- 1Limnological Institute, University of Konstanz, Konstanz, Germany
- 2Research Department for Limnology, University of Innsbruck, Innsbruck, Austria
The harmful bloom-forming cyanobacterium Planktothrix is commonly considered to be nutritionally inadequate for zooplankton grazers, resulting in limited top-down control. However, interactions between Planktothrix and zooplankton grazers are poorly understood. The food quality of Planktothrix is potentially constrained by morphological properties (i.e., filament formation), the production of harmful secondary metabolites, and a deficiency in essential lipids (i.e., primarily sterols). Here, we investigated the relative significance of toxin production (microcystins, carboxypeptidase A inhibitors, protease inhibitors) and sterol limitation for the performance of Daphnia feeding on one Planktothrix rubescens and one P. agardhii wild-type/microcystin knock-out mutant pair. Our data suggest that the poor food quality of both Planktothrix spp. is due to deleterious effects mediated by various harmful secondary metabolites and that the impact of sterol limitation is partially or completely superimposed by toxicity. The significance of the different factors seems to depend on the metabolite profile of the considered Planktothrix strain and the Daphnia clone that is used for the experiments. The toxin-responsive gene expression (transporter genes, gpx, and trypsin) and enzyme activity patterns revealed strain-specific food quality constraints and that Daphnia is capable of modulating its physiological responses according to the ingested Planktothrix strain. Future studies need to consider that Planktothrix–grazer interactions are simultaneously modulated by multiple factors to improve our understanding of top-down influences on Planktothrix bloom formation.
Introduction
Cyanobacteria of the genus Planktothrix are widely distributed in lakes (Kurmayer et al., 2011) and well-known to produce secondary metabolites that are potentially harmful for human health and livestock (Rohrlack et al., 2005; Kohler et al., 2014; Kurmayer et al., 2015). In past decades, Planktothrix has become the predominant photoautotrophic organism in many temperate European lakes (Rücker et al., 1997; Davis et al., 2003; Salmaso et al., 2003; Legnani et al., 2005; Rohrlack et al., 2009), presumably favored by global change and altered nutrient ratios due to lake restoration (Posch et al., 2012; Jacquet et al., 2014). In contrast to other bloom-forming cyanobacteria, Planktothrix is more competitive at low light intensities. With the help of gas vesicles, the filaments can adjust their buoyancy in the water column, enabling the establishment of deep chlorophyll maxima in the metalimnion of stratified lakes (Feuillade, 1994; Micheletti et al., 1998; Bright and Walsby, 2000; Humbert and Le Berre, 2001; Zotina et al., 2003). Furthermore, Planktothrix excrete alkaline phosphatases, allowing for the use of dissolved organic phosphorus when phosphate is depleted (Feuillade et al., 1990). Therefore, the success of Planktothrix in past decades has been attributed to a combination of physico-chemical and ecological changes, i.e., longer stratification period due to lake warming, increased water transparency due to decreasing phosphorus loads and thus decreasing phytoplankton biomass, and an advantage over other phytoplankton species that are less competitive for phosphorus (Jacquet et al., 2005, 2014; Posch et al., 2012).
Top-down influences on Planktothrix bloom formation are poorly understood. Multiple food quality constraints have been proposed rendering Planktothrix and other bloom-forming cyanobacteria nutritionally inadequate. These multiple food quality constraints have been studied only independently from each other, thus ignoring their relative significance for consumers. Filament-formation is often considered as an important mechanism hampering the ingestion of Planktothrix by zooplankton grazers. It has been shown, however, that the freshwater key-stone grazer Daphnia (Daphnia hyalina, Daphnia pulicaria) and other zooplankton taxa are able to ingest Planktothrix filaments, albeit the microcystins produced by many Planktothrix strains may act to some extent as feeding deterrents (Kurmayer and Jüttner, 1999; Oberhaus et al., 2007). Ingestion of Planktothrix has been demonstrated to be deleterious to a number of zooplankton taxa (Infante and Abella, 1985; Demott and Moxter, 1991), but the underlying mechanisms remain unclear. A field study conducted in Lake Bourget (France) suggests that Planktothrix rubescens blooms may support pelagic secondary production to a minor extent, presumably via smaller zooplankton (e.g., ciliates) upgrading the nutritionally inadequate P. rubescens for higher trophic levels (Perga et al., 2013).
Microcystins constitute a major group of cyanobacterial toxins which have been extracted from several cyanobacteria taxa, including Planktothrix (Blom et al., 2001, 2006; Baumann and Jüttner, 2008). Microcystins produced by Microcystis aeruginosa are well-known to harm Daphnia (Demott, 1999; Lürling, 2003; Schwarzenberger et al., 2014), and also microcystins produced by Planktothrix have been demonstrated to be deleterious to Daphnia (Blom et al., 2006; Hulot et al., 2012). Besides microcystins, a wide array of harmful secondary metabolites has been detected in Planktothrix strains, among them protease inhibitors (Rohrlack et al., 2009; Kohler et al., 2014; Kurmayer et al., 2016). Protease inhibitors, rather than microcystins, originating from Planktothrix have been linked to an observed mass-mortality of Daphnia in a Swiss lake (Baumann and Jüttner, 2008). In 70 % out of 89 Planktothrix strains investigated, trypsin inhibitors were detected (Rohrlack et al., 2005). Dietary protease inhibitors from M. aeruginosa strains have been shown to negatively affect Daphnia by hampering digestion and thus somatic growth rates (Rohrlack et al., 1999; Lürling, 2003; Schwarzenberger et al., 2010). However, it also has been demonstrated that Daphnia have specific means to cope with dietary protease inhibitors (Schwarzenberger et al., 2010, 2012, 2020; Von Elert et al., 2012; Schwarzenberger and Von Elert, 2013), comprising physiological adaptations potentially resulting in population tolerance (Blom et al., 2006; Baumann and Jüttner, 2008; Schwarzenberger et al., 2017). Anabaenopeptins acting as carboxypeptidase inhibitors represent another group of harmful secondary metabolites produced by Planktothrix (Itou et al., 1999). Specific responses and adaptive mechanisms of Daphnia in response to this toxin type have not been studied yet.
Besides the production of harmful secondary metabolites, cyanobacteria are of low food quality for zooplankton grazers because of a deficiency in essential lipids, i.e., sterols and long-chain polyunsaturated fatty acids (Müller-Navarra et al., 2000; Von Elert et al., 2003; Martin-Creuzburg et al., 2008). Especially, the lack of sterols in cyanobacteria has been identified as a crucial factor determining the food quality of cyanobacteria for Daphnia (Martin-Creuzburg et al., 2008). Like all arthropods, Daphnia are incapable of synthesizing sterols de novo and thus rely on an adequate dietary sterol supply to cover their physiological demands (Martin-Creuzburg et al., 2005). Daphnia take up dietary phytosterols and metabolize them to cholesterol, the main body sterol in most animals (Martin-Creuzburg et al., 2014). It has been proposed that Daphnia require at least 50% of eukaryotic carbon in their diet to compensate for a dietary sterol deficiency imposed by cyanobacteria (Martin-Creuzburg et al., 2005; Schlotz et al., 2014). Hence, the ratio between pro- and eukaryotic carbon in natural seston may determine whether or not Daphnia are limited by sterols, which in turn may affect the efficiency with which prokaryotic, i.e., cyanobacterial, carbon is transferred to higher trophic levels. The role of essential lipids in determining the food quality of Planktothrix for zooplankton grazers has not been explored yet. However, sterol production by fungal parasites (chytrids) infecting Planktothrix filaments has been suggested to upgrade the poor food quality of Planktothrix for higher trophic levels (Gerphagnon et al., 2019).
Aim of this study was to disentangle the effects of different toxin types and sterol limitation on the performance of Daphnia magna on different Planktothrix strains. Therefore, we investigated the food quality of two different microcystin-producing wild-type (WT) Planktothrix strains and their respective microcystin-free knock-out mutants (Mut) in juvenile growth experiments with D. magna. One WT/Mut pair was representing the deep-water ecotype assigned taxonomically to P. rubescens (DeCandolle ex Gomont) Anagnostidis et Komarek 1988 thriving in deep lakes and reservoirs (Kurmayer et al., 2015). The other WT/Mut pair was assigned to P. agardhii (Gomont) Anagnostidis et Komarek 1988 representing the shallow-water ecotype thriving in more shallow and polymictic lakes (Kurmayer et al., 2015). Using D. magna as a model allowed us to draw on an established system for the study of toxin-responsive gene expression. The two Planktothrix WT/Mut pairs were or were not supplemented with cholesterol in order to assess the relative significance of sterol limitation and toxicity. We hypothesized that the growth of D. magna is constrained more on the microcystin-containing wild-types than on the microcystin-free mutants, and that cholesterol supplementation can exert its positive effect on growth especially on the less toxic mutants. However, we also expected to find differences in D. magna growth between the two WT/Mut pairs, because of other potentially harmful secondary metabolites produced by these strains. To assess potential differences in toxicity between the two WT/Mut pairs, we analyzed the metabolite compositions of both wild-type strains and their respective mutants. Furthermore, we measured the specific inhibition of protein-cleaving enzymes (i.e., carboxypeptidase A and trypsins) by Planktothrix extracts and the expression of toxin-responsive genes (i.e., two transporter genes, gpx, carboxypeptidase a2, and trypsin) in D. magna to investigate the specific responses to the combination of different toxin types (i.e., microcystins, carboxypeptidase A inhibitors, protease inhibitors) that had been detected in the Planktothrix strains previously (Kohler et al., 2014). Finally, we compared growth responses of different D. magna clones on one of the two WT/Mut pairs with and without cholesterol supplementation to assess genotype-specific differences in the capacity to cope with the various food quality constraints imposed by Planktothrix.
Materials and Methods
Cultures and Preparation of Food Suspensions
Two microcystin-containing Planktothrix WT strains and their respective microcystin-free mutants were used as food in the Daphnia growth experiments: (i) the green-pigmented strain No79 assigned to P. agardhii (Gomont) Anagnostidis et Komarek 1988, isolated in 2001 from Lake Arresø, Denmark; and (ii) the red-pigmented strain No21/2 assigned to P. rubescens (DeCandolle ex Gomont) Anagnostidis et Komarek 1988, isolated in 1999 from Lake Figur, Austria (Kurmayer et al., 2005). The WT strain 79 contained demethylated microcystin-RR and -LR, whereas WT strain 21/2 contained demethylated microcystin—HtyR and -LR (Figure 1). The mutants were generated by inhibiting microcystin biosynthesis through the insertional inactivation of the mcyD gene via homologous recombination using chloramphenicol resistance as selection marker (1 μg/ml), (Kurmayer and Christiansen, 2009). The strains were grown at 20°C in 150 ml Cyano medium (Von Elert and Jüttner, 1997) in the absence of chloramphenicol at constant illumination (100 μmol quanta m−2 s−1) for 7 days prior to the experiments. The single-celled green alga Scenedesmus obliquus (SAG 276-3a, Culture Collection of Algae, University of Göttingen, Göttingen, Germany) was used as reference food. We did not use a filamentous green alga as reference food because green algal filaments are not comparable to the much softer and more fragile cyanobacterial filaments. The filament lengths of the P. rubescens strains were well within the particle size range of what D. magna is able to ingest (on average 39.9 ± 7.8 μm). Furthermore, microscopic investigations revealed that the guts of the animals were clearly filled with Planktothrix filaments during the experiments, suggesting that the animals did not starve because of filament-mediated mechanical interference of the filtration process. S. obliquus was cultured semi-continuously (dilution rate: 0.2 d−1) in Cyano medium at constant illumination in aerated 5 L flasks. Food suspensions were prepared by centrifugation and resuspension in fresh medium. The carbon concentration of the food suspensions was estimated from carbon–light extinction regressions established prior to the experiment for each WT and for each mutant.
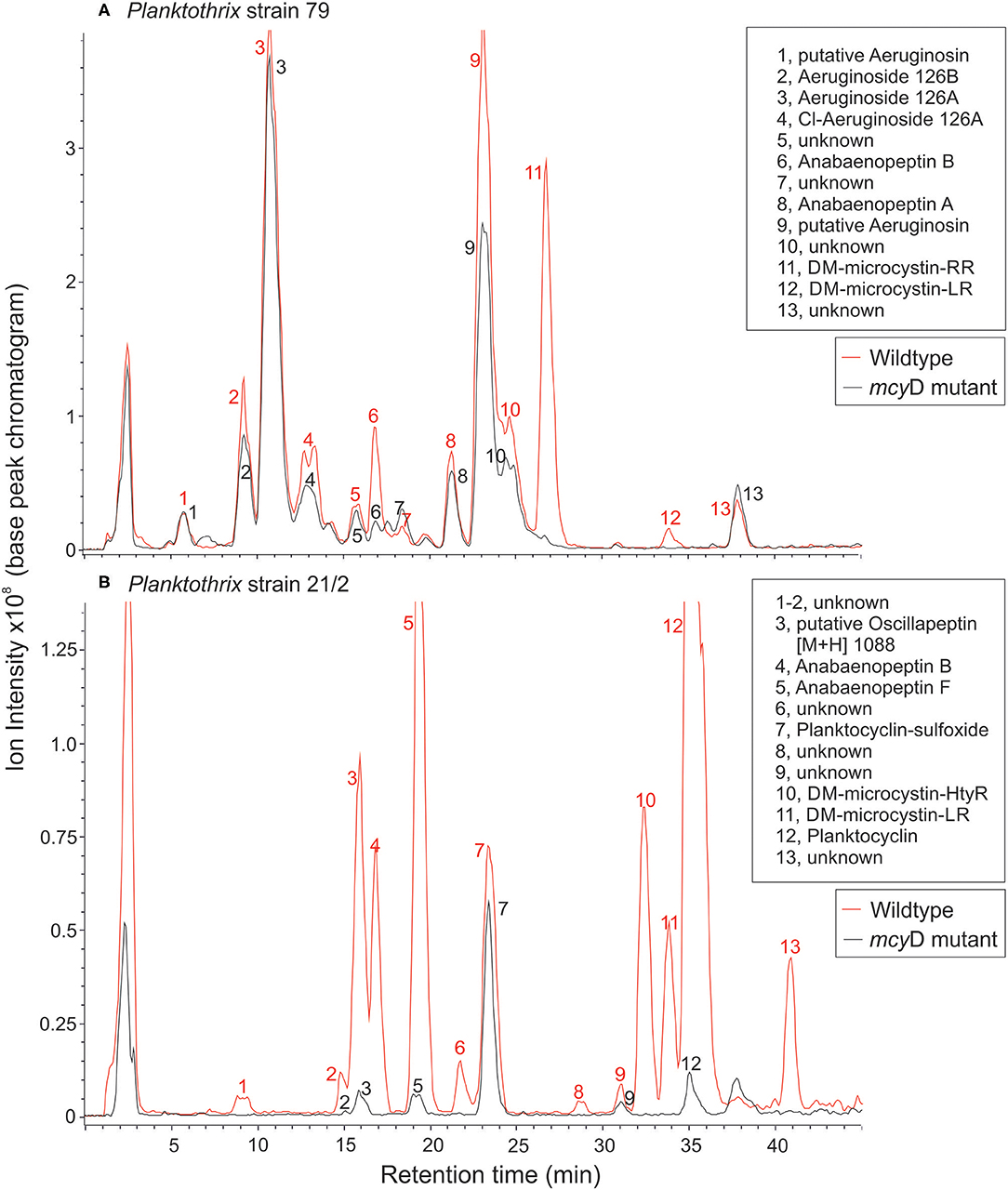
Figure 1. LC-MS chromatograms for (A) P. agardhii strain 79 and (B) P. rubescens strain 21/2 (wild type and mcyD inactivation mutant). For a list of protonated masses recorded for all fractions, see Supplementary Table 1.
Analysis of Microcystins and Other Bioactive Peptides
Planktothrix cells were frozen at −80°C and then freeze-dried. Dried cells (21/2 WT: 14.2 mg, 21/2 Δ mcyD: 2.8 mg, 79 WT: 10.1 mg, 79 Δ mcyD: 12.4 mg) were extracted in aqueous methanol (50/50, v/v) on ice, and the peptide extract was purified from cell debris by centrifugation. Peptide structural variants were separated by HPLC (HP 1100, Agilent) using a linear water/acetonitrile (0.05 % trifluoroacetic acid) gradient from 80:20 to 50:50 in 45 min at a flow rate of 1 ml min−1 in a 30°C oven, LiChrospher 100 octyldecyl silane (ODS) (5 μm particle size) and LiChroCART 250-4 cartridge system (Merck, Darmstadt, Germany). The HPLC system was coupled to an electrospray ionization (ESI) mass spectrometer ion trap (amaZonSL, Bruker) operating in positive ion mode. Nitrogen was used as sheath gas (43 psi, 8 L min−1, 300°C), and helium was used as auxiliary gas (Entfellner et al., 2017). The capillary voltage was set to 5 kV. Under these conditions, peptide structural variants were assigned according to the protonated mass in positive mode (accuracy = 0.15 Da), retention time and specific fragmentation using the enhanced resolution mode (full width at half maximum (FWHM) = 0.35, 50–2,000 Da). To determine the potential limit of detection for microcystin and other peptides, purified anabaenopeptin B (prepared by Judith Blom, Univ. of Zürich, Switzerland) and microcystin-RR, microcystin-YR, and microcystin-LR analytical standards (Cyanobiotech GmbH, Berlin, Germany) were used. Pilot injection tests with anabaenopeptin B [M+H]+ 837, microcystin-RR [M+H]+ 1,038, microcystin-YR [M+H]+ 1,045 and microcystin-LR [M+H]+ 995 revealed a limit of detection (LOD) of 10 ng (amount injected) which is equal to 0.003% of 1 mg dry weight of extracted biomass (Entfellner et al., 2017). Because of the general difficulty to standardize molecule detection in mass spectrometry, the peptide contents were compared as percentage of total peak area calculated from base peak chromatogram (arbitrarily set to 100%). Microcystins were quantified in equivalents of MC-LR. According to the linear regression curves y = 1,617.5x + 1.3696, y is the integrated area in the UV chromatogram (240 nm), and x is μg of MC-LR (0.05–0.5 μg) injected.
Daphnia Growth Experiments
Five different D. magna clones were used for the experiments, i.e., clone B (originating from Großer Binnensee, North Germany; Lampert and Rothhaupt, 1991), clone S5, clone OER 3-3 (collected from a rock pool in Finland; Haag et al., 2011), clone W (originating from a pond near Warsaw; Pijanowska et al., 1993) and clone HO2 (originating from a pond in Hungary; (Ben-Ami et al., 2008). Cohorts of each D. magna clone were raised in 1 liter jars containing filtered (0.2 μm pore-sized membrane filter) and aerated Lake Constance water and 2 mg C L−1 of the green alga S. obliquus as food. Animals were transferred to new jars containing fresh water and food every other day.
All experiments were conducted at 20°C with third-clutch neonates born within 12 h. Neonates were randomly distributed among jars (three to four per jar, three replicates) containing 200 ml of filtered lake water (0.2 μm pore-sized membrane filter) and 1 mg C L−1 of the respective food source. A first experiment was conducted with clone B to assess the overall growth capacity of the animals on Planktothrix using the WT strains as food (S. obliquus as reference food). In the second growth experiment with clone B, both the WT and the Mut strains were used as food either with or without cholesterol supplementation. Cholesterol was used because it is the main body sterol of Daphnia and thus most suitable to assess a potential sterol limitation (Martin-Creuzburg et al., 2014). Cholesterol supplementation was achieved by adding cholesterol-containing liposomes to the experimental jars, resulting in a dietary sterol concentration of 4.5 μg mg C−1. The non-cholesterol supplemented food treatments were supplemented with sterol-free liposomes so that all Planktothrix treatments received the same amount of liposomes. Liposomes were prepared according to Martin-Creuzburg et al. (2014). In the third experiment, the five different D. magna clones were raised on the WT/Mut pair 79 with and without cholesterol supplementation of the mutant. In all experiments, the animals were transferred daily to new jars containing fresh water and food. After four days of feeding, all experiments were terminated, i.e., the Daphnia removed from the jars, rinsed with ultra-pure water, transferred into pre-weighed aluminum boats, and stored at −80°C until they were freeze-dried and weighed (Mettler Toledo XP2U; ±0.1 μg) for subsequent dry mass determination. For gene expression measurements, Daphnia (clone B) were raised in separate jars (three replicates) on both WT/Mut pairs; after 4 days of feeding they were transferred into reaction tubes, stored at −80°C, and freeze dried for subsequent analyses.
Gene Expression
For qPCR analyses of relative gene expression, RNA was extracted from Daphnia using the NucleoSpin RNA Kit (Macherey-Nagel) following the manufacturer's instructions. RNA was immediately reverse-transcribed with the High-capacity cDNA Reverse Transcription Kit (Thermo Fisher Scientific). The integrity of the RNA and RNA concentrations were verified with a NanoDrop (Thermofisher). QPCR and data analyses were performed according to Schwarzenberger et al. (2009): QPCR was conducted on a 7500 Fast Real-Time PCR system (Applied Biosystems). Each reaction contained 10 ng of cDNA template, 10 μL Power SYBR® Green PCR Master Mix (Thermo Fisher Scientific) and 2.5 μM of each primer per primer pair (i.e., (i + ii) abc-transporter and multidrug/pheromone exporter), whose gene expression was demonstrated to be affected by a microcystin-producing Microcystis aeruginosa strain (Schwarzenberger et al., 2014), (iii) gpx (Schlotz et al., 2012) in order to test for putative oxidative stress due to ingestion of microcystins, (iv) trypsin [gene ID: JGI_V11_299571(wfleabase.org); primer forward 5′- ACAGCAAGTCACCGTTCAGA-3′, primer reverse 5′- GCAGGAGTCTTTGCCAGGAT-3′] and (v) carboxypeptidase a2 [gene ID: JGI_V11_214336 (wfleabase.org); primer forward 5′- TGTAGTTGCGCCTCGTCTTG-3′, primer reverse 5′- TTGCATCCTGGACCGGTTC-3′] in a final volume of 20 μL. The carboxypeptidase A2 gene has been shown to be significantly up-regulated in transcriptomes of different Daphnia species reared on cyanobacteria (Asselman et al., 2012; De Koninck et al., 2014; Schwarzenberger et al., 2014).
Each reaction was conducted in three biological replicates. Cycling parameters were 95°C for 10 min to activate the DNA polymerase followed by 40 cycles of 95°C for 15 s and 60°C for 1 min. After the actual analysis, dissociation curves were performed to verify that no primer-dimers and only one product had been amplified. For normalization, three different endogenous controls were analyzed for expression-stability between treatments (alpha-tubulin, ubc, gapdh) prior to the actual QPCR reaction for which ubc was determined as the most stable endogenous control. Relative expression of the target genes was calculated with the 7,500 Software v.2.3. Gene expression on 100% S. obliquus served as calibrator for relative gene expression and was set to 1.
Trypsin Activities
Five D. magna (clone B) grown on S. obliquus for six days were transferred to 100 μL of 0.1 M potassium-phosphate buffer (pH 7.5), homogenized with a pestle, and centrifuged for 3 min at 14,000× g. Trypsin activity was measured photometrically using the artificial substrate N-Benzoyl-Arginine-para-Nitroanilide (BApNA; Sigma-Aldrich), as described in Schwarzenberger et al. (2010). Briefly, 2.8 μL Daphnia homogenate were mixed with 990 μL 0.1 M potassium phosphate buffer (pH 7.5). The buffer contained 0.75 mM BApNA and 3% DMSO. The change in absorption was measured continuously over 3 min at 390 nm and 30°C. Planktothrix cells that were grown for seven days in 150 ml Cyano medium in Erlenmeyer flasks were harvested, frozen and freeze-dried. Fifty milligram of each of the freeze-dried Planktothrix strains were dissolved in 500 μL 60% methanol and then sonicated for 10 min to rupture the cells. The methanolic extracts were centrifuged for 3 min at 12,000× g and 10 μl of each of the supernatants was used for inhibition of trypsin activity in each of the three biological replicates. Uninhibited Daphnia homogenate served as control.
Carboxypeptidase A Activity
Twenty D. magna (clone B) grown on S. obliquus for six days were transferred to 150 μL Millipore water and homogenized with a pestle. The homogenate with a protein concentration of 1,230 μg ml−1 was centrifuged for 3 min at 14,000× g. Carboxypeptidase A activity was measured photometrically using the artificial substrate hippuryl-L-phenylalanin (Sigma-Aldrich) in a plate reader at 254 nm within 1 min after mixing. Two microlitre Daphnia homogenate were mixed with 193 μL of 25 mM Tris/NaCl HCl buffer (pH 7.5) and 6.67 μl of 1 mM hippuryl-L-phenylalanin in ethanol. Two microlitre of each of the methanolic Planktothrix extracts were used for inhibition of carboxypeptidase A activity in each of the three biological replicates.
Data Analyses
Mass-specific juvenile somatic growth rates (g) were determined as the increase in dry mass from the beginning (M0) until day four of the experiment (Mt) with time (t) expressed as age in days:
Somatic growth rates and gene expression data were analyzed using one-way analyses of variance (ANOVA). Clonal differences in growth responses were analyzed using a two-factorial ANOVA. Data met the assumptions of homogeneity of variance. Treatment effects were analyzed using Tukey's HSD post hoc tests. The level of significance was set to p ≤ 0.05. All statistical analyses were conducted using STATISTICA (StatSoft, Inc., 2011, Version 10.0.).
Results
Metabolite Composition
The peptide extracts of Planktothrix WT strains (79 and 21/2) contained both microcystins and additional peptides from different families. Strain 79 contained 1.2 μg/mg DW and 0.03 μg/mg DW of demethylated microcystin-RR and microcystin-LR, each. Strain 21/2 contained 0.42 μg/mg DW and 0.15 μg/mg DW of demethylated microcystin-HtyR and microcystin-LR, each. In addition to microcystins (14.6%) strain 79 produced several structural variants of aeruginosins (70%), anabaenopeptins (6.7%), and unknown peptides (8.7%). Strain 21/2 produced oscillapeptin (9.2%), anabaenopeptins (21.6%), microcystins (12.4%), planktocyclins (50.5%), and unknown peptides (6.4%). Conversely, the peptide extracts of ΔmcyD mutant strain 79 contained aeruginosins (82.8%), anabaenopeptins (5.2%), and unknown peptides (12%), while the ΔmcyD mutant strain 21/2 contained oscillapeptin (6.6%), anabaenopeptins (7.1%), planktocyclins (82%), and unknown peptides (4.2%). Microcystins were not detected in either of the mutants (Figure 1, Supplementary Table 1).
Daphnia Growth Experiments
The first experiment revealed the poor food quality of the two Planktothrix WT strains for D. magna. Somatic growth rates on both WT strains were significantly lower than on S. obliquus, but did not differ significantly between each other (Tukey's HSD after ANOVA, F2, 6 = 937.8, p < 0.001; Figure 2). In the second experiment, somatic growth rates on WT and Mut did not differ significantly in both WT/Mut pairs and cholesterol supplementation did not significantly improve somatic growth rates on both WT strains or their respective mutants (Tukey's HSD after ANOVA: strain 79: F4, 10 = 5.48, p = 0.013; strain 21/2: F4, 10 = 10.9, p = 0.001; Figure 3). However, somatic growth rates on the mutant of strain 79, both with and without cholesterol supplementation, did not differ significantly from that on the control food (S. obliquus), indicating higher somatic growth on the Mut than on the WT strain. Although not significant, somatic growth rates on both WT and Mut of strain 79 slightly increased upon cholesterol supplementation.
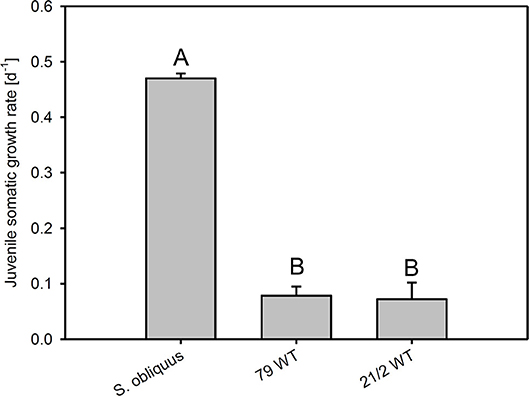
Figure 2. Juvenile somatic growth rates of D. magna reared on the green alga S. obliquus and on two different Planktothrix wild-type strains (79 and 21/2), respectively. Different letters indicate significant differences among treatments (Tukey's HSD, p ≤ 0.05; N = 3, + SD).
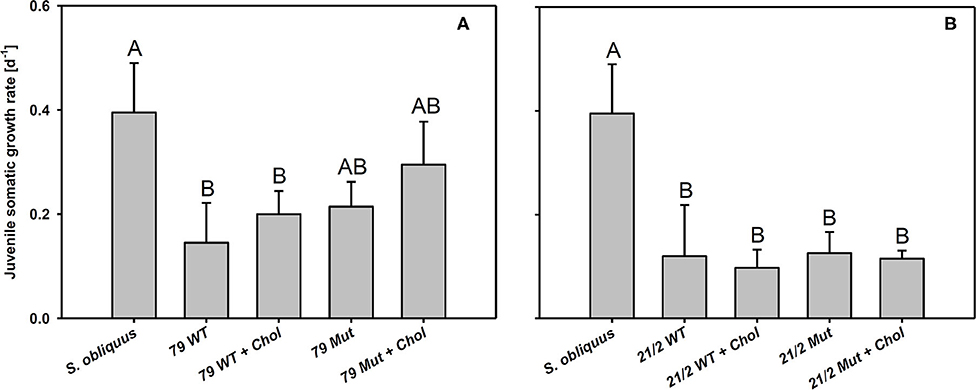
Figure 3. Juvenile somatic growth rates of D. magna reared on the green alga S. obliquus and on the two wild-types and mutants of Planktothrix strains 79 (A) and 21/2 (B) with or without cholesterol supplementation. Different letters indicate significant differences among treatments (Tukey's HSD, p ≤ 0.05; N = 3 + SD).
The third experiment revealed significant differences in growth responses on the WT and the Mut of strain 79 among the five different D. magna clones used here (Tukey's HSD following two-factorial ANOVA; Table 1; Figure 4). Growth on S. obliquus did not differ significantly among clones; and somatic growth rates of all clones were significantly lower on Planktothrix than on S. obliquus (Tukey's HSD, p < 0.05). Only one clone (HO2) achieved significantly higher somatic growth rates on the Mut than on the WT strain (Tukey's HSD, p < 0.05; Figure 4C); all individuals of HO2 died after four days of exposure to the WT strain. Somatic growth rates on the Mut increased significantly upon cholesterol supplementation in two (B and W) out of the five clones tested (Tukey's HSD, p < 0.05; Figure 4 B and W).
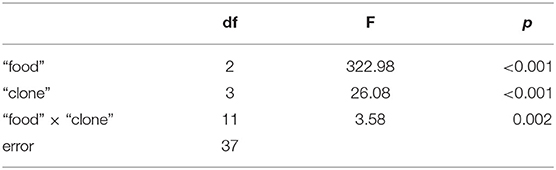
Table 1. Results of the two-factorial ANOVA used to analyse growth rate differences among the five different D. magna clones that were reared on either S. obliquus or the wild-type and the microcystin-free mutant (with and without cholesterol supplementation) of P. agardhii strain 79.
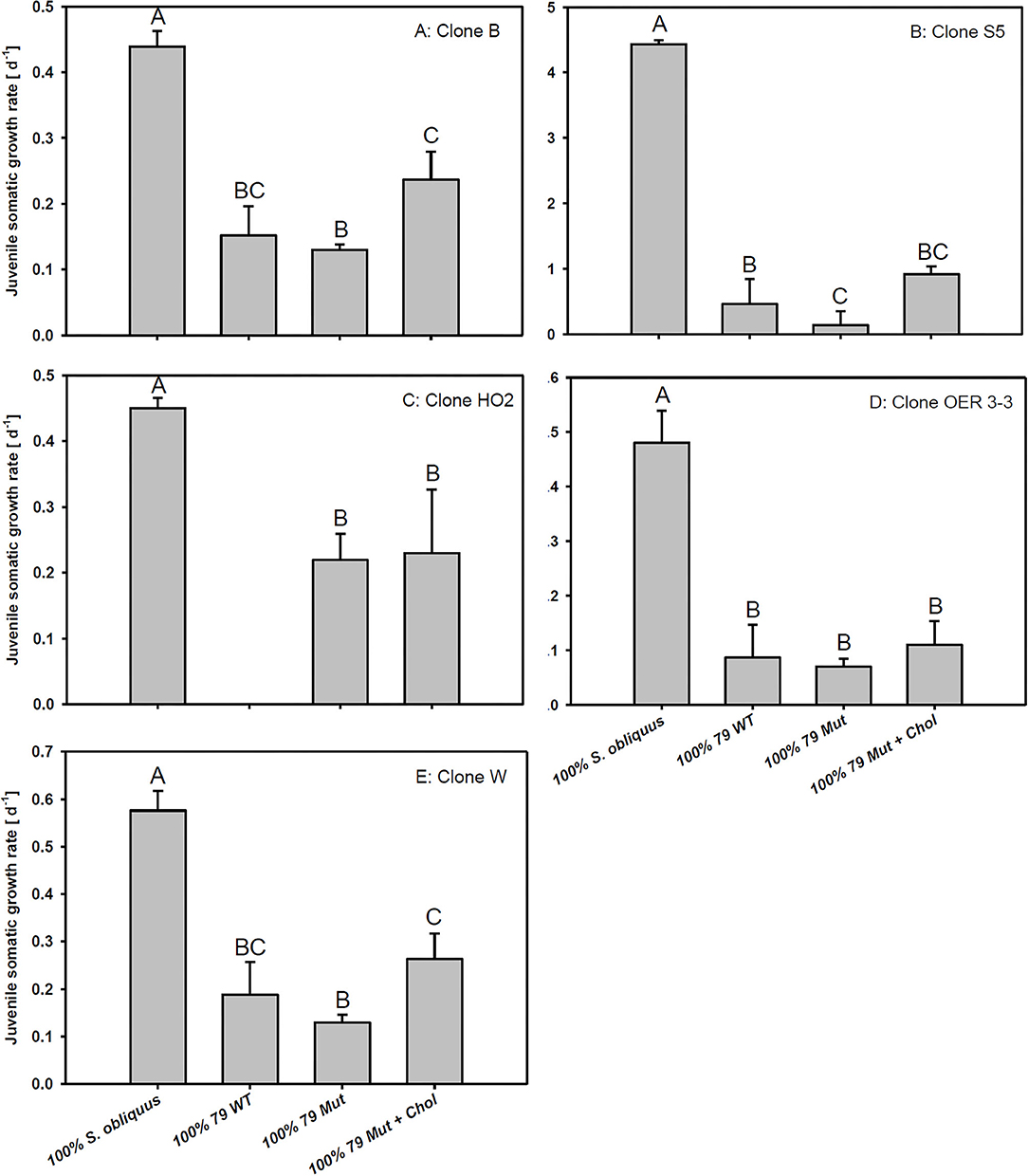
Figure 4. Juvenile somatic growth rates of five different D. magna clones (A–E) reared on the green alga S. obliquus, the wild-type of P. agardhii strain 79, or its mutant with and without cholesterol supplementation. Different letters indicate significant differences among treatments (Tukey's HSD, p ≤ 0.05; N = 3 + SD).
Target Gene Expression, Trypsin, and Carboxypeptidase A Activity
The expression of transporter genes (abc-transporter and multidrug/pheromone exporter) was significantly up-regulated in D. magna reared on the WT strains and their respective mutants (79 and 21/2) relative to S. obliquus (Figures 5A,B). Furthermore, the expression of both transporter genes was significantly higher in D. magna reared on the WT strains than in those reared on the respective mutants (abc-transporter: Tukey's HSD after ANOVA, F4, 10 = 3285.1, p < 0.001; multidrug/pheromone exporter: Tukey's HSD after ANOVA, F4, 10 = 4597.2, p < 0.001; Figures 5A,B). Gpx expression was significantly up-regulated on both WT strains and the mutant of strain 79 but not on the mutant of 21/2 (Tukey's HSD after ANOVA, F4, 10 = 218.2, p < 0.001; Figure 5C).
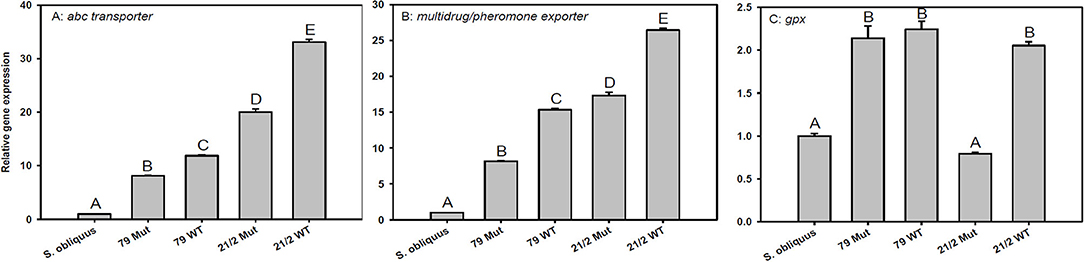
Figure 5. Relative gene expression in D. magna reared on the green alga S. obliquus and on the two different Planktothrix wild-type strains (79 and 21/2), respectively. Shown is the relative expression of (A) abc-transporter, (B) multi-drug/pheromone exporter, and (C) gpx (Tukey's HSD, p ≤ 0.05; N = 3 + SD).
Trypsin expression was also significantly up-regulated in D. magna reared on the WT strains and their respective mutants (79 and 21/2) relative to S. obliquus (Tukey's HSD after ANOVA, F4, 10 = 9929.6, p < 0.001; Figure 6B). Both WT/Mut pairs significantly inhibited trypsin activity, with the WT/Mut pair 21/2 being more efficient (Tukey's HSD after ANOVA, F4, 10 = 4957.5, p < 0.001; Figure 6A). Inhibition of trypsin activity did not differ between WT and Mut in both WT/Mut pairs. Carboxypeptidase a2 expression in D. magna was also significantly up-regulated on both WT strains and their respective mutants, albeit expression levels were higher on strain 21/2, especially on the WT (Tukey's HSD after ANOVA, F4, 10 = 18177.3, p < 0.001; Figure 7B). Carboxypeptidase A activity was significantly inhibited only by the WT of strain 21/2 (Tukey's HSD after ANOVA, F4, 10 = 62.5, p < 0.001; Figure 7A).
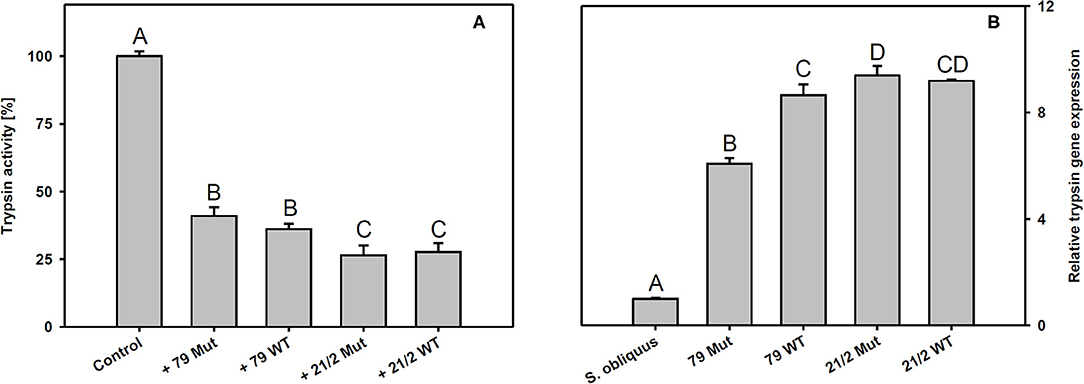
Figure 6. (A) Trypsin activity in D. magna homogenates treated with methanolic extracts gained from the two different Planktothrix wild-type/mutant pairs. The animals were all reared on S. obliquus. Data show the Planktothrix-mediated inhibition of trypsin activity relative to the control without inhibitor. (B) Relative gene expression of trypsin in D. magna reared on S. obliquus or on the two different Planktothrix wild-type/mutant pairs. Different letters indicate significant differences among treatments (Tukey's HSD, p ≤ 0.05; N = 3 + SD).
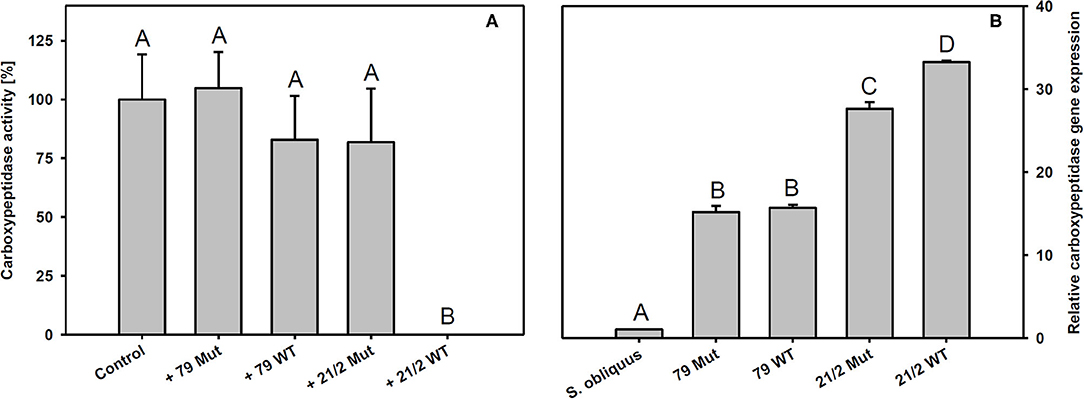
Figure 7. (A) Carboxypeptidase A activity in D. magna homogenates treated with methanolic extracts gained from the two different Planktothrix wild-type/mutant pairs. The animals were all reared on S. obliquus. Data show the Planktothrix-mediated inhibition of carboxypeptidase A activity relative to the control without inhibition. (B) Relative gene expression of carboxypeptidase a2 in D. magna reared on S. obliquus or on the two different Planktothrix wild-type/mutant pairs. Different letters indicate significant differences among treatments (Tukey's HSD, p ≤ 0.05; N = 3 + SD).
Discussion
We reared D. magna on two Planktothrix WT/Mut pairs (79 and 21/2) differing in metabolite composition (Figure 1; Supplementary Table 1). The two WT/Mut pairs allowed us to disentangle food quality effects imposed by microcystins from those mediated by other metabolites, because the WT strains and their respective mutants do not differ in metabolite composition, except for the production of microcystins. Microcystins have been detected in many but not all Planktothrix blooms investigated (Fastner et al., 1999; Davis et al., 2015). Somatic growth rates of D. magna on both Planktothrix WT strains were low, emphasizing the poor food quality of Planktothrix. Although statistically not significant, somatic growth rates on the Mut of strain 79 were higher than those obtained on the respective WT strain, suggesting that the nutritional constraints imposed by the WT strain 79 were at least partially related to microcystin production. Somatic growth rates on the Mut of strain 21/2 were indistinguishable from that of the WT strain, implying that the poor food quality of this strain is due to additional factors than only microcystin production. Supplementation with cholesterol did not significantly improve somatic growth rates of D. magna on both WT/Mut pairs, indicating that the inevitable sterol limitation was superimposed by toxicity, even on the microcystin-free mutants.
This adds to similar results obtained with a WT/Mut pair of M. aeruginosa (PCC7806), for which the microcystins present in the WT strain have been clearly shown to cause high mortality of Daphnia (Rohrlack et al., 1999, 2001; Lürling, 2003). Despite the absence of microcystins, however, somatic growth rates of Daphnia on the mcyD mutant of M. aeruginosa remain low, irrespective of cholesterol supplementation, suggesting that the poor food quality of this microcystin-free mutant is caused by harmful secondary metabolites in addition to microcystins (Lürling, 2003; Martin-Creuzburg et al., 2008). It has been suggested that the poor food quality of microcystin-free M. aeruginosa strains is mediated by an inhibition of the ingestion process by an as yet unknown feeding deterrent (Lampert, 1981; Rohrlack et al., 2001; Lürling, 2003). Mechanical or chemical feeding inhibition might be responsible for the poor food quality of Planktothrix as well, although microscopic examination of our experimental animals did not support this hypothesis. The guts of all animals were clearly filled with Planktothrix filaments and the animals did not show signs of feeding inhibition throughout the experiments, irrespective of whether the WT strains or the mutants were provided as food.
The involvement of glutathione S-transferases (GST) in detoxification of microcystins in Daphnia has been discussed based on results obtained in an in vitro study (Pflugmacher et al., 1998). More recently, however, it has been proposed that Daphnia's GST only play a minor role in microcystin detoxification in vivo and that the excretion of microcystins and potentially other toxic substances via transporters is more important (Sadler and Von Elert, 2014a,b). In Lepidoptera, ABC transporter modifications have been linked to the evolution of resistance to Bacillus thuringiensis toxins (Heckel, 2012). In Daphnia, transporters of the OATP and ABC superfamily have been shown to be involved in the transport of drugs and toxins (Konings et al., 1997; Vasiliou et al., 2009; Sadler, 2014). Moreover, the expression of two transporter genes in D. magna (abc-transporter and multidrug/pheromone exporter), both belonging to the ABC superfamily, has been found to be responsive to microcystins supplemented via liposomes (Schwarzenberger et al., 2014). We show here that the expression of these two transporter genes is also highly responsive to Planktothrix exposure. Feeding on the two Planktothrix WT strains resulted in a significantly higher up-regulation of transporter gene expression in D. magna than feeding on their respective microcystin-free mutants. However, the expression of these transporter genes was also significantly up-regulated in D. magna feeding on the microcystin-free mutants, suggesting that ABC transporter gene expression is responsive to microcystins and other harmful metabolites present in Planktothrix. Differences in transporter gene expression between both WT strains are presumably also linked to differences in their metabolite composition. Assuming that the increase in transporter gene expression actually resulted in an increase in transporter proteins within cell membranes, these findings imply that the animals tried to brace themselves against the multiple harmful metabolites present in Planktothrix by increasing their toxin export capacities. The higher transporter gene expression in D. magna feeding on Planktothrix might well have compensated for further deleterious effects of harmful metabolites on growth or survival.
Besides inhibition of phosphatases, exposure to microcystins can lead to an excessive formation of reactive oxygen species (ROS) in aquatic organisms and thus oxidative stress (Jos et al., 2005; Amado and Monserrat, 2010). Glutathione peroxidase (GPX) is an enzyme that metabolizes fatty-acids oxidized by ROS. Increases in gpx expression and GPX activity in mice and zebra fish in response to microcystin exposure have been proposed to contribute to cellular detoxification (Wiegand et al., 1999; Gehringer et al., 2004; Amado and Monserrat, 2010). Gpx expression was significantly up-regulated in D. magna feeding on the WT strain 21/2 but not in animals feeding on its microcystin-free mutant, suggesting higher oxidative damage in response to microcystin exposure. However, gpx expression increased similarly in animals feeding on the WT strain 79 and in animals feeding on its microcystin-free mutant, thus not supporting this conclusion. The up-regulation of gpx expression in animals feeding on the mutant of strain 79 was most likely caused by other deleterious metabolites generating oxidative stress. It remains to be tested if the up-regulation of gpx expression in animals can be used as a general indicator of oxidative stress induced by harmful cyanobacterial metabolites.
The findings described above suggest that the poor food quality of Planktothrix for Daphnia cannot be solely attributed to the production of microcystins or the lack of sterols. In addition, there must be other secondary metabolites that are harmful to Daphnia, such as the planktocyclins that were found both in the WT and the mutant of strain 21/2 but not in strain 79. Planktocyclins are highly potent protease inhibitors with LC50 values in the lower nanomolar range that have been previously detected in other Planktothrix strains (Baumann et al., 2007). The occurrence of planktocyclins in the WT and the mutant of strain 21/2 may explain why the microcystin-free mutant was still of poor food quality for D. magna. Anabaenopeptins represent another group of potentially harmful metabolites that have been found in the Planktothrix strains investigated here (Supplementary Table 1; Kohler et al., 2014). While some structural variants of anabaenopeptins have been shown to clearly inhibit the activity of carboxypeptidase A, others (anabaenopeptin A, B, and F) have been found to exert little inhibitory activity against carboxypeptidase A (Itou et al., 1999). Schreuder et al. (2016) proposed that enzymatic targets of the more abundant anabaenopeptins B and F resemble carboxypeptidase B rather than carboxypeptidase A. We show here that methanolic extracts prepared from the WT of P. rubescens strain 21/2 significantly inhibit carboxypeptidase A activity in vitro. Surprisingly, extracts prepared from the microcystin-free mutant of strain 21/2 did not significantly inhibit carboxypeptidase A activity and had a low inhibitory effect which was found similar to the methanolic extract of strain 79 (Figure 7A). Thus, the inhibitory effect of peptide variants anabaenopeptin B and anabaenopeptin A (synthesized by strain 79) and anabaenopeptin B and anabaenopeptin F (synthesized by strain 21/2) on carboxypeptidase A is considered relatively weak. For strain 21/2 differences in concentration of unknown carboxypeptidase inhibitors between WT and mutant might be involved which would require further testing. Correspondingly to the complete inhibitory effect the highest carboxypeptidase a2 gene expression was observed in Daphnia exposed to the WT of strain 21/2, indicating that Daphnia were physiologically responsive to the WT 21/2 (Figure 7B). In contrast to strain 21/2, strain 79 did not significantly inhibit carboxypeptidase A activity in vitro. Nevertheless, the up-regulation of carboxypeptidase a2 gene expression in D. magna feeding on the WT and the mutant of strain 79 suggests that the animals were responsive also to yet unknown carboxypeptidase inhibitors present in strain 79. Differences in the capacity to inhibit carboxypeptidase A activity and to modulate gene expression responses in D. magna were presumably due to differences in the concentrations of carboxypeptidase A inhibitors between WTs and their mutants or their efficiency to bind to carboxypeptidase A of D. magna.
Besides anabaenopeptins, P. rubescens strain 21/2 also contains cyanopeptolins (Kohler et al., 2014). Cyanopeptolins have been shown to inhibit trypsin activity in vitro (Schwarzenberger et al., 2012, 2013), and, together with other protease inhibitors (planktocyclins), these peptides have been proposed to cause mass-mortalities of Daphnia in nature (Baumann and Jüttner, 2008). Therefore, we measured trypsin activity in Daphnia homogenates in the absence and presence of methanolic peptide extracts obtained from the two Planktothrix WT/Mut pairs. We found that peptide extracts of both strains inhibited trypsin activity and that the strength of inhibition did not differ between WT and the respective mutant. Moreover, trypsin gene expression was up-regulated in D. magna upon exposure to Planktothrix, which is in accordance with earlier findings for M. aeruginosa and three other trypsin genes (Schwarzenberger et al., 2010). Aeruginosins, peptide metabolites that potentially inhibit different types of serine proteases (Ishida et al., 2007), have been proposed to be responsible for the toxicity of microcystin-deficient Planktothrix strains in acute toxicity assays with the crustacean Thamnocephalus platyurus (Kohler et al., 2014). We found aeruginosins only in the WT and the mutant of strain 79, i.e., the toxicity of strain 21/2 cannot be attributed to aeruginosins. Our data suggest that the entire set of toxic secondary metabolites present in Planktothrix must be considered in order to determine food quality for D. magna. For example the ongoing production of planktocyclins in strain 21/2 Mut might be responsible for any observed effect of sterol addition on somatic growth of D. magna. In contrast, the food quality of strain 79 Mut seemed to increase after eradication of the microcystins (Figure 3).
To assess clonal differences in the susceptibility to Planktothrix, we reared five different D. magna clones on the WT/Mut pair of strain 79 with and without cholesterol supplementation of the mutant and S. obliquus as reference food. The growth responses on the different food sources differed among D. magna clones, indicating genotype-specific differences in the capacity to cope with Planktothrix. The most pronounced result was that all individuals of clone HO2 died within 4 days of exposure to the microcystin-producing wild-type strain 79, whereas all individuals of the other four clones survived on this food until the end of the experiment. No mortality of clone HO2 was observed on the microcystin-free mutant, suggesting that this clone is highly susceptible to microcystin-RR and microcystin-LR. In contrast, growth rates of clones B, OER 3-3, and W did not differ between WT and its microcystin-free mutant, implying that these clones are less sensitive to microcystins. Contrary to expectations, growth rates of clone S5 were even higher on the WT than on the microcystin-free mutant. A possible explanation might be that the disabled microcystin production in the mutant is associated with an increase in the production of other harmful secondary metabolites, such as aeruginosins, to which clone S5 is more sensitive than to microcystins. Cholesterol supplementation significantly increased somatic growth rates of only two out of the five D. magna clones tested, suggesting clone-specific differences in the sensitivity to one or the other toxin type and therewith the capacity of cholesterol to compensate for the toxin-mediated growth inhibition.
Conclusion
Cyanobacterial bloom formation might be modulated by top-down influences, highlighting the importance of studying trophic interactions between cyanobacteria and zooplankton grazers. Trophic interactions between Planktothrix and zooplankton grazers involve multiple nutritional constraints imposed by microcystins and related toxic peptides with strain specific relative significance. Our data suggest that the poor food quality of Planktothrix is due to multiple factors simultaneously impairing the performance of Daphnia. These factors include deleterious effects mediated by harmful secondary metabolites as well as sterol limitation. The significance of the different factors seems to depend on the metabolite profile of the considered Planktothrix strain. As has been shown for the genus Microcystis, the poor food quality of Planktothrix cannot be attributed solely to microcystin production, as indicated by the results we obtained for the microcystin-free Planktothrix mutants. Furthermore, our experiments indicate that the impact of sterol limitation on Daphnia performance is partially or completely superimposed by toxicity, depending on the Planktothrix strain and the Daphnia clone studied. Thus, the absence of sterols in Planktothrix is presumably less relevant for Daphnia than the production of harmful secondary metabolites. Despite pronounced differences in metabolite composition, the two Planktothrix WT strains hardly differed in food quality, i.e., they all resulted in similar low somatic growth rates of D. magna. Although the different combinations of food quality constraints imposed by the P. rubescens strains provoked similar effects on the performance of D. magna, the physiological responses were specific to the respective metabolites. We show here that abc transporter, trypsin, carboxypeptidase a2, and gpx expression in D. magna is highly responsive to Planktothrix exposure. We also show that the digestive trypsin activity in D. magna is affected by trypsin inhibitors and that the carboxypeptidase A activity is affected by carboxypeptidase A inhibitors present in Planktothrix in vitro. Moreover, we provide evidence for clone-specific differences in the tolerance to Planktothrix in D. magna. Using D. magna allowed us to draw on an established model for toxin-responsive gene expression but may have minimized the impact of Planktothrix filaments on the filtration process, assuming that larger Daphnia species are less susceptible to filament-caused mechanical interference with filtration than smaller species. Future studies assessing Planktothrix–grazer interactions need to consider that the top-down impact on Planktothrix blooms is modulated by multiple factors, including sterol limitation and toxicity, which is mediated through a diverse array of harmful secondary metabolites.
Data Availability Statement
All datasets generated for this study are included in the article/Supplementary Material.
Author Contributions
AS and DM-C designed the experiments that were conducted by AS. RK provided the Planktothrix wildtype and mcyD mutant strains and analyzed their metabolites. AS and DM-C wrote the first draft of the manuscript. All authors contributed to data interpretation and the final draft of the manuscript.
Funding
DM-C received funding from the German Research Foundation (DFG; 298726046/GRK2272) and the Ministry of Science, Research and the Arts of the Federal State Baden–Württemberg, Germany, within the Water Research Network project: Challenges of Reservoir Management—Meeting Environmental and Social Requirements. RK was supported by the Austrian Science Fund (FWF; P32193).
Conflict of Interest
The authors declare that the research was conducted in the absence of any commercial or financial relationships that could be construed as a potential conflict of interest.
Acknowledgments
The authors thank Jasminca Behrmann-Godel and Peter Kroth for providing access to their molecular biology laboratories and facilities.
Supplementary Material
The Supplementary Material for this article can be found online at: https://www.frontiersin.org/articles/10.3389/fmicb.2020.586120/full#supplementary-material
References
Amado, L. L., and Monserrat, J. M. (2010). Oxidative stress generation by microcystins in aquatic animals: why and how. Environ. Int. 36, 226–235. doi: 10.1016/j.envint.2009.10.010
Asselman, J., De Coninck, D. I. M., Glaholt, S., Colbourne, J. K., Janssen, C. R., Shaw, J. R., et al. (2012). Identification of pathways, gene networks, and paralogous gene families in Daphnia pulex responding to exposure to the toxic cyanobacterium Microcystis aeruginosa. Environ. Sci. Technol. 46, 8448–8457. doi: 10.1021/es301100j
Baumann, H. I., and Jüttner, F. (2008). Inter-annual stability of oligopeptide patterns of Planktothrix rubescens blooms and mass mortality of Daphnia in Lake Hallwilersee. Limnologica 38, 350–359. doi: 10.1016/j.limno.2008.05.010
Baumann, H. I., Keller, S., Wolter, F. E., Nicholson, G. J., Jung, G., Süssmuth, R. D., et al. (2007). Planktocyclin, a cyclooctapeptide protease inhibitor produced by the freshwater cyanobacterium Planktothrix rubescens. J. Nat. Prod. 70, 1611–1615. doi: 10.1021/np0700873
Ben-Ami, F., Mouton, L., and Ebert, D. (2008). The effects of multiple infections on the expression and evolution of virulence in a Daphnia-endoparasite system. Evol. Int. J. Organ. Evol. 62, 1700–1711. doi: 10.1111/j.1558-5646.2008.00391.x
Blom, J. F., Baumann, H., Codd, G. A., and Jüttner, F. (2006). Sensitivity and adaptation of aquatic organisms to oscillapeptin J and [D-Asp3,(E)-Dhb7]microcystin-RR. Arch. Fuer. Hydrobiol. 167, 547–559. doi: 10.1127/0003-9136/2006/0167-0547
Blom, J. F., Robinson, J. A., and Jüttner, F. (2001). High grazer toxicity of (D-Asp3,(E)-Dhb7)microcystin-RR of Planktothrix rubescens as compared to different microcystins. Toxicon 39, 1923–1932. doi: 10.1016/S0041-0101(01)00178-7
Bright, D. I., and Walsby, A. E. (2000). The daily integral of growth by Planktothrix rubescens calculated from growth rate in culture and irradiance in Lake Zürich. New Phytol. 146, 301–316. doi: 10.1046/j.1469-8137.2000.00640.x
Davis, P. A., Dent, M., Parker, J., Reynolds, C. S., and Walsby, A. E. (2003). The annual cycle of growth rate and biomass change in Planktothrix spp. in Blelham Tarn, english Lake District. Freshwater Biol. 48, 852–867. doi: 10.1046/j.1365-2427.2003.01055.x
Davis, T. W., Bullerjahn, G. S., Tuttle, T., McKay, R. M., and Watson, S. B. (2015). Effects of increasing nitrogen and phosphorus concentrations on phytoplankton community growth and toxicity during Planktothrix blooms in Sandusky Bay, Lake Erie. Environ. Sci. Technol. 49, 7197–7207. doi: 10.1021/acs.est.5b00799
De Koninck, D. I., Asselman, J., laholt, S., Janssen, C. R., Colbourne, J. K., Shaw, J. R., et al. (2014). Genome-wide transcription profiles reveal genotype-dependent responses of biological pathways and gene-families in Daphnia exposed to single and mixed stressors. Environ. Sci. Technol. 48, 3513–3522. doi: 10.1021/es4053363
Demott, W. R. (1999). Foraging strategies and growth inhibition in five daphnids feeding on mixtures of a toxic cyanobacterium and a green alga. Freshw. Biol. 42, 263–274. doi: 10.1046/j.1365-2427.1999.444494.x
Demott, W. R., and Moxter, F. (1991). Foraging on cyanobacteria by copepods - responses to chemical defenses and resource abundance. Ecology 72, 1820–1834. doi: 10.2307/1940981
Entfellner, E., Frei, M., Christiansen, G., Deng, L., Blom, J. F., and Kurmayer, R. (2017). Evolution of anabaenopeptin peptide structural variability in the cyanobacterium Planktothrix. Front. Microbiol. 8:219. doi: 10.3389/fmicb.2017.00219
Fastner, J., Erhard, M., Carmichael, W. W., Sun, F., Rinehart, K. L., Ronicke, H., et al. (1999). Characterization and diversity of microcystins in natural blooms and strains of the genera Microcystis and Planktothrix from German freshwaters. Arch. für Hydrobiol. 1452, 147–163. doi: 10.1127/archiv-hydrobiol/145/1999/147
Feuillade, J. (1994). The cyanobacterium (blue-green alga) Oscillatoria rubescens D.C. Ach. für Hydrobiol. 41, 77–93.
Feuillade, J., Feuillade, M., and Blanc, P. (1990). Alkaline phosphatase activity fluctuations and associated factors in a eutrophic lake dominated by Oscillatoria rubescens. Hydrobiologia, 207, 233–240. doi: 10.1007/BF00041461
Gehringer, M. M., Shephard, E. G., Downing, T. G., Wiegand, C., and Neilan, B. A. (2004). An investigation into the detoxification of microcystin-LR by the glutathione pathway in Balb/c mice. Int. J. Biochem. Cell Biol. 36, 931–941. doi: 10.1016/j.biocel.2003.10.012
Gerphagnon, M., Agha, R., Martin-Creuzburg, D., Bec, A., Perriere, F., Rad-Menéndez, C., et al. (2019). Comparison of sterol and fatty acid profiles of chytrids and their hosts reveals trophic upgrading of nutritionally inadequate phytoplankton by fungal parasites. Environ. Microbiol. 21, 949–958. doi: 10.1111/1462-2920.14489
Haag, K. L., Larsson, J. R., Refardt, D., and Ebert, D. (2011). Cytological and molecular description of Hamiltosporidium tvaerminnensis gen. et sp. nov., a microsporidian parasite of Daphnia magna, and establishment of Hamiltosporidium magnivora comb. nov. Parasitology 138, 447–462. doi: 10.1017/S0031182010001393
Heckel, D. G. (2012). Learning the ABCs of Bt: ABC transporters and insect resistance to Bacillus thuringiensis provide clues to a crucial step in toxin mode of action. Pestic. Biochem. Physiol. 104, 103–110. doi: 10.1016/j.pestbp.2012.05.007
Hulot, F. D., Carmignac, D., Legendre, S., Yéprémian, C., and Bernard, C. (2012). Effects of microcystin-producing and microcystin-free strains of Planktothrix agardhii on long-term population dynamics of Daphnia magna. Ann. Limnol. Int. J. Limnol. 48, 337–347. doi: 10.1051/limn/2012023
Humbert, J. F., and Le Berre, B. (2001). Genetic diversity in two species of freshwater cyanobacteria, Planktothrix (Oscillatoria) rubescens and P. agardhii. Arch. für Hydrobiol. 1150, 97–206. doi: 10.1127/archiv-hydrobiol/150/2001/197
Infante, A., and Abella, S. E. B. (1985). Inhibition of Daphnia by Oscillatoria in Lake Washington. Limnol. Oceanogr. 30, 1046–1052. doi: 10.4319/lo.1985.30.5.1046
Ishida, K., Christiansen, G., Yoshida, W. Y., Kurmayer, R., Welker, M., Valls, N., et al. (2007). Biosynthesis and structure of aeruginoside 126A and 126B, cyanobacterial peptide glycosides bearing a 2-carboxy-6-hydroxyoctahydroindole moiety. Chem. Biol. 14, 565–576. doi: 10.1016/j.chembiol.2007.04.006
Itou, Y., Suzuki, S., Ishida, K., and Murakami, M. (1999). Anabaenopeptins G and H, potent carboxypeptidase a lnhibitors from the cyanobacterium Oscillatoria agardhii (NIES-595). Bioorg. Med. Chem. Lett. 9, 1243–1246. doi: 10.1016/S0960-894X(99)00191-2
Jacquet, S., Briand, J.-F., Leboulanger, C., Avois-Jacquet, C., Oberhaus, L., Tassin, B., et al. (2005). The proliferation of the toxic cyanobacterium Planktothrix rubescens following restoration of the largest natural French lake (Lac du Bourget). Harmful Algae 4, 651–672. doi: 10.1016/j.hal.2003.12.006
Jacquet, S., Kerimoglu, O., Rimet, F., Paolini, G., and Anneville, O. (2014). Cyanobacterial bloom termination: the disappearance of Planktothrix rubescens from Lake Bourget (France) after restoration. Freshw. Biol. 59, 2472–2487. doi: 10.1111/fwb.12444
Jos, A., Pichardo, S., Prieto, A. I., Repetto, G., Vázques, C. M., Moreno, I., et al. (2005). Toxic cyanobacterial cells containing microcystins induce oxidative stress in exposed tilapia fish (Oreochromis sp.) under laboratory conditions. Aquat. Toxicol. 72, 261–271. doi: 10.1016/j.aquatox.2005.01.003
Kohler, E., Grundler, V., Häussinger, D., Kurmayer, R., Gademann, K., Pernthaler, J., et al. (2014). The toxicity and enzyme activity of a chlorine and sulfate containing aeruginosin isolated from a non-microcystin-producing Planktothrix strain. Harmful Algae 39, 154–160. doi: 10.1016/j.hal.2014.07.003
Konings, W. N., Lolkema, J. S., Bolhuis, H., Van Veen, H. W., Poolman, B., and Driessen, A. J. M. (1997). The role of transport processes in survival of lactic acid bacteria, energy transduction and multidrug resistance. Antonie van Leeuw. 71, 117–128. doi: 10.1023/A:1000143525601
Kurmayer, R., Blom, J. F., Deng, L., and Pernthaler, J. (2015). Integrating phylogeny, geographic niche partitioning and secondary metabolite synthesis in bloom-forming Planktothrix. ISME J. 9, 909–921. doi: 10.1038/ismej.2014.189
Kurmayer, R., and Christiansen, G. (2009). The genetic basis of toxin production in cyanobacteria. Freshwater Rev. 2, 31–50. doi: 10.1608/FRJ-2.1.2
Kurmayer, R., Christiansen, G., Gumpenberger, M., and Fastner, J. (2005). Genetic identification of microcystin ecotypes in toxic cyanobacteria of the genus Planktothrix. Microbiology 151, 1525–1533. doi: 10.1099/mic.0.27779-0
Kurmayer, R., Deng, L., and Entfellner, E. (2016). Role of toxic and bioactive secondary metabolites in colonization and bloom formation by filamentous cyanobacteria Planktothrix. Harmful Algae 54, 69–86. doi: 10.1016/j.hal.2016.01.004
Kurmayer, R., and Jüttner, F. (1999). Strategies for the co-existence of zooplankton with the toxic cyanobacterium Planktothrix rubescens in Lake Zurich. J. Plankton Res. 21, 659–683. doi: 10.1093/plankt/21.4.659
Kurmayer, R., Schober, E., Tonk, L., Visser, P., and Christiansen, G. (2011). Spatial divergence in the proportions of genes encoding toxic peptide synthesis among populations of the cyanobacterium Planktothrix in European lakes. FEMS Microbiol. Lett. 317, 127–137. doi: 10.1111/j.1574-6968.2011.02222.x
Lampert, W. (1981). Inhibitory and toxic effects of blue-green algae on Daphnia. Int. Revue der Gesamten Hydrobiol. 66, 285–298. doi: 10.1002/iroh.19810660302
Lampert, W., and Rothhaupt, K. O. (1991). Alternating dynamics of rotifers and Daphnia magna in a shallow lake. Arch. für Hydrobiol. 120, 447–456.
Legnani, E., Copetti, D., Oggioni, A., Tartari, G., Palumbo, M. T., and Morabito, G. (2005). Planktothrix rubescens' seasonal dynamics and vertical distribution in Lake Pusiano (North Italy). J. Limnol. 64, 61–73. doi: 10.4081/jlimnol.2005.61
Lürling, M. (2003). Daphnia growth on microcystin-producing and microcystin-free Microcystis aeruginosa in different mixtures with the green alga Scenedesmus obliquus. Limnol. Oceanogr. 48, 2214–2220. doi: 10.4319/lo.2003.48.6.2214
Martin-Creuzburg, D., Oexle, S., and Wacker, A. (2014). Thresholds for sterol-limited growth of Daphnia magna: a comparative approach using 10 different sterols. J. Chem. Ecol. 40, 1039–1050. doi: 10.1007/s10886-014-0486-1
Martin-Creuzburg, D., Von Elert, E., and Hoffmann, K. H. (2008). Nutritional constraints at the cyanobacteria-Daphnia magna interface: the role of sterols. Limnol. Oceanogr. 53, 456–468. doi: 10.4319/lo.2008.53.2.0456
Martin-Creuzburg, D., Wacker, A., and Von Elert, E. (2005). Life history consequences of sterol availability in the aquatic keystone species Daphnia. Oecologia 144, 362–372. doi: 10.1007/s00442-005-0090-8
Micheletti, S., Schanz, F., and Walsby, A. E. (1998). The daily integral of photosynthesis by Planktothrix rubescens during summer stratification and autumnal mixing in Lake Zürich. New Phytol. 139, 233–246. doi: 10.1046/j.1469-8137.1998.00196.x
Müller-Navarra, D. C., Brett, M. T., Liston, A. M., and Goldman, C. R. (2000). A highly unsaturated fatty acid predicts carbon transfer between primary producers and consumers. Nature 403, 74–77. doi: 10.1038/47469
Oberhaus, L., Gélinas, M., Pinel-Alloul, B., Ghadouani, A., and Humbert, J. F. (2007). Grazing of two toxic Planktothrix species by Daphnia pulicaria: potential for bloom control and transfer os microcystins. J. Plankton Res. 29, 827–838. doi: 10.1093/plankt/fbm062
Perga, M. E., Domaizon, I., Guillard, J., Hamelet, V., and Anneville, O. (2013). Are cyanobacterial blooms trophic dead ends? Oecologia. 172, 551–562. doi: 10.1007/s00442-012-2519-1
Pflugmacher, S., Wiegand, C., Oberemm, A., Beattie, K. A., Krause, E., Codd, G. A., et al. (1998). Identification of an enzymatically formed glutathione conjugate of the cyanobacterial hepatotoxin microcystin-LR: the first step of detoxification. Biochim. Biophys. Acta 1425, 527–533. doi: 10.1016/S0304-4165(98)00107-X
Pijanowska, J., Weider, L. J., and Lampert, W. (1993). Predator-mediated genotypic shifts in a prey population: experimental evidence. Oecologia 96, 40–42. doi: 10.1007/BF00318028
Posch, T., Köster, O., Salcher, M. M., and Pernthaler, J. (2012). Harmful filamentous cyanobacteria favoured by reduced water turnover with lake warming. Nat. Clim. Chang 2:809–13. doi: 10.1038/nclimate1581
Rohrlack, T., Christoffersen, K., and Friberg-Jensen, U. (2005). Frequency of inhibitors of daphnid trypsin in the widely distributed cyanobacterial genus Planktothrix. Environ. Microbiol. 7, 1667–1669. doi: 10.1111/j.1462-2920.2005.00877.x
Rohrlack, T., Dittmann, E., Börner, T., and Christoffersen, K. (2001). Effects of cell-bound microcystins on survival and feeding of Daphnia spp. Appl. Environ. Microbiol. 67, 3523–3529. doi: 10.1128/AEM.67.8.3523-3529.2001
Rohrlack, T., Dittmann, E., Henning, M., Boerner, T., and Kohl, J. (1999). Role of microcystins in poisoning and food ingestion inhibition of Daphnia galeata caused by the cyanobacterium Microcystis aeruginosa. Appl. Environ. Microbiol. 65, 737–739. doi: 10.1128/AEM.65.2.737-739.1999
Rohrlack, T., Skulberg, R., and Skulberg, O. M. (2009). Distribution of oligopeptide chemotypes of the cyanobacterium Planktothrix and their persistance in selected lakes in Fennoscandia. J. Phycol. 45, 1259–1265. doi: 10.1111/j.1529-8817.2009.00757.x
Rücker, J., Wiedner, C., and Zippel, P. (1997). “Factors controlling the dominance of Planktothrix agardhii and Limnothrix redekei in eutrophic shallow lakes,” in Shallow Lakes '95, eds L. Kufel, A. Prejs, and J. I. Rybak (Dordrecht: Springer), 107–115.
Sadler, T. (2014). Interaction of Daphnia Magna With Cyanobacteria: New Insights From Using High-Resolution LC-MS. (Ph.D. thesis), University of Cologne, Germany.
Sadler, T., and Von Elert, E. (2014a). Dietary exposure of Daphnia to microcystins: No in vivo relevance of biotransformation. Aquat. Toxicol. 150, 73–82. doi: 10.1016/j.aquatox.2014.02.017
Sadler, T., and Von Elert, E. (2014b). Physiological interaction of Daphnia and Microcystis with regard to cyanobacterial secondary metabolites. Aquat. Toxicol. 156, 96–105. doi: 10.1016/j.aquatox.2014.08.003
Salmaso, N., Morabito, G., Mosello, R., Garibaldi, L., Simona, M., Buzzi, F., et al. (2003). A synoptic study of phytoplankton in the deep lakes south of the Alps (lakes Garda, Iseo, Como, Lugano and Maggiore). J. Limnol. 62, 207–227. doi: 10.4081/jlimnol.2003.207
Schlotz, N., Pester, M., Freese, H. M., and Martin-Creuzburg, D. (2014). A dietary polyunsaturated fatty acid improves consumer performance during challenge with an opportunistic bacterial pathogen. FEMS Microbiol. Ecol. 90, 467–477. doi: 10.1111/1574-6941.12407
Schlotz, N., Sørensen, J. G., and Martin-Creuzburg, D. (2012). The potential of dietary polyunsaturated fatty acids to modulate eicosanoid synthesis and reproduction in Daphnia magna: a gene expression approach. Comp. Biochem. Physiol. Part A 162, 449–454. doi: 10.1016/j.cbpa.2012.05.004
Schreuder, H, Liesum, A., Lönze, P, Stump, H., Hoffmann, H., Schiell, M, et al. (2016). Isolation, co-crystallization and structure-based characterization of anabaenopeptins as highly potent inhibitors of activated thrombin activatable fibrinolysis inhibitor (TAFIa). Sci. Rep. 6:32958. doi: 10.1038/srep32958
Schwarzenberger, A., Courts, C., and Von Elert, E. (2009). Target gene approaches: gene expression in Daphnia magna exposed to predator-borne kairomones or to microcystin-producing and microcystin-free Microcystis aeruginosa. BMC Genomics 10:527. doi: 10.1186/1471-2164-10-527
Schwarzenberger, A., Hasselmann, M., and Von Elert, E. (2020). Positive selection of digestive proteases in Daphnia: a mechanism for local adaptation to cyanobacterial protease inhibitors. Mol. Ecol. 29, 912–919. doi: 10.1111/mec.15375
Schwarzenberger, A., Keith, N. R., Jackson, C. E., and Von Elert, E. (2017). Copy number variation of a protease gene of Daphnia: its role in population tolerance. J. Exp. Zool. Part A 327, 119–126. doi: 10.1002/jez.2077
Schwarzenberger, A., Kuster, C. J., and Von Elert, E. (2012). Molecular mechanisms of tolerance to cyanobacterial protease inhibitors revealed by clonal differences in Daphnia magna. Mol. Ecol. 12, 4898–4911. doi: 10.1111/j.1365-294X.2012.05753.x
Schwarzenberger, A., Sadler, T., Motameny, S., Ben-Khalifa, K., Frommolt, P., Altmüller, J., et al. (2014). Deciphering the genetic basis of microcystin tolerance. BMC Genomics 15:776. doi: 10.1186/1471-2164-15-776
Schwarzenberger, A., Sadler, T., and Von Elert, E. (2013). Effect of nutrient limitation of cyanobacteria on protease inhibitor production and fitness of Daphnia magna. J. Exp. Biol. 216, 3649–3655. doi: 10.1242/jeb.088849
Schwarzenberger, A., and Von Elert, E. (2013). Cyanobacterial protease inhibitors lead to maternal transfer of increased protease gene expression in Daphnia. Oecologia 172, 11–20. doi: 10.1007/s00442-012-2479-5
Schwarzenberger, A., Zitt, A., Kroth, P., Mueller, S., and Von Elert, E. (2010). Gene expression and activity of digestive proteases in Daphnia: effects of cyanobacterial protease inhibitors. BMC Physiol 10:6. doi: 10.1186/1472-6793-10-6
Vasiliou, V., Vasiliou, K., and Nebert, D. W. (2009). Human ATP-binding cassette (ABC) transporter family. Hum. Genomics 3:281. doi: 10.1186/1479-7364-3-3-281
Von Elert, E., and Jüttner, F. (1997). Phosphorus limitation not light controls the exudation of allelopathic compounds by Trichormus doliolum. Limnol. Oceanogr. 42, 1796–1802. doi: 10.4319/lo.1997.42.8.1796
Von Elert, E., Martin-Creuzburg, D., and Le Coz, J. R. (2003). Absence of sterols constrains carbon transfer between cyanobacteria and a freshwater herbivore (Daphnia galeata). Proc. Biol. Sci. 270, 1209–1214. doi: 10.1098/rspb.2003.2357
Von Elert, E., Zitt, A., and Schwarzenberger, A. (2012). Inducible tolerance in Daphnia magna to dietary protease inhibitors. J. Exp. Biol. 215, 2051–2059. doi: 10.1242/jeb.068742
Wiegand, C., Pflugmacher, S., Oberemm, A., Meems, N., Beattie, K. A., and Steinberg, C. E. W. (1999). Uptake and effects of microcystin-LR on detoxication enzymes of early life stages ofthe zebrafish (Danio rerio). Environ. Toxicol. 14, 89–95. doi: 10.1002/(SICI)1522-7278(199902)14:1<89::AID-TOX12>3.0.CO;2-7
Keywords: carboxypeptidase inhibitors, daphnia-cyanobacteria interactions, enzyme activity, gene expression, microcystins, sterol limitation, protease inhibitors, trypsin inhibitors
Citation: Schwarzenberger A, Kurmayer R and Martin-Creuzburg D (2020) Toward Disentangling the Multiple Nutritional Constraints Imposed by Planktothrix: The Significance of Harmful Secondary Metabolites and Sterol Limitation. Front. Microbiol. 11:586120. doi: 10.3389/fmicb.2020.586120
Received: 22 July 2020; Accepted: 15 September 2020;
Published: 21 October 2020.
Edited by:
Petra M. Visser, University of Amsterdam, NetherlandsReviewed by:
Stéphan Jacquet, Institut National de Recherche pour l'agriculture, l'alimentation et l'environnement (INRAE), FranceAssaf Sukenik, University of Haifa, Israel
Copyright © 2020 Schwarzenberger, Kurmayer and Martin-Creuzburg. This is an open-access article distributed under the terms of the Creative Commons Attribution License (CC BY). The use, distribution or reproduction in other forums is permitted, provided the original author(s) and the copyright owner(s) are credited and that the original publication in this journal is cited, in accordance with accepted academic practice. No use, distribution or reproduction is permitted which does not comply with these terms.
*Correspondence: Anke Schwarzenberger, YW5rZS5zY2h3YXJ6ZW5iZXJnZXJAdW5pLWtvbnN0YW56LmRl