variation in community structure of denitrifying bacteria in arable soil receiving artificial fertilizer and cattle manure by determining T-RFLP of nir gene fragments. FEMS Microbiol. Ecol. 48, 261–271. doi: 10.1016/j.femsec.2004.02.002
Zaprasis, A., Bleisteiner, M., Kerres, A., Hoffmann, T., and Bremer, E. (2015). Uptake of amino acids and their metabolic conversion into the compatible solute proline confers osmoprotection to Bacillus subtilis. Appl. Environ. Microbiol. 81, 250–259. doi: 10.1128/AEM.02797-14
Zhang, H., Gao, S., Lercher, M. J., Hu, S., and Chen, W. H. (2012). EvolView, an online tool for visualizing, annotating and managing phylogenetic trees. Nucleic Acids Res. 40, W569–W572. doi: 10.1093/nar/gks576
Keywords: climate change, agriculture, wheat, PGPR, phosphate solubilization, drought tolerance
Citation: Breitkreuz C, Buscot F, Tarkka M and Reitz T (2020) Shifts Between and Among Populations of Wheat Rhizosphere Pseudomonas, Streptomyces and Phyllobacterium Suggest Consistent Phosphate Mobilization at Different Wheat Growth Stages Under Abiotic Stress. Front. Microbiol. 10:3109. doi: 10.3389/fmicb.2019.03109
Received: 18 March 2019; Accepted: 23 December 2019;
Published: 22 January 2020.
Edited by:
Yusuke Saijo, Nara Institute of Science and Technology (NAIST), JapanReviewed by:
Usman Irshad, COMSATS University Islamabad, Abbottabad Campus, PakistanKei Hiruma, Nara Institute of Science and Technology (NAIST), Japan
Copyright © 2020 Breitkreuz, Buscot, Tarkka and Reitz. This is an open-access article distributed under the terms of the Creative Commons Attribution License (CC BY). The use, distribution or reproduction in other forums is permitted, provided the original author(s) and the copyright owner(s) are credited and that the original publication in this journal is cited, in accordance with accepted academic practice. No use, distribution or reproduction is permitted which does not comply with these terms.
*Correspondence: Claudia Breitkreuz, Y2xhdWRpYS5icmVpdGtyZXV6QHVmei5kZQ==