- 1Laboratory of Environmental Health, Laboratory Sciences and Services Division, International Centre for Diarrhoeal Disease Research, Bangladesh, Dhaka, Bangladesh
- 2Department of Microbiology, Jahangirnagar University, Dhaka, Bangladesh
Vibrio parahaemolyticus is a major foodborne pathogen responsible for significant economic losses in aquaculture and a threat to human health. Here, we explored the incidence, virulence potential, and diversity of V. parahaemolyticus isolates from aquaculture farms in Bangladesh. We examined a total of 216 water, sediment, Oreochromis niloticus (tilapia), Labeo rohita (rui), and Penaeus monodon (shrimp) samples from the aquaculture system where 60.2% (130/216) samples were positive for V. parahaemolyticus. Furthermore, we identified 323 V. parahaemolyticus strains from contaminated samples, 17 of which were found positive for trh, a virulence gene. Four isolates out of the 17 obtained were able to accumulate fluid in the rabbit ileal loop assay. The correlation between the contamination of V. parahaemolyticus and environmental factors was determined by Pearson correlation. The temperature and salinity were significantly correlated (positive) with the incidence of V. parahaemolyticus. Most of the pathogenic isolates (94.1%) were found resistant to ampicillin and amoxicillin. O8: KUT was the predominant serotype of the potentially pathogenic isolates. ERIC-PCR reveals genetic variation and relatedness among the pathogenic isolates. Therefore, this region-specific study establishes the incidence of potential infection with V. parahaemolyticus from the consumption of tilapia, rui, and shrimp raised in farms in Satkhira, Bangladesh, and the basis for developing strategies to reduce the risk for diseases and economic burden.
Introduction
The Gram-negative, facultative anaerobic bacterium Vibrio parahaemolyticus is usually present in tropical and temperate coastal waters, as well as in shrimp aquaculture (Heredia et al., 2009). It is indigenous to the estuarine ecosystem and is associated with human gastrointestinal disease, wound infections, and septicemia (Joseph et al., 1982; Wong et al., 2000). V. parahaemolyticus has been considered an etiological agent of foodborne-related illness throughout the world from the very beginning of its discovery (Yeung and Boor, 2004). Consumption of raw, parboiled, or contaminated seafood, including shrimp, prawn, fish, and shellfish, is the most common reason for human gastrointestinal infections (Yeung and Boor, 2004; Mahmud et al., 2007; Su and Liu, 2007; Sala et al., 2009; Khouadja et al., 2013; Khimmakthong and Sukkarun, 2017). A growing number of V. parahaemolyticus transmissions and outbreaks engendered by strain members of a pandemic clonal complex have been witnessed all through the world from the early 90s (DePaola et al., 2000; Chowdhury et al., 2004; Ansaruzzaman et al., 2005; González-Escalona et al., 2005; Martinez-Urtaza et al., 2005; Quilici et al., 2005; Fuenzalida et al., 2006).
Vibrio-related infections caused by V. parahaemolyticus, which eventually turn into an epidemic, have been observed in Asia, Europe, the United States, Peru, and Chile in the past few decades. These outbreaks were linked with rare, gradual seawater temperature rises along the shoreline (Velazquez-Roman et al., 2014). Several studies performed worldwide implicate environmental factors such as turbidity, temperature, water salinity, amounts of organic matter, and suspended chlorophyll, among others, in the distribution of V. parahaemolyticus and the abundance of the species (López-Hernández et al., 2015). Although the mechanism of V. parahaemolyticus infection in humans is not well known yet, two hemolysins are broadly accepted as pathogenicity indicators, which are the thermostable direct hemolysin (TDH) and the tdh-related hemolysin (TRH) (West et al., 2013; Jones et al., 2014; López-Hernández et al., 2015). tdh is a protein that helps in pore formation, which has been implicated in the invasion of bacteria, and trh plays a major role in virulence. Many V. parahaemolyticus clinical isolates have trh and/or tdh; however, a comparatively limited quantity of environmental isolates harbor such genes (López-Hernández et al., 2015; Leoni et al., 2016).
There are 71 K antigens and 13 O antigens that have been recognized and considered related to gastroenteritis by the Serological Typing Committee of V. parahaemolyticus (Ishibashi et al., 2000). Typically, in the outbreaks, a wide number of serovars (O3:K6, O4:K68, O1:K25, O1:KUT, O1:K26 etc.) are present (Velazquez-Roman et al., 2014). Post-1996, the growing occurrence of gastroenteritis was associated with the pandemic serotype O3:K6 in most of the world (Okuda et al., 1997; Bag et al., 1999). In recent years, the correlation of this serotype O3:K6 with most of the V. parahaemolyticus infections in the United States, South Korea, Thailand, Japan, Laos, Taiwan, and India indicates that this species might have had an uncommon food-borne trait and possess the pandemic ability (Bag et al., 1999). Several molecular typing techniques such as random amplified polymorphic DNA (RAPD), ribotyping, pulsed-field gel electrophoresis (PFGE), and multi-locus sequence typing (MLST) (Bag et al., 1999; Marshall et al., 1999; Wong et al., 1999; González-Escalona et al., 2008; Yang et al., 2008) have been valuable for illustrating the genetic diversity at the strain level (Olive and Bean, 1999). For the subtyping of the V. parahaemolyticus species containing strongly conserved repetitive intergenic consensus sequences, ERIC-PCR has been shown to be efficient (De Bruijn, 1992; Chen et al., 2012).
Shrimp export is positioned as one of the leading earning sectors in Bangladesh. Over 40,000 tons of P. monodon and M. rosenbergii shrimps were exported in 2016 and 2017 along with smaller volume of other species. More than 80% of the shrimp production was shipped to the European Union market, mainly Germany, Netherlands, and Belgium, in 2017. According to the estimation of the fish industry, 5.02 million metric tons of fish production will be achieved within 2020–21. However, the number of detention and rejection cases due to the detection of V. parahaemolyticus from Asia is increasing (Sujeewa et al., 2009).
In multiple ecological studies, the relationship of temperature and salinity with V. parahaemolyticus has been reported, but the extension of this relationship depends on the region and season (Takemura et al., 2014). Similarly, nutrients, pH, and turbidity were incoherent and depended on the region and the variability of these factors. Therefore, it is necessary to conduct region- and area-specific studies to provide a detailed description of the effect of environmental parameters on V. parahaemolyticus concentration (Froelich and Noble, 2016).
Consumption of undercooked and raw seafood is the primary way of V. parahaemolyticus exposure and infection. Fishes and shrimps can accumulate the bacterium from the aquaculture prior to their harvesting process. As the incidence of Vibrio spp. in the environment is unavoidable, their increasing presence in coastal water and sediment create public health issues for the aquaculture industry and the consumers. Therefore, substantial interest has been grown in understanding the biotic and abiotic factors of V. parahaemolyticus. Such data has value for estimating the properties and abundance of the species in aquaculture.
Standing on the abovementioned basic contextual information, we conducted a yearlong field-based study to correlate the abundance of non-pathogenic and pathogenic V. parahaemolyticus with seasons and other factors such as temperature, pH, and salinity. In this study, pathogenic strains were further characterized to determine their virulence potential in a rabbit model.
Materials and Methods
Sample Types, Sources, and Study Sites
Water, sediment, and three different types of fishes (Penaeus monodon—shrimp, Oreochromis niloticus—tilapia, and Labeo rohita—rui) were collected from three sampling sites located in Satkhira Sadar Upazila of Satkhira district (Figure 1), one of the major shrimp-harvesting areas of Bangladesh. The sampling was conducted from the period of May 2017–April 2018, once a month. The sampling sites were (1) low-saline aquaculture: shallow water (22° 38’ 44.9” N 89° 04’ 47.9” E) (2) brackish aquaculture: enclosed river water (22°39’28.9”N 89°04’30.6”E), and (3) brackish water: flowing river water (22°39’45.9”N 89°04’33.4”E). Water and sediment were taken from all three sites, whereas Tilapia was collected from sites (1) and (2), and rui fish and shrimp were collected only from site (1) and site (2), respectively.
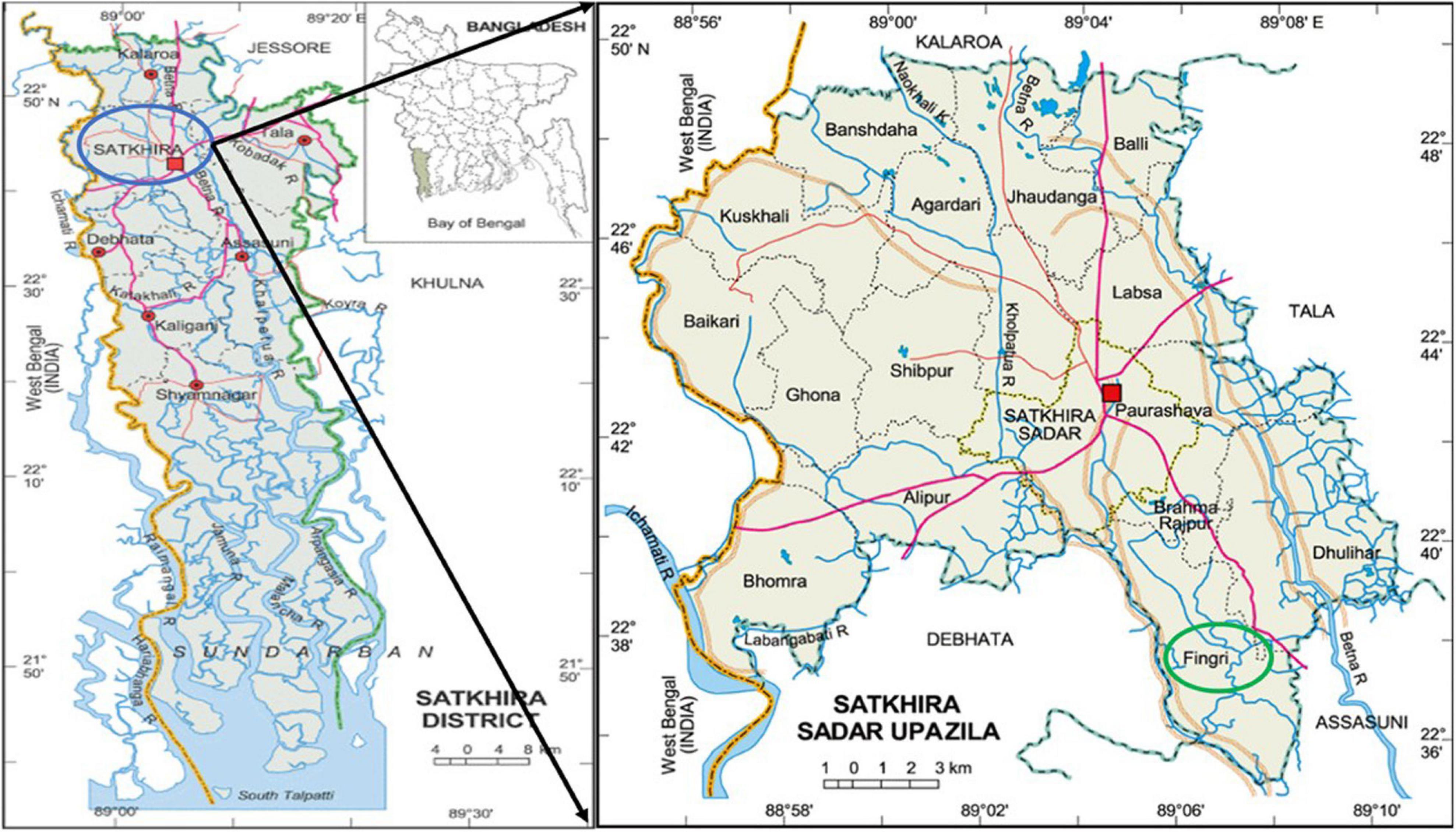
Figure 1. Location of the sample collection sites of the coastal area of Satkhira, Bangladesh. Image courtesy: Banglapedia.
Sample Collection
All the samples were collected in sterile bottles (Nalgene, United States) of 500 ml and 250 ml and a sterile easy-to-close zipper bag (FisherbrandTM Whirl-PakTM, United States). Water and sediment samples were taken from three different spots of each site, and three pieces of each category of fishes were picked up in every sampling time to cover the maximum areas of the site according to the American Public Health Association (APHA) (Federation and American Public Health Association, 2005). After collection, samples were shipped to the Laboratory of Environmental Health (LEH) at the International Centre for Diarrhoeal Disease Research, Bangladesh (icddr,b), in an insulated sample-carrying box maintaining the temperature ranging from 4 to 10°C. Then, the samples were processed within 12 h of collection according to the APHA guideline.
Measurement of Physicochemical Parameters of Water Samples
Salinity and temperature were measured using a conductivity meter (Sens-Ion 5, HACH, United States), and pH of the water samples was measured with a pH meter (Sens-Ion+ pH1, HACH, United States) in each site following the manufacturer’s instructions.
Sample Processing
Inoculation of water and sediment samples on Thiosulfate Citrate Bile Salt Sucrose (TCBS) (DIFCO, BD, United States) agar was done by the spread plate method. Fish samples were washed with sterile 3% NaCl solution to remove surface microorganisms, debris, sand, and slime. Then, the flesh, gut, and gill of the tilapia and rui samples and the flesh and chitin of the shrimp samples were separated aseptically. After that, 5 g of each separated part was homogenized in 45 ml of 3% NaCl solution separately in autoclaved 4 Oz bottles. Then, fish homogenates, water, and sediment samples were enriched in alkaline peptone water (APW) for 18 h. The enrichment broths were then subcultured onto TCBS agar plates and incubated at 37°C overnight. Later on, 4–6 presumptive green or blue-green colonies of 2–3 mm in diameter from each sample were transferred to fresh TCBS agar plates and Chromogenic Vibrio Agar (CVA) (CHROMagar, Paris, France) plates (Hara-Kudo et al., 2001). Isolates showed green to blue green colonies in TCBS, and violet colonies in CVA plates were selected as V. parahaemolyticus. Subsequently, the selected strains were inoculated onto Gelatin Agar (GA) plates to see the gelatinase activity for further confirmation. The isolates were preserved at −80°C in LB broth with 30% glycerol in the icddr,b central bio-repository system and in T1N1 soft agar media at room temperature (Alam et al., 2009). For each experiment, the isolates were subcultured on GA plates from the stocks and kept at 37°C for overnight incubation.
Polymerase Chain Reaction (PCR) Assay
Extraction of chromosomal DNA was done using the boil DNA extraction method according to the procedure reported by Mahmud et al. (2020). Presumptively identified isolates were further confirmed by simplex PCR for the tlh gene, a species-specific gene for V. parahaemolyticus (Taniguchi et al., 1986; Bej et al., 1999; McCarthy et al., 1999; Jones et al., 2012), and the presence of 12 virulent genes was also examined. The presence of all these genes was tested by performing simplex PCR. PCR assays were carried out to detect tdh and trh genes described by Bej et al. (1999); the GS, PGS, orf-8, and HU-α genes described by Li et al. (2016); and the ure, Mtase, VPA 1339 (escC, vscC2), VPA 1346 (yopP, vopP, vopA/P), VPA1321 (vopC), and VPA 1376 genes reported in Caburlotto et al. (2009). All the primers’ sequence and band size are listed in Table 1. The PCR-amplified products were separated in an agarose gel of 1.5% stained in MIDORI green advance and visualized under UV light with a GelDoc Go imaging system (Bio-Rad, United States).
Species Confirmation by VITEK2
All 17 tlh and trh gene-positive V. parahaemolyticus isolates were further confirmed by the VITEK 2 system (bioMérieux, Marcy I’Etoile, France) using a VITEK 2 GN ID card. V. parahaemolyticus (ATCC BAA-238) was used as a positive control for the identification in this system. For VITEK 2 assays, pure isolates were streaked on GA plates and incubated at 37°C overnight. 1–3 isolated colonies were selected from each GA plate and suspended in saline for preparation of inoculum to obtain an absorbance of ∼0.5 McFarland Units before being subjected to VITEK 2 analysis.
Antimicrobial Susceptibility Testing (AST) and Calculation of Multiple Antibiotic Resistance (MAR) Indexes
By following the recommendation of the Clinical and Laboratory Standards Institute (CLSI) guidelines (Patel et al., 2015), the pattern of antibiotic susceptibility for 17 trh-positive isolates was obtained by disk diffusion technique. A bacterial suspension with turbidity comparable to 0.5 McFarland standard was made from bacterial inocula taken from overnight cultured Gelatin Agar (GA) plate and swabbed onto the Mueller-Hinton Agar (MHA) (Difco Detroit, MI, United States). At 37°C, the plates were then kept for overnight incubation aerobically. Total 15 antibiotic discs (Oxoid, United States) including amoxicillin (AML; 10 μg), ampicillin (AMP; 10 μg), cefotaxime (CTX; 30 μg), ceftriaxone (CRO; 30 μg), cefixime (CFM; 5 μg), cefoxitin (FOX; 30 μg), ciprofloxacin (CIP; 5 μg), gentamicin (CN; 10 μg), nalidixic acid (NA; 30 μg), streptomycin (S; 10 μg), tetracycline (TE; 30 μg), trimethoprim/sulfamethoxazole (SXT; 25 μg), piperacillin-tazobactam (TZP; 110 μg), imipenem (IPM; 10 μg), and meropenem (MEM; 10 μg) were used. The inhibition zone surrounding each disk was determined in millimeter (mm) and documented. As per CLSI guidelines, each bacterial species was categorized as resistant (R), intermediate (I), or susceptible (S) (Clinical and Laboratory Standards Institute [CLSI], 2017). The Escherichia coli ATCC 25922 strain was used as a negative control. Calculation of the MAR index was made using formula x/y, where x is the antimicrobial number to which an isolate became resistant and y indicates the antimicrobial number to which the isolate got exposed (Krumperman, 1983).
Serotyping
Serotyping of V. parahaemolyticus toxigenic strains was performed utilizing commercially available V. parahaemolyticus antisera test kit (Denka Seiken, Tokyo, Japan), as defined by Shinoda et al. (1983). The strains were first grown on 3% NaCl-containing Luria-Bertani agar. After incubating for 24 h at 37°C, one loopful inoculum was taken and mixed with 1 ml of normal saline. After boiling for 2 h according to the manufacturers’ instruction, the cell suspension aliquots containing normal saline were utilized for serotyping on the basis of O antigen. The leftover cell suspension (not boiled) was used for K antigen-dependent serotyping.
ERIC-PCR and Genetic Fingerprinting Analysis
To determine the clonal relationship among the isolates based on Enterobacterial Repetitive Intergenic Consensus (ERIC) sequences PCR, two primer sequences ERIC1 (5’-3’ATGTAAGCTCCTGGGGATTCAC) and ERIC2 (5′–3′ AAGTAAGTGACTGG GGTGAGCG) (Versalovic et al., 1991) were used. The conducted amplification conditions were reported by Rivera et al. (1995). Separation of 20 μl PCR product on a 2% agarose gel was performed at 54 volts for 120 min. 1 kb Plus ladders from Invitrogen (Thermo Fisher Scientific, United States) were used as DNA marker. The gel imaging analysis was performed by BioNumerics software (version 4.5) (Applied Maths, Belgium) using the dice coefficient and unweighted-pair group method where average linkages were used to create dendrogram with 1.0% tolerance values.
Rabbit Ileal Loop Study
The rabbit ileal loop assay was performed to examine the induction of fluid accumulation as previously described (Twedt et al., 1980), with minor modifications. The experiment was carried out in duplicate with 1.8–2.2 kg adult New Zealand white rabbits. The pathogenic strains of V. parahaemolyticus (108 CFU/ml) were injected into the ligated ileal loops of rabbits, which was followed by measurement of the fluid accumulation in each loop at 18 h after injection. The fluid accumulation (FA) ratio was calculated by dividing fluid accumulation (in ml) in each loop by the length (in cm) of the loop. Phosphate-buffered saline (PBS) was used as a negative control, and V. cholerae O1 was used as a positive control in this study. Animal Experimental Ethics Committee of International Centre for Diarrhoeal Disease Research, Bangladesh has approved this rabbit ileal loop study (permit number PR-20114).
Histopathology
Histopathological examination of the intestinal tissue of the rabbits was also performed to observe the histopathological features of the intestinal wall. Each ligated intestinal loop was washed in phosphate-buffered saline (PBS), and appropriately 2 cm in length was sectioned and fixed in 10% formalin for histopathological examination under a light microscope. Tissues were dehydrated in a series of graded alcohols, further processed, embedded in paraffin, and mounted into paraffin blocks. Staining of the 4 μm tissue sections was performed with hematoxylin and eosin (H&E). The protocols for H&E staining were as described by Bancroft and Gamble (2008).
Statistical Analysis
Pearson correlation analysis was done to observe the relationship between V. parahaemolyticus contamination and physicochemical parameters. On the other hand, logistic regression analysis was performed to see the relationship between trh-positive V. parahaemolyticus and physicochemical parameters. The odds ratio was calculated from the coefficients of the model to identify significant risk factors. The desired statistical significance level was set at 0.05. The analysis was performed by programming language R (R Core Team, 2013) and statistical software package STATA 13 (StataCorp, 2014).
Results
Variations Among the Sites and Samples
A total of 216 samples from 3 different sites [96 samples from site (1), 96 samples from site (2), and 24 samples from site (3)] were analyzed where 60.2% (130/216) samples were positive for V. parahaemolyticus, and 7.69% (10/130) of total contaminated samples contained trh gene. Later on, from 130 contaminated samples, 323 representative V. parahaemolyticus strains were obtained, of which 17 strains were found positive for the trh gene. The prevalence of V. parahaemolyticus in contaminated samples was highest in samples collected from site (2) at 81.25% (78/96), closely followed by site (3) at 79.16% (19/24). Site (1) presented the lowest incidence of V. parahaemolyticus at 34.38% (33/96). The prevalence of virulence factor, trh, was most prominent in site (3) defined at 12.5% (3/24) followed by site (1) with a 4.17% (4/96) incidence and site (2) at 3.13% (3/96). Among the samples, V. parahaemolyticus was most prevalent in sediment 72.2% (26/36) followed by shrimp 69.4% (25/36), tilapia 66.7% (48/72), water 61.1% (22/36), and rui 25% (9/36) (Table 2).
The surface water temperature at three sampling sites recorded from May 2017 to April 2018 was between 17.3 and 33.8°C. The median temperatures at site (1), site (2), and site (3) were 29, 29.25, and 29.5°C, respectively. The salinity and pH of surface water varied from 0.4 to 16.2 ppt and 7.32 to 8.69, respectively (Figure 2 and Supplementary Table S1). During the summer (March–June) and the rainy season (July–October), V. parahaemolyticus contamination in the samples was higher as compared to the winter seasons (November–February) in all the three sampling sites (Figure 3). In the case of Pearson correlation analysis, a correlation coefficient of 0.41 for salinity indicates a significant (p-value < 0.05) positive relation with V. parahaemolyticus contamination, and similarly, the correlation coefficient of 0.45 for temperature gives a significant (p-value < 0.05) positive relation with V. parahaemolyticus contamination (Table 3). On the other hand, in the case of logistic regression, we observed a significant negative relation between salinity and trh positive V. parahaemolyticus (odds ratio 0.83, p-value < 0.05) (Table 4).
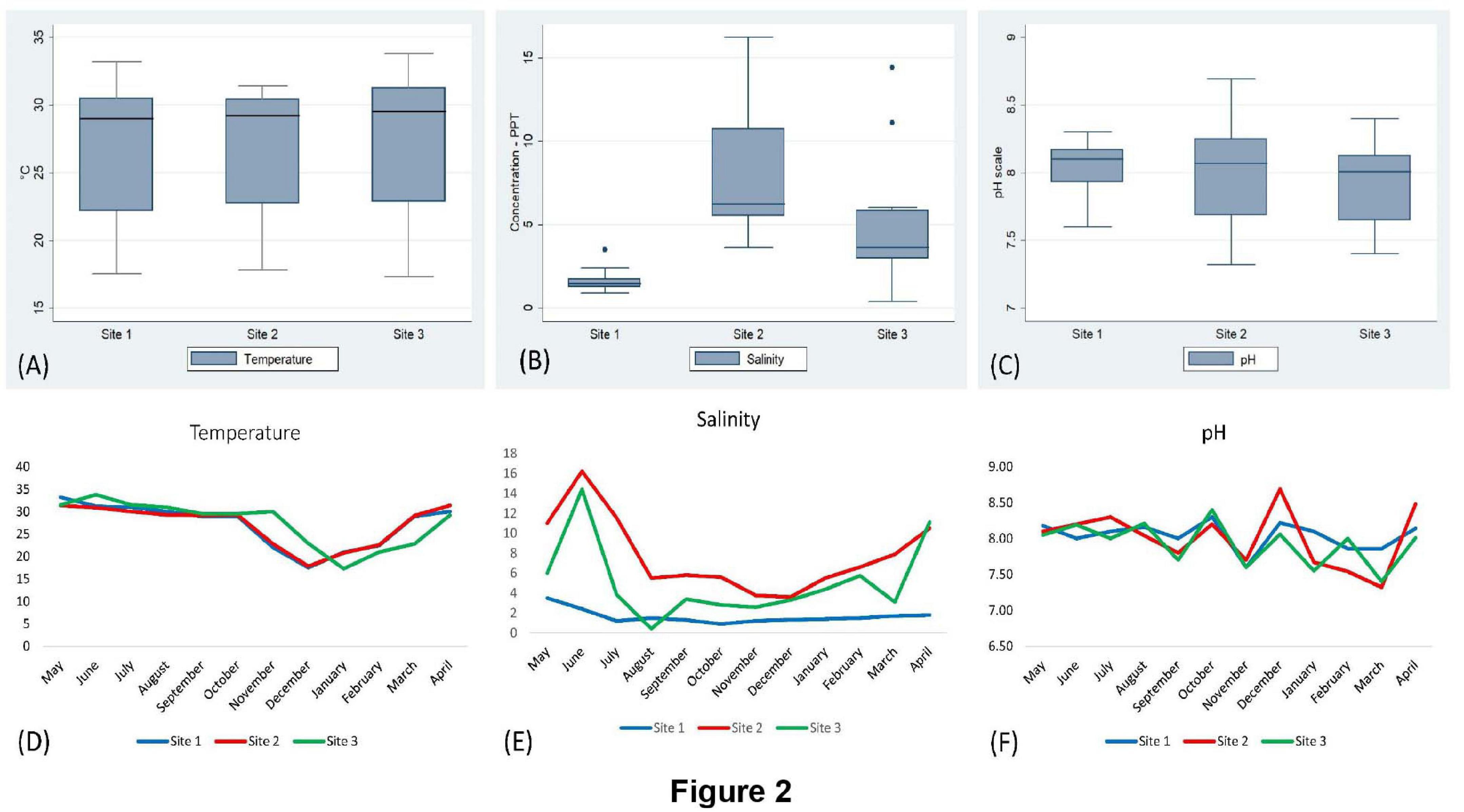
Figure 2. Analysis of physicochemical parameters (temperature, salinity, and pH). Box and whisker plot analysis of temperature (A), salinity (B), and pH (C) in sites (1), (2), and (3). Seasonal variation of temperature (D), salinity (E), and pH (F) in different sites.
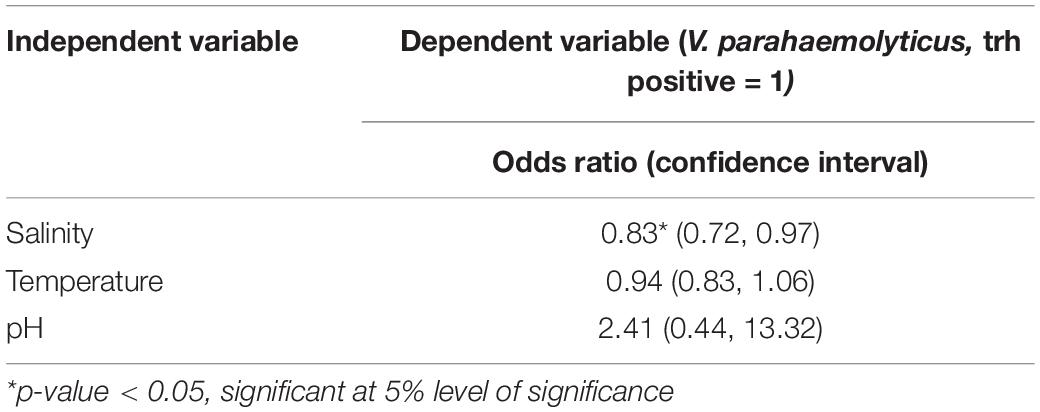
Table 4. Logistic regression analysis of trh-positive V. parahaemolyticus and physicochemical parameters.
Molecular and Phenotypic Confirmation of the Isolates
The presence of tlh (species-specific gene) confirmed the isolates of V. parahaemolyticus. The occurrence of 12 virulence and virulence-associated genes was detected by PCR analysis of the isolates. Most of the isolates were negative for the genes tested. However, 5.26% (17/323) isolates were positive for the trh gene, a major virulence gene for V. parahaemolyticus, considered as pathogenic. All the 17 tlh and trh gene-positive isolates were further confirmed as V. parahaemolyticus species by using the tlh species-specific primer (Supplementary Figure S1) and the VITEK 2 system. Among the trh-positive isolates, 6 (35%), 6 (35%), 4 (24%), and 1 (6%) were from sediment, rui, tilapia, and water samples, respectively (Figure 4). No trh-positive isolate was found from the shrimp sample.
Antibiogram
Antibiotic susceptibility test (AST) was performed on isolated pathogenic V. parahaemolyticus using 15 antibiotics selected from different groups (Supplementary Table S2). Referring to Table 5, V. parahaemolyticus isolates were the most resistant toward ampicillin and amoxicillin (94.1%), followed by cefotaxime (29.4%), and ceftriaxone (17.6%). Some of the antibiotics, such as gentamicin and streptomycin, had a slight resistance of about 11.8% and 5.9%, respectively. All other examined isolates were sensitive to imipenem, sulfamethoxazole-trimethoprim, nalidixic acid, tetracycline, and pipercillin-tezobactam. The next-highest susceptibility rates were found for streptomycin (94.1%), meropenem (88.2%), ciprofloxacin (88.2%), gentamicin (82.4%), cefoxitin (82.4%), ceftriaxone (70.6%), and cefixime (64.7%).
The MAR index was obtained by considering the relation between the number of antibiotics that an isolate is resistant and the total number of antibiotics used. The MAR index range was from 0.07 to 0.27 (Table 6). The maximum MAR index was obtained from two isolates (R06-106, R06-107) from the gut of rui fish, which showed resistance to four antibiotics.
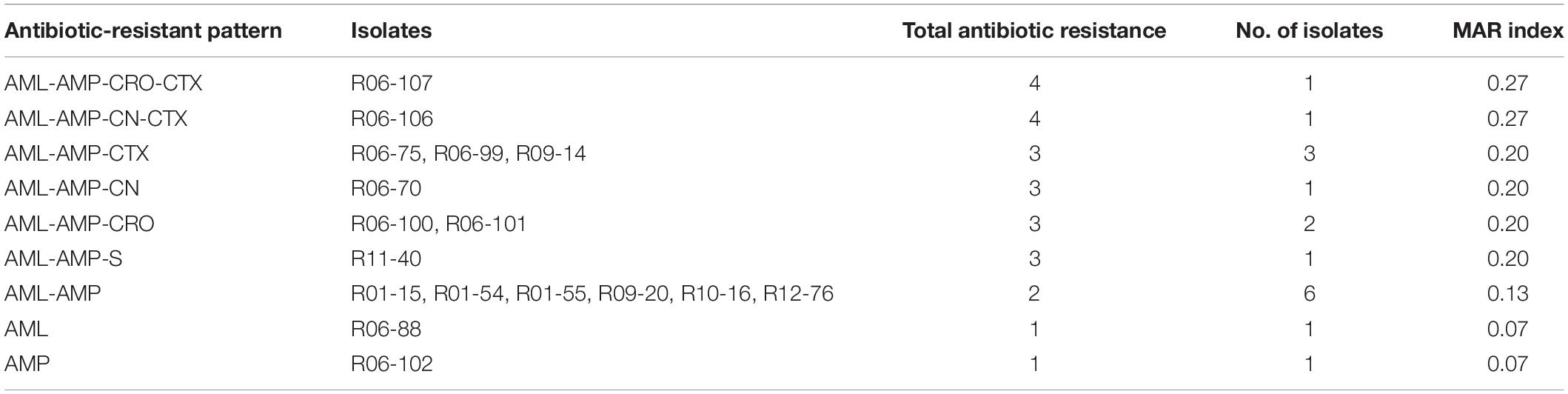
Table 6. Antibiograms and multiple antimicrobial resistance (MAR) indices of V. parahaemolyticus strains.
Serotyping Analysis
All the 17 pathogenic V. parahaemolyticus isolates were serotyped and categorized into 5 different O groups (O1, O3, O5, O8, and O11). Groups of O8 (47.1%), O5 (23.53%), O11 (11.76%), O3 (11.76%), and O1 (5.88%) were found in this study. Among the isolates, only one was determined by specific K-typing, whereas the rest of the isolates were K-typing untypable. O8: KUT (with eight isolates) was the most common serotype among them. Other O: K serogroups included four O5: KUT, two O11: KUT, one O3: KUT, O1: KUT, and O3: K20 each (Table 7).
ERIC-PCR
ERIC-PCR of the 17 pathogenic isolates resulted in 11–25 amplification bands with a molecular size ranging from 100 to 3,000 bp, where 420, 520, and 1,500 bp were common to most of the isolates. However, considering the similarity coefficient of 0.68, the isolates were divided into 7 clusters designated as A, B, C, D, E, F, and G. Most of the isolates were distributed between the C and D clusters. ERIC-PCR banding patterns showed that all the isolates were genetically diverse except one isolate pair (R06-70 and R06-75). Only one strain (R11-40) from sediment is clustered in group F. The reference strain ATCC BAA-238 was in cluster G alone (Figure 5).
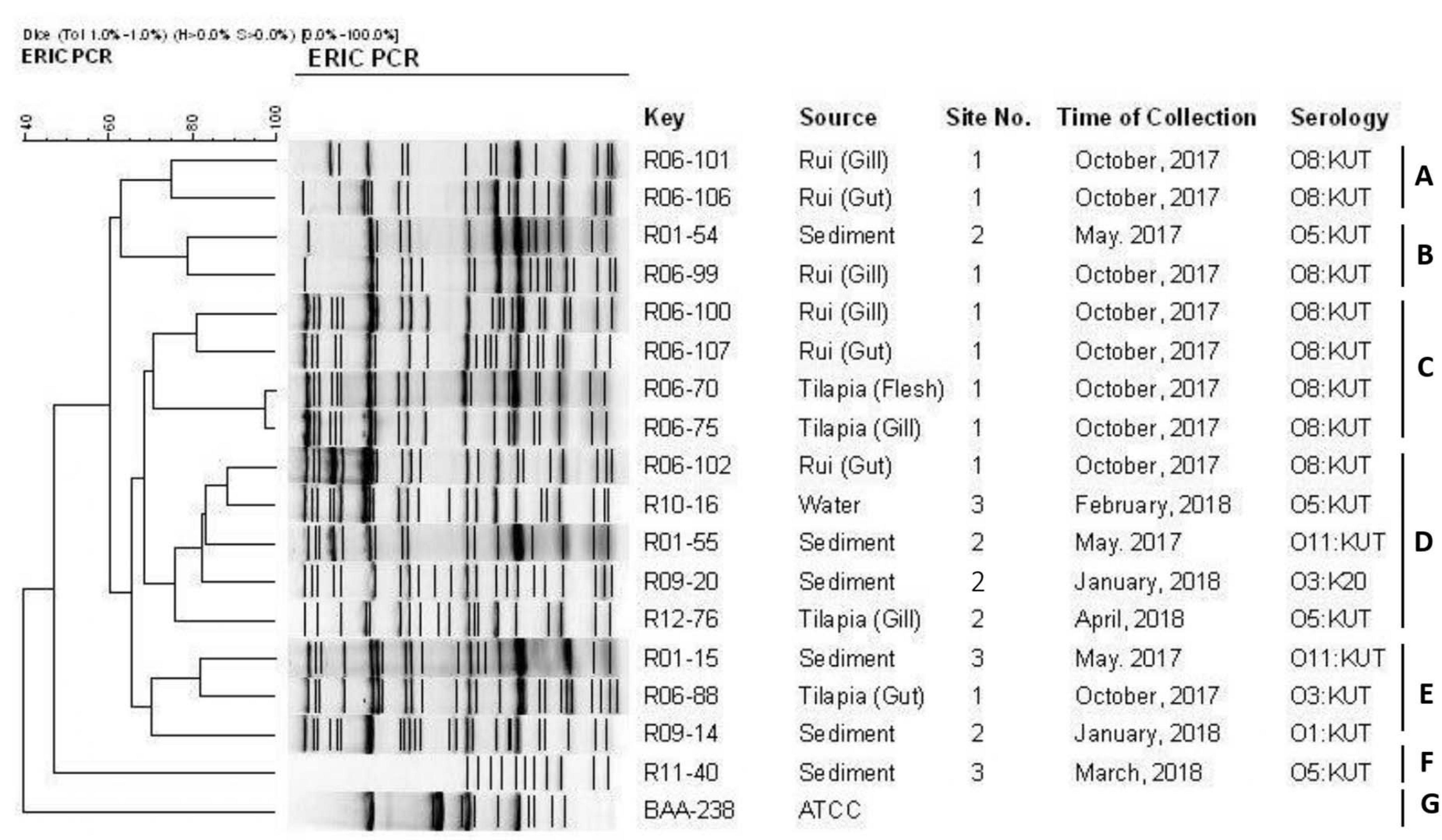
Figure 5. ERIC-PCR DNA fingerprint analysis of trh-positive V. parahaemolyticus isolates in different samples (fish, sediment, water) from Satkhira, Bangladesh.
Ileal Loop Study and Histopathology
Four out of 17 pathogenic V. parahaemolyticus showed fluid accumulation in the rabbit ileal loop. The fluid accumulation (FA) ratio was 2.0, 1.4, 1.6, and 2.2 ml/cm in strains R01-15, R06-75, R06-101, and R12-76, respectively (Figure 6). Among the four strains, R06-101 showed more serosal hemorrhage than the other three strains, characterized by gross bloody mucous in the accumulated fluid. Histopathological features of all four strains showed nearly similar findings, including a moderately degenerated, damaged, and sloughed ileal mucosa (Figure 7; Inside Square); significant/obvious obstruction of blood vessels and infiltration of polymorphonuclear neutrophils (PMN) in the lamina propria and at the crypt epithelium surface were observed at higher magnification (Figure 7; Inside Circle).
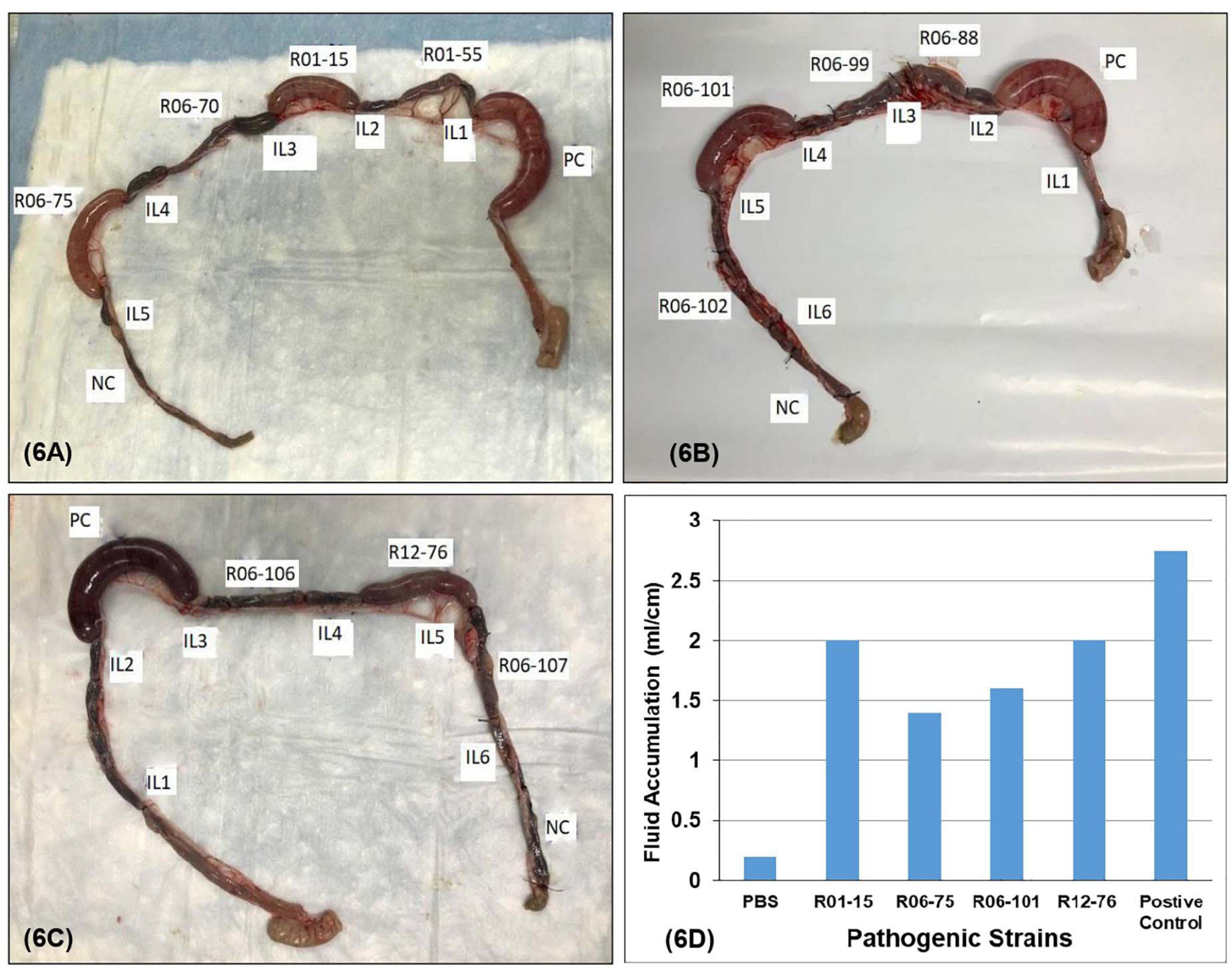
Figure 6. Rabbit ileal loop assay to assess enterotoxigenic activity. Pictorial view of rabbit ileal loop of different environmental strains. Analysis of fluid accumulation of different V. parahaemolyticus strain isolated from the environment (D). Rabbit ileal loops were inoculated with 108 CFU/ml of each trh-positive V. parahaemolyticus strain (A–C). Results are expressed as fluid accumulation (FA; in milliliters) per loop length (in centimeters). PBS and the V. cholerae O1 were taken as controls in this assay.
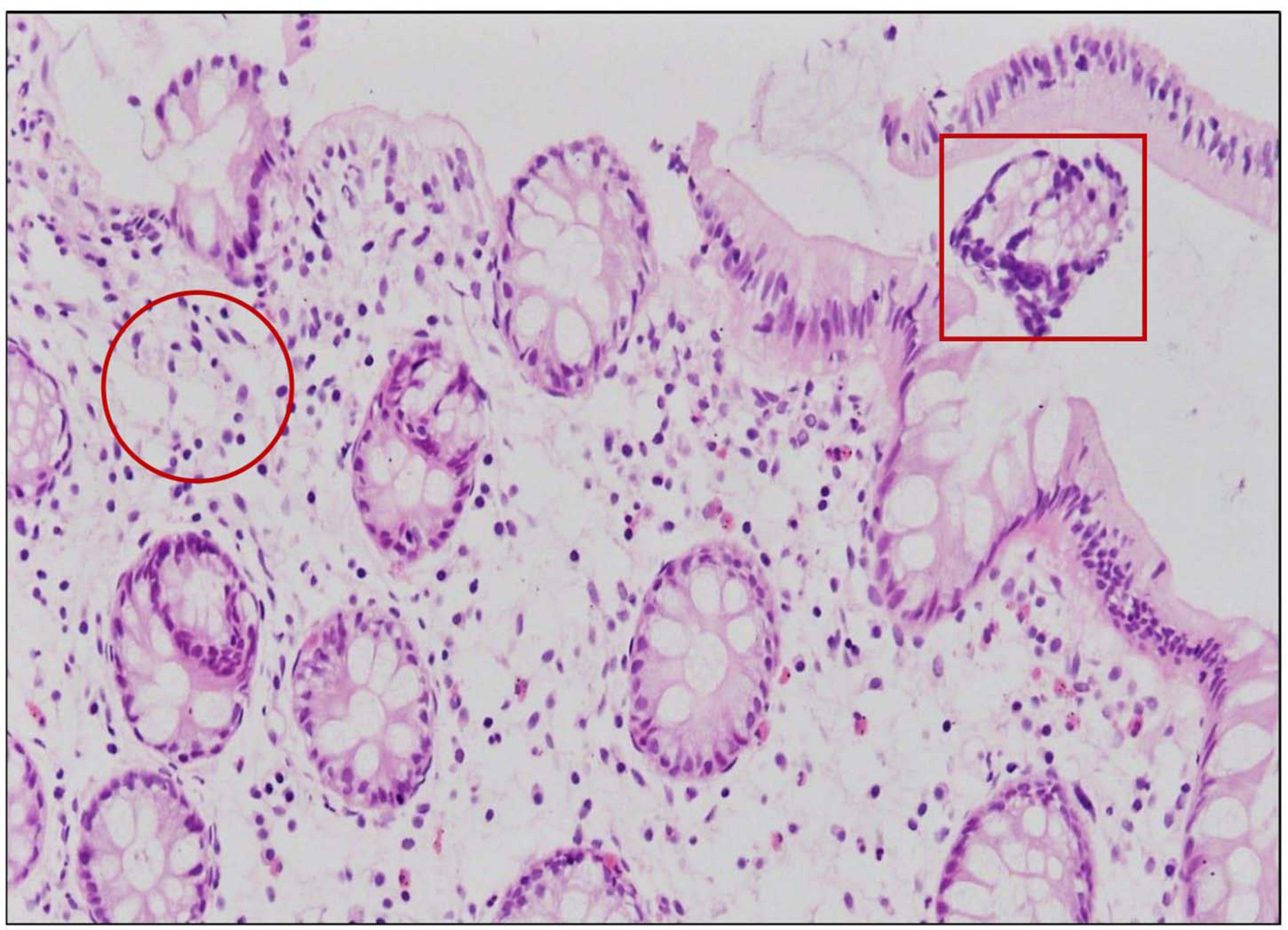
Figure 7. Ileal tissue sections obtained from rabbits infected with 108 CFU/ml of trh positive V. parahaemolyticus showed severe histopathological changes in the mucosa represented by infiltration with PMN in the lamina propria (inside circle) and degeneration, damage, and sloughing (inside square).
Discussion
The occurrence of V. parahaemolyticus has been reported previously in temperate and tropical coastal waters as well as in aquaculture (Heredia et al., 2009; Pui et al., 2014). The incidence of V. parahaemolyticus in shrimp, water, fish, and sediments was investigated all year round using cultural and molecular methods. In our study, V. parahaemolyticus was present year-round in site (2) and site (3), whereas it was absent in site (1) during the winter-period. Surprisingly, the presence of the pathogenic (trh) gene was more frequent during October from the rui fish sample than others. The seasonal and geographical distribution of V. parahaemolyticus has been considered to be significantly dependent on temperature in temperate zone shellfish-growing areas (Kaneko and Colwell, 1975; Kiiyukia et al., 1989; Mahmud et al., 2006).
Based on the year-long assessment of water sample salinity gradients, for which sample collection was done from the three study locations, a salinity-dependent area demarcation can then be made. During summer, maximum salinity was encountered in site (2) and site (3) due to an increased brackish water inflow, whereas in site (1), low salinity was present throughout the year as it was collected from underground shallow water, which is not affected by seawater. Contrariwise, minimum salinities in site (2) and site (3) were reported in the rainy season due to heavy rainfalls associated with the blending of floodwater from the nearby regions. At all three study locations, the temperature of water varied consistently, the highest temperature was reported in the summer, followed by the rainy season, and the lowest in the winter; the pH was somewhat unchanged (7.99 ± 0.31) within its intrinsic alkaline status. However, in the rainy season, the maximum alkalinity may be due to the floodwater inflow from the adjacent areas containing a significant amount of organic residues.
Statistically, the salt tolerance potential (at a salt concentration of 2–10%) can be due to a crucially significant positive correlation between salinity and V. parahaemolyticus (Table 3; Caburlotto et al., 2010; Johnson et al., 2010; Whitaker et al., 2010) but a significant negative association was found between salinity and the pathogenic V. parahaemolyticus, as most of these pathogenic isolates were found in the samples (tilapia, rui) of the site (1), which was quite a low-saline site.
In multiple previous studies, trh-positive V. parahaemolyticus were more frequent than tdh positive in the environmental samples (Bauer et al., 2006; Ellingsen et al., 2008). Although only 1–2% of environmental V. parahaemolyticus strains harbor tdh and/or trh gene, this small amount is sufficient to have a significant impact on public health in tropical developing countries (Cook et al., 2002). In our study, 5.26% (17/323) of the V. parahaemolyticus strains showed pathogenic potential, by the presence of trh gene, but all the isolates were negative for the tdh gene. This result appears similar to the previous study by Letchumanan et al. (2015) but contrary to the earlier reports where a high prevalence of these virulence factors was observed in V. parahaemolyticus strains in natural estuarine and coastal waters (Johnson et al., 2012; Akther et al., 2016).
In this study, V. parahaemolyticus isolates exhibited a high level of resistance to amoxicillin and ampicillin. Resistance to penicillin could probably be due to the extensive use of antibiotics in aquaculture and the effect of residual antibiotics in aquaculture systems. Therefore, the penicillin group is considered ineffective for the management of V. parahaemolyticus infections. However, most of the isolates were susceptible to the majority of the antibiotics used. Susceptibility patterns of V. parahaemolyticus isolates to different antibiotic groups such as quinolone, tetracycline, carbapenem, aminoglycosides, and cephalosporin were analogous with other studies reported in various sample sources (Lesmana et al., 2001; Han et al., 2007; Yano et al., 2011; Ottaviani et al., 2013). In the current study, the MAR index value was observed greater than 0.2 in 8% (2/17) of pathogenic isolates, which indicates that samples originated from a high-risk contamination source where numerous antibiotics are used (Krumperman, 1983). Aquatic bacteria are subjected to environmental contaminations in coastal and estuarine waters, particularly through wastewater treatment plants and agricultural runoff, which might carry a different amount of antimicrobials and heavy metals and subsequently give selective pressure to the aquatic bacteria to be antimicrobial-resistant (Stepanauskas et al., 2006; Gordon et al., 2007). Therefore, continuous surveillance and monitoring programs are essential for both the prevalence and the antimicrobial susceptibility profile to ensure aquaculture safety.
The pathogenic strains were serotyped for epidemiological purposes. The majority of those strains contained O8 antigen, followed by O5, O11, O3, and O1. Most of the strains could not be typed serologically for the K antigen using conventional kits. In our study, serotype O8: KUT was the most frequent, which was also found in the environmental samples in several other studies (Pal and Das, 2010; Jones et al., 2012; Zhang et al., 2013; Haley et al., 2014). Other serotypes found in this study are O5: KUT, O11: KUT, O3: KUT, O1: KUT, and O3: K20. Interestingly, serotype O1:KUT, a serovariant of O3:K6 (González-Escalona et al., 2008), played a major role in causing a massive outbreak of cholerae in Kolkata, India, and afterward found in Asia, Africa, Europe, Latin America, and the United States (Okuda et al., 1997).
The isolates of this study were categorized into 7 clusters at a similarity of 68% based on the ERIC-PCR approach. The clustering of 5 pathogenic strains out of 17 suggested their genetic linkage though they were collected from different sources at a different time interval. Unlike the other strains in this experiment, the ATCC BAA-238 reference strain only belonged to cluster G. One sediment-derived isolate (R11-40) was placed into the cluster F alone and was distinct from the rest of the isolates genetically. ERIC-PCR-based clustering did not correlate with sources of isolation, time, and serotyping. This finding is similar to that of other research, demonstrating the genetic variation within the strains of V. parahaemolyticus (Wong et al., 1999, 2000; Chen et al., 2012; Lu et al., 2016).
Approximately 24% of the pathogenic isolates found in the animal study were associated with fluid accumulation, with/without leading to the death of the rabbit, which implies that all the pathogenic isolates were not able to accumulate fluid in the rabbit intestine. A correlation between enterotoxigenicity of V. parahaemolyticus strains and virulence potential was reported in earlier studies (Hiyoshi et al., 2010). Thus, the presence of the trh virulence gene could not be linked to enterotoxigenic potential in the present experiment. Xu et al. (1994) showed that the deletion of trh led to a minor but noticeable accumulation of fluid in the ligated rabbit small intestine. These findings reveal that tdh and trh alone do not explain the cytotoxicity and enterotoxicity of pathogenic V. parahaemolyticus and propose that certain other mechanisms that have not yet been discovered may contribute to the accumulation of fluid, excluding the factors examined in this study and other studies to date (Park et al., 2004).
This study was limited to only three aquaculture sites due to funding constraints. If an extensive study including a large number of farms were taken, it would be possible to know the scenario better. However, our study provides comprehensive research on pathogenic V. parahaemolyticus isolated from different aquacultures by highlighting the occurrence, virulence, antibiotic resistance pattern, serotyping, genetic diversity, and rabbit ileal loop study. This study suggests the presence of pathogenic V. parahaemolyticus in the aquaculture sample. Moreover, their resistance to multiple antibiotics could be a threat to public health which should not be ignored. The presence of different serotypes including few pandemic serotypes might indicate the diversity and outbreak potential of these isolates. The clustering of the isolates shows their genetic diversity. The fluid accumulation in the ileal loop of rabbit suggests the pathogenicity of those isolates. These data might be of help in assessing human health risk due to the consumption of fish in the southwestern coastal area of Bangladesh. Subsequently, it can be helpful for public health organizations as a future reference for policymaking guidelines.
Data Availability Statement
The original contributions presented in the study are included in the article/Supplementary Material, further inquiries can be directed to the corresponding author/s.
Ethics Statement
The animal study was reviewed and approved by the Animal Experimental Ethics Committee, IRB Secretariat, Research Administration, CMS, International Centre for Diarrhoeal Research, Bangladesh.
Author Contributions
ZM, MM, SI, and SA: conceptualization. AS, MM, and SI: methodology. MD and MA: software. ZM, RI, DM, and AP: validation and investigation. AS, MM, SA, and MD: formal analysis and data curation. ZM and SI: resources. SA: writing—original draft preparation. MM, AS, ZM, SI, RI, and DM: writing—review and editing. MM, SA, MD, and MA: visualization. ZM: supervision, project administration, and funding acquisition. All authors have read and agreed to the published version of the manuscript.
Funding
This research study was funded by the International Centre for Diarrhoeal Disease Research, Bangladesh (icddr,b).
Conflict of Interest
The authors declare that the research was conducted in the absence of any commercial or financial relationships that could be construed as a potential conflict of interest.
Acknowledgments
We gratefully acknowledge our core donors who provide unrestricted support to our research and operations. Current donors providing unrestricted support include the Governments of Bangladesh, Canada, Sweden and the UK. We are also sincerely thankful to Md. Abdus Salam Sarder for providing his aquaculture farm in Satkhira for sampling during the period of the study and Dr. Suman Kumer Paul for cooperating us in the rabbit ileal loop experiment.
Supplementary Material
The Supplementary Material for this article can be found online at: https://www.frontiersin.org/articles/10.3389/fmicb.2021.635539/full#supplementary-material
Supplementary Figure 1 | Gel image of species-specific tlh positive pathogenic V. parahaemolyticus. (Here, serial no represents strain ID in following order: 1 = R01-15, 2 = R01-54, 3 = R01-55, 4 = R06-70, 5 = R06-75, 6 = R06-88, 7 = R06-99, 8 = R06-100, 9 = R06-101, 10 = R06-102, 11 = R06-106, 12 = R06-107, 13 = R09-14, 14 = R09-20, 15 = R10-16, 16 = R11-40, and 17 = R12-76.
References
Akther, F., Neogi, S. B., Chowdhury, W. B., Sadique, A., Islam, A., Akhter, M. Z., et al. (2016). Major tdh+ Vibrio parahaemolyticus serotype changes temporally in the bay of Bengal estuary of Bangladesh. Infect. Genet. Evol. 41, 153–159. doi: 10.1016/j.meegid.2016.04.003
Alam, M., Chowdhury, W. B., Bhuiyan, N. A., Islam, A., Hasan, N. A., Nair, G. B., et al. (2009). Serogroup, virulence, and genetic traits of Vibrio parahaemolyticus in the estuarine ecosystem of Bangladesh. Appl. Environ. Microbiol. 75, 6268–6274. doi: 10.1128/AEM.00266-09
Ansaruzzaman, M., Lucas, M., Deen, J. L., Bhuiyan, N. A., Wang, X.-Y., Safa, A., et al. (2005). Pandemic serovars (O3: K6 and O4: K68) of Vibrio parahaemolyticus associated with diarrhea in Mozambique: spread of the pandemic into the African continent. J. Clin. Microbiol. 43, 2559–2562. doi: 10.1128/JCM.43.6.2559-2562.2005
Bag, P. K., Nandi, S., Bhadra, R. K., Ramamurthy, T., Bhattacharya, S. K., Nishibuchi, M., et al. (1999). Clonal diversity among recently emerged strains ofVibrio parahaemolyticus O3: K6 associated with pandemic spread. J. Clin. Microbiol. 37, 2354–2357. doi: 10.1128/JCM.37.7.2354-2357.1999
Bancroft, J. D., and Gamble, M. (2008). Theory and Practice of Histological Techniques. London: Churchill Livingstone.
Bauer, A., Østensvik, Ø, Florvåg, M., and Ørmen, Ø, and Rørvik, L. M. (2006). Occurrence of Vibrio parahaemolyticus, V. cholerae, and V. vulnificus in Norwegian Blue Mussels (Mytilus edulis). Appl. Environ. Microbiol. 72, 3058–3061. doi: 10.1128/AEM.72.4.3058-3061.2006
Bej, A. K., Patterson, D. P., Brasher, C. W., Vickery, M. C. L., Jones, D. D., and Kaysner, C. A. (1999). Detection of total and hemolysin-producing Vibrio parahaemolyticus in shellfish using multiplex PCR amplification of tl, tdh and trh. J. Microbiol. Methods 36, 215–225. doi: 10.1016/S0167-7012(99)00037-8
Caburlotto, G., Gennari, M., Ghidini, V., Tafi, M., and Lleo, M. M. (2009). Presence of T3SS2 and other virulence-related genes in tdh-negative Vibrio parahaemolyticus environmental strains isolated from marine samples in the area of the Venetian Lagoon, Italy. FEMS Microbiol. Ecol. 70, 506–514. doi: 10.1111/j.1574-6941.2009.00764.x
Caburlotto, G., Haley, B. J., Lleò, M. M., Huq, A., and Colwell, R. R. (2010). Serodiversity and ecological distribution of Vibrio parahaemolyticus in the Venetian Lagoon, Northeast Italy. Environ. Microbiol. Reports 2, 151–157. doi: 10.1111/j.1758-2229.2009.00123.x
Chen, W., Xie, Y., Xu, J., Wang, Q., Gu, M., Yang, J., et al. (2012). Molecular typing of Vibrio parahaemolyticus isolates from the middle-east coastline of China. Int. J. Food Microbiol. 153, 402–412. doi: 10.1016/j.ijfoodmicro.2011.12.001
Chowdhury, A., Ishibashi, M., Thiem, V. D., Tuyet, D. T. N., Van Tung, T., Chien, B. T., et al. (2004). Emergence and serovar transition of Vibrio parahaemolyticus pandemic strains isolated during a diarrhea outbreak in Vietnam between 1997 and 1999. Microbiol. Immunol. 48, 319–327. doi: 10.1111/j.1348-0421.2004.tb03513.x
Clinical and Laboratory Standards Institute [CLSI] (2017). Performance Standards for Antimicrobial Susceptibility Testing. in CLSI Supplement M100. Wayne, PA: Clinical and Laboratory Standards Institute.
Cook, D. W., Bowers, J. C., and DePAOLA, A. (2002). Density of total and pathogenic (tdh+) Vibrio parahaemolyticus in Atlantic and Gulf Coast molluscan shellfish at harvest. J. Food Prot. 65, 1873–1880. doi: 10.4315/0362-028X-65.12.1873
De Bruijn, F. J. (1992). Use of repetitive (repetitive extragenic palindromic and enterobacterial repetitive intergeneric consensus) sequences and the polymerase chain reaction to fingerprint the genomes of Rhizobium meliloti isolates and other soil bacteria. Appl. Environ. Microbiol. 58, 2180–2187. doi: 10.1128/AEM.58.7.2180-2187.1992
DePaola, A., Kaysner, C. A., Bowers, J., and Cook, D. W. (2000). Environmental investigations of Vibrio parahaemolyticus in oysters after outbreaks in Washington, Texas, and New York (1997 and 1998). Appl. Environ. Microbiol. 66, 4649–4654. doi: 10.1128/AEM.66.11.4649-4654.2000
Ellingsen, A. B., Jørgensen, H., Wagley, S., Monshaugen, M., and Rørvik, L. M. (2008). Genetic diversity among Norwegian Vibrio parahaemolyticus. J. Appl. Microbiol. 105, 2195–2202. doi: 10.1111/j.1365-2672.2008.03964.x
Federation, W. E., and American Public Health Association (2005). Standard Methods for the Examination of Water and Wastewater. Washington, DC: American Public Health Association (APHA).
Froelich, B. A., and Noble, R. T. (2016). Vibrio bacteria in raw oysters: managing risks to human health. Philos. Trans. R. Soc. B Biol. Sci. 371:20150209. doi: 10.1098/rstb.2015.0209
Fuenzalida, L., Hernández, C., Toro, J., Rioseco, M. L., Romero, J., and Espejo, R. T. (2006). Vibrio parahaemolyticus in shellfish and clinical samples during two large epidemics of diarrhoea in southern Chile. Environ. Microbiol. 8, 675–683. doi: 10.1111/j.1462-2920.2005.00946.x
González-Escalona, N., Cachicas, V., Acevedo, C., Rioseco, M. L., Vergara, J. A., Cabello, F., et al. (2005). Vibrio parahaemolyticus diarrhea, Chile, 1998 and 2004. Emerg. Infect. Dis. 11:129. doi: 10.3201/eid1101.040762
González-Escalona, N., Martinez-Urtaza, J., Romero, J., Espejo, R. T., Jaykus, L.-A., and DePaola, A. (2008). Determination of molecular phylogenetics of Vibrio parahaemolyticus strains by multilocus sequence typing. J. Bacteriol. 190, 2831–2840. doi: 10.1128/JB.01808-07
Gordon, L., Giraud, E., Ganière, J.-P., Armand, F., Bouju-Albert, A., de la Cotte, N., et al. (2007). Antimicrobial resistance survey in a river receiving effluents from freshwater fish farms. J. Appl. Microbiol. 102, 1167–1176. doi: 10.1111/j.1365-2672.2006.03138.x
Haley, B. J., Kokashvili, T., Tskshvediani, A., Janelidze, N., Mitaishvili, N., Grim, C. J., et al. (2014). Molecular diversity and predictability of Vibrio parahaemolyticus along the Georgian coastal zone of the Black Sea. Front. Microbiol. 5:45. doi: 10.3389/fmicb.2014.00045
Han, F., Walker, R. D., Janes, M. E., Prinyawiwatkul, W., and Ge, B. (2007). Antimicrobial susceptibilities of Vibrio parahaemolyticus and Vibrio vulnificus isolates from Louisiana Gulf and retail raw oysters. Appl. Environ. Microbiol. 73, 7096–7098. doi: 10.1128/AEM.01116-07
Hara-Kudo, Y., Nishina, T., Nakagawa, H., Konuma, H., Hasegawa, J., and Kumagai, S. (2001). Improved method for detection of Vibrio parahaemolyticus in seafood. Appl. Environ. Microbiol. 67, 5819–5823. doi: 10.1128/AEM.67.12.5819-5823.2001
Hiyoshi, H., Kodama, T., Iida, T., and Honda, T. (2010). Contribution of Vibrio parahaemolyticus virulence factors to cytotoxicity, enterotoxicity, and lethality in mice. Infect. Immun. 78, 1772–1780. doi: 10.1128/IAI.01051-09
Ishibashi, M., Ohta, K., Shimada, T., Honda, T., Sugiyama, J., Miwatani, T., et al. (2000). Current status of OK serotype combinations of Vibrio parahaemolyticus. Nippon Saikingaku Zasshi 55:41. doi: 10.3412/jsb.55.539
Johnson, C. N., Bowers, J. C., Griffitt, K. J., Molina, V., Clostio, R. W., Pei, S., et al. (2012). Ecology of Vibrio parahaemolyticus and Vibrio vulnificus in the coastal and estuarine waters of Louisiana, Maryland, Mississippi, and Washington (United States). Appl. Environ. Microbiol. 78, 7249–7257. doi: 10.1128/AEM.01296-12
Johnson, C. N., Flowers, A. R., Noriea, N. F., Zimmerman, A. M., Bowers, J. C., DePaola, A., et al. (2010). Relationships between environmental factors and pathogenic vibrios in the northern Gulf of Mexico. Appl. Environ. Microbiol. 76, 7076–7084. doi: 10.1128/AEM.00697-10
Jones, J. L., Lüdeke, C. H. M., Bowers, J. C., DeRosia-Banick, K., Carey, D. H., and Hastback, W. (2014). Abundance of Vibrio cholerae, V. vulnificus, and V. parahaemolyticus in Oysters (Crassostrea virginica) and Clams (Mercenaria mercenaria) from Long Island Sound. Appl. Environ. Microbiol. 80, 7667–7672. doi: 10.1128/AEM.02820-14
Jones, J. L., Lüdeke, C. H. M., Bowers, J. C., Garrett, N., Fischer, M., Parsons, M. B., et al. (2012). Biochemical, serological, and virulence characterization of clinical and oyster Vibrio parahaemolyticus isolates. J. Clin. Microbiol. 50, 2343–2352. doi: 10.1128/JCM.00196-12
Joseph, S. W., Colwell, R. R., and Kaper, J. B. (1982). Vibrio parahaemolyticus and related halophilic vibrios. CRC Crit. Rev. Microbiol. 10, 77–124. doi: 10.3109/10408418209113506
Kaneko, T., and Colwell, R. R. (1975). Incidence of Vibrio parahaemolyticus in Chesapeake Bay. Appl. Environ. Microbiol. 30, 251–257. doi: 10.1128/AM.30.2.251-257.1975
Khimmakthong, U., and Sukkarun, P. (2017). The spread of Vibrio parahaemolyticus in tissues of the Pacific white shrimp Litopenaeus vannamei analyzed by PCR and histopathology. Microb. Pathog. 113, 107–112. doi: 10.1016/j.micpath.2017.10.028
Khouadja, S., Lamari, F., and Bakhrouf, A. (2013). Characterization of Vibrio parahaemolyticus isolated from farmed sea bass (Dicentrarchus labrax) during disease outbreaks. Int. Aquat. Res. 5:13. doi: 10.1186/2008-6970-5-13
Kiiyukia, C., Venkateswaran, K., Navarro, I. M., Nakano, H., Kawakami, H., and Hashimoto, H. (1989). Seasonal distribution of Vibrio parahaemolyticus serotypes along the oyster beds in Hiroshima coast [Japan]. J. Fac. Appl. Biol. Sci. 28, 49–61.
Krumperman, P. H. (1983). Multiple antibiotic resistance indexing of Escherichia coli to identify high-risk sources of fecal contamination of foods. Appl. Environ. Microbiol. 46, 165–170. doi: 10.1128/AEM.46.1.165-170.1983
Leoni, F., Talevi, G., Masini, L., Ottaviani, D., and Rocchegiani, E. (2016). Trh (tdh-/trh+) gene analysis of clinical, environmental and food isolates of Vibrio parahaemolyticus as a tool for investigating pathogenicity. Int. J. Food Microbiol. 225, 43–53. doi: 10.1016/j.ijfoodmicro.2016.02.016
Lesmana, M., Subekti, D., Simanjuntak, C. H., Tjaniadi, P., Campbell, J. R., and Oyofo, B. A. (2001). Vibrio parahaemolyticus associated with cholera-like diarrhea among patients in North Jakarta, Indonesia. Diagnostic Microbiol. Infect. Dis. 39, 71–75. doi: 10.1016/s0732-8893(00)00232-7
Letchumanan, V., Yin, W.-F., Lee, L.-H., and Chan, K.-G. (2015). Prevalence and antimicrobial susceptibility of Vibrio parahaemolyticus isolated from retail shrimps in Malaysia. Front. Microbiol. 6:33. doi: 10.3389/fmicb.2015.00033
Li, J., Xue, F., Yang, Z., Zhang, X., Zeng, D., Chao, G., et al. (2016). Vibrio parahaemolyticus strains of pandemic serotypes identified from clinical and environmental samples from Jiangsu, China. Front. Microbiol. 7:787. doi: 10.3389/fmicb.2016.00787
López-Hernández, K. M., Pardío-Sedas, V. T., Lizárraga-Partida, L., Williams, J., de, J., Martínez-Herrera, D., et al. (2015). Environmental parameters influence on the dynamics of total and pathogenic Vibrio parahaemolyticus densities in Crassostrea virginica harvested from Mexico’s Gulf coast. Mar. Pollut. Bull. 91, 317–329. doi: 10.1016/j.marpolbul.2014.11.015
Lu, X., Zhou, B., Vogt, R. D., Seip, H. M., Xin, Z., and Ekengren, Ö (2016). Rethinking China’s water policy: the worst water quality despite the most stringent standards. Water Int. 41, 1044–1048. doi: 10.1080/02508060.2016.1219188
Mahmud, Z. H., Kabir, M. H., Ali, S., Moniruzzaman, M., Imran, K. M., Nafiz, T. N., et al. (2020). Extended-spectrum beta-lactamase-producing Escherichia coli in drinking water samples from a forcibly displaced, densely populated community setting in Bangladesh. Front. Public Health 8:228. doi: 10.3389/fpubh.2020.00228
Mahmud, Z. H., Kassu, A., Mohammad, A., Yamato, M., Bhuiyan, N. A., Nair, G. B., et al. (2006). Isolation and molecular characterization of toxigenic Vibrio parahaemolyticus from the Kii Channel, Japan. Microbiol. Res. 161, 25–37. doi: 10.1016/j.micres.2005.04.005
Mahmud, Z. H., Neogi, S. B., Kassu, A., Wada, T., Islam, M. S., Nair, G. B., et al. (2007). Seaweeds as a reservoir for diverse Vibrio parahaemolyticus populations in Japan. Int. J. Food Microbiol. 118, 92–96. doi: 10.1016/j.ijfoodmicro.2007.05.009
Marshall, S., Clark, C. G., Wang, G., Mulvey, M., Kelly, M. T., and Johnson, W. M. (1999). Comparison of Molecular Methods for TypingVibrio parahaemolyticus. J. Clin. Microbiol. 37, 2473–2478. doi: 10.1128/JCM.37.8.2473-2478.1999
Martinez-Urtaza, J., Simental, L., Velasco, D., DePaola, A., Ishibashi, M., Nakaguchi, Y., et al. (2005). Pandemic Vibrio parahaemolyticus O3: K6, Europe. Emerg. Infect. Dis. 11:1319. doi: 10.3201/eid1108.050322
Matsumoto, C., Okuda, J., Ishibashi, M., Iwanaga, M., Garg, P., Rammamurthy, T., et al. (2000). Pandemic spread of an O3: K6 clone of Vibrio parahaemolyticus and emergence of related strains evidenced by arbitrarily primed PCR and toxRS sequence analyses. J. Clin. Microbiol. 38, 578–585. doi: 10.1128/JCM.38.2.578-585.2000
McCarthy, S. A., DePaola, A., Cook, D. W., Kaysner, C. A., and Hill, W. E. (1999). Evaluation of alkaline phosphatase-and digoxigenin-labelled probes for detection of the thermolabile hemolysin (tlh) gene of Vibrio parahaemolyticus. Lett. Appl. Microbiol. 28, 66–70. doi: 10.1046/j.1365-2672.1999.00467.x
Nasu, H., Iida, T., Sugahara, T., Yamaichi, Y., Park, K. -S., Yokoyama, K., et al. (2000). A filamentous phage associated with recent pandemicVibrio parahaemolyticus O3: K6 strains. J. Clin. Microbiol. 38, 2156–2161.
Okuda, J., Ishibashi, M., Hayakawa, E., Nishino, T., Takeda, Y., Mukhopadhyay, A. K., et al. (1997). Emergence of a unique O3: K6 clone of Vibrio parahaemolyticus in Calcutta, India, and isolation of strains from the same clonal group from Southeast Asian travelers arriving in Japan. J. Clin. Microbiol. 35, 3150–3155. doi: 10.1128/JCM.35.12.3150-3155.1997
Okura, M., Osawa, R., Iguchi, A., Takagi, M., Arakawa, E., Terajima, J., et al. (2004). PCR-based identification of pandemic group vibrio parahaemolyticus with a novel group-specific primer pair. Microbiol. Immunol. 48, 787–790. doi: 10.1111/j.1348-0421.2004.tb03596.x
Olive, D. M., and Bean, P. (1999). Principles and applications of methods for DNA-based typing of microbial organisms. J. Clin. Microbiol. 37, 1661–1669. doi: 10.1128/JCM.37.6.1661-1669.1999
Ottaviani, D., Leoni, F., Talevi, G., Masini, L., Santarelli, S., Rocchegiani, E., et al. (2013). Extensive investigation of antimicrobial resistance in Vibrio parahaemolyticus from shellfish and clinical sources, Italy. Int. J. Antimicrob. Agents 42, 191–193. doi: 10.1016/j.ijantimicag.2013.05.003
Pal, D., and Das, N. (2010). Isolation, identification and molecular characterization of Vibrio parahaemolyticus from fish samples in Kolkata. Eur. Rev. Med. Pharmacol. Sci. 14, 545–549.
Park, K.-S., Ono, T., Rokuda, M., Jang, M.-H., Okada, K., Iida, T., et al. (2004). Functional characterization of two type III secretion systems of Vibrio parahaemolyticus. Infect. Immun. 72, 6659–6665. doi: 10.1128/IAI.72.11.6659-6665.2004
Patel, J. B., Cockerill, F. R., and Bradford, P. A. (2015). Performance Standards for Antimicrobial Susceptibility Testing: Twenty-Fifth Informational Supplement. Wayne, PA: Clinical and Laboratory Standards Institute.
Pui, C. F., Bilung, L. M., Zin, N. B. M., Abidin, N. N. B. Z., Micky, V., and Kasing, A. (2014). Risk of acquiring Vibrio parahaemolyticus in water and shrimp from an aquaculture farm. Kuroshio Sci. 8, 59–62.
Quilici, M.-L., Robert-Pillot, A., Picart, J., and Fournier, J.-M. (2005). Pandemic Vibrio parahaemolyticus O3: K6 spread. France. Emerg. Infect. Dis. 11:1148. doi: 10.3201/eid1107.041008
R Core Team (2013). R: A Language and Environment for Statistical Computing. Vienna: R Foundation for Statistical Computing.
Rivera, I. G., Chowdhury, M. A., Huq, A., Jacobs, D., Martins, M. T., and Colwell, R. R. (1995). Enterobacterial repetitive intergenic consensus sequences and the PCR to generate fingerprints of genomic DNAs from Vibrio cholerae O1. O139, and non-O1 strains. Appl. Environ. Microbiol. 61, 2898–2904. doi: 10.1128/AEM.61.8.2898-2904.1995
Sala, M. R., Arias, C., Dominguez, A., Bartolomé, R., and Muntada, J. M. (2009). Foodborne outbreak of gastroenteritis due to Norovirus and Vibrio parahaemolyticus. Epidemiol. Infect. 137, 626–629. doi: 10.1017/S0950268808001040
Shinoda, S., Nakahara, N., Ninomiya, Y., Itoh, K., and Kane, H. (1983). Serological method for identification of Vibrio parahaemolyticus from marine samples. Appl. Environ. Microbiol. 45, 148–152. doi: 10.1128/AEM.45.1.148-152.1983
Stepanauskas, R., Glenn, T. C., Jagoe, C. H., Tuckfield, R. C., Lindell, A. H., King, C. J., et al. (2006). Coselection for microbial resistance to metals and antibiotics in freshwater microcosms. Environ. Microbiol. 8, 1510–1514. doi: 10.1111/j.1462-2920.2006.01091.x
Su, Y.-C., and Liu, C. (2007). Vibrio parahaemolyticus: a concern of seafood safety. Food Microbiol. 24, 549–558. doi: 10.1016/j.fm.2007.01.005
Sujeewa, A. K. W., Sani, N. A., and Laina, M. (2009). Prevalence of toxic genes of Vibrio parahaemolyticus in shrimps (Penaeus monodon) and culture environment. Int. Food Res. J. 16, 89–95.
Takemura, A. F., Chien, D. M., and Polz, M. F. (2014). Associations and dynamics of Vibrionaceae in the environment, from the genus to the population level. Front. Microbiol. 5:38. doi: 10.3389/fmicb.2014.00038
Taniguchi, H., Hirano, H., Kubomura, S., Higashi, K., and Mizuguchi, Y. (1986). Comparison of the nucleotide sequences of the genes for the thermostable direct hemolysin and the thermolabile hemolysin from Vibrio parahaemolyticus. Microb. Pathog. 1, 425–432. doi: 10.1016/0882-4010(86)90004-5
Twedt, R. M., Peeler, J. T., and Spaulding, P. L. (1980). Effective ileal loop dose of Kanagawa-positive Vibrio parahaemolyticus. Appl. Environ. Microbiol. 40, 1012–1016. doi: 10.1128/AEM.40.6.1012-1016.1980
Velazquez-Roman, J., León-Sicairos, N., Hernandez-Diaz, L. D. J., and Canizalez-Roman, A. (2014). Pandemic Vibrio parahaemolyticus O3: K6 on the American continent. Front. Cell. Infect. Microbiol. 3:110. doi: 10.3389/fcimb.2013.00110
Versalovic, J., Koeuth, T., and Lupski, R. (1991). Distribution of repetitive DNA sequences in eubacteria and application to finerpriting of bacterial genomes. Nucleic Acids Res. 19, 6823–6831. doi: 10.1093/nar/19.24.6823
Wang, H., Wong, M. M. L., O’Toole, D., Mak, M. M. H., Wu, R. S. S., and Kong, R. Y. C. (2006). Identification of a DNA methyltransferase gene carried on a pathogenicity island-like element (VPAI) in Vibrio parahaemolyticus and its prevalence among clinical and environmental isolates. Appl. Environ. Microbiol. 72, 4455–4460. doi: 10.1128/AEM.02095-05
West, C. K. G., Klein, S. L., and Lovell, C. R. (2013). High frequency of virulence factor genes tdh, trh, and tlh in Vibrio parahaemolyticus strains isolated from a pristine estuary. Appl. Environ. Microbiol. 79, 2247–2252. doi: 10.1128/AEM.03792-12
Whitaker, W. B., Parent, M. A., Naughton, L. M., Richards, G. P., Blumerman, S. L., and Boyd, E. F. (2010). Modulation of responses of Vibrio parahaemolyticus O3: K6 to pH and temperature stresses by growth at different salt concentrations. Appl. Environ. Microbiol. 76, 4720–4729. doi: 10.1128/AEM.00474-10
Williams, T. L., Musser, S. M., Nordstrom, J. L., DePaola, A., and Monday, S. R. (2004). Identification of a protein biomarker unique to the pandemic O3: K6 clone of vibrio parahaemolyticus. J. Clin. Microbiol. 42, 1657–1665. doi: 10.1128/jcm.42.4.1657-1665.2004
Wong, H.-C., Liu, C.-C., Pan, T.-M., Wang, T.-K., Lee, C.-L., and Shih, D. Y.-C. (1999). Molecular typing of Vibrio parahaemolyticus isolates, obtained from patients involved in food poisoning outbreaks in Taiwan, by random amplified polymorphic DNA analysis. J. Clin. Microbiol. 37, 1809–1812. doi: 10.1128/JCM.37.6.1809-1812.1999
Wong, H.-C., Liu, S.-H., Ku, L.-W., Lee, I.-Y., Wang, T.-K., Lee, Y.-S., et al. (2000). Characterization of Vibrio parahaemolyticus isolates obtained from foodborne illness outbreaks during 1992 through 1995 in Taiwan. J. Food Prot. 63, 900–906. doi: 10.4315/0362-028X-63.7.900
Xu, M., Yamamoto, K., and Honda, T. (1994). Construction and characterization of an isogenic mutant of Vibrio parahaemolyticus having a deletion in the thermostable direct hemolysin-related hemolysin gene (trh). J. Bacteriol. 176, 4757–4760. doi: 10.1128/JB.176.15.4757-4760.1994
Yang, Z., Jiao, X., Zhou, X., Cao, G., Fang, W., and Gu, R. (2008). Isolation and molecular characterization of Vibrio parahaemolyticus from fresh, low-temperature preserved, dried, and salted seafood products in two coastal areas of eastern China. Int. J. Food Microbiol. 125, 279–285. doi: 10.1016/j.ijfoodmicro.2008.04.007
Yano, Y., Hamano, K., Satomi, M., Tsutsui, I., and Aue-umneoy, D. (2011). Diversity and characterization of oxytetracycline-resistant bacteria associated with non-native species, white-leg shrimp (Litopenaeus vannamei), and native species, black tiger shrimp (Penaeus monodon), intensively cultured in Thailand. J. Appl. Microbiol. 110, 713–722. doi: 10.1111/j.1365-2672.2010.04926.x
Yeung, P. S. M., and Boor, K. J. (2004). Epidemiology, pathogenesis, and prevention of foodborne Vibrio parahaemolyticus infections. Foodborne Pathog. Dis. 1, 74–88. doi: 10.1089/153531404323143594
Keywords: Vibrio parahaemolyticus, aquaculture, rabbit ileal loop, histopathology, trh
Citation: Siddique AB, Moniruzzaman M, Ali S, Dewan MdN, Islam MR, Islam MdS, Amin MB, Mondal D, Parvez AK and Mahmud ZH (2021) Characterization of Pathogenic Vibrio parahaemolyticus Isolated From Fish Aquaculture of the Southwest Coastal Area of Bangladesh. Front. Microbiol. 12:635539. doi: 10.3389/fmicb.2021.635539
Received: 30 November 2020; Accepted: 27 January 2021;
Published: 08 March 2021.
Edited by:
Francisco Noé Arroyo López, Consejo Superior de Investigaciones Científicas (CSIC), SpainReviewed by:
Guilherme Campos Tavares, Federal University of Minas Gerais, BrazilLigia Virginia Da Silva, University of Maryland Eastern Shore, United States
Ilenys M. Perez-Diaz, Food Science Research (USDA-ARS), United States
Copyright © 2021 Siddique, Moniruzzaman, Ali, Dewan, Islam, Islam, Amin, Mondal, Parvez and Mahmud. This is an open-access article distributed under the terms of the Creative Commons Attribution License (CC BY). The use, distribution or reproduction in other forums is permitted, provided the original author(s) and the copyright owner(s) are credited and that the original publication in this journal is cited, in accordance with accepted academic practice. No use, distribution or reproduction is permitted which does not comply with these terms.
*Correspondence: Zahid Hayat Mahmud, emhtYWhtdWRAaWNkZHJiLm9yZw==