- 1Institute of Mycorrhizal Biotechnology, Qingdao Agricultural University, Qingdao, China
- 2Key Laboratory of Integrated Crop Pest Management of Shandong Province, College of Plant Health and Medicine, Qingdao Agricultural University, Qingdao, China
This study evaluated the effects and underlying mechanisms of different combinations of plant symbiotic microbes, comprising arbuscular mycorrhizal fungi (AMF), plant growth-promoting rhizobacteria (PGPR), and Trichoderma spp., on tomato Fusarium crown and root rot (TFCRR) resistance. A total of 54 treatments were applied in a greenhouse pot experiment to tomato (Solanum lycopersicum) seedlings inoculated with or without Funneliformis mosseae (Fm), Rhizophagus intraradices (Ri), Trichoderma virens l40012 (Tv), Trichoderma harzianum l40015 (Th), Bacillus subtilis PS1-3 (Bs), Pseudomonas fluorescens PS2-6 (Pf), and Fusarium oxysporum f. sp. radicis-lycopersici (Fo). The symbioses on the tomato root system were well developed, and the composite symbiont generated by AMF + Trichoderma spp. was observed for the first time. Compared with other treatments, Ri + Bs + Tv and Fm + Pf + Tv stimulated the greatest improvements in tomato growth and yield. The combination Ri + Pf + Th + Fo resulted in the strongest biocontrol effects on TFCRR, followed by the treatments Th + Pf + Fo and Ri + Th + Fo. Compared with the Fo treatment, most inoculation treatments improved photosynthetic performance and significantly increased defense enzyme activity in tomato plants, of which the treatment Ri + Pf + Th + Fo showed the highest enzyme activity. Metabolome analysis detected changes in a total of 1,266 metabolites. The number of up-regulated metabolites in tomato plants inoculated with Ri + Pf + Th and Ri + Pf + Th + Fo exceeded that of the Fo treatment, whereas the number of down-regulated metabolites showed the opposite trend. It is concluded that AMF + Trichoderma + PGPR is the most effective combination to promote resistance to TFCRR in tomato. The up-regulation and down-regulation of metabolites regulated by symbiotic microbial genes may be an important mechanism by which root symbiotic microorganisms promote plant growth, increase yield, and improve disease resistance.
Introduction
With the increase in prevalence of multiple cropping regimes and aggravation of obstacles to continuous cropping, soil-borne diseases increasingly restrict tomato (Solanum lycopersicum) production, especially under protected cultivation. Among such diseases, tomato Fusarium crown and root rot (TFCRR) has become a highly destructive soil-borne disease (Kucharek et al., 2000; Hibar et al., 2006; Cao et al., 2018; Li et al., 2018). Tomato production is impacted by TFCRR in more than 10 provinces and cities in China (Li et al., 2018; Wang et al., 2019).
A variety of chemical, cultivation, breeding, microbial, and management measures have been attempted to control TFCRR. At present, fungicides are mainly employed in tomato production to control soil-borne diseases. However, fungicides pollute the environment and directly contribute to toxic residues in food products, thereby endangering human health. Development of green technologies for disease prevention and control is a matter of urgency. Plant metabolites and plant-based derivative treatments have been recently used to reduce incidence of TFCRR and could replace certain chemical fungicides, such as hymexazol and benomyl (Hibar et al., 2007; Murugesan et al., 2011). Selection of resistant cultivars and grafting onto disease-resistant rootstock are effective means of prevention (Thorpe and Jarvis, 1981). However, these methods and approaches have certain limitations. Therefore, it is necessary to develop more efficient and time-saving preventive and control technologies.
Plant roots are colonized with a variety of symbiotic microorganisms, such as mycorrhizal fungi, dark septate endophytes (DSE), Trichoderma spp., Metarhizium spp., and plant growth-promoting rhizobacteria (PGPR). Each of these plant-symbiotic microorganisms may directly or indirectly inhibit infection by pathogens and promote growth of the host plant (Liu and Wang, 2018; Atalla et al., 2020; Mendes et al., 2020; Rana et al., 2020). Although some biological control agents can effectively control TFCRR in a greenhouse, the effect is more efficient when used in combination with other control measures (Colak and Bicici, 2013). Under a field environment, dual inoculation with arbuscular mycorrhizal fungi (AMF) and Bacillus sp. reduces fertilizer application by 50% of the recommended NPK fertilization without compromising crop growth, nutrition, and yield (Nanjundappa et al., 2019). Combined inoculation with Funneliformis mosseae + Bacillus sp. M3-4, or with Glomus versiforme+ Bacillus sp. M3-4 promotes the growth of potato and induces an enhanced defense response to control bacterial wilt disease compared with the benefits of single inoculations (Tan et al., 2015). Liu et al. (2016) evaluated the effects of different combinations of the AMF F. mosseae (Fm), Rhizophagus intraradices (Ri), and G. versiforme (Gv) with PGPR PR2-1, PS1-3, PS1-5, PS2-6, and PS3-2 on plant growth, resistance to Fusarium wilt, and yield of cucumber in a greenhouse pot experiment. The authors concluded that the combinations Fm + PS1-5, Fm + PS3-2, and Gv + PS2-6 were the most effective for control of Fusarium wilt among all tested treatments.
Mutualisms of plant + fungi, plant + bacteria, and fungi + bacteria often represent a reciprocal symbiotic system and form a compound symbiont in a natural ecosystem. Combined symbionts may exert more powerful functions than when inoculated alone. Field experiments show that AMF + Trichoderma harzianum effectively reduces the incidence of soil-borne disease and improves the quality of Salvia miltiorrhiza (Wang et al., 2014). Liu et al. (2019) observed improved control of tomato root knot nematode by the combined inoculation of T. harzianum + Bacillus cereus compared with that of the single strain in a field trial. T. harzianum mainly inhibits Rhizoctonia solani by reparasitism, and Bacillus subtilis inhibits R. solani by an antagonistic mechanism. The combination of these disparate inhibitory mechanisms effectively prevents potato black scurf by R. solani (Pan et al., 2020).
Symbiotic microbial agents in combination may exert their effects by regulating plant physiological metabolism. Inoculation of Fragaria × ananassa plants with the mycorrhizal preparations Mykoflor (Rhizophagus irregularis, F. mosseae, and Claroideoglomus etunicatum), MYC 800 (R. intraradices), and the bacterial preparation Rhizocell C (Bacillus amyloliquefaciens IT45) increases the transpiration rate and CO2 concentration in the intercellular spaces of the leaves, and the total number of bacteria and fungi in the soil is increased. Plants treated with MYC 800 + Rhizocell C show a higher CO2 assimilation rate than that of the control (Mikiciuk et al., 2019). The combination of AMF + PGPR reduces cucumber Fusarium wilt disease incidence and improves the disease resistance of cucumber plants by antagonizing pathogens, promoting synthesis of disease resistance signaling substances, up-regulating defense gene expression, increasing defense enzyme activity, and reducing accumulation of toxic substances in plants (Liu et al., 2017).
Although many previous studies have investigated TFCRR and its prevention, control of soil-borne diseases with chemical pesticides is difficult under a continuous cropping system. Therefore, development of green technologies for prevention and control of soil-borne diseases has attracted considerable research attention. As a symbiotic and active region of plant nutrient acquisition and strong interactions among many organisms, the rhizosphere is an important environment for the development of soil-borne diseases and is the principal location of plant–soil–microorganism interactions. A large number of symbiotic microbes colonize the root zone, restrict and promote each other, and exert diverse physiological and ecological effects. Therefore, it is of theoretical and practical importance to explore the effects and mechanisms of combinations of microbial agents composed of a variety of symbiotic microorganisms. Previously identified biocontrol agents were mostly single strains, but recent studies have revealed that many microbial agents show greater application potential in combination. These results lay a sound theoretical and practical foundation for further research on combination of symbiotic microbial agents. As important members of the plant root symbiotic microbiota, AMF, PGPR, and Trichoderma interact with each other and have been extensively studied. Compared with AMF + PGPR, inoculation with AMF + Trichoderma, Trichoderma + PGPR, and other combinations have been studied less intensively, and even fewer studies have investigated AMF + PGPR + Trichoderma combinations.
Metabolomic profiling is an increasingly important technique in the large field of systems biology (Dearth et al., 2018). Yang et al. (2020) used liquid chromatography combined with time-of-flight mass spectrometry (LC/TOF-MS) to analyze the metabolite profile of Puccinellia tenuiflora seedlings inoculated with or without AMF under alkali stress. Their findings provide insight into the metabolic mechanisms of P. tenuiflora seedlings infected with AMF in alkaline soil and clarify the role of AMF in the molecular regulation of this species under alkali stress. Lu et al. (2020) used ultra-performance liquid chromatography (UPLC) coupled with tandem mass spectrometry (MS/MS) to show that F. mosseae alleviates root rot caused by continuous cropping. The increased activity of certain disease-resistant metabolites may partly account for the disease resistance of the plants. The study provides insight into the molecular mechanism by which AMF alleviates root rot of soybean. Hill et al. (2018) performed the first metabolomic investigation into the impact of AMF colonization by R. irregularis on the chemical defenses in above- and belowground tissues of ragwort (Senecio jacobaea). An increase in the concentrations of four pyrrolizidine alkaloids in roots (but not shoots) of AMF-colonized plants was observed, which may protect colonized plants from attack by belowground organisms.
The objective of the present study was to evaluate the effect of inoculation with AMF, Trichoderma spp., and PGPR, either alone or in combinations, on resistance to TFCRR and to explore the possible mechanisms of the biocontrol effects.
Materials and Methods
Experimental Materials
Seeds of tomato ‘Ginobili’ (a cultivar sensitive to TFCRR) were purchased from Qingdao Wufeng Fruit and Vegetable Development Co. Ltd. (Qingdao, China).
The symbiotic AMF F. mosseae (Fm), R. intraradices (Ri), PGPR B. subtilis PS1-3 (Bs), and P. fluorescens PS2-6 (Pf) were obtained from the Institute of Mycorrhizal Biotechnology, Qingdao Agricultural University (QAU), China. Spores, mycorrhizal root segments, and culture media of AMF were employed as inocula with inoculation potential units determined following the method of Liu and Luo (1994).
The aforementioned PGPR species were inoculated into nutrient broth (3 g beef extract, 10 g peptone, 5 g NaCl, and distilled water to 1,000 ml) and cultured on a rotating shaker at 30°C for 3 days. The PGPR fermentation broth was diluted to 1 × 108 CFU/ml for inoculation.
Trichoderma virens 140012 (Tv), T. harzianum 140015 (Th), and the TFCRR pathogen F. oxysporum f. sp. radicis-lycopersici (Fo) were provided by the College of Plant Medicine, QAU. The Trichoderma spp. were inoculated onto potato dextrose agar (PDA) medium and incubated at 28°C for 10 days. The pellets were filtered, washed, resuspended, and diluted to 1 × 105 CFU/ml for inoculation. Fo was cultured on PDA plates for 5–7 days and a conidia suspension was prepared. The solution was diluted to 107 conidia/ml, stored at 4°C, and inoculated within 24 h.
Nutrient broth and PDA were used for isolation and culture of bacteria and fungi, respectively.
Peat (0–6 mm grade; Pindstrup Mosebrug A/S, Ryomgård, Denmark) was sterilized (121°C for 1 h). The chemical characteristics of the peat were pH 6, electrical conductivity 40 mS/m, NO3–-N 70 g/m3, NH4+-N 50 g/m3, P2O5 140 g/m3, K2O 240 g/m3, MgO 23 g/m3, B 0.4 g/m3, Mo 2.04 g/m3, Cu 1.7 g/m3, Mn 2.9 g/m3, Zn 0.9 g/m3, and Fe 8.4 g/m3. The 2.5-L pots were surface-sterilized before filling with the peat.
Experimental Design, Inoculation, and Management
A greenhouse pot experiment was conducted with tomato seedlings inoculated with (alone or in combination) the symbiotic microbes and the pathogen, and a total of 54 treatments were designed (Supplementary Table 1). A complete randomized block design was used with five replicates for each treatment. The 54 treatments comprised inoculation with or without AMF, PGPR, and Trichoderma, respectively. First, 50 g of AMF inoculum (12,000 inoculation potential units), 10 ml (1 × 108 CFU/ml) of each PGPR species inoculum, and/or 10 ml (1 × 105 CFU/ml) of Trichoderma inoculum were mixed into the peat for the inoculation treatments, respectively. The same volume of sterilized inoculum was added for the non-inoculation treatment (control). Five seeds were sown in each pot. Subsequently, three seedlings were retained in each pot. At the 2–3 leaves stage, the seedlings were inoculated by irrigation with 10 ml of Fo conidial suspension applied to the root zone. Pots were watered three times weekly and, once per week, plants were fertigated with Hoagland’s nutrient solution. The volume of nutrient solution applied to each seedling in one application was 250 ml. During the growing period, the average day/night temperatures were 19°C/27°C.
Determination of AMF Colonization, Physiological Parameters, and Metabolome Analysis of Tomato Plants
AMF Colonization and Associated Indices
Colonization by AMF was determined following the method described by Biermann and Linderman (1981). For PGPR population measurement, 1.0 g dry soil collected from the rhizosphere was added to sterilized water to prepare a soil solution. The PGPR numbers in the rhizosphere soil were counted using a dilution plate method after incubation at 30°C for 3 days (Bashan et al., 1993; Zhao and He, 2002). For Trichoderma colonization measurement, 1 g fine roots were cleaned and surface sterilized, cut into small segments and slices, and placed on PDA for culture at 25°C for 7 days. The number of effective colonies was recorded daily. The disease grade was classified as follows: grade 0, the root showed normal color; grade 1, root slightly discolored with the discolored area as much as 25% of the total root area; grade 2, roots with 26–50% of the total area showing discoloration; grade 3, 51–75% of the total root area showing discoloration; grade 4, more than 76% of the total root area is discolored or the plant is dead. The aforementioned parameters were measured at harvest.
Measurements of Plant Growth Indices and Photosynthetic Characteristics
Conventional methods were used to measure plant height, dry mass weight, fruit yield, and fruit weight per plant. Leaf photosynthetic parameters were measured using a portable photosynthesis system (LI-6400; LI-COR, Inc., Lincoln, NE, United States) between 08:00 and 11:30. Leaf gas exchange measurements were conducted on three leaves. The sampled leaves were frozen in liquid nitrogen and stored at −80°C for subsequent measurements of antioxidant enzyme activities.
Antioxidant Enzyme Activities
Fresh leaf samples (0.5 g) from tomato plants at the fruit-setting stage were homogenized with a pestle in an ice-cold mortar containing 10 ml of ice-cold 50 mM phosphate buffer (pH 7.0). The homogenate was filtered through gauze and then centrifuged at 1,500 × g for 20 min at 4°C. The supernatant (enzyme extract) was collected and used for the measurement of superoxide dismutase (SOD), catalase (CAT), peroxidase (POD), and phenylalanine ammonia-lyase (PAL) activities. Each sample was measured three times. The activities of SOD, CAT, POD, and PAL were determined according to the methods described by Wang (2006).
Metabolome Analysis
Samples of stems with young and mature leaves, and of the fine feeding roots of tomato plants at the fruit-setting stage were frozen in liquid N2 and stored at −80°C. Metabolome analysis was performed by Biomarker Technologies Co., Ltd. (Beijing, China) using non-target liquid chromatography–mass spectrometry (LC-MS; Biomarker Technologies) to identify differences in the metabolite profile among treatments. The specific experimental methods were as follows. The sample (50 mg) was added to 1 ml extract containing an internal standard (1000:2) (methanol/acetonitrile/water, 2:2:1, v/v/v; internal standard concentration 2 mg/L), swirled, and mixed for 30 s. Porcelain beads were added and the sample was homogenized at 45 Hz for 10 min, then treated with ultrasound for 10 min in an ice water bath. After standing for 1 h at −20°C, the homogenate was centrifuged at 4°C and 13,000 × g for 15 min. A sample (500 μl) of the supernatant was carefully removed from the tube. The extract was dried in a vacuum concentrator. An aliquot (150 μl) of the extract (acetonitrile/water, 1:1, v/v) was added to the dried metabolites for resolution. After vortexing for 30 s, the solution was treated with ultrasound for 10 min in an ice water bath. The solution was centrifuged at 4°C and 13,000 × g for 15 min. A sample (120 μl) of the supernatant was carefully transferred to a 2-ml injection flask and mixed with 10 μl from each sample of the QC sample for analysis. KEGG database was used for annotation and enrichment analysis of differential metabolites.
Data Analysis
The data were statistically analyzed by ANOVA with DPS 7.05 software. Fisher’s protected least significant difference (LSD) was used to compare the means at 5% level of significance. The data in the table were mean ± SE.
Results
Root Symbionts Formed With the Symbiotic Microbes
The tested AMF, PGPR, Trichoderma spp., and their combinations colonized tomato roots and the rhizosphere soil. Among these microorganisms, under the condition of single inoculation and Fo inoculation, the colonization frequency of T. virens was significantly higher than that of T. harzianum, which were 1.1 and 1.2 times of that, respectively. Both Fm and Pf increased colonization by the other microorganism. Inoculation with Fm, Ri, and Pf enhanced the colonization frequency of T. harzianum, whereas Fo reduced colonization by AMF, PGPR, and Trichoderma spp. to different degrees. The AMF and Trichoderma sp. simultaneously colonized roots to form dual symbionts (Table 1).
Effects of Symbiotic Microbes on Plant Growth, Development, and TFCRR
Inoculation with Fm, Ri, Bs, Pf, Th, Tv, and their combination treatments increased the growth of tomato plants, and promoted early flowering, percentage fruit set, number of fruit, and yield per plant to various degrees. The treatments Ri + Bs + Tv and Fm + Pf + Tv showed the most highly significant effects. Under the condition of Fo inoculation, the effect of the treatment Ri + Pf + Th was greatest (Table 2).

Table 2. Influences of AMF, PGPR, Trichoderma spp., and Fusarium oxysporum f. sp. radicis-lycopersici on tomato growth and yield.
Single inoculation, and the combinations of two or three symbiotic microorganisms, reduced the incidence and disease index of TFCRR, but the effects differed among treatments. The treatment Ri + Pf + Th + Fo showed the most significant effect, followed by the Th + Pf + Fo and Ri + Th + Fo treatments. Multivariate analysis of variance showed that there were significant interactions among AMF, PGPR, and Trichoderma spp. (Table 3).
Effects of Symbiotic Microbes on Photosynthesis and Antioxidant Enzyme Activities in Tomato Leaves
Most of the photosynthesis parameters, such as net photosynthetic rate (Pn), stomatal conductance (Gs), intercellular carbon dioxide concentration (Ci), and transpiration rate (Tr), of tomato leaves treated with Ri, Bs, Tv + Bs, Tv + Pf, Ri + Bs + Tv, and Ri + Pf + Tv were significantly higher than those of the non-inoculated control. Compared with the Fo treatment, most of the inoculation treatments showed improved photosynthetic performance (Table 4).
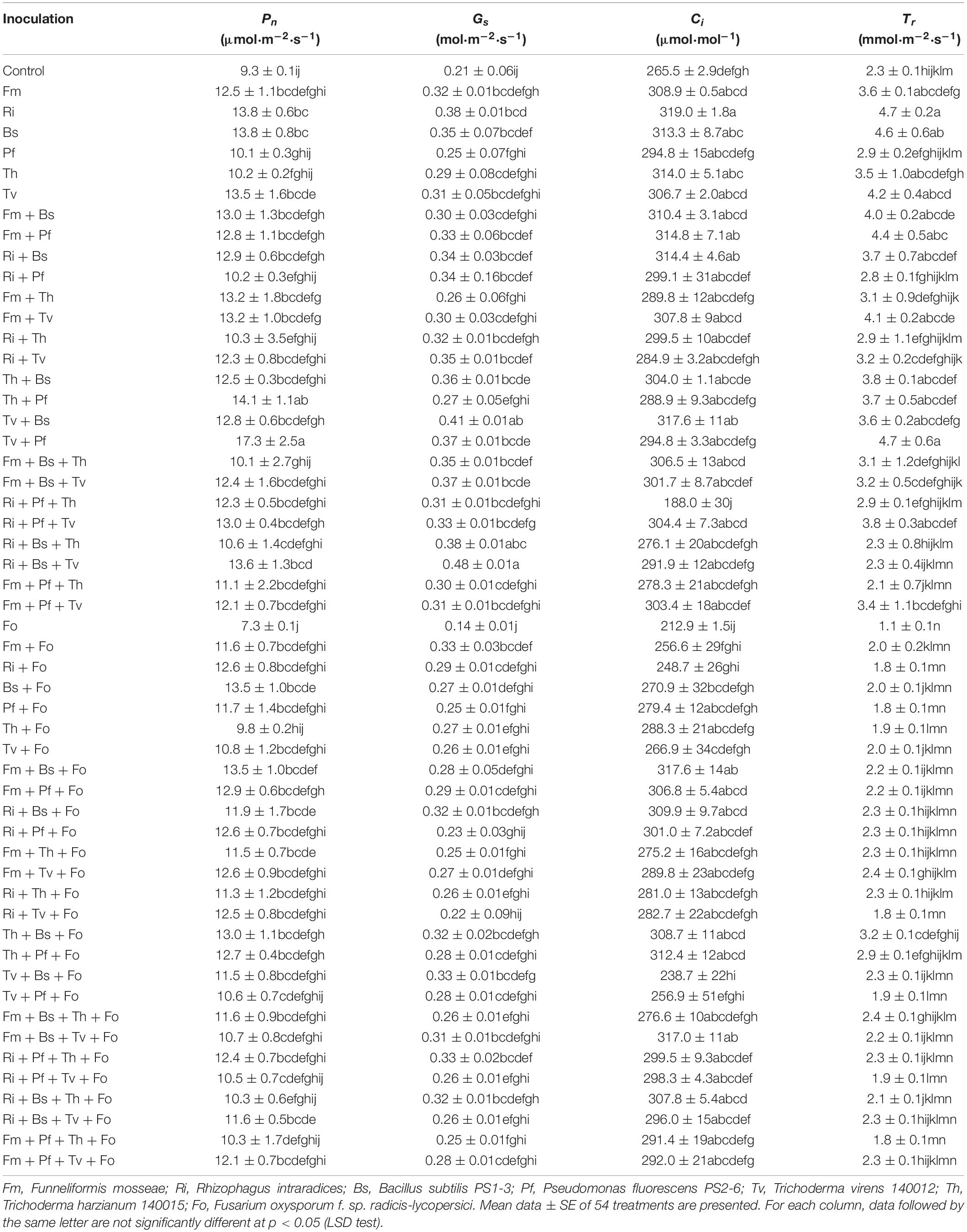
Table 4. Influences of AMF, PGPR, Trichoderma spp., and Fusarium oxysporum f. sp. radicis-lycopersici on tomato leaf photosynthetic performance.
In addition, most of the inoculation treatments significantly increased the antioxidant enzyme activity of tomato plants. Among these enzymes, POD, SOD, CAT, and PAL showed the highest activity in the treatment Ri + Pf + Th + Fo, followed by Fm + Pf + Th + Fo, Fm + Pf + Tv + Fo, Ri + Bs + Tv + Fo, Ri + Th + Fo, and Th + Pf + Fo (Table 5).
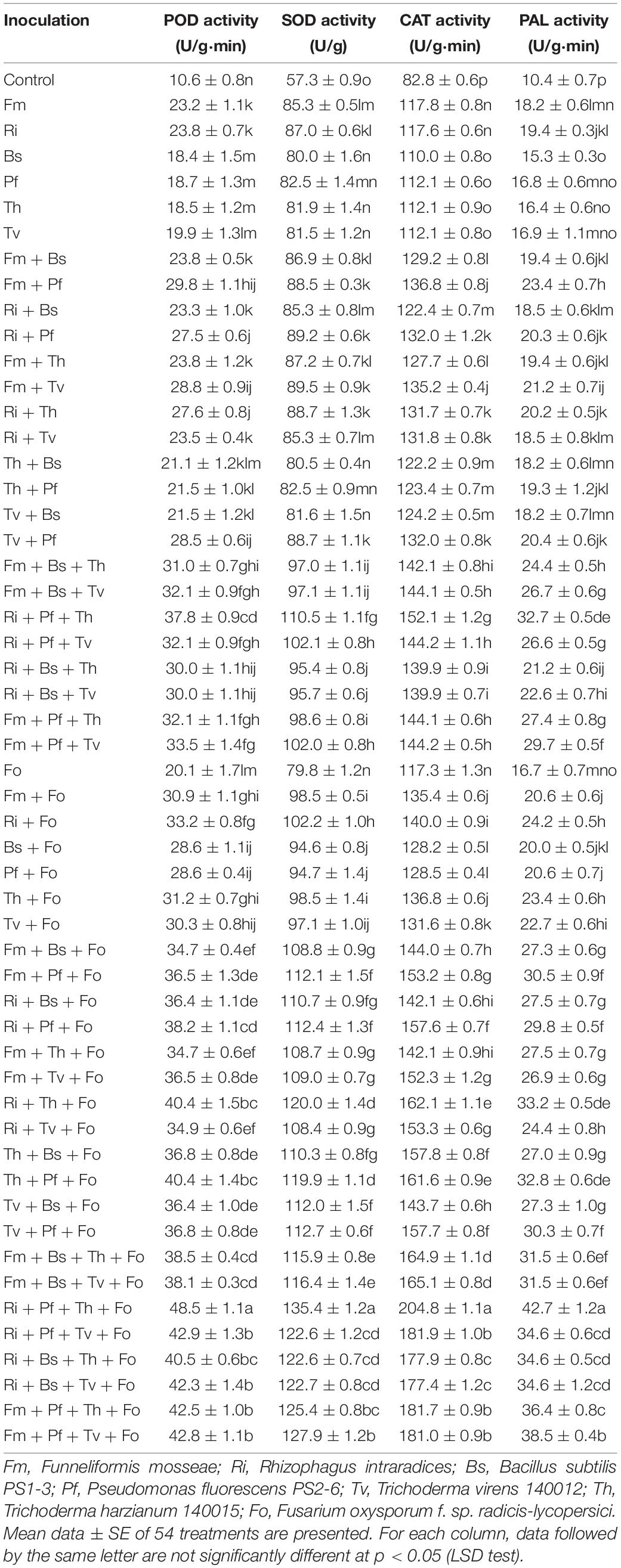
Table 5. Influences of AMF, PGPR, Trichoderma, and Fusarium oxysporum f. sp. radicis-lycopersici on the antioxidant enzyme activity in tomato leaves.
Effects of Symbiotic Microbes on Physiological Metabolism of Tomato Plants
Using non-target LC-MS, metabolome analysis was conducted on the shoots (S) and roots (R) of control (1), Ri + Pf + Th (22), Fo (28), and Ri + Pf + Th + Fo (49) treatments. Principal component analysis (PCA) and partial least squares discriminant analysis were used to analyze the detected MS data to determine the final difference in charge-to-mass ratio, which was then compared with the KEGG metabolite database, and the difference in metabolite content and species among treatments was identified.
Overview of Metabolite Profiles Using PCA and Hierarchical Cluster Analysis
Principal component analysis was performed to reduce the dimensionality of the data and visualize the relationships among the 24 samples. The first principal component (PC1) explained 87.99% of the total variation and the second principal component (PC2) explained 3.24% of the variation across the data set (Figure 1). The responses of the aboveground and underground organs to the different inoculation treatments were well separated by PC1. Regardless of the control group and the treatment group, no significant difference in the metabolites of the aboveground or underground organs was detected, whereas the metabolites differed between the aboveground and underground organs, indicating that aboveground and belowground metabolism of the plant differed significantly. In addition, the biological replicates were projected closely in multidimensional space, which indicated that the replicates showed a strong correlation. Cluster heat map analysis was conducted for 24 samples, of which all samples were clustered into four main groups and the samples from the same organ were clustered together (Figure 2). A higher number of metabolites were up-regulated in the shoot, whereas a greater number of metabolites were down-regulated in the root of tomato plants (Figure 2). Thus, significant differences in metabolism between aboveground and underground organs of the plant were demonstrated. Correlation analysis among the 24 samples showed that each treatment showed high repeatability. Collectively, these results indicated that the data were reliable and the experiment was meaningful.
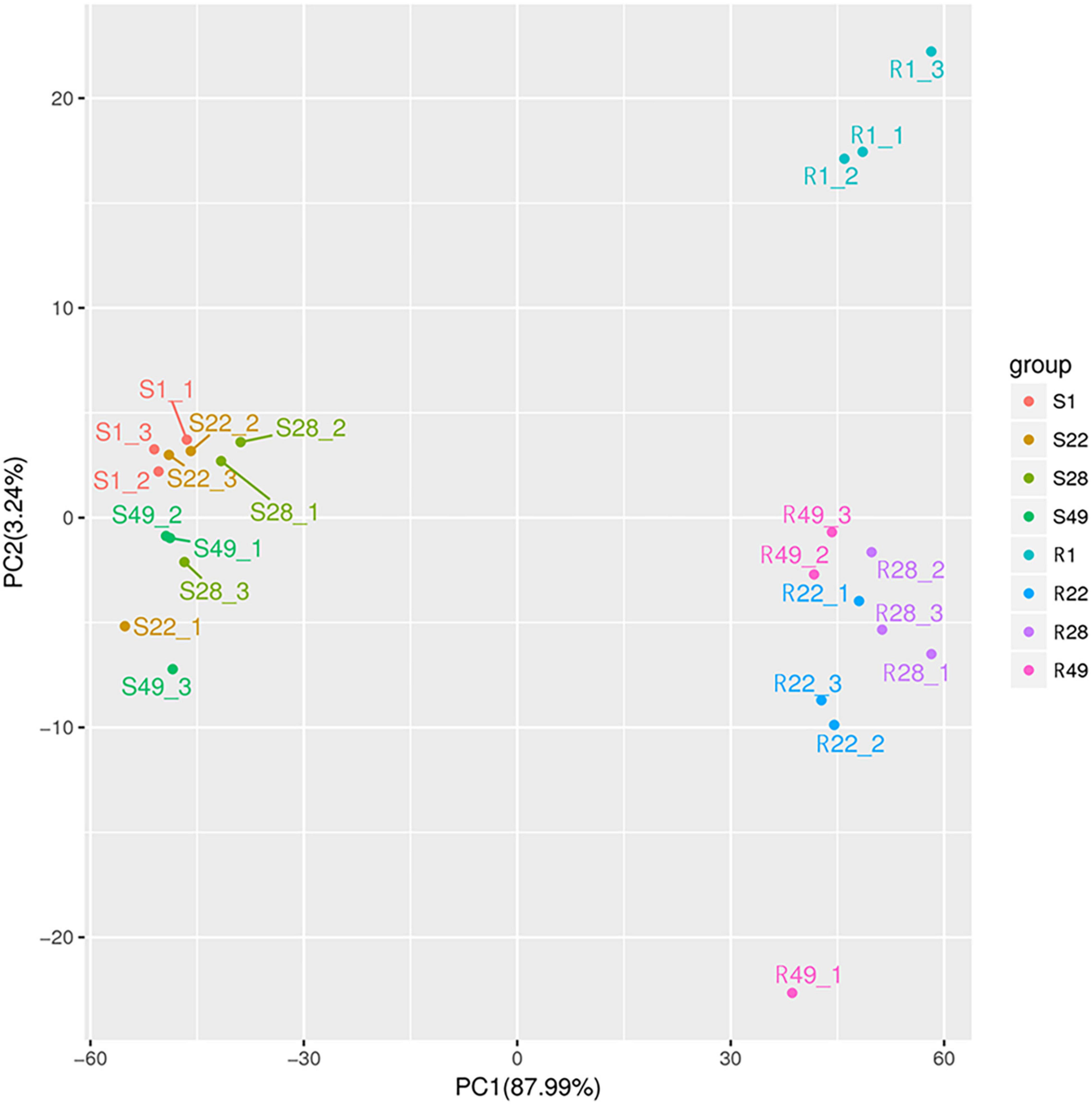
Figure 1. Principal component analysis of tomato shoot (S1, S22, S28, and S49) and root (R1, R22, R28, and R49). 1, Control; 22, Ri + Pf + Th; 28, Fo; 49, Ri + Pf + Th + Fo.
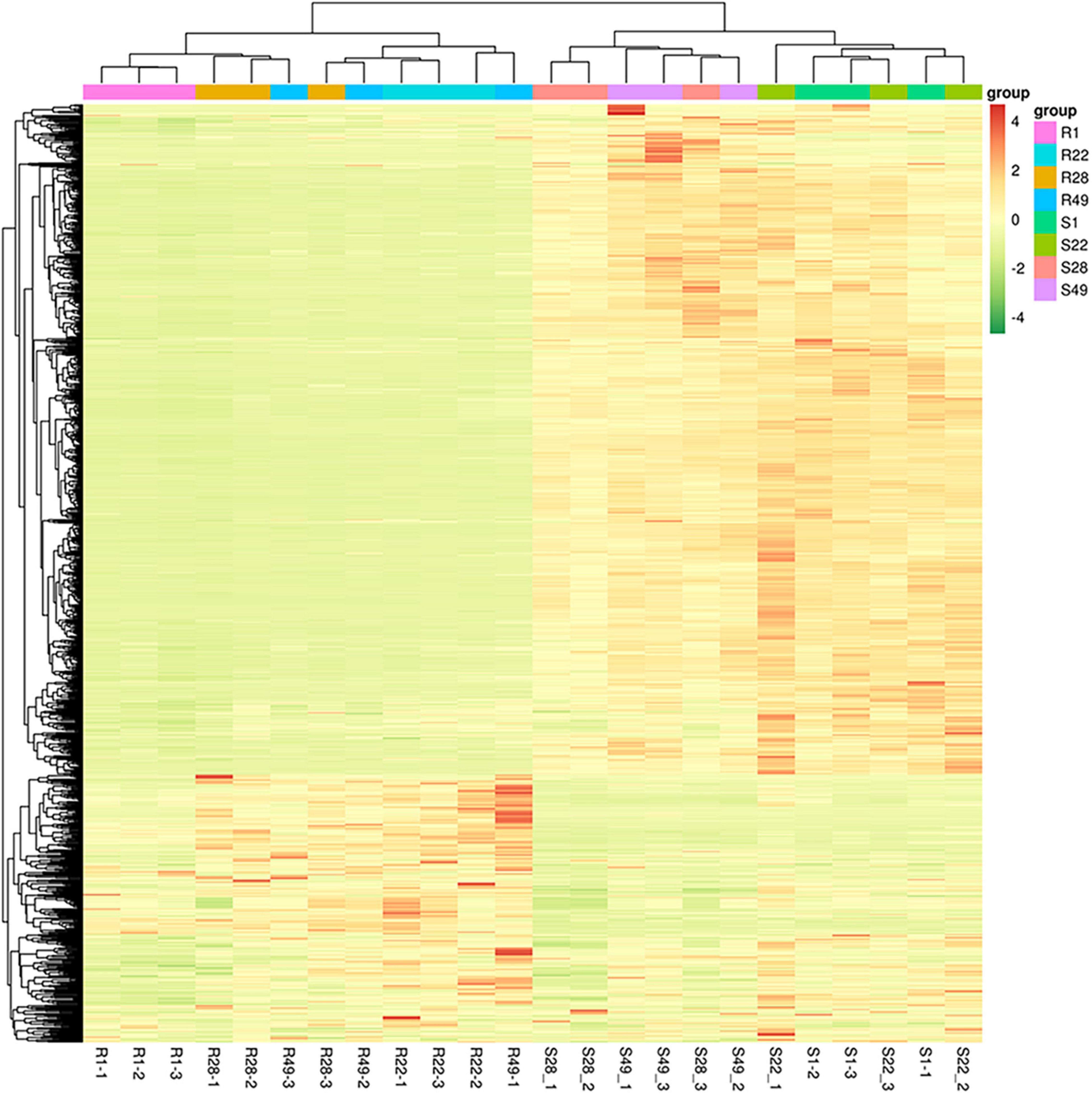
Figure 2. Hierarchical cluster analysis of differential metabolites in tomato shoots (S1, S22, S28, and S49) or roots (R1, R22, R28, and R49). 1, Control; 22, Ri + Pf + Th; 28, Fo; 49, Ri + Pf + Th + Fo.
Differential Metabolite Analysis
A total of 1,266 differential metabolites were detected in the metabolome analysis. With regard to the aboveground organs of tomato, compared with the S1 group, the number of up-regulated metabolites in the S22 treatment group exceeded the number of down-regulated metabolites, whereas the number of down-regulated metabolites in the S28 treatment group was significantly increased and was 44 times higher than that of up-regulated metabolites. The number of metabolites down-regulated in the S49 treatment group was more than the number that were up-regulated. However, after inoculation with Ri + Pf + Th, in tomato shoots the number of down-regulated metabolites decreased and the number of up-regulated metabolites increased (Figure 3 and Supplementary Figure 2).
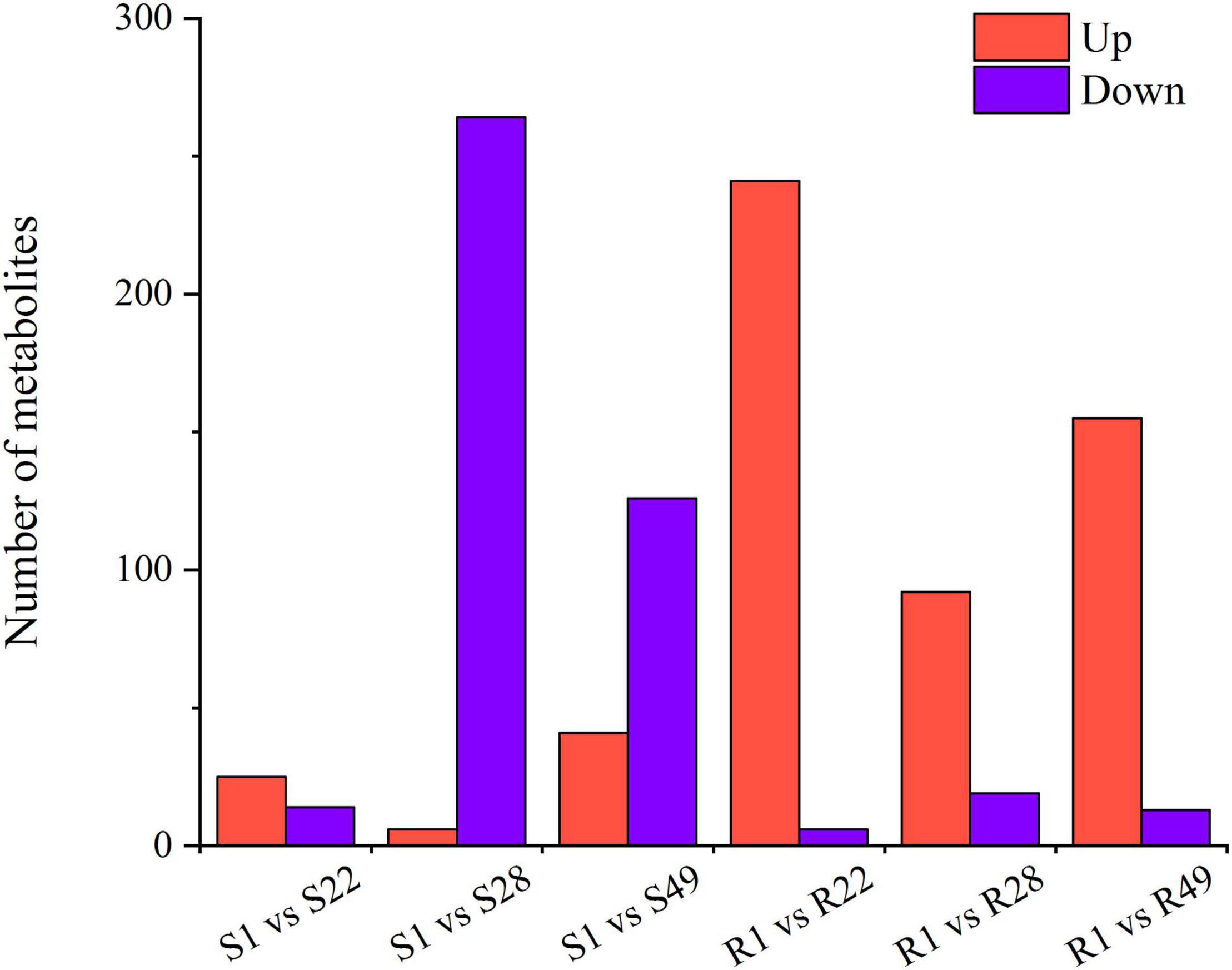
Figure 3. Differential metabolites of S1 vs. S22, S1 vs. S28, S1 vs. S49, R1 vs. R22, R1 vs. R28, and R1 vs. R49. 1, Control; 22, Ri + Pf + Th; 28, Fo; 49, Ri + Pf + Th + Fo.
With regard to the roots of tomato, the number of up-regulated metabolites in the R22, R28, and R49 treatment groups was significantly higher than that in the R1 group, and the number of up-regulated metabolites in the R22 treatment group was 40 times higher than the number of down-regulated metabolites. However, compared with the plants inoculated with Fo, the number of up-regulated metabolites increased by 63 and the number of down-regulated metabolites decreased by 6 in tomato inoculated with Ri + Pf + Th (Figure 3 and Supplementary Figure 2).
Thus, it was noted that symbiotic microorganisms or pathogens mainly affected the amount of down-regulated metabolites in the shoots of tomato and participated in the metabolic regulation of aboveground organs. In contrast, in the roots of tomato plants, the number of up-regulated metabolites was more strongly affected by symbiotic microorganisms or pathogens. In addition, significant differences in secondary metabolism between the shoots and roots of tomato plants were observed (Figure 3 and Supplementary Figure 2).
We screened the differential metabolites using the criteria variable importance plot >1 and p-value < 0.05 based on a partial least squares-discriminant analysis model of the shoots and roots from different treatments (Supplementary Figure 1).
For the shoot of tomato, it was observed that nine of the top 20 differential metabolites were detected in the Ri + Pf + Th treatment compared with the Fo treatment, comprising four up-regulated and five down-regulated metabolites. Inoculation with Ri + Pf + Th promoted the synthesis of acetylspiramycin and 6-hydroxypentadecanedioic acid, but inhibited the production of glucocaffeic acid, vanillic acid-4-O-glucuronide, and 6-caffeoylsucrose (Table 6). In addition, compared with the Fo treatment, 13 of the top 20 differential metabolites were detected in the shoot in the Ri + Pf + Th + Fo group, comprising four up-regulated and nine down-regulated metabolites. Inoculation with Ri + Pf + Th promoted the synthesis of fucosyllactose, zidovudine, and dihydrovaltrate, but inhibited the production of picolinic acid, curcumin, indoleacetic acid, buchanine, and other metabolites (Table 7).
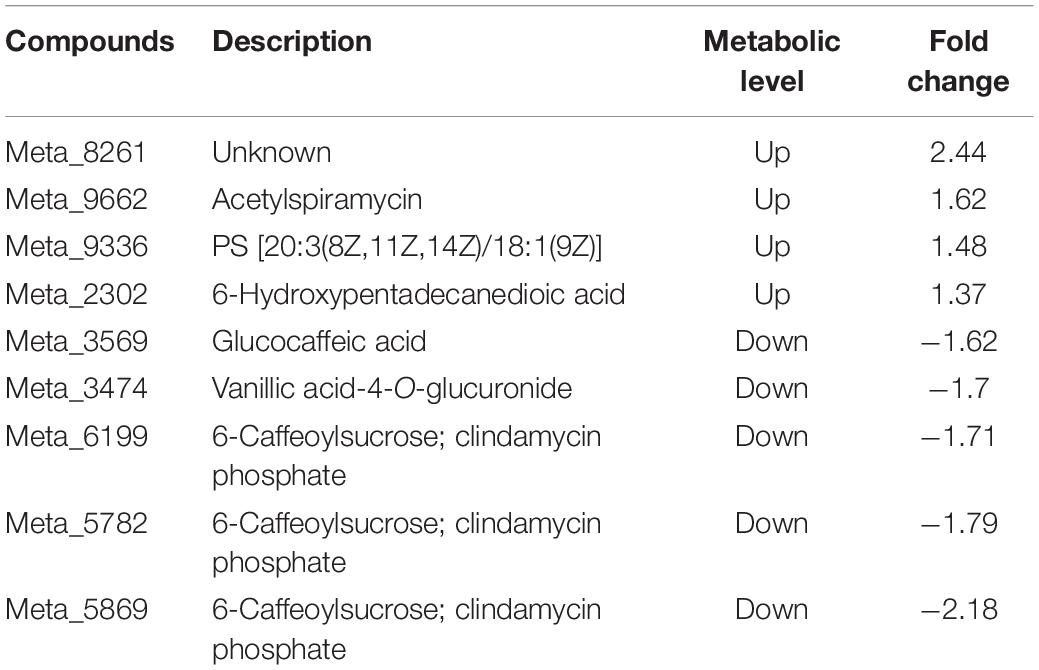
Table 6. Nine differential metabolites detected in the Ri + Pf + Th treatment compared with Fo treatment from tomato shoots.
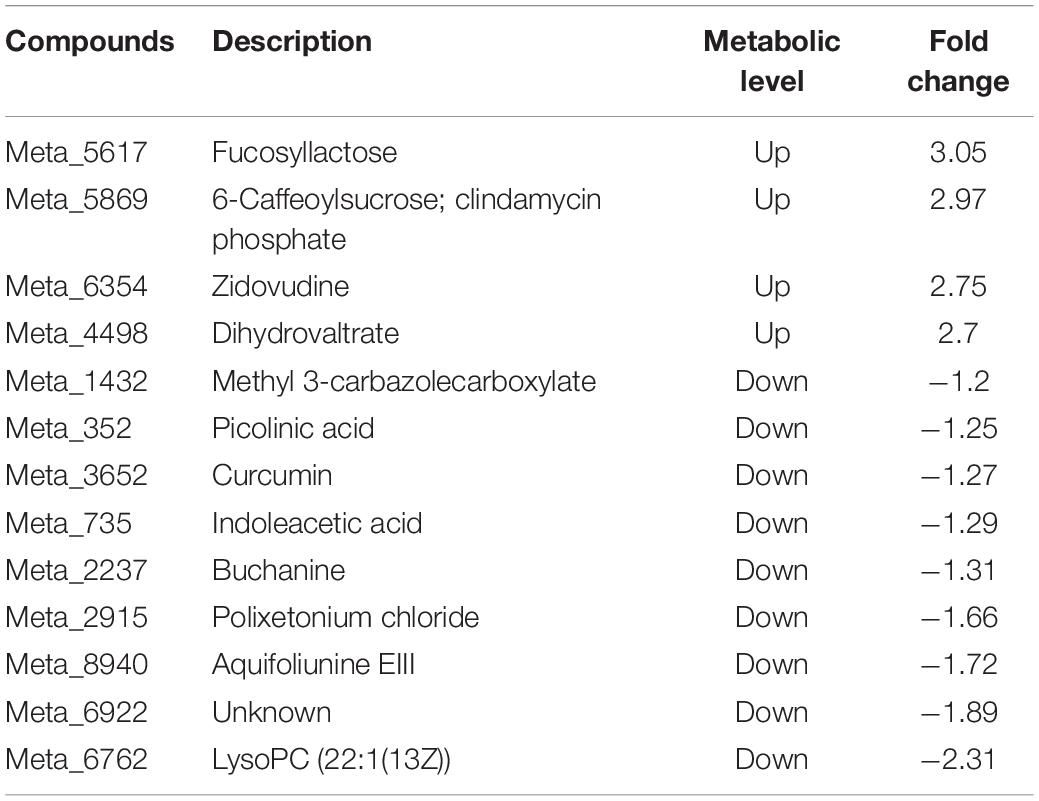
Table 7. Thirteen differential metabolites detected in the Ri + Pf + Th + Fo treatment compared with Fo treatment from tomato shoots.
Compared with inoculation with Fo, 10 of the top 20 differential metabolites were detected in the root after Ri + Pf + Th + Fo inoculation. The metabolites lactosamine, lucuminic acid, cyclomorusin, primidone, icaceine, and 3β,15α-diacetoxylanosta-8,24-dien-26-oic acid were up-regulated, whereas argiopinin I, 16-hydroxyhexadecanoic acid, pantoyllactone glucoside, and sinapoylspermine were down-regulated in the Ri + Pf + Th treatment (Table 8). In addition, compared with the Fo treatment, 11 of the top 20 differential metabolites were detected in the Ri + Pf + Th + Fo treatment. The metabolites lucuminic acid, cyclomorusin, and lactosamine were highly accumulated, whereas quercetin 3-arabinoside 7-glucoside, quercetin 3-glucoside 7-xyloside, and 3-hexadecanoyloleanolic acid were down-regulated in Ri + Pf + Th + Fo treatment (Table 9).
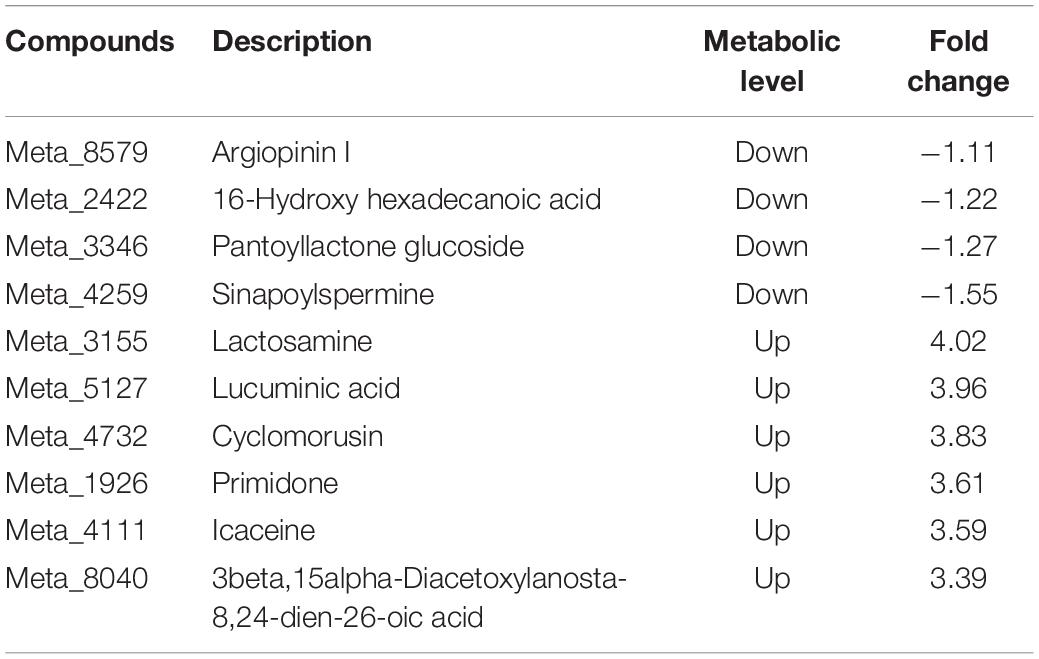
Table 8. Ten differential metabolites detected in the Ri + Pf + Th treatment compared with Fo treatment in tomato roots.
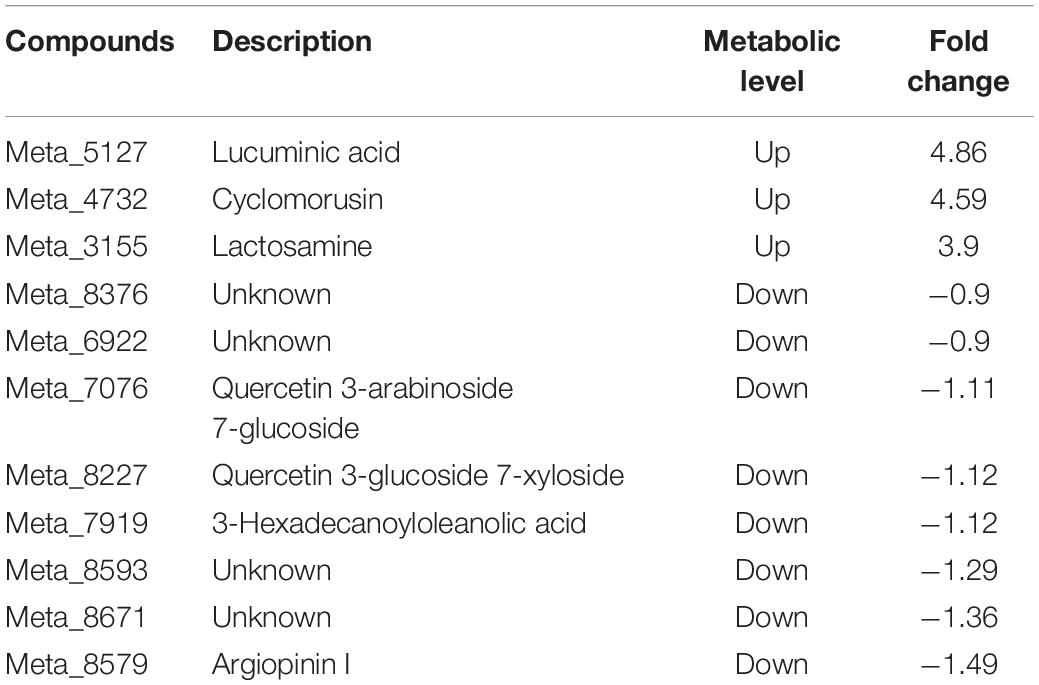
Table 9. Eleven differential metabolites detected in the Ri + Pf + Th + Fo treatment compared with Fo treatment in tomato roots.
A KEGG enrichment analysis was performed (Supplementary Figure 3). Nicotinate and nicotinamide metabolism pathways were the most abundant pathways in tomato shoots after inoculation with Ri + Pf + Th and constituted 28% of the differential metabolites detected. After single inoculation with Fo, arginine and proline metabolism was most strongly enriched pathway in the shoots and comprised 15% of the metabolites, followed by nicotinate and nicotinamide metabolism and butanoate metabolism (12%). After inoculation with Ri + Pf + Th + Fo, 17% of the total metabolites were mainly enriched in the histidine metabolism, glycerophospholipid metabolism, and tyrosine metabolism pathways in tomato shoots (Figure 4).
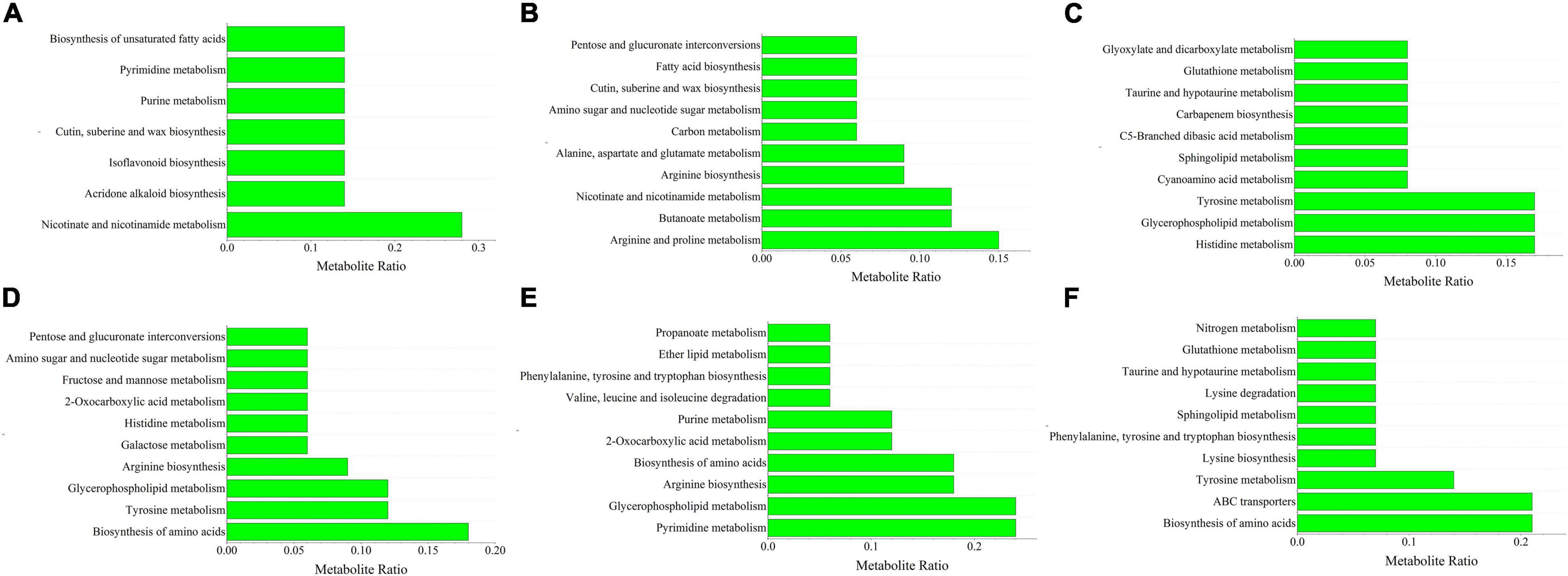
Figure 4. Top 10 metabolites ratio of S1 vs. S22 (A), S1 vs. S28 (B), S1 vs. S49 (C), R1 vs. R22 (D), R1 vs. R28 (E), and R1 vs. R49 (F). 1, Control; 22, Ri + Pf + Th; 28, Fo; 49, Ri + Pf + Th + Fo.
Among the top 10 enriched pathways, 18% of the total metabolites were involved in biosynthesis of amino acids in tomato roots inoculated with Ri + Pf + Th, and 12% of the metabolites were enriched with the tyrosine metabolism and glycerophospholipid metabolism pathways. After single inoculation with Fo, 24% of the metabolites in the roots were enriched in the pyrimidine metabolism and glycerophospholipid metabolism pathways, and 18% of the metabolites were enriched in the arginine biosynthesis and amino acid biosynthesis pathways. The amino acid biosynthesis and ABC transporters pathways were the most strongly enriched pathways in tomato roots after inoculation with Ri + Pf + Th + Fo, accounting for 21% of the total metabolites, whereas 14% of the metabolites were involved in the tyrosine metabolism pathway (Figure 4). It was speculated that the amino acid biosynthesis pathway played an important role in promoting root growth and development of tomato, and the ABC transporters pathway may play a pivotal role in resistance to pathogenic bacteria in tomato roots.
Discussion
Increasing research attention is focused on disease control by symbiotic microbe combinations. Kabdwal et al. (2019) conducted growth promotion and disease control experiments on tomato using T. harzianum (Th43), P. fluorescens (Pf173), R. intraradices, and mancozeb, which was applied in different combinations as a soil application, seedling treatment, or foliar spray. The authors observed that soil treated with Th43 + Pf173 + R. intraradices and seedlings treated with Th43 + Pf173 + three applications of mancozeb as a foliar spray were highly effective in reducing plant mortality, promoting plant growth, and increasing yield. A field trial by Kumar et al. (2018) showed that Glomus mosseae and Glomus fasciculatum + Trichoderma viride (TR) + Azotobacter chroococcum (AZ) combinations had the strongest effect on litchi growth. In the present study, we observed that the combinations Ri + Bs + Tv and Fm + Pf + Tv had the greatest growth-promoting effects on tomato, whereas the combination Ri + Pf + Th had the greatest biological control effect against Fo. Differing from non-symbiotic microbial biocontrol agents, symbiotic microorganisms were used in the present experiment. These AMF, PGPR, and Trichoderma spp. colonized the rhizosphere as well as the root system, thereby forming a mutual symbiosis. Trichoderma spp. not only exist widely in the soil but also colonize the surface and internal tissues of plant roots, stems, and leaves. Zhang et al. (2013) observed that T. harzianum T-E5 colonized the root system of cucumber in a hydroponic culture system; first, the mycelia covered the root surface, then gradually extended into the cortex of the root, and survived in the epidermis and exodermis of the root for a long period. In the present experiment, both T. viride and T. harzianum colonized tomato root cortical cells but differed in their colonization ability, especially after inoculation with a pathogenic fungus. The colonization frequency of T. viride was higher than that of T. harzianum, thus it could be inferred that the colonization ability of the former species was stronger, which may affect the capability to prevent and control disease. The present results also confirmed this point. This finding is of great importance and warrants further systematic investigation. Furthermore, we observed that Trichoderma spp. colonized tomato roots together with AMF to form complex symbionts, and played a synergistic role with AMF, or concurrently exerted an ecological effect in collaboration with AMF + PGPR. This is the first observation of a complex symbiont formed by AMF + Trichoderma. The present experiment not only confirmed the aforementioned effects of Trichoderma but further demonstrated that Trichoderma played a synergistic role in combination with AMF or formed a biologically effective collaboration with AMF + PGPR. Therefore, it is of practical importance to further investigate the physiological and ecological functions and mechanism of the AMF + Trichoderma + PGPR symbiosis.
Symbiotic microorganisms function by direct synthesis of secondary metabolites, such as phytohormones and antibiotics, regulation of plant-related gene expression, and regulation of the community structure of other organisms. In the process of coevolution of plants and pathogens, complex and diverse defense systems have developed. Among these systems, many metabolites produced by plant metabolic pathways are an important material basis of plant resistance and play an important role in the plant defense system. Using LC-MS technology, Tian et al. (2019) reported that resistant and susceptible tobacco cultivars infected by Meloidogyne incognita both showed significant changes in the metabolic pathways associated with disease resistance. The differential metabolites identified included alkaloids, fatty acids, flavonoids, terpenoids, and polyketones. A total of 1,374 proteins and 33 small molecular metabolites in grape leaves were differentially expressed by 1.5 times (p < 0.05) after infection by Botrytis cinerea for 3 days. Infection by B. cinerea had the greatest influence on expression of chloroplast proteins and mainly affected the three signaling pathways of plant disease interaction, plant hormone synthesis, and alkaloid synthesis. In addition, full activation of the disease resistance signaling pathway mediated by salicylic acid was an effective means to resist infection by B. cinerea in grape leaves (Fang et al., 2019). The present metabolome analysis provided results similar to the aforementioned findings, with a total of 1,266 differential metabolites detected and a large number of unknown metabolites. The results showed that AMF + PGPR + Trichoderma inoculation promoted the synthesis of secondary metabolites, including antibiotics and acids, in tomato leaves, but inhibited the synthesis of certain hydrocarbons. Furthermore, this combination promoted the synthesis of antibiotics, acids, and other hydrocarbons in the roots, but inhibited the secretion of acids, glycosides, and certain nitrogen-containing compounds by the roots. In contrast, AMF + PGPR + Trichoderma + Fo co-inoculation promoted the synthesis of secondary metabolites, including saccharides, nucleoside compounds, and hydrocarbons in tomato leaves, but inhibited the synthesis of certain pigments, auxin, and bactericide. Furthermore, the combination promoted the synthesis of glycosides and hydrocarbons in roots, but inhibited secretion of certain glycoside compounds by the roots. The number of metabolites up-regulated and down-regulated in each combination differed significantly, but the specific differences in metabolites and metabolic pathways require further study. The results indicated that a compound microbial agent generated by a certain combination of multiple symbiotic microorganisms may be more effective than a single microbial agent. Therefore, in future research and development of green technologies for prevention and control of TFCRR, the screening and evaluation of compound symbiotic bacteria should be a focus.
The present results may help to develop microbial strain resources and to use the advantages of multiple microbial strains. This will contribute to the development of novel microbial pesticides and biofertilizers for effective pest control. It will be helpful to determine whether there are antagonistic effects between the symbiotic microbes, in addition to their growth-promoting effect, when inoculated in combinations. The present study focused on biological disease prevention as a measure to provide a theoretical basis to eliminate the environmental damage caused by abuse of chemical fertilizers and pesticides. The findings also provide guidance for further research and development of green disease prevention and control technologies and promote agricultural sustainability and economic development.
Given the temporal and spatial limitations of the present experiment, environmental factors such as light, moisture, temperature, and insect pests were not considered but may impact on the biocontrol effectiveness of combinations of AMF, PGPR, and Trichoderma spp. In addition, the metabolome analysis in this experiment has certain shortcomings, such as less processing number. Therefore, single- and dual-inoculation treatments should be analyzed further in future experiments to obtain more complete metabolite profiles and clarify the metabolic pathways that promote tomato growth and resistance to TFCRR. Simultaneous analysis of gene expression and the transcriptome could be conducted. Optimization of the promotive effect of different types of symbiotic microbes and minimization of antagonistic interactions, thereby producing more effective biocontrol bacterial agents, will further contribute to development of green technologies for disease prevention and control.
Conclusion
Applications of symbiotic microbes, alone or in combinations, influence leaf photosynthesis, defense enzyme activities, disease occurrence, growth and development, and yield of tomato. Combinations of AMF, PGPR, and Trichoderma, such as R. intraradices + P. fluorescens + T. harzianum, have strong biocontrol effects on TFCRR. Therefore, this symbiotic microbe combination shows potential for development as an effective biocontrol agent.
Data Availability Statement
The original contributions generated for this study are included in the article/Supplementary Material, further inquiries can be directed to the corresponding author.
Author Contributions
XC and ML conceived, designed, carried out the experiments, and collected and analyzed the data. HZ and CL prepared the experimental materials. XC wrote the first draft of the manuscript. RL and ML revised the manuscript. All authors approved the final version of the article for publication.
Funding
This research was supported by the Major Scientific Innovation Project of Shandong Province (2019JZZY010715) and the Key technology Research and Development of Shandong Province (2019GNC106043).
Conflict of Interest
The authors declare that the research was conducted in the absence of any commercial or financial relationships that could be construed as a potential conflict of interest.
Acknowledgments
We thank Robert McKenzie, from Liwen Bianji, Edanz Editing China (www.liwenbianji.cn/ac), for editing the English text of a draft of this article. We also thank Yinglong Chen, from University of Western Australia for his comments on the draft of this work.
Supplementary Material
The Supplementary Material for this article can be found online at: https://www.frontiersin.org/articles/10.3389/fmicb.2021.629793/full#supplementary-material
Supplementary Figure 1 | Differential multiple histogram. Top 20 differential metabolites of S1 vs. S22 (A), S1 vs. S28 (B), S1 vs. S49 (C), R1 vs. R22 (D), R1 vs. R28 (E), and R1 vs. R49 (F). 1, Control; 22, Ri + Pf + Th; 28, Fo; 49, Ri + Pf + Th + Fo.
Supplementary Figure 2 | Volcanic map of differential metabolites. Differential metabolite volcanic maps of S1 vs. S22 (A), S1 vs. S28 (B), S1 vs. S49 (C), R1 vs. R22 (D), R1 vs. R28 (E), and R1 vs. R49 (F). 1, Control; 22, Ri + Pf + Th; 28, Fo; 49, Ri + Pf + Th + Fo.
Supplementary Figure 3 | The most enriched KEGG pathway of differential metabolites, as analyzed via pairwise comparisons between different treatments. Different metabolites KEGG enrichment maps of S1 vs. S22 (A), S1 vs. S28 (B), S1 vs. S49 (C), R1 vs. R22 (D), R1 vs. R28 (E), and R1 vs. R49 (F). 1, Control; 22, Ri + Pf + Th; 28, Fo; 49, Ri + Pf + Th + Fo.
Supplementary Table 1 | The list of treatments.
Supplementary Table 2 | Metabolites_full_table (NEG).
Supplementary Table 3 | Metabolites_full_table (POS).
References
Atalla, S. M. M., Abdel-Kader, M. M., El-Gamal, N. G., and El-Mougy, N. S. (2020). Using maize wastes, fermented by co-cultures of Trichoderma harzianum and Pseudomonas fluorescens, as grain dressing against m maize diseases under field conditions. Egypt. J. Biol. Pest Control 30:37. doi: 10.1186/s41938-020-00236-x
Bashan, Y., Holguin, G., and Lifshitz, R. (1993). “Isolation and characterization of plant growth-promoting rhizobacteria,” in Methods in Plant Molecular Biology and Biotechnology, eds B. R. Glick and J. E. Thompson (Boca Raton, FL: CRC Press).
Biermann, B., and Linderman, R. G. (1981). Quantifying vercular-arbuscular mycorrhizas: a proposed method towards standardization. New Phytol. 87, 63–67. doi: 10.1111/j.1469-8137.1981.tb01690.x
Cao, H., Li, X., Wang, X., Bai, H., Mu, W., and Liu, F. (2018). Control efficacy of pyraclostrobin and triazole fungicides against tomato crown and root rot. Sci. Agric. Sin. 51, 4065–4075. doi: 10.3864/j.issn.0578-1752.2018.21.006
Colak, A., and Bicici, M. (2013). Integrated disease management of Fusarium crown and root rot of greenhouse-grown tomato in eastern Mediterranean region of turkey. Tarim Bilim. Derg. 19, 89–100.
Dearth, S. P., Castro, H. F., Venice, F., Tague, E. D., Novero, M., Bonfante, P., et al. (2018). Metabolome changes are induced in the arbuscular mycorrhizal fungus Gigaspora margarita by germination and by its bacterial endosymbiont. Mycorrhiza 28, 421–433. doi: 10.1007/s00572-018-0838-8
Fang, X., He, Y., Xi, X., Zha, Q., Zhang, L., and Jiang, A. (2019). Multi-omics reveals the resistance mechanism of grape leaves in response to Botrytis cinerea. J. Zhejiang Univ. (Agric. Life Sci.) 45, 306–316. doi: 10.3785/j.issn.1008-9209.2018.11.121
Hibar, K., Daami-Remadi, M., Ayed, F., and Mahjoub, M. E. (2007). Fusarium crown and root rot of tomato and its chemical control. Int. J. Agric. Res. 2, 687–695. doi: 10.3923/ijar.2007.687.695
Hibar, K., Daami-Remadi, M., Hamada, W., and El-Mahjoub, M. (2006). Bio-fungicides as an alternative for tomato Fusarium crown and root rot control. Tunis. J. Plant Prot. 1, 19–29.
Hill, E. M., Robinson, L. A., Abdul-Sada, A., Vanbergen, A. J., Hodge, A., and Hartley, S. E. (2018). Arbuscular mycorrhizal fungi and plant chemical defence: effects of colonisation on aboveground and belowground metabolomes. J. Chem. Ecol. 44, 198–208. doi: 10.1007/s10886-017-0921-1
Kabdwal, B. C., Sharma, R., Tewari, R., Tewari, A. K., Singh, R. P., and Dandona, J. K. (2019). Field efficacy of different combinations of Trichoderma harzianum, Pseudomonas fluorescens, and arbuscular mycorrhiza fungus against the major diseases of tomato in Uttarakhand (India). Egypt. J. Biol. Pest Control 29:1. doi: 10.1186/s41938-018-0103-7
Kucharek, T., Jones, J. P., Hopkins, D., and Strandberg, I. (2000). Some disease of vegetables and agronomic crops caused by Fusarium in Florida. Circular 1025, Florida Cooperative Extension Service. Gainesville, FL: Institute of Food and Agricultural Sciences, University of Florida.
Kumar, V., Anal, A. K. D., and Nath, V. (2018). Growth response of litchi to arbuscular mycorrhizal co-inoculation with Trichoderma viride, Azotobacter chroococcum and Bacillus megatarium. Indian Phytopath. 71, 65–74. doi: 10.1007/s42360-018-0010-6
Li, J., Sun, Y., Zhao, T., Jiang, J., and Xu, X. (2018). Separation identification and biological characteristics of pathogen causing Fusarium crown and root rot of tomato. J. Northeast Agric. Univ. 49, 22–30.
Liu, B., Wang, Y., Hu, J., Li, J., and Yang, H. (2019). Biocontrol effect evaluation of combined of Trichoderma harzianum and Bacillus cereus against root-knot nematode Meloidogyne ssp. on tomatoes. Shandong Sci. 32, 71–75. doi: 10.3976/j.issn.1002-4026.2019.05.008
Liu, D., Li, M., Sun, W., and Liu, R. (2016). Selection of combinations of arbuscular mycorrhizal fungi and plant growth-promoting rhizobacteria against cucumber Fusarium wilt disease. Acta Phytopathol. Sin. 46, 821–832. doi: 10.13926/j.cnki.apps.2016.06.012
Liu, D., Li, M., Sun, W., and Liu, R. (2017). Mechanism of increasing resistance of cucumber plants to Fusarium wilt disease by combined inoculation with arbuscular mycorrhizal fungi and plant growth-promo- ting rhizobacteria. Acta Phytopathol. Sin. 47, 832–841.
Liu, R., and Luo, X. (1994). A new method to quantify the inoculum potential of arbuscular mycorrhizal fungi. New Phytol. 128, 89–92. doi: 10.1111/j.1469-8137.1994.tb03990.x
Lu, C. C., Guo, N., Yang, C., Sun, H. B., and Cai, B. Y. (2020). Transcriptome and metabolite profiling reveals the effects of Funneliformis mosseae on the roots of continuously cropped soybeans. BMC Plant Biol. 20:479. doi: 10.1186/s12870-020-02647-2
Mendes, J. B. S., da Costa Neton, V. P., de Sousa, C. D. A., de Carvalho Filho, M. R., Rodrigues, A. C., and Bonifacio, A. (2020). Trichoderma and bradyrhizobia act synergistically and enhance the growth rate, biomass and photosynthetic pigments of cowpea (Vigna unguiculata) grown in controlled conditions. Symbiosis 80, 133–143. doi: 10.1007/s13199-019-00662-y
Mikiciuk, G., Sas-Paszt, L., Mikiciuk, M., Derkowska, E., Trzciński, P., Głuszek, S., et al. (2019). Mycorrhizal frequency, physiological parameters, and yield of strawberry plants inoculated with endomycorrhizal fungi and rhizosphere bacteria. Mycorrhiza 29, 489–501. doi: 10.1007/s00572-019-00905-2
Murugesan, S., Vijayakumar, R., and Panneerselvam, A. (2011). Antifungal activity of medicinal plants against plant pathogenic fungus Fusarium oxysporum. J. Pharm. Res. 4, 843–844.
Nanjundappa, A., Bagyaraj, D. J., Saxena, A. K., Kumar, M., and Chakdar, H. (2019). Interaction between arbuscular mycorrhizal fungi and Bacillus spp. in soil enhancing growth of crop plants. Fungal Biol. Biotechnol. 6:23. doi: 10.1186/s40694-019-0086-5
Pan, X., Chang, R., Mu, K., and Chen, Q. (2020). Inhibition effects of Trichoderma harzianum VT9-3r and Bacillus subtilis VT4-1x on three potato pathogens. J. China Agric. Univ. 25, 72–81.
Rana, K. L., Kour, D., Kaur, T., Devi, R., Yadav, A. N., and Yadav, N. (2020). Endophytic microbes: biodiversity, plant growth-promoting mechanisms and potential applications for agricultural sustainability. Antonie van Leeuwenhoek 113, 1075–1107. doi: 10.1007/s10482-020-01429-y
Tan, S., Sun, W., and Liu, R. (2015). Combination of Glomus spp. and Bacillus sp. M3-4 promotes plant resistance to bacterial wilt in potato. Acta Phytopathol. Sin. 45, 661–669.
Thorpe, H. J., and Jarvis, W. R. (1981). Grafted tomatoes escape Fusarium foot and root rot. Can. J. Plant Sci. 61, 1027–1028. doi: 10.4141/cjps81-157
Tian, P., Li, X., He, W., Duan, D., Xu, S., and Yang, T. (2019). Metabonomics analysis of resistant susceptible tobacco varieties before and after infection by Meloidogyne incognita. Acta Tabacaria Sin. 25, 81–92. doi: 10.16472/j.chinatobacco.2018.261
Wang, J., Ren, P., Zhang, F., Hong, B., Chang, Q., Liu, C., et al. (2019). “Isolation and identification of pathogen of tomato Fusarium crown and root rot in greenhouse,” in Proceedings of the 2019 Annual Meeting of the Society for Plant Protection China Society of Plant Protection, 81–86.
Wang, X., Chen, M., Yang, G., Li, X., Li, P., and Chen, M. (2014). Effect of Glomus versiforme and Trichodema harzianum on growth and quality of Salvia miltiorrhiza. China J. Chin. Mater. Med. 39, 1574–1578. doi: 10.4268/cjcmm20140906
Wang, X. K. (2006). Principles and Techniques of Plant Physiological Biochemical Experimental. Beijing: Higher Education Press.
Yang, C. X., Zhao, W. N., Wang, Y. N., Zhang, L., Huang, W. C., and Lin, J. X. (2020). Metabolomics analysis reveals the alkali tolerance mechanism in Puccinellia tenuiflora Plants inoculated with arbuscular mycorrhizal fungi. Microorganisms 8:327. doi: 10.3390/microorganisms8030327
Zhang, F., Zhu, Z., Wang, B., and Wang, P. (2013). Optimization of Trichoderma harzianum T-E5 biomass and determining the degradation sequence of biopolymers by FTIR in solid-state fermentation. Ind. Crops Prod. 49, 619–627. doi: 10.1016/j.indcrop.2013.05.037
Keywords: arbuscular mycorrhizal fungi, plant growth-promoting rhizobacteria, Trichoderma spp., combined microbe agents, tomato, Fusarium crown and root rot
Citation: Cai X, Zhao H, Liang C, Li M and Liu R (2021) Effects and Mechanisms of Symbiotic Microbial Combination Agents to Control Tomato Fusarium Crown and Root Rot Disease. Front. Microbiol. 12:629793. doi: 10.3389/fmicb.2021.629793
Received: 16 November 2020; Accepted: 20 May 2021;
Published: 17 June 2021.
Edited by:
Yong Wang, Guizhou University, ChinaReviewed by:
Zhipeng Hao, Research Center for Eco-Environmental Sciences Chinese Academy of Sciences (CAS), ChinaWei Guo, Chinese Academy of Agricultural Sciences (CAAS), China
Copyright © 2021 Cai, Zhao, Liang, Li and Liu. This is an open-access article distributed under the terms of the Creative Commons Attribution License (CC BY). The use, distribution or reproduction in other forums is permitted, provided the original author(s) and the copyright owner(s) are credited and that the original publication in this journal is cited, in accordance with accepted academic practice. No use, distribution or reproduction is permitted which does not comply with these terms.
*Correspondence: Min Li, bWlubGlAcWF1LmVkdS5jbg==