- 1Department of Infectious Diseases and Hospital Epidemiology, University Hospital Zurich, University of Zurich, Zurich, Switzerland
- 2Department of Immunology and Microbiology, University of Colorado School of Medicine, Aurora, CO, United States
- 3Department of Veterans Affairs Eastern Colorado Health Care System, Denver, CO, United States
Staphylococcus aureus causes chronic and relapsing infections, which may be difficult to treat. So-called small colony variants (SCVs) have been associated with chronic infections and their occurrence has been shown to increase under antibiotic pressure, low pH and intracellular localization. In clinics, S. aureus isolated from invasive infections often show a dysfunction in the accessory gene regulator (agr), a major virulence regulatory system in S. aureus. To assess whether intracellular environment and agr function influence SCV formation, an infection model was established using lung epithelial cells and skin fibroblasts. This allowed analyzing intracellular survival and localization of a panel of S. aureus wild type strains and their isogenic agr knock out mutants as well as a natural dysfunctional agr strain by confocal laser scanning microscopy (CLSM). Furthermore, bacterial colonies were quantified after 1, 3, and 5 days of intracellular survival by time-lapse analysis to determine kinetics of colony appearance and SCV formation. Here, we show that S. aureus strains with an agr knock out predominantly resided in a neutral environment, whereas wild type strains and an agr complemented strain resided in an acidic environment. S. aureus agr mutants derived from an intracellular environment showed a higher percentage of SCVs as compared to their corresponding wild type strains. Neutralizing acidic phagolysosomes with chloroquine resulted in a significant reduction of SCVs in S. aureus wild type strain 6850, but not in its agr mutant indicating a pH dependent formation of SCVs in the wild type strain. The in-depth understanding of the interplay between intracellular persistence, agr function and pH should help to identify new therapeutic options facilitating the treatment of chronic S. aureus infections in the future.
Introduction
Staphylococcus aureus is one of the major human pathogens causing both nosocomial and community-acquired infections. These may range from superficial skin and soft tissue infections to severe invasive infections including pneumonia, sepsis and endocarditis (Lowy, 1998; Petti and Fowler, 2002). Antibiotics usually effectively kill S. aureus. However, chronic and relapsing infections occur even if bacteria are fully susceptible to antibiotics (Welsh et al., 2011; Libraty et al., 2012; Fisher et al., 2017). One reason is the intracellular localization of S. aureus, which was previously considered an exclusively extracellular pathogen. Over the last decade, the relevance of intracellular bacteria as a reservoir in chronic infection has been recognized (Tuchscherr et al., 2011; Fraunholz and Sinha, 2012; Libraty et al., 2012). S. aureus has been shown to actively invade and survive in both professional as well as non-professional phagocytes (Sendi and Proctor, 2009; Fraunholz and Sinha, 2012; Strobel et al., 2016). Once taken up by a eukaryotic cell, the bacterium can undergo different fates: (i) it escapes the phagosome, proliferates in the cytosol and eventually kills its host cell, (ii) it is killed by the host’s defense machinery or (iii) it stays intracellularly without being cleared (Fraunholz and Sinha, 2012). Intracellular localization is a niche for S. aureus, where it is protected from the host’s immune system as well as from antibiotics that act extracellularly. Intracellular S. aureus has been described in cases of recurrent infections such as osteomyelitis and rhinosinusitis (Bosse et al., 2005; Clement et al., 2005; Libraty et al., 2012). Bacteria recovered from chronic infections often show a heterogeneity in colony size (Proctor et al., 2006). The smaller colonies represent a bacterial subpopulation that has previously been referred to as small colony variants (SCVs) (Proctor et al., 1994). Recently, our group showed that one reason for a SCV subpopulation is a delayed growth resumption imposed by a stress such as acidic pH, antibiotic exposure or the intracellular milieu of eukaryotic host cells (Leimer et al., 2016; Vulin et al., 2018). In particular, prolonged intracellular survival of S. aureus has been linked to an increasing percentage of SCVs in a bacterial population (Tuchscherr et al., 2011; Leimer et al., 2016; Rollin et al., 2017). In addition to these extrinsic factors, genetic factors including several S. aureus regulatory systems such as agr, sigB and sarA were shown to influence SCV formation (Kahl et al., 2005). Especially, the accessory gene regulator (agr), a quorum sensing system regulating the expression of adhesins during attachment and virulence factors during infection, is downregulated in SCVs (Moisan et al., 2006; Mitchell et al., 2013). The majority of S. aureus isolated from invasive infections showed reduced toxicity accompanied by a reduction in agr activity as compared to colonizing S. aureus strains (Laabei et al., 2015; Altman et al., 2018). Downregulation of agr has been reported to promote bacteremia and increase mortality in invasive S. aureus infections (Shopsin et al., 2008; DeLeo et al., 2011; Schweizer et al., 2011; Soong et al., 2015). The role of agr deficiency together with the exposure to intracellular pH on SCV formation has not been elucidated yet.
In this study, we show that despite their localization in a neutral intracellular milieu, S. aureus agr mutants have an increased colony size heterogeneity as compared to their corresponding wild type strains suggesting a pH independent formation of SCVs in S. aureus agr mutants.
Materials and Methods
Cell Culture, Bacterial Strains and Growth Conditions
Human alveolar lung epithelial cells A549 and human skin fibroblast cells BJ-5ta were cultured in Dulbecco’s Modified Eagle Medium (DMEM) (Gibco) supplemented with 4.5 g/L glucose, 2 mM L-glutamine (Gibco) and 10% fetal bovine serum (FBS) (Eurobio) at 37°C and 5% CO2. All S. aureus strains used in this study are listed in Table 1. S. aureus overnight cultures were grown in tryptic soy broth (TSB) (BD) at 37°C, 220 rpm. Prior to infection, bacterial overnight cultures were diluted 1:10 in TSB and grown for 2 h to exponential phase. Phage 11 was used to transduce an existing agr:tetM mutation from S. aureus strain RN6911 into strain 6850 (Novick et al., 1993). All agr mutant strains used in this study were whole genome sequenced using an Illumina MiSeq machine and the QIAseq FX DNA Library Kit (Qiagen) to confirm correct replacement of the whole agr system by tetM. Whole genome data analysis was performed using CLC Genomic Workbench (Qiagen) version 10.1.1.
To construct the agr complemented strain, RNAIII and the agrBDCA operon were amplified from USA300 LAC genomic DNA using primers HC735 (5′-GTTGTT GGATCC ACGGCGGGTCTCATAATGAT-3′) and HC736 (5′-GTTGTT AAGCTT TGCGCCATAGGATTGTAGAGT-3′). The PCR product was digested using BamHI and HindIII, and ligated into pLL29 containing the tetracycline resistance gene tetK (Luong and Lee, 2007), generating plasmid pHC175. This plasmid was electroporated into S. aureus 4220 containing pLL2787, and chromosomal integration of plasmid pHC175 at the phage 11 attachment site was selected on TSA containing 1 μg/ml tetracycline. The integrated cassette was transduced into S. aureus LAC using phage 11, and integration was confirmed by PCR. The Δagr:tetM deletion was transduced into this strain, selecting on TSA containing 0.2 μg/ml minocycline. The agr deletion was confirmed by PCR and phenotypes were verified on blood plates. Phage 11 was used to transduce the construct for agr complementation into S. aureus SH1000 agr:tetM.
Bacterial Infection and Intracellular Survival Assay
For all assays, 105 epithelial cells were seeded into 24-well plates. For confocal microscopy, 12 mm coverslips were placed inside the wells before seeding. Epithelial cells were infected at a multiplicity of infection (MOI) of 1 for intracellular survival, microscopy and radial colony growth analysis. After 3 h invasion, epithelial cells were treated with 1 mg/ml flucloxacillin and 20 μg/ml lysostaphin for 1 h (D0), 1 (D1), 3 (D3), or 5 days (D5), respectively. To neutralize the phagolysosomes, 20 μM chloroquine was additionally added. Medium was changed daily. To assess bacterial intracellular survival at the indicated time points, epithelial cells were washed twice with phosphate buffered saline (PBS) (Gibco) and lysed with water. Colony forming units (CFUs) were recovered on tryptic soy agar (TSA) plates.
Radial Colony Growth and SCV Formation
Intracellular S. aureus was recovered 4 h, 1, 3, and 5 days after infection as described above. Radial colony growth on blood agar plates (Columbia + 5% sheep blood, Biomerieux) was monitored at 37°C. For time-lapse imaging, four cameras (Basler, acA5472-17um 20MP with Fujinon Objectives CF16ZA-1S 16 mm/1.8M37.5 × 0.5) were used and images acquired every 10 min for 48 h. Cameras were triggered by Basler’s pylon software suite (Version: 6.0.1). For single time points imaging after 24 h of growth on agar plates, a Canon EOS 1200D reflex camera was used. All images were analyzed with ColTapp, an automated image analysis application for efficient microbial colony growth dynamics quantification (doi: https://doi.org/10.1101/2020.05.27.119040). Appearance time was estimated as the time when a colony reached a radius of 200 μm. Colony growth delay after 1, 3, and 5 days of infection was defined as the difference of the appearance time of a colony compared to the mean appearance time after 4 h infection (day 0). Similarly, radius deviation was defined as the difference in radius of a colony compared to the mean radius at the 4 h infection time point. A colony was defined as a SCV if the area of a colony after 24 h regrowth was smaller than 1/5th of the median area of a colony of the 4 h infection time point after 24 h regrowth (Vulin et al., 2018). A total of 9,693 colonies were analyzed with automated time-lapse analysis and 23,716 colonies with single time point imaging of plates. Plates with less than 15 and more than 350 colonies were excludes in further analyses.
Confocal Microscopy
Fluorescent images of intracellular bacteria were taken with the confocal laser scanning microscope (CLSM) Leica SP8 inverse. Samples were fixed at the indicated time points in 4% paraformaldehyde (PFA). Eukaryotic nuclei and bacterial DNA were stained with Hoechst 33342 (Thermo Scientific), late endosomes and lysosomes were stained with 1 μg/ml mouse α-lysosome associated membrane protein 2 (Lamp-2) (BD Pharmigen) and S. aureus was stained with 2 μg/ml rabbit α-Staphylococcus aureus (EastCoast). As secondary antibodies, 2 μg/ml goat α-mouse Alexa 647 (Invitrogen) and 2 μg/ml goat α-rabbit Alexa 488 (Abcam) were used to label Lamp2 and S. aureus, respectively. To identify acidic compartments, 1 μM LysoTracker Red DND-99 (Invitrogen) was used. Images were deconvolved with Huygens Professional software and further processed with the image analysis software Imaris.
Flow Cytometry-Based pH Monitoring
To monitor intracellular pH, we adapted the method established by Sinha et al. (1999). Briefly, bacterial overnight cultures were pelleted and resuspended in sodium bicarbonate buffer, pH 9. S. aureus strains were labeled with 100 μg/ml fluorescein isothiocyanate (FITC) for 1 h at 37°C, shaking. Epithelial cells were infected at a MOI of 25 for 3 h, followed by 1, 3, or 5 days treatment with 1 mg/ml flucloxacillin and 20 μg/ml lysostaphin. Prior to flow cytometry, epithelial cells were trypsinized, pelleted and resuspended in PBS with 1:500 diluted aqua fluorescent reactive dye (Invitrogen) and 1 μM carbonyl cyanide m-chlorophenyl hydrazone (CCCP) for 15 min at 37°C. Quenching of FITC signal corresponded to an acidic pH. Total internalized bacteria treated with CCCP were calculated according to the formula: AFU = (mean fluorescence intensity BL1-A × percentage of cells in Q3)/1,000. Q3 is the quadrant negative for aqua, but positive for FITC signal. The difference in arbitrary fluorescence units (AFU) between samples treated with CCCP and untreated was calculated as an indirect readout for intracellular pH using the formula: ΔAFU=(AFU−CCCP-AFU+ CCCP)/(AFU+ CCCP× 10,000). Values close to zero indicated neutral pH and larger negative values indicated acidic pH. Positive values were considered as below detection limit and set to zero in the analysis. Samples were measured with the Attune NxT Acoustic Focusing Cytometer (Thermo Fisher Scientific) and analyzed using the software FlowJo.
Cell Viability Assay
To assess bacterial cytotoxicity, a MTT assay was performed, as previously described (Seidl and Zinkernagel, 2013). Epithelial cells were infected at a MOI of 1 as described above. One hour, 1, 3, and 5 days after invasion, the tetrazolium dye MTT (3-[4,5-dimethylthiazole-2-yl]-2,5-diphenyltetrazolium bromide) was added directly to the medium at a final concentration of 1 mg/ml and incubated for 2 h at 37°C and 5% CO2. Medium was aspirated and formazan crystals were dissolved in 0.04 M HCl in isopropanol. Absorbance was measured at 570 nm with the VERSAmax tunable microplate reader (Molecular Devices).
Lactate Dehydrogenase (LDH) Release
To monitor lysis of eukaryotic cells, epithelial cells were infected at a MOI of 1 as described above. Three hours and 24 h after infection cell supernatants were collected. Eukaryotic cell lysis was quantified using the CytoTox 96® Non-Radioactive Cytotoxicity Assay (Promega). Briefly, an equal volume of CytoTox 96® Reagent was added to the supernatant. After 30 min, the Stop Solution was added and absorbance was measured at 490 nm.
Statistical Analysis
All statistical analysis were conducted with R and R Studio Version 3.5.1 (R Core Team, 2018). Figures were produced with ggplot (Wickham, 2016) and ggbeeswarm (Clarke and Sherrill-Mix, 2017), pairwise comparisons were calculated based on estimated marginal means (emm) (Russell, 2018) and p-values were corrected for multiple testing based on Tukey’s or Sidak’s method. We separated the analysis of S. aureus strain Cowan from the analysis of the three wild type and agr mutant pairs. CFU/ml was log10-transformed and linear regression was used to assess the effect of the duration of infection for strain Cowan separately. For the other wild type and agr mutant strains a model incorporating the interaction between days of infection, strain and presence or absence of agr was additionally used. Following, emm pairwise comparisons between wild types and agr mutants averaging over time were calculated per strain. Number of SCV versus normal sized colonies per replicate were modeled with a generalized linear model (glm) based on a quasi-binomial distribution to account for overdispersion. We included the three-way interaction term between days of infection, strain and presence or absence of agr as well as all possible two-way interactions and single parameter effects. Emm pairwise differences were calculated to compare wild types and agr mutants per strain and for each day. Additionally, we investigated the effect of chloroquine treatment at day five (the untreated data was used in two separate analysis). Similarly, a glm with a quasi-binomial distribution and a three-way interaction term including sub-interactions of chloroquine treatment, strain and presence or absence of agr was used. Pairwise differences were calculated to compare wild types and agr mutants per strain and within chloroquine treatment. For strain Cowan, a simple glm with duration of infection as the only term was used. A linear regression model with the three-way interaction term between the three strains, presence or absence of agr and chloroquine treatment was used to analyze pH assay data at day five. Emm pairwise differences were calculated to compare wild types and agr mutants per strain and within chloroquine treatment. For strain Cowan, a simple linear regression with duration of infection as the only term was used.
Results
S. aureus Cowan Resides in an Acidic Environment Over a Period of Five Days
In a first step, we monitored intracellular survival of S. aureus strain Cowan over a period of 5 days within human lung epithelial cells A549. Throughout the infection, the number of intracellular bacteria significantly decreased (p < 0.0001). After 5 days, surviving bacteria decreased by four log, however, intracellular bacteria were not completely eradicated (Figure 1A). Bacteria recovered after 5 days showed a broad colony size heterogeneity (Figure 1B). The probability density function showed a peak of colony size deviation from the mean colony size at day 0 (Supplementary Figure 1) indicating a mean colony size reduction of 20 to 35 μm after 1 and 3 days intracellular survival with 0.3% ± 0.3 SEM and 3.7% ± 1.7 SEM SCVs, respectively. After 5 days, the colony size distribution became broader with a mean colony size reduction of 200 μm. We observed a significant increase in SCV formation from day one to day five (p = 0.017) as well as from day three to day five (p = 0.036) with 11% ± 0.7 SEM SCVs on day five (Figure 1B). The threshold for SCVs was set at 1/5th of the median colony area at day 0. To investigate whether colony size heterogeneity was linked to the exposure of acidic intracellular pH, we used pH dependent quenching of FITC-labeled bacteria as described by Sinha et al. (1999). Throughout the infection, low ΔAFU values were measured indicating exposure of S. aureus strain Cowan to an acidic environment (Figure 1C). Linear regression showed no evidence of a changing intracellular pH over time (p = 0.5835). Intracellular localization of surviving bacteria in an acidic compartment was additionally confirmed by confocal laser scanning microscopy (CLSM). Co-localization of bacteria with lysosome-associated membrane protein 2 (Lamp-2) and LysoTracker signal indicated the localization of bacteria in acidic endosomes (Figure 1D).
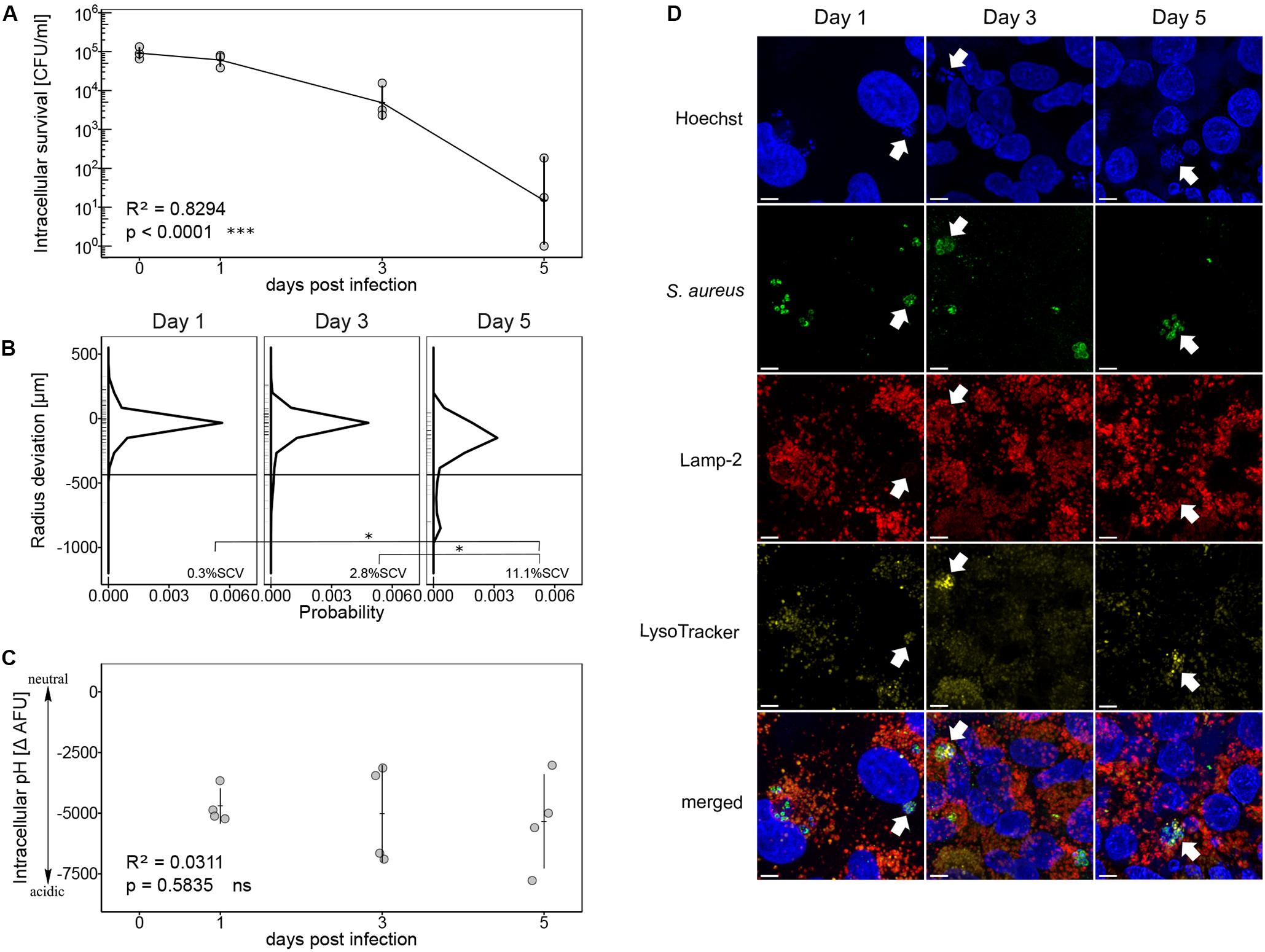
Figure 1. Intracellular survival of S. aureus strain Cowan in acidic endosomes. (A) Recovered bacteria are shown as CFU per ml in log scale over an infection period of 5 days. Three independent experiments in technical triplicates ± SEM are shown. (B) Colony size distribution of recovered bacteria. After 24 h regrowth, colony radius was normalized to the mean colony area at day 0 (Supplementary Figure 1). Radius size deviation from reference is plotted as probability density function. Individual colonies are shown as short lines on y-axis. The threshold for SCVs, indicated as a horizontal line, was calculated as 1/5th of the area of the median area at day 0. The mean percentage of SCVs is written in each panel. Colonies from single images as well as from time-lapse analysis were taken into account. For day one 607 colonies, for day three 362 colonies and for day five 84 colonies were analyzed in two to four biological repeats. Pairwise comparison of day one and day five ∗p = 0.0172, pairwise comparison of day three and day five ∗p = 0.0362. (C) Measurement of intracellular pH after 1, 3, and 5 days of infection. Four independent experiments in technical triplicates ± SEM are shown. (D) CLSM of intracellular S. aureus strain Cowan. Co-localization of S. aureus strain Cowan with Lamp-2-positive compartments and LysoTracker signal are indicated by arrows. Length of scale bar is 5 μm.
Intracellular Survival of S. aureus Wild Type Strains and Their Corresponding agr Mutants
S. aureus wild type strain Cowan shows a natural agr defective phenotype (Grundmeier et al., 2010) and resides in acidic compartments inside epithelial cells (Figure 1D). To evaluate whether agr deficiency or the survival inside acidic compartments contributed to the formation of SCVs, we analyzed a panel of S. aureus wild type strains and their isogenic agr knock out mutants (Table 1). S. aureus strain 6850 was included in this study because it has previously been shown to increase intracellular pH after 6 h of infection of HeLa cells and of human embryonic kidney cells 293 (Lam et al., 2010). JE2 is a highly virulent S. aureus strain representative of the methicillin-resistant USA300 lineage (Tenover and Goering, 2009) and SH1000 is a commonly used laboratory strain of the 8325 lineage (Horsburgh et al., 2002; O’Neill, 2010). Wild type strains and their agr mutants were tested for their ability to invade and reside inside human lung epithelial cells A549. S. aureus CFUs were recovered from eukaryotic cells 1, 3, and 5 days after infection. A significant decrease in intracellular survival over time was observed for all S. aureus strains (p < 0.0001) (Figure 2A). We further performed pairwise comparisons of S. aureus wild types and agr mutants and found that S. aureus wild type strains JE2 and 6850 showed a significant decrease in intracellular survival as compared to their corresponding agr mutants (p = 0.0005 and p = 0.0032, respectively). Although bacterial numbers recovered from epithelial cells decreased over time, neither agr mutants nor wild type strains were eradicated completely. Additionally, we monitored eukaryotic cell survival and cytotoxicity. S. aureus wild type strains showed a higher cytotoxicity as compared to their corresponding agr mutants. Despite the initial cell loss caused by S. aureus wild type strains within the first 24 h (Supplementary Figure 2A), eukaryotic cells showed recovery after 3 and 5 days (Supplementary Figure 2B).
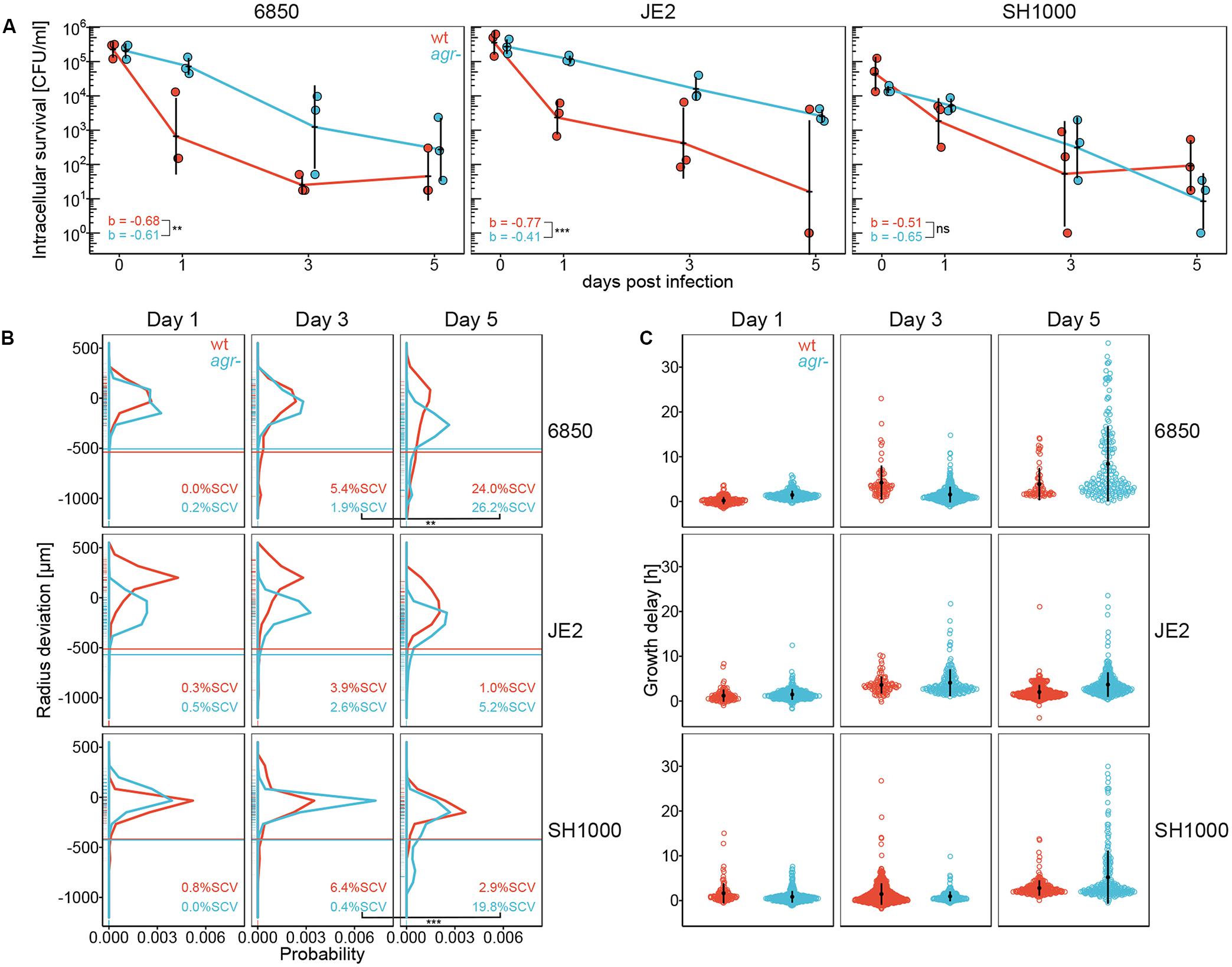
Figure 2. Intracellular survival, colony size distribution and lag time of S. aureus wild type strains (wt) and agr mutants (agr-). S. aureus wild type strains are indicated in red, agr mutants are indicated in blue. (A) Intracellular survival of S. aureus wild type strains and agr mutants in A549 cells. Recovered bacteria are shown as CFU per ml in log scale over an infection period of 5 days. Data points of wild types and agr mutants are offset on x-axis for easier readability. Three independent experiments in technical triplicates ± SEM are shown. **p = 0.0032, ***p = 0.0005. b indicates the slope of the curve. (B) Colony size distribution of recovered bacteria. After 24 h regrowth, colony radius is normalized to the strain specific mean colony area at day 0 (Supplementary Figure 1). Radius size deviation from reference is plotted as probability density function. Individual colonies are shown as short lines on y-axis. The threshold for SCVs, indicated as a horizontal line, was calculated as 1/5th of the area of the median area at day 0. The mean percentage of SCVs is written in each panel. Colonies from single images as well as from time-lapse analysis were taken into account. In total 11,926 colonies in two to seven biological repeats were analyzed. **p = 0.0024, ***p < 0.0001. (C) A subset of the colonies shown in B were monitored with time-lapse imaging on blood agar plates. Appearance time is normalized to the strain specific mean appearance time at day 0 (Supplementary Figure 7). Growth delay is plotted as deviation from reference in hours. Every point of the dot plot represents one colony. In total 3,715 colonies in two to four biological repeats were analyzed. Colony radius at 24 h of time-lapse analysis is included in Figure 2B.
Increased SCVs Due to a Delay in Colony Appearance After Intracellular Survival
The influence of intracellular survival on colony size heterogeneity was determined by quantifying colony radius on blood agar plates after 1, 3, and 5 days of infection. After 3 days of intracellular survival, 6850, JE2 and SH1000 wild type strains showed a higher percentage of SCVs as compared to their agr mutants (Figure 2B). This phenotype switched after 5 days of intracellular survival. All S. aureus agr mutants showed a broader colony size heterogeneity with higher percentage of SCVs as compared to their corresponding wild type strains 5 days after infection (Figure 2B and Supplementary Figure 3, left panel). Pairwise comparisons of wild types and agr mutants over time showed a significant increase in the percentage of SCVs in 6850 and SH1000 agr mutant strains from day three to day five (p = 0.0024 and p < 0.0001, respectively). Similar to the cell line A549 results, we observed a trend toward smaller colony sizes in S. aureus agr mutants as compared to S. aureus wild type strains after 5 days intracellular exposure in the fibroblast cell line BJ5-ta (Supplementary Figures 4A, 5A). Similar to SH1000 wild type strain, complementation of the SH1000 agr mutant resulted in lower SCV numbers as compared to the SH1000 agr mutant (Supplementary Figures 5B, 6C). We further investigated whether smaller colonies were linked to a delay in colony appearance as previously described using automated colony time-lapse analysis (Vulin et al., 2018). To observe differences in appearance time, radial colony growth was monitored and normalized to the mean appearance time at day 0 (Supplementary Figure 7). After 3 days of infection, S. aureus wild type strains 6850 and SH1000 showed more late appearing colonies as compared to their agr mutants, whereas the JE2 agr mutant showed more late appearing colonies than the JE2 wild type (Figure 2C). Similar to the phenotype switch observed in SCV formation from day three to day five, we observed more late appearing colonies for all agr mutants as compared to their corresponding wild type strains after 5 days of infection (Figure 2C). Based on these findings, we conclude that SCVs are linked to the delay in colony appearance.
Acidic Intracellular Milieu Affects Colony Size Heterogeneity of S. aureus Wild Type Strains
To elucidate the correlation between intracellular pH and agr deficiency, intracellular localization of S. aureus wild type strains and agr mutants was investigated by CLSM using LysoTracker to stain acidic compartments. We observed co-localization of LysoTracker signal with S. aureus wild type strains indicating their localization in acidic compartments, but no co-localization of LysoTracker with their respective agr mutants was identified (Figure 3A). We further quantified intracellular pH of infected eukaryotic cells by measuring quenching of FITC-labeled bacteria. Based on a three-way interaction model, we found that S. aureus wild type strains SH1000 and JE2 were exposed to a significantly lower intracellular pH represented as larger negative ΔAFU values as compared to their corresponding agr mutants (p = 0.049 and 0.024, respectively) 5 days after infection (Figure 3B). The same trend was observed for the 6850 wild type strain as compared to its agr mutant (p = 0.091). Similar to our observations in the cell line A549 we observed that S. aureus wild type strains resided in a more acidic intracellular environment as compared to their corresponding agr mutants in the fibroblast cell line BJ5-ta (Supplementary Figure 4B). Complementation of the SH1000 agr mutant resulted in exposure to lower pH, comparable to the ΔAFU values obtained from SH1000 wild type strain (Supplementary Figure 6D). The weak base chloroquine was used as an alkalizing agent to neutralize phagolysosomes. After 5 days of chloroquine treatment there was no significant difference in pH between wild type strains and agr mutants (Figure 3B). ΔAFU values for day one and day three are shown in Supplementary Figure 8. To assess whether intracellular pH influenced colony size heterogeneity and the formation of SCVs in S. aureus, colony radius was measured after 5 days of chloroquine treatment (Figure 3C). Percentage of SCVs and colony size distribution for day one and three after chloroquine treatment are shown in Supplementary Figure 3 (right panel) and Supplementary Figure 9, respectively. Addition of the alkalizing agent chloroquine resulted in significant decrease in percentage of SCVs in S. aureus wild type strain 6850 as compared to its agr mutant (p = 0.0209) after 5 days of infection (Figure 3C). An up to three-fold decrease in the percentage of SCVs was observed in S. aureus strains SH1000 and JE2 after chloroquine treatment.
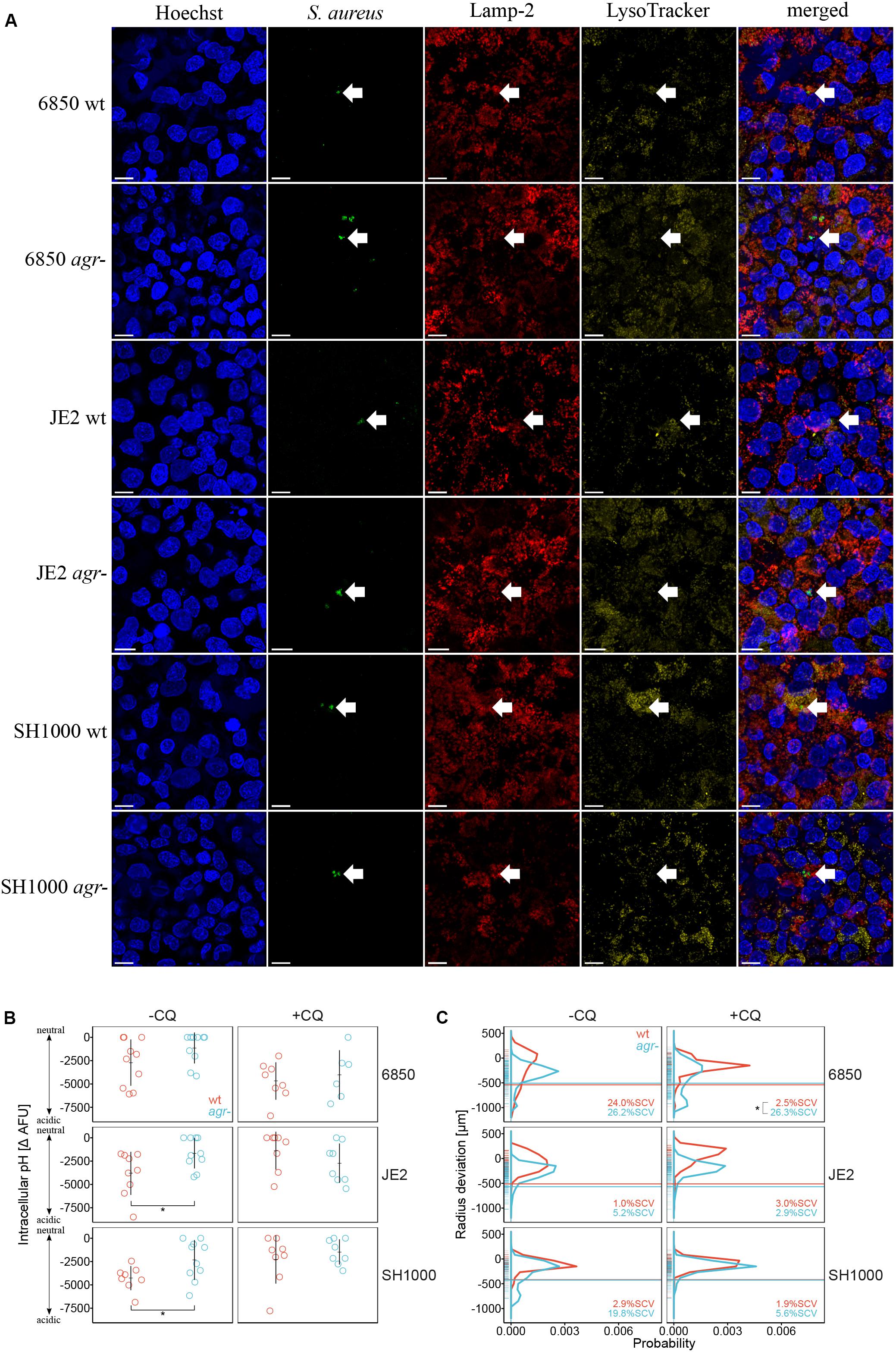
Figure 3. Acidic intracellular pH triggers SCV formation in S. aureus wild type strains. S. aureus wild type strains (wt) are indicated in red, agr mutants (agr-) are indicated in blue. (A) Intracellular localization of S. aureus strains 5 days after infection shown by arrows. Co-localization of S. aureus wild type strains with Lamp-2 and LysoTracker indicate the localization in acidic endosomes. Length of scale bar is 10 μm. (B) Measurement of intracellular pH after 5 days of infection. The alkalizing agent chloroquine (CQ) was used to neutralize phagolysosomes. −CQ indicates no treatment, +CQ indicates chloroquine treatment. Eight to ten independent experiments in technical triplicates ± SEM are shown. Pairwise comparison of JE2 wt and agr- *p = 0.0243, pairwise comparison of SH1000 wt and agr- *p = 0.0486. (C) Comparison of colony size distribution of untreated (−CQ) and chloroquine treated (+CQ) bacteria recovered after 5 days of infection. After 24 h regrowth, colony radius is normalized to the strain specific mean colony area at day 0 (Supplementary Figure 1). Radius size deviation from reference is plotted as probability. The threshold for SCVs, indicated as a horizontal line, was calculated as 1/5th of the area of the median area at day 0. The mean percentage of SCVs is written in each panel. Colonies from single images as well as from time-lapse analysis were taken into account. Colony radius deviation of untreated bacteria is also shown in Figure 2B. In total 9,381 colonies (4,387 untreated and 4,994 chloroquine treated) in two to five biological repeats were analyzed. *p = 0.0209.
Discussion
We found that S. aureus agr knock out strains showed increased intracellular survival and higher SCV numbers after recovery from intracellular exposure as compared to S. aureus wild type strains. Colony size heterogeneity of agr mutants was independent from intracellular pH, whereas an acidic intracellular pH was necessary to trigger SCV formation in wild type strains.
S. aureus wild type strains JE2 and 6850 showed a significant decrease in intracellular survival as compared to their corresponding agr mutants. It has previously been shown that an isogenic agr mutant of JE2 survives at higher rates inside HeLa cells for 24 h as compared to JE2 wild type strain (Munzenmayer et al., 2016). To our knowledge, the effect of prolonged intracellular exposure and its influence on colony size heterogeneity leading to SCV formation in a panel of S. aureus wild type strains and their respective isogenic agr mutants has not been studied in detail so far. A functional agr facilitates bacteria to express phenol soluble modulins (PSMs) among which PSMα acts as a pore-forming toxin to promote escape from endosomes (Schnaith et al., 2007; Giese et al., 2011; Grosz et al., 2014; Strobel et al., 2016). Once in the cytosol, the bacterium is able to multiply and eventually disrupt the host cell (Fraunholz and Sinha, 2012). We and others (Haslinger-Löffler et al., 2006; Strobel et al., 2016; Seidl et al., 2017) previously showed that S. aureus strains JE2 and 6850 are more cytotoxic than Cowan. In general, bacterial cytotoxicity is highly MOI-dependent. As shown by the LDH release assay, JE2 and 6850 efficiently lysed the eukaryotic cells and thus escaped the host cell reaching the medium containing lysostaphin and flucloxacillin, which killed the bacteria. This explains the drastic decrease in CFU counts for S. aureus wild type strains JE2 and 6850. Despite the different rates of CFU reduction, a small population of both S. aureus wild types and agr mutants resided intracellularly up to 5 days after infection. Tuchscherr and colleagues observed intracellular survival of S. aureus strain 6850 even after 28 days of infection of A549 cells (Tuchscherr et al., 2011). The phenotypically agr defective S. aureus strain Cowan showed comparable survival rates to the agr mutants of JE2 and 6850. However, S. aureus wild type strain SH1000 showed similar intracellular survival as its agr mutant. SH1000 carries a 63 bp deletion in the spa-sarS intergenic region that leads to a 75% decrease in the SarS activator steady state level, which is known to influence agr activity (Cheung et al., 2001; Blevins et al., 2002; Oscarsson et al., 2006; Baek et al., 2013). Proteomics analysis of S. aureus strain SH1000 by Strobel and colleagues revealed low levels of hemolysins, PSMs and other toxins under agr control, suggesting a reduced agr activity in SH1000 as compared to other S. aureus wild type strains including 6850 and a USA300 strain (Strobel et al., 2016). The reduced agr activity is a potential explanation for the similarities between SH1000 wild type and its agr mutant regarding intracellular survival. A review by Löffler et al. (2014) summarizes S. aureus intracellular survival inside different cell lines. They conclude that intracellular localization imposes a selective pressure for slow growing bacteria referred to as SCVs. Throughout the infection, we observed increasing colony size heterogeneity in all S. aureus strains tested. After 3 days of infection, S. aureus wild type strains 6850 and SH1000 showed a higher percentage of SCVs as compared to their agr mutants. We investigated colony size heterogeneity in more detail by analyzing colony’s first appearance on blood agar plates and found that the SCV numbers reflected the number of late appearing colonies. Our group previously showed that small colony sizes are indirectly proportional to the delay in colony appearance (Vulin et al., 2018). However, after 5 days of infection, this phenotype switched as agr mutants of JE2, 6850 and SH1000 showed an even broader colony size distribution with higher percentage of SCVs as compared to their corresponding wild type strains. The earlier onset of SCV formation observed for S. aureus wild type strains might be due to the additional pressure of residing in an acidic intracellular environment as compared to S. aureus agr mutants, which were exposed to a less acidic environment. We showed that 6850 and SH1000 wild type strains were exposed to a slightly more acidic intracellular environment as compared to their respective agr mutants already after 1 and 3 days of intracellular survival. Therefore, S. aureus wild type strains were exposed to an acidic milieu for the whole infection period of 5 days. Additionally, CFU numbers of recovered S. aureus wild type strains showed a three log reduction after 3 days of intracellular survival whereas CFU numbers of S. aureus agr mutants only decreased by one log. High numbers of normally growing colonies might mask the population of SCVs. This possibly explains the higher proportion of SCVs in S. aureus wild type strains as compared to their corresponding agr mutants at day three. The factors and mechanism causing this switch in SCV numbers between day three and day five need to be further studied in detail. The increase in SCV formation in S. aureus agr mutants was also reflected by an increase of late appearing colonies 5 days after infection. Intracellular survival and exposure to acidic pH have been shown to trigger the formation of SCVs (Tuchscherr et al., 2011; Leimer et al., 2016; Vulin et al., 2018). Endosomal maturation is characterized by a drop in pH (Maxfield and Yamashiro, 1987; Huotari and Helenius, 2011), therefore phagocytosed bacteria are likely exposed to an acidic pH inside late endosomes. To check whether acidic pH or dysfunctional agr triggers SCV formation, we monitored intracellular pH of S. aureus wild type strains and their agr mutants. We found that JE2 and SH1000 wild type strains were exposed to significantly lower intracellular pH as compared to their corresponding agr mutants 5 days after infection. The same trend toward exposure to more acidic environment was also observed for 6850 wild type strain as compared to its agr mutant. In this study, Cowan is the only strain with a defective agr system that resided in an acidic compartment over the entire infection period of 5 days. In contrast to the agr mutants of 6850, JE2 and SH1000, in which the complete agr system is knocked out and replaced with a tetracycline resistance cassette, the agr system of Cowan is still present in the genome. It was shown by Grundmeier et al. (2010), that Cowan expresses lower levels of hla and agrA as compared to the S. aureus strain 6850 suggesting an agr dysfunction. Nevertheless, baseline levels of agr expression might be sufficient to observe exposure to low intracellular pH comparable to other S. aureus wild type strains. The exposure to acidic intracellular pH resulted in larger colony size heterogeneity and an increased percentage of SCVs. The weak base chloroquine, routinely used for treatment of malaria and rheumatic diseases, was used to alkalize acidic phagolysosomes (Leimer et al., 2016). Alkalization resulted in a nine-fold reduction of SCVs in S. aureus wild type strain 6850, but no reduction in percentage of SCVs was observed in its corresponding agr mutant. Since S. aureus agr mutants were already exposed to a neutral environment inside the host cell, the neutralizing effect of chloroquine on SCV formation was not as strong as compared to the effect on SCV formation in wild type strains. We conclude that an acidic intracellular environment facilitates SCV formation in S. aureus wild type strains. In contrast, agr mutants are able to form high numbers of SCVs in a pH independent manner. These results suggest that a lack of agr regulation promotes the formation of SCVs in the intracellular environment. It was shown by Arvidson and Holme (1971) and by Weinrick et al. (2004) that post-exponential induction of agr-dependent genes is reduced in a nutrient rich media buffered to acidic pH. Therefore, the acidic intracellular milieu and the intracellular environment that S. aureus wild type strains are exposed to, might affect the regulation of the agr system, which in turn could contribute toward the formation of SCVs.
Our findings give more insights into the interplay between intracellular survival, agr function and intracellular pH contributing toward SCV formation. This will allow designing new therapeutic strategies aiming to improve therapy of chronic S. aureus infections in the future.
Data Availability Statement
The datasets generated for this study are available on request to the corresponding author.
Author Contributions
AZ, SM, and NH conceived and planned the experiments. NH carried out the experiments. KS created agr knockout mutants in S. aureus strains JE2 and 6850. HC and AH created the construct for agr complementation and revised the manuscript. JB analyzed the time-lapse data, did the statistical analysis and revised the manuscript. NH, VD, SM, and AZ wrote and revised the manuscript. All authors contributed to the article and approved the submitted version.
Funding
The study was funded by the Swiss National Science Foundation grant 31003A_176252 to AZ. SM was supported by a grant from the Swedish Society for Medical Research (SSMF, P17-0179). AH was supported by a Merit Award (BX002711) from the Department of Veteran Affairs.
Conflict of Interest
The authors declare that the research was conducted in the absence of any commercial or financial relationships that could be construed as a potential conflict of interest.
Acknowledgments
Confocal imaging was performed with support of the Center for Microscopy and Image Analysis, University of Zurich. The following reagent was provided by the Network on Antimicrobial Resistance in Staphylococcus aureus (NARSA) for distribution by BEI Resources, NIAID, NIH: Staphylococcus aureus subsp. aureus, Strain JE2, NR-46543.
Supplementary Material
The Supplementary Material for this article can be found online at: https://www.frontiersin.org/articles/10.3389/fmicb.2020.01415/full#supplementary-material
References
Altman, D. R., Sullivan, M. J., Chacko, K. I., Balasubramanian, D., Pak, T. R., Sause, W. E., et al. (2018). Genome plasticity of agr-defective Staphylococcus aureus during clinical infection. Infect. Immun. 86:e00331-18. doi: 10.1128/IAI.00331-18
Arvidson, S., and Holme, T. (1971). Influence of pH on the formation of extracellular proteins by Staphylococcus aureus. Acta Pathol. Microbiol. Scand. B Microbiol. Immunol. 79, 406–413. doi: 10.1111/j.1699-0463.1971.tb00080.x
Baek, K. T., Frees, D., Renzoni, A., Barras, C., Rodriguez, N., Manzano, C., et al. (2013). Genetic variation in the Staphylococcus aureus 8325 strain lineage revealed by whole-genome sequencing. PLoS One 8:e77122. doi: 10.1371/journal.pone.0077122
Balwit, J. M., van Langevelde, P., Vann, J. M., and Proctor, R. A. (1994). Gentamicin-resistant menadione and hemin auxotrophic Staphylococcus aureus persist within cultured endothelial cells. J. Infect. Dis. 170, 1033–1037.
Blevins, J. S., Beenken, K. E., Elasri, M. O., Hurlburt, B. K., and Smeltzer, M. S. (2002). Strain-dependent differences in the regulatory roles of sarA and agr in Staphylococcus aureus. Infect. Immun. 70, 470–480. doi: 10.1128/iai.70.2.470-480.2002
Bohacek, J., Kocur, M., and Martinec, T. (1971). Deoxyribonucleic acid base composition of serotype strains of Staphylococcus aureus. J. Gen. Microbiol. 68, 109–113. doi: 10.1099/00221287-68-1-109
Bosse, M. J., Gruber, H. E., and Ramp, W. K. (2005). Internalization of bacteria by osteoblasts in a patient with recurrent, long-term osteomyelitis. A case report. J. Bone Joint Surg. Am. 87, 1343–1347. doi: 10.2106/jbjs.d.02649
Cheung, A. L., Schmidt, K., Bateman, B., and Manna, A. C. (2001). SarS, a SarA homolog repressible by agr, is an activator of protein A synthesis in Staphylococcus aureus. Infect. Immun. 69, 2448–2455. doi: 10.1128/iai.69.4.2448-2455.2001
Clarke, E., and Sherrill-Mix, S. (2017). Ggbeeswarm: Categorical scatter (violin point) Plots (Version 0.6. 0).
Clement, S., Vaudaux, P., Francois, P., Schrenzel, J., Huggler, E., Kampf, S., et al. (2005). Evidence of an intracellular reservoir in the nasal mucosa of patients with recurrent Staphylococcus aureus rhinosinusitis. J. Infect. Dis. 192, 1023–1028.
DeLeo, F. R., Kennedy, A. D., Chen, L., Bubeck Wardenburg, J., Kobayashi, S. D., Mathema, B., et al. (2011). Molecular differentiation of historic phage-type 80/81 and contemporary epidemic Staphylococcus aureus. Proc. Natl. Acad. Sci. U.S.A. 108, 18091–18096. doi: 10.1073/pnas.1111084108
Fisher, R. A., Gollan, B., and Helaine, S. (2017). Persistent bacterial infections and persister cells. Nat. Rev. Microbiol. 15, 453–464. doi: 10.1038/nrmicro.2017.42
Fraunholz, M., and Sinha, B. (2012). Intracellular Staphylococcus aureus: live-in and let die. Front. Cell Infect. Microbiol. 2:43. doi: 10.3389/fcimb.2012.00043
Giese, B., Glowinski, F., Paprotka, K., Dittmann, S., Steiner, T., Sinha, B., et al. (2011). Expression of delta-toxin by Staphylococcus aureus mediates escape from phago-endosomes of human epithelial and endothelial cells in the presence of beta-toxin. Cell Microbiol. 13, 316–329. doi: 10.1111/j.1462-5822.2010.01538.x
Grosz, M., Kolter, J., Paprotka, K., Winkler, A. C., Schafer, D., Chatterjee, S. S., et al. (2014). Cytoplasmic replication of Staphylococcus aureus upon phagosomal escape triggered by phenol-soluble modulin alpha. Cell Microbiol. 16, 451–465. doi: 10.1111/cmi.12233
Grundmeier, M., Tuchscherr, L., Bruck, M., Viemann, D., Roth, J., Willscher, E., et al. (2010). Staphylococcal strains vary greatly in their ability to induce an inflammatory response in endothelial cells. J. Infect. Dis. 201, 871–880. doi: 10.1086/651023
Haslinger-Löffler, B., Wagner, B., Bruck, M., Strangfeld, K., Grundmeier, M., Fischer, U., et al. (2006). Staphylococcus aureus induces caspase-independent cell death in human peritoneal mesothelial cells. Kidney Int. 70, 1089–1098.
Horsburgh, M. J., Aish, J. L., White, I. J., Shaw, L., Lithgow, J. K., and Foster, S. J. (2002). sigmaB modulates virulence determinant expression and stress resistance: characterization of a functional rsbU strain derived from Staphylococcus aureus 8325-4. J. Bacteriol. 184, 5457–5467. doi: 10.1128/jb.184.19.5457-5467.2002
Huotari, J., and Helenius, A. (2011). Endosome maturation. EMBO J. 30, 3481–3500. doi: 10.1038/emboj.2011.286
Kahl, B. C., Belling, G., Becker, P., Chatterjee, I., Wardecki, K., Hilgert, K., et al. (2005). Thymidine-dependent Staphylococcus aureus small-colony variants are associated with extensive alterations in regulator and virulence gene expression profiles. Infect. Immun. 73, 4119–4126. doi: 10.1128/iai.73.7.4119-4126.2005
Laabei, M., Uhlemann, A. C., Lowy, F. D., Austin, E. D., Yokoyama, M., Ouadi, K., et al. (2015). Evolutionary Trade-offs underlie the multi-faceted virulence of Staphylococcus aureus. PLoS Biol. 13:e1002229. doi: 10.1371/journal.pbio.1002229
Lam, T. T., Giese, B., Chikkaballi, D., Kuhn, A., Wolber, W., Pane-Farre, J., et al. (2010). Phagolysosomal integrity is generally maintained after Staphylococcus aureus invasion of nonprofessional phagocytes but is modulated by strain 6850. Infect. Immun. 78, 3392–3403. doi: 10.1128/iai.00012-10
Leimer, N., Rachmuhl, C., Palheiros Marques, M., Bahlmann, A. S., Furrer, A., Eichenseher, F., et al. (2016). Nonstable Staphylococcus aureus small-colony variants are induced by low ph and sensitized to antimicrobial therapy by phagolysosomal alkalinization. J. Infect. Dis. 213, 305–313. doi: 10.1093/infdis/jiv388
Libraty, D. H., Patkar, C., and Torres, B. (2012). Staphylococcus aureus reactivation osteomyelitis after 75 years. N. Engl. J. Med. 366, 481–482. doi: 10.1056/nejmc1111493
Löffler, B., Tuchscherr, L., Niemann, S., and Peters, G. (2014). Staphylococcus aureus persistence in non-professional phagocytes. Int. J. Med. Microbiol. 304, 170–176. doi: 10.1016/j.ijmm.2013.11.011
Luong, T. T., and Lee, C. Y. (2007). Improved single-copy integration vectors for Staphylococcus aureus. J. Microbiol. Methods 70, 186–190. doi: 10.1016/j.mimet.2007.04.007
Maxfield, F. R., and Yamashiro, D. J. (1987). Endosome acidification and the pathways of receptor-mediated endocytosis. Adv. Exp. Med. Biol. 225, 189–198. doi: 10.1007/978-1-4684-5442-0_16
Mitchell, G., Fugere, A., Pepin Gaudreau, K., Brouillette, E., Frost, E. H., Cantin, A. M., et al. (2013). SigB is a dominant regulator of virulence in Staphylococcus aureus small-colony variants. PLoS One 8:e65018. doi: 10.1371/journal.pone.0065018
Moisan, H., Brouillette, E., Jacob, C. L., Langlois-Begin, P., Michaud, S., and Malouin, F. (2006). Transcription of virulence factors in Staphylococcus aureus small-colony variants isolated from cystic fibrosis patients is influenced by SigB. J. Bacteriol. 188, 64–76. doi: 10.1128/jb.188.1.64-76.2006
Munzenmayer, L., Geiger, T., Daiber, E., Schulte, B., Autenrieth, S. E., Fraunholz, M., et al. (2016). Influence of Sae-regulated and Agr-regulated factors on the escape of Staphylococcus aureus from human macrophages. Cell Microbiol. 18, 1172–1183. doi: 10.1111/cmi.12577
Novick, R. P., Ross, H. F., Projan, S. J., Kornblum, J., Kreiswirth, B., and Moghazeh, S. (1993). Synthesis of staphylococcal virulence factors is controlled by a regulatory RNA molecule. EMBO J. 12, 3967–3975. doi: 10.1002/j.1460-2075.1993.tb06074.x
O’Neill, A. J. (2010). Staphylococcus aureus SH1000 and 8325-4: comparative genome sequences of key laboratory strains in staphylococcal research. Lett. Appl; Microbiol. 51, 358–361. doi: 10.1111/j.1472-765x.2010.02885.x
Oscarsson, J., Kanth, A., Tegmark-Wisell, K., and Arvidson, S. (2006). SarA is a repressor of hla (alpha-hemolysin) transcription in Staphylococcus aureus: its apparent role as an activator of hla in the prototype strain NCTC 8325 depends on reduced expression of sarS. J. Bacteriol. 188, 8526–8533. doi: 10.1128/jb.00866-06
Petti, C. A., and Fowler, V. G. Jr. (2002). Staphylococcus aureus bacteremia and endocarditis. Infect. Dis. Clin. North Am. 16, 413–435.
Proctor, R. A., Balwit, J. M., and Vesga, O. (1994). Variant subpopulations of Staphylococcus aureus as cause of persistent and recurrent infections. Infect. Agents Dis. 3, 302–312.
Proctor, R. A., von Eiff, C., Kahl, B. C., Becker, K., McNamara, P., Herrmann, M., et al. (2006). Small colony variants: a pathogenic form of bacteria that facilitates persistent and recurrent infections. Nat. Rev. Microbiol. 4, 295–305. doi: 10.1038/nrmicro1384
Rollin, G., Tan, X., Tros, F., Dupuis, M., Nassif, X., Charbit, A., et al. (2017). Intracellular survival of Staphylococcus aureus in endothelial cells: a matter of growth or persistence. Front. Microbiol. 8:1354. doi: 10.3389/fmicb.2017.01354
Russell (2018). L %J R Package Version. Emmeans: Estimated Marginal Means, Aka Least-Squares Means. 1.
Schnaith, A., Kashkar, H., Leggio, S. A., Addicks, K., Kronke, M., and Krut, O. (2007). Staphylococcus aureus subvert autophagy for induction of caspase-independent host cell death. J. Biol. Chem. 282, 2695–2706. doi: 10.1074/jbc.m609784200
Schweizer, M. L., Furuno, J. P., Sakoulas, G., Johnson, J. K., Harris, A. D., Shardell, M. D., et al. (2011). Increased mortality with accessory gene regulator (agr) dysfunction in Staphylococcus aureus among bacteremic patients. Antimicrob. Agents Chemother. 55, 1082–1087. doi: 10.1128/aac.00918-10
Seidl, K., Leemann, M., Palheiros Marques, M., Rachmuhl, C., Leimer, N., Andreoni, F., et al. (2017). High level methicillin resistance correlates with reduced Staphylococcus aureus endothelial cell damage. Int. J. Med. Microbiol. 307, 11–20. doi: 10.1016/j.ijmm.2016.11.009
Seidl, K., and Zinkernagel, A. S. (2013). The MTT assay is a rapid and reliable quantitative method to assess Staphylococcus aureus induced endothelial cell damage. J. Microbiol. Methods 92, 307–309. doi: 10.1016/j.mimet.2012.12.018
Sendi, P., and Proctor, R. A. (2009). Staphylococcus aureus as an intracellular pathogen: the role of small colony variants. Trends Microbiol. 17, 54–58. doi: 10.1016/j.tim.2008.11.004
Shopsin, B., Drlica-Wagner, A., Mathema, B., Adhikari, R. P., Kreiswirth, B. N., and Novick, R. P. (2008). Prevalence of agr dysfunction among colonizing Staphylococcus aureus strains. J. Infect. Dis. 198, 1171–1174.
Sinha, B., Francois, P. P., Nusse, O., Foti, M., Hartford, O. M., Vaudaux, P., et al. (1999). Fibronectin-binding protein acts as Staphylococcus aureus invasin via fibronectin bridging to integrin alpha5beta1. Cell Microbiol. 1, 101–117. doi: 10.1046/j.1462-5822.1999.00011.x
Soong, G., Paulino, F., Wachtel, S., Parker, D., Wickersham, M., Zhang, D., et al. (2015). Methicillin-resistant Staphylococcus aureus adaptation to human keratinocytes. MBio 6:e00289-15. doi: 10.1128/mBio.00289-15
Strobel, M., Pfortner, H., Tuchscherr, L., Volker, U., Schmidt, F., Kramko, N., et al. (2016). Post-invasion events after infection with Staphylococcus aureus are strongly dependent on both the host cell type and the infecting S. aureus strain. Clin. Microbiol. Infect. 22, 799–809. doi: 10.1016/j.cmi.2016.06.020
Tenover, F. C., and Goering, R. V. (2009). Methicillin-resistant Staphylococcus aureus strain USA300: origin and epidemiology. J. Antimicrob. Chemother. 64, 441–446. doi: 10.1093/jac/dkp241
Tuchscherr, L., Medina, E., Hussain, M., Volker, W., Heitmann, V., Niemann, S., et al. (2011). Staphylococcus aureus phenotype switching: an effective bacterial strategy to escape host immune response and establish a chronic infection. EMBO Mol. Med./ 3, 129–141. doi: 10.1002/emmm.201000115
Vulin, C., Leimer, N., Huemer, M., Ackermann, M., and Zinkernagel, A. S. (2018). Prolonged bacterial lag time results in small colony variants that represent a sub-population of persisters. Nat. Commun. 9:4074.
Weinrick, B., Dunman, P. M., McAleese, F., Murphy, E., Projan, S. J., Fang, Y., et al. (2004). Effect of mild acid on gene expression in Staphylococcus aureus. J. Bacteriol. 186, 8407–8423. doi: 10.1128/jb.186.24.8407-8423.2004
Welsh, K. J., Skrobarcek, K. A., Abbott, A. N., Lewis, C. T., Kruzel, M. C., Lewis, E. M., et al. (2011). Predictors of relapse of methicillin-resistant Staphylococcus aureus bacteremia after treatment with vancomycin. J. Clin. Microbiol. 49, 3669–3672. doi: 10.1128/jcm.05287-11
Keywords: Staphylococcus aureus, small colony variants, intracellular survival, agr system, intracellular pH
Citation: Häffner N, Bär J, Dengler Haunreiter V, Mairpady Shambat S, Seidl K, Crosby HA, Horswill AR and Zinkernagel AS (2020) Intracellular Environment and agr System Affect Colony Size Heterogeneity of Staphylococcus aureus. Front. Microbiol. 11:1415. doi: 10.3389/fmicb.2020.01415
Received: 18 January 2020; Accepted: 02 June 2020;
Published: 30 June 2020.
Edited by:
Ying Zhang, Johns Hopkins University, United StatesReviewed by:
Xiancai Rao, Army Medical University, ChinaNadia Berkova, Institut National de la Recherche Agronomique (INRA), France
Copyright © 2020 Häffner, Bär, Dengler Haunreiter, Mairpady Shambat, Seidl, Crosby, Horswill and Zinkernagel. This is an open-access article distributed under the terms of the Creative Commons Attribution License (CC BY). The use, distribution or reproduction in other forums is permitted, provided the original author(s) and the copyright owner(s) are credited and that the original publication in this journal is cited, in accordance with accepted academic practice. No use, distribution or reproduction is permitted which does not comply with these terms.
*Correspondence: Annelies S. Zinkernagel, annelies.zinkernagel@usz.ch
†ORCID: Nicola Häffner, orcid.org/0000-0002-9159-5825; Julian Bär, orcid.org/0000-0003-1929-0338; Vanina Dengler Haunreiter, orcid.org/0000-0002-0850-0363; Srikanth Mairpady Shambat, orcid.org/0000-0002-0527-3978; Kati Seidl, orcid.org/0000-0002-2484-6364; Heidi A. Crosby, orcid.org/0000-0002-0360-0779; Alexander R. Horswill, orcid.org/0000-0002-5568-0096; Annelies S. Zinkernagel, orcid.org/0000-0003-4700-1118