- 1Division of Immunology, Transplantation and Infectious Diseases, IRCCS San Raffaele Scientific Institute, Milan, Italy
- 2Antabio SAS, Labège, France
- 3Nurix Therapeutics, San Francisco, CA, United States
Chronic infection by Pseudomonas aeruginosa in cystic fibrosis (CF) patients is a major contributor to progressive lung damage and is poorly treated by available antibiotic therapy. An alternative approach to the development of additional antibiotic treatments is to identify complementary therapies which target bacterial virulence factors necessary for the establishment and/or maintenance of the chronic infection. The P. aeruginosa elastase (LasB) has been suggested as an attractive anti-virulence target due to its extracellular location, its harmful degradative effects on host tissues and the immune system, and the potential to inhibit its activity using small molecule inhibitors. However, while the relevance of LasB in acute P. aeruginosa infection has been demonstrated, it is still unclear whether this elastase might also play a role in the early phase of chronic lung colonization. By analyzing clinical P. aeruginosa clonal isolates from a CF patient, we found that the isolate RP45, collected in the early phase of persistence, produces large amounts of active LasB, while its clonal variant RP73, collected after years of colonization, does not produce it. When a mouse model of persistent pneumonia was used, deletion of the lasB gene in RP45 resulted in a significant reduction in mean bacterial numbers and incidence of chronic lung colonization at Day 7 post-challenge compared to those mice infected with wild-type (wt) RP45. Furthermore, deletion of lasB in strain RP45 also resulted in an increase in immunomodulators associated with innate and adaptive immune responses in infected animals. In contrast, deletion of the lasB gene in RP73 did not affect the establishment of chronic infection. Overall, these results indicate that LasB contributes to the adaptation of P. aeruginosa to a persistent lifestyle. In addition, these findings support pharmacological inhibition of LasB as a potentially useful therapeutic intervention for P. aeruginosa-infected CF patients prior to the establishment of a chronic infection.
Introduction
Pseudomonas aeruginosa is a Gram-negative opportunistic pathogen with a remarkable and threatening ability to thrive and adapt to various ecological niches (Stover et al., 2000). It causes significant morbidity and mortality, in particular in patients with acute nosocomial airway infections, such as ventilator-associated pneumonia, and with chronic respiratory diseases, including cystic fibrosis (CF), non-CF bronchiectasis, and chronic obstructive pulmonary disease (COPD; Emerson et al., 2002; Jacobs et al., 2020). The high incidence of these infections is ascribable to the large arsenal of virulence factors (e.g., proteases, pyocyanin, exotoxins) secreted by P. aeruginosa, which enables it to cause damage to the host and overcome host immune responses, thus promoting infection (Galdino et al., 2019b). The zinc metalloprotease elastase B (also called LasB or pseudolysin) is the most abundant protease in the P. aeruginosa secretome (Galdino et al., 2019b). Expression of this protease is regulated in a cell population density-dependent manner through cell-to-cell communication, i.e., “quorum sensing” (QS), and, after processing in the periplasm, the mature protein is secreted by the type II secretion system (T2SS; Michel et al., 2011). LasB can degrade components of the extracellular matrix, such as elastin, collagen, fibronectin, and mucins and components of the cellular junctions, such as vascular endothelial cadherin, inducing tissue injury, and bacterial dissemination (Golovkine et al., 2014; Flynn et al., 2017; Galdino et al., 2019b). It can also alter epithelial integrity and repair efficiency (Ruffin and Brochiero, 2019), thus favoring the spread of infection. In addition, LasB can inactivate components of the complement system, such as C3 (Bastaert et al., 2018), degrade other components of the immune defenses (e.g., interleukin 6 – IL-6; Saint-Criq et al., 2018), and interfere with bacterial killing by alveolar macrophages (Kuang et al., 2011; Bastaert et al., 2018), thus manipulating the host response to P. aeruginosa.
In the context of chronic respiratory diseases, lasB is expressed in the airway secretions of CF patients (Storey et al., 1992) and individuals with COPD during exacerbations (Murphy et al., 2008), suggesting a potential role in the progression of lung disease. Recently, LasB has also been shown to reduce expression of the CF transmembrane conductance regulator (CFTR) and its activity in epithelial cells in vitro (Saint-Criq et al., 2018). Defective CFTR functionality has been associated with failure to eradicate inhaled bacteria (Cohen and Prince, 2012); thus, it is conceivable that CFTR impairment by LasB could favor the establishment of chronic P. aeruginosa colonization not only in CF patients but also in patients with COPD and non-CF bronchiectasis. In this regard, deletion of lasB was shown to lead to a less invasive infection in a mouse model of acute pneumonia when compared to the infection caused by the respective wt strain (Golovkine et al., 2014), confirming the relevance of LasB in pathogenesis of P. aeruginosa infection. The most common animal model used so far to assess the relevance of LasB reproduces acute respiratory infection (Bastaert et al., 2018; Qu et al., 2018; Saint-Criq et al., 2018), but a chronic infection model has not been previously used. Thus, it is not clear whether and how LasB is involved in the establishment of chronic colonization.
In this study, we investigated the impact of LasB in chronic P. aeruginosa lung colonization with the aim to (1) determine whether LasB plays a role in the establishment of chronic lung colonization in a mouse model and (2) establish how LasB affects the host immune response.
Materials and Methods
Ethics Statement
Animal studies adhered to the Italian Ministry of Health guidelines for the use and care of experimental animals (IACUC #879). Research with P. aeruginosa isolates from CF individuals and storage of biological materials were approved by the Ethics Commission of Hannover Medical School, Germany.
Bacterial Strains
P. aeruginosa strains included the reference strain PAO1 (Cigana et al., 2020), as well as CF isolate RP45 (Jeukens et al., 2013; Bianconi et al., 2015) and its late pathoadaptive variant RP73 isolated 7 years after RP45 from the same CF patient (Bianconi et al., 2015; Cigana et al., 2016a, 2020).
Construction of lasB-Deleted (∆lasB) Mutants in P. aeruginosa PAO1, RP45, and RP73 Strains
The lasB gene was deleted by homologus recombination according to the method reported by Kaniga et al. (1991). Upstream and downstream regions (700 bp in length) flanking lasB were obtained by gene synthesis (GeneArt®) and cloned into the suicide vector pKNG101 as a BamHI/SpeI fragment. These regions were specific for strains PAO1, RP45, and RP73 as some variations were observed. Escherichia coli CC118λpir was used as host for pKNG101 plasmids. The recombinant plasmids were further transferred into P. aeruginosa strains by conjugation and transconjugants were selected on Pseudomonas isolation agar (PIA) plates containing streptomycin at 2,000 μg/ml. Negative selection was further performed on Luria-Bertani (LB) medium supplemented with 5% sucrose. Allelic exchanges were screened by PCR, and deletion of the1,497 bp lasB gene was confirmed by sequencing.
Elastolytic Assay (Elastin Congo Red Method) to Evaluate LasB Activity in P. aeruginosa Strains
This assay measures the elastolytic activity of secreted LasB in the supernatant of P. aeruginosa cultures using elastin congo red (ECR, Sigma) as substrate. LasB degrades elastin and releases the congo red dye into the supernatant which can then be measured spectrophotometrically. Overnight cultures of P. aeruginosa (PAO1, RP45, and RP73) wt and ∆lasB strains were diluted in LB medium. After reaching an OD600nm of 0.6, cultures were diluted 1:100 and incubated for an additional 24 h in a shaking incubator. The culture supernatants were recovered by centrifugation and filtered through a 0.22 μm filter. The supernatants (diluted 1:10 in LB medium) were then mixed 1:1 with 2X ECR solution, resulting in a final concentration of 10 mg/ml ECR in 50 mM Tris-HCl pH 7.4 and 0.5 mM CaCl2, and incubated for 20 h in a 37°C shaking incubator. The supernatants were recovered by centrifugation, and the released congo red dye was quantitated by its absorbance at 495 nm (OD495nm). As a negative control, supernatants were replaced by LB medium. Statistical analyses were performed according to Student t-test using values obtained with LB medium as reference.
Mouse Model of Chronic Lung Infection
C57BL/6NCrlBR male mice (8–10 weeks, Charles River) were challenged by intratracheal (i.t.) administration with an average of 5.6 × 105 colony forming units (CFUs) of the wt and ∆lasB RP45 strains or 3 × 105 CFUs of the wt and ∆lasB RP73 strains embedded in agar-beads (Bragonzi et al., 2009; Facchini et al., 2014; Cigana et al., 2016b) or with sterile empty agar-beads as control. Body weight and health status were monitored daily. Lung CFUs were analyzed at Days 2 and 7 post-infection. As reported previously, recovery of ≥1,000 CFUs of P. aeruginosa from Day 7 lung cultures is considered evidence that a chronic infection has been established (Lore et al., 2016, 2018). Cytokine/chemokine/growth factor levels were measured in the supernatant of lung homogenates by Bio-Plex Assay (Bio-Rad), as described in the online data supplement. Additional details in accordance with the ARRIVE guidelines (Kilkenny et al., 2010) are reported in the online data supplement.
Statistics
Statistical analyses were performed with GraphPad Prism (GraphPad Software, Inc., San Diego, CA, USA), using Student t-test for the elastolytic activity, a two-way analysis of variance (ANOVA) with Bonferroni’s multiple comparison test for body weight changes, Fisher’s exact test for the incidence of chronic colonization and survival, and nonparametric two-tailed Mann-Whitney test for the CFUs and levels of chemokines/cytokines/growth factors. Outlier data, identified by Grubbs’ test, were excluded from the analysis. A value of p < 0.05 was considered to be statistically significant.
Results
LasB Production and Activity Differ Among P. aeruginosa Strains
The LasB elastolytic activities of culture supernatants from P. aeruginosa reference strain PAO1 and clinical isolates RP45 and RP73, collected from a CF patient at different periods following the onset of lung colonization, were investigated by the ECR method (Figure 1). Whereas the RP45 isolate displayed a strong LasB activity (p = 0.007 for PAO1 vs. LB medium, p = 0.036 for RP45 vs. LB medium), the later clonal variant RP73 (isolated 7 years after RP45) showed no elastolytic activity, despite the presence of LasB protein being confirmed in cultures supernatants by Western blot (Supplementary Figure S1A). The loss of LasB activity is in accordance to the pathoadaptation of P. aeruginosa previously observed for some strains during development of chronic infection in CF (Bianconi et al., 2015). Since all three strains (PAO1, RP45, and RP73) have identical lasB sequences, the lack of activity in the RP73 variant is possibly due to incorrect processing of the mature protein. As expected, all the corresponding ∆lasB mutants displayed no elastolytic activity (Figure 1).
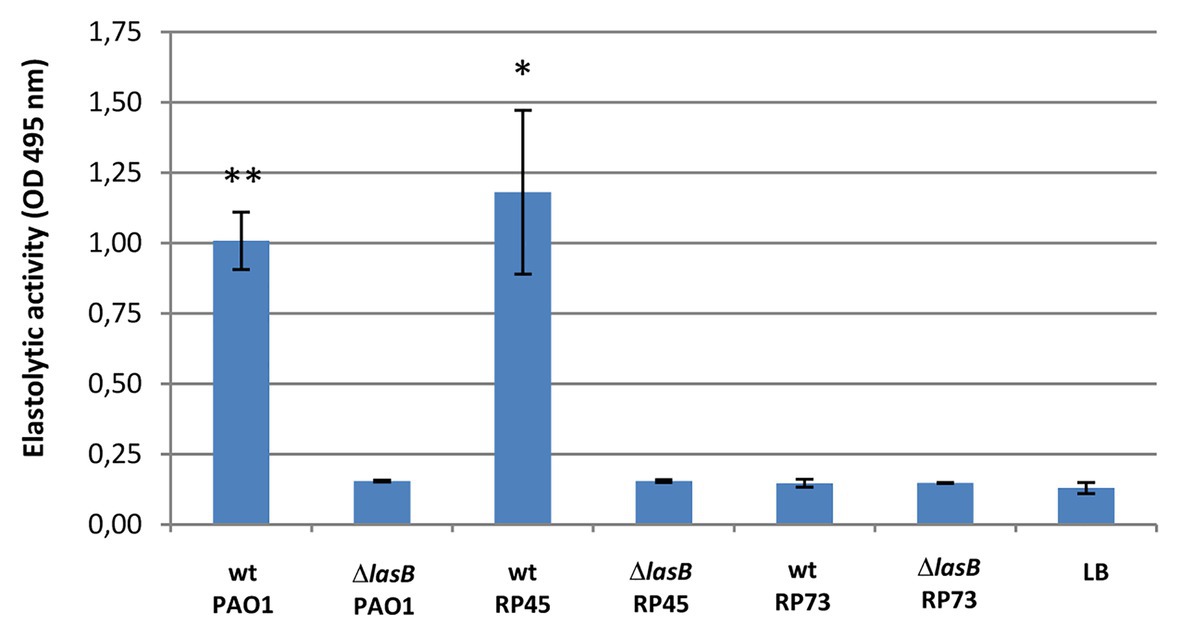
Figure 1. LasB elastolytic activity in culture supernatants from Pseudomonas aeruginosa PAO1 and cystic fibrosis (CF) isolates RP45 and RP73 [wild-type (wt) vs. ∆lasB mutants]. LasB activity in bacterial supernatants (prepared by centrifugation and filtration after 24 h of growth and diluted to 1:10) was evaluated by Elastolytic assay (ECR method, after 20 h of incubation with ECR substrate). Luria-Bertani (LB) growth medium was used as negative control. The data were pooled from two independent experiments in quadruplicate (n = 8 per strain). Statistical significance is indicated as follows: *p < 0.05; **p < 0.01.
LasB Promotes Chronic P. aeruginosa Lung Colonization
In order to investigate the role of LasB in chronic lung colonization by P. aeruginosa, a ∆lasB mutant was generated for the RP45 strain. Mice were infected with wt or ∆lasB RP45 bacteria embedded in agar beads and monitored daily for body weight and health status. Identical rates of survival (60%) were observed for mice infected with the wt RP45 strain and those infected with the ∆lasB mutant counterpart (Figure 2A; Supplementary Figure S2). Both infected groups of animals lost significantly more body weight than mice challenged with sterile empty beads, although no differences were observed in the loss or recovery of body weight between mice infected with wt or ∆lasB RP45 strains (Figure 2B). At Day 2 post-infection, the lung bacterial burden did not differ between mice infected with wt or ∆lasB RP45 strains. However, at Day 7 post-infection, mice infected with the ∆lasB RP45 strain showed significantly lower mean lung CFUs than those infected with the wt counterpart (p = 0.0329, Figure 2C). This reflected the higher capacity of clearance of RP45 ∆lasB (61%) vs. the wt strain (14%), which resulted in a lower incidence of chronic colonization (p = 0.0045, Figure 2D). This suggests that LasB is involved in the establishment of chronic colonization in this model. Mice infected with the wt RP45 strain harboring the highest bacterial load at Day 2 post-infection had detectable LasB by Western blot in lung homogenates (Supplementary Figure S1B) although enzymatic activity could not be detected in these samples (data not shown), perhaps due to the presence of inhibitors in the homogenate and/or limitations in the assay sensitivity. In addition, a ∆lasB mutant was generated for the late adapted strain RP73. The survival rate of mice infected with wt RP73 strain was similar to that of mice infected with ∆lasB RP73 bacteria (≈90%; Figure 3A) but higher than that of mice infected with wt RP45 strain, as previously shown (Bianconi et al., 2015). No difference in changes in body weight was observed between wt or ∆lasB RP73-infected mice (Figure 3B). The lung bacterial burden in mice infected with wt RP73 strain was similar to that in mice infected with ∆lasB RP73 strain (≈5 × 104 median CFUs; Figure 3C) but higher than that in mice infected with wt RP45 bacteria (≈7.7 × 103 median CFUs). When the incidence of chronic colonization was analyzed, no difference was observed between mice infected with wt or ∆lasB RP73 bacteria (Figure 3D), probably due to the absence of LasB activity in RP73. Overall, these results indicate that LasB can contribute to the establishment of chronic lung colonization in CF patients, but its activity could be dispensable in strains already well-adapted to a chronic infection lifestyle.
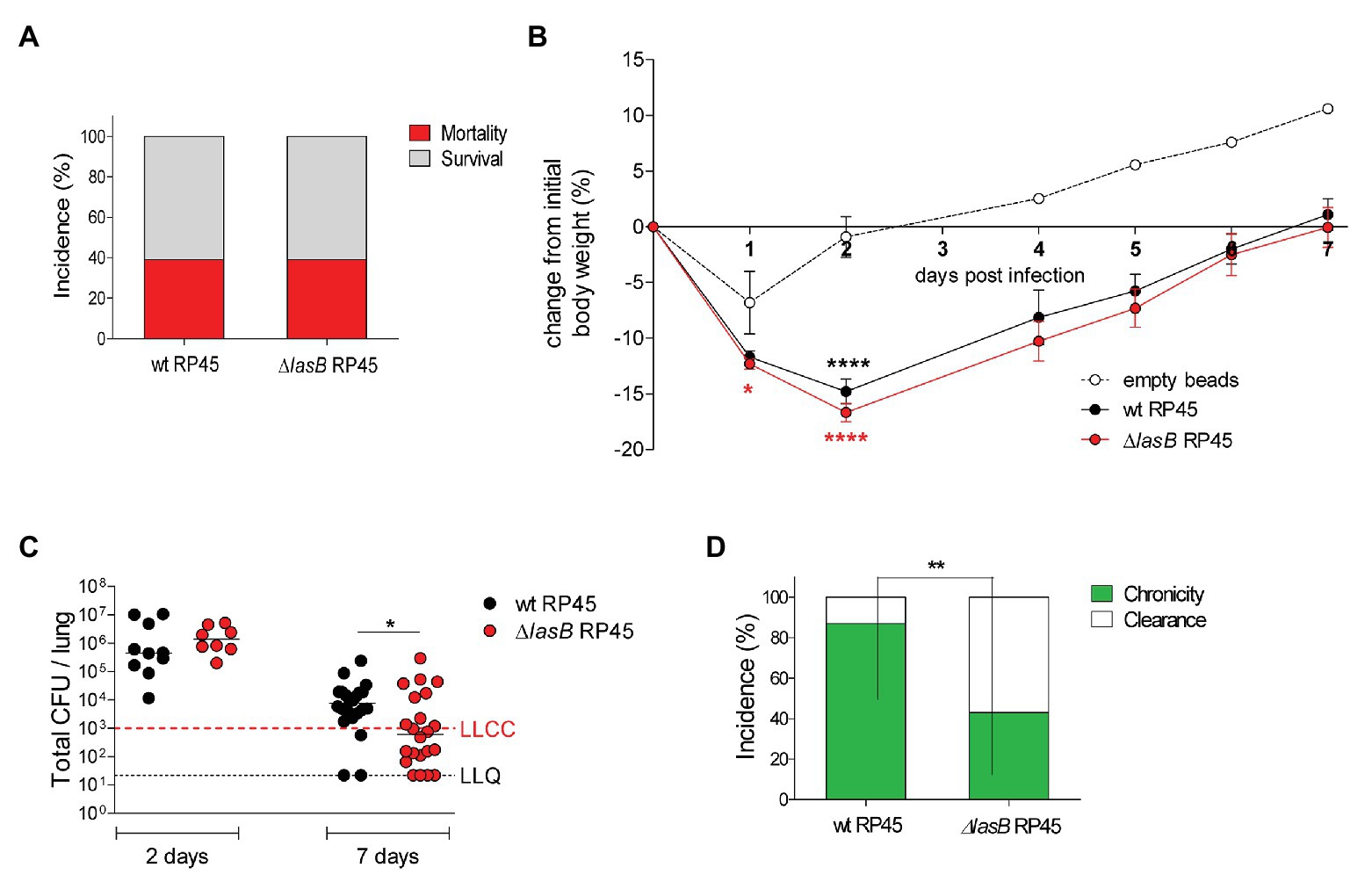
Figure 2. Impact of chronic lung infection by wt and ∆lasB mutant P. aeruginosa RP45 strains on host response. C57BL/6NCrlBR mice were infected with an average of 5.6 × 105 colony forming units (CFUs) embedded in agar beads or with sterile empty beads. Mice were monitored daily for survival and body weight. At Days 2 and 7 post-infection, mice were euthanized, and lungs were excised and homogenized. (A) The incidences of survival and mortality were determined on challenged mice and expressed as percentage. (B) Body weight data are expressed as mean ± standard error of the mean (SEM) of the weight change from the initial body weight. (C) CFUs were evaluated in the lungs after plating onto tryptic soy agar at Days 2 and 7 post-infection. Dots represent values of individual mice, and horizontal lines represent median values. The lower limit of chronic colonization (LLCC) and the lower limit of quantification (LLQ) are indicated. (D) The incidence of clearance (<1,000 P. aeruginosa CFUs in the lung cultures) and capacity to establish chronic airways infection (≥1,000 P. aeruginosa CFUs in the lung cultures) were determined on surviving mice at Day 7 post-infection and expressed as percentage. The data were pooled from three independent experiments (mice nr = 38 per strain). Statistical significance is indicated as follows: *p < 0.05; **p < 0.01; ****p < 0.0001.
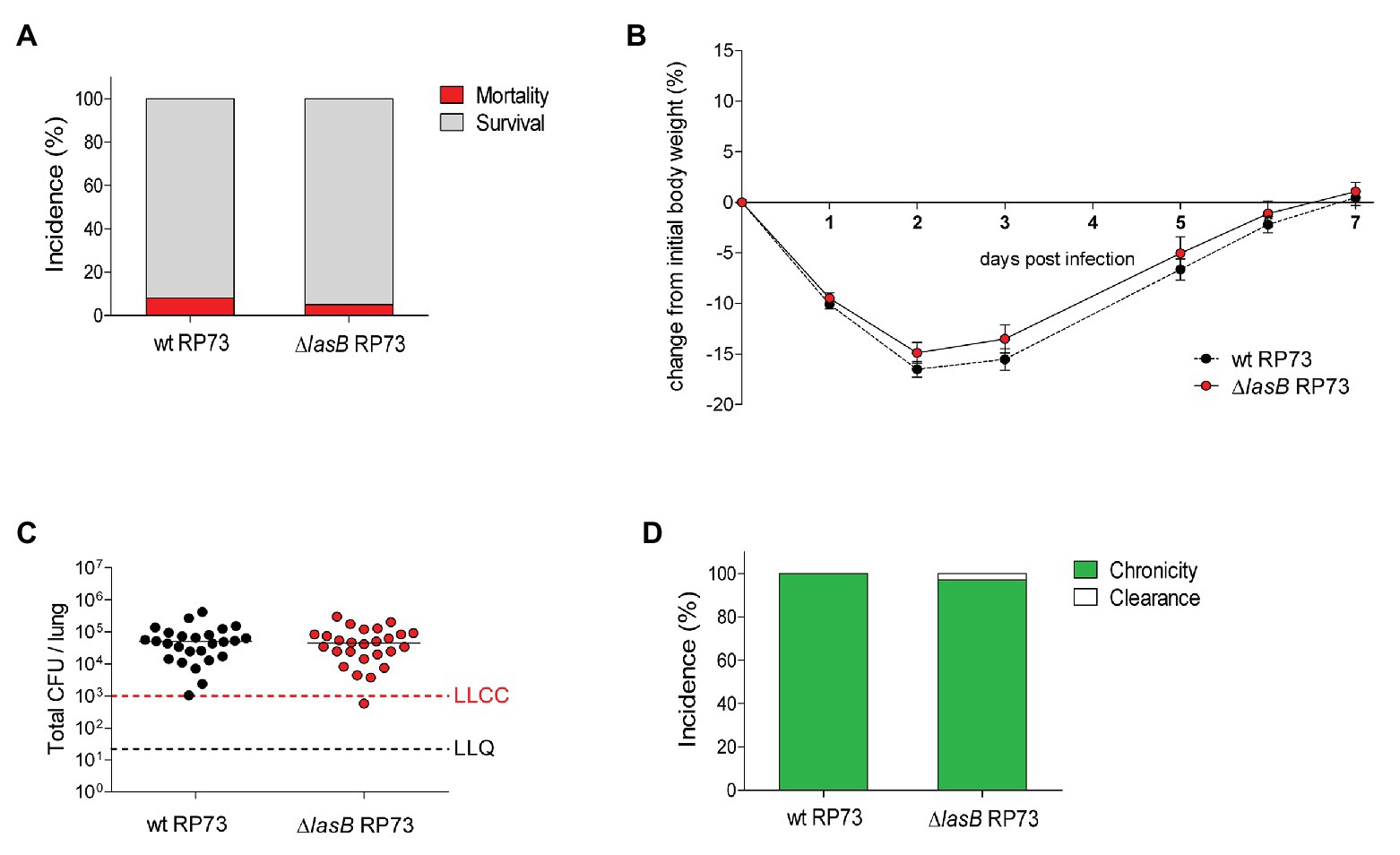
Figure 3. Impact of chronic lung infection by wt and ∆lasB mutant P. aeruginosa RP73 strains on host response. C57BL/6NCrlBR mice were infected with an average of 3 × 105 CFUs/lung embedded in agar beads. Mice were monitored daily for survival and body weight. At Day 7 post-infection, mice were euthanized, and lungs were excised and homogenized. (A) The incidences of survival and mortality were determined on challenged mice and expressed as percentage. (B) Body weight data are expressed as mean ± standard error of the mean (SEM) of the weight change from the initial body weight. (C) CFUs were evaluated in the lungs after plating onto tryptic soy agar at Day 7 post-infection. Dots represent values of individual mice, and horizontal lines represent median values. The lower limit of chronic colonization (LLCC) and the lower limit of quantification (LLQ) are indicated. (D) The incidence of clearance (<1,000 CFUs of P. aeruginosa in the lung cultures) and capacity to establish chronic airways infection (≥1,000 CFUs of P. aeruginosa in the lung cultures) were determined on surviving mice at Day 7 post-infection and expressed as percentage. The data were pooled from three independent experiments (mice nr = 28–29).
LasB Affects Inflammation Induced by Chronic P. aeruginosa Lung Colonization
In order to evaluate whether LasB could affect inflammation, the levels of several chemokines/cytokines and growth factors were measured in the supernatants of lung homogenates from mice infected with wt or ∆lasB RP45 strains at Day 7 post-infection. Four cytokines/chemokines (IL-5, IL-10, IL-13, and granulocyte-macrophage colony-stimulating factor – GM-CSF) were undetectable in the majority of the samples; thus, they were excluded from further analysis. The ∆lasB RP45 strain induced significantly higher levels of IL-1α, IL-1β, IL-3, IL-12p70, IL-17A, eotaxin, granulocyte colony-stimulating factor (G-CSF), macrophage inflammatory protein 1α (MIP-1α), MIP-1β, and RANTES in comparison to the wt counterpart (Table 1), indicating that LasB can downregulate inflammation. These differences did not correlate with the bacterial burden, which is not surprising since mice infected with the ∆lasB RP45 strain showed higher levels of clearance in comparison to those infected with wt RP45. When the analysis was limited to those mice still colonized at Day 7 post-infection, the ∆lasB RP45 strain induced significantly higher levels of IL-1α, IL-1β, IL-9, IL-12p70, G-CSF, MIP-1α, MIP-1β, RANTES, and tumor necrosis factor (TNF)-α in comparison to the wt RP45 strain (Supplementary Table S1), confirming the immunomodulatory potential of LasB.
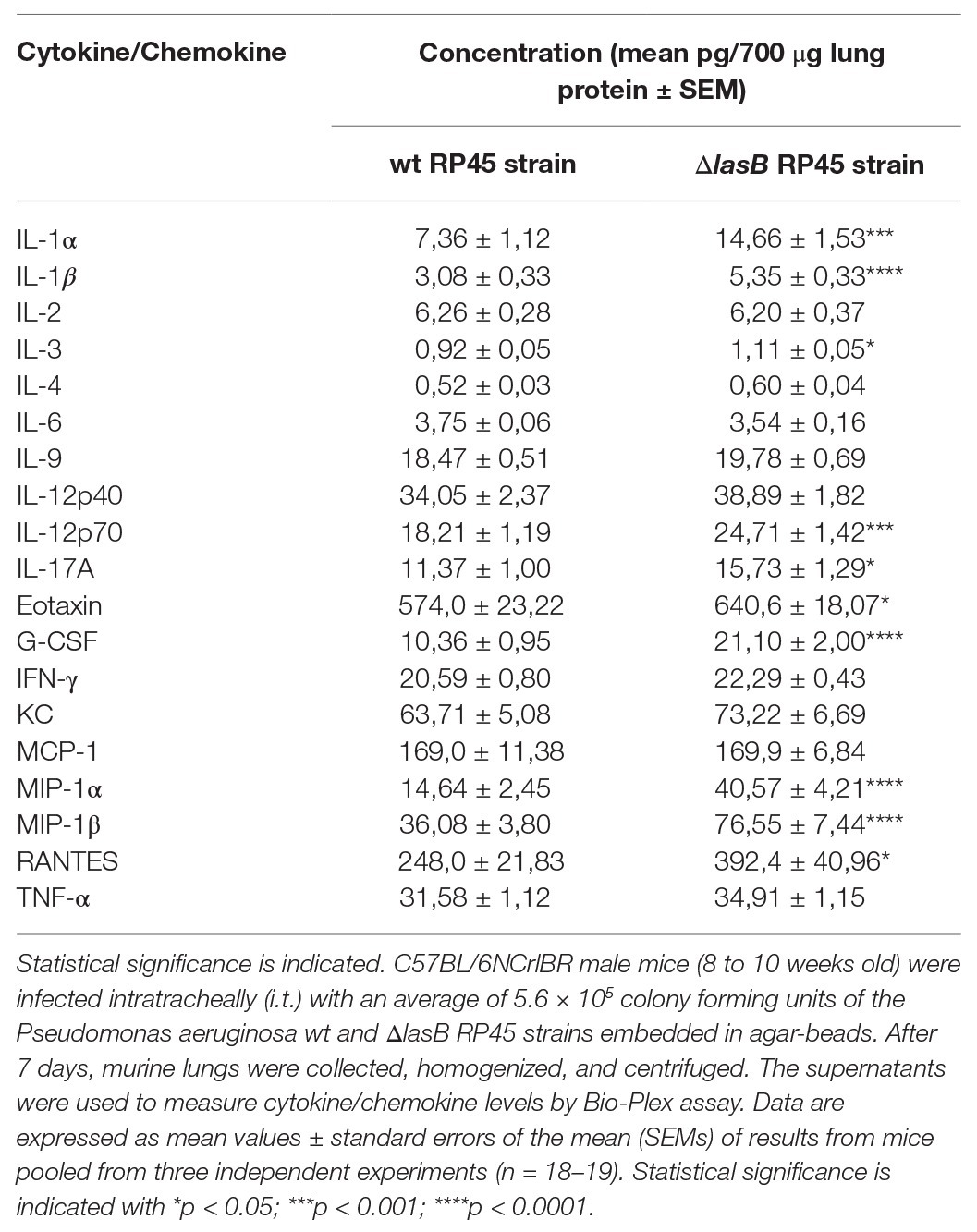
Table 1. Cytokine/chemokine concentrations following chronic airway infection by wt and ΔlasB RP45 strains.
Discussion
P. aeruginosa infections pose a major threat to patients with chronic respiratory infections, such as those with CF or COPD (Emerson et al., 2002; Jacobs et al., 2020). The metalloprotease LasB, the most abundant protein in the P. aeruginosa secretome (Saint-Criq et al., 2018; Galdino et al., 2019b), has been shown to interfere with several pathophysiological pathways. In addition to causing tissue injury and manipulating the immune response (Golovkine et al., 2014; Bastaert et al., 2018; Saint-Criq et al., 2018; Galdino et al., 2019b; Ruffin and Brochiero, 2019), it also reduces the expression and activity of CFTR (Saint-Criq et al., 2018). This evidence assumes considerable relevance as LasB-producing P. aeruginosa strains have been commonly isolated in CF sputum secretions (Storey et al., 1992, 1997; Tingpej et al., 2007). However, most of the evidence supporting the role of LasB is based on in vitro studies or animal models of acute infection. In this work, we exploited the mouse model of chronic lung infection, involving the i.t. inoculation of bacteria embedded in agar-beads, to evaluate the effect of LasB on the establishment of chronic colonization. Agar-beads have been shown to reproduce a microaerobic environment that allows bacteria to grow in the form of microcolonies, similar to growth in the mucus of CF patients (Bragonzi et al., 2005). Two clonal P. aeruginosa clinical isolates, collected from a CF patient at different stages of chronic infection, and their corresponding ∆lasB mutants were used in these studies. We had previously demonstrated that the RP45 strain induced mortality in half of infected mice and caused chronic infection in approximately 80% of the surviving mice, whereas the RP73 strain, isolated 7 years after RP45, possessed several pathoadaptive traits, in that it was non-lethal and exhibited an extraordinary capacity to establish a long-term chronic lung infection (colonizing almost all infected mice; Bianconi et al., 2015). Here, we show that deletion of lasB in the RP45 strain did not affect the rate of survival of infected mice or their body weight as a parameter of mouse health. Surviving mice, including those chronically colonized, recovered body weights, suggesting the establishment of an equilibrium between bacterial growth and containment by the host response. However, despite similar percent survival and loss of body weight, a key finding was that deletion of lasB resulted in a lower incidence of chronic lung colonization, indicating that LasB may play a key role in the early stage of the adaptation of P. aeruginosa to chronic colonization. Interestingly, no difference in the bacterial load, between wt and ∆lasB RP45 infected mice, was observed in those animals with an established chronic infection at Day 7 (defined as those harboring
No difference was observed in the incidence of chronic colonization between mice infected with wt or ∆lasB RP73 strains at Day 7 post-infection. The wt RP73 strain does not produce an active LasB protein. The high incidence of chronic infection is consistent with the RP73 strain being isolated from a patient with a long-term chronic lung infection and thus well adapted to a chronic infection lifestyle in the CF lung. Indeed, P. aeruginosa undergoes a well-known evolutionary adaptation during long-term colonization in the CF lung, which is sustained by bacterial lineages clonal to the initially acquired strain and carrying pathoadaptive phenotypic traits. The phenomenon of adaptation to the CF lung allows bacteria to face a complex environment, characterized by a thick and viscous mucus, in which intense selective pressures are exerted by components of the host immune response, variable availability of nutrients and oxygen, and continuous antibiotic treatments (Rossi et al., 2020). RP73 strain has been shown to possess several features of adaptation to the CF airways that make it highly efficient in chronically colonizing the host (Bianconi et al., 2015). The fact that RP73 no longer requires an active LasB protein in order to cause chronic infection further supports the hypothesis that LasB may only play a role in the early adaptation to a persistent lifestyle and subsequent chronic infection rather than in its maintenance. These results are consistent with works reporting that the LasB expression and activity are more frequently described in early stage sputum isolates compared to those from chronic infections in CF patients (Tingpej et al., 2007; Harmer et al., 2013). A potential mechanistic explanation for this is offered by the observations that (i) LasB expression is required for degradation of complex substrates, such as mucins, during early infections when nutrients are in short supply (Flynn et al., 2017) and (ii) LasB expression is suppressed under anaerobic conditions associated with severe and chronic infections (Lee et al., 2011). Thus, LasB may be important for establishing an early (aerobic) infection, through tissue invasion, degradation of host immune response factors, and supply of growth substrates but is then surplus to requirement during subsequent chronic (anaerobic) infection, characterized by compromised host defenses and abundant free amino-acids.
Analyses of the inflammatory response in the murine lung show that deletion of lasB increased cytokines, chemokines, and growth factors is associated with both innate and adaptive immune responses. In particular, lasB deletion was associated with an increase in the production of factors related to the recruitment of neutrophils (e.g., GM-CSF, MIP-1α), monocytes/macrophages (e.g., MIP-1β), natural killer cells (e.g., IL-12p70, MIP-1β) and T cells (e.g., RANTES), or differentiation of T helper 1 (e.g., IL-12p70) and T helper 17 cells (e.g., IL-17A). This indicates that LasB can cause immunomodulation by either direct degradation of immune mediators or indirect downregulation of their production. However, in contrast to the data reported by Saint-Criq and colleagues (Saint-Criq et al., 2018), we did not observe a downregulation of IL-6 in this study. This could be explained by the timing of the analysis (in addition to the other variables such as strain, mouse model, and inoculum size); whereas Saint-Criq and colleagues measured IL-6 levels after 24 h of acute infection, we analyzed concentrations 7 days after inoculation in the mouse model of chronic lung infection. The cytokine levels observed did not positively correlate with the bacterial loads, which may be because mice infected with the ∆lasB RP45 strain showed higher levels of clearance than those infected with wt RP45. We hypothesize that the increased immune response facilitated by deletion of lasB may have contributed to the higher incidence of bacterial clearance. Further studies, based on the analysis of cellular recruitment and on the inhibition or stimulation of specific immune components, in particular in the early phases of infection/colonization, may be helpful to dissect further the molecular mechanism underlying LasB-mediated immunomodulation. Others have demonstrated that LasB is able to compromise various host immune response factors and that LasB deficiency or inhibition can lead to increased survival or reductions in bacterial burden in mouse models of acute lung infection when a non-lethal bacterial inoculum has been used (Kuang et al., 2011; Bastaert et al., 2018; Qu et al., 2018; Saint-Criq et al., 2018); however, to our knowledge, this is the first study to address the impact of LasB on the incidence of chronic colonization. Future studies could further corroborate its impact on chronic colonization using additional P. aeruginosa clonal lineages.
Overall, our findings indicate that LasB can compromise the immune response, thereby promoting the persistence of P. aeruginosa in the lung. These data support ongoing investigations of LasB-targeted therapeutic approaches to reduce the establishment of chronic P. aeruginosa colonization (Galdino et al., 2019a).
Data Availability Statement
The raw data supporting the conclusions of this article will be made available by the authors, without undue reservation.
Ethics Statement
The animal study was reviewed and approved by IRCCS San Raffaele Scientific Institute (Milan, Italy), Institutional Animal Care and Use Committee (IACUC #733 and #878) and adhered strictly to the Italian Ministry of Health guidelines for the use and care of experimental animals.
Author Contributions
CC, AB, NS, MZ, and ME contributed to conception and design of the study and interpreted the data. CC, MM, SR, BA-F, and AR performed in vivo experiments and collected data. CC and JC analyzed the data, performed statistical analysis, and prepared the figures and tables. JC and LB performed Western blot and evaluated LasB activity. CC wrote the first draft of the manuscript. AB, MZ, and ME critically revised the manuscript. All the authors read, revised and approved the final manuscript.
Funding
JC, NS, LB, MZ, and ME are present or past employees of Antabio. Research reported in this publication is supported by the Cooperative Agreement Number IDSEP160030 from ASPR/BARDA and by awards from Wellcome Trust and Germany’s Federal Ministry of Education and Research, as administrated by CARB-X. The content is solely the responsibility of the authors and does not necessarily represent the official views of the Department of Health and Human Services Office of the Assistant Secretary for Preparedness and Response, other funders, or CARB-X.
Conflict of Interest
The authors declare that this study received funding from Antabio SAS (France) with financial support from Cooperative Agreement Number IDSEP160030 from ASPR/BARDA and by awards from Wellcome Trust and Germany’s Federal Ministry of Education and Research, as administrated by CARB-X. Antabio had the following involvement in the study: study concept and design, interpretation of data, writing of the article. CARB-X was not involved in the study design, collection, analysis, and interpretation of data, the writing of this article or the decision to submit it for publication. The content is solely the responsibility of the authors and does not necessarily represent the official views of the Department of Health and Human Services Office of the Assistant Secretary for Preparedness and Response, other funders, or CARB-X.
Author SR was employed by company Nurix Therapeutics. The remaining authors declare that the research was conducted in the absence of any commercial or financial relationships that could be construed as a potential conflict of interest.
Acknowledgments
We would like to thank B. Tümmler (Hannover Medical School, Hannover, Germany) for supplying the P. aeruginosa strains from CF patients, E. Kessler (Tel Aviv University, Israel) for kindly providing the anti-LasB polyclonal antibody, and Smaltis (Besançon, France) for construction of ∆lasB mutants. We also wish to thank the Wellcome Trust and CARB-X for funding and supporting this research.
Supplementary Material
The Supplementary Material for this article can be found online at: https://www.frontiersin.org/articles/10.3389/fmicb.2020.620819/full#supplementary-material
References
Bastaert, F., Kheir, S., Saint-Criq, V., Villeret, B., Dang, P. M., El-Benna, J., et al. (2018). Pseudomonas aeruginosa LasB subverts alveolar macrophage activity by interfering with bacterial killing through downregulation of innate immune defense, reactive oxygen species generation, and complement activation. Front. Immunol. 9:1675. doi: 10.3389/fimmu.2018.01675
Bianconi, I., Jeukens, J., Freschi, L., Alcala-Franco, B., Facchini, M., Boyle, B., et al. (2015). Comparative genomics and biological characterization of sequential Pseudomonas aeruginosa isolates from persistent airways infection. BMC Genomics 16:1105. doi: 10.1186/s12864-015-2276-8
Bragonzi, A., Paroni, M., Nonis, A., Cramer, N., Montanari, S., Rejman, J., et al. (2009). Pseudomonas aeruginosa microevolution during cystic fibrosis lung infection establishes clones with adapted virulence. Am. J. Respir. Crit. Care Med. 180, 138–145. doi: 10.1164/rccm.200812-1943OC
Bragonzi, A., Worlitzsch, D., Pier, G. B., Timpert, P., Ulrich, M., Hentzer, M., et al. (2005). Nonmucoid Pseudomonas aeruginosa expresses alginate in the lungs of patients with cystic fibrosis and in a mouse model. J. Infect. Dis. 192, 410–419. doi: 10.1086/431516
Cigana, C., Bernardini, F., Facchini, M., Alcala-Franco, B., Riva, C., De Fino, I., et al. (2016a). Efficacy of the novel antibiotic POL7001 in preclinical models of Pseudomonas aeruginosa pneumonia. Antimicrob. Agents Chemother. 60, 4991–5000. doi: 10.1128/AAC.00390-16
Cigana, C., Lore, N. I., Riva, C., De Fino, I., Spagnuolo, L., Sipione, B., et al. (2016b). Tracking the immunopathological response to Pseudomonas aeruginosa during respiratory infections. Sci. Rep. 6:21465. doi: 10.1038/srep21465
Cigana, C., Ranucci, S., Rossi, A., De Fino, I., Melessike, M., and Bragonzi, A. (2020). Antibiotic efficacy varies based on the infection model and treatment regimen for Pseudomonas aeruginosa. Eur. Respir. J. 55:1802456. doi: 10.1183/13993003.02456-2018
Cohen, T. S., and Prince, A. (2012). Cystic fibrosis: a mucosal immunodeficiency syndrome. Nat. Med. 18, 509–519. doi: 10.1038/nm.2715
Emerson, J., Rosenfeld, M., McNamara, S., Ramsey, B., and Gibson, R. L. (2002). Pseudomonas aeruginosa and other predictors of mortality and morbidity in young children with cystic fibrosis. Pediatr. Pulmonol. 34, 91–100. doi: 10.1002/ppul.10127
Facchini, M., De Fino, I., Riva, C., and Bragonzi, A. (2014). Long term chronic Pseudomonas aeruginosa airway infection in mice. J. Vis. Exp. 17:e51019. doi: 10.3791/51019
Flynn, J. M., Phan, C., and Hunter, R. C. (2017). Genome-wide survey of Pseudomonas aeruginosa PA14 reveals a role for the glyoxylate pathway and extracellular proteases in the utilization of mucin. Infect. Immun. 85, e00182–e00117. doi: 10.1128/IAI.00182-17
Galdino, A. C. M., de Oliveira, M. P., Ramalho, T. C., de Castro, A. A., Branquinha, M. H., and Santos, A. L. S. (2019a). Anti-virulence strategy against the multidrug-resistant bacterial pathogen Pseudomonas aeruginosa: pseudolysin (elastase B) as a potential druggable target. Curr. Protein Pept. Sci. 20, 471–487. doi: 10.2174/1389203720666190207100415
Galdino, A. C. M., Viganor, L., de Castro, A. A., da Cunha, E. F. F., Mello, T. P., Mattos, L. M., et al. (2019b). Disarming Pseudomonas aeruginosa virulence by the inhibitory action of 1,10-phenanthroline-5,6-dione-based compounds: elastase B (LasB) as a chemotherapeutic target. Front. Microbiol. 10:1701. doi: 10.3389/fmicb.2019.01701
Golovkine, G., Faudry, E., Bouillot, S., Voulhoux, R., Attree, I., and Huber, P. (2014). VE-cadherin cleavage by LasB protease from Pseudomonas aeruginosa facilitates type III secretion system toxicity in endothelial cells. PLoS Pathog. 10:e1003939. doi: 10.1371/journal.ppat.1003939
Harmer, C., Alnassafi, K., Hu, H., Elkins, M., Bye, P., Rose, B., et al. (2013). Modulation of gene expression by Pseudomonas aeruginosa during chronic infection in the adult cystic fibrosis lung. Microbiology 159, 2354–2363. doi: 10.1099/mic.0.066985-0
Jacobs, D. M., Ochs-Balcom, H. M., Noyes, K., Zhao, J., Leung, W. Y., Pu, C. Y., et al. (2020). Impact of Pseudomonas aeruginosa isolation on mortality and outcomes in an outpatient chronic obstructive pulmonary disease cohort. Open Forum Infect. Dis. 7:ofz546. doi: 10.1093/ofid/ofz546
Jeukens, J., Boyle, B., Bianconi, I., Kukavica-Ibrulj, I., Tummler, B., Bragonzi, A., et al. (2013). Complete genome sequence of persistent cystic fibrosis isolate Pseudomonas aeruginosa strain RP73. Genome Announc. 1, e00568–e00513. doi: 10.1128/genomeA.00568-13
Kaniga, K., Delor, I., and Cornelis, G. R. (1991). A wide-host-range suicide vector for improving reverse genetics in gram-negative bacteria: inactivation of the blaA gene of Yersinia enterocolitica. Gene 109, 137–141. doi: 10.1016/0378-1119(91)90599-7
Kilkenny, C., Browne, W. J., Cuthill, I. C., Emerson, M., and Altman, D. G. (2010). Improving bioscience research reporting: the ARRIVE guidelines for reporting animal research. PLoS Biol. 8:e1000412. doi: 10.1371/journal.pbio.1000412
Kuang, Z., Hao, Y., Walling, B. E., Jeffries, J. L., Ohman, D. E., and Lau, G. W. (2011). Pseudomonas aeruginosa elastase provides an escape from phagocytosis by degrading the pulmonary surfactant protein-A. PLoS One 6:e27091. doi: 10.1371/journal.pone.0027091
Lee, K. M., Yoon, M. Y., Park, Y., Lee, J. H., and Yoon, S. S. (2011). Anaerobiosis-induced loss of cytotoxicity is due to inactivation of quorum sensing in Pseudomonas aeruginosa. Infect. Immun. 79, 2792–2800. doi: 10.1128/IAI.01361-10
Lore, N. I., Cigana, C., Riva, C., De Fino, I., Nonis, A., Spagnuolo, L., et al. (2016). IL-17A impairs host tolerance during airway chronic infection by Pseudomonas aeruginosa. Sci. Rep. 6:25937. doi: 10.1038/srep25937
Lore, N. I., Veraldi, N., Riva, C., Sipione, B., Spagnuolo, L., De Fino, I., et al. (2018). Synthesized heparan sulfate competitors attenuate Pseudomonas aeruginosa lung infection. Int. J. Mol. Sci. 19:207. doi: 10.3390/ijms19010207
Michel, G. P. F., Aguzzi, A., Ball, G., Soscia, C., Bleves, S., and Voulhoux, R. (2011). Role of fimV in type II secretion system-dependent protein secretion of Pseudomonas aeruginosa on solid medium. Microbiology 157, 1945–1954. doi: 10.1099/mic.0.045849-0
Murphy, T. F., Brauer, A. L., Eschberger, K., Lobbins, P., Grove, L., Cai, X., et al. (2008). Pseudomonas aeruginosa in chronic obstructive pulmonary disease. Am. J. Respir. Crit. Care Med. 177, 853–860. doi: 10.1164/rccm.200709-1413OC
Qu, Y., Olonisakin, T., Bain, W., Zupetic, J., Brown, R., Hulver, M., et al. (2018). Thrombospondin-1 protects against pathogen-induced lung injury by limiting extracellular matrix proteolysis. JCI Insight 3:e96914. doi: 10.1172/jci.insight.96914
Rossi, E., La Rosa, R., Bartell, J. A., Marvig, R. L., Haagensen, J. A. J., Sommer, L. M., et al. (2020). Pseudomonas aeruginosa adaptation and evolution in patients with cystic fibrosis. Nat. Rev. Microbiol. doi: 10.1038/s41579-020-00477-5 [Epub ahead of print]
Ruffin, M., and Brochiero, E. (2019). Repair process impairment by Pseudomonas aeruginosa in epithelial tissues: major features and potential therapeutic avenues. Front. Cell. Infect. Microbiol. 9:182. doi: 10.3389/fcimb.2019.00182
Saint-Criq, V., Villeret, B., Bastaert, F., Kheir, S., Hatton, A., Cazes, A., et al. (2018). Pseudomonas aeruginosa LasB protease impairs innate immunity in mice and humans by targeting a lung epithelial cystic fibrosis transmembrane regulator-IL-6-antimicrobial-repair pathway. Thorax 73, 49–61. doi: 10.1136/thoraxjnl-2017-210298
Storey, D. G., Ujack, E. E., Mitchell, I., and Rabin, H. R. (1997). Positive correlation of algD transcription to lasB and lasA transcription by populations of Pseudomonas aeruginosa in the lungs of patients with cystic fibrosis. Infect. Immun. 65, 4061–4067.
Storey, D. G., Ujack, E. E., and Rabin, H. R. (1992). Population transcript accumulation of Pseudomonas aeruginosa exotoxin A and elastase in sputa from patients with cystic fibrosis. Infect. Immun. 60, 4687–4694.
Stover, C. K., Pham, X. Q., Erwin, A. L., Mizoguchi, S. D., Warrener, P., Hickey, M. J., et al. (2000). Complete genome sequence of Pseudomonas aeruginosa PAO1, an opportunistic pathogen. Nature 406, 959–964. doi: 10.1038/35023079
Keywords: Pseudomonas aeruginosa, elastase, LasB, murine chronic lung infection, immune response, cystic fibrosis
Citation: Cigana C, Castandet J, Sprynski N, Melessike M, Beyria L, Ranucci S, Alcalá-Franco B, Rossi A, Bragonzi A, Zalacain M and Everett M (2021) Pseudomonas aeruginosa Elastase Contributes to the Establishment of Chronic Lung Colonization and Modulates the Immune Response in a Murine Model. Front. Microbiol. 11:620819. doi: 10.3389/fmicb.2020.620819
Edited by:
Mario Alberto Flores-Valdez, CONACYT Centro de Investigación y Asistencia en Tecnología y Diseño del Estado de Jalisco (CIATEJ), MexicoReviewed by:
Marta Helena Branquinha, Federal University of Rio de Janeiro, BrazilSinéad Weldon, Queen’s University Belfast, United Kingdom
Copyright © 2021 Cigana, Castandet, Sprynski, Melessike, Beyria, Ranucci, Alcalá-Franco, Rossi, Bragonzi, Zalacain and Everett. This is an open-access article distributed under the terms of the Creative Commons Attribution License (CC BY). The use, distribution or reproduction in other forums is permitted, provided the original author(s) and the copyright owner(s) are credited and that the original publication in this journal is cited, in accordance with accepted academic practice. No use, distribution or reproduction is permitted which does not comply with these terms.
*Correspondence: Cristina Cigana, Y2lnYW5hLmNyaXN0aW5hQGhzci5pdA==