- 1Department of Critical Care Medicine, Peking Union Medical College Hospital, Peking Union Medical College, Chinese Academy of Medical Sciences, Beijing, China
- 2Department of Clinical Laboratory, Peking Union Medical College Hospital, Peking Union Medical College, Chinese Academy of Medical Sciences, Beijing, China
- 3Beijing Key Laboratory for Mechanisms Research and Precision Diagnosis of Invasive Fungal Diseases, Beijing, China
The sustained high morbidity and mortality of Candida sepsis are mainly caused by compromise of host immunity. Clinically, it is often manifested as a significant decrease in CD4+ T cell count, although the mechanism is unclear. We established a lethal mice Candida sepsis model and used Murine Sepsis Score to group mice with different disease severity to establish the influence of T-bet expression on CD4+ T cell count in Candida sepsis. We found that CD4+ T cell count decreased in Candida-infected compared to uninfected mice, and the degree of decrease increased with aggravation of sepsis. Expression of T-bet similarly decreased with worsening of sepsis, but it was significantly enhanced in candidiasis in comparison of naïve state. To clarify its possible mechanism, we measured the activity of mammalian target of rapamycin (mTOR), which is a key regulator of T-bet expression. The mTOR pathway was activated after infection and its activity increased with progression of sepsis. We used mice with T-cell-specific knockout of mTOR or tuberous sclerosis complex (TSC)1 to further inhibit or strengthen the mTOR signaling pathway. We found that mTOR deletion mice had a higher CD4+ T cell count by regulating T-bet expression, and the result in TSC1 deletion mice was reversed. These results demonstrate that T-bet expression mediated by the mTOR pathway influences the CD4+ T cell count in mice with Candida sepsis.
Introduction
Candida albicans is one of the common commensals in humans. However, for immunocompromised and critically ill patients, infection with C. albicans can have disastrous consequences (Kullberg and Arendrup, 2015). Candidemia is the fourth most common bloodstream infection in intensive care units, and mortality of Candida bloodstream infection is close to 40%, which is significantly higher than mortality of sepsis caused by most bacterial pathogens (Wisplinghoff et al., 2004). Septic shock induced by C. albicans can be lethal, with an estimated mortality rate of nearly 90%; three times that of septic shock caused by bacteria (Guzman et al., 2011). Despite the use of antibiotics to which fungi are highly sensitive, and large-scale preventive trials, this situation has not been improved. The incidence and mortality of Candida sepsis remain high (Wenzel and Gennings, 2005; Pappas et al., 2016). Consequently, a lot of research has attempted to address this problem by immunoregulation or enhancing host immunity (Delsing et al., 2014; Sam et al., 2018).
It is well known that the intensity of the adaptive immune response is closely related to the occurrence, development and prognosis of Candida sepsis (Richardson and Moyes, 2015). The adaptive immune response mediated by CD4+ T cells is an essential pathway for the host to quickly eliminate invasive pathogens. A decreased CD4+ T cell count means that the host’s immune system is compromised and the intensity of the immune response is weakened, which are major reasons for poor outcomes in patients with Candida sepsis (Spec et al., 2016). Thus, understanding the molecular mechanisms that cause the decline of CD4+ T cell count in patients with Candida sepsis may provide new insights into improving outcomes.
T-bet is the most well-known specific transcription factor of the T-box family and regulates the differentiation and development of CD4 type 1 helper T (Th1) cells. T-bet directly promotes Th1 differentiation and inhibits differentiation of other Th cells; therefore, it has significant Th1 specificity, which ensures polarization of naïve T cells in the direction of Th1 (Szabo et al., 2000). Many studies have measured the expression of T-bet to reflect the level of CD4+ T cell differentiation and evaluate the host adaptive immune response after infection (Wu et al., 2013; Xu et al., 2019). In addition, T-bet expression is mainly regulated by the mammalian target of rapamycin (mTOR) signaling pathway. As an evolutionarily conserved signaling pathway, mTOR is widely involved in lymphocyte biology. When Candida infection occurs, mTOR is activated, integrating numerous environmental and immune elements, and participates in regulation of lymphocyte development, activation, differentiation and death (Ben-Sahra and Manning, 2017; Mossmann et al., 2018). We have demonstrated that mTOR is involved in CD8+ T cell differentiation that is achieved by regulating expression of transcription factors (Cui et al., 2016; Wang et al., 2018).
Hence, we hypothesized that the mTOR pathway might mediate expression of specific transcription factor T-bet to regulate lymphocyte differentiation during Candida sepsis and influence CD4+ T cell count, leading to compromise of host immune function.
Materials and Methods
Pathogen Preparation
Candida albicans SC5314 was kindly provided by the Department of Clinical Laboratory, Peking Union Medical College Hospital. C. albicans was grown overnight in YPD broth at 37°C. We harvested the cells by centrifugation (1 min, 800 × g, room temperature), washed them with sterile pyrogen-free phosphate-buffered saline (PBS) twice, and resuspended the cells in culture medium (Heather et al., 2014). Finally, we used the turbidity method (Espinel-Ingroff and Kerkering, 1992) to adjust the concentration of conidia to 106 colony-forming units (cfu).
Mice
Male healthy C57BL/6 N mice aged 4–5 weeks weighing 16–18 g were bred under specific pathogen-free conditions in the Animal Laboratory of Peking Union Medical College Hospital. TSC1loxp/loxp, mTORloxp/loxp and Lck-cre mice were generously given by Dr. Yong Zhao (State Key Laboratory of Biomembrane and Membrane Biotechnology, Institute of Zoology, Chinese Academy of Sciences, Beijing, China). Our experiments were approved by the Ethics Committee of Peking Union Medical College Hospital, Chinese academy of medical sciences (Beijing, China) and the approval No is JS-1170. We cross-bred TSC1loxp/loxp and mTORloxp/loxp mice with mice expressing Cre recombinase, respectively, and thus obtained F1 generation Lck-cre; TSC1loxp/– and Lck-cre; mTORloxp/– mice. After that F1 generation mice were interbred in the group and F2 generation Lck-cre; TSC1loxp/loxp and Lck-cre; mTORloxp/loxp mice were obtained. There were 30 mice in each group. Before the experiment, all mice were adapted to a 12-h day–night cycle for >1 week and the food and water were given ad libitum.
C. albicans Infection Model
Wild-type (WT) mice and gene-specific knock-out mice were all injected intravenously through the tail vein with the same dose of C. albicans conidia (1 × 106 cfu/mouse) in 100 μl PBS (Heather et al., 2014) Uninfected control mice were injected with the same dose of saline. Since renal fungal burden at 12 h after challenge has been shown associated with the severity of the disease model (Hurtrel et al., 1980), all mice were euthanized at 12 h after infection and the kidneys, lungs, spleens and livers were removed for histological analysis with Gomori’s methenamine silver (GMS) staining.
Murine Sepsis Score
The Candida intravenous challenge is one of the common models to mimic clinical candidemia. However, because of the different characteristics of the mice and strains used, the severity of many models is not consistent (MacCallum and Odds, 2005; Carreras et al., 2019). In order to ensure the consistency of the model, we used the Murine Sepsis Score (MSS) for severity evaluation (Shrum et al., 2014). MSS includes spontaneous activity, response to touch and auditory stimuli, posture, respiration rate and quality, and appearance in five sections. Each section is assessed on a scale of 0–4 points (Parameters of MSS was provided in Supplementary Material). Mice with >3 points were demonstrated to have sepsis and those with >10 points were associated with a significant increase in mortality. Therefore, at 12 h after challenge, we collected relevant data and performed the MSS on each mouse.
Histopathology
Kidneys, spleens, livers and lungs were stored in 10% neutral-buffered formalin at 4°C after removing from anesthetized and sacrificed mice. After embedding in paraffin and cutting into 4-μm slices, the tissues were stained with GMS. We used Pannoramic DESK (3D HISTECH; Hungary) to scan and image the pathological sections stained with GMS.
Lymphocyte Isolation and Cell Counting
To determine the T-cell count, we took the spleens of the mice, torn up them with eye tweezers and washed with PBS, then used ammonium chloride–potassium lysis buffer (BD) to lyse the red blood cells in the spleens. After that, we resuspended the centrifuged spleen cells (4°C, 500 g, 5 min) in RPMI-1640 medium, used trypan blue to stain the cell suspension and quantified their viability and number with a TC20 automated cell counter (Bio Rad).
Surface Marker Staining and Cell Sorting
Based on the PBMCs of spleen, we detected the ratio of CD4+ T cells. The cell suspension density was adjusted to 1 × 106/ml for flow cytometry and initially gated by forward and side scatter properties (Gating strategy was provided in Supplementary Material). CD4+ T-cells and CD8+ T-cells were then gated. Then we sorted CD4+ T cells and the purity of CD4+ T cells was also detected. The sorted CD4+ T cells were then used for western blotting. The machine we used is BD FACS AriaIII. In the process of cell sorting we used doublet exclusion to ensure the flow cytometry detection of individual cells. The CD4 Monoclonal Antibody (RM4-5), PerCP-Cyanine 5.5, CD8 alpha Monoclonal Antibody (53-6.7), FITC (MA1-10303) added in cell suspension were purchased from eBioscience. The purity was verified as >90%. The sorted cells were used for western blotting.
Western Blotting
To assess the activation of mTOR during Candida sepsis, we used western blotting to detect the levels of p-mTOR and p70S6 kinase. As one of the downstream effector molecules of mTORC1, p70S6 kinase is widely involved in the regulation of mTOR signaling pathway on cellular functions, such as proliferation and differentiation, autophagy and apoptosis, and the transformation of glucose metabolism (Ghosh and Kapur, 2017). In addition, we detected the expression of T-bet through western blotting. First, we used 300 μl RIPA buffer to extract the protein from sorted CD4+ T cells on ice. Secondly, the sample was centrifuged at 12000 rpm at 4°C for 15 min. The supernatant was taken to measure the protein concentration by the bicinchoninic acid method. Thirdly, 30 mg protein was subjected to SDS-PAGE and transferred to polyvinylidene fluoride membranes and 5% nonfat milk was used for blocking the membranes and further incubated them overnight with specific primary antibodies. At last we washed the membranes with TBST buffer for three times, incubated with the corresponding horseradish-peroxidase-conjugated secondary antibody for 1 h and developed on X-ray film with chemiluminescence reagent. Images were captured by Bio-Rad ChemiDoc XRS+, and densitometric analysis was performed using Quantity-One software (Bio-Rad). We used the following antibodies: anti-p-p70S6 (Cell Signaling Technology), anti-phospho (p)-mTOR (Cell Signaling Technology) and anti-T-bets (Cell Signaling Technology); anti-glyceraldehyde-3-phosphate dehydrogenase (GAPDH) (Santa Cruz Biotechnology) was used as a control.
Statistical Analysis
Data were analyzed using SPSS version 24.0 software (SPSS Inc., Armonk, NY, United States) (Relevant data was conducted a normality test and the results were provided in Supplementary Material). Two tailed Student’s t-test or one-way analysis of variance (ANOVA) followed by Dunn’s/Bonferroni’s test were used to determine the statistical significance of differences. P < 0.05 was considered statistically significant.
Results
Model Confirmation of Lethal Sepsis Induced by Intravenous Injection of C. albicans
In this study, we used the established mouse model of lethal Candida sepsis (Wang et al., 2019), which had been verified to express the characteristics of clinical Candida sepsis well. As shown in Figure 1, the hyphae and spores of C. albicans were significantly distributed in the tissues of infected mice.
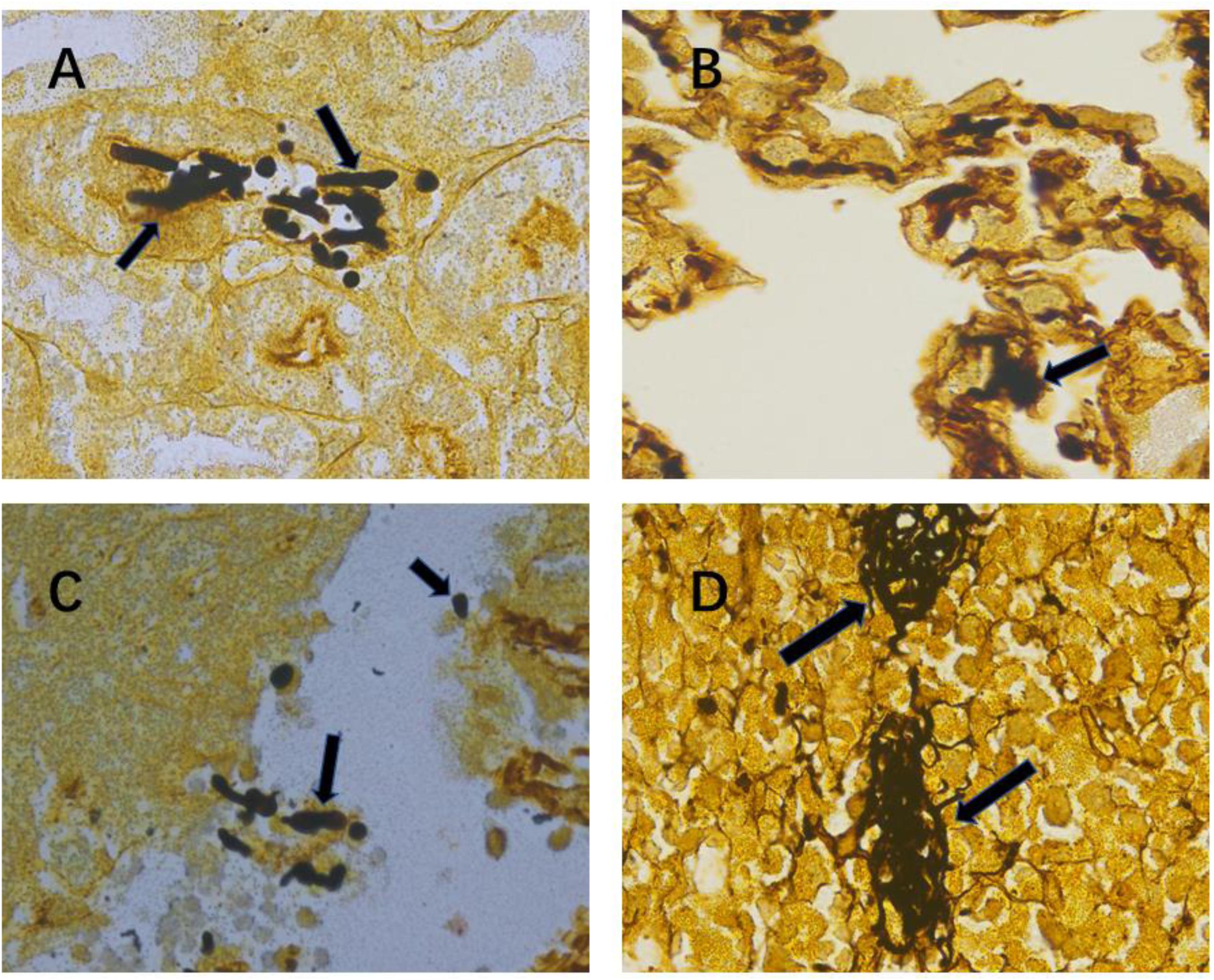
Figure 1. Confirmation of severe invasive Candida albicans multiorgan infection mouse model. Representative kidney (A), lung (B), liver (C) and spleen (D) histological sections at 12 h after intravenous injection of C. albicans were stained with Gomori’s methenamine silver to visualize C. albicans hyphae and spores. Black arrows indicate hyphae and spores. Magnification, 1000×.
Use of MSS to Distinguish Different Degrees of Sepsis
Although the mice were all inoculated with the same dose of C. albicans suspension, the survival time of the mice varied significantly, which implied different degrees of sepsis severity. Since MSS can judge the severity of sepsis to some extent, all mice were scored with MSS at 12 h after C. albicans injection. The differences of the survival curves between any two of three MSS groups were statistically significant (P-value between any two of the three different curves of MSS groups analyzed by log-rank test was <0.0001) (Figure 2A). Mice with different MSS had different survival time. Mice with MSS 4–7 had the longest survival time; mice with MSS >10 had the shortest survival time; and the survival time of the MSS 8–10 group was in the middle (Figure 2B). The MSS had significant negative correlation with the survival time (Figure 2C). These results demonstrate that MSS can distinguish different degrees of fungal sepsis.
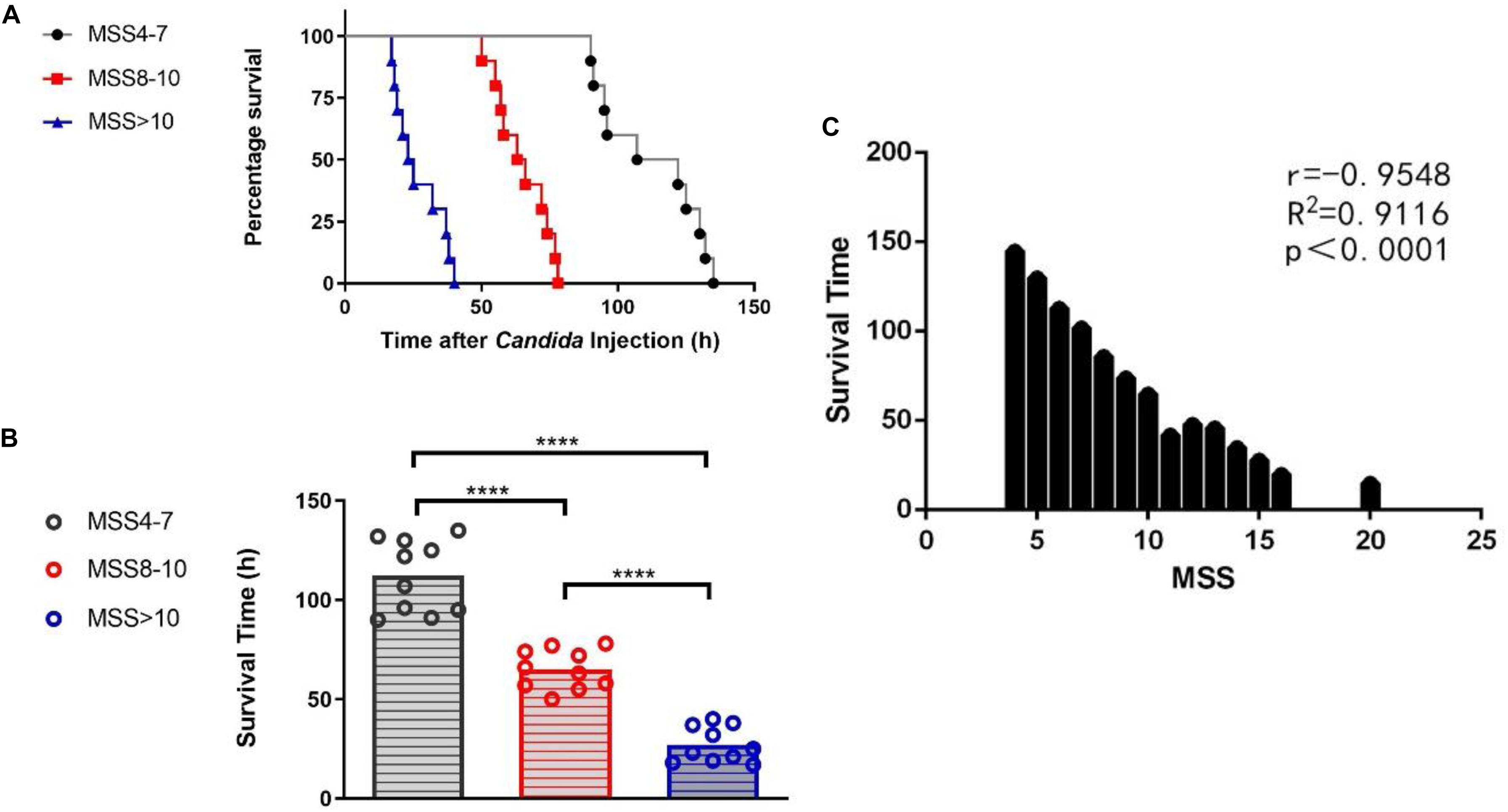
Figure 2. Group difference and correlation between Murine Sepsis Score (MSS) and survival time of mice. Survival rates between MSS 4-7, MSS 8-10 and MSS >10 mice (A). Mice in different MSS group had varied survival time (B). Correlation between MSS and survival time (C). Survival rates were observed for every 1 h after Candida injection. P-value between any two of the three different curves of MSS groups analyzed by log-rank test was <0.0001. MSS was assessed at 12 h after Candida injection. Mean ± SD, 10 mice per group. ****P < 0.0001.
CD4+ T Cell Count and T-Bet Expression in C. albicans-Infected Mice With Aggravation of Sepsis
To determine the effect of Candida sepsis on CD4+ T cells and its underlying mechanism, we separated CD4+ T cells from the spleen by flow cytometry and further measured the expression of T-bet by western blotting (Figure 3A). Compared with uninfected control mice, CD4+ T cell count significantly decreased in all three MSS groups which represented C. albicans-infected mice and continued to decrease with aggravation of sepsis (Figure 3B). The expression of T-bet also decreased with aggravation of sepsis but was significantly enhanced in candidiasis in comparison of naïve state (Figure 3C). These results demonstrate that T-bet might play an important role in regulation of CD4+ T cells during lethal fungal sepsis.
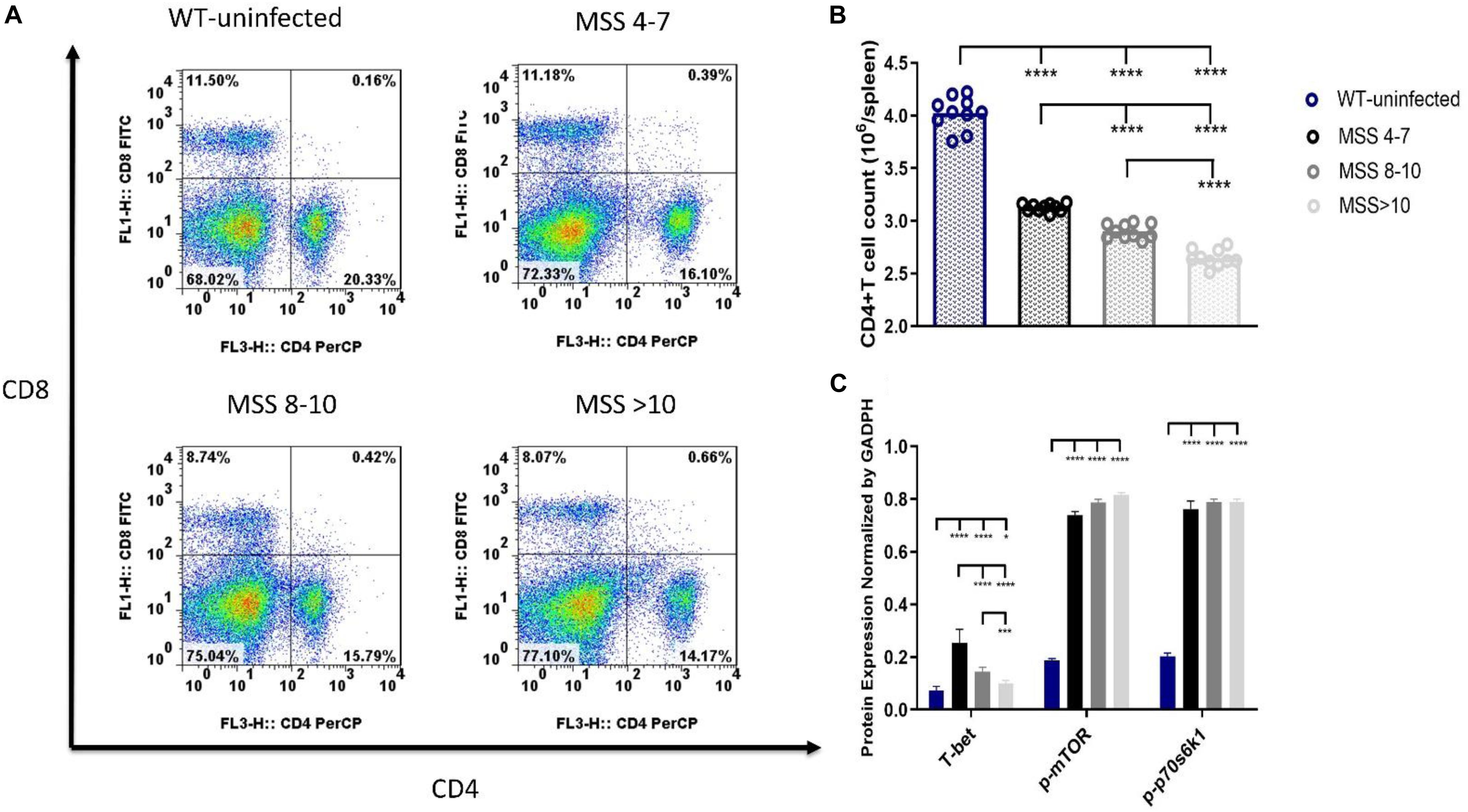
Figure 3. CD4+ T cell count, T-bet expression and mammalian target of rapamycin (mTOR) pathway activation differed according to degrees of sepsis. Splenocytes were obtained at 12 h after Candida injection. (A,B) CD4+ T cells were sorted by flow cytometry analysis. Protein levels of T-bet, phospho (p)-mTOR and p-P70S6 (C) in CD4+ T cells. Levels of T-bet, p-mTOR and p-P70S6 in CD4+ T cells were quantified by western blotting. The amount of each protein level was normalized by GAPDH. Mean ± SD, 10 mice per group, analyzed by one-way analysis of variance multiple comparisons. *P < 0.05, ***P < 0.001, ****P < 0.0001. WT, wild type; (Summarization of the flow cytometry scatter plots was provided in Supplementary Material).
mTOR Pathway Activation Was Elevated in C. albicans-Infected Mice
To assess the activation of mTOR, a key signaling pathway that regulates T-bet expression, in Candida sepsis, we used western blotting to detect the levels of p-mTOR and p70S6 kinase. Compared with uninfected control mice, both were elevated in three MSS groups which represented C. albicans-infected mice and increased with aggravation of sepsis (Figure 3C). These results demonstrate that mTOR is involved in regulating CD4+ T cells in Candida sepsis.
Expression of T-Bet in Different Degrees of Candida Sepsis Is Regulated by mTOR
To further investigate if the mTOR pathway regulated T-bet expression in Candida sepsis, we used T-cell-specific mTOR knockout (Lck-mTOR) mice and T-cell-specific TSC1 knockout (Lck-TSC1) mice. Firstly, we verified mRNA expression by qPCR as the description in our previous study (Wang et al., 2019). Secondly, we detected the protein level of p-mTOR (phosphorylation at Ser2448) and activity of p70S6 kinase (phosphorylation at Thr389), which were both elevated in Lck-TSC1 mice and reduced in Lck-mTOR mice (Figures 4A–C). These results confirmed the gene knockout effect. Thirdly, we detected T-bet expression in gene knockout mice with Candida sepsis (Figures 4A,D). Compared with WT mice, the T-bet expression of mice in the Lck-mTOR group was significantly increased while that in the Lck-TSC1 group was reversed, indicating that the expression of T-bet requires mTOR signaling pathway. At last, we compared the difference of T-bet expression between Lck-mTOR mice and Lck-TSC1 mice under the same infection degree (MSS group) and found that in the same MSS group (Figure 4E), Lck-mTOR mice had more T-bet expression than WT mice, while Lck-TSC1 mice had less expression than WT mice. These results indicated that the expression of T-bet is regulated by the mTOR signaling pathway during Candida sepsis.
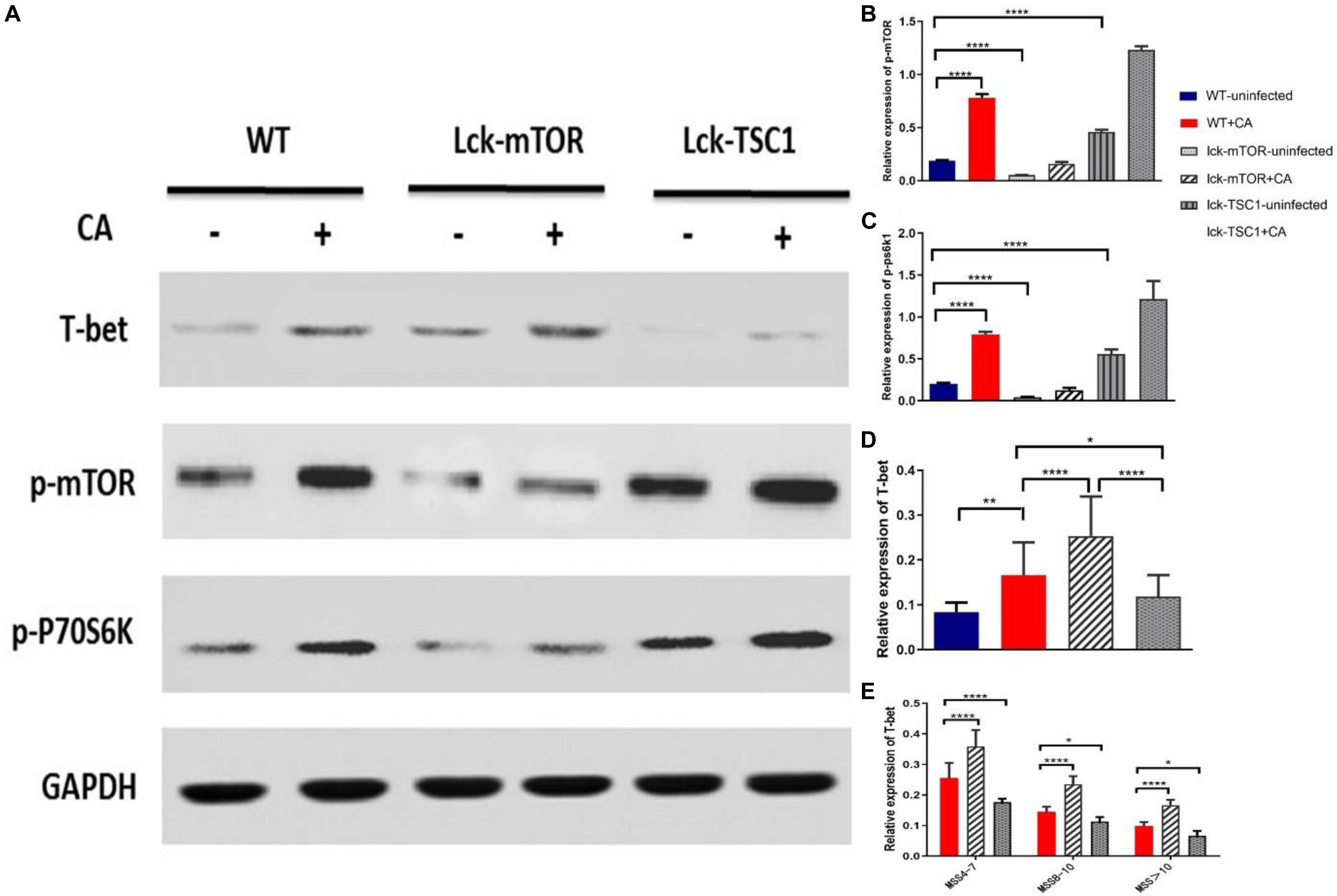
Figure 4. Expression of T-bet in different degrees of Candida sepsis is regulated by mTOR. (A–E) Protein levels of T-bet, phospho (p)-mTOR and p-P70S6 were quantified by western blotting from sorted CD4+ T cells in wild-type (WT), mTOR knockout (Lck-mTOR) and TSC1 knockout (Lck-TSC1) mice. The amount of each protein level was normalized by GAPDH. Mean ± SD, 10 mice per MSS group (“+CA” groups contained all infected mice of three MSS groups), analyzed by one-way analysis of variance multiple comparisons. *P < 0.05, **P < 0.01, ****P < 0.0001.
Variation in CD4+ T Cell Count and T-Bet Expression in T-Cell-Specific mTOR/TSC1 Knockout Mice With Lethal Candida Sepsis
To further demonstrate that CD4+ T cell count is influenced by T-bet expression mediated by mTOR during lethal Candida sepsis, we measured the CD4+ T cell count of gene knockout mice with Candida sepsis (Figures 5A–C). CD4+ T cell count and T-bet expression had the same variation tendency in gene knockout mice with lethal Candida sepsis. In the same MSS group, Lck-mTOR mice had more T-bet expression and CD4+ T cell count than WT mice had, while Lck-TSC1 mice had less than WT mice had. These results demonstrate that the mTOR pathway affects CD4+ T cell count by regulating T-bet expression level during Candida sepsis.
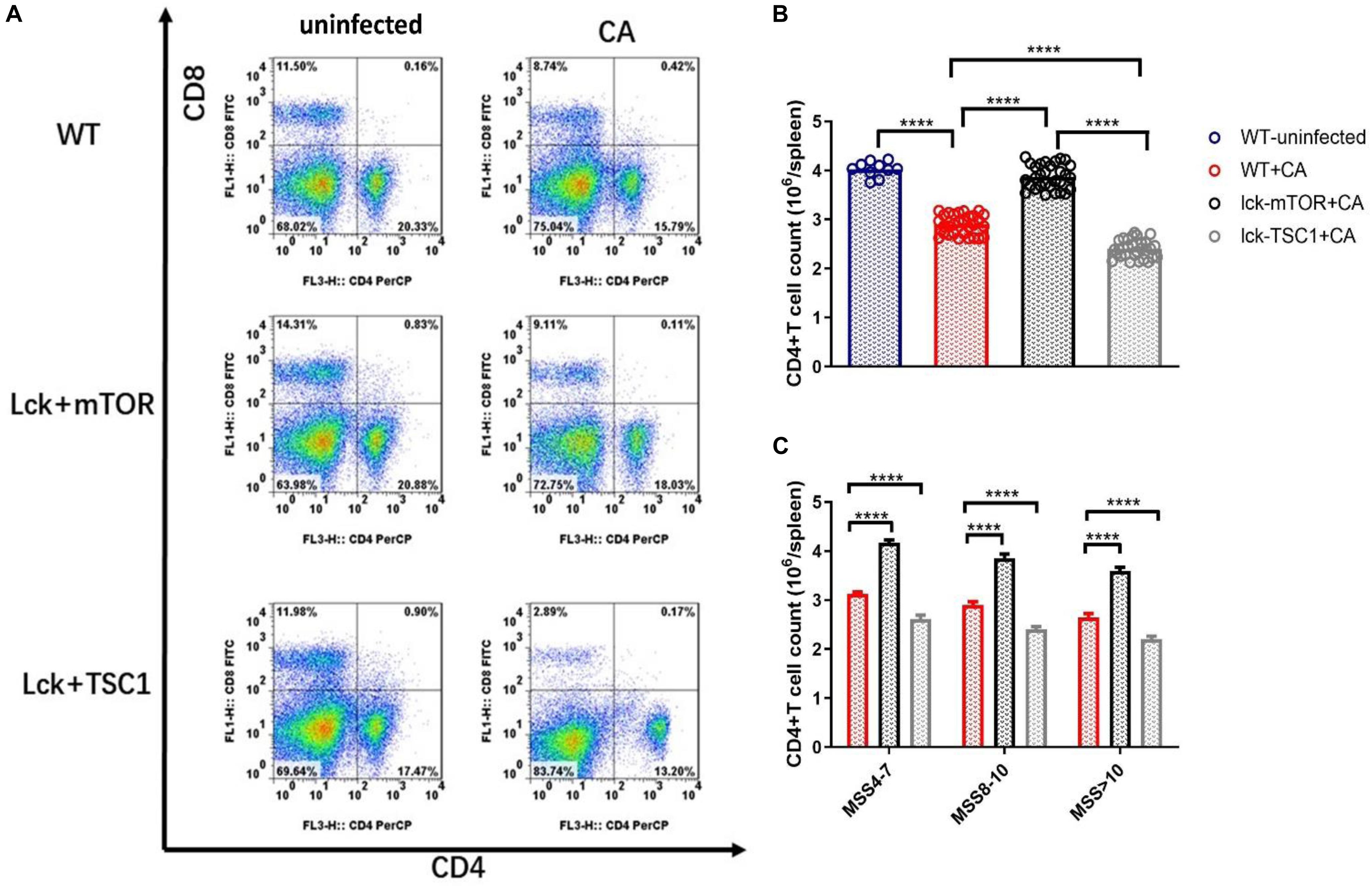
Figure 5. CD4+ T cell count during Candida sepsis is affected by mTOR pathway. Splenocytes of specific gene knockout mice were obtained at 12 h after Candida injection. (A–C) Gating strategy for CD4+CD8– and CD4–CD8+ T cells by flow cytometry analysis. Mean ± SD, 10 mice per MSS group (“+CA” groups contained all infected mice of three MSS groups), analyzed by one-way analysis of variance multiple comparisons. ****P < 0.0001.
Discussion
To our knowledge, this is the first study to demonstrate that expression of CD4-specific transcription factor T-bet regulated by the mTOR signaling pathway influences CD4+ T cell count in lethal Candida sepsis of different severity.
Invasive candidiasis has been a great challenge in clinical practice, especially in critical care, for the past few decades. Despite our efforts to improve early recognition of candidiasis and to prevent or treat it with antifungal agents, it is still common and lethal in immunosuppressed and critically ill patients. Many studies have proved that compromise of host immunity is one of the main reasons (Spec et al., 2016). As a component of the adaptive immune system, CD4+ T cells play a major role in the process of eliminating invasive Candida (Seana et al., 2015). Candida count has been proved to reflect host immune status (Limper et al., 2017). After Candida invades the body, naïve T cells regulated by specific transcription factors begin to differentiate and develop into various effector T helper cells to protect the host against disseminated Candida (Romani, 2011). Thus, a decrease in CD4+ T cell count may be partly caused by disordered differentiation. In the present study, after mice were infected, the CD4+ T cell count decreased significantly and decreased further with aggravation of sepsis, which meant that host immunity was impaired.
T-bet is the main coordinator of gene expression of type 1 immune T cells and a key transcription factor for the differentiation of naïve T cells into Th1 cells. It has been shown that Th1 cells play a central role in eliminating disseminated Candida, mainly by releasing interferon-γ, which mediates cellular immunity and induces delayed hypersensitivity (Delsing et al., 2014). Hence, in the process of Candida sepsis, T-bet acts on CD4+ T cells to regulate the differentiation and growth of its effector cells, thus participating in the clearance of disseminated Candida. In this research, we found that T-bet expression increased after infection but decreased with aggravation of sepsis, suggesting that T-bet plays an important role in the regulation of CD4+ T cells during lethal Candida sepsis. In addition, we found that compared with non-infected mice, the CD4+ T cell count of infected mice decreased, but the expression of T-bet significantly increased. We think the reason is infection induces the initiation of immune response, which promotes the differentiation of naive T cells into effector cells and increases the expression of T-bet. However, when severe infection occurs, the severity of infection has a very important and complex influence on the immune functional differentiation in T cells.
For further analysis, we used MSS to confirm the sepsis model and found that mice with different MSS had different survival time. In this study, mice with high MSS had shorter survival time and lower survival rate, which meant that they had more severe sepsis. This finding is consistent with research by Shrum et al. (2014), in which MSS was used in mice with sepsis, to assess the severity of the sepsis model. As a result, we divided the experimental mice into three MSS groups (4–7, 8–10, and >10) to represent different degrees of sepsis and measured CD4+ T cell count and T-bet expression. We found that mice in MSS group 4–7 had the highest T-bet expression and CD4+ T cell count, while mice in MSS group >10 had the lowest T-bet expression and CD4+ T cell count. This result demonstrated that the more severe Candida sepsis was, the lower the T-bet expression, indicating less Th1 differentiation during this process. This is consistent with a prospective clinical observational study that demonstrated a significant increase in T-bet expression in the sepsis/severe sepsis/septic shock group compared to the uninfected control group, and a gradual decrease in T-bet expression as the disease progressed (Li et al., 2015). In addition, in our study, the MSS >10 group had the lowest number of CD4+ T cells, indicating the most severe immunosuppression and weakest adaptive immune response, which explains why the survival time of this group was the shortest. This finding is consistent with the study of Wu et al. (2013) in which high Th1 differentiation and CD4+ T cell count in septic mice had higher survival rates.
However, in the clinical study by Xu et al. (2019), the sepsis group showed decreased T-bet expression compared to the uninfected control group. The reason for this difference may be that, although all patients had sepsis, most of the pathogens were bacteria, which differed from our study, in which the pathogen was a fungus. It is interesting to note that Xu et al. found that patients in the survival group had higher T-bet expression than those that died, suggesting that the higher the severity of sepsis, the lower the expression of T-bet. This is consistent with our results. We believe that comparison of infected and uninfected groups differs between the two studies because different pathogens caused sepsis, which led to emergence of pathogen-specific host immune response at the initial stage of infection. However, as the infection progresses and worsens into sepsis and even septic shock, the host immune response gradually becomes uniform, that is, immune imbalance caused by simultaneous proinflammatory and anti-inflammatory effects. At this stage, the signaling pathways and mechanisms that regulate the host immune response may be dominated by sepsis, so the same results are obtained.
Indeed, the effector differentiation process of T cells during infection is influenced by multiple signaling channels and transcription factors. This is one of the main issues of our study. T-bet expression is regulated by the mTOR signaling pathway (Chornoguz et al., 2017). In our previous studies, we proved that mTOR also affected CD8+ T cell differentiation in mice with invasive pulmonary aspergillosis by regulating expression of T-bet and Eomes, and mTOR and its downstream S6k1 levels were positively correlated with T-bet and Eomes expression (Cui et al., 2016). Thus, to further uncover the underlying mechanism, we used western blotting to assess activity of mTOR during this lethal fungal sepsis. mTOR activity significantly increased after infection and further increased with aggravation of sepsis, which indicates that mTOR is involved in regulating CD4+ T cells in Candida sepsis. After that, we used gene-specific knockout of mTOR and TSC1 mice to strengthen/inhibit the mTOR signaling pathway. During lethal Candida sepsis, mice in which the mTOR signaling pathway was inhibited by T-cell-specific mTOR deletion had higher expression of T-bet and CD4+ T cell count than WT mice had. Also, mice with T-cell-specific TSC1 deletion had lower expression of T-bet and CD4+ T cell count indicating that the expression of T-bet required mTOR to participate in the regulation, and the regulation affected the CD4+ T cell count in Candida sepsis. This is not consistent with a recent study that showed that expression of T-bet decreased and Th1 cells could not differentiate after inhibition of mTOR by rapamycin (Chornoguz et al., 2017). In that study, the differentiation of CD4+ T cells was achieved in a normal physiological state, while differentiation occurred in a state of lethal Candida sepsis in our study. Different pathological conditions may be a major reason for the different results in the two studies. Many studies have shown that proliferation and differentiation of naïve T cells have significant characteristics under different pathological conditions including infection and tumor, or even in specific cytokine environments (Resende et al., 2013; Saleh et al., 2019). In addition, our result showed even in presence of mTOR deficiency there is a severity-dependent decrease of T-bet and CD4+ T cell count, this is consistent with the study of Jin et al. (2019). They found that when severe infection occurred, under the regulatory of Notch signaling pathway, CD4+ T cell count decreased and continued to decrease with the worsening of sepsis. Thus, we think the change of CD4+ T cell count in our study is due to the joint action of different regulatory mechanisms and changes in a single regulatory pathway may not reflect the overall results of immune differentiation, which needs comprehensive consideration. In a future study we aim to clarify the subpopulation in CD4+ T cell differentiation, such as CD4 type 17 helper T (Th17) cells (Lin et al., 2009), and the relationship between specific transcription and other possible influencing factors in lethal fungal sepsis, to explore the specific mechanism behind this phenomenon.
In summary, our research provides the first preliminary evidence that T-bet expression mediated by the mTOR pathway influences CD4+ T cell count in mice with lethal Candida sepsis, which provides new targets and potential therapeutic strategies for improving immune compromise.
Data Availability Statement
The datasets generated for this study are available on request to the corresponding author.
Ethics Statement
The animal study was reviewed and approved by the Institutional Animal Care and Use Committee (IACUC) of PUMCH.
Author Contributions
NC, HW, GB, and WH together participated in the study design and writing the manuscript and experiments.
Funding
The work was supported by the Clinical and Translational Medicine Research Foundation of Chinese Academy of Medical Sciences (No. 2019XK320040), the National Natural Science Foundation of China (No. 81601657), and Wu Jieping Medical Foundation (No. 320.6750.18419).
Conflict of Interest
The authors declare that the research was conducted in the absence of any commercial or financial relationships that could be construed as a potential conflict of interest.
Acknowledgments
The authors wish to thank Dr. Yong Zhao (State Key Laboratory of Biomembrane and Membrane Biotechnology, Institute of Zoology, Chinese Academy of Sciences, Beijing, China) for generously providing the TSC1loxp/loxp mice, mTORloxp/loxp mice, and Lck-cre mice.
Supplementary Material
The Supplementary Material for this article can be found online at: https://www.frontiersin.org/articles/10.3389/fmicb.2020.00835/full#supplementary-material
References
Ben-Sahra, I., and Manning, B. D. (2017). mTORC1 signaling and the metabolic control of cell growth. Curr. Opin. Cell Biol 45, 72–82. doi: 10.1016/j.ceb.2017.02.012
Carreras, E., Velasco, D. A. M., Orta-Mascaro, M., Simões, I. T., Català, C., Zaragoza, O., et al. (2019). Discordant susceptibility of inbred C57BL/6 versus outbred CD1 mice to experimental fungal sepsis. Cell Microbiol. 21, e12995. doi: 10.1111/cmi.12995
Chornoguz, O., Hagan, R. S., Haile, A., Arwood, M. L., Gamper, C. J., Banerjee, A., et al. (2017). mTORC1 promotes T-bet Phosphorylation to regulate Th1 differentiation. J. Immunol. 198, 3939–3948. doi: 10.4049/jimmunol.1601078
Cui, N., Su, L. X., and Wang, H. (2016). mTOR modulates Lymphocyte differentiation through T-bet and Eomesodermin in response to invasive pulmonary Aspergillosis in rats. Chin. Med. J. Peking 129, 1704–1710. doi: 10.4103/0366-6999.185858
Delsing, C. E., Gresnigt, M. S., Leentjens, J., Preijers, F., Frager, F. A., Kox, M., et al. (2014). Interferon-gamma as adjunctive immunotherapy for invasive fungal infections: a case series. BMC Infect. Dis. 14:166. doi: 10.1186/1471-2334-14-166
Espinel-Ingroff, A., and Kerkering, T. M. (1992). Collaborative comparison of broth macrodilution and microdilution antifungal susceptibility tests. J. Clin. Microbiol. 30, 3138–3145.
Ghosh, J., and Kapur, R. (2017). Role of mTORC1–S6K1 signaling pathway in regulation of hematopoietic stem cell and acute myeloid leukemia. Exp. Hematol. 50, 13–21. doi: 10.1016/j.exphem.2017.02.004
Guzman, J. A., Tchokonte, R., and Sobel, J. D. (2011). Septic shock due to candidemia: outcomes and predictors of shock development. J. Clin. Med. Res. 3, 65–71. doi: 10.4021/jocmr536w
Heather, R., Conti, A. R., Huppler, N., and Whibley, A. (2014). Animal models for Candidiasis. Curr. Protoc. Immunol. 105, 19.6.1-19.6.17.
Hurtrel, B., Lagrange, P. H., and Michel, J. C. (1980). Systemic candidiasis in mice. Ann. Immunol. 131, 93–104.
Jin, B., Liang, Y., Liu, Y., Zhang, L. X., Xi, F. Y., Wu, W. J., et al. (2019). Notch signaling pathway regulates T cell dysfunction in septic patients. Int. Immunopharmacol. 76:105907. doi: 10.1016/j.intimp.2019.105907
Li, J., Li, M., and Su, L. (2015). Alterations of T Helper Lymphocyte subpopulations in sepsis, severe sepsis, and septic shock: a prospective observational study. Inflammation 38, 995–1002. doi: 10.1007/s10753-014-0063-3
Limper, A. H., Adenis, A., Le, T., and Harrison, T. S. (2017). Fungal infections in HIV/AIDS. Lancet Infect. Dis. 17, e334–e343. doi: 10.1016/S1473-3099(17)30303-1
Lin, L., Ibrahim, A. S., Xu, X., Farber, J. M., Avanesian, V., Baquir, B., et al. (2009). Th1-Th17 cells mediate protective adaptive immunity against Staphylococcus aureus and Candida albicans infection in mice. PLoS Pathog. 5:e1000703. doi: 10.1371/journal.ppat.1000703
MacCallum, D. M., and Odds, F. C. (2005). Temporal events in the intravenous challenge model for experimental Candida albicans infections in female mice. Mycoses 48, 151–161. doi: 10.1111/j.1439-0507.2005.01121.x
Mossmann, D., Park, S., and Hall, M. N. (2018). mTOR signalling and cellular metabolism are mutual determinants in cancer. Nat. Rev. Cancer 18, 744–757. doi: 10.1038/s41568-018-0074-8
Pappas, P. G., Kauffman, C. A., Andes, D. R., Clancy, C. J., Marr, K. A., Ostrosky-Zeichner, L., et al. (2016). Clinical practice guideline for the management of Candidiasis: 2016 update by the Infectious Diseases Society of America. Clin. Infect. Dis. 62, e1–e50.
Resende, M., Moreira, D., Augusto, J., Cunha, J., Neves, B., Cruz, M. T., et al. (2013). Leishmania-Infected MHC Class II high Dendritic Cells Polarize CD4 + T Cells toward a Nonprotective T-bet+ IFN-γ+ IL-10+ Phenotype. J. Immunol. 191, 262–273. doi: 10.4049/jimmunol.1203518
Richardson, J. P., and Moyes, D. L. (2015). Adaptive immune responses to Candida albicans infection. Virulence 6:327. doi: 10.1080/21505594.2015.1004977
Romani, L. (2011). Immunity to fungal infections. Nat. Rev. Immunol. 11, 275–288. doi: 10.1038/nri2939
Saleh, M. E., Gadalla, R., Hassan, H., Afifi, A., Götte, M., El-Shinawi, M., et al. (2019). The immunomodulatory role of tumor Syndecan-1 (CD138) on ex vivo tumor microenvironmental CD4+ T cell polarization in inflammatory and non-inflammatory breast cancer patients. PLoS One 14:e217550. doi: 10.1371/journal.pone.0217550
Sam, Q. H., Yew, W. S., and Seneviratne, C. J. (2018). Immunomodulation as therapy for fungal infection: are we closer? Front. Microbiol. 9:1612. doi: 10.3389/fmicb.2018.01612
Seana, D., Leonhardt, I., and Hunniger, K. (2015). Host response to Candida albicans bloodstream infection and sepsis. Virulence 6, 316–326. doi: 10.4161/21505594.2014.988096
Shrum, B., Anantha, R. V., and Xu, S. X. (2014). A robust scoring system to evaluate sepsis severity in an animal model. BMC Res. 7:233. doi: 10.1186/1756-0500-7-233
Spec, A., Shindo, Y., Burnham, C. A., Wilson, S., Ablordeppey, E. A., Beiter, E. R., et al. (2016). T cells from patients with Candida sepsis display a suppressive immunophenotype. Crit. Care 20:15. doi: 10.1186/s13054-016-1182-z
Szabo, S. J., Kim, S. T., Costa, G. L., Zhang, X., Fathman, C. G., Glimcher, L. H., et al. (2000). A novel transcription factor. T-bet, directs Th1 lineage commitment. Cell 100, 655–669.
Wang, H., Bai, G., Cui, N., Han, W., and Long, Y. (2019). T-cell-specific mTOR deletion in mice ameliorated CD4(+) T-cell survival in lethal sepsis induced by severe invasive candidiasis. Virulence 10, 892–901. doi: 10.1080/21505594.2019.1685151
Wang, H., Xiao, Y., Su, L., Yang, F., Xiao, Y., Xiao, M., et al. (2018). mTOR modulates CD8+ T cell differentiation in mice with invasive pulmonary aspergillosis. Open Life Sci. 13, 129–136. doi: 10.4103/0366-6999.205850
Wenzel, R. P., and Gennings, C. (2005). Bloodstream infections due to Candida species in the intensive care unit: identifying especially high-risk patients to determine prevention strategies. Clin. Infect. Dis. 41(Suppl. 6), S389–S393. doi: 10.1086/430923
Wisplinghoff, H., Bischoff, T., Tallent, S. M., Seifert, H., Wenzel, R. P., Edmond, M. B., et al. (2004). Nosocomial bloodstream infections in US hospitals: analysis of 24,179 cases from a prospective nationwide surveillance study. Clin. Infect. Dis. 39, 309–317. doi: 10.1086/421946
Wu, H., Chung, K., and Lin, C. (2013). Associations of T helper 1, 2, 17 and regulatory T lymphocytes with mortality in severe sepsis. Inflamm. Res. 62, 751–763. doi: 10.1007/s00011-013-0630-3
Keywords: T-bet, CD4+ T cell count, lethal Candida sepsis, Murine Sepsis Score, mammalian target of rapamycin
Citation: Bai G, Wang H, Han W and Cui N (2020) T-Bet Expression Mediated by the mTOR Pathway Influences CD4+ T Cell Count in Mice With Lethal Candida Sepsis. Front. Microbiol. 11:835. doi: 10.3389/fmicb.2020.00835
Received: 06 January 2020; Accepted: 07 April 2020;
Published: 05 May 2020.
Edited by:
Christoph Hölscher, Research Center Borstel (LG), GermanyReviewed by:
Abdullah Alqarihi, Lundquist Institute for Biomedical Innovation, United StatesUwe Müller, Leipzig University, Germany
Copyright © 2020 Bai, Wang, Han and Cui. This is an open-access article distributed under the terms of the Creative Commons Attribution License (CC BY). The use, distribution or reproduction in other forums is permitted, provided the original author(s) and the copyright owner(s) are credited and that the original publication in this journal is cited, in accordance with accepted academic practice. No use, distribution or reproduction is permitted which does not comply with these terms.
*Correspondence: Na Cui, cHVtY2hjbkAxNjMuY29t