- 1Department of Medical Microbiology, School of Biology and Basic Medical Science, Medical College of Soochow University, Suzhou, China
- 2Medical Research Center, The People's Hospital of Suzhou New District, Suzhou, China
Salmonella enterica serovar Typhimurium (S). Typhimurium is a primary foodborne pathogen infecting both humans and animals. Salmonella plasmid virulence C (spvC) gene is closely related to S. Typhimurium dissemination in mice, while the mechanisms remain to be fully elucidated. Pyroptosis, a gasdermin-mediated inflammatory cell death, plays a role in host defense against bacterial infection, whereas the effect of spvC on pyroptosis and its function in inflammatory injury induced by S. Typhimurium are rather limited. In our study, C57BL/6 mice and J774A.1 cells infected with S. Typhimurium wild-type strain SL1344, spvC deletion mutant, spvC K136A site-directed mutant, and complemented strain were used to investigate potential pathogenesis of spvC. We verity that SpvC attenuates intestinal inflammation, suppresses pyroptosis through phosphothreonine lyase activity, and reduces pyroptosis in the ceca. Moreover, the reduction of inflammation via spvC results in systemic infection. These findings demonstrate that spvC inhibits pyroptosis and intestinal inflammation to promote bacterial dissemination, which provide new strategies for controlling systemic infection caused by Salmonella and novel insights for the treatment of other corresponding diseases.
Introduction
Salmonella, a facultative intracellular Gram-negative bacterium, is responsible for a wide range of food- and water-borne diseases ranging from gastroenteritis to typhoid fever depending on hosts and serotypes. Salmonella is responsible for a recent global estimated 9% cases of diarrhea annually, which results in disproportionate 41% of all diarrheal-associated mortality (Besser, 2018). The pathogenic factors of Salmonella include endotoxin, enterotoxin, and virulent effectors encoded by bacterial genetic elements. Most pathogenic Salmonella harbor a pSLT virulence plasmid of around 90 kb that contains a highly conserved 8-kb region of five genes (spvRABCD), which have been reported to be implicated in intracellular survival and growth (Guiney and Fierer, 2011; Passaris et al., 2018). Previously, we showed that Salmonella spvB gene could reduce host cell autophagy and regulate intracellular iron homeostasis (Chu et al., 2016; Yang et al., 2019). spvC is another essential factor of Salmonella virulence determinant, and its encoding product, SpvC, shares 63% identity at the amino acid level with OspF of Shigella flexneri and exhibits the same phosphothreonine lyase activity on host mitogen-activated protein kinase (MAPK). spvC leads to attenuation of the intestinal inflammatory response, which is thought to be important during systemic infection of Salmonella (Li et al., 2007; Mazurkiewicz et al., 2008; Haneda et al., 2012).
Pyroptosis, a programmed cell death (PCD) mediated by gasdermin family proteins, is characterized by cell swelling; and the terminal event is represented by rupture of the cell membrane, causing release of cytoplasmic contents of the cell, including pro-inflammatory cytokines, endogenous ligands, alarmins, and other danger-associated molecular patterns (DAMPs) (Gaidt and Hornung, 2016). Pyroptosis is regulated via a Caspase-1-dependent or Caspase-1-independent pathway; the latter is mediated by human Caspase-4, Caspase-5, or mouse Caspase-11 (Man et al., 2017). Canonical inflammasome pathway is activated in response to cytosolic pattern recognition receptors (PRRs) such as nucleotide-binding oligomerization domain, leucine-rich repeat and pyrin domain-containing 3 (NLRP3), and NLR with CARD domain-containing 4 (NLRC4). These receptors assemble Procaspase-1 into multiprotein complexes, which serve as Caspase-1-activating platforms. On the other hand, the non-canonical inflammasome pathway results in the cleavage of Caspase-4/5/11. Caspase-1/4/5/11 functioned as “molecular scissors” cleave not only gasdermin D (GSDMD) but also interleukin (IL)-1β (IL-1β) and IL-18 (Feng et al., 2018; Rathinam et al., 2019). Gasdermin family comprises six paralogous genes: GSDMA, GSDMB, GSDMC, GSDMD, GSDME (also known as DFNA5), and PJVK (also known as DFNB59) and are components of a cell death program (Broz et al., 2020) Both gasdermin B (GSDMB) and gasdermin E (GSMDE) act as tumor suppressors via triggering pyroptosis and promote tumor clearance, while GSDMD is a crucial executor of pyroptosis in anti-infection immunity (Pandeya et al., 2019; Wang et al., 2020; Zhou et al., 2020). Binding of the GSDMD N terminal (GSDMD-NT) domain β1–β2 loops to a common hydrophobic pocket in the GSDMD C terminal (GSDMD-CT) domain compromises auto-inhibition (Liu et al., 2019). The activated fragment GSDMD-NT oligomerizes in the cell membrane by specific binding to phosphoinositides and cardiolipin to form a pore of 10–16 nm in diameter, through which substrates of a smaller diameter, such as IL-1β, are secreted. Host cells not only expose the intracellular pathogens by pyroptosis but also capture the pathogens by pore-induced intracellular traps (PITs), and then neutrophils phagocytize and remove PITs by efferocytosis to prevent the dissemination of bacteria (Jorgensen et al., 2016b; Kovacs and Miao, 2017).
Salmonella enterica serovar Typhimurium (S. Typhimurium) is one of the most common isolates in Salmonella. It could traverse the epithelial cells layer of small intestine, replicate in phagocytes, and disseminate, resulting in systemic infection. In this study, C57BL/6 mice and J774A.1 cells infected with S. Typhimurium-carrying, S. Typhimurium-free, or site-directed mutant spvC were used for in vivo and in vitro assays. We reveal the contribution of spvC to pathogenesis of S. Typhimurium via inhibition of pyroptosis and intestinal inflammation to promote bacterial dissemination.
Materials and Methods
Bacterial Strains and Growth Conditions
Salmonella Typhimurium wild-type strain SL1344 (STM-WT) was kindly provided by Professor Qian Yang (Nanjing Agricultural University, Nanjing, China). STM-WT, spvC deletion mutant (STM-ΔspvC), and spvC site-directed mutant (STM-c-spvC K136A), which lacks phosphothreonine lyase activity and complemented strain (STM-c-spvC), were grown at 37°C in Luria Bertani (LB, Hangwei, China) broth overnight. STM-c-spvC K136A and STM-c-spvC were cultured in the media with 100 μg/ml of ampicillin (Sigma, USA). On the day of infection, S. Typhimurium were subcultured (1:100) for 3 h at 37°C in fresh LB broth to generate bacteria grown to log phase. Both STM-c-spvC K136A and STM-c-spvC were supplemented with 0.2% L-arabinose (Sigma, USA). Bacteria were then washed three times in phosphate-buffered saline (PBS), quantified by OD600.
Construction of spvC Deletion Mutant, Site-Directed Mutant, and Complemented Strain
The primers used for bacterial strains construction are listed in Supplementary Table 1. STM-ΔspvC was constructed using λRed recombination system (Song et al., 2010); and the corresponding plasmids were gifts from Professor Daoguo Zhou (Purdue University, West Lafayette, USA). pBAD/gIII expression system was used to construct STM-c-spvC K136A and STM-c-spvC (Szeliova et al., 2016). spvC K136A allele was amplified by overlap PCR. spvC deletion mutant, site-directed mutant, and complemented strain were identified by PCR and sequencing.
Cell Culture and Bacterial Infection
J774A.1 cells were purchased from the Procell Life Science & Technology Co., Ltd. Cells were routinely cultured in Dulbecco's modified Eagle medium (HyClone Laboratories, Logan, UT, USA) supplemented with 10% (v/v) fetal bovine serum (Biological Industries, Kibbutz Beit-Haemek, Israel) at 37°C in a 5% CO2 atmosphere. Cells were seeded in 12-well plates at a density of 1 × 106 cells per well and co-cultured with S. Typhimurium at the multiplicity of infection (MOI) of 10:1. Cells were washed with PBS; and fresh medium containing amikacin (100 μg/ml, MilliporeSigma, Burlington, MA, USA) was added to kill the extracellular bacteria for 2 h. Afterwards, infected cells were washed and subsequently cultured in fresh medium containing amikacin (10 μg/ml, MilliporeSigma) to limit extracellular replication of bacteria. Proteins were extracted at 8 hpi using radioimmunoprecipitation assay (RIPA) buffer containing protease inhibitors and phosphatase inhibitors (Beyotime Biotechnology).
Mice Experiment
Specific-pathogen-free (SPF) C57BL/6 mice aged 6–8 weeks were purchased from the experimental animal center of Soochow University and bred locally. Mice (fasted for 4 h) were given an oral dose of 100 μl, 200 mg/ml of streptomycin (Sigma, USA) and then resumed normal diet. Mice were randomly divided into three groups, including control, STM-WT, and STM-ΔspvC infection groups; 1 × 108 colony-forming unit (CFU) S. Typhimurium strains, respectively, in 100 μl of PBS were used for oral gavage to mice that were fasted for 4 h before infection. The cecum, liver, spleen, and serum were collected at 24 h post infection (hpi) and 72 hpi for the subsequent experiments.
Histopathological Analysis
Tissue samples of the liver and cecum from infected mice were washed three times with PBS and fixed in 10% formaldehyde solution at 4°C overnight. Then samples were processed with routine histological procedures, dehydration, paraffin embedding, section cutting, and deparaffinization. The sections were stained with hematoxylin–eosin (Baso, Zhuhai, China) and observed under a light microscope (Olympus, Japan).
Bacterial Burden Measurement
Fresh liver and spleen were harvested and immersed in 100 μg/ml of amikacin for 1 h, and tissues were homogenized at 0.3% Triton (Sigma, USA) for 30 min. Tissue homogenate was serially diluted 1:10 in soft agar (55°C) and plated on SS (Salmonella–Shigella) agar plates (Hangwei, Hangzhou, China). Colonies were counted after 16 h.
Western Blot Analysis
Thirty milligrams of the ceca was cut into pieces and lysed in 1 ml of RIPA buffer containing protease inhibitors and phosphatase inhibitors (Beyotime Biotechnology). Samples were homogenized on ice, centrifuged for supernatant at 12,000 rpm for 30 min at 4°C, and heated to 100°C for 5 min. Protein extracts resuspended in sample loading buffer were separated by electrophoresis through 12–15% polyacrylamide gels. Following electrophoretic transfer of proteins onto polyvinylidene difluoride (PVDF) membranes (Millipore), non-specific binding was blocked by incubation with 5% non-fat dry milk (Sangon Biotech Shanghai Co., Ltd.), and then membranes were incubated with primary antibodies anti-phospho-ERK1/2 and anti-phospho-JNK1/2 (1:1,000 dilution, Cell Signaling Technology); anti-NLRP3, anti-NLRC4, anti-Caspase-1, anti-Caspase-11, anti-GSDMD, and anti-histone H3 (1:1,000 dilution, abcam); anti-GAPDH (1:1,000 dilution, Boster); and anti-Tubulin (1:1,000, Beyotime) overnight at 4°C. Membranes were then washed and incubated with the horseradish peroxidase (HRP)-labeled goat anti-rabbit IgG (1:3,000 dilution, Beyotime) for 1 h at room temperature. Proteins were visualized using enhanced chemiluminescence (ECL) reagent (Meilunbio). The grayscale values of the bands were determined by ImageJ launcher broken symmetry software program (National Institutes of Health, Bethesda, MD, USA).
ELISA
To assess cytokine secretion during infection, C57BL/6 mice were bled, and serum IL-1β levels were assessed by ELISA at 24 and 72 hpi according to the kit instructions. ELISA kits were from Beyotime Biotechnology.
Statistical Analysis
Statistical significance was determined by independent Student's t-test for two groups and ANOVA for three or more groups. P < 0.05 was considered to be statistically significant.
Results
Effects of spvC on Salmonella Pathogenesis in Intestinal Inflammatory Response
A streptomycin-pretreated murine model was commonly used for Salmonella diarrhea (Kaiser et al., 2012). Thus, we developed a streptomycin-pretreated murine model to investigate Salmonella-induced intestinal inflammation. A previous study reported that spvC was required by Salmonella to modulate intestinal inflammatory response at 48 h post S. Typhimurium infection (Haneda et al., 2012). To further investigate the dynamic effect of spvC in intestine, we evaluated the pathological changes of the ceca in streptomycin-pretreated mice after S. Typhimurium infection. The expression of spvC in STM-c-spvC is induced by L-arabinose (0.2%); this condition could be fulfilled in vitro, while could not be ensured for in vivo assay. Thus, mice were infected orally with either the STM-WT or the STM-ΔspvC in our study. Macroscopic pictures of the ceca are shown in Figure 1A. The ceca length of mice infected with S. Typhimurium contracts slightly at 24 hpi compared with that of mice in the control group, while the marked contraction of the ceca is observed at 72 hpi. Importantly, shorter cecum is observed in STM-ΔspvC-infected mice than in STM-WT-infected mice at 72 hpi. Histopathological examination of the ceca in these mice supports the above results (Figure 1B). At 24 hpi, mice infected with S. Typhimurium show weak pathological changes in their ceca including slight swelling and infiltration of inflammatory cells into the lamina propria and submucosa compared with those in the uninfected group. The lesions aggravate at 72 hpi. Pronounced cecal inflammation is also observed in mice infected with STM-ΔspvC than in those infected with STM-WT, including epithelial cell swelling and inflammatory cell infiltration in the lamina propria, as well as atrophy in the submucosa. Together, these results indicate that the deletion of spvC leads to an exacerbation of the inflammatory response.
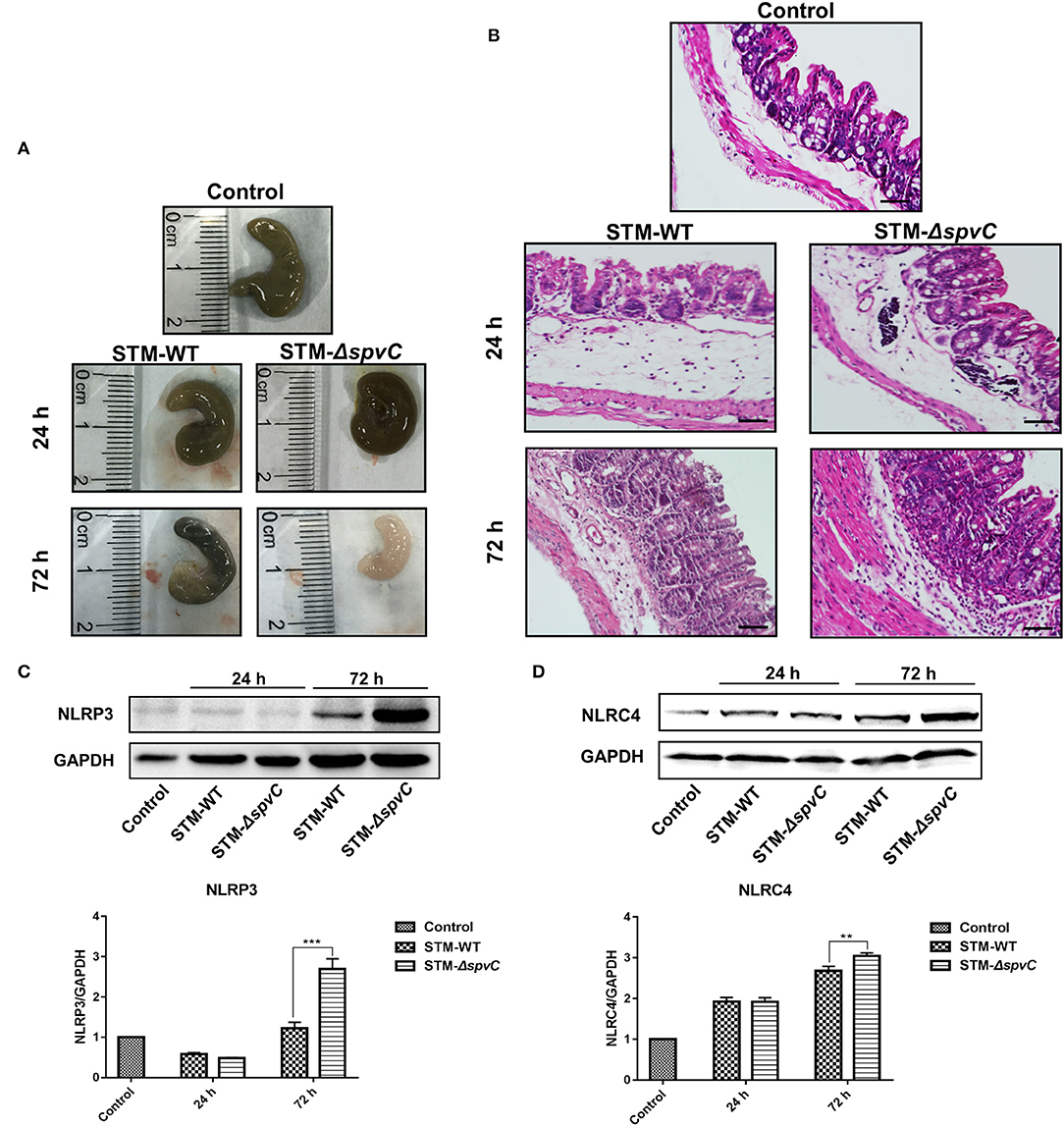
Figure 1. Effects of spvC on Salmonella pathogenesis in intestinal inflammatory response. Mice were infected orally with 1 × 108 colony-forming unit (CFU) of either the STM-WT or the STM-ΔspvC and analyzed at 24 and 72 hpi. (A) Macroscopic pictures of the ceca. (B) Histopathological analysis of the ceca; scale bars, 50 μm. (C) Western blot analysis of whole cecum with specific antibodies to NLRP3 and the control GAPDH. (D) Western blot analysis of whole cecum with specific antibodies to NLRC4 and the control GAPDH. Data were compared by independent Student's t-test. Values are expressed as the means ± SD, and statistically significant differences are indicated. ***P < 0.001; **P < 0.01. Data were from at least three experiments.
PAMPs can function as stimuli to activate inflammatory signaling receptor NLRP3 and NLRC4 (Karki et al., 2018; Swanson et al., 2019). Although the roles of NLRP3 and NLRC4 in intestinal immune defense against S. Typhimurium invasion have been studied, their precise functions of spvC-mediated Salmonella infection in the gut remain elusive (Li et al., 2017; Rauch et al., 2017). To further investigate the underlying mechanisms, NLRP3 and NLRC4 in the ceca of mice infected with S. Typhimurium strains were analyzed by western blot (Figures 1C,D). Results show the increased expression of NLRP3 and NLRC4 at 72 hpi compared with those in the control groups. Notably, higher protein levels of NLRP3 and NLRC4 are found in STM-ΔspvC-infected mice than those in STM-WT-infected mice. These data suggest that spvC regulates NLRP3 and NLRC4 negatively, which may relate to the inhibition of intestinal inflammation.
spvC Mediates Salmonella-Suppressing Pyroptosis in Macrophages via Its Phosphothreonine Lyase Activity
Pyroptosis that could be activated by NLRP3 and NLRC4 strengthened host defense function by its restriction of intracellular bacteria through PITs and disruption of the pathogen replication niche (Shi et al., 2017; Evavold et al., 2018; Karki et al., 2018). Consequently, pathogens as well as PITs released to extracellular space were available to be eliminated by phagocytes and downstream cell intrinsic defenses (Kovacs and Miao, 2017). It is known that spvC encoding protein, SpvC, is a Salmonella effector with phosphothreonine lyase activity. To investigate whether the enzymatic activity of SpvC is involved in its effect on pyroptosis, J774A.1 cells were co-cultured with STM-WT, STM-ΔspvC, STM-c-spvC K136A, which is a directed mutant in the phosphothreonine lyase activity site and STM-c-spvC for 8 hpi. As expected, the levels of NLRP3 and NLRC4 were significantly increased after infection with S. Typhimurium. Both STM-ΔspvC and STM-c-spvC K136A give rise to elevated levels of NLRP3 and NLRC4 in their infected macrophages compared with S. Typhimurium-carrying spvC-infected cells. However, the same levels of NLRP3 and NLRC4 were detected between cells infected with STM-ΔspvC and STM-c-spvC K136A (Figures 2A,B). We next assessed GSDMD, an executor of pyroptosis and its pore-forming domain GSDMD-NT. In line with the changing trend of NLRP3 and NLRC4, levels of GSDMD-NT are significantly higher in cells infected with STM-ΔspvC and STM-c-spvC K136A than in those infected with STM-WT and STM-c-spvC (Figure 2C). Moreover, J774A.1 cells were co-cultured with STM-WT, STM-ΔspvC, and STM-c-spvC after being pretreated with MAPK inhibitors (ERK inhibitor PD98059, JNK inhibitor SP600125, and P38 inhibitor SB203580) to study the change of NLRP3 and NLRC4 protein levels (Supplementary Figure 1). The aforementioned results demonstrate that the phosphothreonine lyase activity of SpvC is required to inhibit pyroptosis.
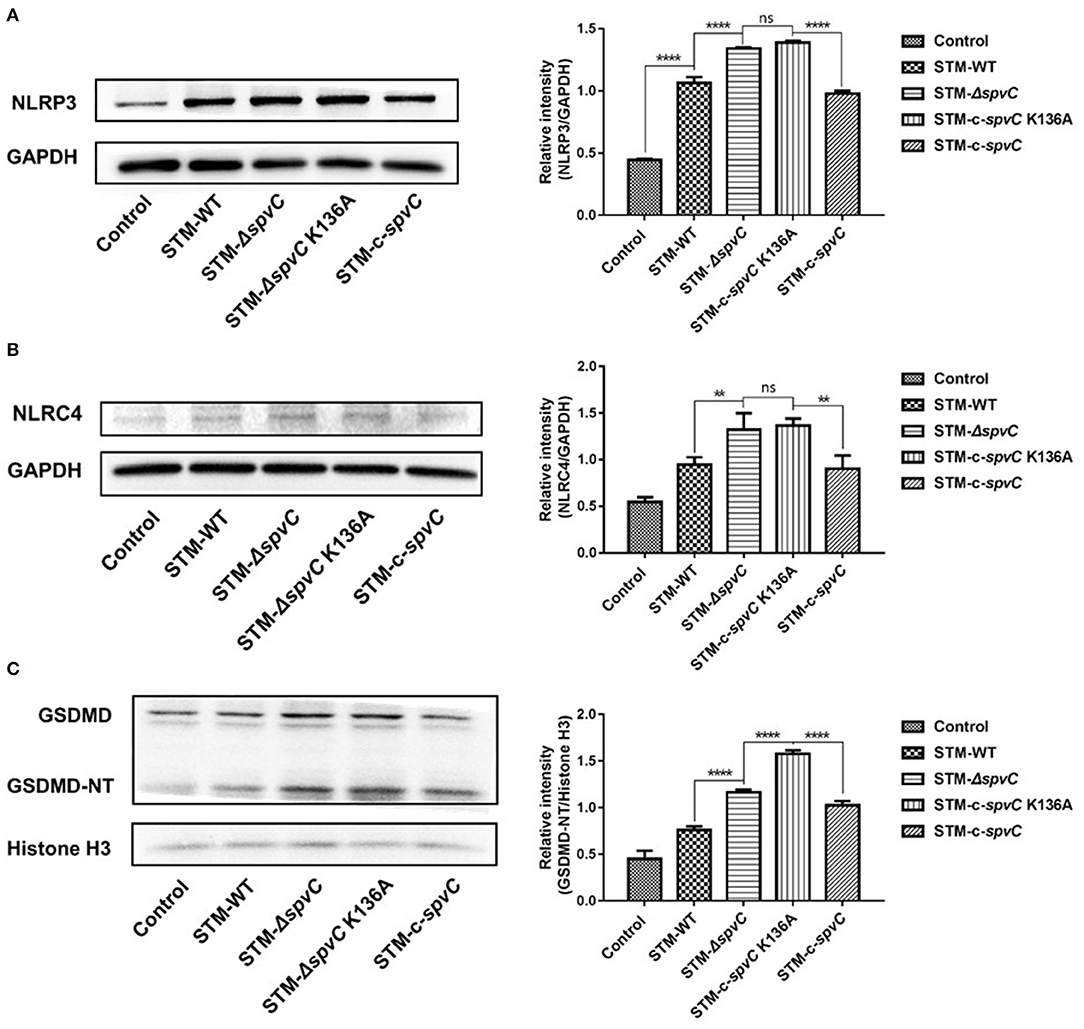
Figure 2. spvC mediates Salmonella-suppressing pyroptosis in macrophages via its phosphothreonine lyase activity. J774A.1 cells were infected with STM-WT, STM-ΔspvC, STM-c-spvC K136A, and STM-c-spvC and analyzed at 8 hpi. (A–C) Western blot analysis of cell lysates with specific antibodies to NLRP3, NLRC4, GSDMD, and the control GAPDH or histone H3. Data were compared by one-way ANOVA. Values are expressed as the means ± SD, and statistically significant differences are indicated. ****P < 0.0001; **P < 0.01; ns, not significant. Data were from at least three experiments.
spvC Attenuates Pyroptosis in the Ceca in Host Defense Against Salmonella Typhimurium Infection
To determine whether spvC attenuates pyroptosis in the ceca against S. Typhimurium invasion, western blot analysis reveals that the expression of GSDMD-NT in S. Typhimurium-infected mouse ceca is five-fold of that in the control group. Moreover, the deletion of spvC results in an increased expression of GSDMD-NT in the ceca (Figure 3A). These results indicate that spvC could attenuate pyroptosis in the ceca.
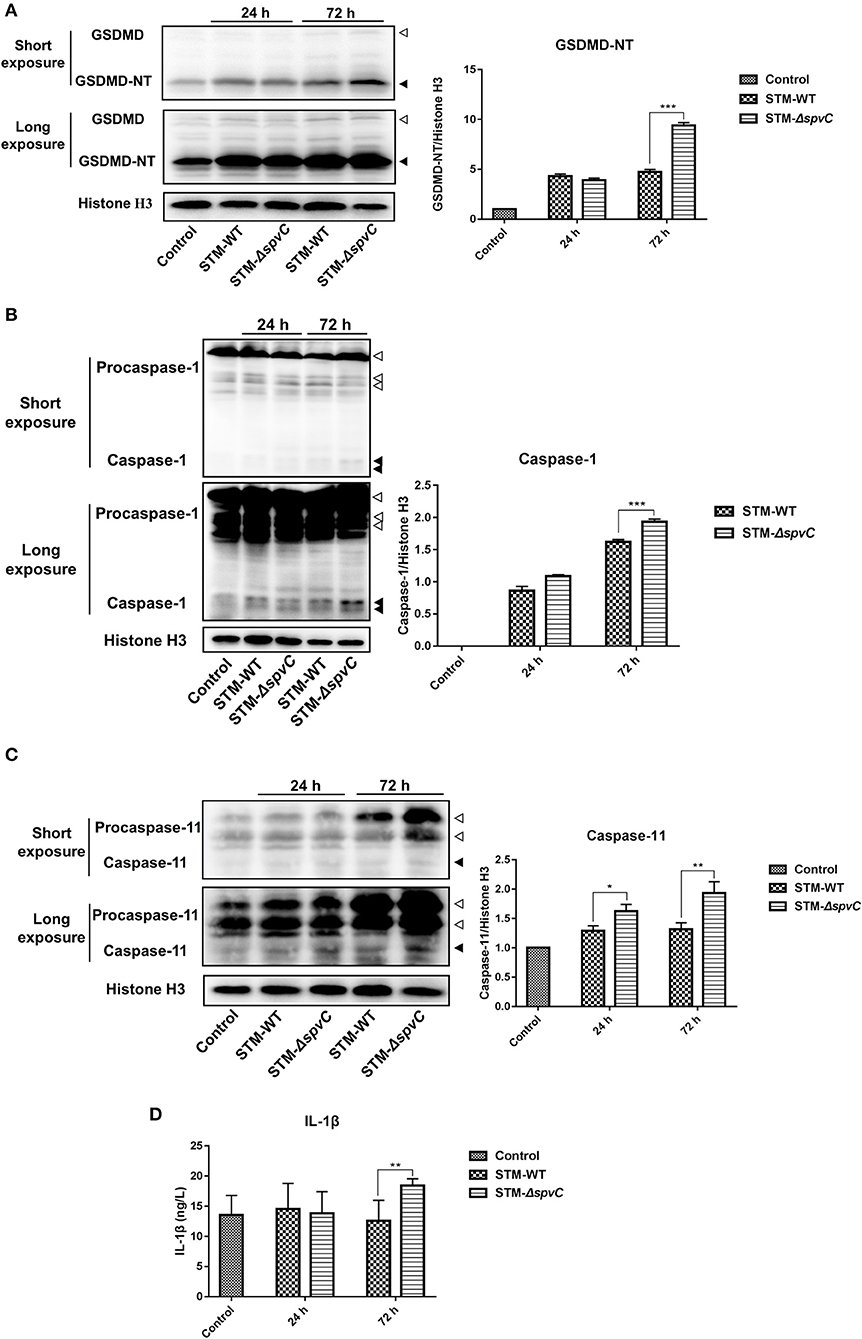
Figure 3. spvC attenuates pyroptosis in the ceca in host defense against Salmonella infection. Mice were infected orally with 1 × 108 colony-forming unit (CFU) of either the STM-WT or the STM-ΔspvC and analyzed at 24 and 72 hpi. Mice uninfected with Salmonella served as control groups. (A) Western blot analysis of whole cecum with specific antibodies to GSDMD and the control histone H3. White arrowheads indicate GSDMD, and black arrowheads indicate GSDMD-NT. (B) Western blot analysis of whole cecum with specific antibodies to Caspase-1 and the control histone H3. White arrowheads indicate Procaspase-1, and black arrowheads indicate Caspase-1. (C) Western blot analysis of whole cecum with specific antibodies to Caspase-11 and the control histone H3. White arrowheads indicate Procaspase-11, and black arrowheads indicate Caspase-11. (A–C) Data were from at least three experiments. (D) ELISA analysis of IL-1β in peripheral blood serums. Control group, n = 2; STM-WT 24 hpi group, n = 6; STM-ΔspvC 24 hpi group, n = 5; STM-WT 72 hpi group, n = 4; STM-ΔspvC 72 hpi group, n = 6. Data were compared by independent Student's t-test. Values are expressed as the means ± SD, and statistically significant differences are indicated. ***P < 0.001; **P < 0.01; *P < 0.05.
Two distinct pathways, named the canonical and non-canonical inflammasome pathways, initiate the assembly of Caspase-1 and Caspase-11 in mice, respectively, which in turn cleave GSDMD (Broz et al., 2020). To investigate which inflammasome pathway was involved in this model, we subsequently determined the hallmarks active fragments of Caspase-1 and Caspase-11. In line with the results obtained in Figure 3A, significantly more cleavage of Caspase-1 and Caspase-11 is determined in S. Typhimurium-infected ceca than that in the control groups. The expression of Caspase-1 and Caspase-11 in the ceca of mice infected with STM-ΔspvC is significantly higher than that in STM-WT-infected mice at 72 hpi (Figures 3B,C). These results reveal that spvC subverts cecal pyroptosis through both canonical and non-canonical inflammasome pathways.
In addition to cleaving GSDMD to render them bioactive and to induce pyroptotic cell death, auto-processed Caspase-1 matures the pro-inflammatory cytokine IL-1β and consequently triggers a robust inflammatory response (Gaidt and Hornung, 2016). Based on this, we find an increasing evidence of the IL-1β secretion in serum at 72 hpi due to the absence of spvC, which is consistent with the level of GSDMD-NT protein (Figure 3D). These findings suggest that spvC suppresses the secretion of IL-1β and attenuates host immune defense against infection.
spvC Is Required for Inflammatory Injury and Salmonella Typhimurium Dissemination
Reduced production of IL-1β and intestinal inflammatory response are responsible for S. Typhimurium systemic infection. A previous study demonstrated that a mutation in spvC did not affect bacterial colonization in the liver of mice infected with S. Typhimurium at 24 hpi, which was in agreement with our results (Haneda et al., 2012). However, with lastingness of infection, we find the exacerbation of inflammatory lesions in the liver of mice infected with S. Typhimurium as compared with control group. Moreover, mice infected with STM-WT have a more severe hepatic inflammation such as inflammatory cell infiltration in the hepatic lobules than those infected with STM-ΔspvC at 72 hpi (Figure 4A). These results indicate that spvC is required for hepatic injury during S. Typhimurium infection. Concomitantly, much more bacteria (~104 CFU/g) from livers are found in mice infected with S. Typhimurium-carrying spvC than those infected with STM-ΔspvC (Figure 4B). We further examine the bacterial loads in the spleen and find that the bacterial number in the spleen coincides with that in the liver (Figure 4C). These data confirm that spvC is required for S. Typhimurium dissemination in systemic organs.
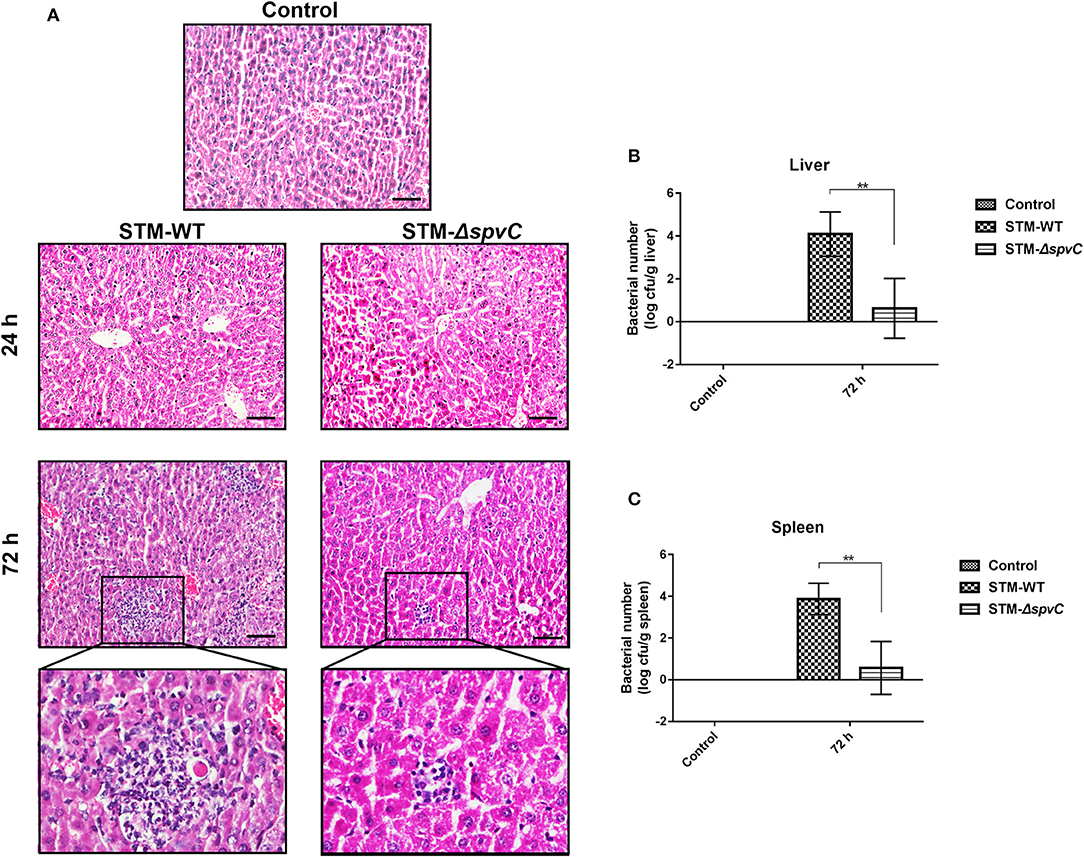
Figure 4. spvC is required for Salmonella dissemination and inflammatory injury. Mice were infected orally with 1 × 108 colony-forming unit (CFU) of either the STM-WT or the STM-ΔspvC and analyzed at 24 and/or 72 hpi. (A) Histopathological analysis of liver; scale bars, 50 μm. Data were from at least three experiments. (B) The bacterial load of livers. (C) The bacterial load of spleens. (B,C) Control group, n = 2; STM-WT 72 hpi group, n = 4; STM-ΔspvC 72 hpi group, n = 5. Data were compared by independent Student's t-test. Values are expressed as the means ± SD, and statistically significant differences are indicated. **P < 0.01.
Discussion
Salmonella are primary enteric pathogens infecting both humans and animals, among which S. Typhimurium is one of the most common isolates in clinical practice (Keestra-Gounder et al., 2015). After ingestion, S. Typhimurium invade preferentially in the cecum and terminal ileum in mice (Kaiser et al., 2012). A previous study showed that spvC could restrain intestinal inflammatory response during the early stages of infection in BALB/c mice infected with Salmonella strain 14,028 (Haneda et al., 2012). While the interaction between bacteria and host is very complex, Salmonella-induced intestinal inflammatory response may be affected by different virulent strains and host background genetics. In this study, we established the in vivo model using C57BL/6 mice infected with Salmonella strain SL1344. Data are in line with the earlier report. In a word, spvC exerts universal anti-inflammatory effect to pathogenesis of S. Typhimurium. To assess a possible correlation between intestinal inflammation and the onset of systemic infection, we extend these studies by monitoring the time course of length and pathology of the ceca. As expected, the inflammatory lesions in the lamina propria and submucosa of the ceca in mice exacerbated at 72 hpi. This suggests that spvC time dependently modulates host immune response by downregulating intestinal inflammation.
Inflammasomes are signaling hubs that activate inflammatory signaling cascades to drive host defense against invading pathogens. A previous study revealed that SPI2 T3SS could disrupt NLRP3 and NLRC4 inflammasome responses (Bierschenk et al., 2017). We further demonstrate that spvC, the effector of SPI2 T3SS, downregulates the expression of NLRP3 and NLRC4 in the ceca of mice infected with S. Typhimurium. Moreover, NLRP3 is uniquely activated by a wide variety of stimuli, including microbial motifs, endogenous danger signals, and environmental irritants (Swanson et al., 2019). In contrast, NLRC4 is mostly triggered by cytosolic flagellin (Duncan and Canna, 2018). This discrepancy probably leads to the susceptibility of NLRP3 in response to Salmonella infection.
After entering the lamina propria at the site of Peyer's patches, S. Typhimurium are taken up by phagocytes (Broz et al., 2012). Migration of these infected phagocytes, predominantly macrophages, facilitates systemic dissemination of the bacteria via the bloodstream to several additional tissues, such as the spleen and liver. SpvC is a phosphothreonine lyase that exerts anti-inflammatory effects by inactivating dual-phosphorylated MAPK through beta elimination (Li et al., 2007). We identify the enzymatic activity of SpvC, contributing to suppress NLRP3, NLRC4, and GSDMD-NT in macrophages, which might confer an advantage to the pathogen during host–pathogen competition. Furthermore, MAPK can transmit signals from the cell membrane to the nucleus, which may provide the first signal for transcription of inflammasomes (Zhao et al., 2019). So MAPK signaling pathway may be involved in the inhibitory effect of spvC on NLRP3 and NLRC4, subsequently suppressing pyroptosis.
Our previous study revealed that S. Typhimurium could cause PCD in several different ways including apoptosis and autophagy for pathogen clearance (Wu et al., 2010; Chu et al., 2014). Besides, Salmonella outer protein B (SopB) suppresses colitis development and increases bacteria pathogenesis via modulating necroptosis (Hu et al., 2019). Notably, experimental evidence reveals that S. Typhimurium trigger pyroptosis through both Caspase-1- and Caspase-11-dependent manner (Shi et al., 2015). In order to investigate the effect of spvC on pyroptosis in the ceca, GSDMD-NT, Caspase-1, and Caspase-11 were detected. The expression of GSDMD-NT, Caspase-1, and Caspase-11 in the ceca of mice infected with STM-ΔspvC increases to a comparable level to those in STM-WT-infected mice. This suggests that spvC inhibits pyroptosis through both canonical and non-canonical inflammasome pathways. Pyroptosis elicits a potent innate immune response resulting in IL-1β secretion (He et al., 2015). This effect functions as a robust host defense by recruiting secondary immune cells working as “scavengers” engulfing either infected dying cells or bacteria released into the extracellular space (Jorgensen et al., 2016a). Of interest, we find that the IL-1β level in serum of mice infected with S. Typhimurium without spvC is significantly higher than that in S. Typhimurium-carrying spvC-infected mice. These findings suggest that spvC decreases IL-1β secretion, which may attenuate host defense. This mechanism was probably the primary cause of the systemic infection by S. Typhimurium. Intriguingly, Caspase-8, a molecular switch for apoptosis, necroptosis, and pyroptosis, regulates an additional pathway controlling GSDMD-driven pyroptosis in Yersinia-infected macrophages (Orning et al., 2018). Whether the Caspase-8/GSDMD pathway is also involved in Salmonella infection deserves deep investigation.
Although gastroenteritis is a localized infection of the intestinal mucosa and mesenteric lymph nodes, the enhanced production of pro-inflammatory cytokines induces acute inflammation and is required for Salmonella clearance (Pradhan et al., 2020). We speculated that the effect of spvC on suppressing IL-1β secretion and attenuating host defense may lead to S. Typhimurium dissemination. Herein, we find that the lesions in livers of mice infected with STM-ΔspvC are slighter than those in STM-WT-infected mice. The viable bacteria isolated from murine livers and spleens infected with STM-WT at 72 hpi are about than 104 of those in STM-ΔspvC-infected mice. Hence, the spread of S. Typhimurium to the liver and spleen, where host defense limits bacterial replication and eliminates pathogen, is eventually increased by spvC. From the host's perspective, NAIP/NLRC4 inflammasome in intestinal epithelial cells (IECs) can prevent systemic dissemination of S. Typhimurium in vivo (Hausmann et al., 2020). To escape from the host, Salmonella in part overcomes this negative regulation of reverse transmigration into the bloodstream with spvC (Gopinath et al., 2019).
Conclusion
In summary, Salmonella infection models in vivo and in vitro were used to investigate potential pathogenesis of spvC gene via modulation of pyroptosis and intestinal inflammation, thereby increasing systemic dissemination. The present study demonstrates that SpvC alleviates intestinal inflammation, downregulates the expression of proteins related to pyroptosis through its enzymatic activity, subsequently inhibits cecal cell pyroptosis through both canonical and non-canonical inflammasome pathways, regulates the secretion of pro-inflammatory cytokines, and finally promotes bacterial dissemination in mice. These findings reveal a novel contribution of spvC to pathogenesis of S. Typhimurium by inhibiting pyroptosis and intestinal inflammation, and this effect may associate with immune strategy of systemic dissemination. Our research has potentially important significance to provide new paradigms for interactions between bacteria and host immune response, which would provide novel insights for controlling Salmonella infection and other infectious diseases.
Data Availability Statement
The original contributions presented in the study are included in the article/Supplementary Materials, further inquiries can be directed to the corresponding authors.
Ethics Statement
The animal study was reviewed and approved by the Animal Experimental Committee of the Soochow University.
Author Contributions
LZu, LZh, and SW designed the research and wrote the manuscript. LZu, LZh, CW, and YW performed the research and conducted the data analysis. YL, RH, and SW supervised the project and edited the manuscript. All authors have read and approved the manuscript.
Funding
The research leading to these results has received funding from the Natural Science Foundation of China (NSFC, Nos. 31970132, 81971899, 81671976 and 31670140) and the Priority Academic Program Development of Jiangsu Higher Education Institutions (PAPD).
Conflict of Interest
The authors declare that the research was conducted in the absence of any commercial or financial relationships that could be construed as a potential conflict of interest.
Supplementary Material
The Supplementary Material for this article can be found online at: https://www.frontiersin.org/articles/10.3389/fmicb.2020.562491/full#supplementary-material
References
Besser, J. M. (2018). Salmonella epidemiology: a whirlwind of change. Food Microbiol. 71, 55–59. doi: 10.1016/j.fm.2017.08.018
Bierschenk, D., Boucher, D., and Schroder, K. (2017). Salmonella-induced inflammasome activation in humans. Mol. Immunol. 86, 38–43. doi: 10.1016/j.molimm.2016.11.009
Broz, P., Ohlson, M. B., and Monack, D. M. (2012). Innate immune response to Salmonella typhimurium, a model enteric pathogen. Gut Microbes 3, 62–70. doi: 10.4161/gmic.19141
Broz, P., Pelegrin, P., and Shao, F. (2020). The gasdermins, a protein family executing cell death and inflammation. Nat. Rev. Immunol. 20, 143–157. doi: 10.1038/s41577-019-0228-2
Chu, Y., Gao, S., Wang, T., Yan, J., Xu, G., Li, Y., et al. (2016). A novel contribution of spvB to pathogenesis of Salmonella Typhimurium by inhibiting autophagy in host cells. Oncotarget 7, 8295–8309. doi: 10.18632/oncotarget.6989
Chu, Y., Yang, Y., Li, Y., Ye, Y., Yan, J., Wang, T., et al. (2014). A Salmonella enterica conjugative plasmid impairs autophagic flux in infected macrophages. Microbes Infect. 16, 553–561. doi: 10.1016/j.micinf.2014.05.001
Duncan, J. A., and Canna, S. W. (2018). The NLRC4 inflammasome. Immunol. Rev. 281, 115–123. doi: 10.1111/imr.12607
Evavold, C. L., Ruan, J., Tan, Y., Xia, S., Wu, H., and Kagan, J. C. (2018). The pore-forming protein gasdermin D regulates interleukin-1 secretion from living macrophages. Immunity 48, 35–44 e36. doi: 10.1016/j.immuni.2017.11.013
Feng, S., Fox, D., and Man, S. M. (2018). Mechanisms of gasdermin family members in inflammasome signaling and cell death. J. Mol. Biol. 430, 3068–3080. doi: 10.1016/j.jmb.2018.07.002
Gaidt, M. M., and Hornung, V. (2016). Pore formation by GSDMD is the effector mechanism of pyroptosis. EMBO J. 35, 2167–2169. doi: 10.15252/embj.201695415
Gopinath, A., Allen, T. A., Bridgwater, C. J., Young, C. M., and Worley, M. J. (2019). The Salmonella type III effector SpvC triggers the reverse transmigration of infected cells into the bloodstream. PLoS ONE 14:e0226126. doi: 10.1371/journal.pone.0226126
Guiney, D. G., and Fierer, J. (2011). The role of the spv genes in Salmonella pathogenesis. Front. Microbiol. 2:129. doi: 10.3389/fmicb.2011.00129
Haneda, T., Ishii, Y., Shimizu, H., Ohshima, K., Iida, N., Danbara, H., et al. (2012). Salmonella type III effector SpvC, a phosphothreonine lyase, contributes to reduction in inflammatory response during intestinal phase of infection. Cell Microbiol. 14, 485–499. doi: 10.1111/j.1462-5822.2011.01733.x
Hausmann, A., Bock, D., Geiser, P., Berthold, D. L., Fattinger, S. A., Furter, M., et al. (2020). Intestinal epithelial NAIP/NLRC4 restricts systemic dissemination of the adapted pathogen Salmonella Typhimurium due to site-specific bacterial PAMP expression. Mucosal Immunol. 13, 530–544. doi: 10.1038/s41385-019-0247-0
He, W. T., Wan, H., Hu, L., Chen, P., Wang, X., Huang, Z., et al. (2015). Gasdermin D is an executor of pyroptosis and required for interleukin-1beta secretion. Cell. Res. 25, 1285–1298. doi: 10.1038/cr.2015.139
Hu, G. Q., Yang, Y. J., Qin, X. X., Qi, S., Zhang, J., Yu, S. X., et al. (2019). Salmonella outer protein B suppresses colitis development via protecting cell from necroptosis. Front. Cell. Infect. Microbiol. 9:87. doi: 10.3389/fcimb.2019.00087
Jorgensen, I., Lopez, J. P., Laufer, S. A., and Miao, E. A. (2016a). IL-1beta, IL-18, and eicosanoids promote neutrophil recruitment to pore-induced intracellular traps following pyroptosis. Eur. J. Immunol. 46, 2761–2766. doi: 10.1002/eji.201646647
Jorgensen, I., Zhang, Y., Krantz, B. A., and Miao, E. A. (2016b). Pyroptosis triggers pore-induced intracellular traps (PITs) that capture bacteria and lead to their clearance by efferocytosis. J. Exp. Med. 213, 2113–2128. doi: 10.1084/jem.20151613
Kaiser, P., Diard, M., Stecher, B., and Hardt, W. D. (2012). The streptomycin mouse model for Salmonella diarrhea: functional analysis of the microbiota, the pathogen's virulence factors, and the host's mucosal immune response. Immunol. Rev. 245, 56–83. doi: 10.1111/j.1600-065X.2011.01070.x
Karki, R., Lee, E., Place, D., Samir, P., Mavuluri, J., Sharma, B. R., et al. (2018). IRF8 regulates transcription of Naips for NLRC4 inflammasome activation. Cell 173, 920–933 e913. doi: 10.1016/j.cell.2018.02.055
Keestra-Gounder, A. M., Tsolis, R. M., and Baumler, A. J. (2015). Now you see me, now you don't: the interaction of Salmonella with innate immune receptors. Nat. Rev. Microbiol. 13, 206–216. doi: 10.1038/nrmicro3428
Kovacs, S. B., and Miao, E. A. (2017). Gasdermins: effectors of pyroptosis. Trends Cell. Biol. 27, 673–684. doi: 10.1016/j.tcb.2017.05.005
Li, H., Xu, H., Zhou, Y., Zhang, J., Long, C., Li, S., et al. (2007). The phosphothreonine lyase activity of a bacterial type III effector family. Science 315, 1000–1003. doi: 10.1126/science.1138960
Li, Y., Liu, M., Zuo, Z., Liu, J., Yu, X., Guan, Y., et al. (2017). TLR9 regulates the NF-kappaB-NLRP3-IL-1beta pathway negatively in Salmonella-induced NKG2D-mediated intestinal inflammation. J. Immunol. 199, 761–773. doi: 10.4049/jimmunol.1601416
Liu, Z., Wang, C., Yang, J., Zhou, B., Yang, R., Ramachandran, R., et al. (2019). Crystal structures of the full-length murine and human gasdermin D reveal mechanisms of autoinhibition, lipid binding, and oligomerization. Immunity 51, 43–49 e44. doi: 10.1016/j.immuni.2019.04.017
Man, S. M., Karki, R., and Kanneganti, T. D. (2017). Molecular mechanisms and functions of pyroptosis, inflammatory caspases and inflammasomes in infectious diseases. Immunol. Rev. 277, 61–75. doi: 10.1111/imr.12534
Mazurkiewicz, P., Thomas, J., Thompson, J. A., Liu, M., Arbibe, L., Sansonetti, P., et al. (2008). SpvC is a Salmonella effector with phosphothreonine lyase activity on host mitogen-activated protein kinases. Mol. Microbiol. 67, 1371–1383. doi: 10.1111/j.1365-2958.2008.06134.x
Orning, P., Weng, D., Starheim, K., Ratner, D., Best, Z., Lee, B., et al. (2018). Pathogen blockade of TAK1 triggers caspase-8-dependent cleavage of gasdermin D and cell death. Science 362, 1064–1069. doi: 10.1126/science.aau2818
Pandeya, A., Li, L., Li, Z., and Wei, Y. (2019). Gasdermin D (GSDMD) as a new target for the treatment of infection. Medchemcomm 10, 660–667. doi: 10.1039/C9MD00059C
Passaris, I., Cambre, A., Govers, S. K., and Aertsen, A. (2018). Bimodal expression of the Salmonella Typhimurium spv operon. Genetics 210, 621–635. doi: 10.1534/genetics.118.300822
Pradhan, D., Pradhan, J., Mishra, A., Karmakar, K., Dhiman, R., Chakravortty, D., et al. (2020). Immune modulations and survival strategies of evolved hypervirulent Salmonella Typhimurium strains. Biochim. Biophys. Acta Gen. Subj. 1864:129627. doi: 10.1016/j.bbagen.2020.129627
Rathinam, V. A. K., Zhao, Y., and Shao, F. (2019). Innate immunity to intracellular LPS. Nat. Immunol. 20, 527–533. doi: 10.1038/s41590-019-0368-3
Rauch, I., Deets, K. A., Ji, D. X., Von Moltke, J., Tenthorey, J. L., Lee, A. Y., et al. (2017). NAIP-NLRC4 inflammasomes coordinate intestinal epithelial cell expulsion with eicosanoid and IL-18 release via activation of Caspase-1 and−8. Immunity 46, 649–659. doi: 10.1016/j.immuni.2017.03.016
Shi, J., Gao, W., and Shao, F. (2017). Pyroptosis: gasdermin-mediated programmed necrotic cell death. Trends Biochem. Sci. 42, 245–254. doi: 10.1016/j.tibs.2016.10.004
Shi, J., Zhao, Y., Wang, K., Shi, X., Wang, Y., Huang, H., et al. (2015). Cleavage of GSDMD by inflammatory caspases determines pyroptotic cell death. Nature 526, 660–665. doi: 10.1038/nature15514
Song, J., Dong, H., Ma, C., Zhao, B., and Shang, G. (2010). Construction and functional characterization of an integrative form lambda Red recombineering Escherichia coli strain. FEMS Microbiol. Lett. 309, 178–183. doi: 10.1111/j.1574-6968.2010.02036.x
Swanson, K. V., Deng, M., and Ting, J. P. (2019). The NLRP3 inflammasome: molecular activation and regulation to therapeutics. Nat. Rev. Immunol. 19, 477–489. doi: 10.1038/s41577-019-0165-0
Szeliova, D., Krahulec, J., Safranek, M., Liskova, V., and Turna, J. (2016). Modulation of heterologous expression from PBAD promoter in Escherichia coli production strains. J. Biotechnol. 236, 1–9. doi: 10.1016/j.jbiotec.2016.08.004
Wang, Q., Wang, Y., Ding, J., Wang, C., Zhou, X., Gao, W., et al. (2020). A bioorthogonal system reveals antitumour immune function of pyroptosis. Nature 579, 421–426. doi: 10.1038/s41586-020-2079-1
Wu, S., Li, Y., Xu, Y., Li, Q., Chu, Y., Huang, R., et al. (2010). A Salmonella enterica serovar Typhi plasmid induces rapid and massive apoptosis in infected macrophages. Cell. Mol. Immunol. 7, 271–278. doi: 10.1038/cmi.2010.17
Yang, S., Deng, Q., Sun, L., Dong, K., Li, Y., Wu, S., et al. (2019). Salmonella effector SpvB interferes with intracellular iron homeostasis via regulation of transcription factor NRF2. FASEB J. 33, 13450–13464. doi: 10.1096/fj.201900883RR
Zhao, W., Ma, L., Cai, C., and Gong, X. (2019). Caffeine inhibits NLRP3 inflammasome activation by suppressing MAPK/NF-kappaB and A2aR signaling in LPS-induced THP-1 macrophages. Int. J. Biol. Sci. 15, 1571–1581. doi: 10.7150/ijbs.34211
Keywords: Salmonella, spvC, pyroptosis, intestinal inflammation, bacterial dissemination
Citation: Zuo L, Zhou L, Wu C, Wang Y, Li Y, Huang R and Wu S (2020) Salmonella spvC Gene Inhibits Pyroptosis and Intestinal Inflammation to Aggravate Systemic Infection in Mice. Front. Microbiol. 11:562491. doi: 10.3389/fmicb.2020.562491
Received: 15 May 2020; Accepted: 16 November 2020;
Published: 15 December 2020.
Edited by:
Mario Alberto Flores-Valdez, CONACYT Centro de Investigación y Asistencia en Tecnología y Diseño del Estado de Jalisco (CIATEJ), MexicoReviewed by:
Serena Ammendola, University of Rome Tor Vergata, ItalyNobuhiko Okada, Kitasato University School of Pharmacy, Japan
Copyright © 2020 Zuo, Zhou, Wu, Wang, Li, Huang and Wu. This is an open-access article distributed under the terms of the Creative Commons Attribution License (CC BY). The use, distribution or reproduction in other forums is permitted, provided the original author(s) and the copyright owner(s) are credited and that the original publication in this journal is cited, in accordance with accepted academic practice. No use, distribution or reproduction is permitted which does not comply with these terms.
*Correspondence: Shuyan Wu, wushuyan@suda.edu.cn; Rui Huang, hruisdm@163.com
†These authors have contributed equally to this work