- 1Unidad de Servicios Analíticos y Metrológicos, Centro de Investigación y Asistencia en Tecnología y Diseño del Estado de Jalisco, Guadalajara, Mexico
- 2Departamento de Tecnología Ambiental, Centro de Investigación y Asistencia en Tecnología y Diseño del Estado de Jalisco, Guadalajara, Mexico
- 3Tecnologico de Monterrey, Escuela de Ingenieria y Ciencias, Zapopan, Mexico
- 4Facultad de Ciencias Químico Biológicas, Universidad Autónoma de Sinaloa, Culiacán Rosales, Mexico
- 5Departamento de Ecología y Recursos Naturales, Universidad de Guadalajara, Autlán de Navarro, Mexico
This study investigated the prevalence, serovar distribution, antimicrobial resistance, and pulsed field gel electrophoresis (PFGE) typing of Salmonella enterica isolated from Lake Zapotlán, Jalisco, Mexico. Additionally, the association of the presence of Salmonella with physicochemical and environmental parameters was analyzed using Pearson correlation analysis and principal component analysis (PCA). Salmonella spp. were identified in 19 of 63 (30.15%) samples. The prevalence of Salmonella was positively correlated with air temperature, electrical conductivity, pH, and dissolved oxygen and negatively correlated with relative humidity, water temperature, turbidity, and precipitation. The predominant serotype identified was Agona (68.48%), followed by Weltevreden (5.26%), Typhimurium (5.26%), and serogroup B (21.05%). Overall, the highest detected antimicrobial resistance was toward colistin (73.68%), followed by sulfamethoxazole (63.15%), tetracycline (57.89%), nalidixic acid (52.63%), and trimethoprim (52.63%). All Salmonella strains were genetically diverse, with a total of 11 XbaI and four BlnI profiles on PFGE. The use of these two enzymes allowed differentiate strains of Salmonella of the same serotype. The results obtained in this study contribute to a better understanding of the Salmonella spp. ecology in an endorheic subtropical lake and provide information for decision makers to propose and implement effective strategies to control point and non-point sources of pathogen contamination.
Introduction
Among the important lakes in Mexico, Lake Zapotlán was recognized by the Convention on Wetlands as the 1466 Ramsar site in 2005. Hence, this lake acquired a status of national action and international cooperation for the conservation and rational use of its wetlands and resources, to recognize its significant value not only for the country, but for humanity (RAMSAR, 2007). This category was awarded because the lake basin is rich in plant and animal species (Comisión Nacional de Áreas Naturales Protegidas [CONANP], 2005). The lake also hosts important migratory waterfowl in the wetland areas that surround the lake (Shear and de Anda, 2009). Furthermore, it represents an important regional water resource for agricultural purposes, small scale fish harvesting, aquatic sports, and other recreational activities (Secretaría de Medio Ambiente y Desarrollo Territorial, 2005, 2013).
The main sources of water pollution in Lake Zapotlán are the scant treatment of municipal sewages discharged by wastewater treatment plants located in the municipalities of Ciudad Guzman (≈225 L/s) and Gomez Farias (≈30 L/s; Comisión Estatal del Agua [CEA], 2015a,b). Additionally, organic and nutrient contamination reaches the lake from agricultural fields and cattle farms settled around the lake, as well as the eroded material dragged down due to accelerated basin deforestation (Ortíz-Jiménez et al., 2005, 2006). In recent years, several national and international companies have dramatically changed the landscape, with extensive deforestation for the industrial use of wood and to introduce avocado and berries plantations (García de Alba-García, 2006). Moreover, rainwater runoff from the entire basin carries other waste from clandestine or poorly regulated urban landfills have contributed to the lake water quality degradation (Diputados Ciudadanos, 2016). Lake Zapotlán presents endorheic features; in other words, it is in an area of land in which the water that falls or runs through that place has no outlet to another river basin, nor to the sea, nor by infiltration intro layers of groundwater (Comisión Nacional de Áreas Naturales Protegidas [CONANP], 2005; Secretaría de Medio Ambiente y Desarrollo Territorial, 2005). Due to these features, the sources of pollution of this lake severely affect the quality of water used for productive and recreational purposes, a factor that increases the risk for human disease through the consumption of contaminated food or thorough contact with the water source (Greenberg et al., 2008; Malczyk and Branfireun, 2015).
Salmonella enterica represent zoonotic bacteria that are distributed throughout the world; they include many serotypes with different host specificity and the apparent ability to cause disease in those hosts is also serovar dependent (Andino and Hanning, 2015). S. enterica is one of the main causes of foodborne diseases, causing gastrointestinal diseases, and is also the etiological agent of more serious systematic diseases such as typhoid and paratyphoid fever (Gal-Mor et al., 2014; Pond and World Health Organization [WHO], 2005). In Mexico, there has been high morbidity associated with salmonellosis, with a total of 129,002 cases in 2012 (Dirección General de Epidemiología [DGEPI], 2012). In Jalisco State, there has been an increase in the number of reported salmonellosis cases, with 76,855 in 2016 and 91,173 in 2017 (Dirección General de Epidemiología [DGEPI], 2016, 2017).
Salmonella is present in marine and fresh environmental surface waters (Heinitz et al., 2000; Crim et al., 2014), but acute contamination of these environments may come from one or multiple routes, such as wastewater treatment plant discharges, urban and/or agricultural runoff pollution, overburdened septic systems, or contact with local and migratory fauna (Borchardt et al., 2003; Brands et al., 2005; Callaway et al., 2005). Due to the persistence of Salmonella in environmental waters, there might be a greater public health concern than previously thought. In some cases, growth outside host organisms leading to the probability of survival between hosts (Cherry et al., 1972; Winfield and Groisman, 2003). In line with Salmonella water pollution, surface waters used for irrigation have been identified as a source of contamination of horticultural crops related to outbreaks, including some in Mexico (Centers for Disease Control and Prevention [CDC], 2008, 2013, 2019). Raw or minimally processed vegetables may be contaminated with Salmonella, with subsequent direct infection of consumers or cross-contamination of other foodstuffs; which could represent a severe health risk (Quiroz-Santiago et al., 2009).
There is a wide variety of stressors to which Salmonella shows a high degree of resistance. Hence, they have a great capacity to persist in changing environments (Winfield and Groisman, 2003), including solar radiation, nutrient starvation, temperature variations, and pH changes, among others. All of these factors influence the survival, persistence, and distribution of these pathogenic bacteria (Xu et al., 1982; Tholozan et al., 1999; Gupte et al., 2003; Cook and Bolster, 2007; McEgan et al., 2013). Rainfall is positively correlated with cases of Salmonella infection in subtropical and tropical regions (Zhang et al., 2010). Particularly intense rainfall events may affect the prevalence and degree of contamination by these bacteria, increasing the transport of Salmonella from point and non-point sources to superficial water sources (Simental and Martinez-Urtaza, 2008; Zhang et al., 2010). However, relationships between the presence of Salmonella in the environment with physicochemical and environmental factors are not yet fully understood.
Phenotypic and genotypic methods are required to determine the origin of the contamination of pathogenic microorganisms in a specific habitat; these techniques allow the identification and characterization of microorganism (Scott et al., 2002). Phenotypic techniques for bacterial characterization include serotyping, biochemical profiling, bacteriophage typing, presence of antigens, and antimicrobial susceptibility profiles (Scott et al., 2002). The appearance of Salmonella serotypes with antimicrobial resistance, especially against drugs that represent the first line of treatment (ampicillin, amoxicillin, chloramphenicol, and trimethoprim/sulfamethoxazole) has become a serious health hazard (Centro Nacional de Excelencia Tecnológica en Salud, 2012; Chen et al., 2013). In Mexico, there has been evidence of the problem since the 1970s (Olarte and Galindo, 1973; González-Cortés et al., 1974; Zaidi et al., 2014). The detection of multiple drug resistance (MDR) in Salmonella isolated from water sources is of great relevance: MDR facilitates the transmission of antibiotic-resistant Salmonella to humans (Li et al., 2014). In Mexico, the increase in antimicrobial resistance in Salmonella strains obtained from clinical samples, domestic animals, and food has been linked to the indiscriminate use of antibiotics (Miranda et al., 2009; Amábile-Cuevas, 2010; Jiménez et al., 2011). However, the emergence of MDR Salmonella strains obtained from natural water sources requires a better characterization.
Pulsed field gel electrophoresis (PFGE) is a genotyping technique used by scientists to produce a DNA fingerprint for the differentiation and grouping of strains of Salmonella spp. or other bacterial isolates; it is also used in surveillance networks for foodborne diseases (Centers for Disease Control and Prevention [CDC], 2004, 2016; Lailler et al., 2002; Swaminathan et al., 2001; Tamada et al., 2001). The analysis can be carried out with one, two, or three restriction enzymes and can be used for reliable molecular typing of the strain(s) under study. In addition, typing methods have been used as an important tool to identify the sources of infection or reservoirs of epidemic outbreaks, to understand the clonality among strains and to determine transmission routes (Bou et al., 2011).
This study investigated the prevalence, serovar distribution, antimicrobial resistance, and PFGE typing of S. enterica isolated from Lake Zapotlán, Jalisco, Mexico, as well as the association between the prevalence and dissemination of Salmonella and different physicochemical and environmental factors. This information may be useful to develop effective strategies to identify sources of water pollution, to prevent contamination of agricultural food products through irrigation, and to reduce the incidence of water borne diseases in analogous freshwater ecosystems.
Materials and Methods
Study Site
Lake Zapotlán is a small (1,100 ha), perennial, and subtropical water body; it is situated in the western part of Mexico (19°45′N 103°29′W), to the south of the State of Jalisco, between the territorial portion of the municipalities of Gomez Farias and Ciudad Guzman, at 1520 m above sea level (Figure 1). Furthermore, it is classified as an endorheic lake, with a total area of 445 km2, a maximum depth of 4.75 m, and an average storage volume of 70.89 Hm3 (Comisión Nacional de Áreas Naturales Protegidas [CONANP], 2005; Greenberg, 2009).
Field Sampling and Monitoring
A total of 63 water samples were collected monthly from June to October 2016 from Lake Zapotlán, from 14 defined sampling stations (A through N) (Figure 1 and Supplementary Table S1). Nine sampling sites (F through N) are located within the main channels that connect the lake to the Gomez Farias and Ciudad Guzman municipalities, which are the main settlements that directly discharge wastewaters into the lake. Furthermore, three sites (C, D, and E) are in the wetlands area and two (A and B) are in the middle of the lake.
Sampling for Microbiological Analysis
Samples for microbiological analysis were taken from the sampling stations in the middle of the lake and in the wetlands area (A, B, C, D, and E) at 1 m depth. Additional samples were taken at different depths (2, 3, and 4 m), depending on the maximum depth of the lacustrine bed at the sampling site (Supplementary Table S1). Samples were taken at different depths to assess the presence of the Salmonella spp. populations through the water column and to infer the potential routes by which it can reach the lake’s biota and humans in contact with the lake’s water.
Samples for microbiological analysis were also taken from the channels that connect the lake to the main settlements (F through N), but these were taken close to the surface (30 cm depth) using an extendible swing sampler (samples could not be taken at different depths at these sites due to the difficult access; Supplementary Table S1). All samples were placed in sterilized bags containing 10 mg sodium thiosulfate tablets, preserved at 4°C in coolers for transportation, and processed in the laboratory in less than 24 h.
Physicochemical and Environmental Parameters
Physicochemical parameters were measured using an environmental multi-parameter probe (YSI 6600 V2), which includes sensors to measure temperature, pH, dissolved oxygen (DO), turbidity (TUR), and electrical conductivity (CON; all are verified sensors by the United States Environmental Protection Agency’s [EPA’s] Environmental Technology Verification Program; U.S. Environmental Protection Agency, 2003). The calibration protocol for all sensors of the probe were carried out before the use of the probe in field according the manufacturer protocol (YSI, 2009).
Physicochemical parameters were measured only in the sampling stations in the wetlands area and in the middle of the lake (A, B, C, D, and E; Figure 1) at different depths (1, 2, 3, and 4 m; Supplementary Table S1). For the remaining sampling stations (F through N), it was not possible to measure the physicochemical parameters with the multi-parameter probe because it was difficult to access the streams in the channels, which were surrounded by an aquatic plant commonly called tule (Schoenoplectus acutus). In addition, August was the only month in which these parameters were not measured in the sampling stations in the wetlands area and in the middle of the lake, because an important surface area of the lake was inaccessible due to the bloom of the aquatic weed commonly called water hyacinth (c; Centro Universitario del Sur, 2015).
Samples were taken during the dry and rainy season of the year to analyze the temporal dynamics of Salmonella spp. populations and understand the influence of climatological patterns of populations dynamics. Data on rainfall (mm), air temperature (°C), and relative humidity (%) for the sampling period were obtained from the National Meteorological Service weather station (ID # 766560) (TuTiempo.net, 2016) in Ciudad Guzman. This data matrix was used to calculate monthly values for each month of the study (June, July, August, September, and October).
Isolation of Salmonella
A 25-mL aliquot of each collected water sample was placed in 225 mL of pre-enrichment medium (buffered peptone water; BD Difco, United States) and incubated at 37°C for 24 h. This culture medium is used to revitalize microorganisms that might be affected by different storage processes or by environmental conditions, such as drying processes, exposure to ultraviolet (UV) radiation from sunlight, and/or the medium’s pH is very low (Luna, 1991; Hurtado, 2001). Subsequently, to stimulate the growth of this bacteria and inhibit the development of intestinal and coliform bacteria, 1- and 10-mL aliquots of the incubated pre-enrichment medium were transferred to 9 and 100 mL, respectively, Rappaport Vassiliadis R10 (BD Difco) medium and Selenite Cystine broth (BD Difco) and incubated at 37°C for 24 h (Iveson et al., 1964; Trichopoulos et al., 1972; Merck, 1994). To select typical Salmonella colonies and to stop the development of other microorganisms, the enrichment broths were streaked onto plates with selective/differential media: xylose lysine deoxycholate agar (XLA; BD Difco) and Hektoen enteric agar (HE; BD Difco) and incubated at 37°C for 24 h (Stanchi, 2007). From the bacterial growth on each plate, 3–4 suspected colony-forming units (CFUs) were selected based on the typical colony morphology—XLA: A typical Salmonella colony has a slightly transparent red halo and a black center, a pink-red zone may be seen in the media surrounding the colonies; HE: Salmonella produces transparent green or blue-green colonies with or without black centers and appears as almost completely black colonies (Global Foodborne Infections Network Country Databank, 2010; BD, 2013)—and re-isolated onto the agar mentioned above. Finally, those CFUs were transferred to 5 mL trypticase soy broth (TSB; Difco, United States) and incubated for 24 h at 37°C (Standard Method ISO 6579:2002, 2002).
Molecular Detection of Salmonella by Polymerase Chain Reaction (PCR)
Salmonella spp. were confirmed by using a previously reported polymerase chain reaction (PCR) protocol (Chiu and Ou, 1996). A 1.5-mL aliquot from the TSB culture was centrifuged at 16,000 g for 5 min, the supernatant was decanted, and 1 mL of molecular grade water was added; this step was repeated twice. After removing the supernatant from the previous step, 200 μL molecular grade water was added to resuspend the cell pellet. Finally, the bacterial suspension was placed in a water bath at 100°C for 5 min to cause cell lysis and release the bacterial DNA. Finally, Salmonella spp. were confirmed by the amplification of a 284 base pair (bp) fragment of the invA gene (Forward: 5′ ACA GTG CTC GTT TAC GAC CTG AAT 3′; Reverse: 3′ AGA CGA CTG GTA CTG ATC GAT AAT 5′; Chiu and Ou, 1996). To confirm the validity of the PCR results, a positive control was included using the bacterial strain S. enterica subspecies enterica serovar Typhimurium ATCC 14028. Escherichia coli ATCC 25922 was used as a negative control.
Statistical Analysis
A one-way analysis of variance (ANOVA) was performed to determine whether there were significant differences between sampling points and between months during the study period with respect to physicochemical and environmental parameters (Statgraphics, 2009). Furthermore, to explore the correlation between physicochemical and environmental parameters and the presence of Salmonella spp., a Pearson correlation matrix was constructed including physicochemical (pH, DO, TUR, and CON), environmental parameters (rainfall [PREC], air temperature [TA], and relative humidity [HUM]) and the prevalence of Salmonella spp. (Murdoch and Chow, 1996; Friendly, 2002).
Principal component analysis (PCA) was performed using the data matrix that comprised the recorded data of the physicochemical and environmental parameters and the prevalence of Salmonella spp. in order to identify the most significant inter-correlated variables (Sidharth et al., 2017). Subsequently, the first two principal components (PCs) were interpreted with the aid of biplots, which provide a visual representation of the correlation between the physicochemical and environmental parameters with the presence of Salmonella spp.
Biplots are a graphical representation of multivariate data (based on the decomposition of the singular value) that simultaneously plot n observations and p independent variables in two dimensions. The superposition of these two types plots provides additional information about the relationships between the variables and observations. The lengths of the vectors reflect the variance of the corresponding variables and the correlation of two variables is reflected by the angle between the two corresponding vectors (smaller angles represent greater correlations) (Jolliffe, 2002; Everitt and Hothorn, 2011; Zelterman, 2015).
Salmonella Serotyping
Serotyping of positive Salmonella strains was performed using a serum agglutination test according to the Kauffman–White scheme. Polyvalent Salmonella O and H antisera were used to obtain a presumptive diagnosis and, subsequently, the definitive designation of the antigen was determined by using monovalent antisera (Popoff and Le-Minor, 2001). Serotyping was carried out at the Enteric Bacteriology Laboratory, from the Bacteriology Department of the Institute of Epidemiological Diagnosis and Reference (“InDRE” by its acronym in Spanish) of the Mexican Ministry of Health in Mexico City.
Antimicrobial Susceptibility
Susceptibility and resistance of all Salmonella strains were carried out through the Kirby–Bauer disk diffusion method according to the guidelines of Clinical and Laboratory Standards Institute (Clinical and Laboratory Standards Institute [CLSI], 2005). An 18–24-h culture grown on TSB was used for antimicrobial resistance testing. A sterile cotton swab was used to create a bacterial suspension mixture in 0.85% saline equivalent to a 0.5 McFarland standard (considered equivalent to approximately 1.5 × 108 CFU/mL). The suspension was then vortexed for 2 s, and a fresh sterile swab was later immersed into the suspension. Excess liquid could drain off by touching the side of the test tube. The entire surface of the Müeller–Hinton agar plate was swabbed edge to edge. Each plate was re-streaked two additional times by rotating the plate a quarter turn to ensure an equal distribution of cells to create a uniform bacterial lawn. Eight classes of antimicrobial agents (aminoglycoside, cephalosporin, penicillin, quinolone, tetracycline, beta-lactam, polypeptide, and sulfonamide; Oxoid, United States) were used in the diffusion assays. The specific antimicrobials used were: chloramphenicol 10 μg (C), nalidixic acid 30 μg (NA), ciprofloxacin 5 μg (CIP), tetracycline 10 μg (TE), ceftazidime/clavulanic acid 30 μg (CAZ), trimethoprim 5 μg (TMP), streptomycin 10 μg (S), sulfamethoxazole/trimethoprim 25 μg (SXT), kanamycin 30 μg (K), amikacin 30 μg (AN), ampicillin 10 μg (AM), gentamicin μg 10 (GM), cephalothin 30 μg (CF), amoxicillin/clavulanic acid 20/10 μg (AMC), colistin 25 μg (CL), and imipenem 10 μg (IPM). Using sterile forceps, antimicrobial disks were placed on the agar surface within 5 min of the application of bacterial cells. The plates were further incubated inverted for 16–18 h at 37°C (Clinical and Laboratory Standards Institute [CLSI], 2005).
Following incubation, the lawn was examined to determine whether growth was uniform over the plate. The diameter of the zones of clearing (including the disk) was measured using a Vernier scale. A single colony or a faint haze was regarded as no growth within the zone of clearing. These results were compared to standardized charts for interpretation, as recommended by the manufacturer of the antimicrobial disks and following the criteria of Clinical Laboratory Standards Institute [CLSI] (2005). Results were recorded as susceptible or resistant. Results showing intermediate resistance were considered susceptible in this study, unless otherwise stated. Strains were considered to be MDR if they demonstrated phenotypic resistance to two or more antimicrobial drugs. E. coli ATCC 25922 was used as a quality control organism to ensure the validity of the susceptibility testing.
PFGE
The molecular subtyping of Salmonella spp. strains was performed according to the 1-day (24–28 h) standardized laboratory protocol for the molecular subtyping of Salmonella by PFGE (Centers for Disease Control and Prevention [CDC], 2017), using S. enterica serovar Braenderup H9812 as a standard strain. Briefly, two agarose-embedded DNA samples were digested, each separately, one with a primary enzyme (XbaI) and the second with a secondary enzyme (BlnI; Roche Diagnostics, Germany) for at least 3 h in an incubator at 37°C. The restriction fragments were separated by electrophoresis in 0.5X Tris-borate-ethylenediaminetetraacetic acid (EDTA; TBE) extended-range buffer (Bio-Rad, United States) with recirculation at 14°C for 20 h and 25 min using a Chef Mapper electrophoresis system (Bio-Rad, United States) with pulse times of 2.20–63.8 s. Following electrophoresis, the gel was stained with GelRed (10,000X; Biotium, United States). Using a gel documenter (Bio-Rad Gel Doc, United States), the DNA bands were visualized under UV light and then photographed and saved as a TIFF image to compare the DNA fragment patterns and cluster analysis, using BioNumerics version 6.6.1.1. software (Applied Maths, Kortrijk, Belgium). Similarity among DNA fragment patterns was determined using the Dice coefficient correlation, and clustering was based on the unweighted pair group method using arithmetic averages (UPGMA) with a band position tolerance and optimization of 1.5%.
Results
Physicochemical and Environmental Parameters
Supplementary Tables S2, S3 show the results of the one-way ANOVA for comparing the physicochemical parameters among sampling months (temporal ANOVA) and sampling points (spatial ANOVA), respectively. Supplementary Table S3 shows the results of the one-way ANOVA for comparing the environmental parameters among sampling months.
The results of the temporal ANOVA in Supplementary Table S2 showed that there were statistically significant differences between sampling months for all evaluated physicochemical parameters except pH. With respect to the spatial ANOVA, there were no significant differences for any evaluated parameter (Supplementary Table S3). The results of the one-way ANOVA comparing the environmental parameters among sampling months showed that there were significant temporal variations in HUM and PREC. There were no statistical differences in TA (Supplementary Table S4).
Presence of Salmonella spp.
A total of 19 Salmonella strains were confirmed from a total of 63 water samples from all sampling sites: 14 strains were detected (73.68%) in June, three strains (15.78%) in July, and the remaining strains in September (Supplementary Table S5). No strains were detected in August or October. It is important to remark that most of the strains (11/19, 57.89%) were isolated from the samples taken from the surface waters (30 cm; F, G, I, and J), three strains were isolated at 1 m depth, two each at 2 and 4 m, and only one strain was isolated at 3 m (Supplementary Table S6). This finding confirms the facultative anaerobic character of the species in the environment (Abulreesh, 2012).
The exploratory correlation analysis (Figure 2) suggested that the presence of Salmonella (SAL) in Lake Zapotlán was negatively correlated with HUM and PREC while it was positively correlated with TA. With respect to physicochemical parameters, Pearson correlation analysis suggested the presence of Salmonella spp. was positively correlated with pH, DO, and CON and negatively correlated with water temperature (WT) and TUR. However, no significant correlations were found between Salmonella spp. and the rest of the physicochemical and environmental parameters (p > 0.05).
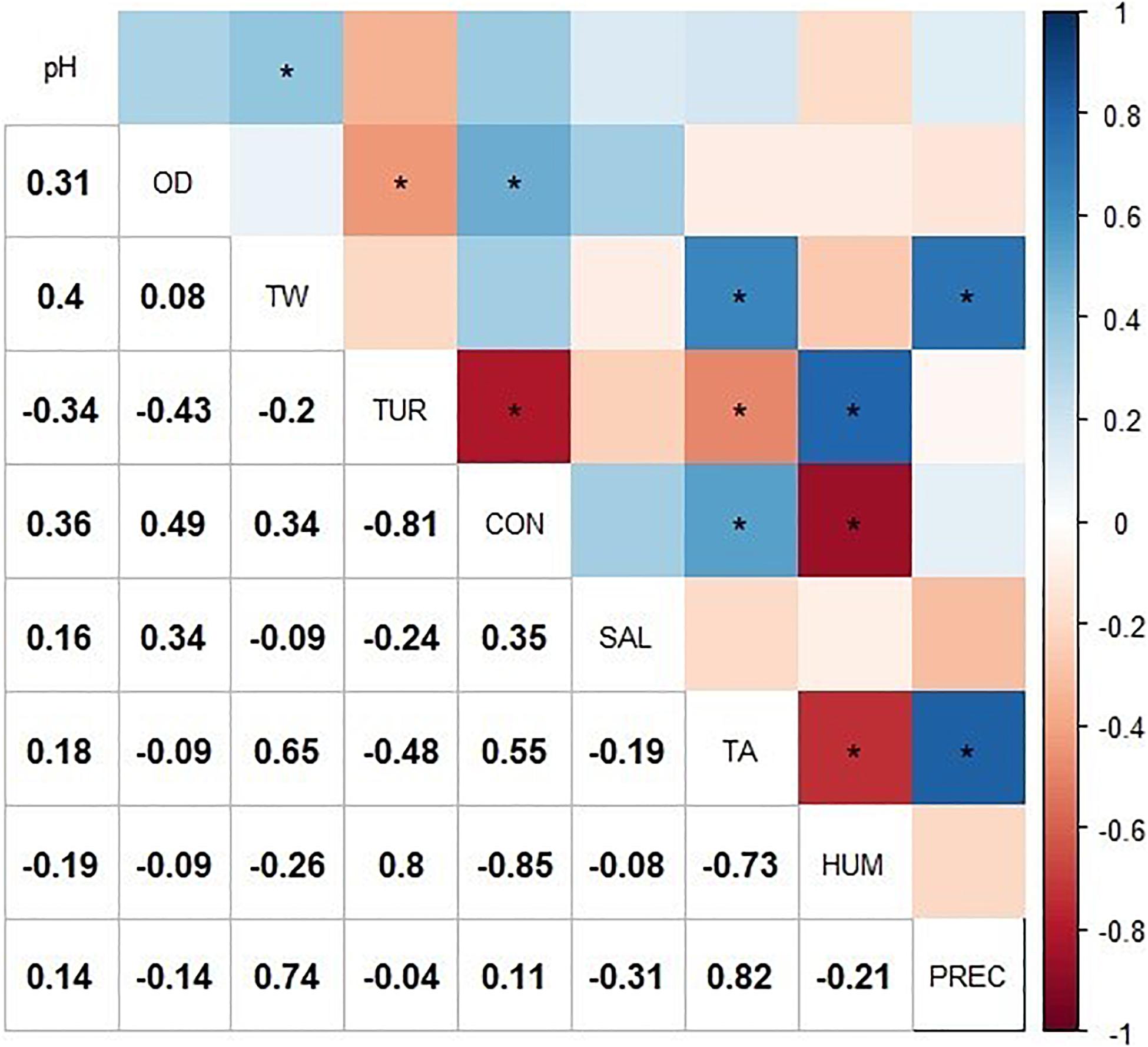
Figure 2. Correlation matrix including the physicochemical and environmental variable values and the presence of Salmonella. The correlogram represents the correlation for all pairs of variables. Positive correlations are displayed in blue and negative correlations in red. The intensity of the color is proportional to the correlation coefficient, so the stronger the correlation (i.e., the closer to −1 or 1), the darker the boxes. “*” indicates significant Pearson correlation (p < 0.05). The color legend on the right side of the correlogram shows the correlation coefficients and the corresponding colors. WT, water temperature; DO, dissolved oxygen; CON, electrical conductivity; TUR, turbidity; TA, air temperature; HUM, relative humidity; PREC, rainfall, and SAL, Salmonella.
A PCA was also applied to explore the relationship between physicochemical and environmental parameters with the presence of Salmonella spp. Four PCs were sufficient to explain the variance of these parameters (Supplementary Table S8). The first four PCs explained 87.37% of the variance (Supplementary Table S7). The highest loadings to PC1 corresponded to DO, HUM, TUR, CON, and TA, all of which are season-dependent variables. The highest loadings of PC2 corresponded WT and PREC, both of which are environmental parameters. PC1 and PC2 accounted for 68.26% of the total data variability. Supplementary Table S8 shows the most influential variables for each of the four components. The graphical analysis of PCA was enhanced by means of biplots, which are the orthogonal projection of the data on the subspace that is spanned by PC1 and PC2 (which contribute the most to the total variance). They describe the importance and correlations between the environmental and physicochemical parameters and the presence of environmental parameters (Gradilla-Hernández et al., 2019). These results reflected the correlation between the presence of Salmonella spp. with some of the evaluated physicochemical and environmental parameters.
On the other hand, the PCA coincided with the results of the correlation analysis. Figure 3 shows how, in a two-dimensional representation composed of PC1 and PC2, the vectors corresponding to the physicochemical and environmental measurements of each sampling month were grouped into clusters. The presence of Salmonella spp. was positively correlated with TA, CON, pH, and DO. Conversely, the presence of these bacteria was negatively correlated with the rest of the analyzed physiochemical (TUR and WT) and environmental parameters (PREC and HUM). As mentioned previously, 73.68% of the Salmonella strains were isolated in the month of June; Figure 3 presents that temporal groupings (samples per month) influenced season-dependent physicochemical and environmental parameters. These observations suggest that the significant variables in PC1 and PC2 vary among months; these two components sufficiently explain the variability so as to classify the observations among the sampling months.
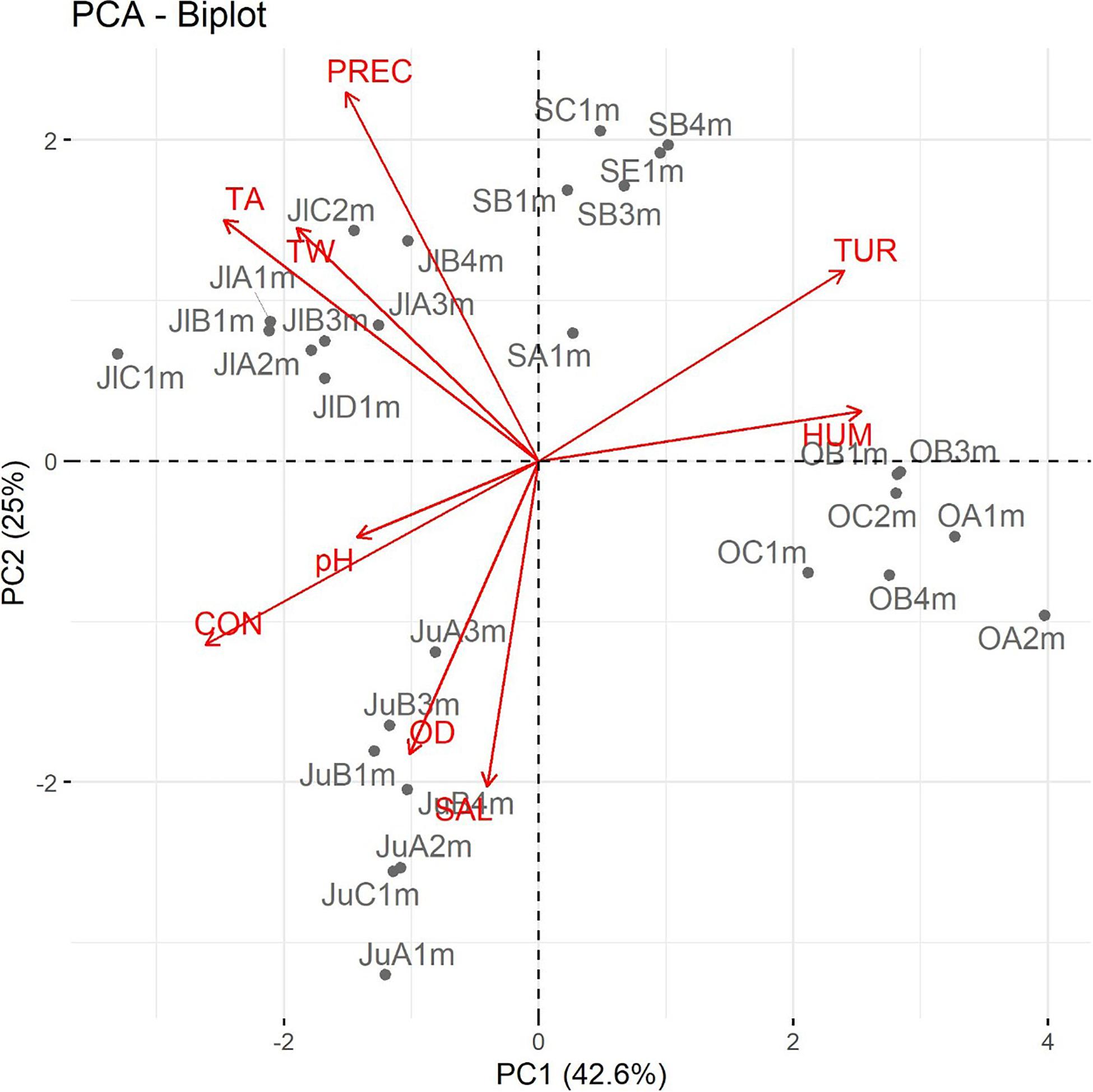
Figure 3. Principal component analysis (PCA) of the physicochemical and environmental parameters; each vector represents a variable, and the correlation of two variables is reflected by the angle between the two corresponding vectors. Abbreviations: WT: water temperature; DO: dissolved oxygen; CON: electrical conductivity; TUR: turbidity; TA: air temperature; HUM: relative humidity; PREC: rainfall; and SAL: Salmonella. Code of the isolated Salmonella strains: First and/or second letter correspond to the sampling month (Ju: June; Jl: July; S: September; and O: October); the second and/or third letter indicate the sampling site (A, B, C, D, or E); and the penultimate number and letter refer to the depth (1, 2, 3, or 4 m).
Serotyping of S. enterica
A total of 19 Salmonella spp. strains were isolated and confirmed; only 15 strains were successfully serotyped by the traditional method. All the strains were identified as S. enterica. Salmonella Agona was the predominant isolated serotype, with 13 of 19 (68.42%) strains; followed by Salmonella serogroup B, with 4 of 19 (21.05%) strains; and finally, Salmonella Typhimurium and Salmonella Weltevreden, each with a single strain.
Antimicrobial Resistance of Isolated Salmonella spp.
The antimicrobial resistance analysis showed that 94.8% (18/19) of the Salmonella spp. isolated from the water of the Lake Zapotlán were resistant to at least one antimicrobial agent and 78.9% (14/19) of the strains had MDR to two or more antimicrobial agents. Only 8 of 16 evaluated antimicrobials (colistin, tetracycline, nalidixic acid, sulfamethoxazole, trimethoprim, gentamicin, kanamycin, and streptomycin) generated resistance in Salmonella strains. Ten of 19 (52.63%) strains showed resistance to colistin, tetracycline, nalidixic acid, sulfamethoxazole, and trimethoprim, while only 5.26% (1/19) of the strains were susceptible to all the tested antimicrobials. Resistance to colistin was prevalent among the tested Salmonella strains (14/19) (Supplementary Figure S1). Our analysis revealed 16 different resistance profiles in the Salmonella strains, with colistin present in all resistance profiles (Supplementary Figure S2).
Genetic Profile of Salmonella Serotypes
The PFGE analysis with the restriction enzyme XbaI allowed inter-serotype differentiation to further obtain specific profiles for each. We found that the three serotypes of Salmonella (Agona, Typhimurium, and Weltevreden) and the analyzed serogroup B presented 11 genetic profiles (designated X01 to X11; Figure 4). Different genetic profiles were observed for S. Agona (five profiles) and serogroup B (three profiles), while the rest (S. Typhimurium and S. Weltevreden) had a single profile. The X09 profile consisted of five strains isolated from the same sampling date with 94.6% similarity. Three of the strains in this profile (A1S2m-2, A1S2m-3, and A1S1m-2) correspond to the same sampling site, but they were isolated at different depths; the rest were obtained from different sites. In addition, three strains (I1S30cm-3, F1S30cm-5, and C1S3m-3) belonging to the X10 profile were obtained from the same sampling date but at a different sampling points; they had 83.5% similarity. There was 97% similarity in the X03 genetic profile, which consisted of two strains (S. Agona and S. serogroup B) and corresponded to the same date and sampling point. The results obtained from this serotyping showed that 94.73% of the strains (18/19) belong to S. serogroup B, while only one strain belongs to serogroup E (S. Weltevreden). However, four of the strains that belong to S. serogroup B were not serotyped.
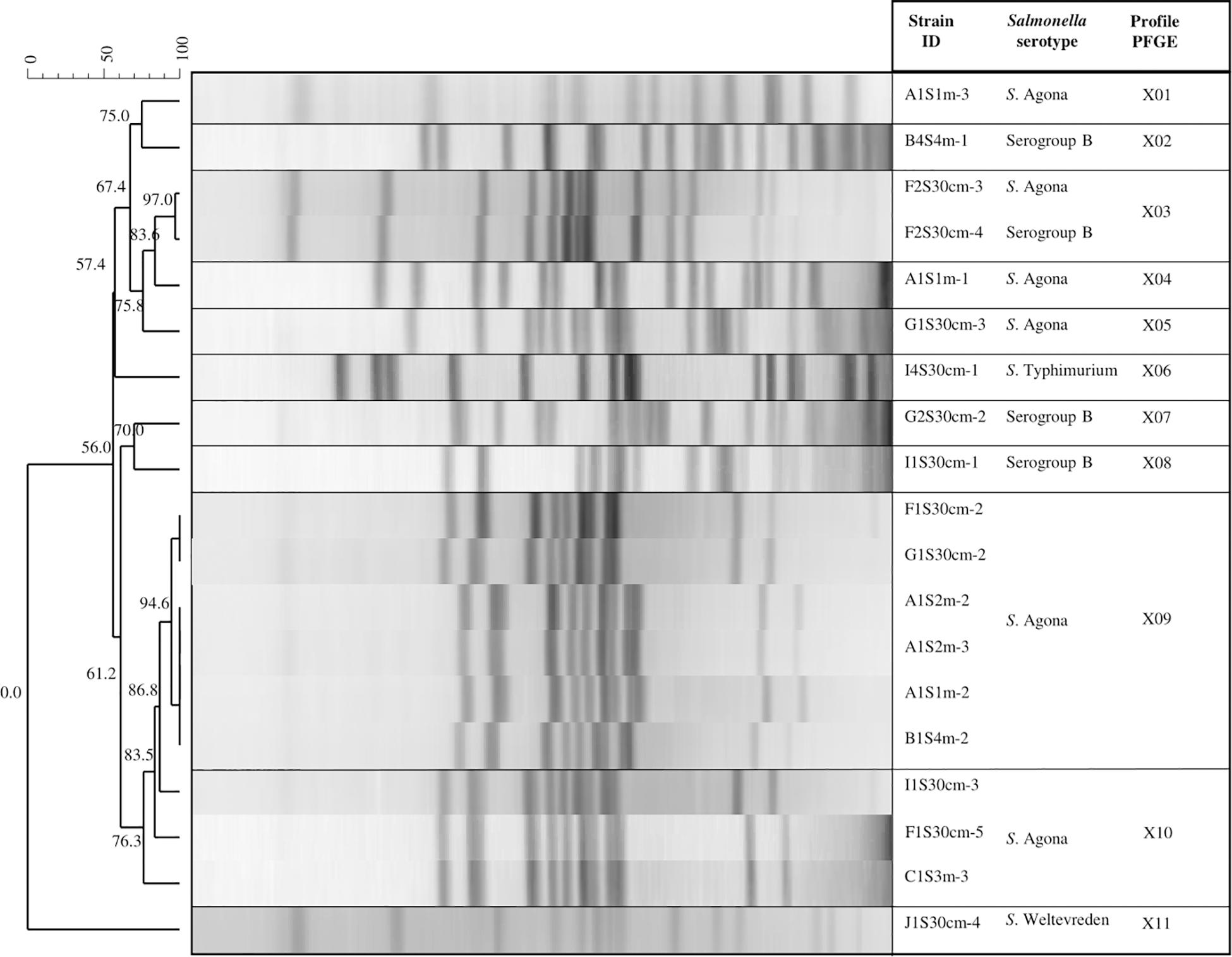
Figure 4. Pulsed field gel electrophoresis (PFGE) XbaI dendrogram showing the relationship between Salmonella strains. The code for the isolated Salmonella strains: The first letter corresponds to the sampling site (A, B, C, F, G, I, or J); the second and third letter indicate the sampling month (1S, 2S, or 4S); the fourth specifies the depth (30 cm, 1, 2, 3, and 4 m); and the dash and number represent an isolated colony within a sample.
The four isolated S. serogroup B strains were distributed in the tributaries that discharge into Lake Zapotlán, while only one strain was isolated from the lake. Profiles X02, X03, X07, and X08 were isolated from different sites and sampling dates. There was no similarity among these profiles: They all had unique band patterns (Figure 4).
All strains were further characterized using a second restriction enzyme (BlnI). The BlnI dendrogram shows that all the PFGE patterns were differentiated into groupings of the same serotype or serogroup, except for the X01 genetic profile, which comprises four strains of S. serogroup B and one of serotype S. Agona; they had 88.6% similarity. These results coincide with the indistinguishable strains of the X03 genetic profile examined with the restriction enzyme XbaI that allowed characterization of serogroup B in S. Agona strains (Figure 5).
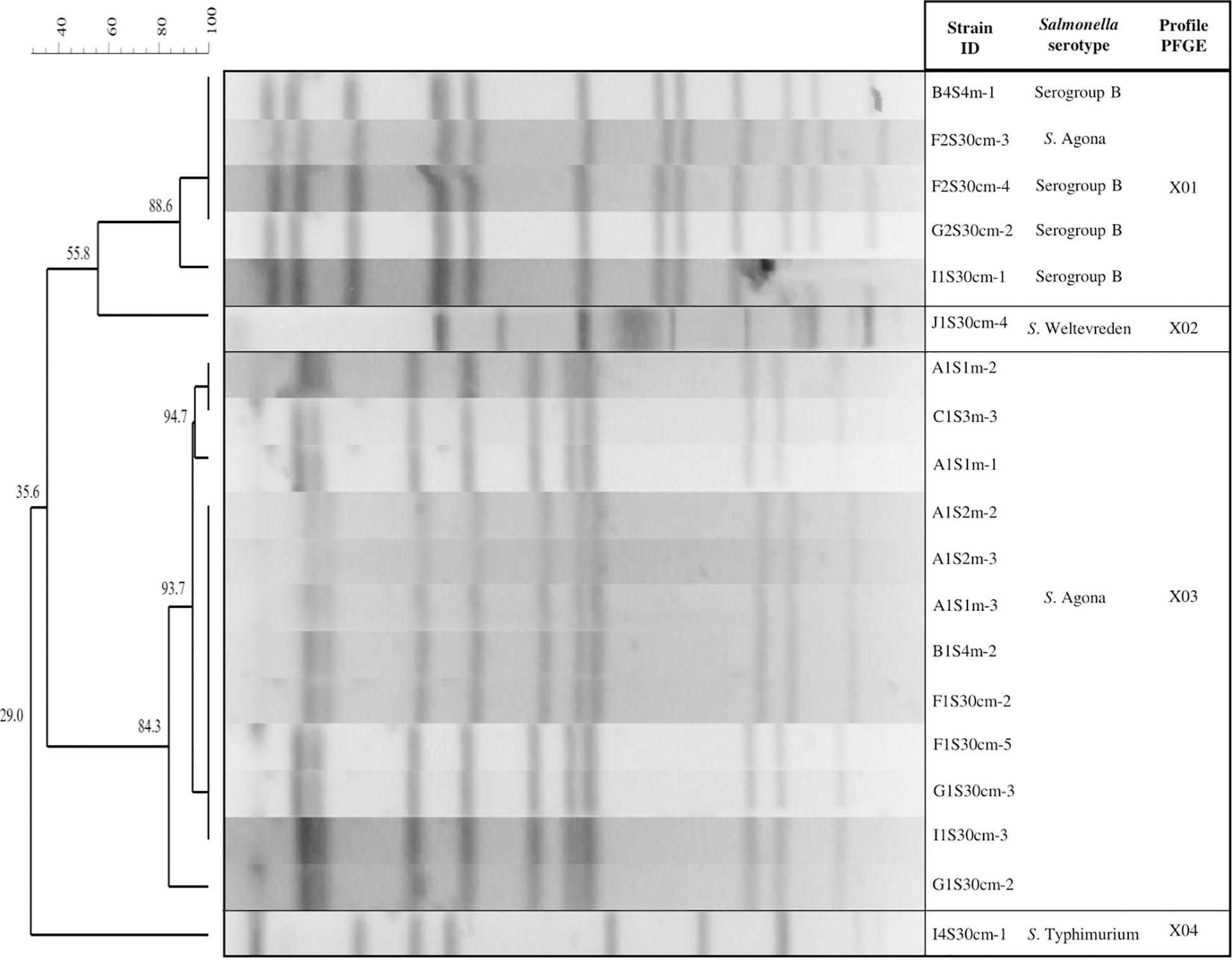
Figure 5. Pulsed field gel electrophoresis (PFGE) BlnI Dendrogram showing the relationship between Salmonella strains. The code for the isolated Salmonella strains: The first letter corresponds to the sampling site (A, B, C, F, G, I, or J); the second and third letters indicate the sampling month (1S, 2S, or 4S); the fourth specifies the depth (30 cm, 1, 2, 3, or 4 m); and the dash and number represent an isolated colony within a sample.
Discussion
Presence of Salmonella spp.
The percentage of isolates of Salmonella spp. obtained in this study were consistent with previously published works, which found a prevalence of approximately 30% when monitoring aquatic environments (Tavechio et al., 2002; Johnson et al., 2003; Arvanitidou et al., 2005). This outcome could be due to the ability of Salmonella to persist in nature, particularly when these bacteria are subject to hostile conditions such as resistance to low nutrient availability, drastic fluctuations in temperature and pH, and UV light radiation; even the serotype can determine its survival (Baudart et al., 2000; Steele and Odumeru, 2004; Wilkes et al., 2011; Wanjugi and Harwood, 2012).
The small settlements located near or around Lake Zapotlán discharge raw wastewaters without treatment (Comisión Estatal del Agua [CEA], 2012), which could be a source of Salmonella spp., as has been previously demonstrated (Thurston-Enriquez et al., 2002). Other sources of Salmonella spp. pollution are manure that result from raising poultry, livestock, pigs, and other domestic animals, all of which are known to be the main reservoirs of Salmonella spp. (Morse and Duncan, 1974). During this study, the presence of these animal reservoirs was observed near the sampling sites. Similarly, Gagliardi et al. (2003) analyzed Salmonella spp. at different stages of the post-harvest process of cantaloupes and isolated these bacteria from irrigation channels and filters through which the water passed. They suggested a possible environmental or domestic origin of the isolated Salmonella. On the contrary, Abulreesh et al. (2004) were unable to isolate Salmonella in water samples from a pond that receives direct fecal contamination from waterfowl; however, they were able to detect these bacteria from sediments in the bottom of the pond. This outcome could be attributed, in part, to the concentration of Salmonella through sedimentation and also to the greater survival of Salmonella in bottom sediments than in water (Fish and Pettibone, 1995; Winfield and Groisman, 2003). These findings possibly suggest that a higher number of Salmonella isolates could be found in the sediments of Lake Zapotlán.
On the other hand, the county of Ciudad Guzman has two wastewater treatment plants. These plants are based on activated sludge processes, with capacities of 50 and 150 L/s, respectively; the treated waste is discharged directly into the lake. According to water managers, the treatment plants frequently surpass their capacity during the rainy season because the sewage system of the city is the same used to drive storm waters. Therefore, it is believed that a large amount of wastewater mixed with stormwater reaches the lake with insufficient treatment (Municipal water managers, personal communication, July 05, 2016; Sistema de agua potable de Zapotlán, 2012).
Relationship Between the Presence of Salmonella spp. With Physicochemical and Environmental Parameters
As mentioned in section 2, the physicochemical parameters were neither measured at all the sampling sites nor in August due to poor accessibility because of the presence of aquatic plants in the channels and in the lake. The statistical analysis lacked these physicochemical measurements, which would have strengthened the interpretations regarding the factors that spatiotemporally influence the presence of Salmonella in Lake Zapotlán. However, with the measurements obtained from several physicochemical parameters in some sampling sites and through the rest of the sampling period, we can suggest a correlation between these parameters and the presence of Salmonella spp. in Lake Zapotlán.
The optimal growth temperature of Salmonella spp. have been reported within the range of 35 to 43°C, however, it can multiply in a wider range of temperatures (5.2–46.2°C; International Commission on Microbiological Specifications for Foods, 1996). In this study, although there was more Salmonella spp. during the month of June (14/19) (Supplementary Table S5), there were no significant differences in WT parameter during the sampling months (Supplementary Table S2), which could favor the growth of these microorganisms. For instance, the highest average CON was reported for the month of June (Supplementary Table S2); nevertheless, no studies have yet reported a correlation between the presence of Salmonella with this variable in aquatic environments. Regarding pH, Salmonella spp. has been reported to survive in an environment with a broad pH range (4.05–9.5; Fatica and Schneider, 2011), with an optimal pH of 7–7.5 (Jay et al., 2003). The average pH recorded during the month of June was 8.2 (Supplementary Table S2); however, there were no significant temporal or spatial differences regarding this variable (Supplementary Tables S2, S3). Similarly, McEgan et al. (2013) found that pH was not correlated with the presence of Salmonella spp. when comparing results for different sampling sites and sampling months in surface waters across central Florida. In the case of TUR, the lowest average value was registered in June (37.85 NTU; Supplementary Table S2). Other studies have reported that TUR does not affect the prevalence of this bacterium (Morales-Morales et al., 2003; Leskinen et al., 2010; Jimenez and Chaidez, 2011).
The highest DO concentrations are usually present at or near the surface of water bodies (Huang et al., 2019). Salmonella spp. is known to be a facultative anaerobic organism for which DO is not a limiting factor for survival (International Commission on Microbiological Specifications for Foods, 1996). In this study, more than 50% of Salmonella spp. strains were isolated from samples taken close to the surface (30 cm depth) at the sampling stations located at the main channels discharging into the lake (sampling stations F through N; Supplementary Table S6). This finding suggests that the proximity to the surface (and higher oxygen availability) may be related to the presence of Salmonella spp. However, in these sites it was not possible analyze the DO, besides the rest of the physicochemical parameters due to the difficulty in accessing them, therefore, as well as OD, other physicochemical parameters could be better for the survival of this bacterium.
The presence of Salmonella spp. in Lake Zapotlán also correlated with environmental parameters (TA, PREC, and HUM). The lowest PREC and HUM were recorded for the months of October and June. As previously mentioned, the greatest prevalence of Salmonella (14/19) was observed in June. By contrast, when higher PREC and HUM were recorded for the months of July and September, there was a lower prevalence of this bacterium (5/19; Supplementary Tables S4, S5). These results are consistent with those reported by McEgan et al. (2013): There was a lower concentration of Salmonella spp., E. coli and fecal coliforms when rainfall was the highest, probably due to the effects of dilution in surface waters. However, it has also been reported that superficial runoff (caused by PREC) may act as an important vehicle for transportation of Salmonella spp. to aquatic environments (Levantesi et al., 2012; H. Liu et al., 2018). Finally, when the TA was higher, the highest presence of Salmonella was recorded (14/19, June; Supplementary Tables S4, S5). Previous studies have also associated a higher Salmonella detection rate in water in warmer seasons (El-Gazzar and Marth, 1992; Polo et al., 1998; Martinez-Urtaza et al., 2004).
Serotyping of S. enterica
In this study, the most predominant serotype was S. Agona. This finding may suggest that this serotype had a more efficient adaptation to the aquatic environment in the Lake Zapotlán. In Mexico, the official epidemiological system (Dirección General de Epidemiología) neither reports nor tracks Salmonella serotypes that cause gastrointestinal infections in the population (Dirección General de Epidemiología [DGEPI], 2017). However, Gutiérrez-Cogco et al. (2000) reported the most frequent serotypes isolated from human sources in Mexico: S. Typhimurium, Salmonella Enteritidis, S. Agona, and Salmonella Typhi were the most frequent; S. Agona represented less than 9% of the total of these isolates. In 2004, the WHO through the GFN (World Health Organization Global Foodborne Infections Network, 2010) reported that only 15 strains of Salmonella spp. isolated from the environment came from Mexico, from which S. Agona was identified five times. Alaniz et al. (1997) highlighted that on farms with cows, pigs, and chickens, the presence of S. enterica presented 25 different serotypes. They noted S. Typhimurium, in addition to finding these serotypes distributed and shared among these animals. Vásquez et al. (2005) reported that chicken farms are infected by Salmonella; the strains isolated from birds were S. Typhimurium and S. Enteritidis. They concluded that the strains isolated from birds are a source of contamination for humans and a route of dissemination of this bacterium. In another study conducted in China in 2015, the distribution of Salmonella serotypes isolated from chickens, pigs, and dairy cows was identified; the most predominant were S. Typhimurium, S. Enteritidis, and S. Agona. The authors mentioned that differences in isolation rates can be interpreted according to the region, sample types, serotypes, collection stations, culture methods, isolation methodologies, culture media, and environmental conditions premises (Kuang et al., 2015).
The diversity of Salmonella found in this study can be attributed to several factors, including the ability to survive in a wide range of hosts (Rabsch et al., 2002). Predominant strains of Salmonella spp. reflect the adaptability of some serotypes to a specific ecosystem; however, there are serotypes that lack the capacity to survive in the environment for prolonged periods of time. This phenomenon is due to the presence of genetic determinants and to the modification of their physiological characteristics, which gives them the ability to adapt to adverse situations (Winfield and Groisman, 2003). In addition, the ubiquitous nature of these bacteria facilitates their passage from a host to the environment and return to a new host (Winfield and Groisman, 2003). Hence, Salmonella is commonly isolated from food, animals, rivers, water, and soil because these reservoirs serve as environments in which bacteria can survive, constituting a permanent source of contamination and facilitating transmission between hosts (Lemarchand and Lebaron, 2002; Winfield and Groisman, 2003).
Antimicrobial Resistance of Salmonella spp.
Salmonella strains isolated in this study with MDR indicate the frequent contact in an environment contaminated with antibiotics (Zansky et al., 2002). Salmonella and other bacterial groups acquire antimicrobial resistance by random chromosomal mutations, by mutations of existing genes, and through transduction, transformation, and conjugation. These mechanisms involve the transfer of antimicrobial resistance genes from a circulating plasmid, such as R factor, conjugative plasmids, or chromosomal elements (Poppe et al., 1996). Furthermore, the factors that promote resistance to antibiotics in microorganisms can be the indiscriminate use of antibiotics in agriculture, animals, and humans, due to inadequate prescription, lack of following indications of use, and deficiencies in government policies that do not limit the use of antibiotics (Boerlin and Reid-Smith, 2008). Lake Zapotlán, as previously mentioned, represents an important part of fishing, artisanal, agricultural, livestock, and tourism activities (Secretaría de Medio Ambiente y Desarrollo Territorial, 2005). Due to all these activities, the resistance encountered of Salmonella spp. to different antimicrobials could be the result of exposure to antibiotics used for human consumption and the control of animals and plants diseases. These diseases could reach the lake through several sources of contamination (point and non-point sources of water pollution; Greenberg et al., 2008; Malczyk and Branfireun, 2015).
In Mexico, antibiotic doses prescribed for therapeutic purposes in humans play a significant role in the increase of resistant strains. Included in this category are self-prescriptions and errors in indications for use, prescriptions when antibiotic use is not justified, and inadequate antibiotic and dose selection, both of which occur when the doctor prescribes antibiotics based only on the patient’s symptoms (Dreser et al., 2008). The frequency of Salmonella strains that are resistant to various antibiotics, including ampicillin, tetracycline, streptomycin, sulfamethoxazole/trimethoprim, chloramphenicol, and sulfonamides, as well as other drugs has been widely established in the literature (Levantesi et al., 2012; Abakpa et al., 2014). In particular, high levels of resistance to these antibiotics have been reported in Salmonella (Voss-Rech et al., 2016). In Mexico, previous studies aimed at the characterization of antimicrobial resistance have described Salmonella strains of clinical (Amábile-Cuevas, 2010) or food (Miranda et al., 2009) origin, or strains that originated in canal waters (López et al., 2009), that are resistant to tetracycline, ampicillin, chloramphenicol, and ceftriaxone. The tetracycline resistance phenotype was also observed in this study: A total of 11 of 19 Salmonella strains were resistant to this antimicrobial agent.
Mhongole et al. (2017) determined the antimicrobial resistance profile of Salmonella isolated from wastewater and tilapia fish in Tanzania. Salmonella serotypes (Kentucky, Chandans, Durban, and Kiambu) were found to be resistant to 8 of 14 tested antimicrobials: sulfamethoxazole (94%), streptomycin (61%), tetracycline (22%), ciprofloxacin and nalidixic acid (17%), trimethoprim (11%), and gentamicin and chloramphenicol (6%). These data coincide with the present study, where 18 strains were resistant to the same antimicrobials. Mhongole et al. (2017) mentioned that wastewater is a source of Salmonella contamination in water bodies and food products, including fish and irrigated crops.
Colistin was the only antimicrobial that was present in all resistance profiles (16 profiles) of this study (Supplementary Figure S2). For decades, colistin has been used in veterinary medicine, mainly for the prevention and treatment of enterobacterial infections (Kempf et al., 2016). Morales et al. (2012) analyzed 124 S. enterica strains from sick pigs; 21% of the isolated strains were resistant to colistin. It has also been widely reported that colistin resistance occurs via point mutation, mostly in genes that affect the lipid bilayer and cell wall biosynthesis (Olaitan et al., 2014). Y.-Y. Liu et al. (2016) first demonstrated a horizontally transferable colistin resistance mechanism: A gene (mcr-1) that is resistant to colistin mediated by a plasmid, in strains of E. coli and Klebsiella pneumoniae originating from different sources (animals, raw meat, and human cases) in China. Doumith et al. (2016) performed a rapid screening of the genomes of S. enterica strains isolated from food (two strains isolated from poultry meat) and humans (10 strains isolated from patients). Six S. enterica isolates were positive for the mcr-1 gene from patients who had recently traveled to Asia. We hypothesize that the colistin resistant species found in this study might have their animal and human origin (as previously reported).
Genetic Profile of Salmonella Serotypes
The diversity of XbaI PFGE profiles for S. Agona and serogroup B might be explained by variations in the genome of the bacterium, because during the process of adaptation to adverse conditions or new environments, the microorganism can integrate new genetic material by the horizontal transfer mechanism (Prosser et al., 2007). Gorski et al. (2011) reported 40 different PFGE profile pulsotypes of S. enterica, serotypes Give, Typhimurium, Montevideo and Infantis, isolated from different sources (water, wildlife, or soil). These data suggest that they were persistent in that environment. These profiles were found scattered around Monterey County in California, an important agricultural region of the United States.
The PFGE technique has shown limited discriminatory power in subtyping some highly clonal serotypes (e.g., S. enterica serotype Enteritidis and S. enterica serotype Hadar; Swaminathan et al., 2001; Lukinmaa et al., 2004; Hyytiä-Trees et al., 2006). Additionally, PFGE often lacks discriminatory power to partition strains into epidemiologically meaningful clusters. Although this technique is still the current fingerprint method used by PulseNet, they are already transitioning toward using sequencing methods (Centers for Disease Control and Prevention [CDC], 2016), which have a higher discriminatory power than PFGE. In addition, the generated sequencing data is non-ambiguous and portable and can be readily compared between laboratories through the curated website (Hyytiä-Trees et al., 2007). In our study, PFGE was quite cumbersome, subjective, and difficult to perform. Sequencing techniques would have permitted us to avoid some drawbacks presented by the PFGE analysis, such as the strict requirements for DNA preparation, the time invested in performing the technique, and the analysis of the images. However, in this study PFGE showed a high discriminatory power by using a second enzyme (BlnI), which allowed us to differentiate PFGE patterns in groups of the same serotype or serogroup. It has been reported that a higher discriminatory power may be achieved by incorporating other potentially more informative enzymes, such as BlnI, SfiI, PacI, NotI, etc. (Zheng et al., 2007, 2010; Zhao et al., 2008; Son et al., 2013).
The distribution of S. Agona and serogroup B can be attributed to the transport and spreading of these bacteria by livestock and wildlife of a locality, through environmental vehicles such as bodies of water (Ferguson et al., 2003). The presence of these animals was observed near the sampling sites during this monitoring; however, it could also be associated to the wastewater that is discharged into the lake. These two sources could have deposited feces of animals and/or infected persons inside the lake and its watercourses, and in this way could have contributed to its contamination. Based on this study, the monitoring of Salmonella might facilitate assessments of temporal and spatial factors that are relevant to the incidence of these bacteria, tracking of the source of contamination, higher resolution subtyping, and comparisons with similar data from other agricultural regions in Jalisco. The PFGE analysis provided useful evidence of the clonality of strains Salmonella strains from Lake Zapotlán; these data will provide a better understanding of transmission patterns.
Conclusion
Lake Zapotlán and in the main bodies of water that discharge into it contain diverse Salmonella strains. The climatic and water quality features of the lake were correlated with the prevalence and dissemination of Salmonella spp. during the study period. The encountered resistance of Salmonella spp. to different antimicrobials might be the result of long-term exposure of the bacteria to waters that receive regular loads of antibiotics used for human consumption and control of animal and plants diseases. Salmonella diversity and its prevalence in the environment might be related to one or a combination of wastewater effluents, agricultural runoff, and direct fecal contamination from natural fauna. Based on these results, future work must study the main routes of dissemination of this pathogen to explore the factors involved in the presence and dissemination of this bacterium in Lake Zapotlán. The findings of this study can be used to understand Salmonella spp. ecology in subtropical regions. This information will aid for proposing and implementing effective strategies to control point and non-point source pollution that is discharged into the lake, as well as to prevent gastrointestinal diseases due to irrigation of food products with contaminated waters.
Data Availability Statement
The datasets generated for this study are available on request to the corresponding author.
Author Contributions
ODT, OYLM, and JDA performed the conceptualization and methodology. ODT, MSGH, and DMR performed the software. ODT and JDA performed the validation, supervision, and project administration. ODT and MSGH performed the formal analysis, data curation, and visualization. ODT, OYLM, JDA, and BAAL performed the investigation. ODT prepared and wrote original draft of the manuscript. ODT, OYLM, JDA, and MSGH performed writing and review and editing of the manuscript. All authors contributed to the article and approved the submitted version.
Funding
This research was financed by Centro de Investigación y Asistencia en Tecnología y Diseño del Estado de Jalisco, A. C. (CIATEJ) Public Research Center of the National Council of Science and Technology (CONACYT, México).
Conflict of Interest
The authors declare that the research was conducted in the absence of any commercial or financial relationships that could be construed as a potential conflict of interest.
Acknowledgments
The authors acknowledge the Centro de Investigación y Asistencia en Tecnología y Diseño del Estado de Jalisco, A. C. for allowing access to the facilities of the molecular microbiology laboratory to carry out this study. The authors also thank MSc Ricardo García de Alba García of the Centro Universitario del Sur of the University of Guadalajara for facilitating the development of the field work, and to Ph.D. Beatriz Quiñones (USDA) and Luis Alberto Arellano García (CIATEJ) for reviewing this manuscript.
Supplementary Material
The Supplementary Material for this article can be found online at: https://www.frontiersin.org/articles/10.3389/fmicb.2020.521146/full#supplementary-material
References
Abakpa, G. O., Umoh, V. J., Ameh, J. B., Yakubu, S. E., Kwaga, J. K. P., and Kamaruzaman, S. (2014). Diversity and antimicrobial resistance of Salmonella enterica isolated from fresh produce and environmental samples. Environ. Nanotechnol. Monitor. Manage. 3, 38–46. doi: 10.1016/j.enmm.2014.11.004
Abulreesh, H. H., Paget, T. A., and Goulder, R. (2004). Waterfowl and the bacteriological quality of amenity ponds. J. Water Health 2, 183–189. doi: 10.2166/wh.2004.0016
Abulreesh, H. H. (2012). Salmonellae in the Environment. Salmonella – Distribution, Adaptation, Control Measures and Molecular Technologies. doi: 10.5772/28201
Alaniz, O. R., Ríos, I. M., Rosas, B. B., and Morales, J. A. (1997). Antimicrobial resistance of strains isolated from animal sources. Vet. México 28, 215–220.
Amábile-Cuevas, C. F. (2010). Antibiotic resistance in Mexico: a brief overview of the current status and its causes. J. Infect. Devel. Count. 4, 126–131. doi: 10.3855/jidc.427
Andino, A., and Hanning, I. (2015). Salmonella enterica: survival, colonization, and virulence differences among serovars. Sci. World J. 2015, 520179. doi: 10.1155/2015/520179
Arvanitidou, M., Kanellou, K., and Vagiona, D. G. (2005). Diversity of Salmonella spp. and fungi in northern Greek rivers and their correlation to fecal pollution indicators. Environ. Res. 99, 278–284. doi: 10.1016/j.envres.2005.01.002
Baudart, J., Lemarchand, K., Brisabois, A., and Lebaron, P. (2000). Diversity of Salmonella strains isolated from the aquatic environment as determined by serotyping and amplification of the ribosomal DNA spacer regions. Appl. Environ. Microbiol. 66, 1544–1552. doi: 10.1128/aem.66.4.1544-1552.2000
BD (2013). BD(Hektoen Enteric Agar (HE Agar). Available online at: https://www.bd.com/resource.aspx?IDX=8970 (accessed June 06, 2020).
Boerlin, P., and Reid-Smith, R. J. (2008). Antimicrobial resistance: its emergence and transmission. Anim. Health Res. Rev. 9, 115–126. doi: 10.1017/S146625230800159X
Borchardt, M. A., Bertz, P. D., Spencer, S. K., and Battigelli, D. A. (2003). Incidence of enteric viruses in groundwater from household wells in Wisconsin. Appl. Environ. Microbiol. 69, 1172–1180. doi: 10.1128/aem.69.2.1172-1180.2003
Bou, G., Fernández-Olmos, A., García, C., Sáez-Nieto, J. A., and Valdezate, S. (2011). Bacterial identification methods in the microbiologı laboratory. Enfermedades Infeccios. Microbiol. Clínica 29, 601–608. doi: 10.1016/j.eimc.2011.03.012
Brands, D. A., Inman, A. E., Gerba, C. P., Maré, C. J., Billington, S. J., Saif, L. A., et al. (2005). Prevalence of Salmonella spp. in oysters in the United States. Appl. Environ. Microbiol. 71, 893–897. doi: 10.1128/AEM.71.2.893-897.2005
Callaway, T. R., Keen, J. E., Edrington, T. S., Baumgard, L. H., Spicer, L., Fonda, E. S., et al. (2005). Fecal prevalence and diversity of Salmonella species in lactating dairy cattle in four states. J. Dairy Sci. 88, 3603–3608. doi: 10.3168/jds.S0022-0302(05)73045-9
Centers for Disease Control and Prevention [CDC] (2004). Outbreak of Salmonella Serotype Enteritidis Infection Associated with Raw Almonds—United States and Canada, 2003—2004. Available online at: https://www.cdc.gov/mmwr/preview/mmwrhtml/mm5322a8.html (accessed November 14, 2018).
Centers for Disease Control and Prevention [CDC] (2008). Multistate outbreak of Salmonella Saintpaul Infections Linked to Raw Produce. Available online at: https://www.cdc.gov/salmonella/2008/raw-produce-8-28-2008.html (accessed February 28, 2019).
Centers for Disease Control and Prevention [CDC] (2013). Multistate Outbreak of Salmonella Saintpaul Infections Linked to Imported Cucumbers 2008. Available online at: https://www.cdc.gov/Salmonella/saintpaul-04-13/index.html (accessed February 28, 2019).
Centers for Disease Control and Prevention [CDC] (2016). Pulsed-Field Gel Electrophoresis (PFGE). Available online at: https://www.cdc.gov/pulsenet/pathogens/pfge.html (accessed June 16, 2020).
Centers for Disease Control and Prevention [CDC] (2017). The CDC PulseNet one-day (24-28 h) Standardized Laboratory Protocol for Molecular Subtyping of Escherichia coli O157:H7, Non-typhoidal Salmonella serotypes, and Shigella sonnei by pulsed Field Gel Electrophoresis (PFGE). Available online at: https://www.cdc.gov/pulsenet/pdf/ecoli-shigella-salmonella-pfge-protocol-508c.pdf (accessed February 11, 2020).
Centers for Disease Control and Prevention [CDC] (2019). Outbreak of Salmonella Infections Linked to Cavi Brand Whole, Fresh Papayas. Avilable at https://www.cdc.gov/salmonella/uganda-06-19/index.html (accessed June 04, 2020).
Centro Nacional de Excelencia Tecnológica en Salud (2012). Prevención, Diagnóstico Y Tratamiento de Fiebre Paratifoidea y Otras Salmonelosis en Niños y Adolescentes en Primero y Segundo Nivel de Atención. Available online at: http://dcs.uqroo.mx/paginas/guiasclinicas/gpc/docs/ISSSTE-253-12-ER.pdf (accessed June 08, 2019).
Centro Universitario del Sur (2015). Universitarios Inician Extracción de Lirio en La Laguna de Zapotlán. Available online at: http://www.cusur.udg.mx/es/noticia/universitarios-inician-extraccion-de-lirio-en-la-laguna-de-zapotlan (accessed July 31, 2019).
Chen, H. M., Wang, Y., Su, L. H., and Chiu, C. H. (2013). Nontyphoid Salmonella infection: microbiology, clinical features, and antimicrobial therapy. Pediatr. Neonatol. 54, 147–152. doi: 10.1016/j.pedneo.2013.01.010
Cherry, W. B., Hanks, J. B., Thomason, B. M., Murlin, A. M., Biddle, J. W., and Croom, J. M. (1972). Salmonellae as an index of pollution of surface waters. Appl. Microbiol. 24, 334–340. doi: 10.1128/aem.24.3.334-340.1972
Chiu, C., and Ou, J. T. (1996). Rapid identification of Salmonella serovars in feces by specific detection of virulence genes, invA and spvC, by an enrichment broth culture-multiplex PCR combination assay. J. Clin. Microbiol. 34, 2619–2622. doi: 10.1128/jcm.34.10.2619-2622.1996
Clinical and Laboratory Standards Institute [CLSI] (2005). Performance Standards for Antimicrobial Susceptibility Testing. 15th Informational Supplement, M100-S15, Wayne, PA: Clinical and Laboratory Standard Institute.
Comisión Estatal del Agua [CEA] (2012). La Laguna de Zapotlán vuelve a ser Un Vertedero de Aguas Negras. Available online at: http://www.jornada.unam.mx/2012/02/02/estados/033n1est (accessed June 01, 2019).
Comisión Estatal del Agua [CEA] (2015a). Ficha Técnica Hidrológica del Municipio de Zapotlán el Grande. Available online at: https://www.ceajalisco.gob.mx/doc/fichas_hidrologicas/region9/zapotlan%20el%20grande.pdf (accessed June 05, 2020).
Comisión Estatal del Agua [CEA] (2015b). Ficha Técnica Hidrológica del Municipio de Gómez Farías. Available online at: https://www.ceajalisco.gob.mx/doc/fichas_hidrologicas/region9/gomez%20farias.pdf (accessed June 05, 2020).
Comisión Nacional de Áreas Naturales Protegidas [CONANP] (2005). Programa de Conservación y Manejo de la Laguna de Zapotlán. Available online at: http://www.conanp.gob.mx/conanp/dominios/ramsar/docs/sitios/FIR_RAMSAR/Jalisco/Laguna_de_Zapotlan/Laguna%20de%20Zapotlan.pdf (accessed June 08 2020).
Cook, K. L., and Bolster, C. H. (2007). Survival of Campylobacter jejuni and Escherichia coli in groundwater during prolonged starvation at low temperatures. J. Appl. Microbiol. 103, 573–583. doi: 10.1111/j.1365-2672.2006.03285.x
Crim, S. M., Iwamoto, M., Huang, J. Y., Griffin, P. M., Gilliss, D., Cronquist, A. B., et al. (2014). Incidence and trends of infection with pathogens transmitted commonly through food—foodborne diseases active surveillance network, 10 U.S. sites, 2006–2013. MMWR Morbid. Mortal. Weekly Rep. 63, 328–332.
Diputados Ciudadanos (2016). Laguna de Zapotlán. La SEMARNAT debe Implementar un Programa de Restauración en la Laguna de Zapotlán. Available online at: https://diputadosciudadanos.mx/boletines/laguna-de-zapotlan (accessed June 06, 2020).
Dirección General de Epidemiología [DGEPI] (2012). Boletín Epidemiológico. Dirección General de Epidemiología. Secretaría de Salud. México, D.F. Available online at: https://www.gob.mx/salud/acciones-y-programas/boletinepidemiologico-2012 (accessed March 23, 2019).
Dirección General de Epidemiología [DGEPI] (2016). Boletín Epidemiológico. Dirección General de Epidemiología. Secretaría de Salud. México, D.F. Available online at: https://www.gob.mx/cms/uploads/attachment/file/285868/sem52.pdf (accessed March 23, 2019).
Dirección General de Epidemiología [DGEPI] (2017). Boletín Epidemiológico. Dirección General de Epidemiología. Secretaría de Salud. México, D.F. Available online at: https://www.gob.mx/cms/uploads/attachment/file/425972/sem52.pdf (accessed March 23, 2019).
Doumith, M., Godbole, G., Ashton, P., Larkin, L., Dallman, T., et al. (2016). Detection of the plasmid-mediated mcr-1 gene conferring colistin resistance in human and food isolates of Salmonella enterica and Escherichia coli in England and Wales. J. Antimicrob. Chemother. 71, 2300–2305. doi: 10.1093/jac/dkw093
Dreser, A., Wirtz, V. J., Corbett, K. K., and Echániz, G. (2008). Antibiotic use in Mexico: review of probles and policies. Salud Pública de México 50, S480–S487. doi: 10.1590/S0036-36342008001000009
El-Gazzar, F. E., and Marth, E. H. (1992). Salmonellae, salmonellosis, and dairy foods: a review. J. Dairy Sci. 75, 2327–2343. doi: 10.3168/jds.S0022-0302(92)77993-4
Everitt, B., and Hothorn, T. (2011). An Introduction to Applied Multivariate Analysis with R, Use R!. New York, NY: Springer-Verlag.
Fatica, M. K., and Schneider, K. R. (2011). Salmonella and produce: survival in the plant environment and implications in food safety. Virulence 2, 573–579. doi: 10.4161/viru.2.6.17880
Ferguson, C., De Roda, H. A. M., Altavilla, N., Deere, D., and Ashbolt, N. (2003). Fate and transport of surface water pathogens in watersheds. Crit. Rev. Environ. Sci. Technol. 33, 299–361. doi: 10.1080/10643380390814497
Fish, J. T., and Pettibone, G. W. (1995). Influence of freshwater sediment on the survival of Escherichia coli and Salmonella sp. as measured by three methods of enumeration. Lett. Appl. Microbiol. 20, 277–281. doi: 10.1111/j.1472-765x.1995.tb00445.x
Gagliardi, J. V., Millner, P. D., Lester, G., and Ingram, D. (2003). On-Farm and postharvest processing sources of bacterial contamination to melon rinds. J. Food Prot. 66, 82–87. doi: 10.4315/0362-028x-66.1.82
Gal-Mor, O., Boyle, E. C., and Grassl, G. A. (2014). Same species, different diseases: how and why typhoidal and non-typhoidal Salmonella enterica serovars differ. Front. Microbiol. 5:391. doi: 10.3389/fmicb.2014.00391
García de Alba-García, R. (2006). Forest Activity in Southern Jalisco and its Influence on the Generation of Environmental Problems in the Zapotlán basin. [Unpublished master’s thesis]., Instituto Politécnico Nacional, Mexico.
Global Foodborne Infections Network Country Databank (2010). Global Foodborne Infections Network Country Databank. Available online at: http://thor.dfvf.dk/portal/page?_pageid=53,1&_dad=portal&_schema=PoRTAL (accessed February 23, 2019).
González-Cortés, A., Bessudo, D., Sánchez-Leyva, R., Fragoso, R., Hinojosa, M., and Becerril, P. (1974). Water-borne transmission of chloramphenicol-resistant Salmonella typhi in Mexico. Lancet 302, 605–607. doi: 10.1016/s0140-6736(73)92427-6
Gorski, L., Parker, C. T., Liang, A., Cooley, M. B., Jay-Russell, M. T., Gordus, A. G., et al. (2011). Prevalence, distribution, and diversity of Salmonella enterica in a major produce region of California. Appl. Environ. Microbiol. 77, 2734–2748. doi: 10.1128/aem.02321-10
Gradilla-Hernández, M. S., de Anda, J., Garcia-Gonzalez, A., Meza-Rodríguez, D., Yebra Montes, C., and Perfecto-Avalos, Y. (2019). Multivariate water quality analysis of Lake Cajititlán, Mexico. Environ. Monit. Assess 192:5. doi: 10.1007/s10661-019-7972-4
Greenberg, T. A. (2009). Nutrient Cycling and Water Pollution in Lake Zapotlán, Mexico [Unpublished master’s thesis]., University of Toronto, Toronto.
Greenberg, T. A., Shear, H., de Anda-Sánchez, J., and Ortíz-Jiménez, M. A. (2008). Preliminary analysis of water pollution in a small lake in Western Mexico. WIT Trans. Ecol. Environ. Water Pollut. IX 111, 13–21.
Gupte, A. R., De Rezende, C. L., and Joseph, S. W. (2003). Induction and resuscitation of viable but nonculturable Salmonella enterica serovar typhimurium DT104. Appl. Environ. Microbiol. 69, 6669–6675. doi: 10.1128/aem.69.11.6669-6675.2003
Gutiérrez-Cogco, L., Montiel-Vázquez, E., Aguilera-Pérez, P., and González-Andrade, M. C. (2000). Salmonella serotypes isolated in Mexico’s health services. Salud Publica de Mexico 42, 490–495.
Heinitz, M. L., Ruble, R. D., Wagner, D. E., and Tatini, S. R. (2000). Incidence of Salmonella in fish and seafood. J. Food Prot. 63, 579–592. doi: 10.4315/0362-028x-63.5.579
Huang, Y., Yang, C., Wen, C., and Wen, G. (2019). S-type dissolved oxygen distribution along water depth in a canyon-shaped and algae blooming water source reservoir: reasons and control. Int. J. Environ. Res. Public Health 16, 987. doi: 10.3390/ijerph16060987
Hurtado, F. (2001). Obtainment a Protein Hydrolysate Using Surpluses from the Fishing and Agricultural Industry. [Unpublished bachelor’s thesis]. Ecuador: Escuela Superior Politécnica del Litoral. Facultad de Ingeniería Mecánica y Ciencias de la Producción.
Hyytiä-Trees, E., Smole, S. C., Fields, P. A., Swaminathan, B., and Ribot, E. M. (2006). Second generation subtyping: a proposed PulseNet protocol for multiple-locus variable-number tandem repeat analysis of Shiga toxin-producing Escherichia coli O157 (STEC O157). Foodborne Pathog. Dis. 3, 118–131. doi: 10.1089/fpd.2006.3.118
Hyytiä-Trees, E. K., Cooper, K., Ribot, E. M., and Gerner-Smidt, P. (2007). Recent developments and future prospects in subtyping of foodborne bacterial pathogens. Fut. Microbiol. 2, 175–185. doi: 10.2217/17460913.2.2.175
International Commission on Microbiological Specifications for Foods (1996). Microorganisms in Food 5: Microbiological Specifications of Food Pathogens. Blackie Academic and Professional.
Iveson, J. B., Kovacs, N., and Laurie, W. M. (1964). An improved method of isolating Salmonellae from contaminated desiccated coconut. J. Clin. Pathol. 17, 75–78. doi: 10.1136/jcp.17.1.75
Jay, L. S., Davos, D., Dundas, M., Frankish, E., and Lightfoot, D. (2003). “Salmonella,” in Foodborne Microorganisms of Public Health Significance, 6th Edn, ed. A. D. Hocking (North Ryde, NSW: Australian Institute of Food Science and Technology (NSW Branch)), 207–266.
Jimenez, M., and Chaidez, C. (2011). Improving Salmonella determination in Sinaloa rivers with ultrafiltration and most probable number methods. Environ. Monit. Assess 184, 4271–4277. doi: 10.1007/s10661-011-2262-9
Jiménez, M., Martínez-Urtaza, J., and Chaidez, C. (2011). Geographical and temporal dissemination of Salmonellae isolated from domestic animal hosts in the Culiacan Valley, Mexico. Microb. Ecol. 61, 811–820. doi: 10.1007/s00248-010-9792-5
Johnson, J. Y., Thomas, J. E., Graham, T. A., Townshend, I., Byrne, J., Selinger, L. B., et al. (2003). Prevalence of Escherichia coli O157:H7 and Salmonella spp. in surface waters of southern Alberta and its relation to manure sources. Can. J. Microbiol. 49, 326–335. doi: 10.1139/w03-046
Kempf, A. J., Hulsebus, H. J., and Akbar, S. (2016). Multiple plasmids contribute to antibiotic resistance and macrophage survival in vitro in CMY2-Bearing Salm- onella enterica. Foodborne Pathog. Dis. 13, 398–404. doi: 10.1089/fpd.2015.2067
Kuang, X., Hao, H., Dai, M., Wang, Y., Ahmad, I., Liu, Z., et al. (2015). Serotypes and antimicrobial susceptibility of Salmonella spp. isolated from farm animals in China. Front. Microbiol. 6:602. doi: 10.3389/fmicb.2015.00602
Lailler, R., Grimont, F., Jones, Y., Sanders, P., and Brisabois, A. (2002). Subtyping of Salmonella typhimurium by pulsed-field gel electrophoresis and comparisons with phage types and resistance types. Pathol. Biol. 50, 361–368. doi: 10.1016/s0369-8114(02)00321-8
Lemarchand, K., and Lebaron, P. (2002). Influence of mutation frequency on the persistence of Salmonella enterica serotypes in natural waters. FEMS Microbiol. Ecol. 41, 125–131. doi: 10.1111/j.1574-6941.2002.tb00973.x
Leskinen, S. D., Brownell, M., Lim, D. V., and Harwood, V. J. (2010). Hollow-fiber ultrafiltration and PCR detection of human-associated genetic markers from various types of surface water in Florida. Appl. Environ. Microbiol. 76, 4116–4117. doi: 10.1128/AEM.00025-10
Levantesi, C., Bonadonna, L., Briancesco, R., Grohmann, E., Toze, S., and Tandoi, V. (2012). Salmonella in surface and drinking water: occurrence and water-mediated transmission. Food Res. Int. 45, 587–602. doi: 10.1016/j.foodres.2011.06.037
Li, B., Vellidis, G., Liu, H., Jay-Russell, M., Zhao, S., Hu, Z., et al. (2014). Diversity and antimicrobial resistance of Salmonella enterica isolates from surface water in Southeastern United States. Appl. Environ. Microbiol. 80, 6355–6365. doi: 10.1128/AEM.02063-14
Liu, H., Whitehouse, C. A., and Li, B. (2018). Presence and persistence of Salmonella in water: the impact on microbial quality of water and food safety. Front. Public Health 6:159. doi: 10.3389/fpubh.2018.00159
Liu, Y.-Y., Wang, Y., Walsh, T. R., Yi, L.-X., Zhang, R., Spencer, J., et al. (2016). Emergence of plasmid-mediated colistin resistance mechanism MCR-1 in animals and human beings in China: a microbiological and molecular biological study. Lancet Infect. Dis. 16, 161–168. doi: 10.1016/S1473-3099(15)00424-7
López, O., León, J., Jiménez, M., and Chaidez, C. (2009). Detection and antibiotic resistance of Escherichia coli and Salmonella in water and agricultural soil. Rev. Fitotecn. Mexic. 32, 119–126.
Lukinmaa, S., Nakari, U. M., Eklund, M., and Siitonen, A. (2004). Application of molecular genetic methods in diagnostics and epidemiology of food-borne bacterial pathogens. APMIS 112, 908–929. doi: 10.1111/j.1600-0463.2004.apm11211-1213.x
Luna, G. (1991). Manual Operativo de Análisis Microbiológicos para Alimentos, 1st Edn. Bogotá: Fundación Universidad de Bogotá Jorge Tadeo Lozano.
Malczyk, E. A., and Branfireun, B. A. (2015). Mercury in sediment, water, and fish in a managed tropical wetland-lake ecosystem. Sci. Total Environ. 52, 260–268. doi: 10.1016/j.scitotenv.2015.04.015
Martinez-Urtaza, J., Liebana, E., Garcia-Migura, L., Perez-Piñeiro, P., and Saco, M. (2004). Characterization of Salmonella enterica serovar Typhimurium from marine environments in coastal waters of Galicia (Spain). Appl. Environ. Microbiol. 70, 4030–4034. doi: 10.1128/AEM.70.7.4030-4034.2004
McEgan, R., Mootian, G., Goodridge, L. D., Schaffner, D. W., and Danyluk, M. D. (2013). Predicting Salmonella populations from biological, chemical, and physical indicators in Florida surface waters. Appl. Environ. Microbiol. 79, 4094–4105. doi: 10.1128/AEM.00777-13
Mhongole, O. J., Mdegela, R. H., Kusiluka, L. J. M., Forslund, A., and Dalsgaard, A. (2017). Characterization of Salmonella spp. from wastewater used for food production in Morogoro, Tanzania. World J. Microbiol. Biotechnol. 33, 42. doi: 10.1007/s11274-017-2209-6
Miranda, J. M., Mondragón, A. C., Martínez, B., Guarddon, M., and Rodríguez, J. A. (2009). Prevalence and antimicrobial resistance patterns of Salmonella from different raw foods in Mexico. J. Food Prot. 72, 966–971. doi: 10.4315/0362-028x-72.5.966
Morales, A. S., Fragoso, de Araújo, J., de Moura Gomes, V. T., Reis Costa, A. T., and dos Prazeres et al. (2012). Colistin resistance in Escherichia coli and Salmonella enterica strains isolated from swine in Brazil. Sci. World J. 2012, 109795. doi: 10.1100/2012/109795
Morales-Morales, H. A., Vidal, G., Olszewski, J., Rock, C. M., Dasgupta, D., Oshima, K. H., et al. (2003). Optimization of a reusable hollow-fiber ultrafilter for simultaneous concentration of enteric bacteria, protozoa, and viruses from water. Appl. Environ. Microbiol. 69, 4098–4102. doi: 10.1128/aem.69.7.4098-4102.2003
Morse, E. V., and Duncan, M. A. (1974). Salmonellosis –an environmental health problem. J. Am. Vet. Med. Assoc. 165, 1015–1019.
Murdoch, D. J., and Chow, E. D. (1996). A graphical display of large correlation matrices. Am. Statist. 50, 178–180. doi: 10.2307/2684435
Olaitan, A. O., Morand, S., and Rolain, J. M. (2014). Mechanisms of polymyxin resistance: acquired and intrinsic resistance in bacteria. Front. Microbiol. 5:643. doi: 10.3389/fmicb.2014.00643
Olarte, J., and Galindo, E. (1973). Salmonella typhi resistant to chloramphenicol, ampicillin, and other antimicrobial agents: strains isolated during an extensive typhoid fever epidemic in Mexico. Antimicrob. Agents Chemother. 4, 597–601. doi: 10.1128/aac.4.6.597
Ortíz-Jiménez, M. A., de Anda, J., and Shear, H. (2005). Hydrologic balance of lake zapotlán, México. J. Environ. Hydrol. 13, 1–16.
Ortíz-Jiménez, M. A., de Anda, J., and Shear, H. (2006). Nutrients/food chain model for Lake Zapotlán (Mexico). Int. J. River Basin Manage. 4, 125–135. doi: 10.1080/15715124.2006.9635282
Polo, F., Figueras, M., Inza, I., Sala, J., Fleisher, J., and Guarro, J. (1998). Relationship between presence of Salmonella and indicators of faecal pollution in aquatic habitats. FEMS Microbiol. Lett. 160, 253–256. doi: 10.1111/j.1574-6968.1998.tb12919.x
Pond, K., and World Health Organization [WHO] (2005). Water, Sanitation and Health Team?. Water Recreation and Disease: Plausibility of Associated Infections: Acute effects, Sequelae and Mortality. Available online at: https://apps.who.int/iris/handle/10665/43338 (accessed January 12, 2019).
Popoff, M. Y., and Le-Minor, L. (2001). Antigenic Formulas of the Salmonella serovars, 8th rev. Edn. Paris: Institut Pasteur.
Poppe, C., McFadden, K. A., and Demczuk, W. H. B. (1996). Drug resistance, plasmids, biotypes and susceptibility to bacteriophages of Salmonella isolated from poultry in Canada. Int. J. Food Microbiol. 30, 325–344. doi: 10.1016/0168-1605(96)00960-9
Prosser, J. I., Bohannan, B. J., Curtis, T. P., Ellis, R. J., Firestone, M. K., Freckleton, M. P., et al. (2007). The role of ecological theory microbial ecology. Nat. Rev. Microbiol. 5, 384–392. doi: 10.1038/nrmicro1643
Quiroz-Santiago, C., Rodas-Suárez, O. R., Vázquez, Q. C. R., Fernández, F. J., Quiñones-Ramírez, E. I., and Vázquez-Salinas, C. (2009). Prevalence of Salmonella in vegetables from Mexico. J. Food Prot. 72, 1279–1282. doi: 10.4315/0362-028x-72.6.1279
Rabsch, W., Andrews, H. L., Kingsley, R. A., Prager, R., Tschäpe, H., Adams, L. G., et al. (2002). Salmonella enterica serotype typhimurium and its host adapted variants. Infect. Immun. 70, 2249–2255. doi: 10.1128/iai.70.5.2249-2255.2002
RAMSAR (2007). The Annotated Ramsar List: Mexico. Available online at: http://www.ramsar.org/profile/profiles_mexico.htm (accessed June 11, 2020).
Scott, T. M., Rose, J. B., Jenkins, T. M., Farrah, S. R., and Lukasik, J. (2002). Microbial source tracking: current methodology and future directions. Appl. Environ. Microbiol. 68, 5796–5803. doi: 10.1128/aem.68.12.5796-5803.2002
Secretaría de Medio Ambiente y Desarrollo Territorial (2005). Programa de conservación y Manejo de la Laguna de Zapotlán. Available online at: https://semadet.jalisco.gob.mx/sites/semadet.jalisco.gob.mx/files/programa_de_conservacion_y_manejo.pdf (accessed June 24, 2019).
Secretaría de Medio Ambiente y Desarrollo Territorial (2013). 1466 Laguna de Zapotlán. Available online at: https://semadet.jalisco.gob.mx/medio-ambiente/biodiversidad/comite-estatal-humedales/92 (accessed March 16, 2019).
TuTiempo.net (2016). Clima Ciudad Guzmán. Datos climáticos 2016. Available online at: https://www.tutiempo.net/clima/2016/ws-766560.html (accessed August 25, 2020).
Shear, H., and de Anda, J. (2009). Preliminary selection of sustainability indicators for a small lake basin in Western México. Local Environ. 14, 557–574. doi: 10.1080/13549830902904144
Sidharth, P. M., Uttam, S., Subhash, T., Sanjay, D., Devi, P. S., Reshma, S., et al. (2017). Multivariate statistical data analysis – principal component analysis (PCA). Int. J. Livestock Res. 7, 60–78. doi: 10.5455/ijlr.20170415115235
Simental, L., and Martinez-Urtaza, J. (2008). Climate patterns governing the presence and permanence of Salmonellae in coastal areas of Bahia de Todos Santos, Mexico. Appl. Environ. Microbiol. 74, 5918–5924. doi: 10.1128/AEM.01139-08
Sistema de agua potable de Zapotlán (2012). Plan de Desarrollo del Sistema de Agua Potable de Zapotlán. Available online at: https://sapaza.gob.mx/images/documentos/planeacion-estrategica/plan%20general%20institucional%20del%20poder.pdf (accessed June 14, 2020).
Son, I., Zheng, J., Keys, C. E., Zhao, S., Meng, J., and Brown, E. W. (2013). Analysis of pulsed field gel electrophoresis profiles using multiple enzymes for predicting potential source reservoirs for strains of Salmonella Enteritidis and Salmonella typhimurium isolated from humans. Infect. Genet. Evol. 16, 226–233. doi: 10.1016/j.meegid.2013.01.020
Standard Method ISO 6579:2002 (2002). Microbiology of Food and Animal Feeding Stuffs Horizontal Method for the Detection of Salmonella spp. Switzerland: International Organization for Standardization.
Statgraphics. One-way Anova (2009). Statgraphics. One-way ANOVA. Available online at: https://cdn2.hubspot.net/hubfs/402067/PDFs/One-Way_ANOVA.pdf (accessed June 08, 2020).
Steele, M., and Odumeru, J. (2004). Irrigation water as source of foodborne pathogens on fruit and vegetables. J. Food Prot. 67, 2839–2849. doi: 10.4315/0362-028x-67.12.2839
Swaminathan, B., Barrett, T. J., Hunter, S. B., and Tauxe, R. V. (2001). PulseNet: the molecular subtyping network for foodborne bacterial disease surveillance, United States. Emerg. Infect. Dis 7, 382–389. doi: 10.3201/eid0703.010303
Tamada, Y., Nakaoka, Y., Nishimori, K., Doi, A., Kumaki, T., Uemura, N., et al. (2001). Molecular typing and epidemiological study of Salmonella enterica serotype typhimurium isolates from cattle by fluorescent amplified-fragment length polymorphism fingerprinting and pulsed-field gel electrophoresis. J. Clin. Microbiol. 39, 1057–1066. doi: 10.1128/JCM.39.3.1057-1066.2001
Tavechio, A. T., Ghilardi, ÂC. R., Peresi, J. T. M., Fuzihara, T. O., Yonamine, E. K., Jakabi, M., et al. (2002). Salmonella serotypes isolated from nonhuman sources in São Paulo, Brazil, from 1996 through 2000. J. Food Prot. 65, 1041–1044. doi: 10.4315/0362-028x-65.6.1041
Tholozan, J. L., Cappelier, J. M., Tissier, J. P., Delattre, G., and Federighi, M. (1999). Physiological characterization of viable-but-nonculturable Campylobacter jejuni cells. Appl. Environ. Microbiol. 65, 1110–1116. doi: 10.1128/aem.65.3.1110-1116.1999
Thurston-Enriquez, J. A., Watt, P., Dowd, S. E., Enriquez, R., Pepper, I. L., and Gerba, C. P. (2002). Detection of protozoan parasites and microsporidia in irrigation waters used for crop production. J. Food Prot. 65, 378–382. doi: 10.4315/0362-028x-65.2.378
Trichopoulos, D., Daskalopoulo, G., Kalapothaki, V., Kalandidi, A., and Vassiliadis, P. (1972). Secondary enrichment in rappaport medium in the isolation of Salmonella, from pig organs. Z. Bakteriol. Parasitenk. Infektionskrankheiten 2193, 306–312.
U.S. Environmental Protection Agency (2003). Environmental Technology Verification Report. YSI Incorporated 6600 EDS Multi-Parameter Water Quality Probe/Sonde. Available online at: https://archive.epa.gov/nrmrl/archive-etv/web/pdf/01_vr_ysi.pdf (accessed June 12, 2020).
Vásquez, E., Mattar, S., Mossos, N., Mogollón, D., and Poutou, R. A. (2005). Caracterización molecular de cepas colombianas de Salmonella spp. a través de RFLP-IS 200. NOVA-Publ. Científ. 3, 1–120.
Voss-Rech, D., Potter, L., Vaz, C. S. L., Pereira, D. I. B., Sangioni, L. A., Vargas, A. C., et al. (2016). Antimicrobial resistance in nontyphoidal Salmonella isolated from human and poultry-related samples in Brazil: 20-Year meta-analysis. Foodborne Pathog. Dis. 14, 116–124. doi: 10.1089/fpd.2016.2228
Wanjugi, P., and Harwood, V. J. (2012). The influence of predation and competition on the survival of commensal and pathogenic fecal bacteria in aquatic habitats. Environ. Microbiol. 15, 517–526. doi: 10.1111/j.1462-2920.2012.02877.x
Wilkes, G., Edge, T. A., Gannon, V. P. J., Jokinen, C., Lyautey, E., Neumann, N. F., et al. (2011). Associations among pathogenic bacteria, parasites, and environmental and land use factors in multiple mixed-use watersheds. Water Res. 45, 5807–5825. doi: 10.1016/j.watres.2011.06.021
Winfield, M. D., and Groisman, E. A. (2003). Role of nonhost environments in the lifestyles of Salmonella and Escherichia coli. Appl. Environ. Microbiol. 69, 3687–3694. doi: 10.1128/aem.69.7.3687-3694.2003
World Health Organization Global Foodborne Infections Network (2010). Laboratory Protocol “Isolation of Salmonella spp. from Food and Animal Faeces. Available online at: http://antimicrobialresistance.dk/CustomerData/Files/Folders/6-pdf-protocols/63_18-05-isolation-of-salm-220610.pdf (accessed June 06, 2020).
Xu, H. S., Roberts, N., Singleton, F. L., Attwell, R. W., Grimes, D. J., and Colwell, R. R. (1982). Survival and viability of non-culturable Escherichia coli and Vibrio cholerae in the estuarine and marine environment. Microb. Ecol. 8, 313–323. doi: 10.1007/BF02010671
YSI (2009). 6-Series Multiparameter Water Quality Sondes, user manual. Available online at: https://www.ysi.com/File%20Library/Documents/Manuals/069300-YSI-6-Series-Manual-RevJ.pdf (accessed February 14, 2016).
Zaidi, M. B., Dreser, A., and Figueroa, I. M. (2014). A collaborative initiative for the containment of antimicrobial resistance in Mexico. Zoonoses Public Health 62(Suppl. 1), 52–57. doi: 10.1111/zph.12166
Zansky, S., Wallace, B., Schoonmaker-Bopp, D., Smith, P., Ramsey, F., Painter, J., et al. (2002). From the centers for disease control and prevention, outbreak of multidrug resistant salmonella newport-United States. JAMA 288, 951–953. doi: 10.1001/jama.288.8.951
Zhang, Y., Bi, P., and Hiller, J. E. (2010). Climate variations and Salmonella infection in Australian subtropical and tropical regions. Sci. Total Environ. 408, 524–530. doi: 10.1016/j.scitotenv.2009.10.068
Zhao, S., White, D. G., Friedman, S. L., Glenn, A., Blickenstaff, K., Ayers, S. L., et al. (2008). Antimicrobial resistance in Salmonella enterica serovar Heidelberg isolates from retail meats, including poultry, from 2002 to 2006. Appl. Environ. Microbiol. 74, 6656–6662. doi: 10.1128/AEM.01249-08
Zheng, J., Keys, C. E., Zhao, S., Ahmed, R., Meng, J., and Brown, E. W. (2010). Simultaneous analysis of multiple enzymes increases accuracy of pulsed-field gel electrophoresis in assigning genetic relationships among homogeneous Salmonella strains. J. Clin. Microbiol. 49, 85–94. doi: 10.1128/JCM.00120-10
Keywords: Salmonella distribution, Salmonella serotypes, antimicrobial resistance, pulsed-field gel electrophoresis, lake
Citation: Díaz-Torres O, Lugo-Melchor OY, de Anda J, Gradilla-Hernández MS, Amézquita-López BA and Meza-Rodríguez D (2020) Prevalence, Distribution, and Diversity of Salmonella Strains Isolated From a Subtropical Lake. Front. Microbiol. 11:521146. doi: 10.3389/fmicb.2020.521146
Received: 24 February 2020; Accepted: 17 August 2020;
Published: 10 September 2020.
Edited by:
Zhi Ruan, Zhejiang University, ChinaReviewed by:
Cheryl P. Andam, University of New Hampshire, United StatesJames Pettengill, U.S. Food & Drug Administration, United States
Copyright © 2020 Díaz-Torres, Lugo-Melchor, Anda-Sánchez, Gradilla-Hernández, Amézquita-López and Meza-Rodríguez. This is an open-access article distributed under the terms of the Creative Commons Attribution License (CC BY). The use, distribution or reproduction in other forums is permitted, provided the original author(s) and the copyright owner(s) are credited and that the original publication in this journal is cited, in accordance with accepted academic practice. No use, distribution or reproduction is permitted which does not comply with these terms.
*Correspondence: Ofelia Yadira Lugo-Melchor, eWx1Z29AY2lhdGVqLm14; José de Anda, amFuZGFAY2lhdGVqLm14