- 1Division of Microbiology, Department of Pathology, Sidra Medicine, Doha, Qatar
- 2Weill Cornell Medical College in Qatar, Doha, Qatar
- 3Biomedical Research Centre, Qatar University, Doha, Qatar
- 4Division of Infectious Diseases, Faculty of Medicine, University of British Columbia, Vancouver, BC, Canada
- 5Division of Pediatric Infectious Diseases, Sidra Medicine, Doha, Qatar
Introduction: Although extended-spectrum β-lactamase (ESBL)–producing Enterobacterales are a public health problem in the Arabian Peninsula, data on the molecular characteristic of their antimicrobial resistance determinants in children is limited.
Aim: To determine the molecular characteristics of ESBL-producing Escherichia coli and Klebsiella pneumoniae in the pediatric population of Qatar.
Methods: Whole-genome sequencing was performed on ESBL-producing E. coli and K. pneumoniae isolates recovered from screening and clinical specimens from pediatric patients at Sidra Medicine in Doha from January to December 2018.
Results: A total of 327 ESBL producers were sequenced: 254 E. coli and 73 K. pneumoniae. Non-susceptibility rates to non-β-lactam antibiotics for both species were 18.1 and 30.1% for gentamicin, 0.8 and 4.1% for amikacin, 41.3 and 41.1% for ciprofloxacin, and 65.8 and 76.1% for cotrimoxazole. The most common sequence types (STs) were ST131 (16.9%), ST38 and ST10 (8.2% each) in E. coli and ST307 (9.7%), and ST45 and ST268 (6.9% each) in K. pneumoniae. CTX-M type ESBLs were found in all but one isolate, with CTX-M-15 accounting for 87.8%. Among other β-lactamases, TEM-1B and OXA-1 were coproduced in 41 and 19.6% of isolates. The most common plasmid-mediated quinolone resistance genes cocarried were qnr A/B/E/S (45.3%). Ninety percent of gentamicin non-susceptible isolates harbored genes encoding AAC(3) enzymes, mainly aac(3)-IIa. Only two of 57 isolates harboring aac(6′)-Ib-cr were non-susceptible to amikacin. Chromosomal mutations in genes encoding DNA gyrase and topoisomerase IV enzymes were detected in 96.2% fluoroquinolone-non-susceptible E. coli and 26.7% fluoroquinolone-non-susceptible K. pneumoniae.
Conclusion: Our data show that CTX-M enzymes are largely the most prevalent ESBLs in children in Qatar with a predominance of CTX-M-15. Carbapenem-sparing options to treat ESBL infections are limited, given the frequent coproduction of OXA-1 and TEM-1B enzymes and coresistance to antibiotic classes other than β-lactams.
Introduction
Over the last four decades, extended-spectrum β-lactamases (ESBLs) have presented a major challenge to treating infections caused by Enterobacterales in health care and community settings (Peirano and Pitout, 2019). Resistance to third- and fourth-generation cephalosporins mediated by ESBLs and the often associated coresistance to other antibiotic classes, such as fluoroquinolones, aminoglycosides, and sulfonamides, have led to the increased use of carbapenems worldwide, which in turn is linked to increasing resistance to these agents (Peirano and Pitout, 2019). Currently, the global epidemiology of ESBLs is dominated by CTX-M type enzymes, mainly due to the explosive dissemination of CTX-M-15 among Escherichia coli and Klebsiella pneumoniae. Nevertheless, regional variations in the prevalence, circulating enzyme types, and sequence types (STs) among ESBL producers have been reported among different patient groups in different parts of the world (Bevan et al., 2017).
The Arabian Peninsula is a region with one of the highest prevalence rates of ESBL-producing enterobacterial species (Dandachi et al., 2019). In Qatar, the percentage of ESBL-producing E. coli and K. pneumoniae isolated from urine specimens in children in the outpatient setting increased from 18% in 2010 to 31.7% in 2018; the resistance was mainly due to CTX-M group 1 enzymes (89.2%; Eltai et al., 2018a). Recently, a study using a polymerase chain reaction fingerprinting approach revealed that 22.4% of diarrheagenic E. coli produced ESBLs, mostly CTX-M group 1 (blaCTX–M–15, blaCTX–M–3; Eltai et al., 2020). However, to the best of our knowledge, there are no epidemiological data regarding the STs of ESBL-producing Enterobacterales. As well, the molecular epidemiology of ESBL producers in children has never been studied using high-resolution genomics (Perez-Lopez et al., 2020). Whole-genome sequencing (WGS) has been demonstrated to be useful in antimicrobial resistance surveillance because of its sensitivity and accuracy to detect antimicrobial resistance genes or mutations (Hendriksen et al., 2019).
Our study aimed to use the WGS approach to comprehensively characterize ESBL-producing E. coli and K. pneumoniae recovered from screening and clinical specimens from pediatric patients at Sidra Medicine in Qatar. We also sought to determine to what extent the information generated by WGS analysis can help improve the clinical management of children with ESBL infections in our institution.
Materials and Methods
Study Design, Bacterial Isolates, and Screening Protocol
The study was conducted at Sidra Medicine, a new 400-bed tertiary care women’s and children’s hospital in Doha that has been functioning at full capacity since January 2018. The hospital serves as a referral center for the pediatric population of Qatar for all medical subspecialties, including oncology, neonatal intensive care and pediatric intensive care, and surgical specialties, including cardiac surgery and neurosurgery.
All non-duplicate ESBL-producing E. coli and K. pneumoniae isolates from clinical specimens as well as rectal swabs submitted for carbapenemase-producing organism (CPO) screening from children younger than 18 years with medical or surgical conditions that required an emergency or elective hospital admission were retrospectively studied for a 1-year period, between January and December 2018. When the same ST of an organism was recovered from both screening and clinical specimens, only the clinical strain was counted.
As a part of the infection control surveillance program in our institution, a CPO questionnaire-based risk assessment is performed on emergency and elective admissions. The questionnaire consists of three questions to identify previous colonization with multidrug-resistant organisms and admission and history of invasive procedures in the last 12 months in other health care facilities within or outside of Qatar. Rectal swabs are collected from patients who answered affirmatively to any of the questions or from those who are unsure about the answer to any of the questions. In addition, patients admitted to the neonatal intensive care unit (NICU) and pediatric intensive care unit (PICU) are routinely screened for CPO carriage on admission and then once a week.
Bacterial Identification and Antimicrobial Susceptibility Testing
Screening rectal swabs were directly inoculated onto two selective chromogenic media (CHROMagarTM ESBL and CHROMagarTM mSuperCARBA, CHROMagar, France) to detect ESBL and carbapenemase producers, respectively. Colonies isolated from any of the screening media and Enterobacterales isolated from clinical specimens were identified using matrix-assisted laser-desorption ionization time-of-flight mass spectrometry (Bruker, Germany), Antimicrobial susceptibility testing for amoxicillin/clavulanate, piperacillin/tazobactam, ceftriaxone, ceftazidime, cefepime, aztreonam, ertapenem, meropenem, gentamicin, amikacin, ciprofloxacin, levofloxacin, cotrimoxazole, and nitrofurantoin was performed using the BD PhoenixTM automated identification and susceptibility testing system (Becton Dickinson, United States). Minimum inhibitory concentrations (MICs) for colistin were determined by broth microdilution (ComASPTM Colistin, Liofilchem, Italy). MIC and breakpoints were interpreted according to the Clinical and Laboratory Standards Institute (CLSI) guidelines 2018. Isolates with intermediate susceptibility and resistant to each antibiotic tested were grouped in a single non-susceptible category. The CLSI susceptible-dose-dependent category for cefepime was considered as intermediate for the purpose of this study. Non-susceptible isolates to any of the third-generation cephalosporins, cefepime, or aztreonam were considered as potential ESBL producers. Bacterial samples were stored at -80°C with 10% glycerol.
Whole-Genome Sequencing and Bioinformatic Analysis
Genomic DNA was extracted using the automated platform NucliSENS easyMag (bioMérieux, Marcy-l’Etoile, France) and quantified by Qubit (Thermo Fisher Scientific, Waltham, MA). WGS was performed on all organisms with a susceptibility pattern consistent with ESBL production on the Illumina Miseq platform. Paired-end (PE) DNA libraries were constructed using Nextera XT (Illumina, San Diego, CA). The DNA was tagmented, amplified by index primers, and purified with AMPure XP beads (Beckman Coulter, Brea, CA) to remove the smaller-size fragments. DNA libraries were then normalized, pooled, and sequenced using the in-house MiSeq platform generation 300-bp PE reads (MiSeq Reagent Kit V3) at the Department of Pathology. Raw reads were assessed by Fastqc1 and quality trimmed by Trim Galore2 to eliminate adaptors and low-quality sequences. Trimmed reads were assembled de novo using SPAdes v.3.9.0 (Bankevich et al., 2012), and smaller contigs (<500 bp) were excluded. The assessment of all genome assemblies was performed using QUAST v5.0.2 (Gurevich et al., 2013), contaminant reads were excluded after analysis by Kraken v2 (Wood and Salzberg, 2014). STs and the genes encoding antimicrobial resistance for β-lactams, fluoroquinolones, aminoglycosides, and trimethoprim-sulfamethoxazole (cotrimoxazole) were predicted based on the PubMLST typing schemes implemented in mlst3 and the ResFinder v3.2 database (Zankari et al., 2012) implemented in abricate v0.9.8.4 Mutations in the quinolone resistance–determining regions (QRDR) affecting chromosomal genes encoding DNA gyrase and topoisomerase IV enzymes were characterized using AMRFinderplus v3.8 (Feldgarden et al., 2019). The plasmids were determined and defined based on the PlasmidFinder v2.1 database (Carattoli et al., 2014). The phylogroup and serotypes of E. coli were predicted using ClermonTyping (Beghain et al., 2018) and ECTyper.5 FimH typing was performed using6 (Roer et al., 2017). The genetic relatedness of the major STs in E. coli and K. pneumoniae was inferred based on core genome SNPs using Parsnp v1 (Treangen et al., 2014).
Statistical Analysis
Associations were assessed by the χ2 test with Yates correction for continuity or Fisher exact test when an expected value was < 5. In addition, the analysis of some variables was broken down into three different nursing units based on the CPO screening policy: PICU and NICU, where active surveillance of ESBL carriage was performed on a weekly basis. The remaining units in which ESBL colonization were studied, depending on the result of the risk assessment survey, were grouped into general pediatrics (GPED).
Results
A total of 327 ESBL producers were sequenced, 254 E. coli and 73 K. pneumoniae. Of them, 202 E. coli (79.5%) and 56 K. pneumoniae (76.7%) were recovered from screening specimens. Sixty-nine strains were isolated from clinical specimens [E. coli 52 isolates (25.5%) and K. pneumoniae 17 isolates (31.5%)], including urine [36 isolates (52.2%)], peritoneal fluid [8 isolates (11.6%)], respiratory tract [7 isolates (10.1%)], bloodstream [6 isolates (8.7%)], pus and wound [5 isolates (7.2% each)], and cerebrospinal fluid [2 isolates (2.9%)]. The proportion of clinical isolates in GPED, PICU, and NICU were 27.6% (55 isolates), 8.9% (8 isolates), and 15.8% (6 isolates), respectively (Supplementary Materials).
Overall, non-susceptibility rates among ESBL-producing E. coli and K. pneumoniae isolates were 46.1 and 72.6% for amoxicillin/clavulanate, 12.6 and 30.1% for piperacillin/tazobactam, 99.2 and 98.6% for ceftriaxone, 59.8 and 87.7% for ceftazidime, 68.5 and 74% for cefepime, 84.2 and 93.5% for aztreonam, 5.1 and 9.6% for ertapenem, 3.1 and 8.2% for meropenem, 18.1 and 30.1% for gentamicin, 0.8 and 4.1% for amikacin, 41.3 and 41.1% for ciprofloxacin, 42.4 and 17.9% for levofloxacin, 65.8 and 76.1% for cotrimoxazole, and 7.9 and 58.9% for nitrofurantoin. In addition, two E. coli strains isolated from rectal screening swabs were resistant to colistin (MIC between 4 and 8 mg/L). Except for the higher non-susceptibility rates to cefepime among clinical isolates of both species and higher non-susceptibility to nitrofurantoin among screening E. coli isolates, there were no significant antimicrobial susceptibility differences between clinical and screening isolates (Table 1).
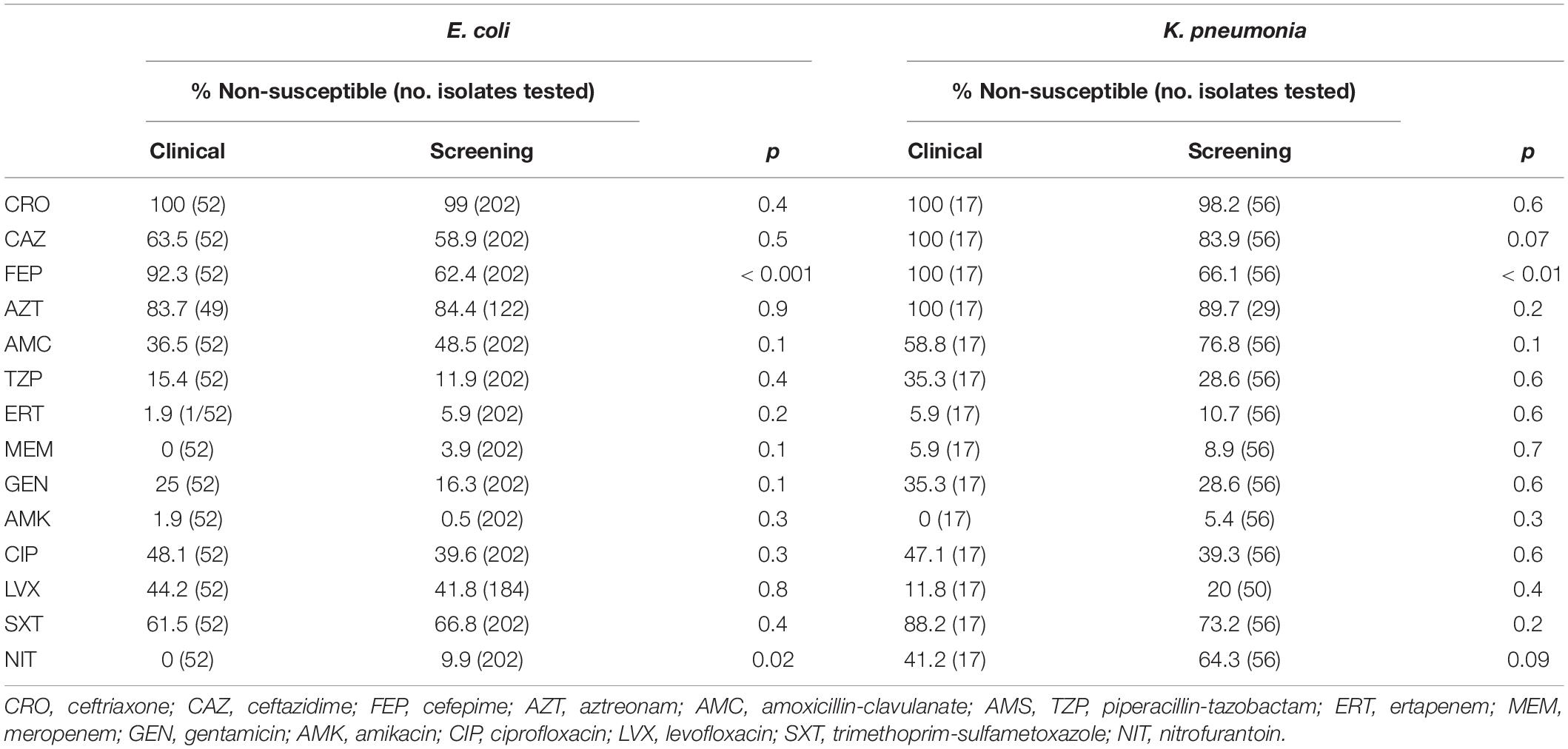
Table 1. Comparison of non-susceptibility rates between clinical and screening ESBL-producing E. coli and K. pneumoniae.
Ninety-seven different STs were detected in E. coli, whereas 40 STs were found in K. pneumoniae. The five most prevalent STs in E. coli were ST131 [43 isolates (16.9%)], followed by ST38 and ST10 [21 isolates (8.2% each)], ST1193 [10 isolates (3.9%)], and ST73 [8 isolates (3.1%)]. All ST131 isolates belonged to phylogroup B2. O25:H4 accounted for 54.8% of the 131 isolates. Twenty-two (51.2%) of the E. coli ST131 belonged to the subclone H30, and 13 (30.2%) of them to the clade H30Rx. Among E. coli ST131, non-susceptibility to fluoroquinolones was significantly higher in H30 subclone (90.9%; 20/22) compared with other subclones (47.6%; 10/21; P < 0.01). Among the other most prevalent E. coli STs, non-susceptibility to fluoroquinolones was particularly remarkable in ST1193 isolates (100%; Supplementary Materials).
The three most common STs among K. pneumoniae isolates were ST307 [7 isolates (9.7%)] followed by ST45 and ST268 [5 isolates (6.9% each)]. Non-susceptibility to fluoroquinolones was significantly higher in ST307 [85.7% (6/7)] than in other STs [36.4% (24/66)] (P = 0.02; Supplementary Materials). Phylogenetic analysis presented in Supplementary Figures S1, S2 indicates genetic variations among and within major STs in both species. For instance, two genetic groups were recognized in E. coli ST131 (Supplementary Figure S1B).
Eighty percent of NICU isolates, including all K. pneumoniae isolates, were detected during hospital stay. Carriage acquisition rate and infection acquisition rate in NICU during hospitalization for the study period were 3.28/1,000 and 0.82/1,000 patient-days. Twenty-nine percent of PICU isolates were detected during hospitalization. Carriage acquisition rate and infection acquisition rate in PICU were 5.82/1,000 and 0.76/1,000 patient-days. Cross-transmission of bacteria with the same ST between patients was not observed in critical care units. Overall, only three patients (4.3%) initially detected by screening swabs developed subsequent clinical infections caused by the same ST during the study period.
WGS revealed that all 327 isolates except one carried CTX-M genes. The predominant gene detected was blaCTX–M–15 in 287 isolates (87.8%), followed by blaCTX–M–14 and blaSHV-106 in nine isolates each (2.8%). ESBL-producing E. coli and ESBL-producing K. pneumoniae were isolated simultaneously from 17 samples with blaCTX–M–15 detected in both species in 16 of those samples.
Sixty-seven percent of isolates coproduced one or more β-lactamases. The most common genes were blaTEM–1B and blaOXA–1 in 134 (41%) and 64 (19.6%) isolates, respectively. CTX-M-15 was present in all isolates carrying blaOXA–1. In addition, 12 E. coli isolates and 6 K. pneumoniae isolates coproduced carbapenemases, Only two types of carbapenemases were detected: OXA-48 type (6 isolates) and NDM type (12 isolates; Table 1 and Supplementary Materials). Supplementary Table 1 shows the association between co-carriage of TEM-1B and OXA-1 and non-susceptibility to amoxicillin/clavulanate and piperacillin/tazobactam.
At least one plasmid-mediated quinolone resistance (PMQR) gene was detected in 199 isolates (60.9%). PMQR genes found were qnr A/B/E/S in 148 isolates (45.3%), aac(6′)-Ib-cr in 57 isolates (17.4%), and qepA in 2 isolates (0.6%). oqxAB was detected in one E. coli isolate only. Fifteen different aminoglycoside-modifying enzyme genes were detected. Ninety percent of isolates non-susceptible to gentamicin carried genes encoding AAC(3) enzymes (61/68 isolates). Conversely, only 2 (3.5%) of the 57 isolates harboring aac(6′)-Ib-cr were non-susceptible to amikacin. All four isolates with high-level resistance to amikacin carried 16S rRNA methylase genes (two rmtB and two armA). Genes encoding fosfomycin-modifying enzymes (fos genes) were detected in 73 isolates (22%). These genes were carried by 89% of K. pneumoniae and 3% of E. coli strains. The simultaneous carriage of blaOXA–1, aac(6′)-Ib-cr, and aac(3)-II was strongly associated (P < 0.0001). Eighty-nine percent of the isolates harboring the dfrA17 gene also carried the aadA5 gene, whereas all isolates harboring dfrA12 carried aadA2 genes (Table 2 and Supplementary Materials). The plasmid-mediated colistin resistance gene mcr-1 was detected in the two colistin-resistant E. coli isolates (Supplementary Materials).
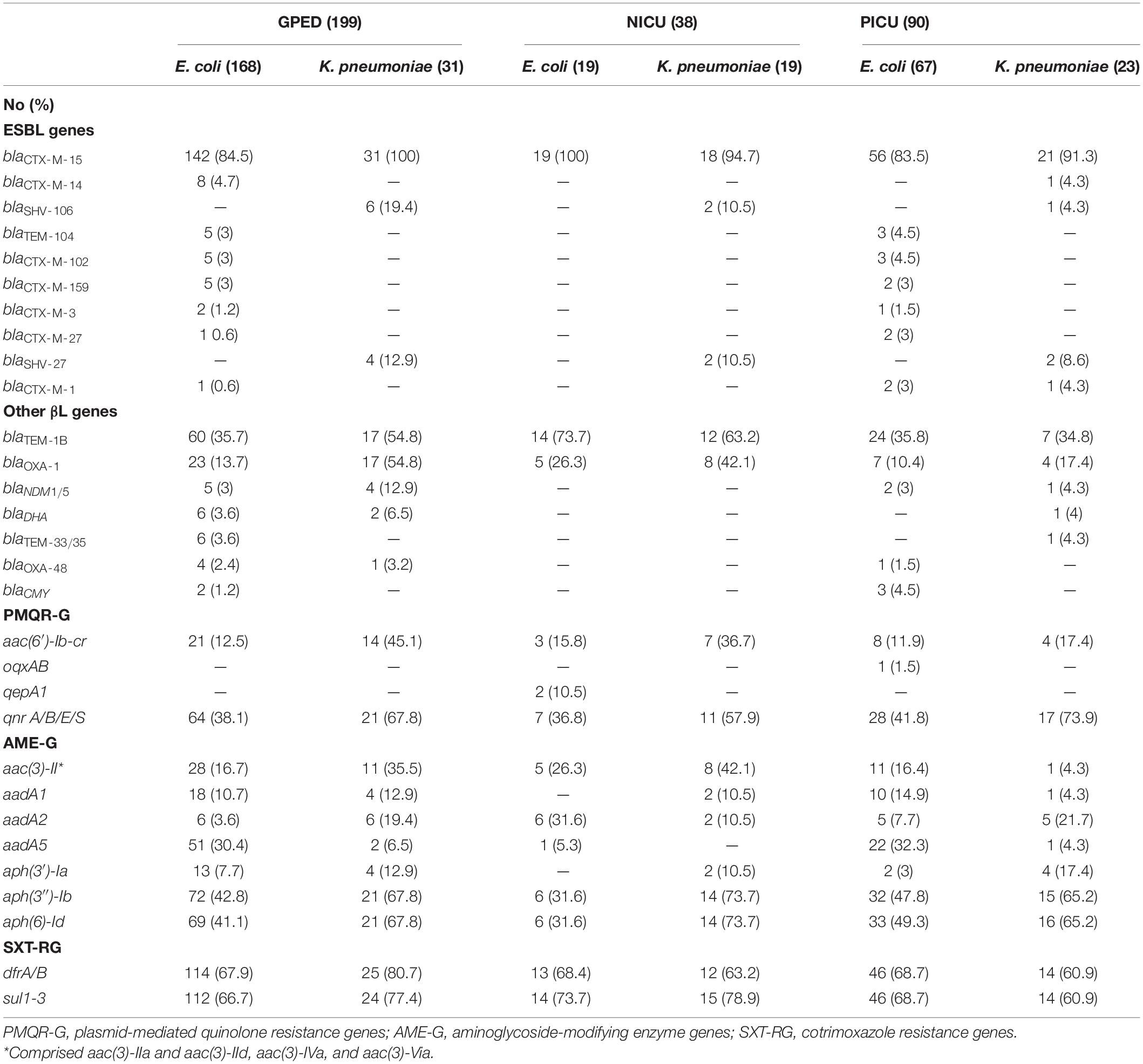
Table 2. Distribution of β-lactamase, quinolone, aminoglycoside, and cotrimoxazole resistance genes among ESBL-producing E. coli and K. pneumoniae.
QRDR mutations (gyrA, parC, and parE) were detected QRDR mutations (gyrA, parC, and parE) were detected in 96.2% (101/105) of fluoroquinolone-non-susceptible E. coli isolates and 26.7% (8/30) of fluoroquinolone-non-susceptible K. pneumoniae isolates. Conversely, QRDR mutations were present in 30.2% (45/149) of fluoroquinolone-susceptible E. coli isolates and 7% (3/43) of fluoroquinolone-susceptible K. pneumoniae isolates. Overall, 69.8% (30/43) E. coli ST131 isolates carried ≥ 4 QRDR mutations compared with 27.5% (58/211) non-ST131 isolates (P < 0.001). Five identical QRDR mutations in gyrA (S83L and D87N), parC (S80I and E84V), and parE (I529L) were detected in 90.9% (20/22) of ST131-H30 isolates. Consistently, all E. coli ST1193 isolates had the same mutations in gyrA (S83L and D87N) and parC (S80I). All of the seven K. pneumoniae ST307 isolates carried two mutations in gyrA (S83I) and parC (80I) genes. In contrast, only two isolates (3%) belonging to other STs (ST967, ST2096) had two QRDR mutations (P < 0.001) (Supplementary Materials).
Narrow host-range IncF plasmids were present in 278 isolates (85%). FIB was the most common F replicon found in 243 isolates (74.3%) followed by FII in 163 isolates (49.7%) and FIA 99 isolates (30.3%). In addition, 10 more Inc replicon types were detected, mostly as a part of multiple combinations with F replicons. The three most prevalent were as follows: IncI1 [64 isolates (19.6%)], IncX [27 isolates (8.3%)], and IncB/O/K/Z [25 isolates (7.6%)]. On the other hand, 189 isolates (57.8%) contained col-type plasmids. RNAI [119 isolates (36.4%)], col156 [88 isolates (26.9%)], and MG828 [46 isolates (14%)] were the most common representative plasmids within this group. Plasmid location of blaCTX–M genes could be determined in 22 isolates (Table 3). Twenty-one isolates (6.4%) did not have any plasmid detected. Both mcr-1 genes found in our collection were carried by IncI2 plasmids (Supplementary Materials).
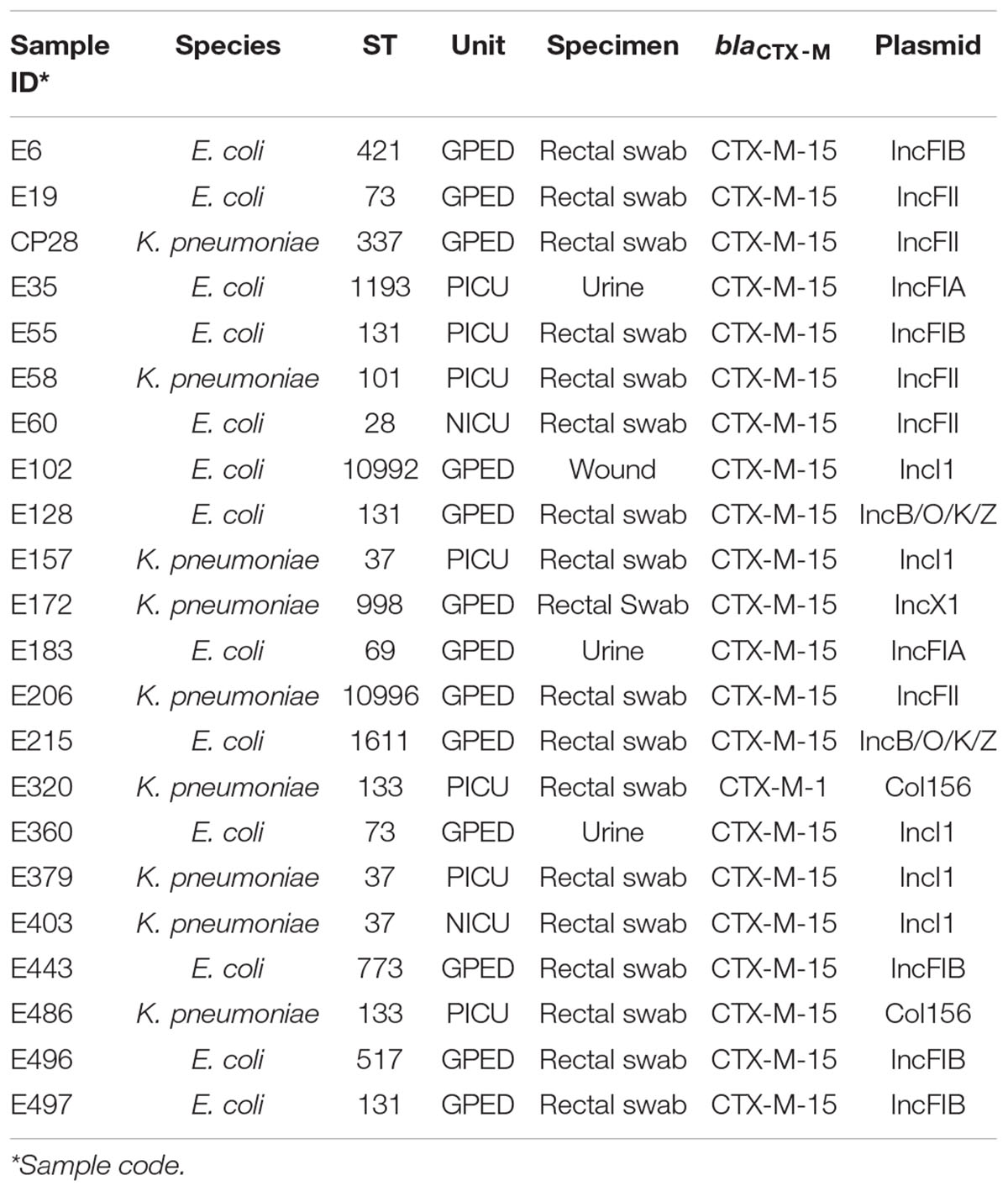
Table 3. Plasmid location of blaCTX–M genes in 22 ESBL-producing E. coli and K. pneumoniae isolates.
Discussion
Our data show that CTX-M-type ESBLs are largely disseminated in the pediatric population in Qatar with CTX-M-15, the most widespread ESBL type worldwide (Bevan et al., 2017; Peirano and Pitout, 2019), as the main driving force. Our study also points out that narrow host range plasmids, mainly those belonging to the IncF family, are likely the main vehicle for the dissemination of blaCTX–M–15 along with other resistance genes such as blaOXA–1, aac(6′)-Ib-cr, and aac(3)-II among a great diversity of genotypes within E. coli and K. pneumoniae (Carattoli, 2009). It is reasonable to hypothesize that our epidemiological landscape has been shaped by a high rate of community carriage among a fast-growing expatriate population, which currently comprises around 87% of Qatar’s population. It is also important to note that the largest segment of the expatriate population in Qatar is constituted by a young labor force from the Indian subcontinent (De Bel-Air, 2019), where rates of CTX-M-15 among fecal isolates from healthy individuals and clinical isolates have been reported to be greater than 90% (Ensor et al., 2006; Lina et al., 2014; Chakraborty et al., 2015; Sherchan et al., 2015; Islam et al., 2019). In contrast, our NICU had a variety of genotypes of both species without subsequent significant cross-transmission, suggesting a sporadic colonization/infection within a group that is at particular high risk of ESBL acquisition (Li et al., 2017).
Sequence analysis revealed that the management of ESBL infections in our pediatric population is challenging, particularly concerning carbapenem-sparing options. The effect of clavulanic acid and tazobactam in inhibiting CTX-M enzymes is often counteracted in our isolates by the coproduction of TEM-1B and OXA-1 (Livermore et al., 2019) and to a lesser extent by other enzymes such as plasmid-mediated AmpC β-lactamases and carbapenemases. For example, amoxicillin/clavulanate does not seem to be a reliable agent for the treatment of uncomplicated ESBL lower urinary tract infections (UTIs). Fortunately, nitrofurantoin remains a good option in this scenario, especially in cases caused by E. coli, as all urinary isolates of E. coli were susceptible to this agent. On the other hand, although TEM-1B seems to have a poor inhibitory effect against tazobactam, the common coproduction of OXA-1 alone or accompanied by TEM-1B makes piperacillin/tazobactam an unreliable agent for the empirical or definitive treatment of systemic ESBL infections, irrespective of the unresolved controversy concerning the usefulness of this agent in high-inoculum infections (Retamar et al., 2013; Harris et al., 2018). Although ertapenem seems to be an appealing option, resistance to carbapenems due to coproduction of carbapenemases was not negligible. It was not surprising that OXA-48 type and NDM type, mainly associated with the coproduction of CTX-M-15, were the only carbapenemases identified in our strains, given that the molecular epidemiology of carbapenemases in E. coli and K. pneumoniae across countries of the Gulf Cooperation Council is largely dominated by these enzymes (Zowawi et al., 2014; Sonnevend et al., 2015; Dandachi et al., 2019; Touati and Mairi, 2020). Interestingly, the international high-risk clone of K. pneumoniae ST258 responsible for the dissemination of genes encoding KPC-type carbapenemases elsewhere was not detected in our collection concurring with earlier studies in this part of the Arabian Peninsula in which it was seldom found (Dandachi et al., 2019; Touati and Mairi, 2020). It should be also noted that 3 of 20 non-susceptible isolates to ertapenem lacked genes encoding carbapenemases or AmpC β-lactamases, suggesting that resistance to ertapenem might have been caused by impaired penetration through the outer membrane due to porin loss (Nicolas-Chanoine et al., 2018). Particularly noteworthy was high proportion of isolates susceptible to amikacin despite the presence aac(6′)-Ib-cr genes in 17% of strains. Although monotherapy with amikacin has been proposed to treat febrile UTI in children in regions with low resistance rates among ESBL producers (Madhi et al., 2018), it should be kept in mind that it is not uncommon to find susceptible isolates according to the CLSI breakpoints (MIC ≤ 16 mg/L) harboring aac(6′)-Ib-cr with MICs between 4 and 8 mg/L (Fernández-Martínez et al., 2015; Livermore et al., 2019). The treatment with amikacin of ESBL infections caused by isolates with MIC > 4 mg/L may become ineffective by compromising the Cmax/MIC ratio, the main pharmacodynamic parameter that predicts bacterial killing in aminoglycosides (Alqahtani et al., 2018). Likewise, resistance to cotrimoxazole among our isolates was significantly high, rendering this combination ineffective at least as empirical therapy. Interestingly, the strong association between dfrA17 and aadA5 genes and between dfrA12 and aadA2 genes suggests that class 1 integrons were not uncommon in our isolates. Class 1 integrons containing these gene cassette combinations are often located in IncF plasmids along with resistance genes such as blaCTX–M–15, blaOXA–1, and aac(6′)-Ib-cr (Oliveira-Pinto et al., 2017). Finally, the detection of mcr-1 genes in two E. coli isolates resistant to colistin, the last resort for the treatment of Enterobacterales resistant to broad-spectrum cephalosporins and carbapenems, was worrying but not surprising as this gene has been sporadically reported in clinical specimens in the Arabian Peninsula carried by IncI2 and IncHI2 plasmids (Dandachi et al., 2019). It is worth noting that these isolates belonged to the STs ST115 and ST540, which have mainly been detected from the poultry and environment (Tsui et al., 2020). As fecal carriage of ESBL-producing E. coli has also been described from food handlers in Qatar (Eltai et al., 2018b), our findings suggest that a silent transmission from animals to humans via the food chain may be also playing a role in the spread of ESBL and other resistance determinants among our population.
Because fluoroquinolones are rarely prescribed in our institution, the high rate of non-susceptibility to these agents among a quinolone-naive population deserves a special mention. On the one hand, the high proportion of PMQR determinants in our isolates suggests that transmission of these genes among commensal intestinal flora through the epidemic plasmids reported here is facilitating the selection of isolates with multiple mutations in the QRDR resulting in high-level resistance to fluoroquinolones among community isolates (Yuan et al., 2012; Yassine et al., 2019). On the other hand, fluoroquinolone resistance has been a major driver for the selection and spread of successful clones that frequently harbor blaCTX–M–15 such as E. coli ST131-H30Rx, E. coli ST1193, and K. pneumoniae ST307 (Tóth et al., 2014; Johnson et al., 2015; Wyres et al., 2019; Fuzi et al., 2020; Peirano et al., 2020).
Our study has several limitations. Because MICs were determined by the Phoenix automated system, which does not always provide an exact value within the susceptibility range, low-level resistance conferred by certain genes could not be assessed when MICs did not exceed susceptible breakpoints, for instance, low-level amikacin resistance in isolates carrying aac(6′)-Ib-cr or low-level piperacillin/tazobactam resistance in the presence of OXA-1 enzyme. Moreover, the activity of other potential useful agents available in Qatar, particularly ceftazidime–avibactam, ceftolozane–tazobactam, and fosfomycin, could not be assessed because susceptibility cards used in this study did not contain any of these antibiotics. Despite this limitation, it could be speculated that ceftazidime–avibactam would have been effective against our isolates in the absence of coproduction of NDM-type carbapenemases, and fosfomycin would have been a suitable option for uncomplicated ESBL lower UTI caused by E. coli in the absence of other resistance mechanisms, as all isolates recovered from clinical specimens lacked fos genes (Cattoir and Guérin, 2018; Peirano and Pitout, 2019). It should be also taken into account that the population studied mostly comprised children at risk of acquisition of multidrug-resistant bacteria, which may have biased the actual burden of some resistance determinants in our entire pediatric population. Finally, plasmid replicons were analyzed using genome assemblies derived from short-read sequence data, which hindered reliable plasmid sequence reconstruction and elucidation of plasmid location of CTX-M genes in the majority of isolates. In fact, plasmid sequences were not detected by PlasmidFinder database in 22 isolates. Although this could indicate the challenge on the inference of plasmids from fragmented, small-sized contigs or the presence of non-typable plasmids, it could also suggest a potential chromosomal integration of blaCTX–M-type genes, a phenomenon increasingly reported in clinical isolates (Rodríguez et al., 2014; van Aartsen et al., 2019).
In summary, our study showed that CTX-M enzymes are overwhelmingly prevalent in the pediatric population of Qatar with CTX-M-15 as the major driving force. High community carriage rates among a multinational population appear to be the main reservoir for blaCTX–M–15 and other resistance genes, which are likely spread by epidemic plasmids. Our study also highlighted the promising value of NGS-based technologies to improve antibiotic prescribing practices by uncovering resistance mechanisms not detected by conventional phenotypic antimicrobial susceptibility testing. To the best of our knowledge, this is the first study characterizing ESBL in children using WGS in the Arabian Peninsula. Because demographic profiles in other countries of the Gulf Cooperation Council are similar to Qatar, we believe that our results could be extrapolated at least to the middle and eastern part of the region.
Data Availability Statement
The original contributions presented in the study are publicly available. This data can be found in NCBI under accession numbers PRJNA599369 and PRJNA599387.
Ethics Statement
This study was granted exempt status by the Institutional Review Board of Sidra Medicine (Protocol Number: 1804022140) as it only involved pathology specimens. Written informed consent for participation was not required for this study in accordance with the national legislation and the institutional requirements.
Author Contributions
AP-L designed the study, analyzed the data, and drafted the manuscript. SS performed WGS, supported HA-M and MS in collecting isolates and performing antimicrobial susceptibility testing and revised the manuscript. HA-M supported MS in collecting isolates and performing antimicrobial susceptibility testing on selected isolates, supported SS in performing WGS, carried out the statistical analysis, and revised the manuscript. KT performed the bioinformatic analysis and revised the manuscript. MH supervised WGS process and bioinformatic analysis and revised the manuscript. MS performed identification and antimicrobial susceptibility testing on all isolates. EA and MJ collaborated with AP-L in the clinical interpretation of the WGS analysis. PT coordinated and supervised the execution of the study and substantially revised the manuscript. All authors read and approved the final version of the manuscript.
Funding
This study was funded by a Sidra Internal Research Funding grant (Project ID: SIRF_200040).
Conflict of Interest
The authors declare that the research was conducted in the absence of any commercial or financial relationships that could be construed as a potential conflict of interest.
Acknowledgments
This manuscript has been released as a pre-print at Research Square. Available at: https://www.researchsquare.com/article/rs-29509/v1. The authors would like to thank Will Hsiao and Jun Duan (BCCDC Public Health Laboratory, Vancouver, Canada) for technical assistance.
Supplementary Material
The Supplementary Material for this article can be found online at: https://www.frontiersin.org/articles/10.3389/fmicb.2020.581711/full#supplementary-material
Footnotes
- ^ http://www.bioinformatics.babraham.ac.uk/projects/fastqc/
- ^ http://www.bioinformatics.babraham.ac.uk/projects/trim_galore/
- ^ https://github.com/tseemann/mlst
- ^ https://github.com/tseemann/abricate
- ^ https://github.com/phac-nml/ecoli_serotyping
- ^ https://cge.cbs.dtu.dk/services/FimTyper/
References
Alqahtani, S., Abouelkheir, M., Alsultani, A., Elsharawy, Y., Alkoraishi, A., Reem, O., et al. (2018). Optimizing amikacin dosage in pediatrics based on population pharmacokinetic/pharmacodynamic modeling. Pediatr. Drugs 20, 265–272. doi: 10.1007/s40272-018-0288-y
Bankevich, A., Nurk, S., Antipov, D., Gurevich, A. A., Dvorkin, M., Kulikov, A. S., et al. (2012). SPAdes: a new genome assembly algorithm and its applications to single-cell sequencing. J. Comput. Biol. 19, 455–477. doi: 10.1089/cmb.2012.0021
Beghain, J., Bridier-Nahmias, A., Le Nagard, H., Denamur, E., and Clermont, O. (2018). ClermonTyping: an easy-to-use and accurate in silico method for Escherichia genus strain phylotyping. Microb. Genom. 4:e000192.
Bevan, E. R., Jones, A. M., and Hawkey, P. M. (2017). Global epidemiology of CTX-M β-lactamases: temporal and geographical shifts in genotype. J. Antimicrob. Chemother. 72, 2145–2155. doi: 10.1093/jac/dkx146
Carattoli, A. (2009). Resistance plasmid families in Enterobacteriaceae. Antimicrob. Agents Chemother. 53, 2227–2238. doi: 10.1128/aac.01707-08
Carattoli, A., Zankari, E., García-Fernández, A., Voldby-Larsen, M., Lund, O., Villa, L., et al. (2014). In silico detection and typing of plasmids using PlasmidFinder and plasmid multilocus sequence typing. Antimicrob. Agents Chemother. 58, 3895–3903. doi: 10.1128/aac.02412-14
Cattoir, V., and Guérin, F. (2018). How is fosfomycin resistance developed in Escherichia coli? Future Microbiol. 13, 1693–1696. doi: 10.2217/fmb-2018-0294
Chakraborty, A., Adhikari, P., Shenoy, S., and Saralaya, V. (2015). Clinical significance and phylogenetic background of extended spectrum β-lactamase producing Escherichia coli isolates from extra-intestinal infections. J. Infect. Public Health 8, 248–253. doi: 10.1016/j.jiph.2014.10.001
Dandachi, I., Chaddad, A., Hanna, J., Matta, J., and Daoud, Z. (2019). Understanding the epidemiology of multi-drug resistant gram-negative bacilli in the Middle East using a one health approach. Front. Microbiol. 10:1941. doi: 10.3389/fmicb.2019.01941
De Bel-Air, F. D. (2019). Demography, Migration, and Labour Market in Qatar. No. 3/2017. Available online at: http://gulfmigration.org/media/pubs/exno/GLMM_EN_2017_03.pdf (accessed July 09, 2020).
Eltai, N. O., Al Thani, A. A., Al-Ansari, K., Deshmukh, A. S., Wehedy, E., Al-Hadidi, S. H., et al. (2018a). Molecular characterization of extended spectrum beta-lactamases Enterobacteriaceae causing lower urinary tract infection among pediatric population. Antimicrob. Resist. Infect. Control 7:90.
Eltai, N. O., Yassine, H. M., Al Thani, A. A., Abu Madi, M. A., Ismail, A., Ibrahim, E., et al. (2018b). Prevalence of antibiotic resistant Escherichia coli isolates from fecal samples of food handlers in Qatar. Antimicrob. Resist. Infect. Control 7:78.
Eltai, N. O., Al-Thani, A. A., Al-Hadidi, S. H., Al-Ansari, K., and Yassine, H. M. (2020). Antibiotic resistance and virulence patterns of pathogenic Escherichia coli strains associated with acute gastroenteritis among children in Qatar. BMC Microbiol. 20:54. doi: 10.1186/s12866-020-01732-8
Ensor, V. M., Shahid, L., Evans, T., and Hawkey, P. M. (2006). Occurrence, prevalence and genetic environment of CTX-M β-lactamases in Enterobacteriaceae from Indian hospitals. J. Antimicrob. Chemother. 58, 1260–1263. doi: 10.1093/jac/dkl422
Feldgarden, M., Brover, V., Haft, D. H., Prasad, A. B., Slotta, D. J., Tolstoy, I., et al. (2019). Validating the NCBI AMRFinder tool and resistance gene database using antimicrobial resistance genotype-phenotype correlations in a collection of NARMS isolates. Antimicrob. Agents Chemother. 63:e0483-19.
Fernández-Martínez, M., Miró, E., Ortega, A., Bou, G., González-López, J. J., Oliver, A., et al. (2015). Molecular identification of aminoglycoside-modifying enzymes in clinical isolates of Escherichia coli resistant to amoxicillin/clavulanic acid isolated in Spain. Int. J. Antimicrob. Agents 46, 157–163. doi: 10.1016/j.ijantimicag.2015.03.008
Fuzi, M., Rodríguez-Baño, J., and Tóth, Á (2020). Global evolution of pathogenic bacteria with extensive use of fluoroquinolone agents. Front. Microbiol. 11:271. doi: 10.3389/fmicb.2019.00271
Gurevich, A., Saveliev, V., Vyahhi, N., and Tesler, G. (2013). QUAST: quality assessment tool for genome assemblies. Bioinformatics 29, 1072–1075. doi: 10.1093/bioinformatics/btt086
Harris, P. N. A., Tambyah, P. A., Lye, D. C., Mo, Y., Lee, T. H., Yilmaz, M., et al. (2018). Effect of piperacillin-tazobactam vs meropenem on 30-day mortality for patients with E. coli or Klebsiella pneumoniae bloodstream infection and ceftriaxone resistance: a randomized clinical trial. JAMA 320, 984–994. doi: 10.1001/jama.2018.12163
Hendriksen, R. S., Bortolaia, V., Tate, H., Tyson, G. H., Aarestrup, F. M., and McDermott, P. F. (2019). Using genomics to track global antimicrobial resistance. Front. Public Health 7:242. doi: 10.3389/fmicb.2019.00242
Islam, M. A., Amin, M. B., Roy, S., Asaduzzaman, M., Islam, M. R., Navab-Daneshmand, T., et al. (2019). Fecal colonization with multidrug-resistant E. coli among healthy infants in rural Bangladesh. Front. Microbiol. 10:640. doi: 10.3389/fmicb.2019.00640
Johnson, J. R., Johnston, B., Kuskowski, M. A., Sokurenko, E. V., and Tchesnokovac, V. (2015). Intensity and mechanisms of fluoroquinolone resistance within the H30 and H30Rx subclones of Escherichia coli sequence type 131 compared with other fluoroquinolone-resistant E. coli. Antimicrob. Agents Chemother. 59, 4471–4480. doi: 10.1128/aac.00673-15
Li, X., Xu, X., Yang, X., Luo, M., Liu, P., Su, K., et al. (2017). Risk Factors for infection and/orcolonisation with extended-spectrum β-lactamase-producing bacteria in the neonatal intensive care unit: a meta-analysis. Int. J. Antimicrob. Agents 50, 622–628. doi: 10.1016/j.ijantimicag.2017.06.027
Lina, T. T., Khajanchi, B. K., Azmi, I. J., Islam, M. A., Mahmood, B., Akter, M., et al. (2014). Phenotypic and molecular characterization of extended-spectrum beta-lactamase-producing Escherichia coli in Bangladesh. PLoS One 9:e108735. doi: 10.1371/journal.pone.0108735
Livermore, D. M., Day, M., Cleary, P., Hopkins, K. L., Toleman, M. A., Wareham, D. W., et al. (2019). OXA-1 β-lactamase and non-susceptibility to penicillin/β-lactamase inhibitor combinations among ESBL-producing Escherichia coli. J. Antimicrob. Chemother. 74, 326–333. doi: 10.1093/jac/dky453
Madhi, F., Jung, C., Timsit, S., Levy, C., Biscardi, S., Lorrot, M., et al. (2018). Febrile urinary-tract infection due to extended-spectrum beta-lactamase-producing Enterobacteriaceae in children: a French prospective multicenter study. PLoS One 13:e0190910. doi: 10.1371/journal.pone.0190910
Nicolas-Chanoine, M. H., Mayer, N., Guyot, K., Dumont, E., and Pagès, J. M. (2018). Interplay between membrane permeability and enzymatic barrier leads to antibiotic-dependent resistance in Klebsiella pneumoniae. Front. Microbiol. 29:1422. doi: 10.3389/fmicb.2019.01422
Oliveira-Pinto, C., Diamantino, C., Oliveira, P. L., Reis, M. P., Costa, P. S., Paiva, M. C., et al. (2017). Occurrence and characterization of class 1 integrons in Escherichia coli from healthy individuals and those with urinary infection. J. Med. Microbiol. 66, 577–583. doi: 10.1099/jmm.0.000468
Peirano, G., Chen, L., Kreiswirth, B. N., and Pitout, J. D. D. (2020). Emerging antimicrobial resistant high- risk clones among Klebsiella pneumoniae: ST307 and ST147. Antimicrob. Agent Chemother. doi: 10.1128/AAC.01148-20 [Epub ahead of print].
Peirano, G., and Pitout, J. D. D. (2019). Extended-spectrum β-lactamase-producing Enterobacteriaceae: update on molecular and epidemiology and treatment options. Drugs 79, 1529–1541. doi: 10.1007/s40265-019-01180-3
Perez-Lopez, A., Sundararaju, S., Al-Mana, H., Tsui, K. M., Hasan, M., and Tang, P. (2020). Molecular characterization of extended-spectrum β-lactamase-producing Escherichia coli and Klebsiella pneumoniae among the pediatric population in Qatar. Research Square [Preprint], Available online at: https://www.researchsquare.com/article/rs-29509/v1 (accessed July 12, 2020).
Retamar, P., López-Cerero, L., Muniain, M. A., Pascual, A., and Rodríguez-Baño, J. ESBL- REIPI/GEIHGroup (2013). Impact of the MIC of piperacillin-tazobactam on the outcome of patients with bacteremia due to extended-spectrum-β-lactamase-producing Escherichia coli. Antimicrob. Agents Chemother. 57, 3402–3404. doi: 10.1128/aac.00135-13
Rodríguez, I., Thomas, K., Van Essen, A., Schink, A. K., Day, M., Chattaway, M., et al. (2014). Chromosomal location of blaCTX-M genes in clinical isolates of Escherichia coli from Germany, The Netherlands and the UK. Int. J. Antimicrob. Agents 43, 553–557. doi: 10.1016/j.ijantimicag.2014.02.019
Roer, L., Tchesnokova, V., Allesoe, R., Muradova, M., Chattopadhyay, S., Ahrenfeldt, J., et al. (2017). Development of a web tool for Escherichia coli subtyping based on fimh alleles. J. Clin. Microbiol. 55, 2538–2543. doi: 10.1128/jcm.00737-17
Sherchan, J. B., Hayakawa, K., Miyoshi-Akiyama, T., Ohmagari, N., Kirikae, T., Nagamatsu, M., et al. (2015). Clinical epidemiology and molecular analysis of extended-spectrum-β-lactamase producing Escherichia coli in Nepal: characteristics of sequence types 131 and 648. Antimicrob. Agents Chemother. 59, 3424–3432. doi: 10.1128/aac.00270-15
Sonnevend, Á, Ghazawi, A. A., Hashmey, R., Jamal, W., Rotimi, V. O., Shibl, A. M., et al. (2015). Characterization of carbapenem-resistant Enterobacteriaceae with high rate of autochthonous transmission in the Arabian Peninsula. PLoS One 25:e0131372. doi: 10.1371/journal.pone.0131372
Tóth, Á, Kocsis, B., Damjanova, I., Kristóf, K., Jánvári, L., Pászti, J., et al. (2014). Fitness cost associated with resistance to fluoroquinolones is diverse across clones of Klebsiella pneumoniae and may select for CTX-M-15 type extended-spectrum β-lactamase. Eur. J. Clin. Microbiol. Infect. Dis. 33, 837–843. doi: 10.1007/s10096-013-2022-6
Touati, A., and Mairi, A. (2020). Epidemiology of carbapenemase-producing Enterobacterales in the Middle East: a systematic review. Expert. Rev. Anti. Infect. Ther. 18, 241–250. doi: 10.1080/14787210.2020.1729126
Treangen, T. J., Ondov, B. D., Koren, S., and Phillippy, A. M. (2014). The Harvest suite for rapid core genome alignment and visualization of thousands of intraspecific microbial genomes. Genome. Biol. 15:524.
Tsui, C. K. M., Sundararaju, S., Al Mana, H., Hasan, M. R., Tang, P., and Perez-Lopez, A. (2020). Plasmid-mediated colistin resistance encoded by mcr-1 gene in Escherichia coli co-carrying blaCTX-M-15 and blaNDM-1 genes in pediatric patients in Qatar. J. Glob. Antimicrob. Resist. 22, 662–663. doi: 10.1016/j.jgar.2020.06.029
van Aartsen, J. J. C., Moore, C. E., Parry, C. M., Turner, P., Phot, N., Mao, S., et al. (2019). Epidemiology of paediatric gastrointestinal colonisation by extended spectrum cephalosporin-resistant Escherichia coli and Klebsiella pneumoniae isolates in north-west Cambodia. BMC Microbiol. 19:59. doi: 10.1186/s12866-019-1431-9
Wood, D. E., and Salzberg, S. L. (2014). Kraken: ultrafast metagenomic sequence classification using exact alignments. Genome Biol. 15:R46.
Wyres, K. L., Hawkey, J., Hetland, M. A. K., Fostervold, A., Wick, R. R., Judd, L. M., et al. (2019). Emergence and rapid global dissemination of CTX-M-15-associated Klebsiella pneumoniae strain ST307. J. Antimicrob. Chemother. 74, 577–581. doi: 10.1093/jac/dky492
Yassine, I., Rafei, R., Osman, M., Mallat, H., Dabboussi, F., and Hamze, M. (2019). Plasmid-mediated quinolone resistance: mechanisms, detection, and epidemiology in the Arab countries. Infect. Genet. Evol. 4:104020. doi: 10.1016/j.meegid.2019.104020
Yuan, J., Xu, X., Guo, Q., Zhao, X., Ye, X., Guo, Y., et al. (2012). Prevalence of the oqxAB gene complex in Klebsiella pneumoniae and Escherichia coli clinical isolates. J. Antimicrob. Chemother. 67, 1655–1659. doi: 10.1093/jac/dks086
Zankari, E., Hasman, H., Cosentino, S., Vestergaard, M., Rasmussen, S., Lund, O., et al. (2012). Identification of acquired antimicrobial resistance genes. J. Antimicrob. Chemother. 67, 2640–2644. doi: 10.1093/jac/dks261
Zowawi, H. M., Sartor, A. L., Balkhy, H. H., Walsh, T. R., Al Johani, S. M., AlJindan, R. Y., et al. (2014). Molecular characterization of carbapenemase-producing Escherichia coli and Klebsiella pneumoniae in the countries of the Gulf cooperation council: dominance of OXA-48 and NDM producers. Antimicrob. Agents Chemother. 58, 3085–3090. doi: 10.1128/aac.02050-13
Keywords: children, whole-genome sequencing, E. coli, K. pneumoniae, extended-spectrum β-lactamases, CTX-M-15, fluoroquinolones
Citation: Perez-Lopez A, Sundararaju S, Al-Mana H, Tsui KM, Hasan MR, Suleiman M, Janahi M, Al Maslamani E and Tang P (2020) Molecular Characterization of Extended-Spectrum β-Lactamase–Producing Escherichia coli and Klebsiella pneumoniae Among the Pediatric Population in Qatar. Front. Microbiol. 11:581711. doi: 10.3389/fmicb.2020.581711
Received: 09 July 2020; Accepted: 12 October 2020;
Published: 11 November 2020.
Edited by:
Miklos Fuzi, Semmelweis University, HungaryReviewed by:
Abdelaziz Touati, University of Béjaïa, AlgeriaRafael Vignoli, Universidad de la República, Uruguay
Khalid Zerouali, University of Hassan II Casablanca, Morocco
Copyright © 2020 Perez-Lopez, Sundararaju, Al-Mana, Tsui, Hasan, Suleiman, Janahi, Al Maslamani and Tang. This is an open-access article distributed under the terms of the Creative Commons Attribution License (CC BY). The use, distribution or reproduction in other forums is permitted, provided the original author(s) and the copyright owner(s) are credited and that the original publication in this journal is cited, in accordance with accepted academic practice. No use, distribution or reproduction is permitted which does not comply with these terms.
*Correspondence: Andres Perez-Lopez, YXBlcmV6bG9wZXpAc2lkcmEub3Jn