- 1Dipartimento di Malattie Infettive, Istituto Superiore di Sanità, Rome, Italy
- 2Vector Biology, Max Planck Institute for Infection Biology, Berlin, Germany
- 3Department of Theory and Bio-Systems, Max Planck Institute of Colloids and Interfaces, Potsdam, Germany
The egress and fertilization of Plasmodium gametes and development of a motile ookinete are the first crucial steps that mediate the successful transmission of the malaria parasites from humans to the Anopheles vector. However, limited information exists about the cell biology and regulation of this process. Technical impediments in the establishment of in vitro conditions for ookinete maturation in Plasmodium falciparum and other human malaria parasites further constrain a detailed characterization of ookinete maturation. Here, using fluorescence microscopy and immunolabeling, we compared P. falciparum ookinete maturation in Anopheles coluzzii mosquitoes in vivo and in cell culture in vitro. Our results identified two critical steps in ookinete maturation that are regulated by distinct mosquito factors, thereby highlighting the role of the mosquito environment in the transmission efficiency of malaria parasites.
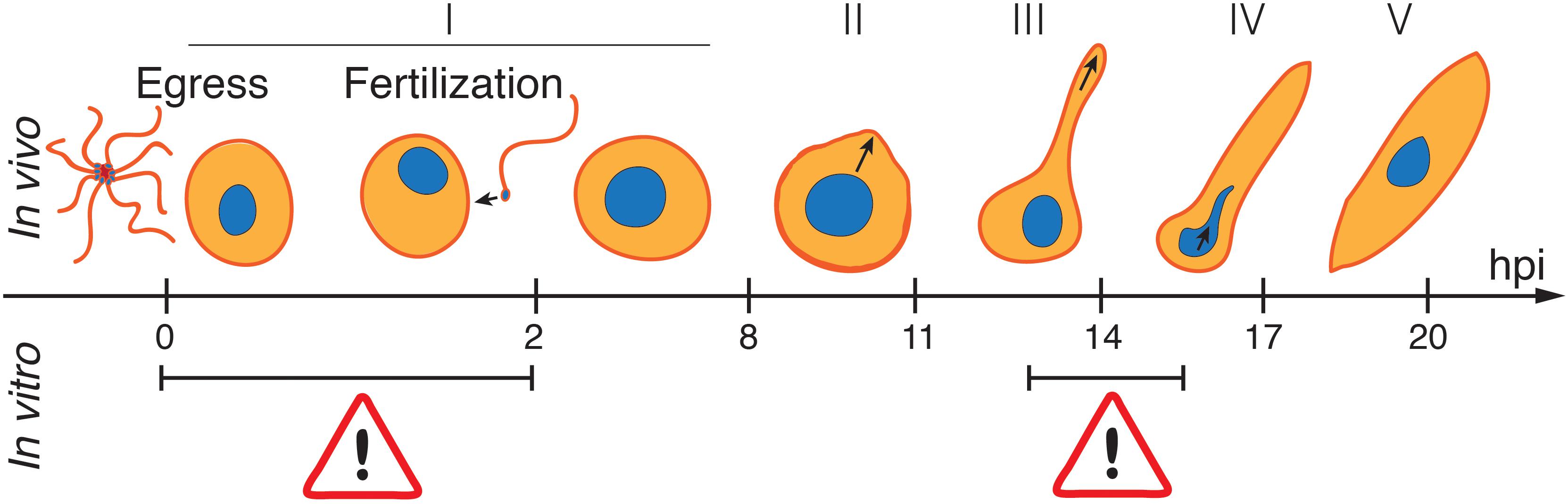
Graphical Abstract.Ookinete development within the mosquito blood bolus is a critical step in malaria transmission. Using time-series approaches, we identified two limiting steps of Plasmodium falciparum ookinete development in vitro: (i) inhibition of transition from round forms (stage I) to knobs (stage II), likely caused by an inefficient fertilization, and (ii) arrest of transition between stages III and IV, associated with morphological abnormalities during protuberance elongation and enlargement. The abnormal protuberance at stage IV prevents further nuclear migration from the round body to the appendage.
Introduction
Malaria is a mosquito-borne disease, mainly caused by the protozoan parasite Plasmodium falciparum, which kills 429,000 people annually (World Health Organization [WHO], 2018). A key component of the global initiative to eliminate malaria is the transmission block of malaria parasites from humans to the mosquito vector. Plasmodium transmission is initiated within minutes after blood ingestion by the Anopheles mosquito. Within the mosquito midgut, the gametocytes transform into mature extracellular female and male gametes. The product of fertilization, the zygote, takes one day to transform into a motile ookinete, which then traverses the midgut epithelium to establish oocyst infection on the basal side. About 10–12 days later, each oocyst releases thousands of sporozoites that migrate to and invade the salivary glands, ready to be injected into a human at the next mosquito bite. During the first 24 h after blood meal, the parasites undergo a series of radical tightly regulated morphological changes: gamete roundup, egress and exflagellation, formation of a zygote, emergence of an elongated protuberance and transformation of a round zygote into a crescent-shaped motile ookinete. The early stages of gametocyte-to-gamete transformation are triggered by the mosquito-derived metabolite xanthurenic acid, which together with the temperature drop signals for gamete egress and exflagellation (Billker et al., 1998). Although fertilization and post-fertilization steps are critical for Plasmodium establishment in the mosquito, very little is known about molecular mechanisms and signals that orchestrate parasite sexual reproduction. Field studies suggested that pre-fertilization events do not reliably predict the outcome of mosquito infection, as the numbers of the blood-circulating gametocytes do not always correlate with the donor infectiousness to the insect vector (Bousema and Drakeley, 2011). Are post-fertilization events (the transition from zygote to ookinete) a more accurate proxy to forecast the efficiency of Plasmodium transmission?
To better characterize the post-fertilization events, several studies attempted to establish P. falciparum ookinete cultures in vitro (Carter et al., 1987; Warburg and Schneider, 1993; Bounkewa et al., 2010; Ghosh et al., 2010; Delves et al., 2017). While such cultures were successful for the rodent malaria parasite Plasmodium berghei (Janse et al., 1985; Sinden et al., 1985), the efficiency of P. falciparum conversion from round-shaped parasites to mature ookinetes is surprisingly low (0.45 to 16%) as compared to the estimated 50% efficiency in vivo (Carter et al., 1987; Bounkewa et al., 2010; Delves et al., 2017). The poor efficiency of P. falciparum gamete fertilization was identified as a major obstacle to efficient ookinete production in vitro (Ghosh et al., 2010; Delves et al., 2017). As the factors that promote fertilization in vivo remained obscure, a series of culture conditions (lipids, glucose, mosquito pupal extract, and red blood cell lysate additives, variations in hematocrit, pH, and gas; etc.) were tested to rescue the block in fertilization, albeit without success (Ghosh et al., 2010; Delves et al., 2017).
In spite of low efficiency, culturing in vitro provided a first classification of ookinete development based on morphology of Giemsa-stained cells (Ghosh et al., 2010). Besides the round-shaped zygote, five ookinete stages have been described: (I and II) retorts with a short protuberance attached to the round body; (III) retort with enlarged but not fully elongated protuberance; (IV) retorts with the thin protuberance at maximum elongation; (V) crescent or fluke-shaped mature ookinetes. The drawback of this classification was the unspecific basis of the Giemsa staining, which could not distinguish between residual non-activated gametocytes, developmentally blocked degenerated parasites, and mature ookinetes. Application of monoclonal antibodies that bound the zygote/ookinete surface antigen Pfs25 and/or the ookinete-specific intracellular enzyme chitinase helped the identification of mature ookinetes (Carter et al., 1987; Bounkewa et al., 2010; Ghosh et al., 2010; Delves et al., 2017). However, the antibodies were used only to count the mature ookinetes at a late time point or at the very beginning and very end of the process, thereby preventing a faithful reconstruction of the ookinete maturation stages.
Here, using live immunofluorescence microscopy, we report the temporal characterization of the zygote to ookinete development in the P. falciparum-infected Anopheles coluzzii mosquitoes and in cell culture in vitro. By comparing the time series of the zygote development in vivo and in vitro, we demonstrate a requirement of mosquito-derived factor(s) for gamete fertilization and identify a new critical stage of ookinete maturation.
Materials and Methods
Parasite Cultures
Plasmodium falciparum NF54 parasites were cultured in O+ human red blood cells (Haema, Berlin), at 37°C, in a 3% O2, 5% CO2, and 92% N2 atmosphere. Asexual cultures (0.5–5% of parasitemia) were maintained at 3–4% hematocrit in the complete medium composed of RPMI 1640 with L-glutamine and 25 mM HEPES, supplemented with 10% human A+ serum (Haema, Berlin), 10 mM hypoxanthine (c-c-Pro), and 20 μg/ml of gentamicin (Sigma). For gametocyte production, asynchronous asexual parasites were seeded at 1% parasitemia and 5% hematocrit, and complete medium without gentamicin was replaced daily for 15 days until mosquito infections. Stage V gametocytes were enumerated in a counting chamber (Neubauer) 14 days post seeding. On the same day, a sample from the culture was incubated for 15 min at room temperature in the presence of 20 μM of xanthurenic acid (Sigma), and exflagellating clusters were enumerated in a counting chamber (Neubauer). The exflagellation rate was calculated as follows:
P. falciparum NF54 clone originated from Prof. Sauerwein’s laboratory, Radboud University Medical Center, Nijmegen, The Netherlands, and was authenticated for Pfs47 by PCR on genomic DNA. P. falciparum asexual cultures were monthly tested for Mycoplasma contamination.
Mosquito Rearing and Parasite Infections
Anopheles coluzzii (Ngousso S1 strain) was maintained at 29°C 70–80% humidity 12/12-h day/night cycle. For P. falciparum infections, mosquitoes were fed for 15 min on a membrane feeder with stage V gametocytes diluted in fresh red blood cells and human serum to 3.7 × 106 stage V gametocytes/ml and 50% hematocrit. Infected mosquitoes were kept in a secured S3 laboratory at 26°C for up to 11 days according to national regulations (Landesamt für Gesundheit und Soziales, project number 297/13). Unfed mosquitoes were removed shortly after infection.
Mosquito Dissections
For ookinete analyses, midguts of decapitated mosquitoes were dissected in 1 × phosphate-buffered saline (PBS) at the indicated time points (20 midguts per time point for each experimental condition). For oocyst counts, mosquitoes were killed in ethanol 70% at 11 dpi, midguts were dissected and incubated in 1% mercurochrome (Sigma) in water for 5–10 min. The oocyst numbers were enumerated under a light microscope Leica DM2000 LED.
Ookinete Cultures in vitro and ex vivo
For in vitro experiments, ookinete cultures were set up by diluting gametocyte cultures 15 days post seeding in fresh complete medium to 5 × 106 stage V gametocytes/ml, supplemented with 20 μM xanthurenic acid and incubated at 26°C in a siliconized tube until analyses.
For ex vivo experiments, midguts of infected mosquitoes were homogenized in complete medium containing 40 μg/ml of gentamicin and 5 μg/ml of Fungizone (Gibco) to decrease the microbial charge, incubated for 2 min at room temperature, washed (300 g, 4 min), resuspended in 500 μl of complete medium containing 40 μg/ml of gentamicin (Alpha Laboratories), and incubated at 26°C in a siliconized tube for an additional 21 h. Control in vivo samples were collected from mosquito bolus 23 h post infection (hpi), while control in vitro ookinete cultures were set up with the same gametocyte suspension prepared for mosquito infections, diluted 1:25 in complete medium without gentamicin and incubated at 26°C for 23 h. As mock control, one aliquot from in vitro cultures was subjected 2 h post seeding to the same gentamicin/Fungizone treatment used ex vivo and then cultured for an additional 21 h (Supplementary Figure 4).
Ookinete Staining and Quantification
Hybridoma cells expressing anti-Pfs25 IgG (clone 4B7) were obtained from BEI Resources. IgG fraction was purified using Protein G Sepharose columns (GE Healthcare) and labeled to Alexa Fluor 568 (Life Technologies) in the Central Laboratory Facility (Deutsches Rheuma-Forschungszentrum, Berlin). Ookinetes were stained with anti-Pfs25-Alexa Fluor 568 antibody (7.5 μg/ml at 2 hpi and 1.5 μg/ml at 23 hpi) and Hoechst 1:500 (Molecular Probes) for 30 min at 4°C in PBS. Samples were washed (300 g, 4 min) and resuspended in PBS. The numbers of Pfs25-positive parasites were enumerated in a counting chamber (Neubauer) using a Leica DMRB fluorescence microscope and classified into stages using an Axio Observer Z1 fluorescence microscope equipped with an Apotome module (Zeiss) (Figure 1).
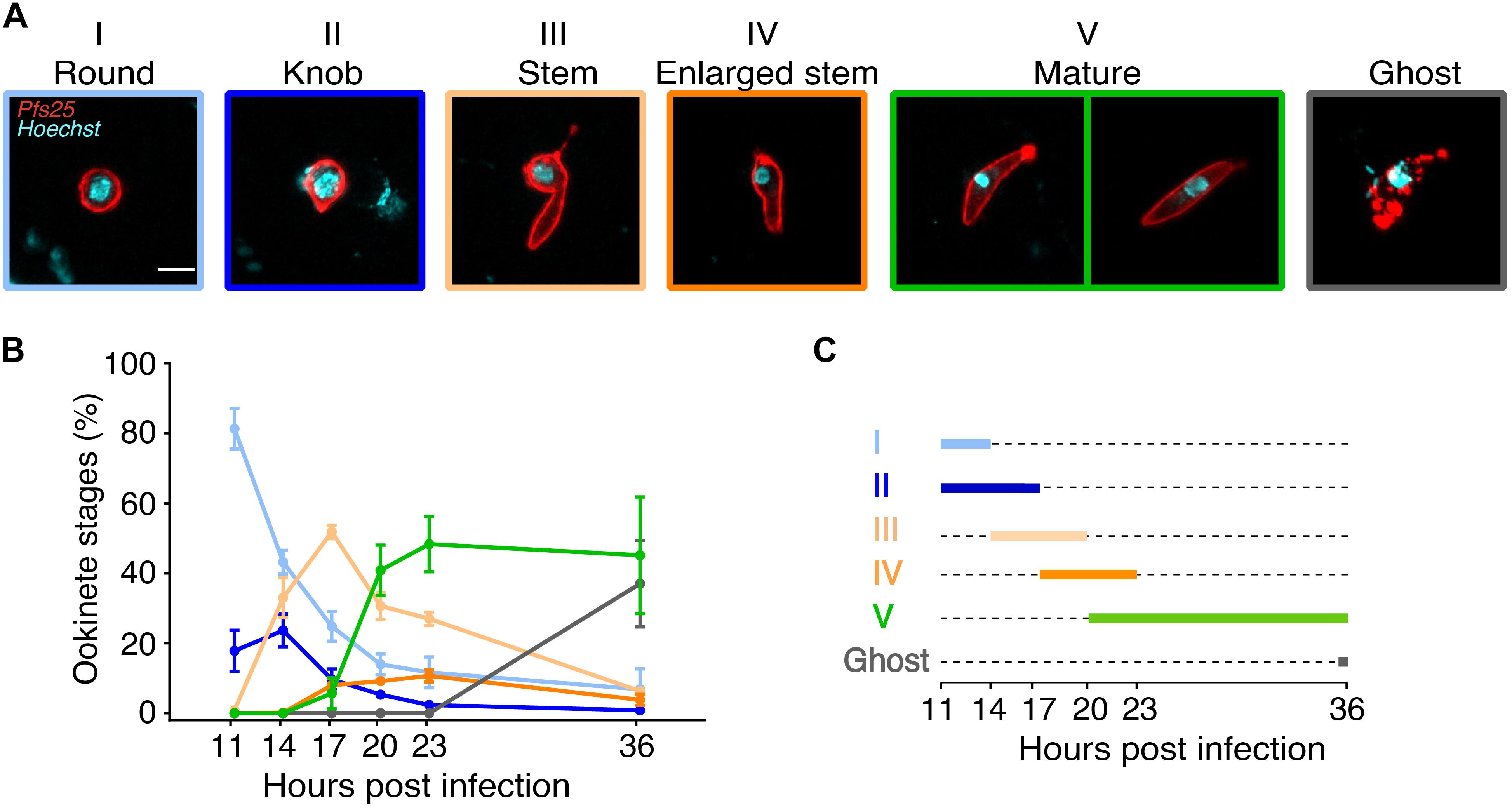
Figure 1. Kinetics of Plasmodium falciparum ookinete maturation in the mosquito bolus. Anopheles coluzzii mosquitoes were infected with P. falciparum gametocytes. Live parasites were isolated from a pool of 20 mosquito boluses and stained with anti-Pfs25 antibody at 11, 14, 17, 20, 23, and 36 h post infection (hpi). (A) Representative pictures of Pfs25-positive and Hoechst-stained ookinete stages as referred throughout this study (scale bar: 5 μm). Stage I: Round, indistinguishable from egressed female gamete. Stage II: Knob, small protuberance not exceeding in length the radius of the main spherical body. Stage III: Stem, elongated protuberance longer than the radius of the main spherical body. Stage IV: Enlarged stem, the elongated protuberance–still containing the nucleus–increasing in width. Stage V: Mature, terminally differentiated elongated form with or without residual spherical body; the nucleus has migrated from the residual spherical body to the stem. Ghost: elongated degenerated forms with non-continuous surface Pfs25 signal. (B) In vivo ookinete forms were classified as in (A). Proportion of ookinete stages out of total Pfs25-positive parasites is indicated for each time point. Every point represents the mean ± SEM of three independent experiments (N = 3, n = 200 parasites counted per time point). (C) Schematic representation of the duration of ookinete stages in the mosquito vector described in (A).
Ookinete conversion was defined as follows:
The proportion of mature ookinete was defined as follows:
Representative pictures were acquired with the Apotome module, and images were deconvoluted and corrected for phase contrast and local bleaching using ZEN 2012 software (Zeiss).
Statistical Analysis
No samples were excluded from the analyses. Mosquitoes from the same batches were randomly allocated to the experimental groups (age range: 1–2 days). The experimenters were not blinded to the group allocation during the experiment and/or when assessing the outcome. Statistical analysis for Figure 2 was performed with GraphPad Prism 8, and p-values below 0.05 were considered significant (∗p < 0.05) as indicated in the figures. The specific tests used are indicated in the figure legend.
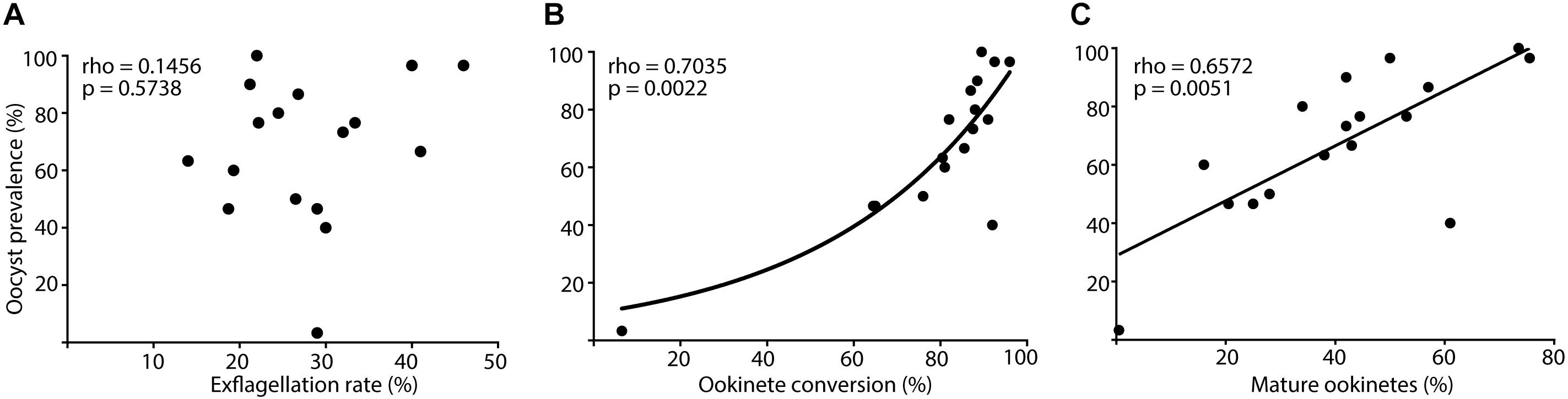
Figure 2. Correlation between male gamete activation and ookinete development rates with oocyst prevalence. Anopheles coluzzii mosquitoes were fed with Plasmodium falciparum gametocytes. Live parasites were isolated from the mosquito bolus and stained with anti-Pfs25 antibody at 24 h post infection (hpi), while oocysts were stained with mercurochrome and counted at 11 days post infection (dpi). Correlation between oocyst prevalence and (A) exflagellation rate (exflagellation clusters ml–1/gametocyte stage V ml–1) of gametocyte cultures 24 h before infection, (B) percentage of ookinete conversion (stages II to V ookinetes/total Pfs25-positive parasites), and (C) percentage of mature ookinetes (mature stage V ookinetes/total Pfs25-positive parasites). Every dot represents one independent experiment (N = 17), Pfs25-positive parasites (n = 200) were counted in pooled samples of 20 blood boluses, and oocyst prevalence was analyzed in dissected midguts (n = 30) per experiment. Rho- and p-values (Spearman correlation) are indicated on the top left corner of every graph, and lines show data trends for significant correlations (B), exponential growth curve; (C), linear regression.
Statistical analyses for Figures 3, 4 were performed by comparing the fraction of each single ookinete stage between two growth conditions at the same time point. The method (see below) takes both the biological variability and the sample sizes into account and allows estimation of the errors associated with the average percentages, which are then compared using a z-test. We defined f1, f2, and f3 as the fractions of the morphology for three independent experiments under one growth condition and s1, s2, and s3 as the corresponding sample sizes. For each of the three sample sizes, we take one fraction at random and generate one number of ookinetes from a binomial distribution with probability given by the chosen fraction and population size given by the sample size. As an illustrative example, suppose that we associate f3 to s1, f1 to s2, and f1 to s3 for a certain ookinete stage (e.g., knob) at a certain time (e.g., 17 hpi) and a certain condition (e.g., in vivo). We then generate three random numbers from binomial distributions with the parameters given by the fraction and sample size. These three numbers (n1, n2, and n3) are the number of ookinetes found for the three samples. This corresponds to a simulated replica of the same experiment under the condition that the fractions (f1, f2, and f3) and the sample sizes (s1, s2, and s3) are the same as found in the experiment. The corresponding fraction of ookinete will then be g1 = n1/s1, g2 = n2/s2, and g3 = n3/s3.
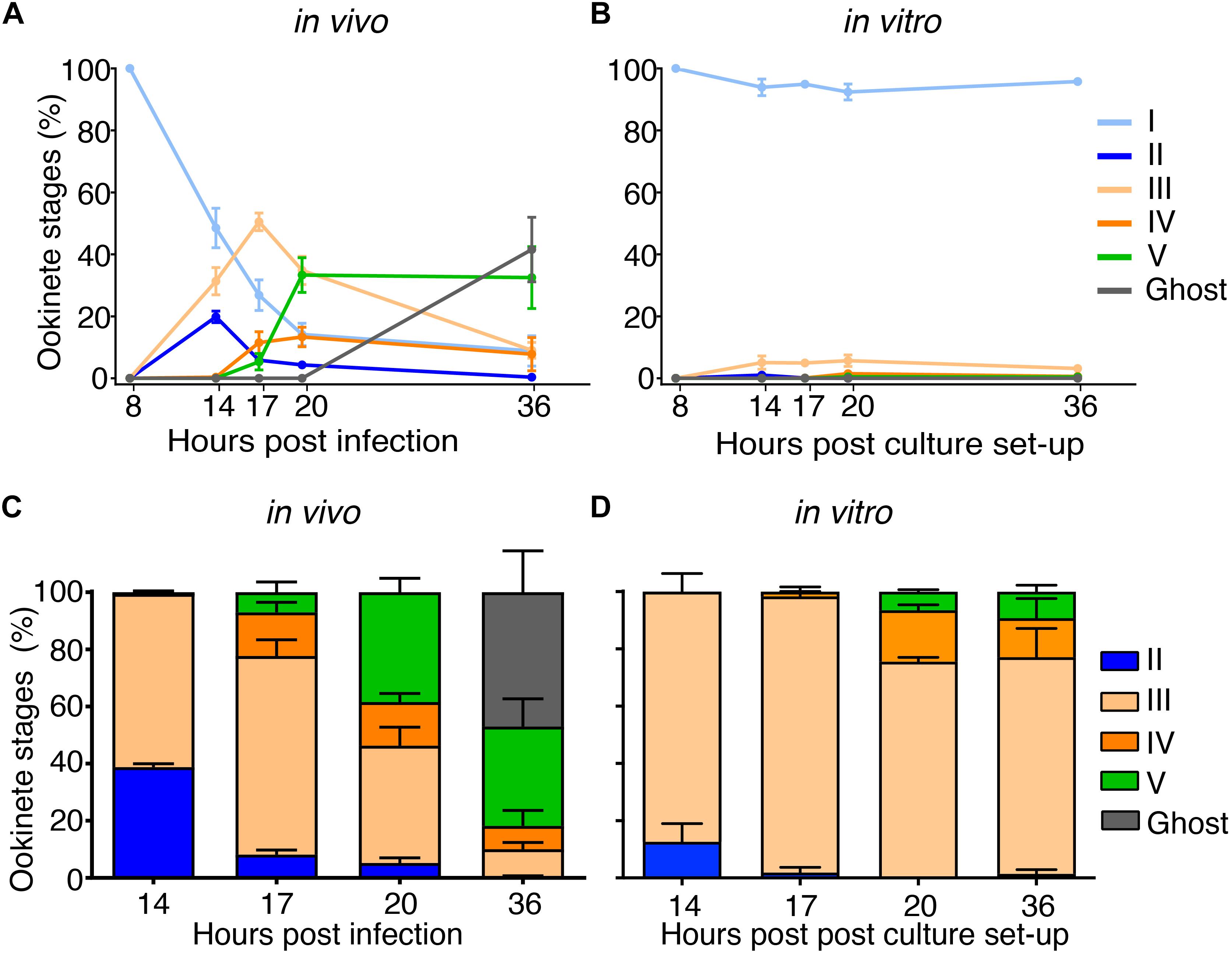
Figure 3. Parallel time course highlights two limiting steps in in vitro ookinete development. The same preparation of Plasmodium falciparum gametocytes was diluted and fed to mosquitoes or induced to undergo gametogenesis in a parallel time course of in vivo versus in vitro development. Parasites were isolated from a pool of 20 mosquito boluses or collected from the in vitro culture and stained with anti-Pfs25 antibody at 8, 14, 17, 20, and 36 h after feeding or culture setup. Ookinete forms were classified as in Figure 2A, and the proportions of ookinete stages on total Pfs25-positive parasites in vivo (A) or in vitro (B) are indicated for each time point. Every point represents the mean ± SEM of three independent experiments (A: n = 200, B: n = 500 parasites counted per time point). Relative proportions of ookinete stages out of total Pfs25-posititve parasites excluding round forms (stages II to V and ghosts) per each time point are shown in vivo (C) and in vitro (D). Bar plots represent the mean proportions ± SEM of three independent experiments (C), n = 309, 439, 515, and 449 and (D), n = 91, 76, 114, and 63 Pfs25-positive parasites were analyzed per time point. The z-test was used to compare the mean proportion of each ookinete stage and its variances in vivo versus in vitro.
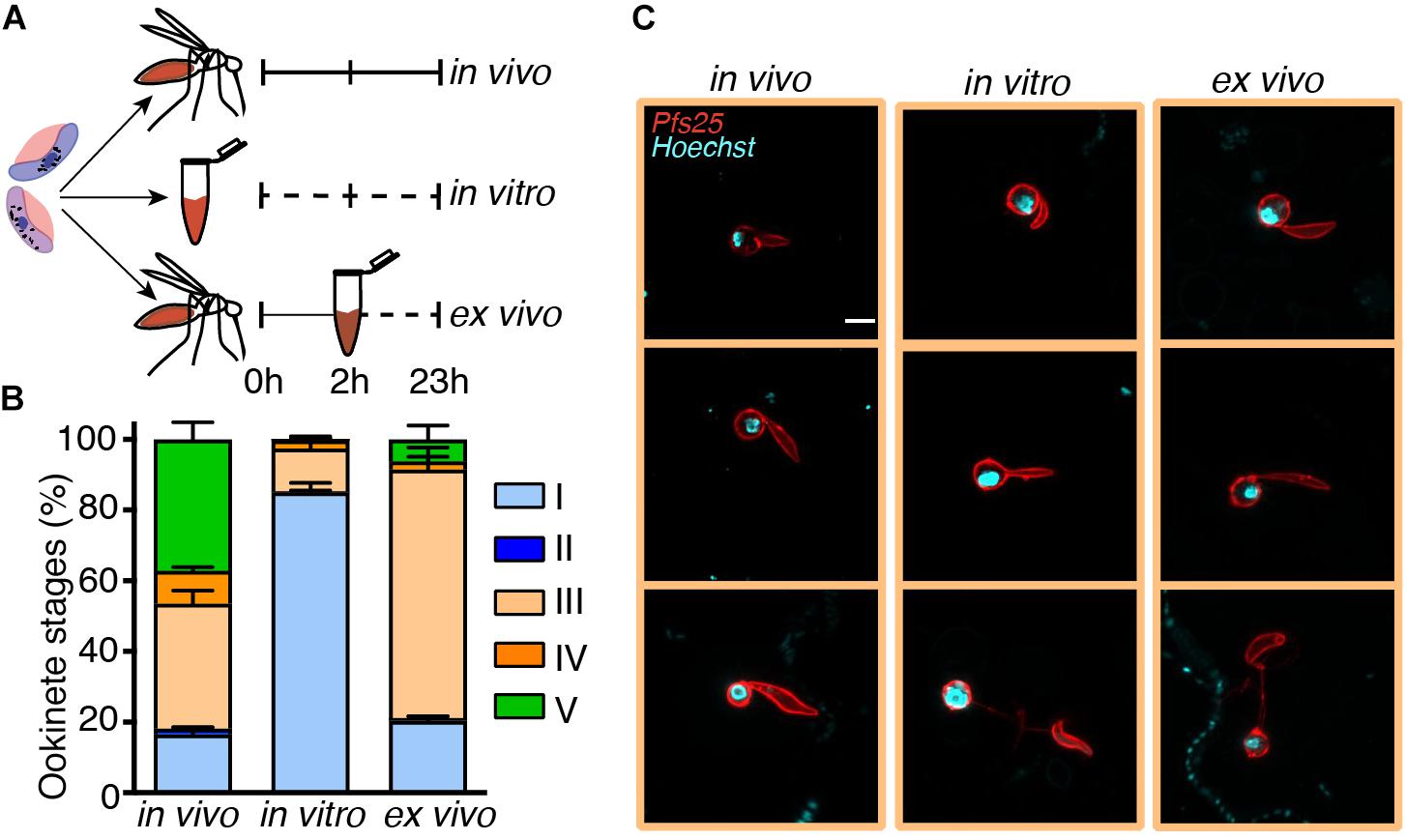
Figure 4. Ookinete development in vitro is critically impaired at the stage III-to-IV transition. The same Plasmodium falciparum gametocyte culture was used for mosquito infections or for in vitro ookinete development. To bypass the fertilization block, 20 mosquito blood boluses per time point were pooled and dissected 2 h post infection (hpi), and the residing parasites were transferred into in vitro cultures and incubated for 21 h (ex vivo conditions). Parasites developed in the mosquito bolus or in cultures in vitro were stained with anti-Pfs25 antibody at 2 and 23 hpi or post culture setup and were classified according to stages. (A) Schematic overview of the experiment. (B) Proportional composition of Pfs25-positive parasite stages developed in vivo, in vitro, and ex vivo 23 hpi or post culture as depicted in (A). Bar plots represent the mean proportions ± SEM (N = 3, n = 200 parasites counted per time point). (C) Representative images of anti-Pfs25-positive and Hoechst-stained stage III ookinetes 23 hpi or post culture developed in three conditions (scale bar-5 μm). The z-test was used to perform pairwise comparison of mean proportions of each ookinete stage and their variances in vivo, in vitro, and ex vivo.
We repeat this procedure M times, for M = 3e+6. After this procedure, the data contain M triplets of random numbers g1, g2, and g3 that represent simulated outcomes of the same experiments given the data obtained in the experimental work. The average of each of the triplets produces M average fractions E[g] = (g1 + g2 + g3)/3. The mean E[E(g)] of all these M average fractions and the variance Var[E(g)] of the M average fractions are the mean fraction and its variance associated with the ookinete stage at that given time point and under the given condition. We repeat this procedure for the each ookinete stage, time point, and condition and then compare the means of two conditions in pairs, taking the variances thus computed into account. The z-test applied to the two means and their variances finally delivers the p-value. Based on the above-mentioned procedure, the generated p-values are summarized in the Table 1 (refer to Figures 3C,D) and Table 2 (refer to Figure 4). The raw data used for this analysis are reported in Supplementary Tables 4–6.
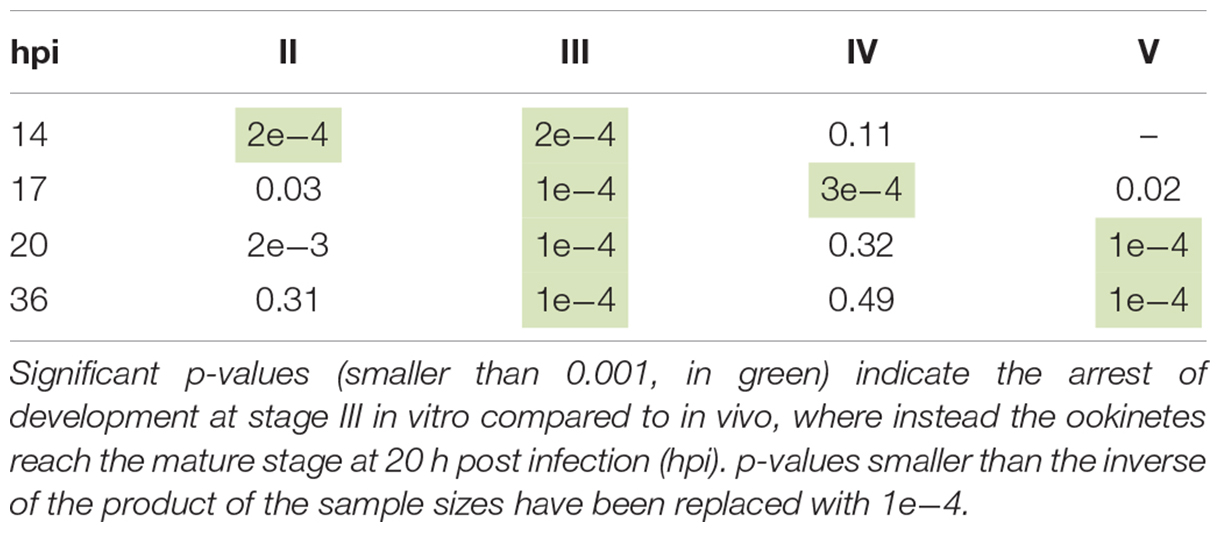
Table 1. p-values computed from the z-test for the comparison in vivo versus in vitro as shown in Figures 3C,D.
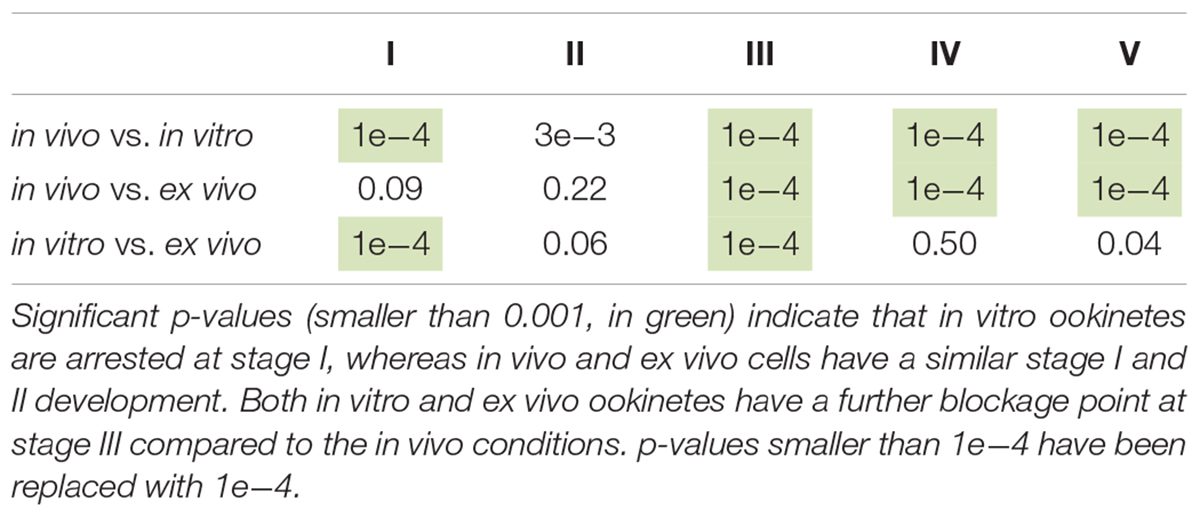
Table 2. p-values computed with a z-test for the comparison in vivo, in vitro, and ex vivo at 23 h post infection (hpi) as shown in Figure 4B.
Results
Stages of P. falciparum Ookinete Development in A. coluzzii
To characterize the major stages of zygote development, we performed a time course fluorescence live microscopy analysis of the blood bolus content of A. coluzzii mosquitoes infected with gametocyte cultures of P. falciparum. Live parasites within the mosquito bolus were dissected at 11, 14, 17, 20, 23, and 36 hpi and stained with the Hoechst nuclear dye and anti-Pfs25 antibodies. We identified five parasite developmental stages based on morphology, fluorescence patterns, and nuclear position (Figure 1A). Specifically, all immature stages were round shaped and displayed differences in the length of the protuberance that eventually gave the elongated shape to mature ookinetes. Stage I lacked the protuberance and was indistinguishable from egressed female gametes; stage II featured a short protuberance that did not exceed in length the radius of the main spherical body (knob); stage III had an elongated well-developed protuberance of at least the length of the radius of the main spherical body (stem); stage IV had a round nucleated body of reduced size and a wide long protuberance; stage V acquired the characteristic elongated shape with or without attached residual spherical body (Figure 1A). Importantly, stages I–IV displayed nuclear staining in the round body. Nuclear translocation from the residual round body to the well-formed elongated protuberance was observed during the transition from stage IV to stage V (Supplementary Figure 1). We also detected parasites that were suggestive of a degenerated form of a mature ookinete with disrupted Pfs25 surface signal and named them ghosts (Figure 1A). As these forms were only observed after appearance of mature ookinetes at the late time point of ookinete development in vivo, we speculate that ghosts represent ookinetes undergoing degradation probably due to the harsh environment of the mosquito midgut. This classification was used to measure the proportion of each stage in the mosquito blood bolus at six time points after infection. We found no obvious morphological changes in the round-shaped parasites until 11 hpi. During the next 3–14 h, the zygotes progressively developed protuberance, translocated the nucleus from the shrinking round body to the protuberance, and converted into mature ookinetes. We established the following kinetics of the ookinete development: stage II dominated at 14 hpi, stage III at 17 hpi, and stage IV between 17 and 23 hpi (Figures 1B,C and Supplementary Table 2). Mature ookinetes appeared at 17 hpi, peaked at 23 hpi, and persisted in the blood bolus until 36 hpi, whereas the ghost forms accumulated between 23 and 36 hpi (Figures 1B,C and Supplementary Table 2). The absolute number of Pfs25-positive parasites per mosquito blood bolus remained constant until 23 hpi but decreased at 36 hpi (Supplementary Figure 2 and Supplementary Table 2), likely reflecting the ookinete traversal of the mosquito midgut. Interestingly, only half of bolus-resident parasites developed into ookinetes, suggesting that at some stages, parasite development was restricted by the mosquito environment. We concluded that the observed progressive changes in proportions of developmental stages were not caused by parasite mortality but represented the dynamics of ookinete development in the mosquito.
Development of Mature Ookinetes in the Mosquito Blood Bolus Reliably Predicts Successful Mosquito Infection
As only half of the ingested parasites transformed into mature ookinetes (Figure 1B), we next evaluated how well the exflagellation rate of a given gametocyte culture, the conversion rate of egressed gametes into all ookinete stages (stages II to V) or into mature ookinetes only (stage V), predicted the success of oocyst formation. Ookinete development was monitored by anti-Pfs25 staining at 24 hpi as described above, whereas oocysts were labeled by mercurochrome staining for counting at 11 days post infection (dpi). In line with the field studies, gametocyte exflagellation rate (accounting only for male gametocytes) was a poor predictor of oocyst prevalence (Figure 2A). We found that oocyst prevalence (number of oocyst-positive mosquitoes/total number of blood fed mosquitoes) correlated significantly with the ookinete conversion rate [number of converted ookinetes (stages II to V)/total Pfs25-positive parasites] and the proportion of mature ookinetes [number of mature ookinetes (stage V)/total Pfs25-positive parasites] (Figures 2B,C and Supplementary Table 3). We concluded that similar to natural infections, ookinete conversion is a more reliable predictor of parasite development in the mosquito than exflagellation rate, pointing to some critical steps that regulate gamete fusion and early stages of zygote development.
Critical Stages of Ookinete Development in vitro
To identify developmental bottlenecks in ookinete conversion, we compared the dynamics of in vivo and in vitro ookinete development using the same initial gametocyte culture for mosquito infections and ookinete culture. At selected time points, parasites were isolated from the mosquito bolus or collected from the culture, stained with anti-Pfs25 antibodies and staged as described above. The absolute number of Pfs25-positive parasites stayed overall constant in all conditions until 23 hpi (Supplementary Figure 3). As expected, at 23 hpi, the majority of zygotes in the mosquito bolus developed protuberances, and 40% of them converted into mature ookinetes. In contrast, at the same time point in vitro, most of the detected Pfs25-positive forms remained at stage I (Figures 3A,B). As gametocytes efficiently egressed in both conditions (Supplementary Table 1), our findings are consistent with the previous reports that proposed a block in gamete fertilization in vitro. Focusing the analysis on the small proportion of ookinetes that developed from stage II onward, we observed an accumulation of stage III parasites. While this stage was dominant at the 14 h post culture setup, the corresponding samples in vivo showed a higher proportion of stage II parasites (Figures 3C,D, p-values = 0.0002, paired z-test). Interestingly, stage III parasites continued to accumulate in cultures in vitro at later time points, and only a small proportion of the parasites developed into mature ookinetes. In contrast, slower development of stage III forms in vivo was accompanied by swift progression to stage IV and V ookinetes. Our results identified a specific critical step in ookinete development after fertilization–the rate of stage III to stage IV transition–morphologically corresponding to a proper enlargement of the ookinete protuberance.
We next attempted to rescue ex vivo the critical step of ookinete development blocked in vitro. In these experiments, mosquitoes were infected with the gametocyte cultures, and 2 h later, the parasites were extracted from the insect gut and put into culture in vitro for an additional 21 h. In parallel, we examined the dynamics of ookinete development from the same gametocyte culture in vivo and in vitro as described above (Figure 4A). As the mosquito environment is not sterile, the ex vivo isolated parasites were treated with an antibiotic/antimycotic mix to prevent the overgrowth of the microbes inhabiting the mosquito midgut. This antimicrobial treatment had no effect on parasite development (Supplementary Figure 4). We found that the proportion of ookinetes that bypassed stage I was not significantly different in in vivo versus ex vivo conditions, demonstrating that the first 2 h of in vivo development rescued the fertilization block in vitro to the levels observed in vivo (Figure 4B, p-value = 0.09, paired z-test). However, the majority of the ex vivo parasites remained at stage III at 23 hpi, with only a minor fraction developing into mature ookinetes (p-value = 0.0001, paired z-test). The arrested stage III parasites featured a much thinner protuberance than those obtained in vivo (Figure 4C). Altogether, our results describe a new critical step in ookinete development. Further studies should investigate cellular mechanisms that control protuberance emergence and enlargement in P. falciparum.
Discussion
The human-to-mosquito Plasmodium transition is essential for transmission of malaria parasites and a promising target of drug- or antibody-mediated transmission-blocking interventions. With both pharmacological and immunological interventions receiving increased attention, the absence of robust ookinete in vitro culturing methods poses a major roadblock for identification of potent inhibitory targets. Here, by comparing the dynamics of ookinete development in vivo and in vitro, we characterize mosquito-regulated critical steps in early ookinete maturation. The first mosquito factor shaping host-to-vector transmission was identified almost 40 years ago and was shown to increase exflagellation efficiency of male gametocytes in the avian malaria parasite Plasmodium gallinaceum (Nijhout, 1979). Later on, this factor was purified from mosquito extracts and identified as xanthurenic acid (Billker et al., 1998). The results reported here postulate an existence of additional mosquito factors that promote gamete fertilization and zygote morphogenesis.
We found that the rate of gamete conversion to ookinetes was a good predictor of oocyst production in the mosquito, whereas the rate of production of male gametes (exflagellation) was not. These observations indicate that the first 24 h are crucial for Plasmodium establishment in its vector. These results are in line with the field studies that showed that numbers of circulating gametocytes do not always predict parasite infectiousness to the insect vector (Bousema and Drakeley, 2011). A meta-analysis of the infectiousness of P. falciparum-infected blood samples from children from Burkina Faso and Tanzania to A. gambiae, and a similar study with P. vivax and A. dirus, revealed a non-linear relationship between the gametocyte titers in the donors’ blood and infection prevalence in the mosquito (Churcher et al., 2013; Kiattibutr et al., 2017). Furthermore, a recent meta-analysis conducted on P. falciparum gametocyte carriers in Mali, Burkina Faso, and Cameroon revealed a broad positive correlation between circulating female gametocytes and infected mosquitoes, while circulating male gametocytes contributed to mosquito infection only at low gametocyte densities (Bradley et al., 2018). These findings provide an additional proof that the Plasmodium exflagellation rate, which corresponds to the fraction of mature male gametocytes, is a poor predictor of carrier infectivity to mosquitoes.
We propose that the poor predictive properties of gamete activation are linked to variability in mosquito factors that control fertilization and zygote development. The importance of the mosquito environment is supported by two observations: (i) rescue of gamete fertilization block by within-mosquito development during the first 2 h after infection and (ii) abnormal morphogenesis of stage III parasites in vitro that results in narrow protuberance, which may block nuclear migration at stage IV. Interestingly, the defined here ordering of stages III and IV differs from that proposed by Ghosh et al. (2010), where the ookinetes with enlarged stem (stage IV here) were positioned before the forms with the elongated stem (stage III here). Therefore, the systematic time-series approach applied here contributed to a better understanding of the timing and order of the crucial steps in ookinete development.
The synchronous comparison of in vivo and in vitro ookinete maturation confirmed that P. falciparum gamete fertilization is very inefficient in vitro (Delves et al., 2017) (Graphical Abstract). Here, we demonstrate that this block is completely reverted by allowing in vivo parasite within-mosquito development within the first 2 h. Our cultures in vitro contained xanthurenic acid, and we detected no differences in gamete egress between in vivo and in vitro conditions. Therefore, we propose that gamete fusion requires other mosquito factor(s). Currently, the nature of these factors is unknown. Interestingly, our conclusions are in line with an earlier study which used a computational approach to model ookinete development (Lawniczak and Eckhoff, 2016).
In contrast to fertilization, the short pulse of in vivo development did not rescue the second block in the ookinete maturation in vitro. Instead of delay, we observed faster development of the protuberance and, hence, accumulation of stage III in vitro. However, this faster development translated into morphological abnormalities, as stage III developed thinner protuberance in vitro. This observation could be explained by higher concentrations of some permissive factor(s) in the culture medium as compared to the natural mosquito environment. Identification of the factor(s) not only will allow optimization of in vitro conditions but also will inform how within-mosquito variability in this factor affects ookinete development in vivo. In conclusion, the results presented here suggest that successful P. falciparum fertilization and ookinete maturation require the continuous presence of multiple as yet unknown mosquito factors. Identification of these factors should benefit the establishment of P. falciparum ookinete culture in vitro and uncover the mechanisms that regulate early stages of transmission of this deadly parasite.
Data Availability Statement
All datasets generated for this study are included in the articleSupplementary Material.
Author Contributions
GS, GC, and PS-C conceived and performed the experiments, analyzed the results, and wrote the manuscript. AV performed statistical analyses and wrote the manuscript. PA and EL conceived experiments, analyzed the data, and wrote the manuscript.
Conflict of Interest
The authors declare that the research was conducted in the absence of any commercial or financial relationships that could be construed as a potential conflict of interest.
Funding
GS was supported by the grant ISARP “Farmaci di nuova generazione contro la trasmissione di Plasmodium falciparum per la eradicazione della malaria” from the Italian Ministry of Foreign Affairs and International Cooperation and Istituto Superiore di Sanità. This study has received funding from the European Union’s Horizon 2020 Research and Innovation Programme under grant agreement no. 731060.
Acknowledgments
We thank M. Andres, D. Eyermann, H. Ahmed, and L. Spohr (Vector Biology Unit, Max Planck Institute for Infection Biology, Berlin) for mosquito rearing, parasite culturing, and P. falciparum infections. The following reagent was obtained through BEI Resources, NIAID, NIH: Hybridoma 4B7 Anti-Plasmodium falciparum 25 kDa Gamete Surface Protein (Pfs25), MRA-315, contributed by Louis H. Miller and Allan Saul. We thank Tuula Geske (Central Laboratory Facility, Deutsches Rheuma-Forschungszentrum, Berlin) for anti-Pfs25 IgG production, purification, and labelling.
Supplementary Material
The Supplementary Material for this article can be found online at: https://www.frontiersin.org/articles/10.3389/fmicb.2020.00269/full#supplementary-material
References
Billker, O., Lindo, V., Panico, M., Etienne, A. E., Paxton, T., Dell, A., et al. (1998). Identification of xanthurenic acid as the putative inducer of malaria development in the mosquito. Nature 392, 289–292. doi: 10.1038/32667
Bounkewa, V., Li, F., and Vinetz, J. M. (2010). In vitro generation of Plasmodium falciparum ookinetes. Am. J. Trop. Med. Hyg. 83, 1187–1194. doi: 10.4269/ajtmh.2010.10-0433
Bousema, T., and Drakeley, C. (2011). Epidemiology and infectivity of Plasmodium falciparum and Plasmodium vivax gametocytes in relation to malaria control and elimination. Clin. Microbiol. Rev. 24, 377–410. doi: 10.1128/CMR.00051-10
Bradley, J., Stone, W., Da, D. F., Morlais, I., Dicko, A., Cohuet, A., et al. (2018). Predicting the likelihood and intensity of mosquito infection from sex specific Plasmodium falciparum gametocyte density. eLife 7:e34463. doi: 10.7554/eLife.34463
Carter, E. H., Suhrbier, A., Beckers, P. J., and Sinden, R. E. (1987). The in vitro cultivation of P. falciparum ookinetes and their enrichment on Nycodenz density gradients. Parasitology 95, 25–30. doi: 10.1017/s0031182000057516
Churcher, T. S., Bousema, T., Walker, M., Drakeley, C., Schneider, P., Ouédraogo, A. L., et al. (2013). Predicting mosquito infection from Plasmodium falciparum gametocyte density and estimating the reservoir of infection. eLife 2:e00626. doi: 10.7554/eLife.00626
Delves, M. J., Marques, S. R., Ruecker, A., Straschil, U., Miguel-Blanco, C., López-Barragá, M. J., et al. (2017). Failure of in vitro differentiation of Plasmodium falciparum gametocytes into ookinetes arises because of poor gamete fertilisation. bioRxiv[Preprint]
Ghosh, A. K., Dinglasan, R. R., Ikadai, H., and Jacobs-Lorena, M. (2010). An improved method for the in vitro differentiation of Plasmodium falciparum gametocytes into ookinetes. Malar. J. 9:194. doi: 10.1186/1475-2875-9-194
Janse, C. J., Mons, B., Rouwenhorst, R. J., Van der Klooster, P. F., Overdulve, J. P., and Van der Kaay, H. J. (1985). In vitro formation of ookinetes and functional maturity of Plasmodium berghei gametocytes. Parasitology 91(Pt 1), 19–29. doi: 10.1017/s0031182000056481
Kiattibutr, K., Roobsoong, W., Sriwichai, P., Saeseu, T., Rachaphaew, N., Suansomjit, C., et al. (2017). Int. J. Parasitol. 47, 163–170. doi: 10.1016/j.ijpara.2016.10.006
Lawniczak, M. K., and Eckhoff, P. A. (2016). A computational lens for sexual-stage transmission, reproduction, fitness and kinetics in Plasmodium falciparum. Malar J. 15, 487. doi: 10.1186/s12936-016-1538-5
Nijhout, M. M. (1979). Plasmodium gallinaceum: exflagellation stimulated by a mosquito factor. Exp. Parasitol. 48, 75–80. doi: 10.1016/0014-4894(79)90056-0
Sinden, R. E., Hartley, R. H., and Winger, L. (1985). The development of Plasmodium ookinetes in vitro: an ultrastructural study including a description of meiotic division. Parasitology 91, 227–244. doi: 10.1017/s0031182000057334
Warburg, A., and Schneider, I. (1993). In vitro culture of the mosquito stages of Plasmodium falciparum. Exp Parasitol. 76, 121–126. doi: 10.1006/expr.1993.1014
Keywords: malaria parasite transmission, Plasmodium falciparum, Anopheles coluzzii, ookinete maturation, time course of zygote development, developmental bottleneck
Citation: Siciliano G, Costa G, Suárez-Cortés P, Valleriani A, Alano P and Levashina EA (2020) Critical Steps of Plasmodium falciparum Ookinete Maturation. Front. Microbiol. 11:269. doi: 10.3389/fmicb.2020.00269
Received: 21 November 2019; Accepted: 06 February 2020;
Published: 17 March 2020.
Edited by:
Rhoel Dinglasan, University of Florida, United StatesReviewed by:
Michael Delves, University of London, United KingdomRyan Smith, Iowa State University, United States
Henrique Silveira, New University of Lisbon, Portugal
Copyright © 2020 Siciliano, Costa, Suárez-Cortés, Valleriani, Alano and Levashina. This is an open-access article distributed under the terms of the Creative Commons Attribution License (CC BY). The use, distribution or reproduction in other forums is permitted, provided the original author(s) and the copyright owner(s) are credited and that the original publication in this journal is cited, in accordance with accepted academic practice. No use, distribution or reproduction is permitted which does not comply with these terms.
*Correspondence: Pietro Alano, cGlldHJvLmFsYW5vQGlzcy5pdA==; Elena A. Levashina, bGV2YXNoaW5hQG1waWliLWJlcmxpbi5tcGcuZGU=
†These authors have contributed equally to this work