- 1Departamento de Biotecnología Microbiana y de Plantas, Centro de Investigaciones Biológicas (CSIC), Madrid, Spain
- 2CIBER de Enfermedades Respiratorias (CIBERES), Madrid, Spain
- 3Intrahospital Infections Laboratory, National Centre for Microbiology, Instituto de Salud Carlos III (ISCIII), Majadahonda, Spain
The molecular basis of the pathogenesis of the opportunistic invasive infections caused by isolates of the Gemella genus remains largely unknown. Moreover, inconsistencies in the current species assignation were detected after genome-level comparison of 16 public Gemella isolates. A literature search detected that, between the two most pathogenic species, Gemella morbillorum causes about twice the number of cases compared to Gemella haemolysans. These two species shared their mean diseases – sepsis and endocarditis – but differed in causing other syndromes. A number of well-known virulence factors were harbored by all species, such as a manganese transport/adhesin sharing 83% identity from oral endocarditis-causing streptococci. Likewise, all Gemellae carried the genes required for incorporating phosphorylcholine into their cell walls and encoded some choline-binding proteins. In contrast, other proteins were species-specific, which may justify the known epidemiological differences. G. haemolysans, but not G. morbillorum, harbor a gene cluster potentially encoding a polysaccharidic capsule. Species-specific surface determinants also included Rib and MucBP repeats, hemoglobin-binding NEAT domains, peptidases of C5a complement factor and domains that recognize extracellular matrix molecules exposed in damaged heart valves, such as collagen and fibronectin. Surface virulence determinants were associated with several taxonomically dispersed opportunistic genera of the oral microbiota, such as Granulicatella, Parvimonas, and Streptococcus, suggesting the existence of a horizontally transferrable gene reservoir in the oral environment, likely facilitated by close proximity in biofilms and ultimately linked to endocarditis. The identification of the Gemella virulence pool should be implemented in whole genome-based protocols to rationally predict the pathogenic potential in ongoing clinical infections caused by these poorly known bacterial pathogens.
Introduction
Opportunistic pathogens are organisms that can become pathogenic only after certain perturbations to the host, such as disease or injury prior infection, alteration of the immune system, or aging (Brown et al., 2012). Such species may have remained overlooked in the clinical setting due to their intrinsic difficulty in being identified by ordinary microbiological and/or molecular methods. Nevertheless, the advent of new techniques for the identification of bacterial species, such as matrix-assisted laser desorption ionization–time of flight mass spectrometry (MALDI-TOF MS), has been instrumental in detecting infections caused by unusual pathogens (Seng et al., 2013). The implementation of other techniques such as whole-genomic sequencing of bacterial clinical isolates, is greatly encouraged to increase identification resolution, particularly with rare bacteria (Quainoo et al., 2017).
Gemella sp. represents one of the bacterial groups that exemplify the irruption of relatively frequent, opportunistic pathogens in the clinical laboratory. Gemellae are facultatively anaerobic, cytochrome oxidase- and catalase-negative Gram-positive cocci with a DNA G+C content (mol%) as low as 30–34 (Collins and Falsen, 2015). Bacterial species are commonly defined by applying a set of predetermined criteria, most frequently including phenotypic data and 16S rRNA gene sequence similarity. When required, DNA–DNA hybridization experiments as well as genome-based criteria such as average nucleotide identity (ANI) are also employed. The identification of Gemella isolates represents a challenge to clinical laboratories. Manual or commercial phenotypic methods may result in misidentification of Gemella spp. as viridans group streptococci or other related organisms and vice versa (Christensen and Ruoff, 2015). Also 16S rRNA gene sequencing is frequently employed to identify these microorganisms (Woo et al., 2003 and references therein). To date, 98.65% 16S rRNA gene sequence similarity is the threshold for differentiating two species (Kim et al., 2014). It is noteworthy that, for example, the type strains of Gemella parahaemolysans (NTUH_1465T) and Gemella taiwanensis (NTUH_5572T) displayed 99.77 and 100% 16S rRNA gene sequence similarity with Gemella haemolysans ATCC 10379T (Hung et al., 2014). In such cases, phylogenetic analysis of concatenated sequences of two or more housekeeping genes must be performed for a detailed identification (Hung et al., 2010). Nevertheless, if a pathogen cannot be identified by conventional methods, MALDI-TOF MS should be considered (Welker et al., 2019), although it should be noted that some rare isolates may not be included in the database at the time of analysis (Fonkou et al., 2018). Inconsistencies with MALDI-TOF MS results based on potential biases in phenotypic typing data from various protein expression levels must also be considered. Even though MALDI-TOF MS was able to distinguish outbreak strains with shorter turnaround times, whole-genome sequencing (WGS) analysis provided far-higher discriminatory power (recently reviewed by Hayashi Sant’Anna et al., 2019), which ultimately allowed an improved understanding of transmission events. It has been proposed that in an outbreak scenario, MALDI-TOF MS could be used to complement WGS as a rapid initial analysis tool until WGS data are generated (Quainoo et al., 2017). A very recent study has highlighted the appropriateness of WGS to investigate the presence of microorganisms (including Gemellae) in biopsies from patients with endophthalmitis (Deshmukh et al., 2019).
Currently, G. haemolysans is the type species of the genus which currently contains eleven different species (last date accessed, 10 January 2020); these include nine species with standing in nomenclature (Parte, 2018) and two more recently described species (“Gemella massiliensis”) (Fonkou et al., 2018) and “Gemella muriseptica” (Eaton et al., 2019). Gemella isolates have been found as commensals in metagenomic approaches of the oral cavity, the upper respiratory tract, the intestine, the breastmilk of healthy mothers and the female genital tracts of humans (van de Wijgert et al., 2014; Boix-Amorós et al., 2016; Takayasu et al., 2017; Esposito and Principi, 2018; Villmones et al., 2018).
Gemellae are related to the Mitis and Salivarius groups of streptococci (Facklam and Elliott, 1995; Ruoff, 2002) that are also members of the oral microbiota. These streptococci, such as Streptococcus gordonii, Streptococcus mitis, and Streptococcus sanguinis, are well-known opportunistic pathogens able to cause invasive diseases like sepsis and endocarditis (Abranches et al., 2018). Notably, Gemella carriage has also been associated with allergies and asthma in children (Dzidic et al., 2018). Evidence of horizontal gene transfer (HGT) to Gemella of some genes associated with virulence in related streptococci is being accumulated. These include genes encoding mainly paralogous zinc metalloproteases (namely iga, zmpB, and zmpC) from Streptococcus sp. (Takenouchi-Ohkubo et al., 2006; Bek-Thomsen et al., 2012).
Despite this information, a detailed study of the virulence potential of Gemella spp. at a genomic scale is, to the best of our knowledge, lacking. Gemella genomic sequences have been produced since the first sequence reported in 2008. With the aim of closing the current gap between genome availability and acquisition of biological value for this pathogen, a global genome analysis and identification of potential virulence factors have been approached in the present study. Results may help to understand the pathogenicity of the Gemella genus in opportunistic infections.
Materials and Methods
Literature Search
Reports were detected by searching the term “Gemella” in PubMed (last date accessed, December 31, 2018), followed by manual revision to verify unequivocal case reports involving Gemella spp. without considering co-infections.
Genome and Proteome Management
All the original genomic and proteomic information was downloaded from the ASSEMBLY database (Kitts et al., 2016) hosted by the National Center for Biotechnology Information (NCBI). Gene sequences were extracted from the whole genome sequence using the coordinate information. ANI comparisons were carried out using ORTHOANIU (Yoon et al., 2017). Correspondence analysis of codon usage was carried out with CODONW.
16S rRNA sequences were downloaded from the SILVA database (Quast et al., 2013), and from original genomic sequences. Approximately 1300 nucleotides were required. MultiLocus Sequence Analysis (MLSA) was carried out after concatenation of groEL, recA and rpoB complete or fragment sequences. Sequences were aligned with Clustal Omega (Sievers and Higgins, 2018) and subjected to phylogeny analysis using MEGA 10.0.5 (Kumar et al., 2018).
Homolog Detection and Analysis
Overlapping between Gemella proteomes was carried out by clustering with CD-HIT v4.6 (Fu et al., 2012) applying on a ≥ 70% identity and bidirectional 80% alignment length basis. Virulence factors were detected by BLAST against the virulence factor database (VFDB) virulence factor list applying ≥40% identity, ≥70% of the alignment length and E-value ≤10–20, as thresholds. The closest non-Gemella species – not necessarily the donor – to a Gemella protein or domain was selected as the first one to reach three hits as sorted by E-value, using BLASTp of NCBI against the non-redundant protein sequences with default parameters and a bidirectional length alignment coverage of ≥80%. Sequences were aligned by Clustal Omega (Sievers and Higgins, 2018). Phylogenetic analyses were carried out with Mega 10.0.5 (Kumar et al., 2018).
Surface Protein Identification and Analysis
Surface proteins were defined as those containing anchoring domains provided by Pfam v31 (El-Gebali et al., 2019), Prosite 2018_09 and TIGRFAM databases: choline-binding domains (CBDs) (PF01473 Pfam domain and PS51170 PROSITE rule), LPxTG (PF00746/PS50847/TIGR01167) or LysM (PF01476/PS51782/-) domains. Pfam and TIGRFAM profiles were detected using Pfamscan, whereas PROSITE rules were applied using the ps-scan script (de Castro et al., 2006), in all cases using the recommended gathering thresholds. Best taxon hits were identified for whole protein and domain Gemella sequences by BLAST against the non-redundant (nr) database of the NCBI. The species/taxon with the first three hits (highest scores) was considered.
To search for potential competence genes, Gemella proteomes were screened through BLAST to competence-related proteins in UniProt plus those manually added corresponding to the DNA uptake machinery (Kovács et al., 2009; Straume et al., 2015) such as ComGA–ComGG in Bacillus subtilis 168, and CglA–CglD in Streptococcus pneumoniae TIGR4. Relaxed thresholds of 20% identity covering ≥50–60% alignment length of the original protein were applied.
Results
Epidemiological Survey of the Current Literature Indicates That Gemellae Are Versatile Opportunistic Pathogens
To assess the epidemiological importance of Gemella, all PubMed abstracts concerning case reports associated with species of this genus were manually collected and supervised. A total of 212 reports were found until 2018, where nearly all articles concerned one single patient. The clinical case report is a popular genre in medical writing (Nissen and Wynn, 2014a), which advantages and disadvantages have been discussed in detail previously (Ostrowsky, 2007; Nissen and Wynn, 2014b; Johnson, 2018). As case reports are the clinical description of single patients, these findings may be atypical and not generalizable to other populations. Moreover, this type of study cannot lead to conclusions regarding causality. Nevertheless, case reports are inexpensive and useful in planning natural history studies, forming hypotheses and describing clinical experience. Frequently, phenomena observed in clinical practice provide the first clues of more generalized etiologies or risks and provide valuable suggestions for further study.
Gemellae were involved in up to 41 distinct syndromes with a disparate range of anatomic sites, occurrences, invasiveness and immunocompetence of the patient. According to these published data, it appears that co-morbidities, immunosuppressive therapy and particular situations in previously immunocompetent individuals, such as dental treatments, are more important than age or gender in contracting Gemella diseases. Nine syndromes involved more than five literature reports, from which “endocarditis of the native valve” excelled (62 reports, 29% of the total) (Figure 1A). Reports were steady from 1995 until the present for the two species that accounted for the vast majority of clinical cases: Gemella morbillorum (129 cases, 64%, 5.1 yearly reports) and G. haemolysans (60 reports, 30%, 2.0 yearly reports) (Figure 1B). Comparatively, the clinical literature impact of Gemella sanguinis (8 reports) and, in particular, Gemella bergeri (4 reports) was scarce. While the proportion of cases involving the two most pathogenic species is maintained for bacteremia, endocarditis (with or without prosthetic valves) and brain abscesses, there were discrepancies for other diseases. In particular, G. haemolysans caused more eye infections (endophthalmitis and crystalline keratopathy) and meningitis whereas G. morbillorum produced more liver abscesses, pleural empyemas and septic arthritides (Figure 1C). Although there is an unavoidable bias toward publication of most serious cases, our literature screening supports that Gemella is a relatively uncommon, albeit recurrent and versatile pathogen, mostly in immunocompromised patients, with remarkable epidemiological differences between species.
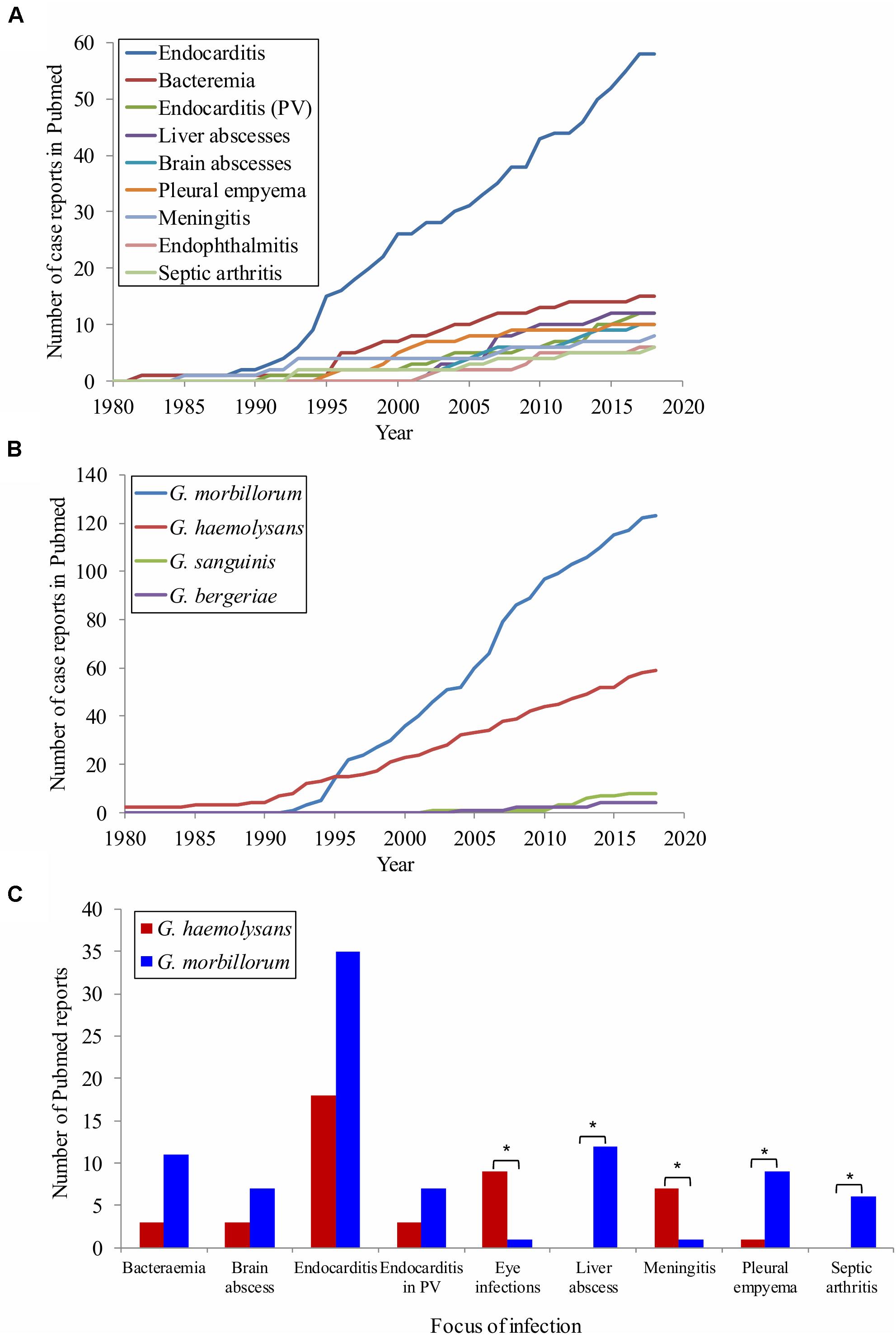
Figure 1. Gemella clinical reports in the literature. (A) Cumulative number of reported cases per year. (B) Cumulative number of reported cases per species over time. The number of reports by disease (A) and by species (B) was sorted in the legend in descending order. (C) Report occurrence for the two most virulent species for diseases with >5 reported cases. Statistical significance was calculated for G. morbillorum by two-tailed Fisher’s exact test considering the observed cases respect to the expected cases based on the relative occurrence observed in G. haemolysans. *P ≤ 0.05.
Genome-Wide Comparisons Reveal Unnoticed Relationships Between Gemella Isolates
In a first attempt to support the observed epidemiological trends, the global organization of the genus was studied in detail from a genomic perspective. The 16 publicly available Gemella genomes (Supplementary Table S1) (last date accessed December 31, 2018) were analyzed, although only four of them were complete. Most genomes belong to oral metagenomic samples. Genome sizes ranged 1.60–2.05 Mb with G+C content 29.0−30.9%. The exception was Gemella assacharolytica whose genome was probably incomplete (only 1.29 Mb), and that has a G+C content of 26.7%.
The G. morbillorum and G. sanguinis isolates satisfied the ≈94–96% ANI threshold, which is the current gold standard for prokaryotic species circumscriptions at the genomic level (Konstantinidis and Tiedje, 2005; Richter and Roselló-Móra, 2009). Unexpectedly, the genome of the proposed “G. massiliensis” strain also shared >99% ANI with that of strains W2231 and 6198 Gemella sp. isolates, indicating that all of them belong to a close phylotype of the same species. Moreover, those three genomes showed 94.9% ANI with that of the type species (T) of G. bergeri, strongly suggesting that they actually represent members of the same species. In contrast, the genome of G. haemolysans strain M341 only showed ≈87% ANI with respect to the remaining three isolates of G. haemolysans from which, in turn, DNF011367 only reached 93.6% ANI between them. Therefore, we propose to combine all these isolates into a potential “Haemolysans group” rather than being members of a single species. On the other hand, Gemella cuniculi is a zoonotic species that was not further considered. Genome data indicate therefore the existence of four principal Gemella taxons in humans: G. bergeri, the “Haemolysans group,” G. morbillorum, and G. sanguinis, visualized as dark color squares along the diagonal of heatmaps calculated from ANI data (Figure 2).
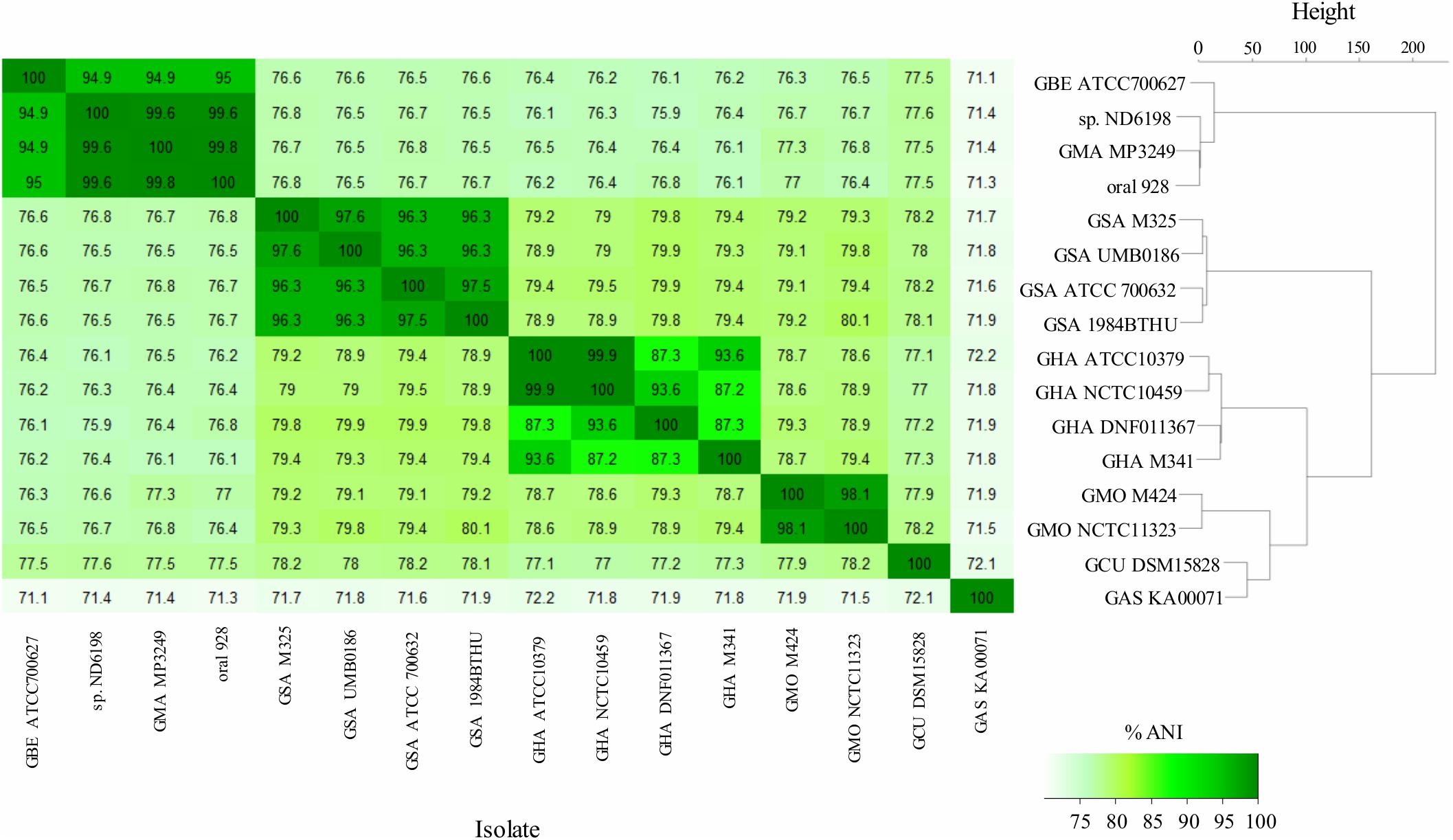
Figure 2. Genomic comparison between Gemella isolates. ANI matrix heatmap (left) and hierarchical clustering based on ANI values (right). Prefixes: GAS: G. assacharolytica; GBE: G. bergeri; GCU: G. cuniculi; GHA: G. haemolysans; GMA: G. massiliensis; GMO: G. morbillorum; GSA: G. sanguinis.
The relationship between isolates was validated by 16S rRNA and MLSA (groEL, recA, and rpoB genes) phylogeny, which essentially rendered the same genus structure (Supplementary Figure S1). The only remarkable exception was the “Haemolysans group” which was not so clearly resolved compared to the whole-genome approach. In addition, three other Gemella spp. associated with disease or human colonization, but without available genome sequences, were analyzed by at least one of these methods. By doing so, G. parahaemolysans (Hung et al., 2014) and G. taiwanensis (Hikone et al., 2017) were also members of the “Haemolysans group,” whereas Gemella palaticanis (Hoyles et al., 2000) had its own clade.
The Properties of Gemella Accessory Proteomes Suggest Acquisition by Horizontal Gene Transfer
It would be expected that syndromes caused by any Gemella isolate may fall on the protein pool shared by all species, whereas epidemiologic particularities of the two most virulent species can be attributed to their unique protein subsets. To explore this hypothesis, core and accessory proteomes for the whole genus and the respective “species groups” were analyzed. Up to 860 proteins (≈50% of a Gemella proteome) (Figure 3A) were shared by all isolates in the four taxons considered. Specific core proteomes ranged from 62 to 70 proteins whereas the accessory proteomes reached 278–550 proteins, where the “Haemolysans group” stands out as expected from its taxonomic range wider than a single species. The 751 proteins of the panproteome that were in other situations, i.e., those shared by different species groups but not in their core genomes in all cases, were classified into the “Other” class. Among them, 57 proteins were exclusively shared by all isolates of the pathogenic species while only 6–17 proteins were shared by any of the three-species core combinations that include the low-pathogenicity control G. bergeri.
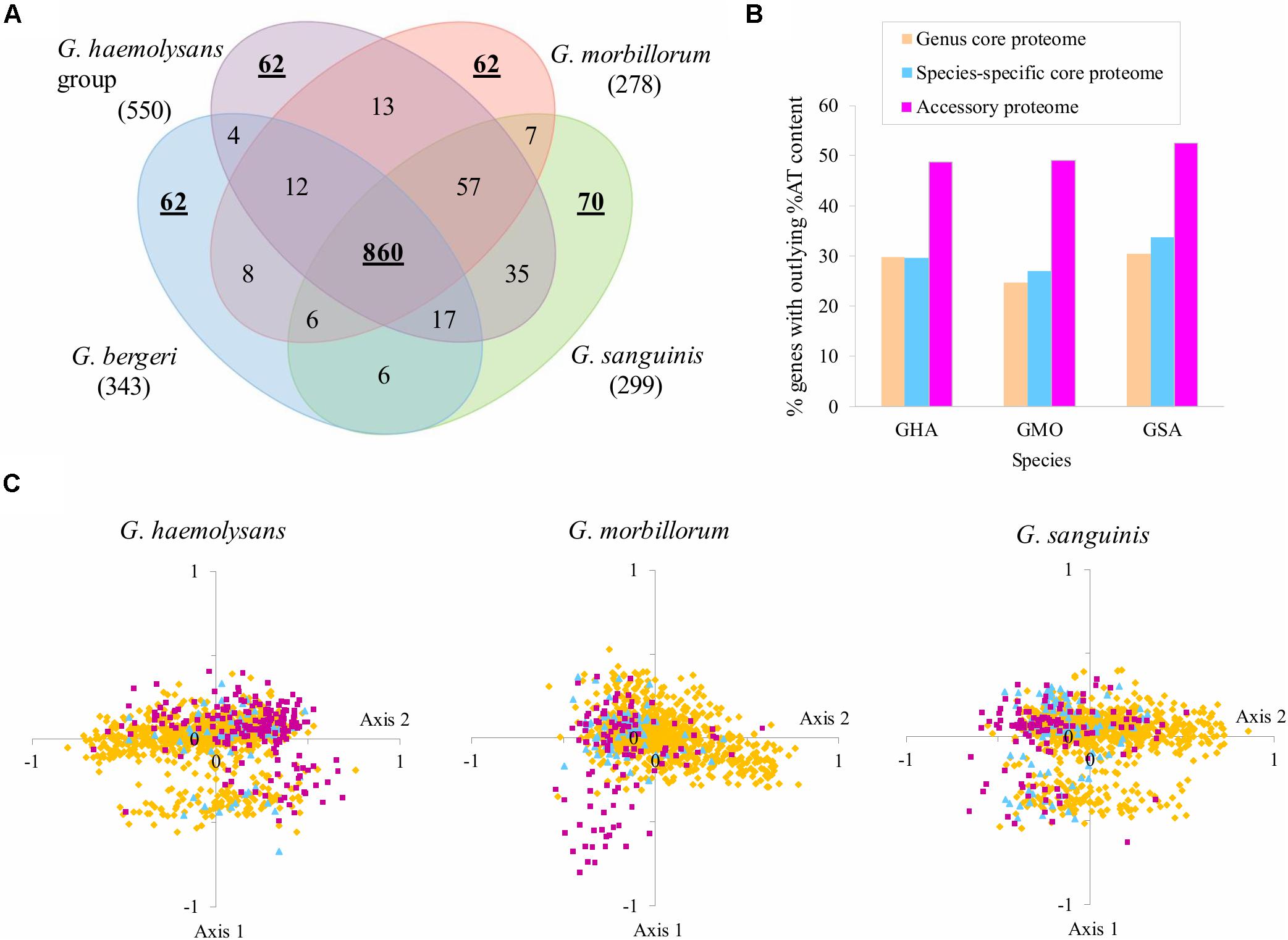
Figure 3. Proteome comparison between Gemella taxons. (A) Venn diagram quantifying the degree of overlapping between the core proteomes of the four Gemella taxons. The number of exclusive accessory proteins is indicated in brackets. (B) Percentage of genes with outlying %GC composition defined as those showing at least one standard deviation (z score) higher or lower than the average in representative species isolates. The z scores were calculated using the average ± SD of the GC content of genes in the representative species for the three taxons considered as pathogenic that was 31.0 ± 3.9% for the G. haemolysans ATCC 10379 (GHE), 31.2 ± 4.0% for G. morbillorum M424 (GMO), and 29.9 ± 3.7% for G. sanguinis ATCC 700632 (GSA). (C) Correspondence analysis of codon usage. Panel for representative isolates for each species. Each point represents the location of a gene for the two axes showing the highest non-redundant variability for codon composition. Genes are labeled according to colors corresponding to genus core, species core and isolate-accessory genes as in panel (B).
The percentage of genes encoding either the species-specific or the genus core proteomes that shows an atypical GC content, defined as those with a difference above one standard deviation with respect to the average, was ∼30%. However, these outlying cases reached around half of the genes encoding species accessory proteins (Figure 3B). A correspondence analysis of codon usage revealed that species-core and accessory genes tend to concentrate into a particular subsection of the sparse gene plot (Figure 3C). Overall, the taxon-stratified analyses of proteomic and genomic features indicated that species and isolates acquired foreign genetic material that may contribute to the different clinical outcomes caused by different Gemella spp.
Gemella Isolates Carry Common and Specific Virulence Factors
To gain rational insight into the molecular basis of syndromes common to all Gemella spp. as well as the species-specific virulence propensities, the putative pathogenic arsenal of Gemella was identified. A comparison of the Gemella proteomes to the VFDB (Liu et al., 2019) and the analysis of surface proteins – full sequences or their constituent domains – was carried out. Firstly, homologs for 34 non-redundant VFDB proteins from different pathogens were detected in at least one Gemella isolate (Supplementary Table S2). However, only 14 of them were represented in all Gemella groups, even when the demanded coverage threshold was relaxed to ≥80% isolates. This suggested that the core virulence arsenal of Gemella is relatively small and very likely subjected to gene gain and loss events.
It should be taken into account that global resources, such as the VFDB, may fail to detect novel, species-specific pathogenic determinants. Bacterial surface proteins greatly determine the behavior of the pathogen by directly interacting with different host molecules (Rohde and Chhatwal, 2013; Hammerschmidt et al., 2019). Each Gemella isolate analyzed contained between 10 and 30 predicted surface proteins, according to the presence of universal anchor domains (Dramsi and Bierne, 2017). With some exceptions, however, the intricate domain composition of these proteins prevents its straightforward inference to pathogenesis by comparison to bona fide virulence factors. Surface proteins were therefore decomposed into domains and independently scrutinized. From the 35 domains exposed in the surface proteins detected, some of them – or their combinations – were exclusively present in proteins from the species-specific core and accessory proteomes of the Gemella spp. causing the majority of clinical cases (Supplementary Table S3). The most notable putative virulence determinants are analyzed below.
Capsular Gene Clusters
The capsule is crucial in permitting the survival of many different pathogens in the bloodstream as it prevents opsonophagocytosis by minimizing complement deposition onto the bacterial surface (Lindberg, 1999). Despite early studies reporting the presence of a capsule in Gemella (Reyn et al., 1966), a genetic explanation for this feature is missing. With the remarkable exception of G. morbillorum, putative capsular gene clusters containing 14–17 loci were found in all the Gemella spp. considered (Figure 4A). With the exception of flanking genes, these capsular clusters were organized in a way similar to that of S. pneumoniae (Bentley et al., 2006; Yother, 2011) (Supplementary Tables S4–S6). The four upstream and the most downstream genes of the clusters showed GC contents of 34–36%. Excluding the gene located immediately upstream of the first gene of the cluster, the genes located at the extremes of such clusters were conserved among isolates of the same Gemella species but differed between species. At an intra-taxon level, except from the G. bergeri group for which capsular clusters were essentially identical (>90% amino acid identity), several deletion, gene substitution, and sequence divergence (70–90% identity) events were observed. Such alterations affected sugar-modifying enzymes such as glycosyltransferases and epimerases. All capsular genes showed a putative streptococcal origin with identities ranging from 47 to 80%. However, the closest organism (putative donor) involved a mixed myriad of streptococcal species (data not shown), suggesting multiple independent recombinant events. Notably, the five most 3′-terminal genes of the “Haemolysans group” had a putative unique S. pneumoniae origin.
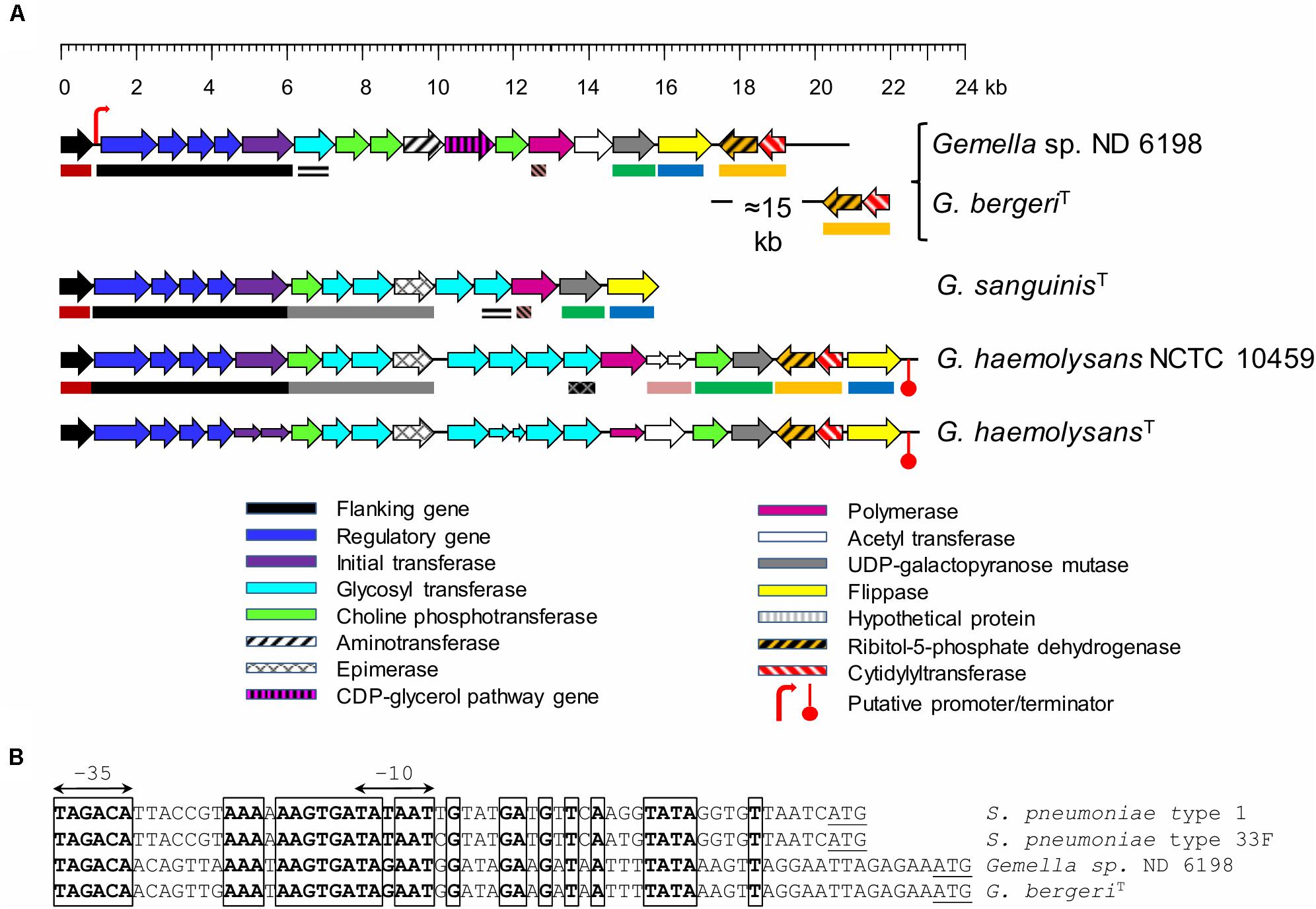
Figure 4. Genetic organization of capsular gene loci in Gemella spp. (A) Capsular gene clusters. Thick and thin arrows represent complete and interrupted open reading frames respectively. The arrows show the direction of transcription. Below the gene clusters, horizontal bars indicate regions that share 70–80% of nucleotide identity with those having identical color and shading. The proposed function of the corresponding gene products is also shown. (B) Putative promoter region of the Gemella capsular gene cluster. As a comparison, the corresponding region of S. pneumoniae types 1 and 33F is also shown. Nucleotides conserved in all the sequences are boxed and the ATG initiation codon of the first gene of the capsular operon is shown underlined.
Concerning regulation, a short region similar to that containing the proved promoter of pneumococcal capsular genes for serotype 1 and 33F was found upstream of the putative G. bergeri capsular operon (Figure 4B). However, this region was apparently deleted and/or reorganized in other Gemellae and a canonical promoter region appears to be missing (data not shown) or located elsewhere.
Phosphorylcholine and Choline-Binding Proteins in the Cell Walls
Several lines of evidence have suggested the existence of phosphorylcholine (PCho)-containing teichoic acids (TAs) and choline-binding proteins (CBPs) among Gemellae. First, using a mouse monoclonal anti-PCho antibody, Gillespie and co-workers noted the presence of PCho residues on the surface of some (but not all) isolates of G. haemolysans (Gillespie et al., 1996). More recently, sequence comparisons have revealed that some Gemella spp. encode orthologs of the S. pneumoniae LytB glucosaminidase (Bai et al., 2014). LytB, a member of the CBP family of proteins, is a chain-dispersing enzyme and an important pneumococcal virulence factor (López and García, 2004; Ramos-Sevillano et al., 2011; Corsini et al., 2016). The presence of pCho-TAs in Gemella was fully sustained by sequence comparisons (Supplementary Tables S7, S8). In particular, the proteins from the whole pathway (LicA, LicB, LicC, TarI, and TarJ) involved in Cho import, phosphorylation and binding of P-Cho residues to nascent TA chains in S. pneumoniae (Johnston et al., 2016) showed ≥60% sequence identity (≥75% similarity) with those from Gemellae. Such high identities and comparable features between species indicate the acquisition of these genes already in the Gemella ancestor. A schematic representation of the genes involved in TA biosynthesis in S. pneumoniae, and presumably, in three Gemella strains is shown in Figure 5A. An advantage of the presence of PCho in the cell wall is that choline itself is an important virulence factor by binding to the platelet-activating factor receptor, which enhances the adhesive capacities to several host cells (Iovino et al., 2013).
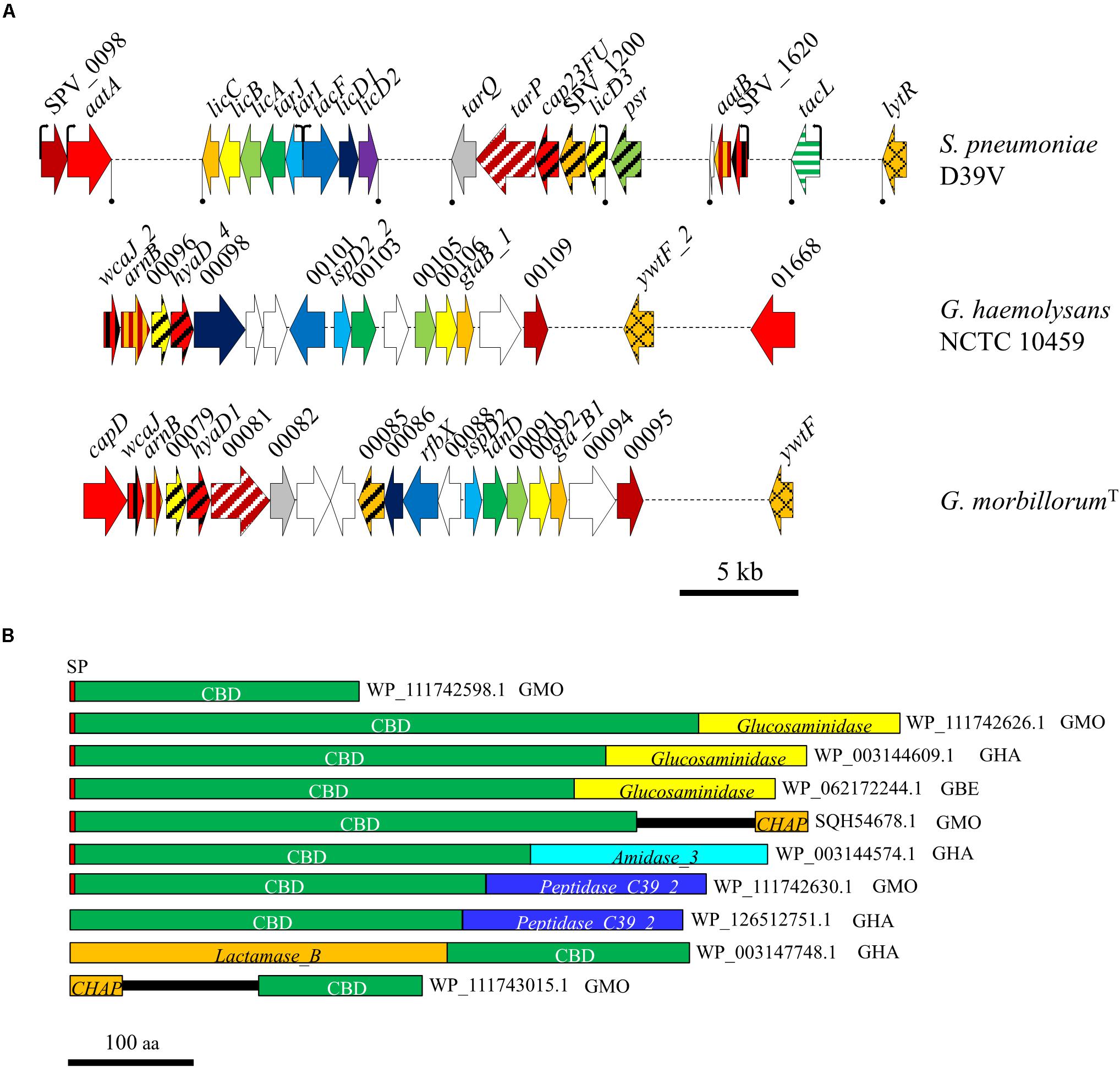
Figure 5. Genes involved in the synthesis of choline-containing teichoic acids and CBPs in Gemella. (A) Genes with predicted similar functions in different genomes are indicated by identical color and shading. (B) Predicted CBPs encoded in Gemella genomes. GBE, G. bergeri; GHA, G. haemolysans; GMO. G. morbillorum. Domains are designated as in the Pfam database (Amidase_3, PF01520; CHAP, PF05257; Glucosaminidase, PF01832; Lactamase_B, PF00753; Peptidase_C39_2, PF13529). The CBD consists of a variable number of repeats, each about 20-amino acid residues long (CW_binding_1, PF01473). SP, signal peptide.
In agreement with the probable presence of PCho-containing TAs in the Gemella cell wall, up to six potential CBPs could be found (Figure 5B). Taking into account the high similarities between these CBPs and those of S. pneumoniae, a variety of functions could be attributed to some of those proteins (see Galán-Bartual et al., 2015 for a review): (1) LytB-like glucosaminidases; (2) N-acetylmuramoyl-L-alanine amidases and/or cysteine, histidine-dependent amidohydrolases/peptidases; (3) peptidases; (4) PCho esterases with a metallo-β-lactamase protein fold; and (5) other CBPs that lack any other domains and that can be involved in structural and/or regulatory functions, as described for the pneumococcal CbpF protein. Of note, the domain CHAP-containing (Cysteine, Histidine Amidohydrolase/Peptidase) protein WP_111743015.1 (Figure 5B) most probably corresponds to the endolysin encoded by a putative prophage present in G. morbillorumT (data not shown). Curiously, in all but two cases in Figure 5B, the CBD constituted by the Cho-binding repeats (CBRs), was most similar to those found in Granulicatella proteins (40–59% identity). This suggests a distinct origin for the choline metabolism and the CBD itself. In this respect, it is also worth mentioning that the majority of the CBRs found in the CBPs of Gemella spp. does not follow the consensus sequence of most pneumococcal CBPs: GWxK-X4–5-WYY-φ-x3–5GxMx2–3, where x is any residue and φ is hydrophobic (Galán-Bartual et al., 2015). Instead, the CBRs of Gemellae consist of a series of long and short repeats that are strikingly similar to those reported only in CbpL, a CBP with relevance in pneumococcal pathogenesis (Gutiérrez-Fernández et al., 2016) (Supplementary Figure S2).
Toxins
Homologs of pneumolysin (Ply) – a toxin with β-hemolysin activity under anaerobic conditions and that is central in pneumococcal virulence –, were found. Of note, the potential Gemella cytolysin is 55% identical (75% similar) to S. pneumoniae Ply, but contains a putative signal peptide that is always absent in Ply (data not shown). However, pneumolysin homologs were observed only in G. bergeri (WP_062172157, WP_021753470) and G. cuniculi (WP_027130155), which suggests that these toxins do not play an important role in most Gemella-caused diseases. Although weak β-hemolysis observed in G. haemolysans has been proposed as a criterion for differentiating this species from G. morbillorum (Berger and Pervanidis, 1986), no genetic determinant that justify such meta-phenotype has been identified so far. In any case, it should be noted that some strains of Gemellae are β-hemolytic; for example some strains of G. bergeri (three of six) were hemolytic on horse blood agar (Collins et al., 1998). On trypticase soy sheep blood agar, most strains are α-or non-hemolytic. It is well recognized that the expression of β-hemolysis in Gemellae depends on the choice of blood and agar base (Collins and Falsen, 2015).
Recognition of Receptors and Extracellular Matrix Components by Adhesins
Gram-positive pathogens rely on exposed functional elements in microbial surface component recognizing adhesive matrix molecules (MSCRAMMs), as those exposed in damaged/inflamed tissues of heart valves (Fayet et al., 2007; Mahler and Butcher, 2011), and receptors.
As is the case for the fibronectin-binding protein PavA (Pneumococcal adherence and virulence factor A) of streptococci, all Gemella isolates contained polytopic proteins with an FbpA (Fibronectin binding protein A) domain plus a DUF814 (currently redefined as “NFACT_RNA-bind” domain). PavA is one of the three virulence proteins that are universally present in a set of 40 S. mitis and Streptococcus oralis endocarditis isolates, suggesting a prominent role in this disease (Rasmussen et al., 2017). The universal pathogenic involvement of this homolog has been tested in Streptococcus anginosus, which harbors a gene (fbp62) encoding a PavA ortholog. Notably, a Δfbp62 mutant of S. anginosus showed a reduced ability to form abscesses in an animal model of infection (Kodama et al., 2018). It should be noted, however, that Gemella homologs of PavA share 40–41% identity to those of Bacillus species like Bacillus circulans, a microorganism also able to cause endocarditis (Krause et al., 1999).
In contrast, many MSCRAMMs arise after shuffling of functional domains. Among them, some proteins from several Gemella isolates contained distinct combinations of Cna-B (Collagen adhesin B, Pfam Accession: PF05738), FctA (Fibronectin and collagen binding, and T antigen protein, PF12892) and/or collagen_bind (PF05737) domains, which have been associated to fibronectin and collagen-binding in Staphylococcus aureus (Symersky et al., 1997; Deivanayagam et al., 2000). However, we found several differences between the proteins from pathogenic and low-pathogenic taxons (Table 1). First, the exclusive FctA–collagen_bind combination are in the species core of G. morbillorum and in the G. haemolysans M341 isolate, which in both cases have a very likely S. oralis origin (69% identity, whole length). When combined with CnaB, the collagen_bind domain shows distinct putative origins for G. morbillorum (Parvimonas and Mogibacterium, 48% identity), and G. sanguinis (Streptococcus parasanguinis, 32% identity) compared to isolates of the low-pathogenic G. bergeri group (Streptococcus intermedius, 46–48% identity). This pattern strongly suggests that this domain was introduced into the Gemella genus by independent events and, probably, show different ligand affinities and biological contexts.
Several Gemella proteins also contained one or two SSURE (Streptococcal SUrface REpeat) motifs (sharing in all cases 86–87% identity to repeats of S. mitis proteins). Such repeats are also linked to fibronectin and plasminogen-binding in the PavB (Pneumococcal adherence and virulence factor B) pneumococcal protein (Jensch et al., 2010). Only one Gemella protein, that of G. haemolysans DNF01167, contained a von Willebrand factor type A (vWFA_2) domain (sharing 33–37% identity to protein sections of oral Olsenella, Selenomonas and Parvimonas). The vWF is a huge multimeric human protein that triggers platelet adhesion in areas of vascular damage (Shahidi, 2017), which may favor endocarditis when present in pathogens due to platelet and fibronectin recruitment.
Other proteins appear to be involved in adhesion in a matrix-independent manner. PsaA (Pneumococcal surface adhesin A), a lipoprotein involved in cellular adhesion via E-cadherin (Anderton et al., 2007), and manganese transport (Dintilhac et al., 1997) in S. pneumoniae was one of the VFDB members detected in all Gemellae. PsaA, which is a member of a Gram-positive adhesin family associated to saliva binding (Ganeshkumar et al., 1993) and endocarditis (Lowe et al., 1995), encompasses several homologs in Gram-positive pathogens (Papp-Wallace and Maguire, 2006). A phylogenetic analysis showed that the Gemella PsaA homolog is a member of the SsaB/ScaA subfamily of proteins of S. gordonii and S. sanguinis with which it shares an outstanding identity (82–83%) (Supplementary Figure S3A). Notably, these two viridans streptococci are major causative agents of endocarditis from oral origin (Vogkou et al., 2016) and, therefore, this protein may play a highly selective dual role in the oral and cardiac environments.
While proteins containing one or two MucBP (Mucin Binding Protein) repeats, which participate in the colonization of the upper respiratory tract (Du et al., 2011), were found in isolates of all Gemella groups, the number of such repeats is much higher, up to 17, in G. haemolysans (showing 86% identity with a S. mitis protein). Besides that, proteins with Rib (Resistance to proteases, immunity, group B) repeats, involved in epithelial adhesion in the main virulence factor of Streptococcus agalactiae (Baron et al., 2004), were exclusive from the core species proteomes of pathogenic Gemella. In G. morbillorum, these proteins carried only 2–3 Rib repeats (35–41% identity to Lactobacillus and Aerococcus proteins). In contrast, G. haemolysans proteins showed up to 8 Rib repeats and were part of six isolate-specific independent domain architectures, all related to S. mitis proteins (60–80% identity). Tentatively, MucBP and Rib G. haemolysans proteins may require more repeats than their G. morbillorum counterparts due to the need to cross the polysaccharide capsule.
Proteases
Surface proteolytic domains also play key roles in pathogenesis by inactivating host immune components and proteolysis of host tissues during invasion. Some of the Gemella proteins with confident homologs in the VFDB content were proteases, such as IgA (Immunoglobulin A protease) and ZmpC, whose presence in Gemella had been already reported. The member IgA1 of the Zmp (Zinc metalloproteinase) superfamily is able to cleave human immunoglobulin A at the hinge region, thereby eliminating an important aspect of host defense at mucosal sites (Mistry and Stockley, 2006). Despite the Zmp superfamily owns complex architectures, homologs were detected in Gemellae by Pfam analysis (Peptidase_M26_N and C domains) and relaxed BLAST search thresholds to S. pneumoniae representative sequences. With the exception of ZmpB, G. haemolysans isolates carried fully Zmp complements (IgA, ZmpC and ZmpD) where IgA may have a S. mitis origin (64% identity). In contrast, G. morbillorum and G. sanguinis only harbored ZmpB, and G. bergeri lacked any Zmp homolog (Supplementary Figure S3B). Unfortunately, the relevance of these differences can only be speculated since the substrates of ZmpB/C/D are still unknown despite its recognized association with invasive disease (Bek-Thomsen et al., 2012).
Another important family of proteases present in Gemella spp. was ClpP (Caseinolytic protease Proteolytic subunit), showing 82% identity to Staphylococcus homologs (Supplementary Figure S3C). ClpP is involved in many proteolytic processes and related to bacterial virulence (Bhandari et al., 2018). Finally, the peptidase_S8 and Fn3_5 domain fusion was found in proteins of G. haemolysans, G. morbillorum and G. sanguinis (Table 1). Comparable architectures have been found in ScpC (Streptococcal chemokine protease C) homologs of S. agalactiae and Streptococcus pyogenes, playing inflammation control, colonization and invasion roles in these bacteria (Hidalgo-Grass et al., 2006). This peptidase is critically involved in soft tissue infection by degrading the complement C5a component and IL-8, two important factors for neutrophil recruitment (Sjolinder et al., 2008). The bacterium with the most confident hits for the three species (51–63% identities) was S. gordonii.
Metal Cofactor Uptake
Manganese and iron are essential cofactors for enzymes of pathogens, that are scarce or in complexed forms in the host. Therefore, pathogens must acquire them during colonization and infection by specialized surface proteins. Among them, the aforementioned PsaA adhesin is also a Mn2+ transport important to face the oxidative environment of the upper respiratory and digestive tracts. In addition, “Near iron transporter” (NEAT) domains are able to capture heme-related molecules as a putative source of iron during invasive disease (Sheldon and Heinrichs, 2015) (Table 1). G. haemolysans and G. morbillorum isolates carry two or more long surface proteins with a NEAT domain. Moreover, one of these proteins in all G. haemolysans isolates harbor a protein with the “LRR_5 – NEAT” alternative architecture, also observed in other pathogens as Bacillus cereus (Daou et al., 2009). The G. haemolysans NEAT domain shares 63% identity to protein domains of Peptostreptococcus anaerobius, another commensal bacterium causing endocarditis (Cone et al., 2003). Instead, the G. morbillorum NEAT repeats share 49% identity to Peptoniphilus sp. proteins, a gut species that also causes blood stream infections (Brown et al., 2014), again suggesting distinct acquisition events and adaptive pressure for protein virulence determinants in Gemella even when playing comparable functions.
Absence of Complete Competence Gene Complements in Gemella sp.
The puzzling pathogenomic patterns of Gemella isolates described above strongly suggests that many virulence factors were acquired by Gemella isolates through HGT events instead of by vertical – i.e., speciation – inheritance. One of the commonest HGT mechanisms in Gram-positive bacteria is natural genetic transformation, with B. subtilis and S. pneumoniae being the two paradigms (Claverys et al., 2009). However, all Gemella spp. analyzed only contained close homologs to the central DNA repair proteins RecA, RadA, RnjA, and Ssb of both B. subtilis and S. pneumoniae (Supplementary Table S9). Therefore, Gemella seems to have tools involved in DNA recombination although by induction and uptake mechanisms independent from the current knowledge acquired from the two Gram-positive competence paradigms. This is not fully surprising since competence, in contrast to capsular polysaccharide, PCho acquisition and other virulence factors, is an extraordinarily specific and complex issue, where the genes involved show strong species-specific sequence divergence.
Discussion
In this study, the Gemella genome sequences have been analyzed through the lens of our current understanding of molecular pathobiology of Gram-positive pathogens. Beyond its unquestionable utility, general virulence databases may be limited as a unique strategy to cover the full pathogenicity of new species, even when related to primary pathogens. As judging from genomic data, Gemellae appear to have a high capacity to incorporate exogenous DNA probably by, given the apparent absence of competence genes, conjugation or phage transduction. These HGT pathways may be followed by adaption to lifestyle by extensive recombination and/or mutation, which may account for the observed inter-species clinical differences. A salient example is the presence of a S. pneumoniae-like capsule in the “Haemolysans group” isolates. Since S. pneumoniae is the streptococcus with the highest invasive potential and that CPS is considered a central factor in such phenotype (Kadioglu and Andrew, 2004), this feature may be one of the factors that increase the potential of the “Haemolysans group” to cause this sort of diseases. Moreover, the gene content divergence of the capsular clusters in this taxon would very likely produce CPSs with diverse thickness and compositions. These findings suggest adaptive events leading to the existence of serotypes that might eventually circumvent cross-protection in hosts previously infected with other G. haemolysans isolates.
Several exposed proteins bind to the host extracellular matrix in the principal Streptococcus and Staphylococcus pathogenic species during infection (Hammerschmidt et al., 2019). These are also essential factors for binding to oral surfaces of viridans streptococci (Jenkinson and Lamont, 2005). Equivalents to these determinants, proteins with potential for binding to collagen, fibronectin and/or plasminogen were also found in Gemella spp. While there are virulence factors common to all Gemellae, e.g., the lipoprotein PsaA, exclusive surface protein pools of G. haemolysans and G. morbillorum – including adhesive, evasive and nutrient scavenging factors – may be responsible for their higher pathogenic potential. Such combination of exclusive factors may favor the colonization and biofilm formation in damaged native valves by recognizing the exposed extracellular matrix components (Fayet et al., 2007), a process leading to endocarditis.
In contrast to G. haemolysans, G. morbillorum appears to be unable to synthesize CPS. Nevertheless, this feature may enable a different and higher exposure of their proteinaceous surface arsenal to compensate the decrease in antiphagocytic protection provided by the capsule during invasive disease. It is tempting to speculate that this condition might favor some pathogenic outcomes, such as liver abscesses, whereas it may disfavor other, such as meningitis. For example, the exact “collagen_bind – [Cna-B]3 – gram_pos_anchor” domain combination was only found in G. morbillorum M424. Importantly, this architecture is identical to the one from the Pil1 pilus subunit of Streptococcus gallolyticus subsp. gallolyticus, the etiological agent of 10–15% of all infective endocarditis (Liesman et al., 2017). Like G. morbillorum, this streptococcus is present in the digestive tract and the reported endocarditis cases have been associated with colon cancer (Lopez-Dupla et al., 1996; FitzGerald et al., 2006; Beck et al., 2008). This G. morbillorum protein may therefore exhibit the same capital functions of Pil1 such as recognition of collagen type I in heart valves to create biofilms (Danne et al., 2011; Boleij and Tjalsma, 2013) and activation of the contact system triggering the coagulation cascade (Isenring et al., 2018). G. morbillorum and S. gallolyticus subsp. gallolyticus may therefore have undergone convergent evolutionary processes into comparable pathophenotypes.
Technical advances have permitted the routine identification of bacteria previously assumed to be mere commensals as the etiological agents of infections. A vast majority of these cases are produced in the human population under a pregnant, dental surgery or the chronic immunocompromised status. The term “pathobiont” has been coined to cover microorganisms that can show either pathogenic or commensal behaviors as a result of complex bacteria-host interactions. Gemella appears as an ideal model to study this fine equilibrium since it exemplifies the case of a bacterium with a mild virulence gene pool that, while not as large as that of primary pathogens, may lead to a recurrent track of diverse cases. Several facts displace this balance from harmless to pathogenic. On the pathogen’s side, Gemella genomes can steadily aggregate virulence determinants for persistence and virulence to convert into lineages found to have more intrinsic chances to cause disease and disseminate, as previously reported (Cerdá Zolezzi et al., 2007). On the host’s side, alterations such as the exposure of extracellular matrix molecules in damaged native valves, the implant of artificial heart valves and neutrophil depletion are, in most cases, a prerequisite to ease the way for Gemella infection. Experimental options to study these aspects would be the utilization of bacterial knockouts in cellular models of human endocardial endothelium (Bao et al., 2017), rat models of endocarditis (Santoro and Levison, 1978) and anti-Ly6G monoclonal antibody to induce neutrophil depletion in mouse models of sepsis (Visan et al., 2018). VFs detected in this study apparently suffice to establish some genomic trends underlying Gemella virulence. However, the availability of genome sequences of more Gemella isolates from pathogenic and microbiota sources would be necessary to ascertain this proposal. In this respect, isolates from other species with reported virulence potential such as G. palaticanis, G. parahaemolysans, and G. taiwanensis should be included to complete the genomic view of the genus.
Given that only a small fraction of Gemella isolates cause disease, what is the nature of the selective pressure that make virulence factors persist under non-invasive conditions? Around two thirds of VF genes are also found in bacteria not considered genuine pathogens (Niu et al., 2013). This indicates that genes possibly contribute to a different kind of host relationship when found in other genomic contexts. A plausible answer to this question might be that Gemella factors play distinct biological roles in the oral and other environments that reconcile, respectively, commensal and pathogenic lifestyles. By doing so, the same gene products (e.g., adhesins) that are maintained in genomes of these microorganisms to neutralize the shearing forces in the harassing environment of the oral cavity (Jenkinson and Lamont, 2005) may be recalled during invasive stages in the eventuality the bacteria have to counteract the bloodstream to colonize the valve endocardium and, probably, other niches. In fact, Gemellae and viridans streptococci are the oral bacteria most associated with the mucosa fraction respect to the saliva fraction (Diaz et al., 2012), and these two groups are commonly linked to opportunistic endocarditis despite their evolution to commensality involves the absence of many VFs observed in common pathogens (Kilian et al., 2014). As a corollary, evidences point toward adaptation to the oral cavity involves pre-adaption to endocarditis (Brouqui and Raoult, 2001).
The capacity of Gemellae to acquire (and potentially to donate) exogenous DNA encoding VFs from a number of sources implies that Gemella may also act as a repository of virulence determinants, as also suggested for antibiotic resistance factors (Cerdá Zolezzi et al., 2007). Gemella and Streptococcus show ecological – even with physical contact inside biofilms (Mira, 2008) – and genetic proximity, which would facilitate DNA exchange and its conservation given that the selective forces are similar. Notably, such gene transfer appears to involve also other commensal oral genera from different taxonomic families with a comparable virulence pattern to Gemella, such as Abiotrophia, Granulicatella, and Parvimonas (Ohara-Nemoto et al., 2005; Baghban and Gupta, 2016; Chowdhury and German, 2018). Altogether, data suggest the existence of a gene pool in the oral bacterial environment permitting these species to increase their abilities to survive and cause invasive diseases such as endocarditis, sepsis, abscesses and/or joint infections.
Caution should be maintained when genomic information is translated into the clinical context, in particular when sequenced isolates are not the same as those causing the reported infections. Nevertheless, many of the activities associated with virulence detected in Gemella are common to the whole genus or species, and compatible with similar diseases caused by well-studied pathogens of other Gram-positive genera such as Streptococcus. The analysis of the VF pool of these species may find its application in the clinical setting by inter-species vaccine development (Mira, 2008) and the high-precision prognosis of opportunistic infections. Besides, because of the similarities between the VFs of Gemellae and other pathogens, the real impact of the diseases caused by Gemella sps. might be potentially overestimated. In addition, recent molecular and culture-independent methods have revealed that some infections reported to be caused by Gemellae (or by many other bacteria) are currently recognized as polymicrobial in nature (García-Lechuz et al., 2002; Imirzalioglu et al., 2014; Yamagishi et al., 2018). This is particularly true in environments like the nasopharynx (Mittal et al., 2019).
As already mentioned, identification of Gemella isolates in the clinical laboratory has been routinely carried out using MALDI-TOF MS (Schulthess et al., 2013), 16S RNA sequencing (Woo et al., 2003), API® ID systems (BioMérieux, France) (Milnik et al., 2013) and/or pulsed-field gel electrophoresis (Cerdá Zolezzi et al., 2007). However, the accuracy of these techniques can be currently outperformed, in a cost-effective manner, by whole genome analysis. For example, 16S rRNA analysis failed to reveal the relative divergence within the “Haemolysans” group by its lower resolution and, by design, the explicit identification of the virulence pool. This study aims to lay the foundations for predicting the chances of commuting from commensal to pathogen, in particular for G. haemolysans and G. morbillorum, which represent together ca. 95% of clinical cases. Moreover, when combined with clinical metadata of the patient – concerning the cardiac, immunological and metabolic status – the genomic information of the isolate would permit the rationalization of the disease from a double, host and pathogen, perspective. This valuable information would assist clinical management of these infections with an unprecedented personalized precision in the near future.
Data Availability Statement
All datasets generated for this study are included in the article/Supplementary Material.
Author Contributions
EG and AM-G designed the study, conducted the analyses, and wrote the manuscript.
Conflict of Interest
The authors declare that the research was conducted in the absence of any commercial or financial relationships that could be construed as a potential conflict of interest.
Acknowledgments
We thank Alex Mira (FISABIO Institute) and Sylvia Valdezate (ISCIII) for carefully revising the article and suggestions. This research was supported by grants MPY 509/19 from the Instituto de Salud Carlos III (ISCIII) and SAF2017-88664-R from the Spanish Ministerio de Economía, Industria y Competitividad (MEICOM). The Centro de Investigación Biomédica en Red de Enfermedades Respiratorias (CIBERES) is an initiative of the ISCIII. AM-G is the recipient of a Miguel Servet contract by the ISCIII.
Supplementary Material
The Supplementary Material for this article can be found online at: https://www.frontiersin.org/articles/10.3389/fmicb.2020.00524/full#supplementary-material
References
Abranches, J., Zeng, L., Kajfasz, J. K., Palmer, S. R., Chakraborty, B., Wen, Z. T., et al. (2018). Biology of oral streptococci. Microbiol. Spectr. 6:GPP3-0042-2018. doi: 10.1128/microbiolspec.GPP3-0042-2018
Anderton, J. M., Rajam, G., Romero-Steiner, S., Summer, S., Kowalczyk, A. P., Carlone, G. M., et al. (2007). E-cadherin is a receptor for the common protein pneumococcal surface adhesin A (PsaA) of Streptococcus pneumoniae. Microb. Pathog. 42, 225–236. doi: 10.1016/j.micpath.2007.02.003
Baghban, A., and Gupta, S. (2016). Parvimonas micra: a rare cause of native joint septic arthritis. Anaerobe 39, 26–27. doi: 10.1016/j.anaerobe.2016.02.004
Bai, X.-H., Chen, H.-J., Jiang, Y.-L., Wen, Z., Huang, Y., Cheng, W., et al. (2014). Structure of pneumococcal peptidoglycan hydrolase LytB reveals insights into the bacterial cell wall remodeling and pathogenesis. J. Biol. Chem. 289, 23403–23416. doi: 10.1074/jbc.M114.579714
Bao, X., Bhute, V. J., Han, T., Qian, T., Lian, X., and Palecek, S. P. (2017). Human pluripotent stem cell-derived epicardial progenitors can differentiate to endocardial-like endothelial cells. Bioeng. Transl. Med. 2, 191–201. doi: 10.1002/btm2.10062
Baron, M. J., Bolduc, G. R., Goldberg, M. B., Auperin, T. C., and Madoff, L. C. (2004). Alpha C protein of group B Streptococcus binds host cell surface glycosaminoglycan and enters cells by an actin-dependent mechanism. J. Biol. Chem. 279, 24714–24723. doi: 10.1074/jbc.M402164200
Beck, M., Frodl, R., and Funke, G. (2008). Comprehensive study of strains previously designated Streptococcus bovis consecutively isolated from human blood cultures and emended description of Streptococcus gallolyticus and Streptococcus infantarius subsp. coli. J. Clin. Microbiol. 46, 2966–2972. doi: 10.1128/JCM.00078-08
Bek-Thomsen, M., Poulsen, K., and Kilian, M. (2012). Occurrence and evolution of the paralogous zinc metalloproteases IgA1 protease, ZmpB, ZmpC, and ZmpD in Streptococcus pneumoniae and related commensal species. mBio 3:e00303-12. doi: 10.1128/mBio.00303-12
Bentley, S. D., Aanensen, D. M., Mavroidi, A., Saunders, D., Rabbinowitsch, E., Collins, M., et al. (2006). Genetic analysis of the capsular biosynthetic locus from all 90 pneumococcal serotypes. PLoS Genet. 2:e31. doi: 10.1371/journal.pgen.0020031
Berger, U., and Pervanidis, A. (1986). Differentiation of Gemella haemolysans (Thjøtta and Bøe 1938) Berger 1960, from Streptococcus morbillorum (Prevot 1933) Holdeman and Moore 1974. Zentralbl. Bakteriol. Mikrobiol. Hyg. A 261, 311–321. doi: 10.1016/s0176-6724(86)80048-7
Bhandari, V., Wong, K. S., Zhou, J. L., Mabanglo, M. F., Batey, R. A., and Houry, W. A. (2018). The role of ClpP protease in bacterial pathogenesis and human diseases. ACS Chem. Biol. 13, 1413–1425. doi: 10.1021/acschembio.8b00124
Boix-Amorós, A., Collado, M. C., and Mira, A. (2016). Relationship between milk microbiota, bacterial load, macronutrients, and human cells during lactation. Front. Microbiol. 7:492. doi: 10.3389/fmicb.2016.00492
Boleij, A., and Tjalsma, H. (2013). The itinerary of Streptococcus gallolyticus infection in patients with colonic malignant disease. Lancet Infect. Dis. 13, 719–724. doi: 10.1016/S1473-3099(13)70107-5
Brouqui, P., and Raoult, D. (2001). Endocarditis due to rare and fastidious bacteria. Clin. Microbiol. Rev. 14, 177–207. doi: 10.1128/cmr.14.1.177-207.2001
Brown, K., Church, D., Lynch, T., and Gregson, D. (2014). Bloodstream infections due to Peptoniphilus spp.: report of 15 cases. Clin. Microbiol. Infect. 20, O857–O860. doi: 10.1111/1469-0691.12657
Brown, S. P., Cornforth, D. M., and Mideo, N. (2012). Evolution of virulence in opportunistic pathogens: generalism, plasticity, and control. Trends Microbiol. 20, 336–342. doi: 10.1016/j.tim.2012.04.005
Cerdá Zolezzi, P., Goñi Cepero, P., Ruiz, J., Millán Laplana, L., Rubio Calvo, C., and Gómez-Lus, R. (2007). Molecular epidemiology of macrolide and tetracycline resistances in commensal Gemella sp. isolates. Antimicrob. Agents Chemother. 51, 1487–1490. doi: 10.1128/AAC.01374-06
Chowdhury, S., and German, M. L. (2018). Rare but not infrequent: infective endocarditis caused by Abiotrophia defectiva. Case Rep. Infect. Dis. 2018:5186520. doi: 10.1155/2018/5186520
Christensen, J. J., and Ruoff, K. L. (2015). “Aerococcus, Abiotrophia, and other aerobic catalase-negative, Gram-positive cocci,” in Manual of Clinical Microbiology, 11th Edn, eds J. Jorgensen, M. Pfaller, K. Carroll, G. Funke, M. Landry, S. Richter, et al. (Washington, DC: ASM Press), 422–436. doi: 10.1128/9781555817381.ch24
Claverys, J.-P., Martin, B., and Polard, P. (2009). The genetic transformation machinery: composition, localization, and mechanism. FEMS Microbiol. Rev. 33, 643–656. doi: 10.1111/j.1574-6976.2009.00164.x
Collins, M., and Falsen, E. (2015). “Gemella,” in Bergey’s Manual of Systematics of Archaea and Bacteria, eds W. B. Whitman, F. Rainey, P. Kämpfer, M. Trujillo, J. Chun, P. DeVos, et al. (Hoboken, NJ: John Wiley & Sons, Inc., in association with Bergey’s Manual Trust), 511–518. doi: 10.1002/9781118960608.gbm00578
Collins, M. D., Hutson, R. A., Falsen, E., Sjöden, B., and Facklam, R. R. (1998). Gemella bergeriae sp. nov., isolated from human clinical specimens. J. Clin. Microbiol 36, 1290–1293. doi: 10.1128/jcm.36.5.1290-1293.1998
Cone, L. A., Battista, B. A., and Shaeffer, C. W. J. (2003). Endocarditis due to Peptostreptococcus anaerobius: case report and literature review of peptostreptococcal endocarditis. J. Heart Valve Dis. 12, 411–413.
Corsini, B., Aguinagalde, L., Ruiz, S., Domenech, M., Antequera, M. L., Fenoll, A., et al. (2016). Immunization with LytB protein of Streptococcus pneumoniae activates complement-mediated phagocytosis and induces protection against pneumonia and sepsis. Vaccine 34, 6148–6157. doi: 10.1016/j.vaccine.2016.11.001
Danne, C., Entenza, J. M., Mallet, A., Briandet, R., Debarbouille, M., Nato, F., et al. (2011). Molecular characterization of a Streptococcus gallolyticus genomic island encoding a pilus involved in endocarditis. J. Infect. Dis. 204, 1960–1970. doi: 10.1093/infdis/jir666
Daou, N., Buisson, C., Gohar, M., Vidic, J., Bierne, H., Kallassy, M., et al. (2009). IlsA, a unique surface protein of Bacillus cereus required for iron acquisition from heme, hemoglobin and ferritin. PLoS Pathog. 5:e1000675. doi: 10.1371/journal.ppat.1000675
de Castro, E., Sigrist, C. J. A., Gattiker, A., Bulliard, V., Langendijk-Genevaux, P. S., Gasteiger, E., et al. (2006). ScanProsite: detection of PROSITE signature matches and ProRule-associated functional and structural residues in proteins. Nucleic Acids Res. 34, W362–W365. doi: 10.1093/nar/gkl124
Deivanayagam, C. C. S., Rich, R. L., Carson, M., Owens, R. T., Danthuluri, S., Bice, T., et al. (2000). Novel fold and assembly of the repetitive B region of the Staphylococcus aureus collagen-binding surface protein. Structure 8, 67–78. doi: 10.1016/S0969-2126(00)00081-2
Deshmukh, D., Joseph, J., Chakrabarti, M., Sharma, S., Jayasudha, R., Sama, K. C., et al. (2019). New insights into culture negative endophthalmitis by unbiased next generation sequencing. Sci. Rep. 9:844. doi: 10.1038/s41598-018-37502-w
Diaz, P. I., Dupuy, A. K., Abusleme, L., Reese, B., Obergfell, C., Choquette, L., et al. (2012). Using high throughput sequencing to explore the biodiversity in oral bacterial communities. Mol. Oral Microbiol. 27, 182–201. doi: 10.1111/j.2041-1014.2012.00642.x
Dintilhac, A., Alloing, G., Granadel, C., and Claverys, J. P. (1997). Competence and virulence of Streptococcus pneumoniae: Adc and PsaA mutants exhibit a requirement for Zn and Mn resulting from inactivation of putative ABC metal permeases. Mol. Microbiol. 25, 727–739. doi: 10.1046/j.1365-2958.1997.5111879.x
Dramsi, S., and Bierne, H. (2017). Spatial organization of cell wall-anchored proteins at the surface of Gram-positive bacteria. Curr. Top Microbiol. Immunol. 404, 177–201. doi: 10.1007/82_2016_4
Du, Y., He, Y.-X., Zhang, Z.-Y., Yang, Y.-H., Shi, W.-W., Frolet, C., et al. (2011). Crystal structure of the mucin-binding domain of Spr1345 from Streptococcus pneumoniae. J. Struct. Biol. 174, 252–257. doi: 10.1016/j.jsb.2010.10.016
Dzidic, M., Abrahamsson, T. R., Artacho, A., Collado, M. C., Mira, A., and Jenmalm, M. C. (2018). Oral microbiota maturation during the first 7 years of life in relation to allergy development. Allergy 73, 2000–2011. doi: 10.1111/all.13449
Eaton, V. E., Pettit, S., Elkinson, A., Houseknecht, K. L., King, T. E., and May, M. (2019). Polymicrobial abscess following ovariectomy in a mouse. BMC Vet. Res. 15:364. doi: 10.1186/s12917-019-2125-0
El-Gebali, S., Mistry, J., Bateman, A., Eddy, S. R., Luciani, A., Potter, S. C., et al. (2019). The Pfam protein families database in 2019. Nucleic Acids Res. 47, D427–D432. doi: 10.1093/nar/gky995
Esposito, S., and Principi, N. (2018). Impact of nasopharyngeal microbiota on the development of respiratory tract diseases. Eur. J. Clin. Microbiol. Infect. Dis. 37, 1–7. doi: 10.1007/s10096-017-3076-7
Facklam, R., and Elliott, J. A. (1995). Identification, classification, and clinical relevance of catalase-negative, gram-positive cocci, excluding the streptococci and enterococci. Clin. Microbiol. Rev. 8, 479–495. doi: 10.1128/cmr.8.4.479
Fayet, C., Bendeck, M. P., and Gotlieb, A. I. (2007). Cardiac valve interstitial cells secrete fibronectin and form fibrillar adhesions in response to injury. Cardiovasc. Pathol. 16, 203–211. doi: 10.1016/j.carpath.2007.02.008
FitzGerald, S. F., Moloney, A. C., Maurer, B. J., and Hall, W. W. (2006). Gemella endocarditis: consider the colon. J. Heart Valve Dis. 15, 833–835.
Fonkou, M. D. M., Bilen, M., Cadoret, F., Fournier, P.-E., Dubourg, G., and Raoult, D. (2018). Enterococcus timonensis’ sp. nov., ‘Actinomyces marseillensis’ sp. nov., ‘Leptotrichia massiliensis’ sp. nov., ‘Actinomyces pacaensis’ sp. nov., ‘Actinomyces oralis’ sp. nov., ‘Actinomyces culturomici’ sp. nov. and ‘Gemella massiliensis’ sp. nov., new bacterial species isolated from the human respiratory microbiome. New Microbes New Infect. 22, 37–43. doi: 10.1016/j.nmni.2017.12.005
Fu, L., Niu, B., Zhu, Z., Wu, S., and Li, W. (2012). CD-HIT: accelerated for clustering the next-generation sequencing data. Bioinformatics 28, 3150–3152. doi: 10.1093/bioinformatics/bts565
Galán-Bartual, S., Pérez-Dorado, I., García, P., and Hermoso, J. A. (2015). “Structure and functions of choline binding proteins,” in Streptococcus Pneumoniae. Molecular Mechanisms of Host-Pathogen Interactions, eds J. Brown, S. Hammerschimdt, and C. Orihuela (Amsterdam: Academic Press), 207–230. doi: 10.1016/B978-0-12-410530-0.00011-9
Ganeshkumar, N., Arora, N., and Kolenbrander, P. E. (1993). Saliva-binding protein (SsaB) from Streptococcus sanguis 12 is a lipoprotein. J. Bacteriol. 175, 572–574. doi: 10.1128/jb.175.2.572-574.1993
García-Lechuz, J. M., Cuevas-Lobato, O., Hernángomez, S., Hermida, A., Guinea, J., Marín, M., et al. (2002). Extra-abdominal infections attributable to Gemella species. Int. J. Infect. Dis 6, 78–82. doi: 10.1016/S1201-9712(02)90142-6
Gillespie, S. H., Ainscough, S., Dickens, A., and Lewin, J. (1996). Phosphorylcholine-containing antigens in bacteria from the mouth and respiratory tract. J. Med. Microbiol. 44, 35–40. doi: 10.1099/00222615-44-1-35
Gutiérrez-Fernández, J., Saleh, M., Alcorlo, M., Gómez-Mejía, A., Pantoja-Uceda, D., Treviño, M. A., et al. (2016). Modular architecture and unique teichoic acid recognition features of choline-binding protein L (CbpL) contributing to pneumococcal pathogenesis. Sci. Rep. 6:38094. doi: 10.1038/srep38094
Hammerschmidt, S., Rohde, M., and Preissner, K. T. (2019). “Extracellular matrix interactions with gram-positive pathogens,” in Gram-Positive Pathogens: Microbiol Spectrum, 3rd Edn, Vol. 7, eds V. Fischetti, R. Novick, J. Ferretti, D. Portnoy, M. Braunstein, and J. Rood (Washington, DC: ASM Press), 108–124. doi: 10.1128/microbiolspec.GPP3-0041-2018
Hayashi Sant’Anna, F., Bach, E., Porto, R. Z., Guella, F., Sant’Anna, E., and Passaglia, L. M. P. (2019). Genomic metrics made easy: what to do and where to go in the new era of bacterial taxonomy. Crit. Rev. Microbiol. 45, 182–200. doi: 10.1080/1040841X.2019.1569587
Hidalgo-Grass, C., Mishalian, I., Dan-Goor, M., Belotserkovsky, I., Eran, Y., Nizet, V., et al. (2006). A streptococcal protease that degrades CXC chemokines and impairs bacterial clearance from infected tissues. EMBO J. 25, 4628–4637. doi: 10.1038/sj.emboj.7601327
Hikone, M., Sakamoto, N., Ota, M., Washino, T., Kobayashi, K., Iwabuchi, S., et al. (2017). The first case report of infective endocarditis caused by Gemella taiwanensis. J. Infect. Chemother. 23, 567–571. doi: 10.1016/j.jiac.2017.02.012
Hoyles, L., Foster, G., Falsen, E., and Collins, M. D. (2000). Characterization of a Gemella-like organism isolated from an abscess of a rabbit: description of Gemella cunicula sp. nov. Int. J. Syst. Evol. Microbiol. 50, 2037–2041. doi: 10.1099/00207713-50-6-2037
Hung, W.-C., Chen, H.-J., Tasai, J.-C., Tseng, S.-P., Lee, T.-F., Hsueh, P.-R., et al. (2014). Gemella parahaemolysans sp. nov. and Gemella taiwanensis sp. nov., isolated from human clinical specimens. Int. J. Syst. Evol. Microbiol. 64, 2060–2065. doi: 10.1099/ijs.0.052795-0
Hung, W.-C., Tseng, S.-P., Chen, H.-J., Tsai, J.-C., Chang, C.-H., Lee, T.-F., et al. (2010). Use of groESL as a target for identification of Abiotrophia, Granulicatella, and Gemella species. J. Clin. Microbiol 48, 3532–3538. doi: 10.1128/jcm.00787-10
Imirzalioglu, C., Sethi, S., Schneider, C., Hain, T., Chakraborty, T., Mayser, P., et al. (2014). Distinct polymicrobial populations in a chronic foot ulcer with implications for diagnostics and anti-infective therapy. BMC Res. Notes 7:196. doi: 10.1186/1756-0500-7-196
Iovino, F., Brouwer, M. C., van de Beek, D., Molema, G., and Bijlsma, J. J. E. (2013). Signalling or binding: the role of the platelet-activating factor receptor in invasive pneumococcal disease. Cell. Microbiol. 15, 870–881. doi: 10.1111/cmi.12129
Isenring, J., Kohler, J., Nakata, M., Frank, M., Jans, C., Renault, P., et al. (2018). Streptococcus gallolyticus subsp. gallolyticus endocarditis isolate interferes with coagulation and activates the contact system. Virulence 9, 248–261. doi: 10.1080/21505594.2017.1393600
Jenkinson, H. F., and Lamont, R. J. (2005). Oral microbial communities in sickness and in health. Trends Microbiol. 13, 589–595. doi: 10.1016/j.tim.2005.09.006
Jensch, I., Gamez, G., Rothe, M., Ebert, S., Fulde, M., Somplatzki, D., et al. (2010). PavB is a surface-exposed adhesin of Streptococcus pneumoniae contributing to nasopharyngeal colonization and airways infections. Mol. Microbiol. 77, 22–43. doi: 10.1111/j.1365-2958.2010.07189.x
Johnson, L. L. (2018). “Design of observational studies,” in Principles and Practice of Clinical Research, 4th Edn, eds J. I. Gallin, F. P. Ognibene, and L. L. Johnson (London: Academic Press), 231(248.
Johnston, C., Hauser, C., Hermans, P. W., Martin, B., Polard, P., Bootsma, H. J., et al. (2016). Fine-tuning of choline metabolism is important for pneumococcal colonization. Mol. Microbiol. 100, 972–988. doi: 10.1111/mmi.13360
Kadioglu, A., and Andrew, P. W. (2004). The innate immune response to pneumococcal lung infection: the untold story. Trends Immunol. 25, 143–149. doi: 10.1016/j.it.2003.12.006
Kilian, M., Riley, D. R., Jensen, A., Bruggemann, H., and Tettelin, H. (2014). Parallel evolution of Streptococcus pneumoniae and Streptococcus mitis to pathogenic and mutualistic lifestyles. mBio 5:e01490-14. doi: 10.1128/mBio.01490-14
Kim, M., Oh, H.-S., Park, S.-C., and Chun, J. (2014). Towards a taxonomic coherence between average nucleotide identity and 16S rRNA gene sequence similarity for species demarcation of prokaryotes. Int. J. Syst. Evol. Microbiol 64, 346–351. doi: 10.1099/ijs.0.059774-0
Kitts, P. A., Church, D. M., Thibaud-Nissen, F., Choi, J., Hem, V., Sapojnikov, V., et al. (2016). Assembly: a resource for assembled genomes at NCBI. Nucleic Acids Res. 44, D73–D80. doi: 10.1093/nar/gkv1226
Kodama, Y., Ishikawa, T., Shimoyama, Y., Sasaki, D., Kimura, S., and Sasaki, M. (2018). The fibronectin-binding protein homologue Fbp62 of Streptococcus anginosus is a potent virulence factor. Microbiol. Immunol. 62, 624–634. doi: 10.1111/1348-0421.12646
Konstantinidis, K. T., and Tiedje, J. M. (2005). Genomic insights that advance the species definition for prokaryotes. Proc. Natl. Acad. Sci. U.S.A. 102, 2567–2572. doi: 10.1073/pnas.0409727102
Kovács, A. T., Smits, W. K., Mirończuk, A. M., and Kuipers, O. P. (2009). Ubiquitous late competence genes in Bacillus species indicate the presence of functional DNA uptake machineries. Environ. Microbiol. 11, 1911–1922. doi: 10.1111/j.1462-2920.2009.01937.x
Krause, A., Gould, F. K., and Forty, J. (1999). Prosthetic heart valve endocarditis caused by Bacillus circulans. J. Infect. 39, 160–162. doi: 10.1016/s0163-4453(99)90011-7
Kumar, S., Stecher, G., Li, M., Knyaz, C., and Tamura, K. (2018). MEGA X: molecular evolutionary genetics analysis across computing platforms. Mol. Biol. Evol. 35, 1547–1549. doi: 10.1093/molbev/msy096
Liesman, R. M., Pritt, B. S., Maleszewski, J. J., and Patel, R. (2017). Laboratory diagnosis of infective endocarditis. J. Clin. Microbiol. 55, 2599–2608. doi: 10.1128/JCM.00635-17
Lindberg, A. A. (1999). Polyosides (encapsulated bacteria). C. R. Acad. Sci. III 322, 925–932. doi: 10.1016/s0764-4469(00)87188-7
Liu, B., Zheng, D., Jin, Q., Chen, L., and Yang, J. (2019). VFDB 2019: a comparative pathogenomic platform with an interactive web interface. Nucleic Acids Res. 47, D687–D692. doi: 10.1093/nar/gky1080
López, R., and García, E. (2004). Recent trends on the molecular biology of pneumococcal capsules, lytic enzymes, and bacteriophage. FEMS Microbiol. Rev. 28, 553–580. doi: 10.1016/j.femsre.2004.05.002
Lopez-Dupla, M., Creus, C., Navarro, O., and Raga, X. (1996). Association of Gemella morbillorum endocarditis with adenomatous polyps and carcinoma of the colon: case report and review. Clin. Infect. Dis. 22, 379–380. doi: 10.1093/clinids/22.2.379
Lowe, A. M., Lambert, P. A., and Smith, A. W. (1995). Cloning of an Enterococcus faecalis endocarditis antigen: homology with adhesins from some oral streptococci. Infect. Immun. 63, 703–706. doi: 10.1128/iai.63.2.703-706.1995
Mahler, G. J., and Butcher, J. T. (2011). Inflammatory regulation of valvular remodeling: the good(?), the bad, and the ugly. Int. J. Inflam. 2011:721419. doi: 10.4061/2011/721419
Milnik, A., Gazis, A., Tammer, I., and Bartels, C. (2013). Immunocompetent young man with cerebral abscess and cortical venous infarction mimicking cerebritis caused by Gemella morbillorum. BMJ Case Rep. 2013:bcr2012007252. doi: 10.1136/bcr-2012-007252
Mira, A. (2008). “Horizontal gene transfer in oral bacteria,” in Molecular Oral Microbiology, ed. A. H. Rogers (Norfolk: Caister Academic Press), 61–81.
Mistry, D., and Stockley, R. A. (2006). IgA1 protease. Int. J. Biochem. Cell Biol. 38, 1244–1248. doi: 10.1016/j.biocel.2005.10.005
Mittal, R., Sanchez-Luege, S. V., Wagner, S. M., Yan, D., and Liu, X. Z. (2019). Recent perspectives on gene-microbe interactions determining predisposition to otitis media. Front. Genet. 10:1230. doi: 10.3389/fgene.2019.01230
Nissen, T., and Wynn, R. (2014a). The history of the case report: a selective review. JRSM Open 5, 1–5. doi: 10.1177/2054270414523410
Nissen, T., and Wynn, R. (2014b). The clinical case report: a review of its merits and limitations. BMC Res. Notes 7:264. doi: 10.1186/1756-0500-7-264
Niu, C., Yu, D., Wang, Y., Ren, H., Jin, Y., Zhou, W., et al. (2013). Common and pathogen-specific virulence factors are different in function and structure. Virulence 4, 473–482. doi: 10.4161/viru.25730
Ohara-Nemoto, Y., Kishi, K., Satho, M., Tajika, S., Sasaki, M., Namioka, A., et al. (2005). Infective endocarditis caused by Granulicatella elegans originating in the oral cavity. J. Clin. Microbiol. 43, 1405–1407. doi: 10.1128/JCM.43.3.1405-1407.2005
Ostrowsky, B. (2007). “Epidemiology of health-associated infections,” in Bennett & Brachman’s Hospital Infections, ed. W. R. Jarvis (Philadelphia, PA: Lippincott Williams & Wilkins), 3–24.
Papp-Wallace, K. M., and Maguire, M. E. (2006). Manganese transport and the role of manganese in virulence. Annu. Rev. Microbiol. 60, 187–209. doi: 10.1146/annurev.micro.60.080805.142149
Parte, A. C. (2018). LPSN – List of prokaryotic names with standing in nomenclature (bacterio.net), 20 years on. Int. J. Syst. Evol. Microbiol. 68, 1825–1829. doi: 10.1099/ijsem.0.002786
Quainoo, S., Coolen, J. P. M., van Hijum, S. A. F. T., Huynen, M. A., Melchers, W. J. G., van Schaik, W., et al. (2017). Whole-genome sequencing of bacterial pathogens: the future of nosocomial outbreak analysis. Clin. Microbiol. Rev. 30, 1015–1063. doi: 10.1128/CMR.00016-17
Quast, C., Pruesse, E., Yilmaz, P., Gerken, J., Schweer, T., Yarza, P., et al. (2013). The SILVA ribosomal RNA gene database project: improved data processing and web-based tools. Nucleic Acids Res. 41, D590–D596. doi: 10.1093/nar/gks1219
Ramos-Sevillano, E., Moscoso, M., García, P., García, E., and Yuste, J. (2011). Nasopharyngeal colonization and invasive disease are enhanced by the cell wall hydrolases LytB and LytC of Streptococcus pneumoniae. PLoS One 6:e23626. doi: 10.1371/journal.pone.0023626
Rasmussen, L. H., Højholt, K., Dargis, R., Christensen, J. J., Skovgaard, O., Justesen, U. S., et al. (2017). In silico assessment of virulence factors in strains of Streptococcus oralis and Streptococcus mitis isolated from patients with infective endocarditis. J. Med. Microbiol. 66, 1316–1323. doi: 10.1099/jmm.0.000573
Reyn, A., Birch-Andersen, A., and Lapage, S. P. (1966). An electron microscope study of thin sections of Haemophilus vaginalis (Gardner and Dukes) and some possibly related species. Can. J. Microbiol. 12, 1125–1136. doi: 10.1139/m66-154
Richter, M., and Roselló-Móra, R. (2009). Shifting the genomic gold standard for the prokaryotic species definition. Proc. Natl. Acad. Sci. U.S.A. 106, 19126–19131. doi: 10.1073/pnas.0906412106
Rohde, M., and Chhatwal, G. S. (2013). Adherence and invasion of streptococci to eukaryotic cells and their role in disease pathogenesis. Curr. Top. Microbiol. Immunol. 368, 83–110. doi: 10.1007/82_2012_281
Ruoff, K. L. (2002). Miscellaneous catalase-negative, gram-positive cocci: emerging opportunists. J. Clin. Microbiol. 40, 1129–1133. doi: 10.1128/jcm.40.4.1129-1133.2002
Santoro, J., and Levison, M. E. (1978). Rat model of experimental endocarditis. Infect. Immun. 19, 915–918. doi: 10.1128/iai.19.3.915-918.1978
Schulthess, B., Brodner, K., Bloemberg, G. V., Zbinden, R., Böttger, E. C., and Hombach, M. (2013). Identification of Gram-positive cocci by use of matrix-assisted laser desorption ionization-time of flight mass spectrometry: comparison of different preparation methods and implementation of a practical algorithm for routine diagnostics. J. Clin. Microbiol. 51, 1834–1840. doi: 10.1128/JCM.02654-12
Seng, P., Abat, C., Rolain, J. M., Colson, P., Lagier, J.-C., Gouriet, F., et al. (2013). Identification of rare pathogenic bacteria in a clinical microbiology laboratory: impact of matrix-assisted laser desorption ionization-time of flight mass spectrometry. J. Clin. Microbiol. 51, 2182–2194. doi: 10.1128/JCM.00492-13
Shahidi, M. (2017). Thrombosis and von Willebrand factor. Adv. Exp. Med. Biol. 906, 285–306. doi: 10.1007/5584_2016_122
Sheldon, J. R., and Heinrichs, D. E. (2015). Recent developments in understanding the iron acquisition strategies of gram positive pathogens. FEMS Microbiol. Rev. 39, 592–630. doi: 10.1093/femsre/fuv009
Sievers, F., and Higgins, D. G. (2018). Clustal Omega for making accurate alignments of many protein sequences. Protein Sci. 27, 135–145. doi: 10.1002/pro.3290
Sjolinder, H., Lovkvist, L., Plant, L., Eriksson, J., Aro, H., Jones, A., et al. (2008). The ScpC protease of Streptococcus pyogenes affects the outcome of sepsis in a murine model. Infect. Immun. 76, 3959–3966. doi: 10.1128/IAI.00128-08
Straume, D., Stamsås, G. A., and Håvarstein, L. S. (2015). Natural transformation and genome evolution in Streptococcus pneumoniae. Infect. Genet. Evol. 33, 371–380. doi: 10.1016/j.meegid.2014.10.020
Symersky, J., Patti, J. M., Carson, M., House-Pompeo, K., Teale, M., Moore, D., et al. (1997). Structure of the collagen-binding domain from a Staphylococcus aureus adhesin. Nat. Struct. Biol. 4, 833–838. doi: 10.1038/nsb1097-833
Takayasu, L., Suda, W., Takanashi, K., Iioka, E., Kurokawa, R., Shindo, C., et al. (2017). Circadian oscillations of microbial and functional composition in the human salivary microbiome. DNA Res. 24, 261–270. doi: 10.1093/dnares/dsx001
Takenouchi-Ohkubo, N., Mortensen, L. M., Drasbek, K. R., Kilian, M., and Poulsen, K. (2006). Horizontal transfer of the immunoglobulin A1 protease gene (iga) from Streptococcus to Gemella haemolysans. Microbiology 152, 2171–2180. doi: 10.1099/mic.0.28801-0
van de Wijgert, J. H. H. M., Borgdorff, H., Verhelst, R., Crucitti, T., Francis, S., Verstraelen, H., et al. (2014). The vaginal microbiota: what have we learned after a decade of molecular characterization? PLoS One 9:e105998. doi: 10.1371/journal.pone.0105998
Villmones, H. C., Haug, E. S., Ulvestad, E., Grude, N., Stenstad, T., Halland, A., et al. (2018). Species level description of the human ileal bacterial microbiota. Sci. Rep. 8:4736. doi: 10.1038/s41598-018-23198-5
Visan, L., Rouleau, N., Proust, E., Peyrot, L., Donadieu, A., and Ochs, M. (2018). Antibodies to PcpA and PhtD protect mice against Streptococcus pneumoniae by a macrophage- and complement-dependent mechanism. Hum. Vaccin. Immunother. 14, 489–494. doi: 10.1080/21645515.2017.1403698
Vogkou, C. T., Vlachogiannis, N. I., Palaiodimos, L., and Kousoulis, A. A. (2016). The causative agents in infective endocarditis: a systematic review comprising 33,214 cases. Eur. J. Clin. Microbiol. Infect. Dis. 35, 1227–1245. doi: 10.1007/s10096-016-2660-6
Welker, M., Van Belkum, A., Girard, V., Charrier, J.-P., and Pincus, D. (2019). An update on the routine application of MALDI-TOF MS in clinical microbiology. Expert Rev. Proteomics 16, 695–710. doi: 10.1080/14789450.2019.1645603
Woo, P. C. Y., Lau, S. K. P., Fung, A. M. Y., Chiu, S. K., Yung, R. W. H., and Yuen, K. Y. (2003). Gemella bacteraemia characterised by 16S ribosomal RNA gene sequencing. J. Clin. Pathol. 56, 690–693. doi: 10.1136/jcp.56.9.690
Yamagishi, T., Hikone, M., Sugiyama, K., Tanabe, T., Wada, Y., Furugaito, M., et al. (2018). Purpura fulminans with Lemierre’s syndrome caused by Gemella bergeri and Eikenella corrodens: a case report. BMC Infect. Dis. 18:523. doi: 10.1186/s12879-018-3437-6
Yoon, S.-H., Ha, S.-M., Lim, J., Kwon, S., and Chun, J. (2017). A large-scale evaluation of algorithms to calculate average nucleotide identity. Antonie Van Leeuwenhoek 110, 1281–1286. doi: 10.1007/s10482-017-0844-4
Keywords: emergent pathogen, Granulicatella, horizontal gene transfer, Parvimonas, virulence factor
Citation: García López E and Martín-Galiano AJ (2020) The Versatility of Opportunistic Infections Caused by Gemella Isolates Is Supported by the Carriage of Virulence Factors From Multiple Origins. Front. Microbiol. 11:524. doi: 10.3389/fmicb.2020.00524
Received: 05 November 2019; Accepted: 11 March 2020;
Published: 31 March 2020.
Edited by:
Lisa Sedger, University of Technology Sydney, AustraliaReviewed by:
Erik Senneby, Lund University, SwedenRosalia Cavaliere, University of Technology Sydney, Australia
Copyright © 2020 García López and Martín-Galiano. This is an open-access article distributed under the terms of the Creative Commons Attribution License (CC BY). The use, distribution or reproduction in other forums is permitted, provided the original author(s) and the copyright owner(s) are credited and that the original publication in this journal is cited, in accordance with accepted academic practice. No use, distribution or reproduction is permitted which does not comply with these terms.
*Correspondence: Antonio J. Martín-Galiano, bWdhbGlhbm9AaXNjaWlpLmVz