- 1UR-EGP, Faculté des Sciences, Université Saint-Joseph de Beyrouth, Beirut, Lebanon
- 2Université Paris-Saclay, INRAE, AgroParisTech, Micalis Institute, Jouy-en-Josas, France
- 3Department of Pharmacy, Centre for Integrative Microbial Evolution (CIME), Faculty of Mathematics and Natural Sciences, University of Oslo, Oslo, Norway
Antimicrobial peptides (AMPs) are essential effectors of the host innate immune system and they represent promising molecules for the treatment of multidrug resistant microbes. A better understanding of microbial resistance to these defense peptides is thus prerequisite for the control of infectious diseases. Here, using a random mutagenesis approach, we identify the fliK gene, encoding an internal molecular ruler that controls flagella hook length, as an essential element for Bacillus thuringiensis resistance to AMPs in Drosophila. Unlike its parental strain, that is highly virulent to both wild-type and AMPs deficient mutant flies, the fliK deletion mutant is only lethal to the latter’s. In agreement with its conserved function, the fliK mutant is non-flagellated and exhibits highly compromised motility. However, comparative analysis of the fliK mutant phenotype to that of a fla mutant, in which the genes encoding flagella proteins are interrupted, indicate that B. thuringiensis FliK-dependent resistance to AMPs is independent of flagella assembly. As a whole, our results identify FliK as an essential determinant for B. thuringiensis virulence in Drosophila and provide new insights on the mechanisms underlying bacteria resistance to AMPs.
Introduction
The continuous emergence of new infectious agents, together with the worrying rise in antibiotic resistance in pathogens, constitutes a threat to human health with predictions that these will account for 20% of deaths over the coming decades (ECDC/EMEA, 2009). Indeed, in the last 60 years, no fewer than 335 new infectious diseases of humans have emerged (Jones et al., 2008) and 30% of all emerging infections were comprised of pathogens transmitted through food (Kuchenmuller et al., 2009). Seventy percent of germs responsible for infections in hospitals are resistant to at least one class of antibiotics and the emergence of strains resistant to several classes of antibiotics is beginning to pose serious therapeutic problems (Levy and Marshall, 2004). These data highlight the urgent need to implement new therapeutic strategies and develop new classes of antimicrobial agents to control infections. In this perspective, the discovery in the 90s of antimicrobial peptides (AMPs) in animals as part of their innate immune defenses has paved the way for a new and very broad field of research. AMPs are small, cationic, usually amphipathic peptides with a broad spectrum of activity against Gram-positive and Gram-negative bacteria, fungi, viruses, and protozoa (Imler and Bulet, 2005; Guani-Guerra et al., 2010; Marxer et al., 2016; Rolff and Schmid-Hempel, 2016; Haney et al., 2017; Hanson and Lemaitre, 2020). Ex-vivo studies have shown that their potent antimicrobial activity is exhibited mostly by perturbing negatively charged microbial membranes, although some AMPs can also act at intracellular targets preventing the synthesis of essential elements for biological functioning and the survival of microorganisms (Park et al., 1998; Kragol et al., 2001; Brogden, 2005; Rahnamaeian et al., 2015; Scocchi et al., 2016; Yang et al., 2019). Therefore, AMPs constitute a promising alternative therapeutic option that should make it possible to provide new classes of molecules with a broad spectrum of antimicrobial activity to possibly minimize or delay the onset of resistance (Andres and Dimarcq, 2004; Zaiou, 2007). However, if AMPs are taken out of their natural environment and used as antimicrobial agents in a conventional clinical setting, they could exert a strong and continuous selection pressure on the bacterial population leading to rapid selection of resistant strains. Such a scenario could have extremely serious consequences if it resulted in the development of cross-resistance with innate human AMPs (Bell and Gouyon, 2003; Perron et al., 2006). Therefore, an accurate knowledge of the mechanisms of resistance to these compounds, and a better understanding of the environmental constraints encountered by pathogenic bacteria during the infectious process, are prerequisites to their intensive use.
The Bacillus cereus group includes a growing number of species which range from no hazardous to deadly dangerous (Carroll et al., 2020). To date 21 genomospecies have been described (Liu et al., 2017). These include B. anthracis, the infamous causing agent of the anthrax disease, the entomopathogen B. thuringiensis that is widely used as a biological control agent to combat insect pests of agriculture or vectors of diseases and B. cereus sensu stricto an opportunistic human pathogen which causes gastroenteritis and is now considered as the third most important cause of collective food poisoning incidents in Europe (EFSA, 2009). These Gram-positive bacteria are ubiquitous in nature and their spores are highly resistant to common sterilizing techniques which make them of a high concern to the food industry. The ability of B. cereus group members to form biofilms adds complexity and persistence to bacteria on industrial and biomedical devices which are currently recognized as defining sources for nosocomial infections (Stenfors Arnesen et al., 2007; Kuroki et al., 2009; Bottone, 2010; Glasset et al., 2018). Although B. cereus food-borne poisoning is generally mild, it is capable of causing serious gastrointestinal diseases in humans, such as bloody diarrhea and emetic poisoning, eventually leading to some fatal cases (Mahler et al., 1997; Lund et al., 2000; Dierick et al., 2005), especially in immunocompromised patients and preterm neonates (Stenfors Arnesen et al., 2008). Most alarming is the fact that B. cereus is now also increasingly diagnosed as a cause of severe, frequently fatal, non-gastrointestinal infections such as: bacteremia (Hernaiz et al., 2003), osteomyelitis (Sliman et al., 1987), septicemia (Matsumoto et al., 2000), pneumonia (Gray et al., 1996; Miller et al., 1997), liver abscess (Latsios et al., 2003). More recently, a novel food pathogen, B. cytotoxicus (Lund et al., 2000; Fagerlund et al., 2007; Auger et al., 2008; Lapidus et al., 2008; Guinebretiere et al., 2013), as well as B. cereus “anthracis-like” strains were also characterized (Hoffmaster et al., 2004; Klee et al., 2010), showing emergence of new pathotypes in the group. Although distinctive pathogenic features of the B. cereus group species are linked to plasmid-born genes specifying their susceptible hosts, these bacteria share a common genetic background with several genes associated with the expression of their virulence phenotypes (Ehling-Schulz et al., 2019). Indeed, the opportunistic properties of B. cereus and B. thuringiensis have been explored in animal models underlying the requirement of common genetic determinant in the pathogenic properties of these bacteria (Salamitou et al., 2000; Gohar et al., 2008; Ramarao et al., 2012; Ramarao and Sanchis, 2013). In agreement with this hypothesis, some B. thuringiensis strains have been reported to cause infections in immunocompromised patients (Green et al., 1990; Damgaard et al., 1997; Hernandez et al., 1998; Helgason et al., 2000; Kuroki et al., 2009). These data emphasize the necessity of an in-depth investigation of the common genetic determinants involved in the determination of the pathogenic potential of B. cereus species.
Previous studies have reported the prominent feature of B. cereus and B. thuringiensis to rapidly develop in insect models upon septic injury infection (Stephens, 1952; Kushner and Heimpel, 1957; Salamitou et al., 2000; Buisson et al., 2019). These data presumably underlined the potent capacity of these bacteria to overcome host innate immune defenses. In agreement with these findings, we have previously reported that B. cereus is highly resistant to AMPs (Abi Khattar et al., 2009). This resistance relies on the D-alanyl esterification of its cell wall teichoic acids (TAs) through the activity of the genes products of the dltXABCD operon that is highly conserved among Gram-positive bacteria (Heaton and Neuhaus, 1992; Perego et al., 1995; Peschel et al., 1999; Abachin et al., 2002; Kristian et al., 2005; Abi Khattar et al., 2009; Kamar et al., 2017). The D-alanylation of TAs reduces their net negative charge, thus lowering the attraction of cationic AMPs to the bacterial cell wall (Kristian et al., 2003, 2005; Fabretti et al., 2006; Kovacs et al., 2006; Perea Velez et al., 2007). We have further explored the relevance of B. cereus resistance to AMPs in the context of its exposure to the host immune defenses using the Drosophila model. Indeed, in insects as in mammals, sensing of Microbial Associated Molecular Patterns (MAMPs) by cognate host innate immune Pattern Recognition Receptors (PRRs) triggers a highly conserved immune response that is prerequisite to counter the infections (Janeway, 1989; Medzhitov, 2007). In Drosophila, sensing of Bacilli Diaminopimelic acid (DAP)-type peptidoglycan triggers the activation of the immune deficiency (IMD) pathway which controls the activation of the NF-κB transcription factor, Relish, thus driving the expression of several immune genes including those encoding AMPs (Leulier et al., 2003; Kaneko et al., 2004; Stenbak et al., 2004). Seven AMP families have been characterized in Drosophila (Imler and Bulet, 2005; Hanson and Lemaitre, 2020). Their highly induced expression upon microbial challenge, provides microbicidal concentrations that are far above the concentration necessary to kill bacteria (Hanson et al., 2019; Hanson and Lemaitre, 2020). Using a septic injury infection model, we have shown that B. thuringiensis is highly resistant to the Drosophila Relish-dependent systemic antimicrobial response. Remarkably, contrary to the wild-type (wt) bacterial strain, that is equally virulent to both wt and relish mutant flies, a B. thuringiensis Δdlt mutant only exhibited high pathogenicity in relish immunodeficient flies (Kamar et al., 2017).
Here, taking advantage of this Drosophila model of bacteremia which easily allows the discrimination between virulent and attenuated bacterial strains, together with the recent development of an AMP-deficient Drosophila strain (Hanson et al., 2019), we aimed at further exploring physiological and genetic factors that make B. cereus resistant to host AMPs in vivo. Using a random mutagenesis approach, we identified the fliK gene, which encodes a protein with a flagellar hook length control motif, as an essential determinant for B. thuringiensis resistance to AMPs and virulence in Drosophila. In agreement with its highly conserved function, the fliK mutant is non-flagellated and exhibits highly compromised motility. However, by combining both in vitro and in vivo analysis, we show that B. thuringiensis FliK-dependent resistance to AMPs is independent of the flagellar motility. As a whole, our results, provide new insights on the strategies developed by bacteria to overcome host innate immune defenses, in particular AMPs, which could be exploited for potential therapeutic approaches to decrease viability of pathogens.
Results
A Two-Step Screening Strategy Identifies Genes Required for the Resistance of Bt407 to Polymyxin B and to Innate Immune Defenses in Drosophila
In an attempt to identify new genes involved in the resistance of B. cereus to innate immune defenses, and more specifically to AMPs, we generated, by insertion and mobilization of a mini-Tn10 transposon, a random mutagenesis library of the B. thuringiensis Bt407 strain (Lereclus et al., 1989) that we screened in a two-step strategy as described in the following. In the first step of the screen, Bt407mini–Tn10 insertion mutants were screened on LB-Agar plates supplemented with 200 μg/ml of polymyxin B in order to select clones with a growth delay comparable to that of the dlt operon mutant, Bt407ΔdltX (Kamar et al., 2017). Out of 3,200 tested clones, this primary screen allowed the selection of 10 Bt407mini–Tn10 insertion mutants with a significant growth delay on polymyxin B as compared to the wt strain. The phenotype of each of the selected clones was confirmed by three independent experiments. To exclude the possibility that the growth delay phenotype observed in the selected Bt407mini–Tn10 insertion mutants was due to a growth deficiency rather than an increased sensitivity to polymyxin B, their growth was assessed in LB broth medium at 30°C. All mutants grew in the same way as the Bt407 strain in the absence of polymyxin B. In contrast, their growth was affected during exponential phase when the culture medium was supplemented by polymyxin B at 200 μg/ml. Mapping of the transposon insertion sites (see section “Materials and Methods”) revealed that, in all mutants, the transposon was inserted in an open reading frame (ORF) flanked by 9 bp duplication at each end, which is a mini-Tn10 insertion characteristic. The 10 insertions mapped to four distinct ORFs that were identified and designated PSC1 to PSC4 (for Polymyxin B Sensitive Clone). The insertions in the identified ORFs hit the following genes, respectively, BTB_c16930 encoding a hypothetical protein with a flagellar hook length control protein motif, the BTB_c20220 gene encoding the cell wall-associated hydrolase LytF1, the gene BTB_c28480 encoding the NprM bacillolysin and the BTB_c32720 gene encoding a hypothetical protein with a ß-lactamase motif characteristic of penicillin-binding proteins (Table 1).
To address the primary objective of this study, which is the identification of genes involved in the resistance to innate immune defenses, in the second step of the screen, we evaluated the virulence of the four PSC insertion mutants upon a septic injury infection in adult Drosophila. This infection model, previously established on the wt and the dlt mutant strains of Bt407, allows the discrimination between virulent and attenuated strains (Kamar et al., 2017; Figure 1). Thus, we compared the survivals of wt flies infected with the PSC clones to those of flies infected with the wt and the dlt mutant strains of Bt407. The results indicate that, similarly to the Bt407Δdlt mutant, the PSC1 and PSC4 clones had an attenuated virulence when compared to the parental Bt407 strain (Figure 1). Overall, these results allowed the selection of two candidate genes required for the resistance of Bt407 to polymyxin B in culture medium and to host defenses in Drosophila. Based on gene mapping and homology sequence analysis, the candidate genes interrupted by the mini-Tn10 transposon in the PSC1 and PSC4 clones are likely involved in flagellar and cell wall assembly, respectively. Several studies have associated the flagellum to different aspects of bacterial virulence (Duan et al., 2013). In Campylobacter jejuni, locomotion and resistance to AMPs have also been correlated (Cullen and Trent, 2010; Cullen et al., 2012). These traits were separately attributed to the dual function of a phosphoethanolamine transferase (EptC) which modifies both the flagellar rod protein FlgG and the lipid A domain of lipooligosaccharide, respectively (Cullen et al., 2012). However, a direct link between flagella and the resistance of bacteria to AMPs remains largely unexplored. Therefore, in this study we aimed at investigating the role of flagella in the resistance to AMPs with a particular focus on the role of the BTB_c16930 gene.
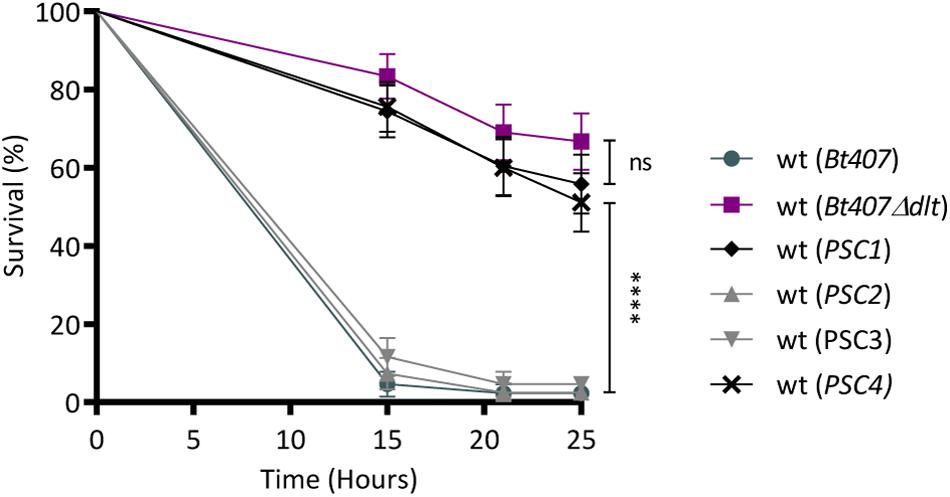
Figure 1. Identification of novel genes involved in Bt407 virulence in Drosophila. Survival of wild-type flies (wt) to an infection with Bt407, Bt407Δdlt or the four Bt mutants preselected as Polymyxin B Sensitive Clones (PSC). Data are representative of three independent experiments (mean ± SD). Statistical tests were performed using Log Rank test (ns: p > 0.05, ****p < 0.0001).
The fliK Gene Is Required for Bt407 Flagellar Filament Assembly and Function
The bacterial flagellum is a complex organelle that projects from the inside-out of the cell. It comprises three functional parts: (1) the basal body, which is embedded in the cell envelope, and that comprises a stator, a rotor, and an axle-like rod that extends through the cell wall peptidoglycan and houses a type III secretion system that exports the more distal components of the organelle, (2) the hook, which constitutes a flexible universal joint connecting the basal body to the last part of the flagellum, and (3) the helical filament which acts as a propeller (Guttenplan and Kearns, 2013; Evans et al., 2014; Mukherjee and Kearns, 2014). According to the KEGG database (Kyoto Encyclopedia of Genes and Genomes), the BTB_c16930 gene encoding a hypothetical protein is the ortholog of fliK in B. thuringiensis HD1011 strain with 91.1% of protein sequence identity. Functional analysis performed in Bacillus subtilis and Gram-negative bacteria have shown that fliK encodes an internal molecular ruler which controls hook length (Silverman and Simon, 1972; Patterson-Delafield et al., 1973; Minamino et al., 1999b, 2004; Journet et al., 2003; Shibata et al., 2007; Chevance and Hughes, 2008; Erhardt et al., 2010; Courtney et al., 2012). Furthermore, as the secretion of flagellar components is sequential, FliK was also shown to be required for switching the specificity of the export machinery from rod-hook substrates to flagellin, the protein products of the fla genes in Bt407, thereby promoting extracellular filament assembly (Moriya et al., 2006; Minamino et al., 2009; Houry et al., 2010; Erhardt et al., 2011; Mizuno et al., 2011; Hughes, 2012a,b; Evans et al., 2013). This is achieved once the hook has reached its mature length.
In order to confirm the role of the fliK gene in the assembly of flagella in Bt407, and to avoid any possible effect arising from the mini-Tn10 mobilization or unrelated secondary mutation, we constructed a de novo Bt407ΔfliK deletion mutant harboring a precise deletion of the BTB_c16930 gene (Figure 2A) and its complemented strain Bt407ΔfliKΩfliK. Using scanning atomic force microscopy (AFM), we examined the presence of flagella on Bt407ΔfliK. As shown in Figure 2B, unlike the wt Bt407 strain, the Bt407ΔfliK deletion mutant completely failed to produce detectable flagellar filaments as shown in amplitude images (Figure 2B). No loose fragments of flagella were observed in the mutant cellular preparation as shown in amplitude images. In agreement with these findings, we showed that, similarly to the Bt407Δfla mutant (Houry et al., 2010), in which the genes encoding flagellar proteins had been interrupted, the Bt407ΔfliK mutant shows a highly compromised swimming motility as compared to the wt Bt407 strain (Figure 2C). Both flagellar assembly and swimming motility are restored by the functional complementation of the Bt407ΔfliK deletion mutant by the fliK ORF (Figures 2B,C). Altogether, these results confirm the essential function of the predicted FliK protein in the flagellar assembly and associated motility of Bt407. Since flagella mediated motility was shown to promote biofilm formation for several bacterial species including B. thuringiensis (O’Toole and Kolter, 1998; Pratt and Kolter, 1998; Klausen et al., 2003; Wood et al., 2006; Lemon et al., 2008; Houry et al., 2010, 2012; Guttenplan and Kearns, 2013; Fagerlund et al., 2014; Majed et al., 2016) and since biofilms are known to enhance bacterial resistance to antimicrobial effectors (Costerton, 2004; Hall-Stoodley and Stoodley, 2009), we sought to evaluate the capacity of the Bt407ΔfliK mutant to form a biofilm at the air-liquid interface in a glass tube. Quantification of biofilm biomasses presented in Figure 2D clearly indicates that, similarly to the Bt407Δfla mutant, biofilm formation is highly compromised for the Bt407ΔfliK mutant. This phenotype was reverted in the complemented Bt407ΔfliKΩfliK strain (Figure 2D). This result underlies the requirement of the FliK protein for flagellar associated biofilm formation in Bt407.
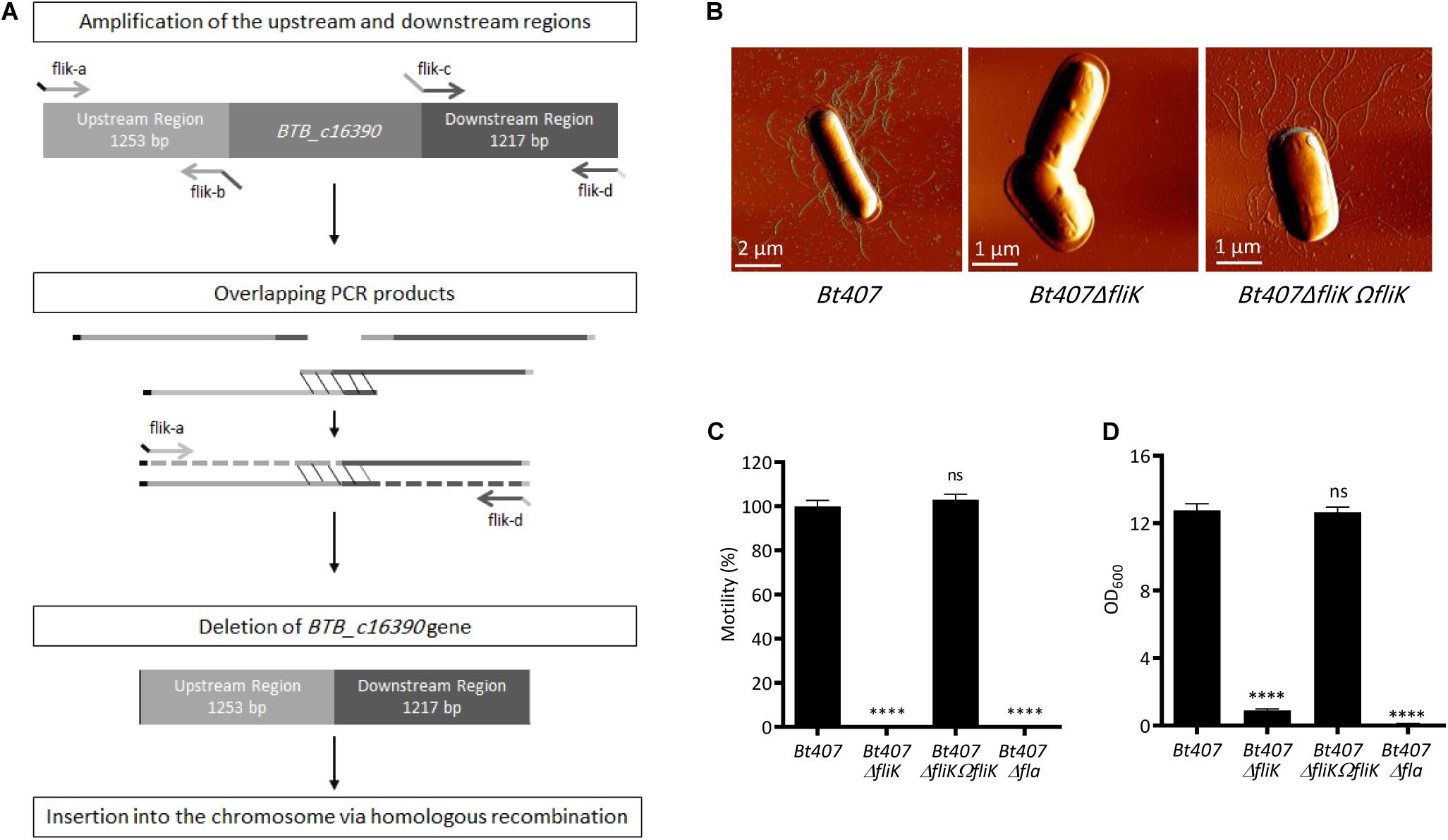
Figure 2. FliK is required for Bt407 flagellar assembly. (A) Schematic diagram showing the construction of an in-frame deletion of the BTB_c16930 gene by Splicing by Overlap Extension (SOE). (B) Scanning Atomic Force Microscopy (AFM) of exponential growth phase cells of Bt407, Bt407ΔfliK, and Bt407ΔfliKΩfliK. (C) Bacterial motility on LB soft agar (0.25%) medium. Motility of the Bt407 strain was set to 100%, and values obtained with other strains were set as fold relative to this value. (D) Total biofilm biomass at the air-liquid interface in glass tubes, following 48 h incubation at 30°C. Data obtained from three independent experiments are combined in single value (mean ± SD). Statistical tests were performed using the Mann-Whitney test within Prism software (ns: p > 0.05; ****p < 0.0001).
Beyond Its Role in Flagellar Assembly, FliK Is Essential for the Resistance of Bacillus thuringiensis to Host Anti Microbial Peptides
In order to confirm that the enhanced sensitivity of the PSC1 clone to polymyxin B is due to the compromised function of the fliK gene, we assessed the susceptibility of the Bt407, Bt407ΔfliK, and Bt407ΔfliKΩfliK strains to polymyxin B by determining their half inhibitory concentration (IC50). As presented in Figure 3A, the Bt407ΔfliK mutant is highly sensitive to polymyxin B with an IC50 fourfold lower than that of Bt407 and Bt407ΔfliKΩfliK strains (IC50s of 110, 402, and 425 μg/ml, respectively) (Figure 3A). To check whether the increased sensitivity to polymyxin B observed in Bt407ΔfliK was due to the mutation of the fliK gene or more generally to a flagellar filament production deficiency, we henceforth included in our experiment the Bt407Δfla mutant (Houry et al., 2010). The results indicate that, the growth of the Bt407Δfla mutant is comparable to that of the parental Bt407 strain with an IC50 of 385 μg/ml for polymyxin B in vitro (Figure 3A).
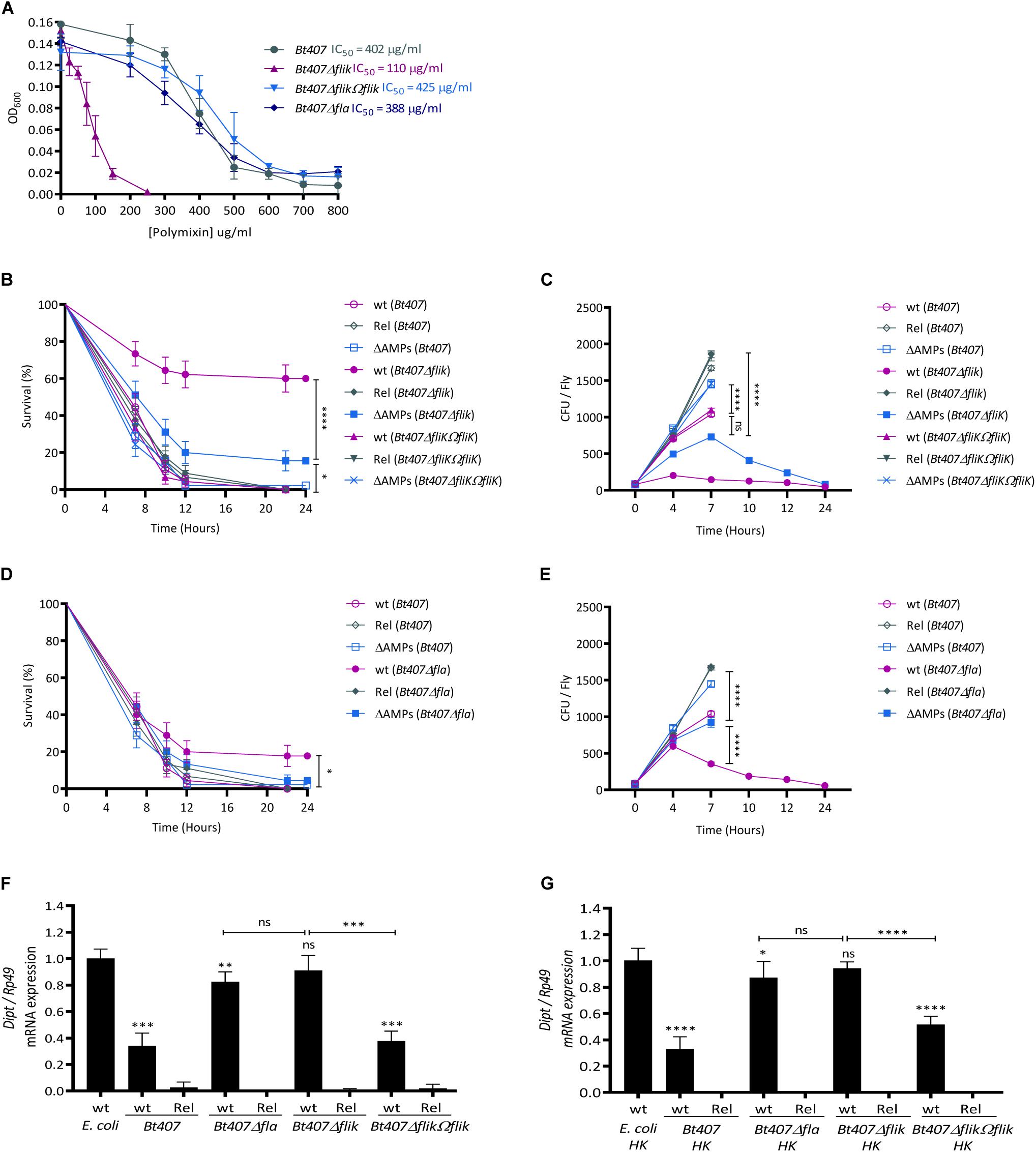
Figure 3. FliK confers Bt407 resistance to Drosophila antimicrobial peptides in a flagella independent manner. (A) The half inhibitory concentration (IC50) of Bt407, Bt407ΔfliK, Bt407ΔfliKΩfliK, and Bt407Δfla. The bacterial growth was scored 6 h post incubation at 30°C in LB medium supplemented with increased concentrations of polymyxin B. (B,D) Survival of adult wild-type (wt), relish (Rel) and antimicrobial peptides (ΔAMPs) mutant flies to an infection with Bt407 (B,D), Bt407ΔfliK (B), Bt407ΔfliKΩfliK (B) or Bt407Δfla (D). (C,E) Internal bacterial loads retrieved from adult flies infected with Bt407 (C,E) Bt407ΔfliK (C), Bt407ΔfliKΩfliK (C), or Bt407Δfla (E). The Colony Forming Unit (CFU) counting was performed only on surviving flies. (F,G) Relative expression of the Diptericin (Dipt) transcripts in wild-type (wt) or relish (Rel) mutant flies induced by living (F) or heat-killed HK (G) Bt407, Bt407ΔfliK, Bt407ΔfliKΩfliK, or Bt407Δfla. Transcripts expression was measured by RT-qPCR in total RNA extracts 4 h upon the induction. Ribosomal protein 49 (Rp49) transcript was used as reference gene. Transcripts levels are compared to that triggered in wt flies infected by living (F) or heat-killed HK (G) E. coli as a control. Data obtained from three independent experiments are combined in single value (mean ± SD). Statistical tests were performed using the Log Rank test for the survival assays and Mann-Whitney test for the CFU counting and the Dipt expression evaluation within Prism software (ns: p > 0.05; ∗0.01 < p < 0.05; ∗∗0.001 < p < 0.01; ∗∗∗0.0001 < p < 0.001; ****p < 0.0001).
We then sought to check whether the attenuated virulence of the Bt407ΔfliK was also due to the FliK-dependent resistance of Bt407 to host AMPs. Therefore, we assessed the survival of wt and ΔAMPs mutant flies, which are devoid of 10 out of the 14 known Drosophila immune inducible AMPs encoding genes, to an infection by Bt407, Bt407ΔfliK, Bt407ΔfliKΩfliK, or Bt407Δfla (Hanson et al., 2019). We also included in the analysis relish mutants which are unable to mount an IMD-dependent systemic humoral response upon the infection of flies by Bacillus spp. (Leulier et al., 2003). As shown in Figure 3B, unlike the wt Bt407 strain, which is highly resistant to the Relish-dependent AMPs response in flies thus killing wt, ΔAMPs and relish mutants with the same kinetics (approximately 100% of lethality 12 h post-infection), the fliK mutant displays an attenuated virulence in immunocompetent flies (37% of lethality at 12 h post-infection) while remaining fully virulent in immunodeficient flies sharing the same lethality kinetics with its parental strain (Figure 3B). The survival of ΔAMPs mutant flies largely mirrored that of relish mutants thus attesting of the sensitivity of this mutant to AMPs effectors among all Relish-immune induced genes. The slight difference between the survival of relish and ΔAMPs mutants could be explained by the expression in the latter of the four remaining genes encoding antibacterial Cecropins which are known to be induced by the IMD pathway (Leulier et al., 2000). The sensitivity of the Bt407ΔfliK mutant to AMPs is further attested by the quantification of bacterial loads in the hemolymph of ΔAMPs mutants as compared to wt flies (Figure 3C). Indeed, whereas Bt407ΔfliK is unable to grow in wt flies, its growth in ΔAMPs mutants is equivalent to that of the parental Bt407 strain in wt flies up to 7 h post infection (Figure 3C). Passing this time point, the growth of Bt407ΔfliK in the hemolymph of ΔAMPs flies is likely compromised by the accumulated expression of Cecropins. This hypothesis is further supported by its accentuated growth in the hemolymph of relish mutants which are devoid of all IMD-dependent AMPs, and the concomitant lethality of these flies to the infection. These phenotypes are strictly dependent on the activity of FliK as revealed by the virulence phenotype and the growth of the Bt407ΔfliKΩfliK complemented strain in both wt and ΔAMPs mutant (Figures 3B,C). Interestingly, in agreement with its resistance to polymyxin B phenotype, the Bt407Δfla mutant showed a virulence phenotype that contrasts with that of the Bt407ΔfliK. In particular, the Bt407Δfla mutant remained highly virulent with wt flies dying most similarly to ΔAMPs and relish mutants up 12 h post infection (Figure 3D). Comparative analysis of the growth of bacterial strains in the hemolymph of these flies, shows that unlike Bt407ΔfliK, Bt407Δfla grows similarly to the wt strain up to 4 h after the infection (Figure 3E). This result correlates with an identical lethality rate of the flies 7 h after the infection. Passing this time point, a slightly attenuated virulence is observed with only 20% of wt flies surviving 12 h post-infection (Figure 3D). This decreased virulence of the Bt407Δfla is correlated with a decrease of its growth in the hemolymph of wt flies as compared to the parental strain (Figures 3D,E). Thus, compared to the Bt407ΔfliK mutant that is completely compromised by the relish-dependent immune response, a Bt407Δfla mutant is only sensitive to this response at later time points of the infection when the bacterial virulence phenotype is already prominently expressed (compare bacterial growth in wt and relish mutant flies). These data suggest that the additive expression of Relish-dependent AMPs encoding genes might account for the slight, although significant reduced virulence of Bt407Δfla in wt as compared to relish mutants’ flies. Interestingly, the early growth of Bt407Δfla is similar to that of the parental Bt407 strain in the hemolymph of wt flies. Thus, it is tempting to speculate that the continuing growth of the wt Bt407 strain, passing 4 h of the infection, would account for its accentuated virulence on wt flies as compared to that of Bt407Δfla (Figures 3D,E). Interestingly, the growth of the parental Bt407 strain also seems to be slowed down by the Relish-dependent immune response 7 h after the infection (Figures 3C,E). However, the resistance of Bt407 to this response is manifested by its continuous albeit attenuated growth but also by its fully virulent phenotype on wt, ΔAMPs and relish mutant flies (Figures 3B–E). Although these results provide clear evidence of a role of FliK in the resistance of B. thuringiensis to the Drosophila systemic AMPs Relish-dependent humoral response, this hypothesis is challenged by the possibility that the wt and mutant bacteria strains might induce a different immune response in the infected flies. In order to check for this possibility, we compared the immune response induced by the different bacterial strains, by quantifying the expression of Diptericin, an AMP-encoding gene, which is conventionally used as a readout of the Relish-dependent immune response in Drosophila. As shown in Figure 3F, Bt407 induces a mild-immune response in Drosophila compared to the Gram-negative bacterium Escherichia coli that is commonly used as an IMD pathway inducer (Figure 3F). Both Bt407Δfla and Bt407ΔfliK mutants trigger an enhanced immune response as compared to the parental or Bt407ΔfliKΩfliK strains. The reduced immune response triggered by Bt407 is not due to an inhibitory mechanism depending on the activity of the flagella as the immune induced response profile remained unchanged whether the flies were treated with living or heat-killed bacteria (Figures 3F,G). Thus, these results rule out the possibility that the different virulence phenotypes of Bt407ΔfliK (37% of lethality) and Bt407Δfla (80% of lethality) are due to a more prominent induction of immune effector genes in the host by the former. However, these data could potentially explain the slightly reduced virulence of Bt407Δfla (20% of survivors) compared to that of the wt Bt407 strain (0% survivors) at later timepoints of the infection where the higher and cumulative production of immune effector molecules might exert an additive effect on the bacteria thus limiting their growth in the hemolymph of the infected flies. Altogether, our results so far confirm a role of FliK in the resistance of B. thuringiensis to polymyxin B in vitro and to the Drosophila AMPs in vivo independently of its function in the establishment of flagella.
Discussion
AMPs are produced by virtually all living organisms and their widespread role in innate immune defense has been largely recognized. They allow unicellular organisms to successfully compete with other organisms sharing their habitats and constitute key effectors of the innate immune system in metazoans (Schmitt et al., 2016; Ageitos et al., 2017). By acting at the frontline of host defenses, these microbicidal molecules play an essential role in limiting the infections. The development of resistance mechanisms to this immune arsenal is thus considered as a major virulence phenotype providing successful human pathogens the potential to produce serious invasive infections (Koprivnjak and Peschel, 2011; Cole and Nizet, 2016). Several studies have shown that bacterial pathogens have evolved different resistance mechanisms to AMPs, mainly by surface charge modification (Peschel et al., 2001; Thedieck et al., 2006; Samant et al., 2009; Nawrocki et al., 2014; Kamar et al., 2017). Here, we have combined in vitro and in vivo approaches for the identification of novel genes required for the resistance of B. thuringiensis to cationic AMPs. Screening of 3,200 clones of a random mini-T10 insertional mutagenesis library allowed the selection of four clones with a compromised resistance to polymyxin B in vitro. Sequence analysis of the insertion sites of the transposon showed that these proteins were mainly secreted, or cell wall proteins associated with functional aspects of the cell wall and cell envelope. Of these mutants, only two exhibited a reduced virulence compared to the Bt407 parental strain in a systemic infection model in Drosophila. These results emphasize the existence of various AMP resistance mechanisms in B. thuringiensis. These are likely to support the ubiquitous nature of this bacterium that is highly spread in the environment. These results also advance the importance of using in vivo infection models for the evaluation of the significance to pathogenesis of genetic variants that impact bacterial resistance to AMPs while confronted to the complex immune defenses in eukaryotic hosts (Bauer and Shafer, 2015).
In this study, we focused on one mutant that has a transposon insertion in the gene annotated as fliK. Phenotypic characterization of a precise Bt407ΔfliK deletion mutant allowed us to confirm that the fliK gene is a defining element of B. thuringiensis virulence in Drosophila with a pleiotropic phenotype. Indeed, unlike its parental strain, the Bt407ΔfliK mutant is non-flagellated and exhibits compromised motility and biofilm formation. These data are in agreement with the conserved essential function of fliK in flagellar assembly that was previously described for several bacterial species (Silverman and Simon, 1972; Patterson-Delafield et al., 1973; Journet et al., 2003; Minamino et al., 2004; Shibata et al., 2007; Chevance and Hughes, 2008; Erhardt et al., 2010; Courtney et al., 2012). The novelty of this report relies on the demonstration that the reduced pathogenicity of the Bt407ΔfliK mutant is largely due to its high sensitivity to AMPs independently of its role in flagellar assembly. Indeed, comparative analysis reveals striking difference between the phenotypes of Bt407ΔfliK and Bt407Δfla mutants in terms of virulence in the Drosophila systemic infection model while both mutants share similar traits in terms of absence of flagella, reduced motility and biofilm formation. The highly attenuated virulence of Bt407ΔfliK compared to that of Bt407Δfla is nonetheless associated with a marked enhanced susceptibility of the former (and not of the latter) to AMPs both in vitro and in vivo. In particular, our data show that Flik is essential for the resistance of wt Bt407 strain to AMPs thus providing it with the advantage of a promoted growth from the early time of the infection. Indeed, timescale analysis indicates that the induced AMP humoral response efficiently inhibits the growth of Bt407ΔfliK in the hemolymph of infected flies but is unable to contain the early growth of the Bt407Δfla mutant which remained comparable to that of the wt Bt407 strain. Although the induced AMP response partially contains the growth of Bt407Δfla in the hemolymph of wt flies during the progress of the infection, the early bacterial growth is sufficient to induce significant lethality of the flies in a manner very similar to those infected by the wt Bt407 strain (Figures 3D,E). These results highlight the essential role of the FliK-dependent resistance to host AMPs in the virulence of Bt407 in the Drosophila model, independently of its role in flagellar assembly and motility. Nevertheless, unsuccessful flagellar assembly seems to influence the sensing of bacteria by the host immune system. Indeed, our results show that Bt407 induces a mild AMP-humoral response to which it is highly resistant. Surprisingly, this response is significantly enhanced and to the same level in flies infected by Bt407Δfla or Bt407ΔfliK (Figure 3F). These results suggest that the absence of flagella makes Bt407 more vulnerable to immune sensing in Drosophila. We do not presently have a clear understanding of how this is performed. However, as DAP-type PGN is known to be the major trigger of the immune response upon Bacillus spp. infection in Drosophila (Leulier et al., 2003), three scenarios have been proposed for their sensing by cognate host receptors: (1) accessibility of the receptor to cell wall PGN; (2) the release of PGN fragments through the activity of enzymes produced by bacteria, or by (3) host effector molecules such as AMPs or lysozyme (Chaput and Boneca, 2007; Humann and Lenz, 2009; Atilano et al., 2011; Vaz et al., 2019). We have so far verified whether PGN is already further released from the mutant Bt407Δfla or Bt407ΔfliK bacteria as compared to the wt strain by dosing the IMD response triggered in flies injected with supernatant of bacterial overnight cultures. The results presented in Supplementary Figure 1A. A clearly indicate that the supernatant of both Bt407ΔfliK and Bt407Δfla are more immunostimulatory than that of the wt or Bt407ΔfliKΩfliK complemented strains. This enhanced immunostimulatory effect is not due to an enhanced autolysis of the mutant strains (Supplementary Figure 1B). These results clearly suggest that the absence of flagella, somehow, perturbates the cell wall integrity thus resulting in an enhanced release of immunostimulatoty PGN fragments (Supplementary Figure 1A). The partial controlled growth of Bt407Δfla by the AMP response it induces in the infected flies could be explained by the enhanced expression of these immune effectors by the host immune response. Nonetheless, we cannot exclude the possibility that a flagellar dependent mechanism would provide successfully growing bacteria an accentuated resistance to the accumulated production of AMPs in vivo.
Although our data put forward FliK as an essential element for the enhanced resistance of B. thuringiensis to AMPs, the molecular mechanism underlying this particular function remains to be clarified. The flagellar apparatus is a complex self-assembling nanomachine that contains its own type III protein export apparatus that has various substrate specificities. During flagellar morphogenesis, the export apparatus switches substrate specificity from the rod-/hook-type substrate to the filament-type substrate (Minamino et al., 1999a,b). In B. subtilis, this switch in export specificity also results in the export of the anti-sigma factor FlgM that inhibits late-class flagellar gene expression by sequestering a flagellar-specific sigma factor (Gillen and Hughes, 1991; Kutsukake and Iino, 1994; Daughdrill et al., 1997; Karlinsey et al., 2000a,b; Kalir et al., 2001; Barembruch and Hengge, 2007). Secreted FlgM is further degraded extracellularly by the proteases Epr and WprA (Calvo and Kearns, 2015). It is currently well known that FliK plays an essential role in switching the substrate specificity of the flagellar export apparatus by modifying the export gate proteins FlhA and FlhB that control flagellar protein export (Aizawa, 2012; Hughes, 2012a,b; Evans et al., 2014; Minamino, 2018; Minamino et al., 2019; Kinoshita et al., 2020). This modification allows specificity switch for the secretion among others of filament class proteins (Ghelardi et al., 2002; Bouillaut et al., 2005; Minamino, 2018). Based on these data, it is tempting to speculate that FliK might be involved in the expression or secretion of proteins/effectors that would account for the enhanced resistance of B. thuringiensis to AMPs. This hypothesis is supported by previous studies that have pinpointed the role of flagella in the secretion of virulence factors (Young et al., 1999; Duan et al., 2013). For example, in B. thuringiensis the expression of some secreted virulence determinants was shown to be dependent on a functional flagellar export apparatus (Bouillaut et al., 2005; Fagerlund et al., 2010). In particular, a Bt407 mutant carrying a mutation of the flhA gene, which encodes an essential protein of the flagellar secretion apparatus, is defective for the secretion of some virulence factors and was shown to exhibit a decreased expression of these virulence factors and to be less virulent in the Galleria mellonella insect model by both force-feeding and intrahemocoelic injection (Ghelardi et al., 2002; Bouillaut et al., 2005; Fagerlund et al., 2010). Likewise, FlhA was proposed as defining element for the regulation of virulence factors in C. jejuni (Carrillo et al., 2004). We strongly believe that investigation of FliK’s cellular functions deserves a more detailed study and are aiming, in the future, to examine its impact on the molecular composition of the cell envelope and the regulation of the transcription/secretion program in B. thuringiensis. In sum, by identifying FliK as prerequisite for the resistance of B. thuringiensis to host AMPs, this study sets the way for in depth studies useful for the identification of potential novel targets for the development of new antibacterial therapeutic strategies.
Materials and Methods
Bacterial Strains, Growth Conditions, and Bacterial Products
The acrystalliferous strain B. thuringiensis 407 Cry-(Bt407) (Lereclus et al., 1989) was used as wild-type throughout this study. E. coli K-12 strain TG1 was used as a host for cloning experiments. E. coli strain ET12567 (dam–; dcm–), was used to generate unmethylated plasmid DNA for Bt407 electrotransformation. Bt407 and E. coli strains were transformed as previously described (Dower et al., 1988; Lereclus et al., 1989).
A B. thuringiensis strain containing a fliK deletion was generated by precise, in frame allelic exchange and deletion replacement without antibiotic resistance cassettes. The thermosensitive plasmid MAD (pMAD) was used in these experiments. The 1.253 kb sequence immediately upstream from fliK was amplified with the primers fliK-a and fliK-b, and a 1.217 kb sequence immediately downstream from fliK was amplified with the primers fliK-c and fliK-d. The primers fliK-b and fliK-c introduce overlapping PCR products. The two amplicons were then subjected to another PCR cycle with the primers fliK-a and fliK-d, such that an amplicon, from which the 1.096 kb of fliK gene has been deleted, was amplified. This amplicon was digested with BamHI and NcoI and was introduced between the corresponding cloning sites of pMAD. Bt407 was then transformed with 10 μg of the recombinant plasmid by electroporation as previously described (Lereclus et al., 1989). Transformants were subjected to allelic exchange by homologous recombination and bacteria sensitive to erythromycin, resulting from double crossing over event in which the chromosomal fliK copy was replaced with the overlapping sequences, were selected. The procedure for selection of mutants by allelic exchange via double crossover has been described previously (Bravo et al., 1996). The chromosomal allelic exchange in the fliK mutant was checked by PCR using the appropriate primer couples (fliK-a and fliK-d) and confirmed by DNA sequencing of the PCR fragments generated from the primer pairs fliK-a and fliK-d. The resulting fliK-deficient strain was designated Bt407ΔfliK. For selection and CFU counting, we transformed Bt407 and Bt407ΔfliK with the unmethylated plasmid pHT315paphA3gfp containing ampicillin and erythromycin resistance cassettes. The primers fliK-a, fliKb, fliKc, and fliK-d are listed in the Supplementary Table 1.
The complemented strain of Bt407ΔfliK was constructed as follows: From Bt407 genomic DNA, the intergenic region immediately located upstream the operon, in which fliK is the second gene, was amplified and then cloned upstream the entire fliK gene by using the splicing by overlap extension technique (SOE). The primer CompfliK-a included a restriction site for BamHI and was used with CompfliK-b to amplify the intergenic region. The fliK gene was amplified from Bt407 genomic DNA by using CompfliK-c as forward primer and CompfliK-d that included a restriction site for EcoRI as reverse primer. The primers CompfliK-b and CompfliK-c introduce overlapping PCR products, which then served as matrix to a final PCR using CompfliK-a and CompfliK-d as primers. The resulting 1.274 kb fragment consisting of the full-length fliK gene downstream the intergenic region was digested with BamHI and EcoRI, gel-purified, and ligated to the pHT304-18 shuttle vector previously digested with the same enzymes. An aliquot of ligation mixture (∼50 ng DNA) was used to transform E. coli K-12 strain TG1 by electroporation as described previously (Lereclus et al., 1989). The resulting construct, pHT304-18ΩfliK, was verified by sequencing using the pHT304-18 specific primers PU and PR and then transferred into E. coli ET12567 by electroporation. Unmethylated plasmid pHT304-18ΩfliK from E. coli ET12567 was then introduced into strain Bt407ΔfliK by electroporation to generate the complemented Bt407ΔfliKΩfliK strain. Plasmid extraction was performed from the complemented strain, and the presence of pHT304-18ΩfliK was checked by sequencing using PU and PR primers. The primers CompfliK-a, CompfliK-b, CompfliK-c, CompfliK-d, PU, and PR are listed in Supplementary Table 1.
Bt407ΔdltX and Bt407Δdlt have been described (Kamar et al., 2017; Attieh et al., 2019). Bt407Δfla mutant was provided by Dr. Michel Gohar (Université Paris-Saclay, INRAE, AgroParisTech, Micalis Institute, Jouy-en-Josas, France) (Houry et al., 2010). E. coli DH5αGFP (Bonnay et al., 2013), commonly used as agent to induce Drosophila IMD pathway, was a kind gift from Pr. Jean Marc Reichhart (UPR9022—CNRS—Université de Strasbourg). All strains were grown in Luria-Bertani (LB) broth, with vigorous shaking, at 37°C for E. coli strains and at 30°C for Bt407 strains. The antibiotic concentrations used for bacterial selection were as follows: 100 μg/ml of ampicillin for E. coli; 100 μg/ml of spectinomycin for E. coli and 300 μg/ml of spectinomycin for B. thuringiensis and 10 μg/ml of erythromycin for Bt407 and its derivatives. β-Galactosidase production was detected on LB plates supplemented with X-Gal (5-bromo-4-chloro-3-indolyl-β-d-galactopyranoside) at 100 μg/ml.
Heat killing of bacteria was performed as described (El Chamy et al., 2008). Briefly, bacterial solutions followed two steps of 20 min of incubation at 95°C separated by 20 min of cooling on ice. Killing was verified by plating 100 μl of each bacterial solution on LB agar plates.
DNA Manipulations
Chromosomal DNA was extracted from B. thuringiensis using the Puregene Yeast/Bact. Kit B (QIAgen, France). Plasmid DNA was extracted from E. coli by standard alkaline lysis by using QIAprep spin columns (QIAgen, France). Restriction enzymes and T4 DNA ligase were used as recommended by the manufacturer (New England Biolabs). Oligonucleotide primers (Supplementary Table 1) were synthesized by Sigma Proligo (Paris, France). PCRs were performed in an Applied Biosystems 2720 Thermal cycler (Applied Biosystem, United States) with Phusion High-Fidelity or Taq DNA Polymerase (New England Biolabs). Amplified DNA fragments were purified with the QIAquick PCR purification kit (QIAgen, France). Digested DNA fragments were separated by electrophoresis and purified from agarose gels using the QIAquick gel extraction kit (QIAgen, France). All constructions were confirmed by DNA sequencing by GATC Biotech (Konstanz, Deutschland).
Generation of a B. thuringiensis Strain 407 (Cry–) Transposon Library
A mini-Tn10 derivative of Salmonella enterica serovar Typhimurium Tn10 transposon, carried out by the thermosensitive plasmid pIC333, as a transposon delivery system, was used for random insertion mutagenesis (Supplementary Figure 2; Petit et al., 1990; Steinmetz and Richter, 1994). Outside the transposon, the plasmid pIC333 contains the thermosensitive replication origin of pE194 (pE194 ts) plasmid, a gene conferring resistance to erythromycin (ermR) as well as a Tn10 transposase allele tnpA with relaxed target specificity to increase the transposition randomness. The mini-Tn10 transposon itself, in pIC333, is a 2.4 kb element composed of the two ends of Tn10 flanking a spectinomycin resistance gene (specR) and a pColE1-type origin of replication (Ori pColE1). An insertion library was constructed in Bt407 as described previously (Gominet et al., 2001; Espinasse et al., 2002; Fedhila et al., 2004; Kamoun et al., 2009). After Bt407 transformation with the pIC333 at 30°C, transformants were selected for their resistance to erythromycin. Subsequently, an upshift to the non-permissive temperature (37°C) under selection for spectinomycin resistance allowed the pIC333 elimination and the selection of derivatives in which the miniTn10 had integrated into the chromosome. Briefly, the culture was diluted 1:100 into fresh LB medium only supplemented with spectinomycin (150 μg/ml), and grown overnight while shaking at 37°C. This step was repeated 6–8 times. Appropriate dilutions of the growing cells were then plated on LB agar containing Spectinomycin (150 μg/ml) and incubated at 40°C for the selection of insertion mutants. Approximately 20,000 spectinomycin-resistant colonies were randomly selected and stocked as individual clones at −80°C.
Screening the B. thuringiensis Strain 407 (Cry–) Transposon Library for Sensitivity to Polymyxin B
The insertion mutants were then screened for increased sensitivity to polymyxin B, and those with higher sensitivity were selected. Appropriate dilutions of the mutant library were plated on LB agar medium supplemented with spectinomycin and incubated at 40°C overnight. All SpecR clones were then replicated on LB—agar medium containing erythromycin. The SpecR Erms clones (about 70% of the SpecR clones were Erms) were considered a mini-Tn10 insertion mutant, which were selected, inoculated on LB-Agar Petri dishes supplemented or not with 200 μg/ml of polymyxin B (50 colonies per plate) and then incubated at 30°C for 48 h. Bt407 and Bt407ΔdltX (Kamar et al., 2017) were used as positive and negative controls, respectively. Strains were considered as insensitive to 200 μg/ml of polymyxin B when colonies appeared after the above incubation times.
Marker Rescue of Flanking Genomic DNA and Identification of Transposon Insertion Site
In order to determine the mini-Tn10 insertion site within Bt407 mutant clones’ genome, a plasmid rescue has been conducted. Chromosomal DNA was extracted, digested with EcoRI or HindIII (The mini-Tn10 element contains no restriction sites for these enzymes) and the digestion products were self-ligated using T4 DNA ligase. E. coli TG1 cells were than transformed with the ligation mixture, hence allowing the selection of transformed clones with a plasmid containing the mini-Tn10 element and the DNA fragments flanking the insertion locus (The mini-Tn10 element harbors an E. coli replicon and a gene conferring resistance to spectinomycin). Putative clones obtained by selection for their resistance to spectinomycin were subjected to plasmid DNA extraction. In order to confirm the presence of mini-Tn10 in the selected clones genome, the restriction map of the plasmid was determined by the presence of a 2.2 kb BamHI characteristic fragment of mini-Tn10. As to determine the mini-Tn10 insertion site, the chromosomal DNA flanking the insertion locus in the plasmid was sequenced, using primers E1 and E3 (Supplementary Table 1) matching the transposon extremities. Sequencing reads were mapped to Bt407 genome by using the BLAST program of the National Center for Biotechnology Information Genbank (NCBI).
Atomic Force Microscopy (AFM) Analysis
Bacteria were visualized by AFM. The strains Bt407, Bt407ΔfliK, and Bt407ΔfliKΩfliK were taken from a frozen stock and grown overnight in 5 ml of liquid LB medium at 30°C and 220 rpm. The next day, cultures were diluted to 10–6 and 100 μl of each was spread on LB plates and incubated overnight at 30°C. One colony of each of the strains was then inoculated in 5 ml of liquid LB medium and grown overnight at 30°C and 220 rpm. The next day, 0.2 ml of each of the three strain cultures was inoculated into 20 ml of LB and grown as described above; samples were withdrawn for density measurements at 30 min intervals, until an OD600 of 1.0 was reached. One millilitre from each culture was then centrifuged at 4,000 rpm for 3 min. The supernatant was thrown away, and the pellet carefully resuspended in 1 ml PBS. Bacterial cells were centrifuged once more and resuspended again, in 1 ml MilliQ water. Twenty microlitre of sample was then added to 5 μl (100 mM) Tris/Mg2+ buffer and 25 μl MilliQ water. Ten microlitre of this solution was applied on a newly cleaved Mica (glued to a microscope slide), incubated at room temperature for 10 min, and then washed 10 times with 100 μl MilliQ water, before being air dried for some minutes and finally dried with a soft N2 gas stream. The slide was then scanned in an Atomic force microscope (JPK NanoWizard instrument, Berlin, Germany). The preparations were scanned using Intermittent contact (Ic) mode and a Silicon NSC35/AlBS cantilever (MicroMasch Spain). All scans were performed in air. The Error images obtained in Ic mode are named Amplitude images.
Sensitivity to Antimicrobial Peptides
Polymyxin B was used as a prototype antimicrobial compound for the large-scale screening of the collection. The test was performed in 96-well microplates containing seven concentrations of polymyxin B (Sigma) from 200 to 800 μg/ml for Bt407, Bt407Δfla, and Bt407ΔfliKΩfliK and from 25 to 250 μg/ml for Bt407ΔfliK. Bacterial growth was scored after inoculation with strains at an initial OD600 = 0.1 and incubation at 30°C for 6 h. Susceptibility to polymyxin B was evaluated by determining the half inhibitory concentration (IC50), corresponding to the concentration of polymyxin B halving inoculum viability. IC50 was determined by examining the dose-response curves obtained with the various concentrations of polymyxin B.
Motility Assay
The swimming ability of Bt407 strains was determined on LB soft agar plates (0.25% agar, final concentration) by measuring the bacterial growth area. A volume of 5 μl of an OD600 = 1 bacterial culture was spotted in the agar plate center and then incubated at 37°C. The colony diameters were measured every 6 h.
Biofilm Formation
The ability of Bt407 and its derivatives to form biofilms was determined as previously described (Fagerlund et al., 2014). Briefly, cultures in the exponential phase were diluted into HCT medium to an OD600 of 0.01. UV-sterilized 6 ml glass tubes were inoculated with 2 ml of the diluted cultures and then incubated for 48 h at 30°C without shaking. At the end of the incubation time, the 2 ml culture medium was removed using a Pasteur pipette and the OD600 of the biofilm, thoroughly vortexed in 2 ml PBS, was measured.
Autolysis Test
The autolysis test was performed as previously described (Abi Khattar et al., 2009). Briefly, bacterial solutions prepared from bacterial cells in exponential growth phase, washed twice with cold PBS, and resuspended in the same buffer supplemented with 0.05% Triton X-100, were incubated in 96-well plate at 37°C without shaking and autolysis was monitored by measuring the OD600 every 30 min.
Drosophila melanogaster Stocks and Maintenance
DrosDel isogenic w1118 (iso w1118) (Ryder et al., 2004) and OregonR were used as wild-type. The antimicrobial peptides mutant (ΔAMPs) and its wt control iso w1118 (Hanson et al., 2019) are a kind gift from Pr. Bruno Lemaitre (Global Health Institute, School of Life Sciences, École Polytechnique Fédérale de Lausanne, Lausanne, Switzerland). The IMD pathway mutant relishE20 (Rel) has been described (Hedengren et al., 1999). Fly stocks were raised on cornmeal-agar medium rich in yeast (7.25%) at 25°C.
Survival Experiments, Injections, and Immune Induction
Survival experiment were performed on a total of ≥ 45 adult females per genotype (15–20 individuals per each of the three biological replicates). Batches of 15–20 female flies, aged between 2 and 4 days old, were pricked with a tungsten needle previously dipped into a bacterial solution prepared from an overnight culture that was washed and diluted in PBS (1×) to a final OD600 = 2. The infected flies were incubated at 29°C. The Diptericin expression was measured by RT-qPCR 4 h post infection.
Sixty nine nanoliter of culture supernatant were injected into the thorax of batches of 20–25 female flies (aged 2–4 days old) with a Nanoject apparatus (Drummond, Broomall, PA). These flies were incubated 4 h at 29°C then Diptericin expression was quantified in RNA extracts by RT-qPCR.
Fly Internal Bacterial Load Quantification
The CFU counting was performed by plating serial dilutions of lysates obtained from 10 infected flies as described previously on LB agar medium containing the appropriate antibiotic to each strain.
Quantitative Real Time PCR
Total RNA was extracted with TRI Reagent (Sigma-Aldrich) from 15 to 25 adult of each genotype per biological replicate. The reverse transcription was performed on 1 μg of RNA by using the RevertAid RT Reverse Transcription Kit (Thermo Fisher Scientific). cDNA was used as template in PCR reaction with three 10 μl technical replicates of each biological sample. qPCR was performed on an iQ5 Real Time PCR detection system (Biorad) using iTaq Universal Syber Green supermix (Biorad). The amount of RNA detected was normalized to that of the house keeping gene rp49. Primers for Diptericin and rp49 genes are listed in the Supplementary Table 1. Relative gene expression levels between control and experimental samples was determined using the ΔΔCT method. Each experimental sample was compared to each wt sample.
Statistical Analysis
All data analysis was performed using GraphPad Prism 8.0.2 software and statistical tests used for each data set are indicated in figure legends.
Data Availability Statement
The original contributions presented in the study are included in the article/Supplementary Material, further inquiries can be directed to the corresponding author/s.
Author Contributions
ZA conceived, designed and performed the experiments, analyzed the data, contributed to the manuscript writing, and reviewed and edited the manuscript. CM, AR, and IJ performed the experiments. SP designed and constructed the Bt407ΔfliK mutation. IH and OØ conceived experiments, analyzed data, and reviewed and edited the manuscript. MKA conceived and designed the experiments, supervised the work, analyzed the data, and reviewed and edited the manuscript. VS-B and LEC conceived and designed the study, conceived and designed the experiments, supervised the work, analyzed the data, and wrote the manuscript. All authors contributed to the article and approved the submitted version.
Funding
This work was funded by the Research Council of the Saint-Joseph University of Beirut (FS73) and the Lebanese National Council of Scientific Research (CNRS-L/02-05-16) and has benefited from the support of the CEDRE program (32942VD) and the Institut National de Recherche pour l’Agriculture, l’Alimentation et l’Environnement (INRAE). ZA was supported by a Ph.D. fellowship from the CNRS-L and has also benefited from the SAFAR program and the international mobility scholarship from AgroParisTech (Bourse de mobilite Abies). CM was supported by a Ph.D. fellowship from the Research Council of the Saint-Joseph University of Beirut (FS139).
Conflict of Interest
The authors declare that the research was conducted in the absence of any commercial or financial relationships that could be construed as a potential conflict of interest.
Acknowledgments
We thank Prof. Bruno Lemaitre for providing the ΔAMPs mutant and its isogenic wild-type control and Dr. Michel Gohar for providing the Bt407Δfla mutant.
Supplementary Material
The Supplementary Material for this article can be found online at: https://www.frontiersin.org/articles/10.3389/fmicb.2020.611220/full#supplementary-material
References
Abachin, E., Poyart, C., Pellegrini, E., Milohanic, E., Fiedler, F., Berche, P., et al. (2002). Formation of D-alanyl-lipoteichoic acid is required for adhesion and virulence of Listeria monocytogenes. Mol. Microbiol. 43, 1–14. doi: 10.1046/j.1365-2958.2002.02723.x
Abi Khattar, Z., Rejasse, A., Destoumieux-Garzon, D., Escoubas, J. M., Sanchis, V., Lereclus, D., et al. (2009). The dlt operon of Bacillus cereus is required for resistance to cationic antimicrobial peptides and for virulence in insects. J. Bacteriol. 191, 7063–7073. doi: 10.1128/JB.00892-09
Ageitos, J. M., Sanchez-Perez, A., Calo-Mata, P., and Villa, T. G. (2017). Antimicrobial peptides (AMPs): ancient compounds that represent novel weapons in the fight against bacteria. Biochem. Pharmacol. 133, 117–138. doi: 10.1016/j.bcp.2016.09.018
Aizawa, S.-I. (2012). Mystery of FliK in length control of the flagellar hook. J. Bacteriol. 194, 4798–4800. doi: 10.1128/jb.06239-11
Andres, E., and Dimarcq, J. L. (2004). Cationic antimicrobial peptides: update of clinical development. J. Intern. Med. 255, 519–520. doi: 10.1046/j.1365-2796.2003.01278.x
Atilano, M. L., Yates, J., Glittenberg, M., Filipe, S. R., and Ligoxygakis, P. (2011). Wall teichoic acids of Staphylococcus aureus limit recognition by the Drosophila peptidoglycan recognition protein-SA to promote pathogenicity. PLoS Pathog. 7:e1002421. doi: 10.1371/journal.ppat.1002421
Attieh, Z., Awad, M. K., Rejasse, A., Courtin, P., Boneca, I. G., Chapot-Chartier, M.-P., et al. (2019). D-alanylation of Teichoic Acids in Bacilli impedes the immune sensing of peptidoglycan in Drosophila. BioRxiv doi: 10.1101/631523
Auger, S., Galleron, N., Bidnenko, E., Ehrlich, S. D., Lapidus, A., and Sorokin, A. (2008). The genetically remote pathogenic strain NVH391-98 of the Bacillus cereus group is representative of a cluster of thermophilic strains. Appl. Environ. Microbiol. 74, 1276–1280. doi: 10.1128/AEM.02242-07
Barembruch, C., and Hengge, R. (2007). Cellular levels and activity of the flagellar sigma factor FliA of Escherichia coli are controlled by FlgM-modulated proteolysis. Mol. Microbiol. 65, 76–89. doi: 10.1111/j.1365-2958.2007.05770.x
Bauer, M. E., and Shafer, W. M. (2015). On the in vivo significance of bacterial resistance to antimicrobial peptides. Biochim. Biophys. Acta Biomembranes 1848, 3101–3111. doi: 10.1016/j.bbamem.2015.02.012
Bell, G., and Gouyon, P.-H. (2003). Arming the enemy: the evolution of resistance to self-proteins. Microbiology 149, 1367–1375. doi: 10.1099/mic.0.26265-0
Bonnay, F., Cohen-Berros, E., Hoffmann, M., Kim, S. Y., Boulianne, G. L., Hoffmann, J. A., et al. (2013). big bang gene modulates gut immune tolerance in Drosophila. Proc. Natl. Acad. Sci. U.S.A. 110, 2957–2962. doi: 10.1073/pnas.1221910110
Bottone, E. J. (2010). Bacillus cereus, a volatile human pathogen. Clin. Microbiol. Rev. 23, 382–398. doi: 10.1128/CMR.00073-09
Bouillaut, L., Ramarao, N., Buisson, C., Gilois, N., Gohar, M., Lereclus, D., et al. (2005). FlhA influences Bacillus thuringiensis PlcR-regulated gene transcription, protein production, and virulence. Appl. Environ. Microbiol. 71, 8903–8910. doi: 10.1128/aem.71.12.8903-8910.2005
Bravo, A., Agaisse, H., Salamitou, S., and Lereclus, D. (1996). Analysis of cryIAa expression in sigE and sigK mutants of Bacillus thuringiensis. Mol. Gen. Genet. 250, 734–741. doi: 10.1007/bf02172985
Brogden, K. A. (2005). Antimicrobial peptides: pore formers or metabolic inhibitors in bacteria? Nat. Rev. Microbiol. 3, 238–250. doi: 10.1038/nrmicro1098
Buisson, C., Gohar, M., Huillet, E., and Nielsen-LeRoux, C. (2019). Bacillus thuringiensis spores and vegetative bacteria: infection capacity and role of the virulence regulon PlcR following intrahaemocoel injection of Galleria mellonella. Insects 10:129. doi: 10.3390/insects10050129
Calvo, R. A., and Kearns, D. B. (2015). FlgM is secreted by the flagellar export apparatus in Bacillus subtilis. J. Bacteriol. 197, 81–91. doi: 10.1128/jb.02324-14
Carrillo, C. D., Taboada, E., Nash, J. H., Lanthier, P., Kelly, J., Lau, P. C., et al. (2004). Genome-wide expression analyses of Campylobacter jejuni NCTC11168 reveals coordinate regulation of motility and virulence by flhA. J. Biol. Chem. 279, 20327–20338. doi: 10.1074/jbc.m401134200
Carroll, L. M., Wiedmann, M., and Kovac, J. (2020). Proposal of a taxonomic nomenclature for the Bacillus cereus group which reconciles genomic definitions of bacterial species with clinical and industrial phenotypes. mBio 11:e00034-20.
Chaput, C., and Boneca, I. G. (2007). Peptidoglycan detection by mammals and flies. Microbes Infect. 9, 637–647. doi: 10.1016/j.micinf.2007.01.022
Chevance, F. F., and Hughes, K. T. (2008). Coordinating assembly of a bacterial macromolecular machine. Nat. Rev. Microbiol. 6, 455–465. doi: 10.1038/nrmicro1887
Cole, J. N., and Nizet, V. (2016). Bacterial evasion of host antimicrobial peptide defenses. Microbiol. Spectr. 4, 413–443.
Costerton, J. W. (2004). “A short history of the development of the biofilm concept,” in Microbial Biofilms, eds M. Ghannoum and G. O’Toole (Washington, DC: American Society of Microbiology), 4–19. doi: 10.1128/9781555817718.ch1
Courtney, C. R., Cozy, L. M., and Kearns, D. B. (2012). Molecular characterization of the flagellar hook in Bacillus subtilis. J. Bacteriol. 194, 4619–4629. doi: 10.1128/JB.00444-12
Cullen, T. W., Madsen, J. A., Ivanov, P. L., Brodbelt, J. S., and Trent, M. S. (2012). Characterization of unique modification of flagellar rod protein FlgG by Campylobacter jejuni lipid A phosphoethanolamine transferase, linking bacterial locomotion and antimicrobial peptide resistance. J. Biol. Chem. 287, 3326–3336. doi: 10.1074/jbc.m111.321737
Cullen, T. W., and Trent, M. S. (2010). A link between the assembly of flagella and lipooligosaccharide of the Gram-negative bacterium Campylobacter jejuni. Proc. Natl. Acad. Sci. U.S.A. 107, 5160–5165. doi: 10.1073/pnas.0913451107
Damgaard, P. H., Granum, P. E., Bresciani, J., Torregrossa, M. V., Eilenberg, J., and Valentino, L. (1997). Characterization of Bacillus thuringiensis isolated from infections in burn wounds. FEMS Immunol. Med. Microbiol. 18, 47–53.
Daughdrill, G. W., Chadsey, M. S., Karlinsey, J. E., Hughes, K. T., and Dahlquist, F. W. (1997). The C-terminal half of the anti-sigma factor, FlgM, becomes structured when bound to its target, σ 28. Nat. Struct. Biol. 4, 285–291. doi: 10.1038/nsb0497-285
Dierick, K., Van Coillie, E., Swiecicka, I., Meyfroidt, G., Devlieger, H., Meulemans, A., et al. (2005). Fatal family outbreak of Bacillus cereus-associated food poisoning. J. Clin. Microbiol. 43, 4277–4279. doi: 10.1128/JCM.43.8.4277-4279.2005
Dower, W. J., Miller, J. F., and Ragsdale, C. W. (1988). High efficiency transformation of E. coli by high voltage electroporation. Nucleic Acids Res 16, 6127–6145. doi: 10.1093/nar/16.13.6127
Duan, Q., Zhou, M., Zhu, L., and Zhu, G. (2013). Flagella and bacterial pathogenicity. J. Basic Microbiol. 53, 1–8. doi: 10.1002/jobm.201100335
ECDC/EMEA (2009). ECDC/EMEA Joint Technical Report—The Bacterial Challenge: Time to React. Solna: ECDC.
EFSA (2009). The community summary report on food-borne outbreaks in the European Union in 2007. EFSA J. 7:271r.
Ehling-Schulz, M., Lereclus, D., and Koehler, T. M. (2019). The Bacillus cereus group: Bacillus species with pathogenic potential. Microbiol. Spectr. 7, 875–902. doi: 10.1128/microbiolspec.GPP3-0032-2018
El Chamy, L., Leclerc, V., Caldelari, I., and Reichhart, J. M. (2008). Sensing of ‘danger signals’ and pathogen-associated molecular patterns defines binary signaling pathways ‘upstream’ of Toll. Nat. Immunol. 9, 1165–1170. doi: 10.1038/ni.1643
Erhardt, M., Hirano, T., Su, Y., Paul, K., Wee, D. H., Mizuno, S., et al. (2010). The role of the FliK molecular ruler in hook-length control in Salmonella enterica. Mol. Microbiol. 75, 1272–1284. doi: 10.1111/j.1365-2958.2010.07050.x
Erhardt, M., Singer, H. M., Wee, D. H., Keener, J. P., and Hughes, K. T. (2011). An infrequent molecular ruler controls flagellar hook length in Salmonella enterica. EMBO J. 30, 2948–2961. doi: 10.1038/emboj.2011.185
Espinasse, S., Gohar, M., Lereclus, D., and Sanchis, V. (2002). An ABC transporter from Bacillus thuringiensis is essential for β-exotoxin I production. J. Bacteriol. 184, 5848–5854. doi: 10.1128/jb.184.21.5848-5854.2002
Evans, L. D., Hughes, C., and Fraser, G. M. (2014). Building a flagellum outside the bacterial cell. Trends Microbiol. 22, 566–572. doi: 10.1016/j.tim.2014.05.009
Evans, L. D., Poulter, S., Terentjev, E. M., Hughes, C., and Fraser, G. M. (2013). A chain mechanism for flagellum growth. Nature 504, 287–290. doi: 10.1038/nature12682
Fabretti, F., Theilacker, C., Baldassarri, L., Kaczynski, Z., Kropec, A., Holst, O., et al. (2006). Alanine esters of enterococcal lipoteichoic acid play a role in biofilm formation and resistance to antimicrobial peptides. Infect. Immun. 74, 4164–4171. doi: 10.1128/IAI.00111-06
Fagerlund, A., Brillard, J., Furst, R., Guinebretiere, M. H., and Granum, P. E. (2007). Toxin production in a rare and genetically remote cluster of strains of the Bacillus cereus group. BMC Microbiol. 7:43. doi: 10.1186/1471-2180-7-43
Fagerlund, A., Dubois, T., Økstad, O.-A., Verplaetse, E., Gilois, N., Bennaceur, I., et al. (2014). SinR controls enterotoxin expression in Bacillus thuringiensis biofilms. PLoS One 9:e96707. doi: 10.1371/journal.pone.0096707
Fagerlund, A., Lindbäck, T., and Granum, P. E. (2010). Bacillus cereus cytotoxins Hbl, Nhe and CytK are secreted via the Sec translocation pathway. BMC Microbiol. 10:304. doi: 10.1186/1471-2180-10-304
Fedhila, S., Guillemet, E., Nel, P., and Lereclus, D. (2004). Characterization of two Bacillus thuringiensis genes identified by in vivo screening of virulence factors. Appl. Environ. Microbiol. 70, 4784–4791. doi: 10.1128/aem.70.8.4784-4791.2004
Ghelardi, E., Celandroni, F., Salvetti, S., Beecher, D. J., Gominet, M., Lereclus, D., et al. (2002). Requirement of flhA for swarming differentiation, flagellin export, and secretion of virulence-associated proteins in Bacillus thuringiensis. J. Bacteriol. 184, 6424–6433. doi: 10.1128/jb.184.23.6424-6433.2002
Gillen, K. L., and Hughes, K. T. (1991). Molecular characterization of flgM, a gene encoding a negative regulator of flagellin synthesis in Salmonella typhimurium. J. Bacteriol. 173, 6453–6459. doi: 10.1128/jb.173.20.6453-6459.1991
Glasset, B., Herbin, S., Granier, S. A., Cavalie, L., Lafeuille, E., Guerin, C., et al. (2018). Bacillus cereus, a serious cause of nosocomial infections: epidemiologic and genetic survey. PLoS One 13:e0194346. doi: 10.1371/journal.pone.0194346
Gohar, M., Faegri, K., Perchat, S., Ravnum, S., Okstad, O. A., Gominet, M., et al. (2008). The PlcR virulence regulon of Bacillus cereus. PLoS One 3:e2793. doi: 10.1371/journal.pone.0002793
Gominet, M., Slamti, L., Gilois, N., Rose, M., and Lereclus, D. (2001). Oligopeptide permease is required for expression of the Bacillus thuringiensis plcR regulon and for virulence. Mol. Microbiol. 40, 963–975. doi: 10.1046/j.1365-2958.2001.02440.x
Gray, E. W., Adler, P. H., and Noblet, R. (1996). Economic impact of black flies (Diptera: Simuliidae) in South Carolina and development of a localized suppression program. J. Am. Mosq. Control Assoc. 12, 676–678.
Green, M., Heumann, M., Sokolow, R., Foster, L. R., Bryant, R., and Skeels, M. (1990). Public health implications of the microbial pesticide Bacillus thuringiensis: an epidemiological study, Oregon, 1985-86. Am. J. Public Health 80, 848–852. doi: 10.2105/ajph.80.7.848
Guani-Guerra, E., Santos-Mendoza, T., Lugo-Reyes, S. O., and Teran, L. M. (2010). Antimicrobial peptides: general overview and clinical implications in human health and disease. Clin. Immunol. 135, 1–11. doi: 10.1016/j.clim.2009.12.004
Guinebretiere, M. H., Auger, S., Galleron, N., Contzen, M., De Sarrau, B., De Buyser, M. L., et al. (2013). Bacillus cytotoxicus sp. nov. is a novel thermotolerant species of the Bacillus cereus group occasionally associated with food poisoning. Int. J. Syst. Evol. Microbiol. 63(Pt 1), 31–40. doi: 10.1099/ijs.0.030627-0
Guttenplan, S. B., and Kearns, D. B. (2013). Regulation of flagellar motility during biofilm formation. FEMS Microbiol. Rev. 37, 849–871. doi: 10.1111/1574-6976.12018
Hall-Stoodley, L., and Stoodley, P. (2009). Evolving concepts in biofilm infections. Cell Microbiol. 11, 1034–1043. doi: 10.1111/j.1462-5822.2009.01323.x
Haney, E. F., Mansour, S. C., and Hancock, R. E. (2017). Antimicrobial peptides: an introduction. Methods Mol. Biol. 1548, 3–22. doi: 10.1007/978-1-4939-6737-7_1
Hanson, M. A., Dostalova, A., Ceroni, C., Poidevin, M., Kondo, S., and Lemaitre, B. (2019). Synergy and remarkable specificity of antimicrobial peptides in vivo using a systematic knockout approach. eLife 8:e44341.
Hanson, M. A., and Lemaitre, B. (2020). New insights on Drosophila antimicrobial peptide function in host defense and beyond. Curr. Opin. Immunol 62, 22–30. doi: 10.1016/j.coi.2019.11.008
Heaton, M. P., and Neuhaus, F. C. (1992). Biosynthesis of D-alanyl-lipoteichoic acid: cloning, nucleotide sequence, and expression of the Lactobacillus casei gene for the D-alanine-activating enzyme. J. Bacteriol. 174, 4707–4717. doi: 10.1128/jb.174.14.4707-4717.1992
Hedengren, M., Asling, B., Dushay, M. S., Ando, I., Ekengren, S., Wihlborg, M., et al. (1999). Relish, a central factor in the control of humoral but not cellular immunity in Drosophila. Mol. Cell. 4, 1–20. doi: 10.1016/0008-8749(75)90078-7
Helgason, E., Caugant, D. A., Olsen, I., and Kolstø, A.-B. (2000). Genetic structure of population of Bacillus cereus and B. thuringiensis isolates associated with periodontitis and other human infections. J. Clin. Microbiol. 38, 1615–1622. doi: 10.1128/jcm.38.4.1615-1622.2000
Hernaiz, C., Picardo, A., Alos, J. I., and Gomez-Garces, J. L. (2003). Nosocomial bacteremia and catheter infection by Bacillus cereus in an immunocompetent patient. Clin. Microbiol. Infect. 9, 973–975. doi: 10.1046/j.1469-0691.2003.00682.x
Hernandez, E., Ramisse, F., Ducoureau, J.-P., Cruel, T., and Cavallo, J.-D. (1998). Bacillus thuringiensis subsp. konkukian (serotype H34) superinfection: case report and experimental evidence of pathogenicity in immunosuppressed mice. J. Clin. Microbiol. 36, 2138–2139. doi: 10.1128/jcm.36.7.2138-2139.1998
Hoffmaster, A. R., Ravel, J., Rasko, D. A., Chapman, G. D., Chute, M. D., Marston, C. K., et al. (2004). Identification of anthrax toxin genes in a Bacillus cereus associated with an illness resembling inhalation anthrax. Proc. Natl. Acad. Sci. U.S.A. 101, 8449–8454. doi: 10.1073/pnas.0402414101
Houry, A., Briandet, R., Aymerich, S., and Gohar, M. (2010). Involvement of motility and flagella in Bacillus cereus biofilm formation. Microbiology 156(Pt 4), 1009–1018. doi: 10.1099/mic.0.034827-0
Houry, A., Gohar, M., Deschamps, J., Tischenko, E., Aymerich, S., Gruss, A., et al. (2012). Bacterial swimmers that infiltrate and take over the biofilm matrix. Proc. Natl. Acad. Sci. U.S.A. 109, 13088–13093. doi: 10.1073/pnas.1200791109
Hughes, K. T. (2012a). Flagellar hook length is controlled by a secreted molecular ruler. J. Bacteriol. 194, 4793–4796. doi: 10.1128/jb.00343-12
Hughes, K. T. (2012b). Rebuttal: mystery of flik in length control of the flagellar hook. J. Bacteriol. 194:4801. doi: 10.1128/JB.06454-11
Humann, J., and Lenz, L. L. (2009). Bacterial peptidoglycan-degrading enzymes and their impact on host muropeptide detection. J. Innate Immun. 1, 88–97. doi: 10.1159/000181181
Imler, J. L., and Bulet, P. (2005). Antimicrobial peptides in Drosophila: structures, activities and gene regulation. Chem. Immunol. Allergy 86, 1–21. doi: 10.1159/000086648
Janeway, C. A. Jr. (1989). Approaching the asymptote? Evolution and revolution in immunology. Cold Spring Harb. Symp. Quant. Biol. 54(Pt 1), 1–13. doi: 10.1101/sqb.1989.054.01.003
Jones, K. E., Patel, N. G., Levy, M. A., Storeygard, A., Balk, D., Gittleman, J. L., et al. (2008). Global trends in emerging infectious diseases. Nature 451, 990–993. doi: 10.1038/nature06536
Journet, L., Agrain, C., Broz, P., and Cornelis, G. R. (2003). The needle length of bacterial injectisomes is determined by a molecular ruler. Science 302, 1757–1760. doi: 10.1126/science.1091422
Kalir, S., McClure, J., Pabbaraju, K., Southward, C., Ronen, M., Leibler, S., et al. (2001). Ordering genes in a flagella pathway by analysis of expression kinetics from living bacteria. Science 292, 2080–2083. doi: 10.1126/science.1058758
Kamar, R., Rejasse, A., Jehanno, I., Attieh, Z., Courtin, P., Chapot-Chartier, M. P., et al. (2017). DltX of Bacillus thuringiensis is essential for D-Alanylation of teichoic acids and resistance to antimicrobial response in insects. Front. Microbiol. 8:1437. doi: 10.3389/fmicb.2017.01437
Kamoun, F., Fguira, I. B., Tounsi, A., Abdelkefi-Mesrati, L., Sanchis, V., Lereclus, D., et al. (2009). Generation of Mini-Tn 10 transposon insertion mutant library of Bacillus thuringiensis for the investigation of genes required for its bacteriocin production. FEMS Microbiol. Lett. 294, 141–149. doi: 10.1111/j.1574-6968.2009.01559.x
Kaneko, T., Goldman, W. E., Mellroth, P., Steiner, H., Fukase, K., Kusumoto, S., et al. (2004). Monomeric and polymeric gram-negative peptidoglycan but not purified LPS stimulate the Drosophila IMD pathway. Immunity 20, 637–649. doi: 10.1016/s1074-7613(04)00104-9
Karlinsey, J. E., Lonner, J., Brown, K. L., and Hughes, K. T. (2000a). Translation/secretion coupling by type III secretion systems. Cell 102, 487–497. doi: 10.1016/s0092-8674(00)00053-2
Karlinsey, J. E., Tanaka, S., Bettenworth, V., Yamaguchi, S., Boos, W., Aizawa, S. I., et al. (2000b). Completion of the hook–basal body complex of the Salmonella typhimurium flagellum is coupled to FlgM secretion and fliC transcription. Mol. Microbiol. 37, 1220–1231. doi: 10.1046/j.1365-2958.2000.02081.x
Kinoshita, M., Tanaka, S., Inoue, Y., Namba, K., Aizawa, S.-I., and Minamino, T. (2020). The flexible linker of the secreted FliK ruler is required for export switching of the flagellar protein export apparatus. Sci. Rep. 10:838.
Klausen, M., Heydorn, A., Ragas, P., Lambertsen, L., Aaes-Jørgensen, A., Molin, S., et al. (2003). Biofilm formation by Pseudomonas aeruginosa wild type, flagella and type IV pili mutants. Mol. Microbiol. 48, 1511–1524. doi: 10.1046/j.1365-2958.2003.03525.x
Klee, S. R., Brzuszkiewicz, E. B., Nattermann, H., Bruggemann, H., Dupke, S., Wollherr, A., et al. (2010). The genome of a Bacillus isolate causing anthrax in chimpanzees combines chromosomal properties of B. cereus with B. anthracis virulence plasmids. PLoS One 5:e10986. doi: 10.1371/journal.pone.0010986
Koprivnjak, T., and Peschel, A. (2011). Bacterial resistance mechanisms against host defense peptides. Cell Mol. Life Sci. 68, 2243–2254. doi: 10.1007/s00018-011-0716-4
Kovacs, M., Halfmann, A., Fedtke, I., Heintz, M., Peschel, A., Vollmer, W., et al. (2006). A functional dlt operon, encoding proteins required for incorporation of d-alanine in teichoic acids in gram-positive bacteria, confers resistance to cationic antimicrobial peptides in Streptococcus pneumoniae. J. Bacteriol. 188, 5797–5805. doi: 10.1128/JB.00336-06
Kragol, G., Lovas, S., Varadi, G., Condie, B. A., Hoffmann, R., and Otvos, L. Jr. (2001). The antibacterial peptide pyrrhocoricin inhibits the ATPase actions of DnaK and prevents chaperone-assisted protein folding. Biochemistry 40, 3016–3026. doi: 10.1021/bi002656a
Kristian, S. A., Datta, V., Weidenmaier, C., Kansal, R., Fedtke, I., Peschel, A., et al. (2005). D-alanylation of teichoic acids promotes group a streptococcus antimicrobial peptide resistance, neutrophil survival, and epithelial cell invasion. J. Bacteriol. 187, 6719–6725. doi: 10.1128/JB.187.19.6719-6725.2005
Kristian, S. A., Lauth, X., Nizet, V., Goetz, F., Neumeister, B., Peschel, A., et al. (2003). Alanylation of teichoic acids protects Staphylococcus aureus against Toll-like receptor 2-dependent host defense in a mouse tissue cage infection model. J. Infect. Dis. 188, 414–423. doi: 10.1086/376533
Kuchenmuller, T., Hird, S., Stein, C., Kramarz, P., Nanda, A., and Havelaar, A. H. (2009). Estimating the global burden of foodborne diseases–a collaborative effort. Euro. Surveill. 14:19195. doi: 10.2807/ese.14.18.19195-en
Kuroki, R., Kawakami, K., Qin, L., Kaji, C., Watanabe, K., Kimura, Y., et al. (2009). Nosocomial bacteremia caused by biofilm-forming Bacillus cereus and Bacillus thuringiensis. Intern. Med. 48, 791–796. doi: 10.2169/internalmedicine.48.1885
Kushner, D. J., and Heimpel, A. M. (1957). Lecithinase production by strains of Bacillus cereus Fr. and Fr. pathogenic for the larch sawfly, Pristiphora erichsonii (Htg). Can. J. Microbiol. 3, 547–551. doi: 10.1139/m57-059
Kutsukake, K., and Iino, T. (1994). Role of the FliA-FlgM regulatory system on the transcriptional control of the flagellar regulon and flagellar formation in Salmonella typhimurium. J. Bacteriol. 176, 3598–3605. doi: 10.1128/jb.176.12.3598-3605.1994
Lapidus, A., Goltsman, E., Auger, S., Galleron, N., Segurens, B., Dossat, C., et al. (2008). Extending the Bacillus cereus group genomics to putative food-borne pathogens of different toxicity. Chem. Biol. Interact. 171, 236–249. doi: 10.1016/j.cbi.2007.03.003
Latsios, G., Petrogiannopoulos, C., Hartzoulakis, G., Kondili, L., Bethimouti, K., and Zaharof, A. (2003). Liver abscess due to Bacillus cereus: a case report. Clin. Microbiol. Infect. 9, 1234–1237. doi: 10.1111/j.1469-0691.2003.00795.x
Lemon, K., Earl, A., Vlamakis, H., Aguilar, C., and Kolter, R. (2008). Biofilm development with an emphasis on Bacillus subtilis. Curr. Top. Microbiol. Immunol. 322, 1–16. doi: 10.1007/978-3-540-75418-3_1
Lereclus, D., Arantes, O., Chaufaux, J., and Lecadet, M. (1989). Transformation and expression of a cloned delta-endotoxin gene in Bacillus thuringiensis. FEMS Microbiol. Lett. 51, 211–217. doi: 10.1016/0378-1097(89)90511-9
Leulier, F., Parquet, C., Pili-Floury, S., Ryu, J. H., Caroff, M., Lee, W. J., et al. (2003). The Drosophila immune system detects bacteria through specific peptidoglycan recognition. Nat. Immunol. 4, 478–484. doi: 10.1038/ni922
Leulier, F., Rodriguez, A., Khush, R. S., Abrams, J. M., and Lemaitre, B. (2000). The Drosophila caspase Dredd is required to resist Gram-negative bacterial infection. Embo Rep. 1, 353–358. doi: 10.1093/embo-reports/kvd073
Levy, S. B., and Marshall, B. (2004). Antibacterial resistance worldwide: causes, challenges and responses. Nat. Med. 10(12 Suppl.), S122–S129. doi: 10.1038/nm1145
Liu, Y., Du, J., Lai, Q., Zeng, R., Ye, D., Xu, J., et al. (2017). Proposal of nine novel species of the Bacillus cereus group. Int. J. Syst. Evol. Microbiol. 67, 2499–2508. doi: 10.1099/ijsem.0.001821
Lund, T., De Buyser, M. L., and Granum, P. E. (2000). A new cytotoxin from Bacillus cereus that may cause necrotic enteritis. Mol. Microbiol. 38, 254–261. doi: 10.1046/j.1365-2958.2000.02147.x
Mahler, H., Pasi, A., Kramer, J. M., Schulte, P., Scoging, A. C., Bar, W., et al. (1997). Fulminant liver failure in association with the emetic toxin of Bacillus cereus. N. Engl. J. Med. 336, 1142–1148. doi: 10.1056/nejm199704173361604
Majed, R., Faille, C., Kallassy, M., and Gohar, M. (2016). Bacillus cereus biofilms-same. Only different. Front. Microbiol. 7:1054. doi: 10.3389/fmicb.2016.01054
Marxer, M., Vollenweider, V., and Schmid-Hempel, P. (2016). Insect antimicrobial peptides act synergistically to inhibit a trypanosome parasite. Philos. Trans. R. Soc. Lond. B Biol. Sci. 371:20150302. doi: 10.1098/rstb.2015.0302
Matsumoto, S., Suenaga, H., Naito, K., Sawazaki, M., Hiramatsu, T., and Agata, N. (2000). Management of suspected nosocomial infection: an audit of 19 hospitalized patients with septicemia caused by Bacillus species. Jpn. J. Infect. Dis. 53, 196–202.
Medzhitov, R. (2007). Recognition of microorganisms and activation of the immune response. Nature 449, 819–826. doi: 10.1038/nature06246
Miller, J. M., Hair, J. G., Hebert, M., Hebert, L., and Roberts, F. J. Jr., et al. (1997). Fulminating bacteremia and pneumonia due to Bacillus cereus. J. Clin. Microbiol. 35, 504–507. doi: 10.1128/jcm.35.2.504-507.1997
Minamino, T. (2018). Hierarchical protein export mechanism of the bacterial flagellar type III protein export apparatus. FEMS Microbiol. Lett. 365:fny117.
Minamino, T., Doi, H., and Kutsukake, K. (1999a). Substrate specificity switching of the flagellum-specific export apparatus during flagellar morphogenesis in Salmonella typhimurium. Biosci. Biotechnol. Biochem. 63, 1301–1303. doi: 10.1271/bbb.63.1301
Minamino, T., Gonzalez-Pedrajo, B., Yamaguchi, K., Aizawa, S. I., and Macnab, R. M. (1999b). FliK, the protein responsible for flagellar hook length control in Salmonella, is exported during hook assembly. Mol. Microbiol. 34, 295–304. doi: 10.1046/j.1365-2958.1999.01597.x
Minamino, T., Kawamoto, A., Kinoshita, M., and Namba, K. (2019). Molecular organization and assembly of the export apparatus of flagellar type III secretion systems. Curr. Top. Microbiol. Immunol. 427, 91–107. doi: 10.1007/82_2019_170
Minamino, T., Moriya, N., Hirano, T., Hughes, K. T., and Namba, K. (2009). Interaction of FliK with the bacterial flagellar hook is required for efficient export specificity switching. Mol. Microbiol. 74, 239–251. doi: 10.1111/j.1365-2958.2009.06871.x
Minamino, T., Saijo-Hamano, Y., Furukawa, Y., Gonzalez-Pedrajo, B., Macnab, R. M., and Namba, K. (2004). Domain organization and function of Salmonella FliK, a flagellar hook-length control protein. J. Mol. Biol. 341, 491–502. doi: 10.1016/j.jmb.2004.06.012
Mizuno, S., Amida, H., Kobayashi, N., Aizawa, S.-I., and Tate, S.-I. (2011). The NMR structure of FliK, the trigger for the switch of substrate specificity in the flagellar type III secretion apparatus. J. Mol. Biol. 409, 558–573. doi: 10.1016/j.jmb.2011.04.008
Moriya, N., Minamino, T., Hughes, K. T., Macnab, R. M., and Namba, K. (2006). The type III flagellar export specificity switch is dependent on FliK ruler and a molecular clock. J. Mol. Biol. 359, 466–477. doi: 10.1016/j.jmb.2006.03.025
Mukherjee, S., and Kearns, D. B. (2014). The structure and regulation of flagella in Bacillus subtilis. Annu. Rev. Genet. 48, 319–340.
Nawrocki, K. L., Crispell, E. K., and McBride, S. M. (2014). Antimicrobial peptide resistance mechanisms of gram-positive bacteria. Antibiotics 3, 461–492. doi: 10.3390/antibiotics3040461
O’Toole, G. A., and Kolter, R. (1998). Flagellar and twitching motility are necessary for Pseudomonas aeruginosa biofilm development. Mol. Microbiol. 30, 295–304. doi: 10.1046/j.1365-2958.1998.01062.x
Park, C. B., Kim, H. S., and Kim, S. C. (1998). Mechanism of action of the antimicrobial peptide buforin II: buforin II kills microorganisms by penetrating the cell membrane and inhibiting cellular functions. Biochem. Biophys. Res. Commun. 244, 253–257. doi: 10.1006/bbrc.1998.8159
Patterson-Delafield, J., Martinez, R. J., Stocker, B., and Yamaguchi, S. (1973). A new fla gene in Salmonella typhimurium—flaR—and its mutant phenotype-superhooks. Archiv. Mikrobiol. 90, 107–120. doi: 10.1007/bf00414513
Perea Velez, M., Verhoeven, T. L., Draing, C., Von Aulock, S., Pfitzenmaier, M., Geyer, A., et al. (2007). Functional analysis of D-alanylation of lipoteichoic acid in the probiotic strain Lactobacillus rhamnosus GG. Appl. Environ. Microbiol. 73, 3595–3604. doi: 10.1128/AEM.02083-06
Perego, M., Glaser, P., Minutello, A., Strauch, M. A., Leopold, K., and Fischer, W. (1995). Incorporation of D-alanine into lipoteichoic acid and wall teichoic acid in Bacillus subtilis. Identification of genes and regulation. J. Biol. Chem. 270, 15598–15606. doi: 10.1074/jbc.270.26.15598
Perron, G. G., Zasloff, M., and Bell, G. (2006). Experimental evolution of resistance to an antimicrobial peptide. Proc. R. Soc. B Biol. Sci. 273, 251–256. doi: 10.1098/rspb.2005.3301
Peschel, A., Jack, R. W., Otto, M., Collins, L. V., Staubitz, P., Nicholson, G., et al. (2001). Staphylococcus aureus resistance to human defensins and evasion of neutrophil killing via the novel virulence factor MprF is based on modification of membrane lipids with l-lysine. J. Exp. Med. 193, 1067–1076. doi: 10.1084/jem.193.9.1067
Peschel, A., Otto, M., Jack, R. W., Kalbacher, H., Jung, G., and Gotz, F. (1999). Inactivation of the dlt operon in Staphylococcus aureus confers sensitivity to defensins, protegrins, and other antimicrobial peptides. J. Biol. Chem. 274, 8405–8410. doi: 10.1074/jbc.274.13.8405
Petit, M. A., Bruand, C., Janniere, L., and Ehrlich, S. D. (1990). Tn10-derived transposons active in Bacillus subtilis. J. Bacteriol. 172, 6736–6740. doi: 10.1128/jb.172.12.6736-6740.1990
Pratt, L. A., and Kolter, R. (1998). Genetic analysis of Escherichia coli biofilm formation: roles of flagella, motility, chemotaxis and type I pili. Mol. Microbiol. 30, 285–293. doi: 10.1046/j.1365-2958.1998.01061.x
Rahnamaeian, M., Cytrynska, M., Zdybicka-Barabas, A., Dobslaff, K., Wiesner, J., Twyman, R. M., et al. (2015). Insect antimicrobial peptides show potentiating functional interactions against Gram-negative bacteria. Proc. Biol. Sci. 282:20150293. doi: 10.1098/rspb.2015.0293
Ramarao, N., Nielsen-Leroux, C., and Lereclus, D. (2012). The insect Galleria mellonella as a powerful infection model to investigate bacterial pathogenesis. J. Vis. Exp. 70:e4392. doi: 10.3791/4392
Ramarao, N., and Sanchis, V. (2013). The pore-forming haemolysins of bacillus cereus: a review. Toxins 5, 1119–1139. doi: 10.3390/toxins5061119
Rolff, J., and Schmid-Hempel, P. (2016). Perspectives on the evolutionary ecology of arthropod antimicrobial peptides. Philos. Trans. R. Soc. Lond. B Biol. Sci. 371:20150297. doi: 10.1098/rstb.2015.0297
Ryder, E., Blows, F., Ashburner, M., Bautista-Llacer, R., Coulson, D., Drummond, J., et al. (2004). The DrosDel collection: a set of P-element insertions for generating custom chromosomal aberrations in Drosophila melanogaster. Genetics 167, 797–813. doi: 10.1534/genetics.104.026658
Salamitou, S., Ramisse, F., Brehelin, M., Bourguet, D., Gilois, N., Gominet, M., et al. (2000). The plcR regulon is involved in the opportunistic properties of Bacillus thuringiensis and Bacillus cereus in mice and insects. Microbiology 146(Pt 11), 2825–2832. doi: 10.1099/00221287-146-11-2825
Samant, S., Hsu, F.-F., Neyfakh, A. A., and Lee, H. (2009). The Bacillus anthracis protein MprF is required for synthesis of lysylphosphatidylglycerols and for resistance to cationic antimicrobial peptides. J. Bacteriol. 191, 1311–1319. doi: 10.1128/jb.01345-08
Schmitt, P., Rosa, R. D., and Destoumieux-Garzon, D. (2016). An intimate link between antimicrobial peptide sequence diversity and binding to essential components of bacterial membranes. Biochim. Biophys. Acta 1858, 958–970. doi: 10.1016/j.bbamem.2015.10.011
Scocchi, M., Mardirossian, M., Runti, G., and Benincasa, M. (2016). Non-membrane permeabilizing modes of action of antimicrobial peptides on bacteria. Curr. Top. Med. Chem. 16, 76–88. doi: 10.2174/1568026615666150703121009
Shibata, S., Takahashi, N., Chevance, F. F., Karlinsey, J. E., Hughes, K. T., and Aizawa, S. (2007). FliK regulates flagellar hook length as an internal ruler. Mol. Microbiol. 64, 1404–1415. doi: 10.1111/j.1365-2958.2007.05750.x
Silverman, M. R., and Simon, M. I. (1972). Flagellar assembly mutants in Escherichia coli. J. Bacteriol. 112, 986–993. doi: 10.1128/jb.112.2.986-993.1972
Sliman, R., Rehm, S., and Shlaes, D. M. (1987). Serious infections caused by Bacillus species. Medicine 66, 218–223. doi: 10.1097/00005792-198705000-00005
Steinmetz, M., and Richter, R. (1994). Easy cloning of mini-Tn10 insertions from the Bacillus subtilis chromosome. J. Bacteriol. 176, 1761–1763. doi: 10.1128/jb.176.6.1761-1763.1994
Stenbak, C. R., Ryu, J. H., Leulier, F., Pili-Floury, S., Parquet, C., Herve, M., et al. (2004). Peptidoglycan molecular requirements allowing detection by the Drosophila immune deficiency pathway. J. Immunol. 173, 7339–7348. doi: 10.4049/jimmunol.173.12.7339
Stenfors Arnesen, L. P., Fagerlund, A., and Granum, P. E. (2008). From soil to gut: Bacillus cereus and its food poisoning toxins. FEMS Microbiol. Rev. 32, 579–606. doi: 10.1111/j.1574-6976.2008.00112.x
Stenfors Arnesen, L. P., O’Sullivan, K., and Granum, P. E. (2007). Food poisoning potential of Bacillus cereus strains from Norwegian dairies. Int. J. Food Microbiol. 116, 292–296. doi: 10.1016/j.ijfoodmicro.2006.12.021
Stephens, J. M. (1952). Disease in codling moth larvae produced by several strains of Bacillus cereus. Can. J. Zool. 30, 30–40. doi: 10.1139/z52-002
Thedieck, K., Hain, T., Mohamed, W., Tindall, B. J., Nimtz, M., Chakraborty, T., et al. (2006). The MprF protein is required for lysinylation of phospholipids in listerial membranes and confers resistance to cationic antimicrobial peptides (CAMPs) on Listeria monocytogenes. Mol. Microbiol. 62, 1325–1339. doi: 10.1111/j.1365-2958.2006.05452.x
Vaz, F., Kounatidis, I., Covas, G., Parton, R. M., Harkiolaki, M., Davis, I., et al. (2019). Accessibility to peptidoglycan is important for the recognition of Gram-positive bacteria in Drosophila. Cell Rep. 27, 2480–2492.
Wood, T. K., Barrios, A. F. G., Herzberg, M., and Lee, J. (2006). Motility influences biofilm architecture in Escherichia coli. Appl. Microbiol. Biotechnol. 72, 361–367. doi: 10.1007/s00253-005-0263-8
Yang, S., Lee, C. W., Kim, H. J., Jung, H. H., Kim, J. I., Shin, S. Y., et al. (2019). Structural analysis and mode of action of BMAP-27, a cathelicidin-derived antimicrobial peptide. Peptides 118:170106. doi: 10.1016/j.peptides.2019.170106
Young, G. M., Schmiel, D. H., and Miller, V. L. (1999). A new pathway for the secretion of virulence factors by bacteria: the flagellar export apparatus functions as a protein-secretion system. Proc. Natl. Acad. Sci. U.S.A. 96, 6456–6461. doi: 10.1073/pnas.96.11.6456
Keywords: antimicrobial peptides, resistance, Bacillus thuringiensis, Drosophila melanogaster, virulence, FliK, flagella
Citation: Attieh Z, Mouawad C, Rejasse A, Jehanno I, Perchat S, Hegna IK, Økstad OA, Kallassy Awad M, Sanchis-Borja V and El Chamy L (2020) The fliK Gene Is Required for the Resistance of Bacillus thuringiensis to Antimicrobial Peptides and Virulence in Drosophila melanogaster. Front. Microbiol. 11:611220. doi: 10.3389/fmicb.2020.611220
Received: 28 September 2020; Accepted: 18 November 2020;
Published: 18 December 2020.
Edited by:
Jørgen J. Leisner, University of Copenhagen, DenmarkReviewed by:
Ioannis Eleftherianos, George Washington University, United StatesSamir Jaoua, Qatar University, Qatar
Copyright © 2020 Attieh, Mouawad, Rejasse, Jehanno, Perchat, Hegna, Økstad, Kallassy Awad, Sanchis-Borja and El Chamy. This is an open-access article distributed under the terms of the Creative Commons Attribution License (CC BY). The use, distribution or reproduction in other forums is permitted, provided the original author(s) and the copyright owner(s) are credited and that the original publication in this journal is cited, in accordance with accepted academic practice. No use, distribution or reproduction is permitted which does not comply with these terms.
*Correspondence: Vincent Sanchis-Borja, dmluY2VudC5zYW5jaGlzLWJvcmphQGlucmFlLmZy; Laure El Chamy, bGF1cmUuY2hhbXlAdXNqLmVkdS5sYg==