- 1Department of Microbiology, Daiichi Sankyo India Pharma Private Limited, Gurgaon, India
- 2Research Department, Sidra Medicine, Doha, Qatar
- 3Department of Pharmacokinetics and Metabolism, Daiichi Sankyo India Pharma Private Limited, Gurgaon, India
Pseudomonas aeruginosa forms biofilms in the lungs of chronically infected cystic fibrosis patients, which are tolerant to both the treatment of antibiotics and the host immune system. Normally, antibiotics are less effective against bacteria growing in biofilms; azithromycin has shown a potent efficacy in cystic fibrosis patients chronically infected with P. aeruginosa and improved their lung function. The present study was conducted to evaluate the effect of azithromycin on P. aeruginosa biofilm. We show that azithromycin exhibited a potent activity against P. aeruginosa biofilm, and microscopic observation revealed that azithromycin substantially inhibited the formation of solid surface biofilms. Interestingly, we observed that azithromycin restricted P. aeruginosa biofilm formation by inhibiting the expression of pel genes, which has been previously shown to play an essential role in bacterial attachment to solid-surface biofilm. In a rat model of chronic P. aeruginosa lung infection, we show that azithromycin treatment resulted in the suppression of quorum sensing-regulated virulence factors, significantly improving the clearance of P. aeruginosa biofilms compared to that in the placebo control. We conclude that azithromycin attenuates P. aeruginosa biofilm formation, impairs its ability to produce extracellular biofilm matrix, and increases its sensitivity to the immune system, which may explain the clinical efficacy of azithromycin in cystic fibrosis patients.
Introduction
Pseudomonas aeruginosa is the most common bacterial pathogen that causes biofilm-mediated chronic lung infections among cystic fibrosis (CF) patients (Faure et al., 2018; Maurice et al., 2018). P. aeruginosa has an innate propensity to attach to different solid surfaces and form biofilms, which enables the bacteria to resist both the host’s innate immune system and treatments with antibiotics (Mulcahy et al., 2014). The treatment options for nosocomial Gram-negative infections are very limited. The antibiotics of choice for Gram-negative pathogens are parenteral carbapenems, such asimipenem and meropenemor quinolones (Bassetti et al., 2018). However, the poor activities of these antibiotics on bacterial biofilms and the increasing prevalence of multidrug-resistant P. aeruginosa (Cabot et al., 2012; Ciofu and Tolker-Nielsen, 2019; Kumar et al., 2019) leave the physicians with very limited choices to effectively treat these patients.
Although macrolides are commonly used for Gram-positive pathogens, azithromycin is being extensively used to treat chronic respiratory tract infections caused by P. aeruginosa especially in cystic fibrosis patients (Hansen et al., 2005; Wagner et al., 2005). In addition to the direct anti-pseudomonas effects (Tateda et al., 1996; Marvig et al., 2012), multiple clinical studies have highlighted the beneficial non-antibiotic effects of azithromycin, including modulation of the aberrant immune response and improving lung function in CF patients (Equi et al., 2002; Saiman et al., 2003; Clement et al., 2006; Principi et al., 2015; Cogen et al., 2018). Taken together, multiple mechanisms of azithromycin have been suggested, such as inhibiting biofilm formation by modulating the synthesis of quorum sensing (QS) molecules (Nalca et al., 2006; Swatton et al., 2016) or stimulating the anti-inflammatory effect during prolonged exposure (Zimmermann et al., 2018).
P. aeruginosa poses different QS signaling systems (LasI/LasR and Rh1I/Th1R)that regulate different cellular processes, including cell-to-cell communication, extracellular matrix synthesis, and biofilm formation (de Kievit and Iglewski, 2000; Smith and Iglewski, 2003). A large number of genes, including those involved in virulence and biofilm formation, are modulated by two acyl-homoserine lactone molecules synthesized by QS systems, namely, 3-O-C12homoserine lactone (HSL) synthesized by lasI and C4-HSL synthesized by Rh1I (Ding et al., 2018). The QS signaling molecule 3-O-C12 HSL has been shown to be essential for extracellular matrix modulation and biofilm formation since the lasI mutant was found to be defective in extracellular biofilm matrix and biofilm formation (Sakuragi and Kolter, 2007). In contrast, the Rh1I/Rh1R signaling system was found to be important for the survival of bacterial cells during anaerobic conditions in biofilms (Yoon et al., 2002; Sakuragi and Kolter, 2007). Interestingly, an increasing level of 3-O-C12 HSL has been reported in both P. aeruginosa biofilm formed in vitro and in CF patients chronically infected with P. aeruginosa (Geisenberger et al., 2000; Singh et al., 2000; Barr et al., 2015). Azithromycin has been shown to inhibit the synthesis of 3-O-C12 HSL signaling QS molecules (Favre-Bonte et al., 2003) without influencing the growth of planktonic cells (Chalmers, 2017).
However, data concerning the mechanism of biofilm inhibition by azithromycin and in vivo efficacy in chronic pulmonary infection, to support the clinical utilities of azithromycin in CF patients, are scarce. In this study, we evaluated the efficacy of azithromycin against in vitro and in vivo P. aeruginosa biofilm formation in a chronic pulmonary rat infection model at a human-equivalent dose. Our study revealed the potential mechanism of biofilm inhibition by azithromycin.
Materials and Methods
Bacterial Strains and Antibiotics
P. aeruginosa strain PAO1, PA-14 (highly virulent clinical isolate), and ATCC 700829 (standard strain for biofilm formation) were used for all in vitro and in vivo biofilm experiments. A recombinant P. aeruginosa G2 strain having a single chromosomal copy of promoterless lacZ gene, under promoter control of the pelA gene, was used for the mechanism of action studies. The bacterial isolates were obtained from the American Type Culture Collection (Manassas, VA, United States) or from our proprietary collection of clinical isolates. Levofloxacin was synthesized at Daiichi Sankyo Co., Tokyo. Azithromycin and clindamycin were obtained from a commercial source. All strains were stored at −80°C in 20% glycerol in trypticase soy broth (TSB) (Becton, Dickinson, and Company, Cockeysville, MD). All bacteriological media were procured from Becton Dickinson and Company; the reagents and chemicals were purchased from Sigma-Aldrich Co., LLC, United States.
In vitro Biofilm Activity
The effect of azithromycin and clindamycin on P. aeruginosa biofilm formation was assessed as described previously (Sharma et al., 2009; Barman et al., 2016; Kumar et al., 2019). Briefly, the overnight-grown culture of P. aeruginosa PAO1 in TSB was pelleted and resuspended in Luria–Bertani broth containing 0.2% glucose (107 CFU/ml). The bacterial suspension was spiked with different concentrations (16–0.015 μg/ml) of azithromycin or clindamycin in 96-well plates and incubated at 26 ± 2°C. The effects of azithromycin and clindamycin on inhibition of biofilm formation were assayed after 24 h of exposure. The wells were emptied and washed three times with phosphate-buffered saline (PBS; pH 7.3), and the adherent biofilm was stained with 200 μl of 1% crystal violet for 30 min and rinsed with distilled water. The adherent biofilm in the wells was solubilized with 200 μl of 30% acetic acid, and the optical density at 600 nm (OD600) was measured to quantify the formed biofilms. Each experiment was performed thrice, and the mean percent inhibition and standard deviations were calculated. The relative inhibition of biofilms, expressed as a mean percentage, was determined using the following formula:
The efficacy of azithromycin was also evaluated on a pre-formed biofilm of P. aeruginosa PAO1 to assess the therapeutic or eradicative effect of azithromycin as described earlier (Macia et al., 2014). The bacterial suspension was prepared as described above and inoculated at 200 μl/well in a 96-well plate. After 24 h of incubation, the wells were emptied and washed with PBS and then spiked with different concentrations (16–0.015 μg/ml) of azithromycin prepared in LB broth containing 0.2% glucose. The plate was further incubated for 24 h, and the effect of azithromycin was assessed as described above.
To further evaluate the effect of azithromycin on planktonic cells and biofilm formation, a time-kill kinetic study was performed. Briefly, 10 ml of LB broth containing 0.2% glucose and a sterile 2-cm-long piece of the catheter were pre-warmed at 26 ± 2°C in reaction tubes, and the different concentrations of azithromycin (2 or 16 μg/ml) were added to tubes. The bacterial inoculum of P. aeruginosa PA-14 was prepared as described above and added to the tubes to achieve a final inoculum of ∼1 × 105 CFU/ml. The tubes were incubated at 26 ± 2°C. The planktonic cell counts were determined at various time-points by serial dilution plating onto a trypticase soy agar plate. After 24 h of incubation, the catheter pieces from each tube were removed, non-adherent bacteria were washed from the catheter pieces, and the number of CFU embedded in biofilm per catheter was determined as described earlier (Kumar et al., 2016, 2019). The experiment was performed in triplicate, and the mean log10 killing of planktonic cells and biofilm inhibition were calculated for each concentration. To further assess biofilm formation in the absence (drug-free control) or presence of azithromycin, the catheter pieces were also sectioned and processed for scanning electron microscopy (SEM).
β-Galactosidase Activity
To understand the molecular mechanism of biofilm inhibition, a recombinant mutant P. aeruginosa G2-containing promoterless lacZ gene under promoter control of pelA was gifted to us by Prof. Kilter (Sakuragi and Kolter, 2007). The β-galactosidase activity was performed in the absence and presence of different concentrations of azithromycin and levofloxacin, as described earlier (Sakuragi and Kolter, 2007).
In vivo Studies
Specific pathogen-free female Sprague–Dawley (SD) rats, weighing 200 ± 20 g (age 8 weeks), were used for the study. The rats were procured from Vivo Biotech Pvt. Ltd., Hyderabad, India. The animals were housed in a pathogen-free animal facility and allowed 5 days of acclimatization in the study room before initiating the experiment. Feed and water were provided ad libitum during acclimatization and the study periods. All animal studies were approved by the institutional animal ethics committee. The animal studies were conducted under the strict guidelines of the committee for the Purpose of Control and Supervision of Experiments on Animals, Ministry of Environment, Forests and Climate Change, New Delhi, Government of India, and under the supervision of the institutional committee.
To determine the in vivo efficacy of azithromycin, a chronic biofilm model of P. aeruginosa was established in SD rats, which mimic the human chronic infection (Hoover et al., 2017; Chawanpaiboon et al., 2019). A semisolid 0.25% w/v agar beads suspension was prepared and autoclaved a day before the in vivo experiment and stored overnight at 4°C. To cause a chronic biofilm infection, P. aeruginosa PA-14 was grown overnight on Mueller–Hinton agar (MHA; Becton, Dickinson and Company), and three to four isolated bacterial colonies were picked and suspended in PBS buffer to prepare the bacterial suspension. The turbidity of bacterial suspension was adjusted to 1 McFarland, diluted 1:10 with PBS. To prepare the inoculum, bacterial suspension was embedded in agar beads as detailed in a previously described method, which yield beads of mean size approximately 200–300 μm and a bead suspension of 2.5 × 107 CFU/ml (Hraiech et al., 2012). The animals were anesthetized by injecting 100 μl of xylazine (5 mg/kg) and ketamine (100 mg/kg) mixture intramuscularly. Using an 18-gauge blunt needle, 150 μl of bead suspension was injected intratracheally as described earlier (Barman et al., 2011). To cause a chronic biofilm infection, the animals were kept untreated for 3 days. Before starting the treatment, the animals were randomized using their body weight, and n = 6 animals were kept in each group. Azithromycin was administered orally using agavage at 75 mg/kg, q24h for 4 days. The rats were then euthanized 22 h after the last dose, and the lungs’ bacterial load was determined by plating diluted lung homogenates on MHA.
Pharmacokinetics Studies of Azithromycin in P. aeruginosa-Infected Rats
The concentrations of azithromycin were assessed in the plasma and lung epithelial lining fluid (ELF) of the rats infected with P. aeruginosa PA-14. Intratracheal infection in rats was performed as described above. A single dose of 75 mg/kg of azithromycin was administered orally through gavage. Blood and broncho-alveolar lavage (BAL) fluid (with 2 ml of normal saline wash) were collected at different time-points up to 24 h post-dose from each animal by gross dissection. For the collection of BAL, 2 ml of sterile cold PBS was infused into the animal through the trachea and into the bronchial cavity and aspirated out. Plasma was harvested from blood samples by centrifugation. The azithromycin concentrations in plasma and BAL samples were determined using liquid chromatography with tandem mass spectrometry. The apparent volume of ELF (VELF) in BAL fluid was determined by the urea dilution method using the urea assay kit from BioChain (Hayward, CA). The concentration of azithromycin in ELF (CELF) was determined as
where CBAL is the measured concentration of azithromycin in BAL fluid.
Statistical Analysis
All data were analyzed using GraphPad Prism (version 8; GraphPad Software, San Diego, CA, United States). The statistical significance of the difference between the numbers of viable organisms recovered from the lungs of the treated group and those for the untreated control group or biofilm inhibition as compared to control (no drug control) was evaluated by non-parametric Mann–Whitney analysis. A difference between the treated group and the untreated control group was considered to be statistically significant if the P < 0.05.
Results
In vitro Biofilm Activity
Azithromycin exhibited a potent activity against biofilms produced by different isolates of P. aeruginosa. Azithromycin showed a strong biofilm inhibition as compared to the drug-free control and clindamycin with a biofilm preventive concentration (BPC50) value of 0.122 μg/ml against P. aeruginosa PAO1 (Figure 1) and also exhibited a similar biofilm activity against P. aeruginosa ATCC 700829 (standard strain used for biofilm formation) (Supplementary Figure 1). In contrast, azithromycin exhibited a comparatively reduced activity against the pre-formed biofilm with a minimum biofilm eradication concentration (MBEC50) value of 7.49 μg/ml against P. aeruginosa PAO1 (Supplementary Figure 2).
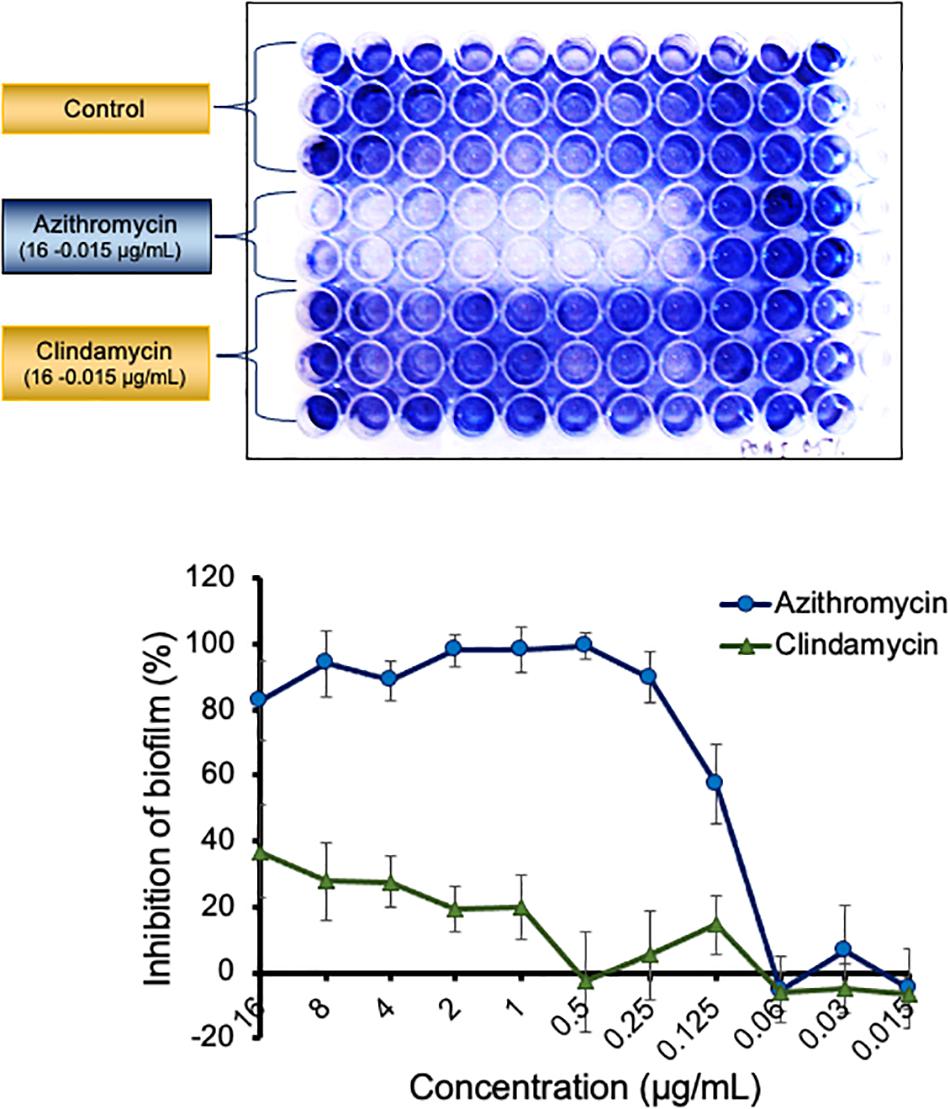
Figure 1. Activity of azithromycin and clarithromycin against biofilm of P. aeruginosa PAO1 The adherent biofilm in presence of azithromycin, clindamycin or in control well (drug free) was stained with 1% crystal violet and measured by OD600 after dissolving in 30% acetic acid. The inhibition of biofilm in presence of antibiotics was calculated by comparing the biofilm formation in control well. Values shown in the figure are average of three independent experiments.
To further determine the effect of azithromycin against planktonic cells and biofilm formation on a solid surface, we performed a time-kill kinetic study using a catheter piece as a solid surface objective, as described earlier (Kumar et al., 2019), although azithromycin showed a substantial 1.86 log10 reduction in planktonic cell counts at 6 h as compared to the drug-free control and but no effect at 24 h post-incubation (Figure 2A and Supplementary Figure 3). Possibly, over-expression of multidrug efflux pumps could be the reason of its poor activity against P. aeruginosa in LB media, as potent-activity of azithromycin has been observed in the presence of known efflux pump inhibitors or eukaryotic media (RPMI 1640) (Buyck et al., 2012). Despite the high bacterial cell counts in LB media (Figure 2A), azithromycin exhibited a strong activity against P. aeruginosa PA-14 biofilm (Figure 2B). A simple macroscopic and quantitative analysis 24 h after incubation revealed that P. aeruginosa PA-14 formed a strong solid-surface biofilm on catheter pieces (Figures 2C,D), whereas in the presence of azithromycin, no solid-surface biofilm formed, suggesting that, despite having a limited activity against the planktonic cells of P. aeruginosa in LB media, azithromycin efficiently impeded the planktonic cells from leading to biofilm formation, possibly by inhibiting the synthesis of the extracellular biofilm matrix required for solid-surface biofilm formation. To confirm our hypothesis, we used SEM to visualize biofilm formation at the cellular level in a drug-free control and the presence of azithromycin. As shown in Figure 2C, P. aeruginosa cells were embedded in an extracellular matrix to form a solid-surface biofilm in a drug-free control, whereas no extracellular matrix was observed in the presence of azithromycin (Figure 2D). Hence, biofilm formation was significantly inhibited. These lines of evidence support the hypothesis that azithromycin inhibits biofilm formation by impairing the ability of P. aeruginosa to produce its biofilm matrix.
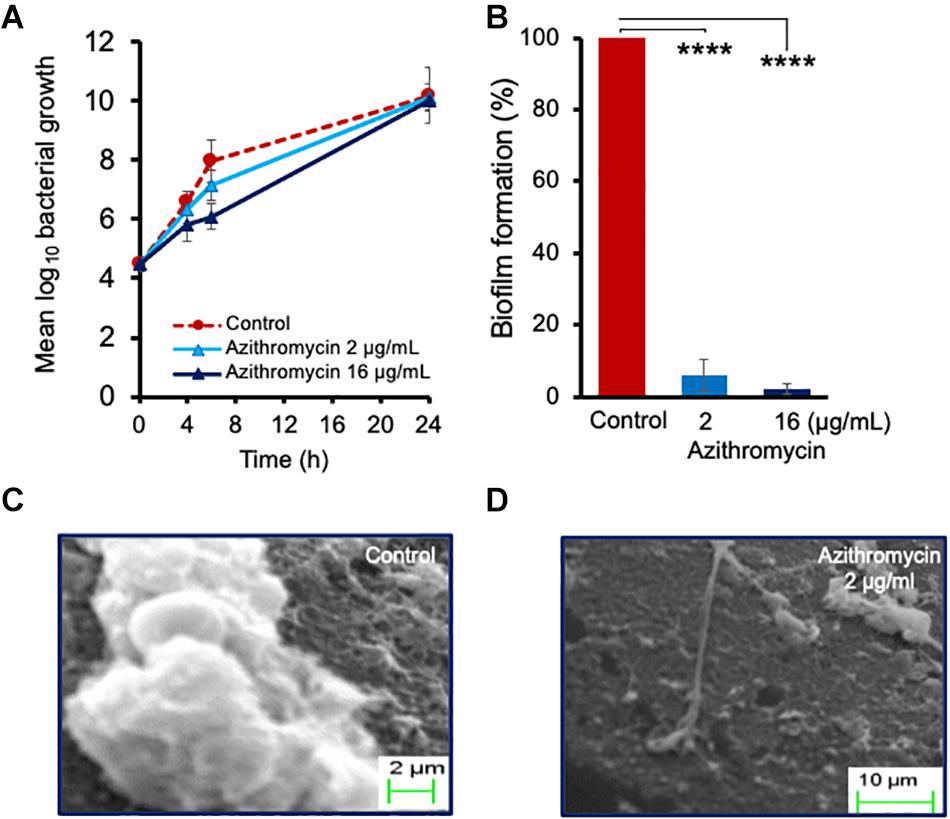
Figure 2. Activity of azithromycin against planktonic cells of P. aeruginosa PA14 (A), and biofilm formation (B) at 24 h. Scanning electron micrographs revealing biofilm formation at 24 h in absence of drug (C) or in presence of azithromycin (D). The catheter piece in drug-free control well shows production of extracellular matrix and biofilm formation, while no extracellular biofilm matrix and biofilm formation observed in presence of azithromycin. Each value is the average of triplicate assay where presented data is mean ± SD. Statistical analysis were calculated using one way ANOVA and ****p < 0.0001 (n = 3).
Development of the solid-surface-associated biofilm is controlled by pel genes in P. aeruginosa, as a pel gene mutant was found defective in synthesizing an extracellular biofilm matrix and biofilm formation (Sakuragi and Kolter, 2007), and the expression of pel genes is regulated by 3-O-C12 homoserine lactone (autoinducer of the las QS signaling system, synthesized by lasI) (Sakuragi and Kolter, 2007).
To understand the biofilm inhibition mechanism of azithromycin, we evaluated the β-galactosidase activity of a recombinant mutant containing a promoter less lacZ gene under promoter control of pel gene (Sakuragi and Kolter, 2007), in the absence and presence of different azithromycin concentrations. Azithromycin showed a dose-dependent inhibition of β-galactosidase activity with IC50 0.170 μg/ml (Figure 3). In addition, to confirm the selectively of azithromycin from the Las QS system, we also evaluated the sub-MIC concentrations of levofloxacin. As expected, levofloxacin did not influence the β-galactosidase activity of mutant (Figure 3A). Taken together, these results indicate that azithromycin inhibits pel gene transcription by inhibiting the las QR signaling system (Figures 3B,C), resulting in the inhibition of P. aeruginosa biofilm formation.
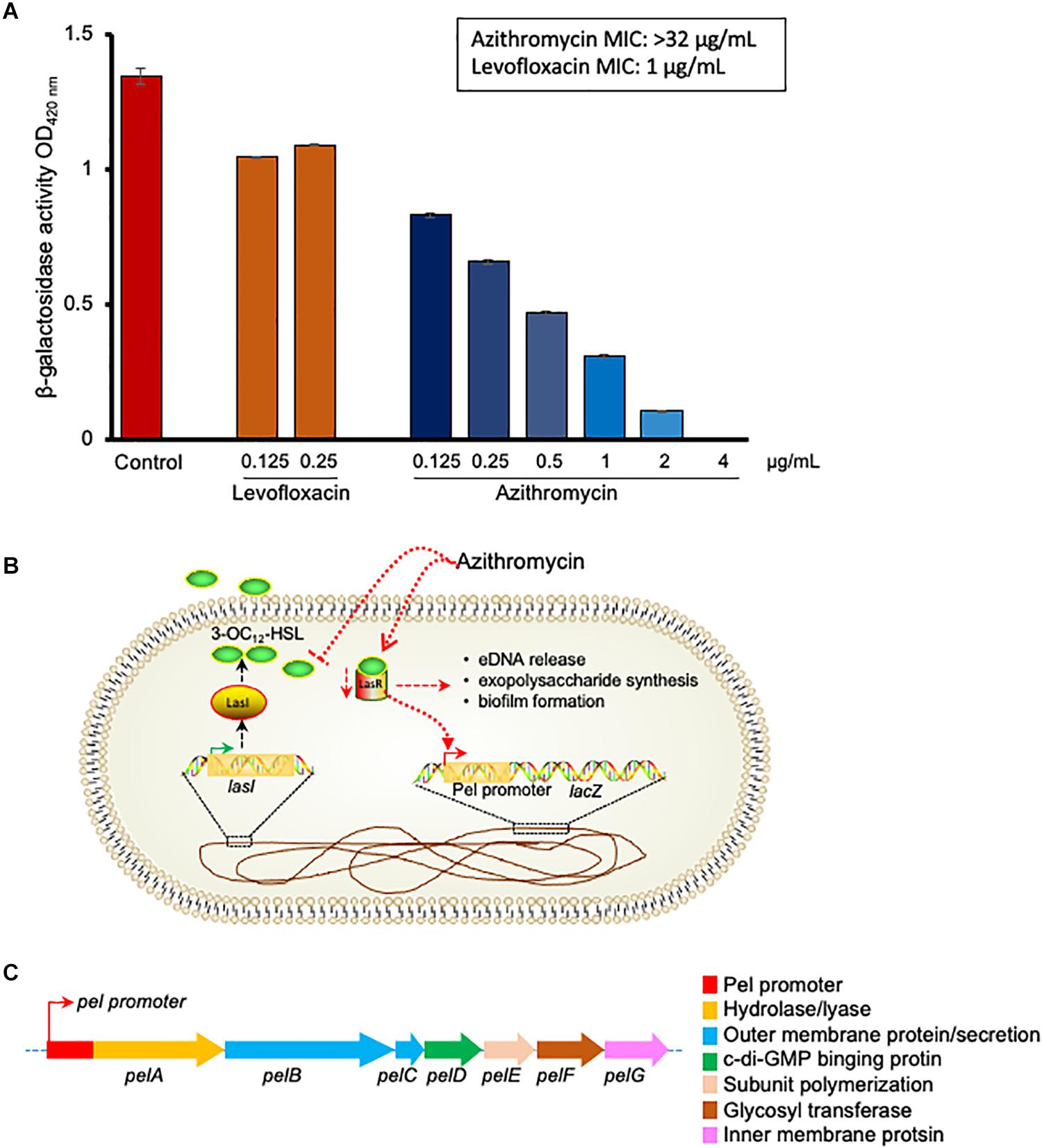
Figure 3. p-galactosidase activity of P. aeruginosaG2 recombinant construct containing pel promoter-lacZ transcriptional fusion in presence of different concentration of azithromycin, levofloxacin or no-drug control (A). The MIC of azithromycin and levofloxacin was > 32 and 1 ng/mL, respectively, against P. aeruginosaG2. Proposed mechanism of biofilm and pel genes inhibition by azithromycin (B).Genetic structure of pel genes in P. aeruginosa (C).
In vivo Efficacy Against P. aeruginosa in a Rat Biofilm Model
The experimental plan used for testing the efficacy of azithromycin at a human-equivalent dose is shown in Figure 4. The treatment was implemented 3 days post-infection and given once daily for a period of 4 days (Figure 4A). All the infected animals in the control group exhibited consistent bacterial counts. At 4 days, the placebo treatment resulted in no significant change in bacterial load, whereas treatment with azithromycin at 75 mg/kg, q24h resulted in a significant 1.67 log10 reduction in bacterial counts as compared to the placebo control (Figure 4B).
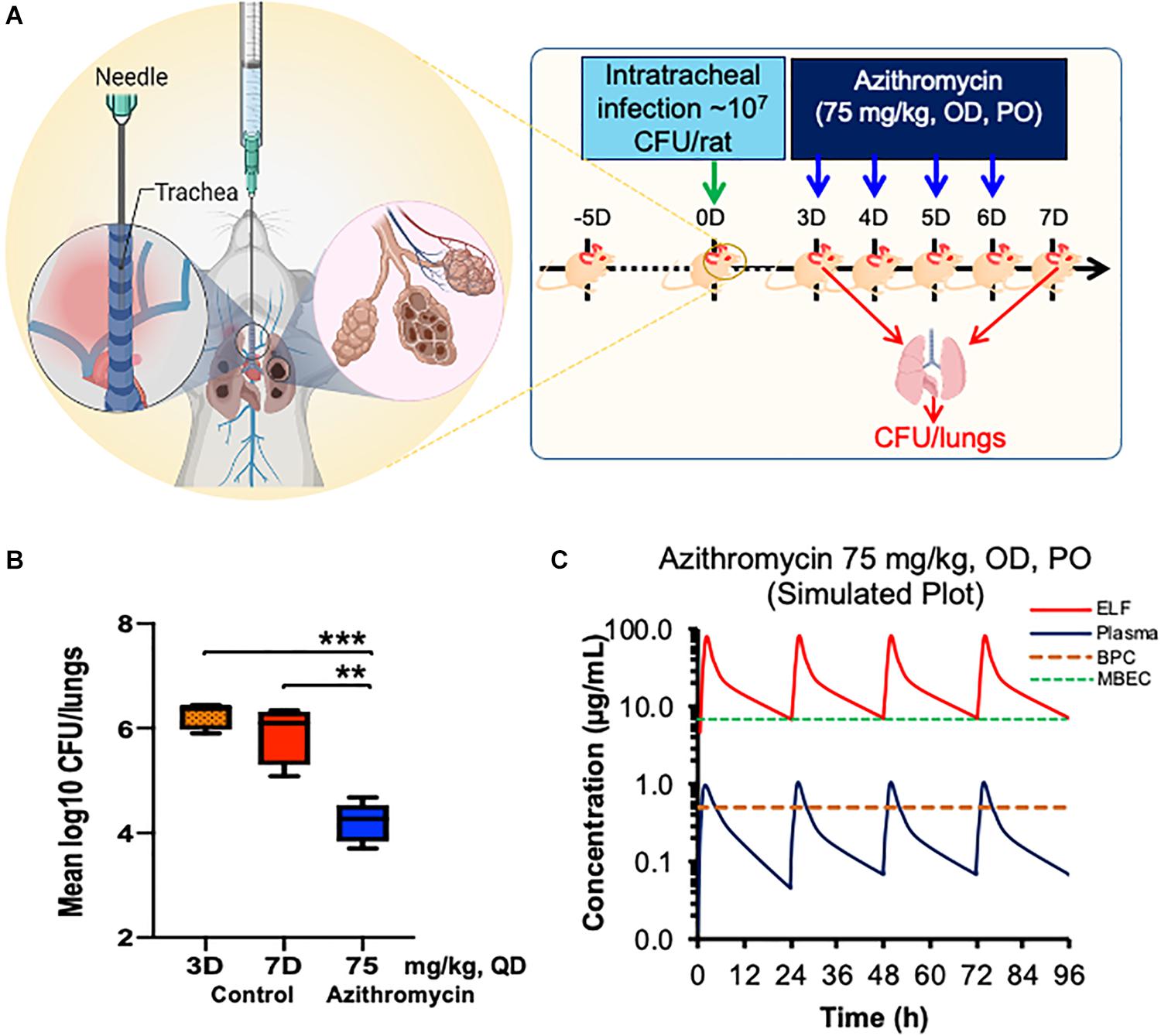
Figure 4. Efficacy of azithromycin in chronic rat pulmonary infection model of P. aeruginosa PA-14.Chronic pulmonary was caused using P aeruginosaPA-14 in rats via the intratracheally route and treatment was started 3 days post infection with azithromycin by PO route (A). Bacterial lungs loads were determined post 22 h of last dose and compared with those of placebo treated (B). Stimulated plasma and ELF concentration of azithromycin at 75 mg/kg, PO for 4 days was calculated using WinNonlin (C). The asterisks indicates a significant difference compared with those of control group (**P < 0.01, ***P < 0.001). CFU, Colony Forming Unit; OD, Once a Day; PO, Per Orem (Oral administration); ELF, Epithelial Lining Fluid; BPC, Biofilm Preventive Concentration; MBEC, Minimal Biofilm Eradication Concentration.
Pharmacokinetics of Azithromycin in P. aeruginosa-Infected Rats
The plasma and lung tissue kinetics of azithromycin in the SD rats infected with P. aeruginosa PA-14 are presented in Figure 4C and Table 1. The plasma and lung tissues were harvested from infected animals at different time-points of up to 24 h post-dosing, and the stimulated plasma and lung tissue (EFL) profile was prepared to understand the pharmacokinetics of azithromycin in infected animals. Although Cmax in plasma and lung tissues was observed 2 h after administration, azithromycin showed comparatively higher concentrations in lung tissues. The lung ELF/plasma ratios of Cmax and AUCinf were 81.05 and 85.96, respectively. The graph shows that the simulated lung concentrations of azithromycin at 75 mg/kg, q24h dose were substantially higher than the BPC and MBEC values of P. aeruginosa biofilms throughout the treatment (Figure 4C).
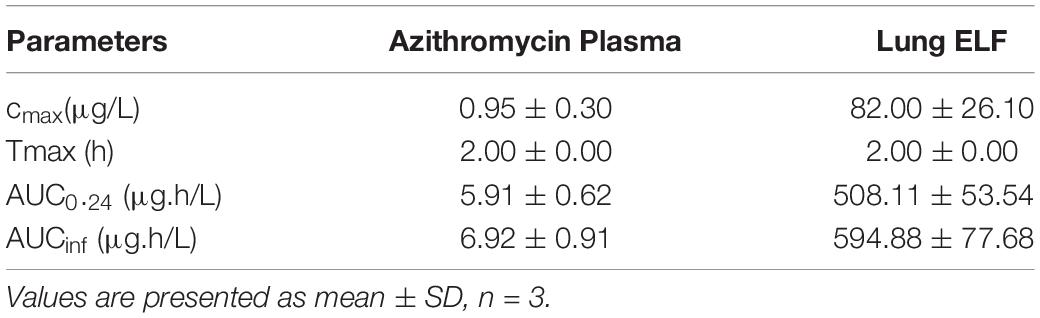
Table 1. Pharmacokinetic parameters of azithromycin at 75 mg/kg, PO dose in Wister rats infected with P. aeruginosa PA-14.
Discussion
Biofilm formation within the lungs of CF patients is the hallmark of P. aeruginosa pathogenesis (Singh et al., 2000), as it substantially reduced the bacterial susceptibility to antimicrobial agents and the host immune system compared to planktonic cells (Smith and Iglewski, 2003; Mulcahy et al., 2014). Biofilm infections (including CF infections) tend to be chronic and difficult to eradicate and remain a matter of great concern, which has generated the need for alternative treatment strategies. The most striking findings with azithromycin in the present investigation were its capacity to inhibit P. aeruginosa biofilm formation and reduce the solid-surface biofilm attachment almost to a lower detection limit. Interestingly, the SEM of solid-surface biofilm in the presence of azithromycin shows a substantially reduced extracellular biofilm matrix and attachment to a solid surface to form a biofilm, which is in accordance with the findings of other studies (Mizukane et al., 1994; Ichimiya et al., 1996). Although azithromycin is well known to show a potent activity against P. aeruginosa biofilm, its evaluation in chronic respiratory P. aeruginosa biofilm infection model has been relatively understudied. There is a pressing need to determine its bactericidal activity on biofilms to facilitate its clinical use.
To evaluate the potential mechanism of azithromycin to inhibit biofilm formation, we developed a refined and chronic lung rat model of P. aeruginosa to mimic the clinical and chronic P. aeruginosa infection conditions in patients (Hraiech et al., 2012; Chawanpaiboon et al., 2019). The most important features of the rat chronic P. aeruginosa infection model were consistent and reproducible bacterial lung counts in infected animals with no acute morbidity but only delayed mortality of infected rats within 7–14 days if left untreated (data not shown), which makes it ideal to evaluate efficacy against chronic disease. These findings align with those of CF patients who suffer from chronic P. aeruginosa infections and show high mortalities. In this study, we evaluated the efficacy of azithromycin at 75 mg/kg, once daily, which was equivalent to 500 mg every 24 h in humans (Beringer et al., 2005; Cipolli et al., 2012; Dalhoff, 2014). To mimic the clinical chronic infection conditions, the treatment was initiated 3 days post-infection with 75 mg/kg, q24h of azithromycin for 4 days by the PO route. This treatment regimen resulted in a significant reduction in the lungs’ bacterial counts compared to the placebo-treated control.
To understand the pharmacodynamics of potent in vivo activity of azithromycin, we performed pharmacokinetics of azithromycin in the SD rats infected with P. aeruginosa. Interestingly, we observed a significantly higher concentration of azithromycin in the lungs of the infected animals, which was found to be well above the BPC and MBEC values of azithromycin. This explains the potent in vivo efficacy of azithromycin in treating the chronic lung infection model of P. aeruginosa. Our data are in accordance with the clinical findings that azithromycin treatment improves lung function in CF patients chronically infected with P. aeruginosa either by inhibiting biofilm formation (Saiman et al., 2003; Hansen et al., 2005) or suppressing the growth of susceptible bacteria.
The bacterial biofilm matrix is generally composed of exopolysaccharides, proteins (Sutherland, 2001), and e-DNA (Cherny and Sauer, 2019), which is required for both structural integrity and protective barrier to the harsh environment (Mulcahy et al., 2014). Three main exopolysaccharides (psl, pel, and alginate) have been identified in P. aeruginosa (Sutherland, 2001). Among these, pel gene cluster regulated by LasI/LasR (3-O-C12 HSL molecule synthesized by lasI) was found important for bacterial attachment to solid surfaces and biofilm formation (Sakuragi and Kolter, 2007). A dose-dependent inhibition of the pel promoter by azithromycin in our study suggests that azithromycin affects biofilm formation by inhibiting the synthesis of the QS molecule (3-O-C12). In addition to the regulation of in vitro biofilm, the QS molecules are also critical for the infectivity of P. aeruginosa, as QS mutant showed impaired chronic infectivity compared to wild-type P. aeruginosa (Willcox et al., 2008). Interestingly, a comparatively reduced level of QS molecules (a marker of biofilm formation) in the ELF of animals treated with azithromycin further suggests the important role of the QS system during chronic P. aeruginosa infection (Figure 5) and indicates that azithromycin modulates the biofilm by inhibiting the synthesis of 3-O-C12 HSL, a known marker of biofilm formation (Barr et al., 2015). Our results with rats with chronic P. aeruginosa infection demonstrated that azithromycin significantly improved the pulmonary P. aeruginosa clearance, possibly by interfering with QS systems and crippling of the exopolysaccharides of the biofilm synthesized by the pel proteins. Besides inhibiting the virulence factors, azithromycin is known to have multiple effects against P. aeruginosa: (a) enhanced activity in the presence of serum, which could contribute to bacterial killing during chronic pulmonary infection (Lucchi et al., 2008), (b) anti-inflammatory activity that could help to reduce the inflammation at infection sites (Zeng et al., 2016; Zimmermann et al., 2018), (c) biofilm inhibitory or eradicative activity by modulating the synthesis of signaling molecules, such as the QS system, ci-di-GMP, and AHL (Favre-Bonte et al., 2003; Nalca et al., 2006; Barr et al., 2015; Ghosh et al., 2020), and (d) anti-virulence activity that could impair P. aeruginosa cells to produce extracellular biofilm matrix. These multiple effects of azithromycin could together be important mediators for its potent in vivo efficacy, or is there something else that drives the efficacy against P. aeruginosa in chronic CF patients? Could it be that host immune modulation leads to killing of bacterial cells or inhibition/eradication of biofilm and exposing the bacterial cells to an accumulated azithromycin concentration in the lung? These could be interesting avenues for investigation for future research.
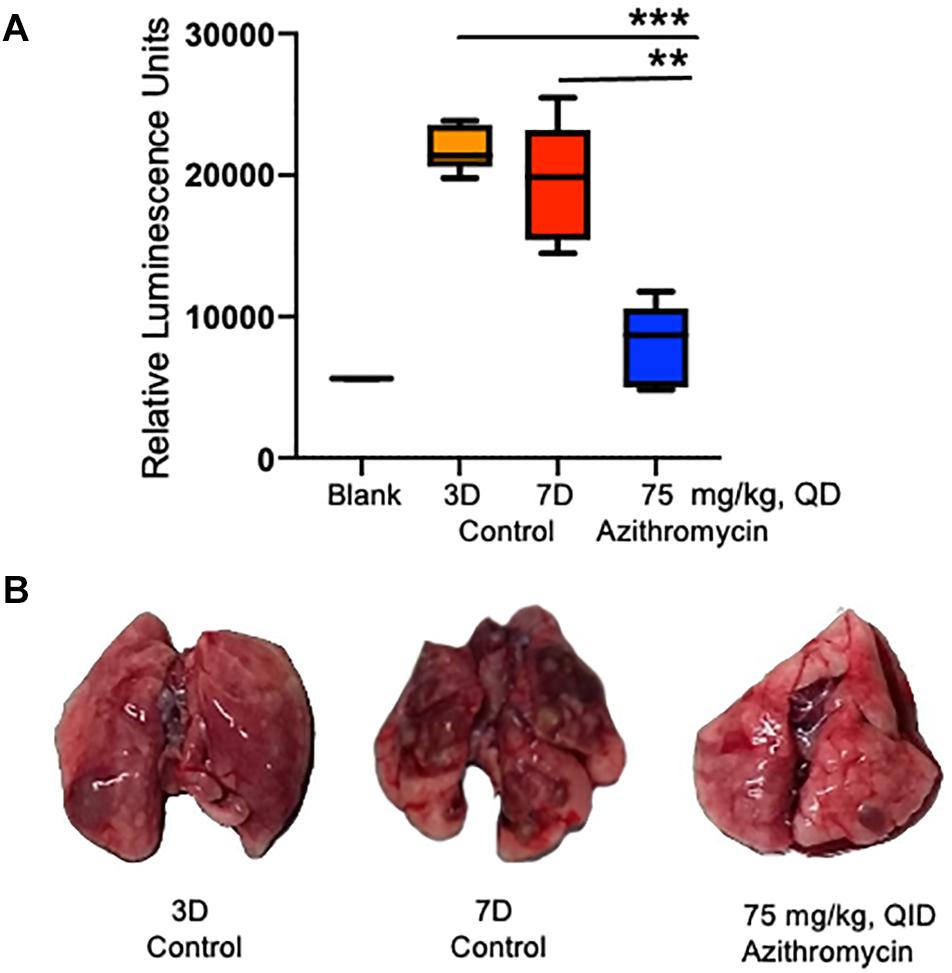
Figure 5. Detection of QS molecule in the epithelial lining fluid (ELF) of infected rats. QS molecule was determined 3 days post-infection, when the treatment was started and at 7 days post-infection in the placebo control and azithromycin treated rats (A). Representative lungs photos of 3D, 7D and azithromycin treated rats (B). The asterisks indicates a significant difference compared with those of 7D control group (**P < 0.01, ***P < 0.001).
In the present study, we reported the detailed biofilm preventive effect of azithromycin. However, our study has several limitations. First, the in vitro biofilm assays revealed that azithromycin shows better BPC than the MPEC, which was also supported by the reduced production of extracellular biofilm matrix in the presence of azithromycin, while the in vivo efficacy of azithromycin was evaluated against chronic P. aeruginosa infection (preformed biofilms). Second, the in vivo study was performed only at a single human-equivalent dose. Therefore, further detailed studies are required to access the preventive and eradicative biofilm effect of azithromycin at multiple doses to understand its clinical utilities in CF patients.
In summary, we demonstrated that azithromycin repressed the expression of 3-O-C12 (QS system) and inhibits biofilm matrix production by influencing the expression of pel genes. Furthermore, azithromycin treatment of rats with chronic P. aeruginosa infection significantly improved the bacterial clearance from chronic infection compared to placebo controls. Taken together, the repression of bacterial virulence, the bactericidal effect on biofilm formation, and the enhanced serum sensitivity after a prolonged exposure to azithromycin may be responsible for the potent efficacy observed in our rats with chronic infection and in patients with CF.
Data Availability Statement
The original contributions presented in the study are included in the article/Supplementary Material, further inquiries can be directed to the corresponding author/s.
Ethics Statement
The animal study was reviewed and approved by the Institutional Animal Ethics Committee at Daiichi Sankyo Pharma Pvt. Ltd., Gurgaon.
Author Contributions
MK and MR conceived the study, performed statistical analyses, and generated graphs. MK, MR, TM, TKB, VJ, SS, and MP performed the in vitro and in vivo studies. TC performed the PK studies. MP, DJU, and NM did the project management. MK, MR, and SA wrote the first draft of manuscript. All authors contributed to manuscript editing, discussed the results, and approved the final submitted version.
Funding
We declare that this study received funding from Daiichi Sankyo Pharma Pvt., Ltd., Gurgaon. The funder was not involved in the study design, the collection, analysis, and interpretation of data, the writing of this article, or the decision to submit it for publication. We obtained permission for the publication of this manuscript from the funder before submitting the manuscript to the journal. We thank Daiichi Sankyo Co., Ltd., for financial assistance and support to this project.
Conflict of Interest
MK, MR, TM, TKB, VJ, TC, SS, MP, DJU, and NM were employed by the company Daiichi Sankyo Pharma Pvt. Ltd., Gurgaon.
The remaining author declares that the research was conducted in the absence of any commercial or financial relationships that could be construed as a potential conflict of interest.
Acknowledgments
We thank the Institute of Genomics and Integrative Biology, Delhi, India, for performing SEM studies and their interpretation. We were also grateful to Prof. Kilter of Harvard Medical School, 200 Longwood Avenue, Boston, for providing the P. aeruginosa G2.
Supplementary Material
The Supplementary Material for this article can be found online at: https://www.frontiersin.org/articles/10.3389/fmicb.2021.603151/full#supplementary-material
References
Barman, T. K., Kumar, M., Mathur, T., Chaira, T., Ramkumar, G., Kalia, V., et al. (2016). In vitro and in vivo activities of a Bi-Aryl Oxazolidinone. RBx 11760, against Gram-Positive Bacteria. Antimicrob. Agents Chemother. 60, 7134–7145.
Barman, T. K., Rao, M., Bhati, A., Kishore, K., Shukla, G., Kumar, M., et al. (2011). Non invasive real-time monitoring of bacterial infection & therapeutic effect of anti-microbials in five mouse models. Indian J. Med. Res. 134, 688–695.
Barr, H. L., Halliday, N., Camara, M., Barrett, D. A., Williams, P., Forrester, D. L., et al. (2015). Pseudomonas aeruginosa quorum sensing molecules correlate with clinical status in cystic fibrosis. Eur. Respir. J. 46, 1046–1054.
Bassetti, M., Vena, A., Croxatto, A., Righi, E., and Guery, B. (2018). How to manage Pseudomonas aeruginosa infections. Drugs Context 7:212527.
Beringer, P., Huynh, K. M., Kriengkauykiat, J., Bi, L., Hoem, N., Louie, S., et al. (2005). Absolute bioavailability and intracellular pharmacokinetics of azithromycin in patients with cystic fibrosis. Antimicrob. Agents Chemother. 49, 5013–5017. doi: 10.1128/aac.49.12.5013-5017.2005
Buyck, J. M., Plesiat, P., Traore, H., Vanderbist, F., Tulkens, P. M., and Van Bambeke, F. (2012). Increased susceptibility of Pseudomonas aeruginosa to macrolides and ketolides in eukaryotic cell culture media and biological fluids due to decreased expression of oprM and increased outer-membrane permeability. Clin. Infect. Dis. 55, 534–542. doi: 10.1093/cid/cis473
Cabot, G., Ocampo-Sosa, A. A., Dominguez, M. A., Gago, J. F., Juan, C., Tubau, F., et al. (2012). Genetic markers of widespread extensively drug-resistant Pseudomonas aeruginosa high-risk clones. Antimicrob. Agents Chemother. 56, 6349–6357. doi: 10.1128/aac.01388-12
Chalmers, J. D. (2017). Macrolide resistance in Pseudomonas aeruginosa: implications for practice. Eur. Respir. J. 49:1700689. doi: 10.1183/13993003.00689-2017
Chawanpaiboon, S., Vogel, J. P., Moller, A. B., Lumbiganon, P., Petzold, M., Hogan, D., et al. (2019). Global, regional, and national estimates of levels of preterm birth in 2014: a systematic review and modelling analysis. Lancet Glob. Health 7, e37–e46.
Cherny, K. E., and Sauer, K. (2019). Pseudomonas aeruginosa requires the DNA-specific endonuclease EndA to degrade extracellular genomic DNA to disperse from the biofilm. J. Bacteriol. 201:e00059-19.
Ciofu, O., and Tolker-Nielsen, T. (2019). Tolerance and resistance of Pseudomonas aeruginosa biofilms to antimicrobial agents-how P. aeruginosa can escape antibiotics. Front. Microbiol. 10:913. doi: 10.3389/fmicb.2019.00913
Cipolli, M., Cazzola, G., Novelli, A., Cassetta, M. I., Fallani, S., and Mazzei, T. (2012). Azithromycin concentrations in serum and bronchial secretions of patients with cystic fibrosis. Clin. Drug Invest. 21, 353–360. doi: 10.2165/00044011-200121050-00005
Clement, A., Tamalet, A., Leroux, E., Ravilly, S., Fauroux, B., and Jais, J. P. (2006). Long term effects of azithromycin in patients with cystic fibrosis: a double blind, placebo controlled trial. Thorax 61, 895–902.
Cogen, J. D., Onchiri, F., Emerson, J., Gibson, R. L., Hoffman, L. R., Nichols, D. P., et al. (2018). Chronic Azithromycin use in cystic fibrosis and risk of treatment-emergent respiratory pathogens. Ann. Am. Thorac. Soc. 15, 702–709.
Dalhoff, A. (2014). Pharmacokinetics and pharmacodynamics of aerosolized antibacterial agents in chronically infected cystic fibrosis patients. Clin. Microbiol. Rev. 27, 753–782. doi: 10.1128/cmr.00022-14
de Kievit, T. R., and Iglewski, B. H. (2000). Bacterial quorum sensing in pathogenic relationships. Infect. Immun. 68, 4839–4849. doi: 10.1128/iai.68.9.4839-4849.2000
Ding, F., Oinuma, K. I., Smalley, N. E., Schaefer, A. L., Hamwy, O., Greenberg, E. P., et al. (2018). The Pseudomonas aeruginosa orphan quorum sensing signal receptor QscR regulates global quorum sensing gene expression by activating a single linked operon. mBio 9: e01274-18.
Equi, A., Balfour-Lynn, I. M., Bush, A., and Rosenthal, M. (2002). Long term azithromycin in children with cystic fibrosis: a randomised, placebo-controlled crossover trial. Lancet 360, 978–984. doi: 10.1016/s0140-6736(02)11081-6
Faure, E., Kwong, K., and Nguyen, D. (2018). Pseudomonas aeruginosa in chronic lung infections: how to adapt within the host? Front. Immunol. 9:2416. doi: 10.3389/fimmu.2018.02416
Favre-Bonte, S., Kohler, T., and Van Delden, C. (2003). Biofilm formation by Pseudomonas aeruginosa: role of the C4-HSL cell-to-cell signal and inhibition by azithromycin. J. Antimicrob. Chemother. 52, 598–604. doi: 10.1093/jac/dkg397
Geisenberger, O., Givskov, M., Riedel, K., Hoiby, N., Tummler, B., and Eberl, L. (2000). Production of N-acyl-L-homoserine lactones by P. aeruginosa isolates from chronic lung infections associated with cystic fibrosis. FEMS Microbiol. Lett. 184, 273–278. doi: 10.1016/s0378-1097(00)00059-8
Ghosh, A., Jayaraman, N., and Chatterji, D. (2020). Small-molecule inhibition of bacterial biofilm. ACS Omega 5, 3108–3115. doi: 10.1021/acsomega.9b03695
Hansen, C. R., Pressler, T., Koch, C., and Hoiby, N. (2005). Long-term azitromycin treatment of cystic fibrosis patients with chronic Pseudomonas aeruginosa infection; an observational cohort study. J. Cyst. Fibros. 4, 35–40. doi: 10.1016/j.jcf.2004.09.001
Hoover, J. L., Lewandowski, T. F., Mininger, C. L., Singley, C. M., Sucoloski, S., and Rittenhouse, S. (2017). A robust pneumonia model in immunocompetent rodents to evaluate antibacterial efficacy against S. pneumoniae, H. influenzae, K. pneumoniae, P. aeruginosa or A. baumannii. J. Vis. Exp. 119:e55068. doi: 10.3791/55068
Hraiech, S., Bregeon, F., Brunel, J. M., Rolain, J. M., Lepidi, H., Andrieu, V., et al. (2012). Antibacterial efficacy of inhaled squalamine in a rat model of chronic Pseudomonas aeruginosa pneumonia. J. Antimicrob. Chemother. 67, 2452–2458. doi: 10.1093/jac/dks230
Ichimiya, T., Takeoka, K., Hiramatsu, K., Hirai, K., Yamasaki, T., and Nasu, M. (1996). The influence of azithromycin on the biofilm formation of Pseudomonas aeruginosa in vitro. Chemotherapy 42, 186–191. doi: 10.1159/000239440
Kumar, M., Rao, M., Barman, T. K., Pandya, M., Dube, S., and Purnapatre, K. P. (2016). In-vivo efficacy of a novel Leu-t-RNA synthatase inhibitor compound a against MDR Pseudomonas aeruginosa 1594965 in a foreign body associated urinary tract infection model. Int. J. Infect. Dis. 45:129. doi: 10.1016/j.ijid.2016.02.320
Kumar, M., Rao, M., Purnapatre, K. P., Barman, T. K., Joshi, V., Kashyap, A., et al. (2019). DS86760016, a Leucyl-tRNA synthetase inhibitor with activity against Pseudomonas aeruginosa. Antimicrob. Agents Chemother. 63: e02122-18.
Lucchi, M., Damle, B., Fang, A., de Caprariis, P. J., Mussi, A., Sanchez, S. P., et al. (2008). Pharmacokinetics of azithromycin in serum, bronchial washings, alveolar macrophages and lung tissue following a single oral dose of extended or immediate release formulations of azithromycin. J. Antimicrob. Chemother. 61, 884–891. doi: 10.1093/jac/dkn032
Macia, M. D., Rojo-Molinero, E., and Oliver, A. (2014). Antimicrobial susceptibility testing in biofilm-growing bacteria. Clin. Microbiol. Infect. 20, 981–990. doi: 10.1111/1469-0691.12651
Marvig, R. L., Sondergaard, M. S., Damkiaer, S., Hoiby, N., Johansen, H. K., Molin, S., et al. (2012). Mutations in 23S rRNA confer resistance against azithromycin in Pseudomonas aeruginosa. Antimicrob. Agents Chemother. 56, 4519–4521. doi: 10.1128/aac.00630-12
Maurice, N. M., Bedi, B., and Sadikot, R. T. (2018). Pseudomonas aeruginosa biofilms: host response and clinical implications in lung infections. Am. J. Respir. Cell Mol. Biol. 58, 428–439.
Mizukane, R., Hirakata, Y., Kaku, M., Ishii, Y., Furuya, N., Ishida, K., et al. (1994). Comparative in vitro exoenzyme-suppressing activities of azithromycin and other macrolide antibiotics against Pseudomonas aeruginosa. Antimicrob. Agents Chemother. 38, 528–533. doi: 10.1128/aac.38.3.528
Mulcahy, L. R., Isabella, V. M., and Lewis, K. (2014). Pseudomonas aeruginosa biofilms in disease. Microb. Ecol. 68, 1–12.
Nalca, Y., Jansch, L., Bredenbruch, F., Geffers, R., Buer, J., and Haussler, S. (2006). Quorum-sensing antagonistic activities of azithromycin in Pseudomonas aeruginosa PAO1: a global approach. Antimicrob. Agents Chemother. 50, 1680–1688. doi: 10.1128/aac.50.5.1680-1688.2006
Principi, N., Blasi, F., and Esposito, S. (2015). Azithromycin use in patients with cystic fibrosis. Eur. J. Clin. Microbiol. Infect. Dis. 34, 1071– 1079.
Saiman, L., Marshall, B. C., Mayer-Hamblett, N., Burns, J. L., Quittner, A. L., Cibene, D. A., et al. (2003). Azithromycin in patients with cystic fibrosis chronically infected with Pseudomonas aeruginosa: a randomized controlled trial. JAMA 290, 1749–1756. doi: 10.1001/jama.290.13.1749
Sakuragi, Y., and Kolter, R. (2007). Quorum-sensing regulation of the biofilm matrix genes (pel) of Pseudomonas aeruginosa. J. Bacteriol. 189, 5383–5386. doi: 10.1128/jb.00137-07
Sharma, S., Khan, I. A., Ali, I., Ali, F., Kumar, M., Kumar, A., et al. (2009). Evaluation of the antimicrobial, antioxidant, and anti-inflammatory activities of hydroxychavicol for its potential use as an oral care agent. Antimicrob. Agents Chemother. 53, 216–222. doi: 10.1128/aac.00045-08
Singh, P. K., Schaefer, A. L., Parsek, M. R., Moninger, T. O., Welsh, M. J., and Greenberg, E. P. (2000). Quorum-sensing signals indicate that cystic fibrosis lungs are infected with bacterial biofilms. Nature 407, 762–764. doi: 10.1038/35037627
Smith, R. S., and Iglewski, B. H. (2003). Pseudomonas aeruginosa quorum sensing as a potential antimicrobial target. J. Clin. Invest. 112, 1460–1465. doi: 10.1172/jci200320364
Sutherland, I. (2001). Biofilm exopolysaccharides: a strong and sticky framework. Microbiology 147, 3–9. doi: 10.1099/00221287-147-1-3
Swatton, J. E., Davenport, P. W., Maunders, E. A., Griffin, J. L., Lilley, K. S., and Welch, M. (2016). Impact of Azithromycin on the quorum sensing-controlled proteome of Pseudomonas aeruginosa. PLoS One 11:e0147698. doi: 10.1371/journal.pone.0147698
Tateda, K., Ishii, Y., Matsumoto, T., Furuya, N., Nagashima, M., Matsunaga, T., et al. (1996). Direct evidence for antipseudomonal activity of macrolides: exposure-dependent bactericidal activity and inhibition of protein synthesis by erythromycin, clarithromycin, and azithromycin. Antimicrob. Agents Chemother. 40, 2271–2275. doi: 10.1128/aac.40.10.2271
Wagner, T., Soong, G., Sokol, S., Saiman, L., and Prince, A. (2005). Effects of azithromycin on clinical isolates of Pseudomonas aeruginosa from cystic fibrosis patients. Chest 128, 912–919. doi: 10.1378/chest.128.2.912
Willcox, M. D. P., Zhu, H., Conibear, T. C. R., Hume, E. B. H., Givskov, M., Kjelleberg, S., et al. (2008). Role of quorum sensing by Pseudomonas aeruginosa in microbial keratitis and cystic fibrosis. Microbiology (Reading) 154, 2184–2194. doi: 10.1099/mic.0.2008/019281-0
Yoon, S. S., Hennigan, R. F., Hilliard, G. M., Ochsner, U. A., Parvatiyar, K., Kamani, M. C., et al. (2002). Pseudomonas aeruginosa anaerobic respiration in biofilms: relationships to cystic fibrosis pathogenesis. Dev. Cell 3, 593–603.
Zeng, J., Zhang, N., Huang, B., Cai, R., Wu, B., E, S., et al. (2016). Mechanism of azithromycin inhibition of HSL synthesis in Pseudomonas aeruginosa. Sci. Rep. 6:24299.
Keywords: Gram-negative bacteria, PA-14, PAO1, multidrug resistance, respiratory tract infection, pel genes, extracellular biofilm matrix, quorum sensing molecule
Citation: Kumar M, Rao M, Mathur T, Barman TK, Joshi V, Chaira T, Singhal S, Pandya M, Al Khodor S, Upadhyay DJ and Masuda N (2021) Azithromycin Exhibits Activity Against Pseudomonas aeruginosa in Chronic Rat Lung Infection Model. Front. Microbiol. 12:603151. doi: 10.3389/fmicb.2021.603151
Received: 05 September 2020; Accepted: 15 March 2021;
Published: 23 April 2021.
Edited by:
Mark Smeltzer, University of Arkansas for Medical Sciences, United StatesReviewed by:
Giuseppantonio Maisetta, University of Pisa, ItalyOana Ciofu, University of Copenhagen, Denmark
Copyright © 2021 Kumar, Rao, Mathur, Barman, Joshi, Chaira, Singhal, Pandya, Al Khodor, Upadhyay and Masuda. This is an open-access article distributed under the terms of the Creative Commons Attribution License (CC BY). The use, distribution or reproduction in other forums is permitted, provided the original author(s) and the copyright owner(s) are credited and that the original publication in this journal is cited, in accordance with accepted academic practice. No use, distribution or reproduction is permitted which does not comply with these terms.
*Correspondence: Manoj Kumar, bWt1bWFyQHNpZHJhLm9yZw==
†These authors have contributed equally to this work