- 1Faculty of Natural Resources and Marine Sciences, Tarbiat Modares University, Tehran, Iran
- 2Laboratory of Environmental Microbiology, Institute of Microbiology, Czech Academy of Sciences, Prague, Czechia
- 3Microbial Biotechnology Department, Agricultural Biotechnology Research Institute of Iran, Agricultural Research, Education and Extension Organization, Karaj, Iran
- 4Human Genetics Research Centre, Baqiyatallah University of Medical Sciences, Tehran, Iran
The diverse chemical, biological, and microbial properties of litter and organic matter (OM) in forest soil along an altitudinal gradient are potentially important for nutrient cycling. In the present study, we sought to evaluate soil chemical, biological, microbial, and enzymatic characteristics at four altitude levels (0, 500, 1,000, and 1,500 m) in northern Iran to characterize nutrient cycling in forest soils. The results showed that carbon (C) and nitrogen (N) turnover changed with altitude along with microbial properties and enzyme activity. At the lowest altitude with mixed forest and no beech trees, the higher content of N in litter and soil, higher pH and microbial biomass nitrogen (MBN), and the greater activities of aminopeptidases affected soil N cycling. At elevations above 1,000 m, where beech is the dominant tree species, the higher activities of cellobiohydrolase, arylsulfatase, β-xylosidase, β-galactosidase, endoglucanase, endoxylanase, and manganese peroxidase (MnP) coincided with higher basal respiration (BR), substrate-induced respiration (SIR), and microbial biomass carbon (MBC) and thus favored conditions for microbial entropy and C turnover. The low N content and high C/N ratio at 500-m altitude were associated with the lowest microbial and enzyme activities. Our results support the view that the plain forest with mixed trees (without beech) had higher litter quality and soil fertility, while forest dominated by beech trees had the potential to store higher C and can potentially better mitigate global warming.
Introduction
In terrestrial ecosystems, forest soils, as the largest carbon (C) reservoir, play a pivotal role in C cycling (Nottingham et al., 2020) and can potentially affect nitrogen (N) dynamics (Ndossi et al., 2020). Many studies have indicated that geography, soil, and litter properties (soil pH, nutrient content, and water content) and aboveground biotic factors (stand composition and diversity) are important predictors of soil C and N stocking (Delgado-Baquerizo et al., 2017; Walker et al., 2018). In addition, altitude has long been considered a potential driver of soil nutrient turnover through its influence on the biotic and abiotic components of forest ecosystems (Gebrewahid et al., 2018). Although altitude may not have a direct impact on soil organic matter (SOM) turnover, it can still influence this process indirectly by shaping soil, climatic, and vegetation conditions (Sierra and Causeret, 2018; Devi and Sherpa, 2019). Climatic conditions (i.e., decreased temperature and humidity) are heavily influenced by altitude, which in turn affects vegetation distribution/composition and alters the quality and quantity of litter/soil characteristics and C loss (Kotas et al., 2018; Quan et al., 2019). Recent studies have documented how changes in climatic conditions and forest canopy composition associated with altitude can affect the litter decomposition rate (Cardelli et al., 2019), soil microbial/enzyme activities (Feng et al., 2019; D’Alò et al., 2021), and nutrient storage in different ways (Bello et al., 2015).
As the main source of SOM, tree species composition and diversity along an altitudinal gradient may have a strong impact on C and N cycling (Gebrewahid et al., 2018; Sierra and Causeret, 2018). Despite the importance of soil nutrient cycling in the characterization of soil biogeochemistry under varying forest ecosystems (Bello et al., 2015; Frouz, 2018), the nature of soil nutrient reservoirs and their turnover in different regions are largely unknown. SOM decomposition involves both chemical and biological processes, including enzymatic catalysis (Sierra and Causeret, 2018; Nottingham et al., 2019), which generally decrease with increasing altitude, resulting in slower decomposition of organic matter (OM) (D’Alò et al., 2021).
Soil extracellular enzyme activities are essential for C and N cycling/stocking and litter decomposition and thus for microbial life (Baldrian and Štursová, 2011; Šnajdr et al., 2013; D’Alò et al., 2021). They also play an essential role in soil fertility as useful indicators for soil management (Baldrian et al., 2010; Cardelli et al., 2019). Changes in tree species composition along an altitudinal gradient alter soil microbial activity and thus the activity of soil enzymes (Feng et al., 2019; Ndossi et al., 2020). In fact, enzyme activity is sensitive to changes in vegetation and is closely related to soil primary quality, and health (Šnajdr et al., 2013; Nottingham et al., 2019). Soil nutrients (Cardelli et al., 2019), forest stands (Šnajdr et al., 2013), and climate (Walker et al., 2018) are factors affecting extracellular enzyme activity along altitudinal gradients. Recent studies have shown that the activity of C- and N-dependent enzymes has different trends with increasing altitude (Ndossi et al., 2020; D’Alò et al., 2021). The effects of altitude on soil extracellular enzyme activities are highly variable across individual studies (Lladó et al., 2017), since they do not follow simple biochemical rules (Baldrian et al., 2013). It is widely assumed that extracellular enzyme activity increases with increase in temperature, but in the forest altitudinal gradient their activity is more related to the quality of available OM, vegetation, and nutrient demands of the microbial biomass (Nottingham et al., 2019; D’Alò et al., 2021), which can have different trends with altitude (Lladó et al., 2017; Ndossi et al., 2020). Meng et al. (2020) reported that the higher C content of the soils at the higher altitude may denote a bigger labile C availability for C-dependent extracellular enzymes.
Moreover, knowledge about the function and activity of soil microorganisms along altitude is essential for understanding forest soil productivity (Massaccesi et al., 2020), as soil microbes play a fundamental role in biogeochemical cycling (Walker et al., 2018). Soil microbes play a pivotal role in the mineralization and decomposition of SOM by producing various hydrolytic enzymes (Cardelli et al., 2019). In particular, basal respiration (BR), substrate-induced respiration (SIR), microbial biomass C (MBC), microbial biomass N (MBN), and metabolic and microbial quotients as microbial indicators change with forest vegetation along the altitudinal gradient, while soil organic C (SOC) is often less affected (Walker et al., 2018; Massaccesi et al., 2020). In most cases, MBC and MBN showed different trends with changes of forest types along the altitudinal gradient (Massaccesi et al., 2020; Ndossi et al., 2020). Climatic conditions, vegetation, and edaphic factors also contribute to changes in substrate availabilities and microbial activities (Walker et al., 2018; Ndossi et al., 2020). Trees, as the primary source of plant biomass, affect microbial processes in ecosystems via specific litter chemistry and rhizodeposition (Šnajdr et al., 2013). Moreover, soil microbial activities are directly linked to C and N turnover/stock (Urbanová et al., 2015; Razanamalala et al., 2018) and mineralization of OM (Walker et al., 2018; Nottingham et al., 2019).
Soil fauna represents another important driver of SOM dynamics (García-Palacios et al., 2013; Frouz, 2018). Soil macro- and meso-organisms play key roles in nutrient cycling and SOM decomposition through their own metabolism, stimulation of microbial activity, and bioturbation (Frouz, 2018). Soil fauna is known as the engineer of the soil ecosystem that interacts with the soil microbiome and influences litter decomposition and nutrient cycling (García-Palacios et al., 2013). Climate directly alters litter decay because of the sensitivity of processes mediated by soil fauna to factors such as temperature or precipitation (García-Palacios et al., 2013). Recent studies have identified environmental factors (i.e., temperature and moisture) (García-Palacios et al., 2013), stand composition (Massaccesi et al., 2020), and soil and litter properties (Bayranvand et al., 2017b) as the main factors influencing the activity of soil fauna and flora in natural forests along altitudinal gradients (Bayranvand et al., 2021b).
Hyrcanian forests are located south of the Caspian Sea in northern Iran and are covered by temperate broadleaf forests (Bayranvand et al., 2017b), which are similar to vegetation in parts of Europe, northern Turkey, and the Caucasus (Talebi et al., 2013). Forest stand composition and climate change with altitude (Talebi et al., 2013), which in turn affect soil microbial and enzyme activities, and nutrient turnover (Gebrewahid et al., 2018; Banday et al., 2019). The aim of the present study was to investigate (i) how a shift in forest stand composition along an altitudinal gradient affects litter and soil chemical properties and soil C and N cycling; (ii) how biological (earthworm, nematode, protozoa, and fine-root biomass), microbial (BR, SIR, and microbial biomass C and N), and enzymatic activity patterns change along an elevation gradient; and (iii) which factors determine C and N stocks across transects. We hypothesized that (i) the C stock increases with altitude due to decreased litter decomposition and quality of beech forests and a low soil temperature (ST); (ii) the N stock is greater at low altitudes where tree diversity, pH, and litter quality are high; and (iii) the soil microbial respiration, MBC, and C-dependent enzymes are associated with higher soil and litter C with increasing altitude where beech forest are dominant, while the opposite is assumed for the N stock.
Materials and Methods
Study Area
Soil and litter samples were collected in the Hyrcanian mountain forest along three parallel altitudinal transects from 0 to 1,500 m a.s.l. in Mazandaran Province in northern Iran (57°90′–58°20′ E 43°30′–43°90′ N) (Figure 1). The region has a sub-Mediterranean climate where the mean annual temperature (MAT) decreases from 15 to 5°C (4–5°C decrease in temperature per 1,000 m), and the mean annual precipitation (MAP) decreases from 1,200 to 600 mm along an altitudinal gradient. Approximately 35–45% of the rainfall occurs in autumn, 18–35% in winter, and the rest (10–20%) in summer (Noushahr city meteorological station; for the period 1977–2010). The MAP is approximately 898, 843, 805, and 746 mm at 0, 500, 1,000, and 1,500 m a. s. l., respectively (Karger et al., 2017; Figure 1). The MATs at 0, 500, 1,000, and 1,500 m a. s. l. are 19.2, 16.3, 14, and 11.6°C, respectively. Based on clear changes in tree canopy composition along elevation, four altitude classes based on dominant trees were identified from 0 to 1,500 m a.s.l. (Khaleghi et al., 1997): including (1) plain mixed forests (0 m) with ironwood (Parrotia persica C.A.M.), oak (Quercus castaneifolia C.A.M.), hornbeam (Carpinus betulus L.), alder (Alnus subcordata), and Persian poplar (Populus alba caspica Bornm); (2) low mountainous mixed forests (500 m) with beech (Fagus orientalis Lipsky), ash (Fraxinus excelsior L), and ironwood; (3) middle mountainous mixed forests (1,000 m) with beech, maple (Acer velutinum Boiss), and hornbeam; and (4) high mountainous pure forests (1,500 m) with pure beech.
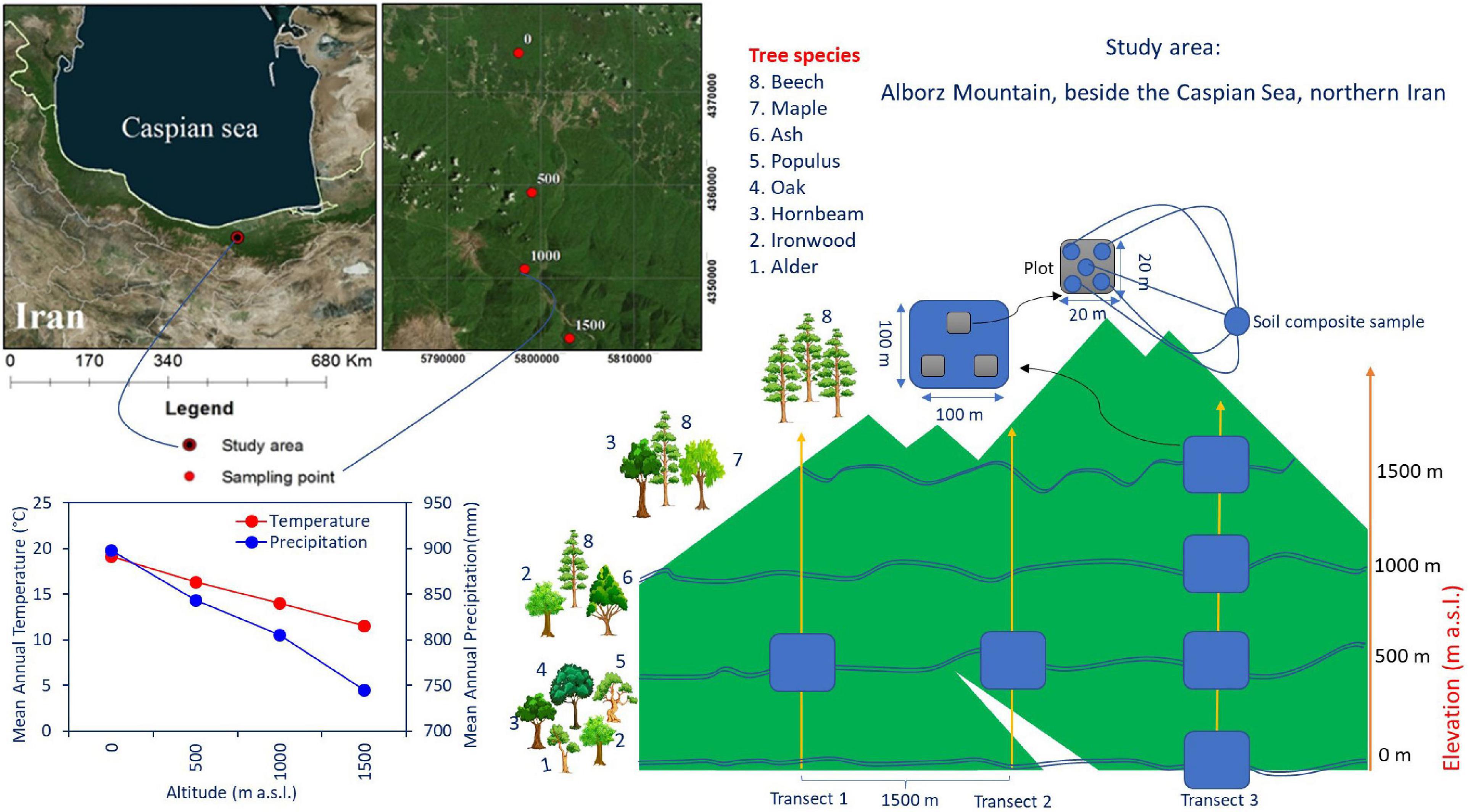
Figure 1. The study site at the Experimental Forest Station, Vaz watershed in the Central Caspian region of northern Iran, and tree species composition along the altitudinal gradient in the Alborz Mountains. The tree species growing in the area were Fagus orientalis Lipsky (beech), Parrotia persica C.A.M. (ironwood), Carpinus betulus L. (hornbeam), Quercus castaneifolia C.A.M. (oak), Fraxinus excelsior L. (ash), Acer velutinum (maple), Alnus subcordata C.A.M. (alder), and Populus alba caspica Bornm (Persian poplar).
Sample Collection and Laboratory Analysis
The study area constitutes an altitude gradient from 0 to 1,500 m along the Albourz Mountains on the southern coast of the Caspian Sea. At each elevation level, three 1-ha plots with a horizontal distance of 1,500 m were sampled. Each of these main plots was subdivided into three random subplots (each 400 m2). Samples were collected from corners and the center of each subplot (five samples per subplot, Figure 1). Elevation at each main plot was recorded using a Garmin model GPSMAP 60Cx. All living trees and the diameter of the trees at breast height (DBH, 1.3 m) were measured in each subplot with diameter tape for calculating of trees basal area (Table 1). Litter and soil samples were collected during May 2018. In total, 180 samples were collected for laboratory analyses (four altitude levels × three main plots × three subplots × five profiles). The soil and litter samples collected in each subplot were pooled separately and processed as an independent replicate for each elevation level.
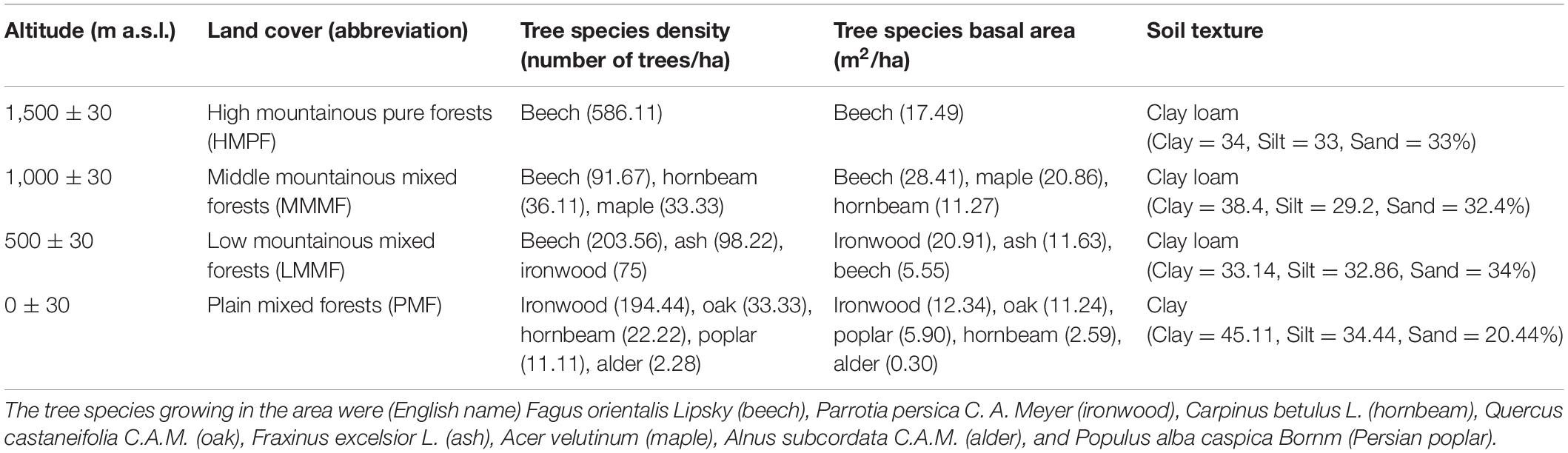
Table 1. Distribution of forest types and properties along an altitudinal gradient in the Hyrcanian forest profile (Vaz catchment, Alborz Mountains, northern Iran).
After digging the profile, organic layer thickness [i.e., OL, OF, and OH (in some profiles)] was measured with tape from the forest-floor surface to the top of the mineral soil (Bayranvand et al., 2021a). Subsequently, composite litter samples including OL and OF layers were obtained (Bayranvand et al., 2017a). After removing organic layers, soil samples were collected with plastic tubes (5 cm diameter). At the same time, earthworms were counted in a metal frame (30 × 30 × 10 cm) in both organic and mineral layers. ST was also recorded with a portable temperature probe (model: TA-288).
Litter samples were transported to the laboratory, dried at 65°C in a fan-assisted oven for 48 h and crushed/homogenized with an electric stamp for subsequent chemical analysis.
Soil samples were kept in a Styrofoam box filled with dry ice and transferred to the laboratory. Soil samples were sieved using a<2-mm mesh and either kept at 4°C for the measurement of chemical and soil respiration or freeze-dried and stored at −20°C for subsequent enzyme activity assays.
Chemical, Biological, and Microbial Properties
The organic C content in litter samples was determined by dry combustion at 450°C for 4 h. The N content was measured using the micro-Kjeldahl technique (Bremner et al., 1982). Soil pH was measured with an Orion Analyser Model 901 pH meter in a 1:2.5 soil: deionized water slurry. Bulk density was calculated by the cold method [soil dry weight/core volume basis (Plaster, 1985)]. Soil moisture (SM) was measured by drying soil samples at 105°C for 24 h. SOC and total N (TN) were measured by the modified method of Walkley–Black (wet oxidation) (Allison, 1975) and micro-Kjeldahl techniques (Bremner et al., 1982). C and N contents were calculated by taking into account soil bulk density and depth (Plaster, 1985). MBC (Anderson and Domsch, 1990) and MBN (Brookes et al., 1985) were assessed by chloroform fumigation extraction using 5 g fresh soil and 20 ml 0.5 M K2SO4. MBC was calculated as the difference between organic C extracted from fumigated and non-fumigated soils using a conversion factor of 0.45. MBN was calculated by subtracting extracted N from fumigated and non-fumigated soils using a conversion factor of 0.54. Soil BR and SIR were measured by trapping CO2 emitted from fresh soil with NaOH in glass tubes during 5 days of incubation at 25°C (Alef, 1995) and titration with HCl. Glucose (1%) was used as a substrate for the SIR measurement. The ratio of the carbon availability index (CAI), metabolic quotient (qCO2), and microbial entropy ratio were calculated by dividing BR to SIR, BR to MBC, and MBC to Corg, respectively (Cheng et al., 1996).
To demonstrate soil biological activity, earthworm, nematode and protozoan population densities as well as fine-root biomass were measured. Earthworms were enumerated in a 30-cm2 area and 10-cm depth (Bayranvand et al., 2017b). Nematode and protozoal densities were estimated in 100 g fresh soil by the modified cotton-wool filter method. After adding 1 g glucose and centrifugation, the numbers of nematodes (Liang et al., 2009) and protozoa (Mayzlish and Steinberger, 2004) were counted under a microscope. To determine the biomass of fine roots (<2 mm diameter), roots were extracted from 10-cm3 soil samples, washed with water, dried at 65°C, and weighed (Neatrour et al., 2005).
Enzyme Activity Assays
In our study, the potential of exocleaving hydrolytic, endocleaving polysaccharide hydrolases, and ligninolytic enzyme activities were measured in laboratory. To assay exocleaving hydrolytic enzymes, 0.5 g of fresh soil was homogenized in 50 ml of 50 mM sodium acetate (pH 5.0) using an UltraTurrax (IKA Labortechnik, Germany) for 3 min at 8,000 rpm in an ice bath. The activities of arylsulfatase (EC 3.1.6.1), 1,4-α-glucosidase (EC 3.2.1.20), cellobiohydrolase (exocellulase; EC 3.2.1.91), 1,4-β-glucosidase (EC 3.2.1.21), 1,4-β-xylosidase (EC 3.2.1.37), N-acetylglucosaminidase (chitinase, EC 3.2.1.30), phosphomonoesterase (PME, EC 3.1.3.2), alanine aminopeptidase (EC 3.4.11.12), and leucine aminopeptidase (EC 3.4.11.1) were measured in homogenate using 4-methylumbelliferol- (MUF) or 7-amido-4-methylcoumarin- (AMC)-based substrates as described previously (Baldrian, 2009). The substrates were dissolved in DMSO at a final concentration of 500 μM. For background fluorescence subtraction, 200 μl of 50 mM sodium acetate (pH 5.0) was combined with 40 μl of MUF standards to correct the results for fluorescence quenching. The multiwell plates were incubated at 40°C, and the fluorescence was recorded after 5 min and until 125 min using a microplate reader (Infinite, TECAN, Austria) at an excitation wavelength of 355 nm and an emission wavelength of 460 nm. Enzymatic activities were calculated based on a standard curve of MUF or AMC. One unit of enzyme activity was defined as the amount of enzyme releasing 1 nmol of MUF or AMC per min and expressed per gram of soil dry mass.
To analyse the activity of endocleaving polysaccharide hydrolases and ligninolytic enzymes, homogenized samples were extracted at 4°C for 2 h on an orbital shaker (100 rpm) with 100 mM phosphate buffer, pH 7 (16: 1 w/v), filtered through Whatman #5 filter paper and desalted using PD-10 desalting columns (Pharmacia, Sweden) according to the supplier’s protocol to remove inhibitory small-molecular mass compounds (Baldrian, 2009). Laccase (EC 1.10.3.2) activity was measured by monitoring the oxidation of 2,2′-azinobis-3-ethylbenzothiazoline-6-sulfonic acid in citrate–phosphate (100 mM citrate, 200 mM phosphate) buffer, pH 5.0, at 420-nm wavelength. Manganese peroxidase (MnP, EC 1.11.1.13) was assayed in succinate–lactate buffer (100 mM, pH 4.5). 3-Methyl-2-benzothiazolinone hydrazone and 3,3-dimethylaminobenzoic acid were oxidatively coupled by the enzymes, and the resulting purple indamine dye was detected spectrophotometrically at 595 nm. The results were corrected using the activities of the samples without manganese (for MnP); the addition of manganese sulfate was substituted by an equimolar amount of ethylenediaminetetraacetic acid (EDTA). Endo-1,4-β-glucanase (endocellulase; EC 3.2.1.4) and endo-1,4-β-xylanase (EC 3.2.1.8) activities were measured with azo-dyed carbohydrate substrates (carboxymethyl cellulose and birch wood xylan, respectively) using the protocol of the supplier (Megazyme, Ireland). The reaction mixture contained 0.2 ml of 2% dyed substrate in 200 mM sodium acetate buffer (pH 5.0) and 0.2 ml of the sample. The reaction mixture was incubated at 40°C for 60 min and stopped by adding 1 ml of ethanol followed by vortexing for 10 s and centrifuging for 10 min at 10,000 × g (Baldrian, 2009). The amount of released dye was measured at 595 nm, and the enzyme activity was calculated according to standard curves correlating the dye release with the release of reducing sugars. One unit of enzyme activity was defined as the amount of enzyme releasing 1 mmol reducing sugars per minute. Enzyme activity was expressed per g soil dry mass.
Statistical Analysis
To test for normality and equality of the variances of variables, Kolmogorov–Smirnov and Levene’s tests were employed, respectively. The differences in litter floor and soil physicochemical, biological, biochemical, and enzyme activities across the four forest types along an altitudinal gradient were analysed using one-way analysis of variance (one-way ANOVA). Means were compared using Tukey tests (HSD test). P-values < 0.05 were considered statistically significant. To examine associations between litter floor, soil data, and enzyme activities, a multivariate ANOVA analysis based on principal component analysis (PCA) was performed using the “ellipse” package in R version 3.3.2 (R Core Team, 2016). The two first axes with higher explained variations were plotted in PCA. Selected for further interpretation of the results, their relationship with all parameters was determined through a correlation matrix.
Results
Litter and Soil Chemical, Biological, and Microbial Properties
As expected, the altitude gradient greatly influenced tree canopy composition, litter quality, and quantity and soil chemical and biological properties, but with different trends (Tables 1, 2). Litter C was significantly elevated at 1,000–1,500-m altitudes, while litter N was significantly higher at the lowest altitude (0 m) than at the other altitude levels. In addition, the lowest litter C/N ratio and thickness were also found at this altitude level and increased with altitude (Table 2). The highest SM was recorded at 1,500 m. ST, however, sharply decreased with increasing altitude. The highest soil pH was recorded at the lowest (0 m) and highest (1,500 m) altitudes. Soil C was higher at elevations above 1,000 m, while soil N was significantly higher at the 0-m altitude (Table 2). The lowest soil C/N ratio was recorded at the 0-m altitude, and its content increased with altitude, particularly in beech forests (Table 2).
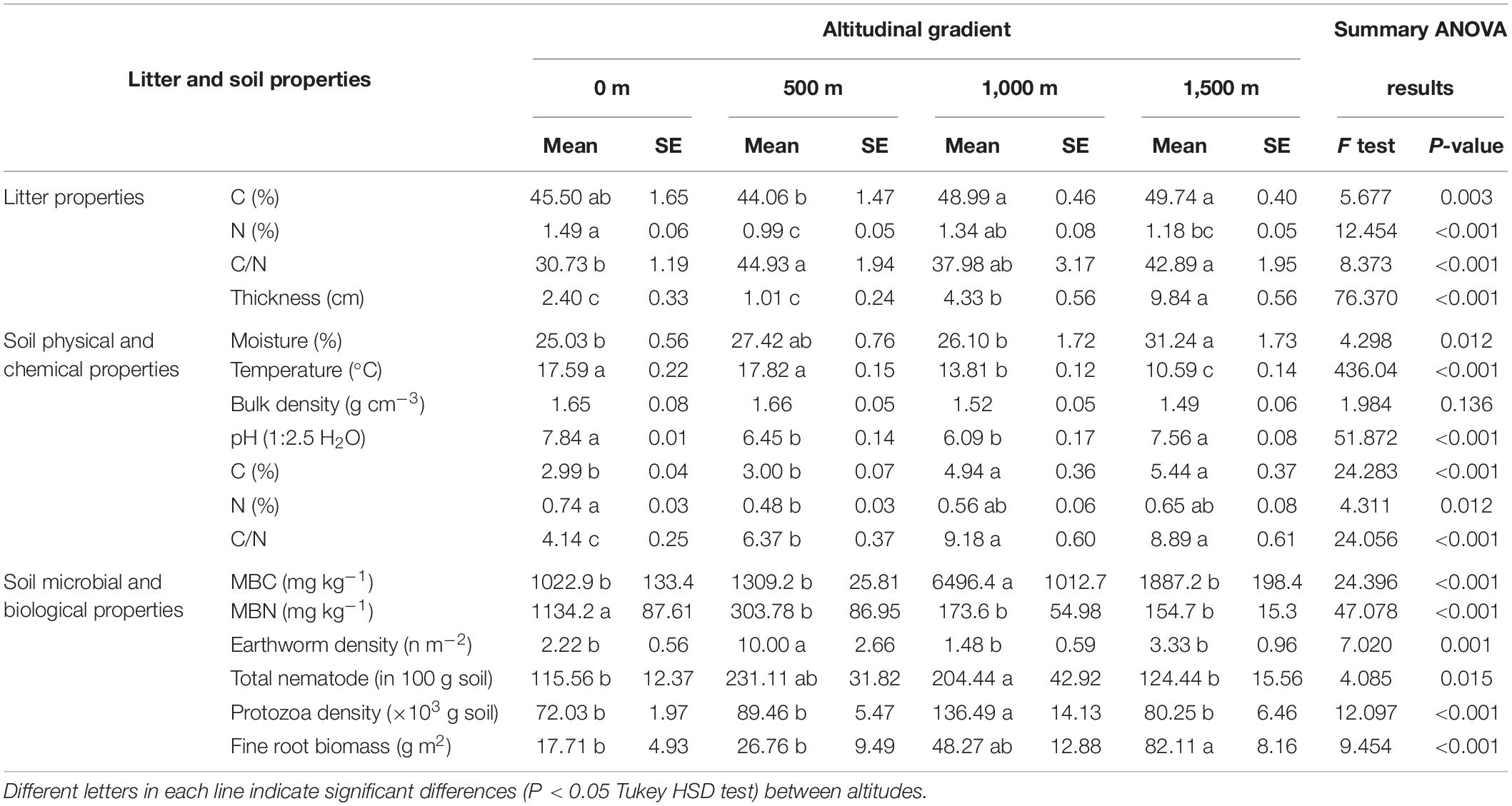
Table 2. Litter and soil chemical, biological, and microbial properties along an altitudinal gradient.
The highest amount of MBC was observed at 1,000 m, while the highest MBN was found at the 0-m altitude level, and the content decreased along the elevation gradient (Table 2). A significantly higher soil BR and SIR were observed at 1,500 m in pure beech forests compared with 0 > 500 ≈ 1,000 m altitude levels (Figure 2A). The CAI ratio did not change along the altitudinal gradient (Figure 2B). Microbial entropy was enhanced at the 1,000-m altitude compared with other altitude levels (Figure 2C), while the lowest altitude (0 m; mixed plain forest) showed the highest qCO2 ratio compared with other levels (mixed and pure beech forests) (Figure 2D). The soil C stock was highest under mixed and pure beech forests at 1,000- to 1,500-m elevation levels compared with the lower altitudes (0–500 m) (Figure 2E). The N stock was significantly higher at 0-m altitude under plain forest than under pure forest (1,500 m) (Figure 2F). The highest density of earthworms was found at 500 m, whereas the total protozoan density was highest at 1,000 m (Table 2). Fine-root biomass also increased along the altitudinal gradient (Table 2).
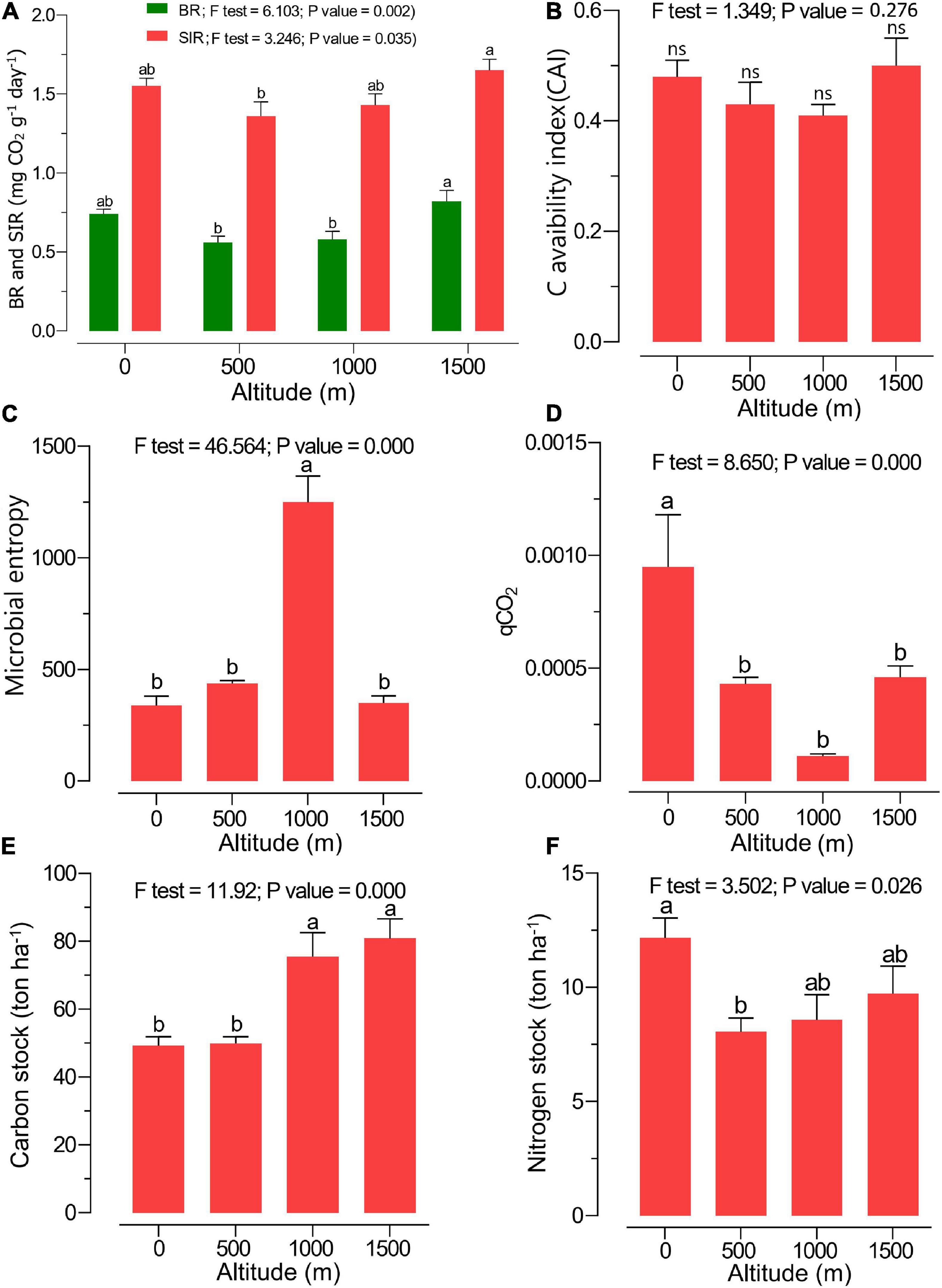
Figure 2. Soil microbial/biochemical properties such as (A) soil basal respiration (BR; green) and substrate-induced respiration (SIR; red), (B) carbon availability index (CAI), (C) microbial entropy ratio, (D) metabolic quotient (qCO2), (E) carbon stock, and (F) nitrogen stock at various altitudes. Different letters indicate a significant difference along the altitudinal gradient [analysis of variance (ANOVA) followed by Tukeys HSD post hoc test; p < 0.05].
Soil Enzyme Activity
The extracellular enzyme activities were significantly different between altitudes with no clear trend (Figures 3A–P). The activities of β-glucosidase (Figure 3A), acid phosphatase (Figure 3B), N-acetylglucosaminidase (Figure 3C), arylsulfatase (Figure 3D), and laccase (Figure 3E) were significantly higher at higher elevation levels (1,000 to 1,500 m). Specifically, higher activities of cellobiohydrolase (Figure 3H), endoglucanase (Figure 3I), endoxylanase (Figure 3K), oxidase (Figure 3F), peroxidase (Figure 3G), and Mn-peroxidase (Figure 3J) were observed at the middle altitude level (1,000 m), where mixed beech forest is dominant. In contrast, the activities of lipase (Figure 3N), alanine aminopeptidase (Figure 3O), and leucine aminopeptidase (Figure 3P) declined with increasing altitude, with the highest activities in plain forests (0 m altitude level). The lowest activity for β-xylosidase (Figure 3L) and β-galactosidase (Figure 3M) was recorded at 500 m with no clear trend across the altitudinal gradient.
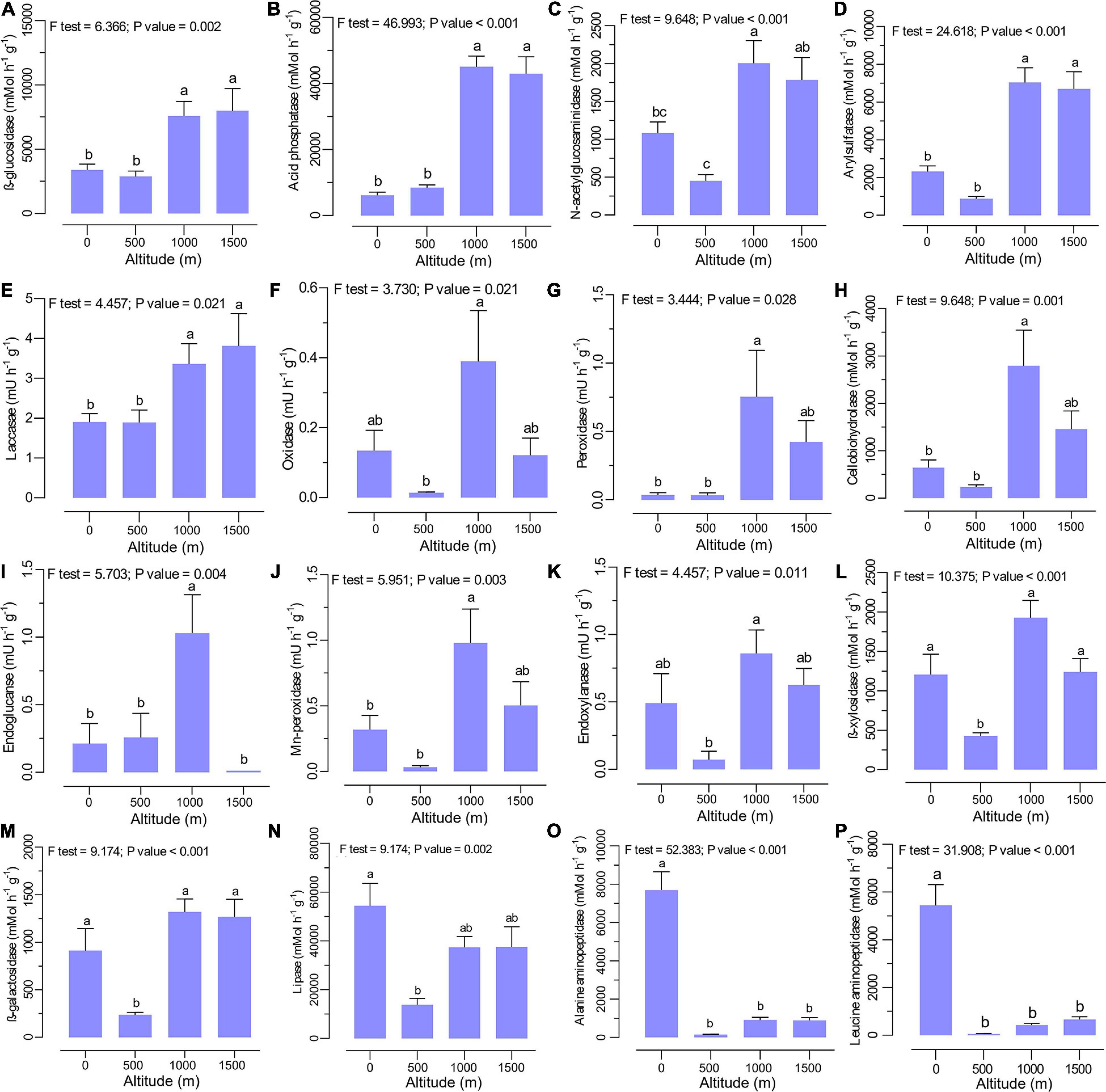
Figure 3. Soil extracellular enzyme activities such as (A) β-glucosidase, (B) acid phosphatase, (C) N-acetylglucosaminidase, (D) arylsulfatase, (E) laccase, (F) oxidase, (G) peroxidase, (H) cellobiohydrolase, (I) endoglucanase, (J) Mn-peroxidase, (K) endoxylanase, (L) β-xylosidase, (M) β-galactosidase, (N) lipase, (O) alanine aminopeptidase, and (P) leucine aminopeptidase at various altitudes. Different letters indicate a significant difference along the altitudinal gradient (ANOVA and Tukey HSD test; p < 0.05).
The Relationship Among All Litter and Soil Characteristics
Considering all litter and soil biological and biochemical properties and enzyme activities, samples were clustered separately by altitude in the PCA plot (Figures 4A,B). The two first axes of PCA covered ∼46% of the variation among samples collected from different altitudes. At the higher elevation (1,500 m), higher litter C, litter thickness, soil C, soil C/N ratio, protozoan density, fine-root biomass, MBC, and microbial entropy were associated with increased C stock and higher activities of β-glucosidase, acid phosphatase, N-acetylglucosaminidase, cellobiohydrolase, arylsulfatase, β-xylosidase, β-galactosidase, endoxylanase, peroxidase, and Mn-peroxidase enzymes (Figures 4A,B). Litter N, soil N, pH, BR, SIR, CAI, and MBN were aggregated at the positive portion of PC2 and were associated with an increased N stock and higher activities of lipase, alanine aminopeptidase, leucine aminopeptidase, β-xylosidase, and β-galactosidase enzymes at the 0-m-altitude level.
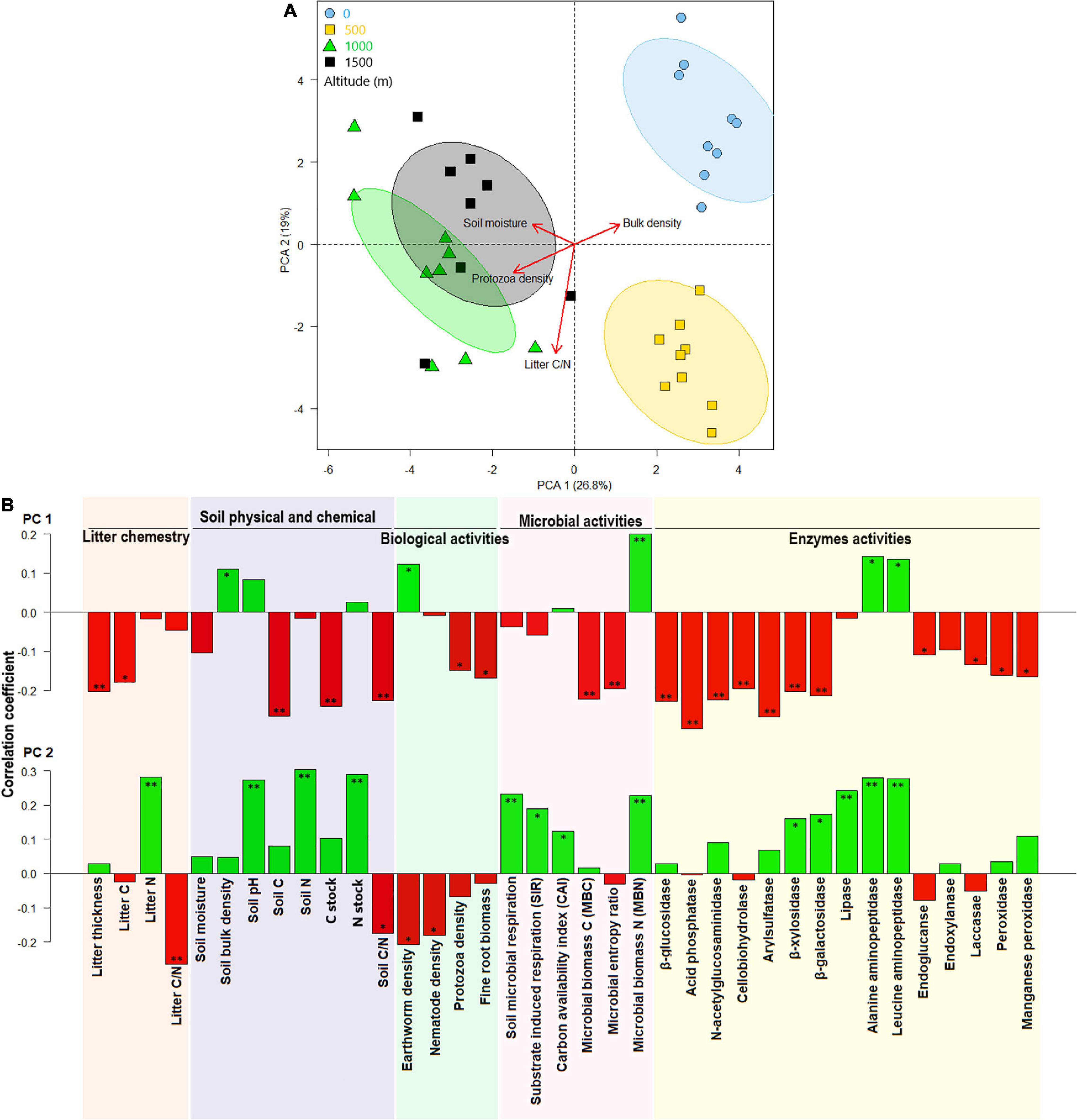
Figure 4. Principal component analysis (PCA) of chemical litter and soil properties and soil biological, biochemical, microbial, and enzyme activities at various altitudes (A) and their correlations with the first two canonical axes (B). * and ** represents correlation coefficients significant level at p < 0.05 and p < 0.01, respectively.
Discussion
The tree composition of forests covering the northern part of the Alborz Mountains changes with altitude (Talebi et al., 2013), a condition that is associated with altered soil C and nutrient stocks. The high C stocks at high altitudes can be due to the continuous accumulation of leaf litter and slower decomposition because of the lower temperatures at the higher elevation levels (Banday et al., 2019). The dominance of F. orientalis Lipsky at high elevations may likely contribute to C sequestration, resulting in a higher litter C/N ratio and higher soil C compared with other deciduous forest stands at lower altitudes (Talebi et al., 2013; Kooch and Bayranvand, 2017). Compared with other tree species found at low altitudes, the beech forest floor is more recalcitrant to decomposition, which could be due to its lower nutrient contents (Delgado-Baquerizo et al., 2017), higher C/N ratio (Bayranvand et al., 2017a), and high lignin content (Kooch and Bayranvand, 2017). The differences in soil C stocks in the two forest types thus originate from their different vegetation types, as the quantity, and quality of litter are crucial drivers that affect the soil microbial biomass and soil pH (Banday et al., 2019; Massaccesi et al., 2020). Low temperatures at higher altitudes are another factor contributing to the higher C stock, as in cooler climates, soils have higher OM due to a slow mineralization rate and a high forest-floor thickness (Gebrewahid et al., 2018; Banday et al., 2019). We found that plain mixed forest with species of P. persica, Q. castaneifolia, and C. betulus at low altitudes had higher litter quality (high N and low C/N), higher soil fertilities and N stocks than beech forest stands at higher altitudes. Krashevska et al. (2017) noted that a high initial litter N concentration and low C/N ratio as well as low lignin and high holo-cellulose concentrations are associated with high litter quality and promote rapid decomposition (Šnajdr et al., 2013).
Previous analyses by Šnajdr et al. (2013) and Feng et al. (2019) suggested that tree species determine the quality and quantity of resources available for soil microorganisms and enzyme functions. Our analyses revealed that higher enzyme activities are typical of higher altitudes under beech forests with high litter thickness, litter, and soil C and C/N ratios under appropriate SM. Šnajdr et al. (2008) studied the relationship between enzyme production and litter decomposition by fungal communities under different forest covers and noted that the decomposition of litter components, such as cellulose and lignin, is often attributed to cellulase enzyme activities. In agreement, enzymes, such as laccase, endoglucanase, β-glucosidase, and β-xylosidase, were reported to be produced in Fagus sylvatica forest by Lepista nuda fungi. Mn-peroxidase and laccase contribute to lignin degradation, which results in both depolymerization and partial mineralization (Valášková et al., 2007). In the present study, the activities of β-glucosidase, acid phosphatase, N-acetylglucosaminidase, arylsulfatase, and laccase were higher at higher elevations (1,000 to 1,500 m) in beech forests where litter thickness, C and C/N ratios, fine-root biomass, and SM were significantly higher (Banday et al., 2019). Increased C-dependent enzyme activity is likely due to a higher availability of aboveground litterfall (with higher C) and fine-root necromass to soil microorganisms (Feng et al., 2019). In addition to a lower decomposition rate, litter quality also decreased with altitude (Marian et al., 2019).
Increased soil enzyme activities could be associated with higher C accumulation since soil microbial biomass and heterotrophic respiration increase with plant density (Feng et al., 2019). Kang et al. (2009) also noted that higher activities of acid phosphatase and oxidase were dependent on the soil C content. In previous studies, it has been reported that the activities of β-glucosidase, cellobiohydrolase, acid phosphatase, and N-acetylglucosaminidase positively correlate with soil C (Štursová and Baldrian, 2011; Xu et al., 2020). Our findings are consistent with recent studies highlighting that higher enzyme activities are positively correlated with altitude and C, particularly under beech forests with high C sources and MBC (Štursová and Baldrian, 2011; Šnajdr et al., 2013; Xiao et al., 2019).
Principal component analysis showed that the higher soil C, moisture, SIR, BR, MBC, and litter thickness at the highest altitude (1,500 m; under beech forests) were associated with C stock, β-glucosidase, N-acetylglucosaminidase, arylsulfatase, β-xylosidase, β-galactosidase, endoxylanase, peroxidase, and Mn-peroxidase enzyme activities. Similarly, Cardelli et al. (2019) showed a positive correlation between C and the β-glucosidase, cellulase, xylosidase, and glucuronidase activities under F. sylvatica. These enzymes are involved in the degradation of cellulose, pectin and lignin, which are the main components of forest-floor plant residues (Cardelli et al., 2019). Previous studies have also reported a correlation of arylsulfatase and acid phosphatase enzyme activities with soil C content in deciduous forests (Baldrian et al., 2013; Kooch and Bayranvand, 2017). We also showed the highest enzyme and biological activities (i.e., nematode and protozoa density) under mixed beech–hornbeam–maple forest with high MBC and moderate litter/soil qualities and thickness. This could be due to a high C in beech litter/soil and a high litter decomposition of hornbeam and maple trees (Kooch and Bayranvand, 2019). Dehydrogenases and oxidases facilitate the degradation of lignin and thus improve C cycling through the release of nutrients from plant residues (Massaccesi et al., 2020). Enzymes such as urease and alanine aminopeptidase are involved in N cycling in terrestrial ecosystems (Šnajdr et al., 2013; Feng et al., 2019).
In this study, the activities of alanine and leucine aminopeptidase were significantly higher in the plain mixed forest with high litter/soil N and pH, while their activities declined at high altitudes and at low temperatures. pH and N are both important factors affecting enzyme activity (Šnajdr et al., 2013). A general decline in qCO2 and N with altitude could be attributed to a decreased ST and pH (Massaccesi et al., 2020). Štursová and Baldrian (2011) showed that the specific activity of leucine aminopeptidase increases with pH and N. A decrease in pH at high altitudes likely results from leaching of bases from the surface due to high precipitation or higher concentrations of H+ ions due to higher litter decomposition (Banday et al., 2019). The PCA showed that the soil N stock was associated with higher litter N, pH, qCO2, MBN, and activities of lipase, alanine and leucine aminopeptidase enzymes. We also noted a clear association of chitinase and leucine-aminopeptidase enzymes with high soil quality (high N and low C/N), consistent with their activities under high fresh OM (Cardelli et al., 2019).
Soil C accumulation is enhanced by low ST and acidic and anaerobic conditions, all of which inhibit the decomposition process (Banday et al., 2019; Devi and Sherpa, 2019). As an important driver of the microbial community and microbial C limitation (Walker et al., 2018), ST was significantly lower at high altitudes than at low altitudes (Cui et al., 2019). Increased soil C and MBC are known to positively correlate with high SM and low ST (Baldrian et al., 2010; Quan et al., 2019).
The C stock was positively correlated with SM, MBC, BR, and SIR but negatively correlated with higher levels of ST. Microbial entropy and MBC were enhanced at middle altitudes (1,000 m), where the dominant tree species were beech, hornbeam, and maple. The activities of soil organisms, including earthworms, protozoa, and nematodes, depend on soil conditions, such as pH, N, and available nutrients, which are affected by the rate of OM decomposition (Kooch and Bayranvand, 2017). Soil N and pH are the main factors affecting litter decomposition, which is the major source of the soil microbial qCO2 under high temperatures (Quan et al., 2019). Hornbeam forest had the highest litter quality, soil fertility, and soil organism activities compared with beech forest. In fact, soils under hornbeam had higher nutrient concentrations and pH that make conditions more favorable for microbial activities, whereas poor beech litter quality was due to a lower N concentration, higher C/N ratio, and higher levels of recalcitrant compounds associated with lower MBN and N sequestration (Kooch and Bayranvand, 2017). Among chemical variables, N had the strongest effect on the microbial biomass in litter and soil (Urbanová et al., 2015). Thus, the higher rate of N mineralization under hornbeam can be linked to the lower C/N ratio of its topsoil (Kooch and Bayranvand, 2019).
Conclusion
Microbial and enzyme activities can be used as sensitive indicators of soil function in response to different products of forest aboveground along altitudinal gradient, which helps to better understand the dynamics of both C and N cycling. Our findings indicate that N stock increases under plain mixed forest, which likely results from increased ST, tree diversity, litter N, MBN, pH, and leucine/alanine aminopeptidase enzymes. At higher altitudes, higher litter thickness, litter/soil C and C/N ratios, BR, SIR, and MBC and β-glucosidase, acid phosphatase, arylsulfatase, and laccase activities were associated with the lowest ST, highest SM, and thus greater C accumulation. In addition, our results showed that decreased litter/soil N and pH were associated with lower soil microbial and enzyme functions, which can be seen well at the 500-m altitude. Plain forests with high tree diversity, which produce high litter quality, had the highest N-dependent enzyme activity and soil fertilities, while mountainous forests with dominant beech trees had the potential to store more C and thus help to increase MBC. This study provides novel insights into different enzyme activities along altitude and improves our understanding of soil C and N cycling in forests.
Data Availability Statement
The original contributions presented in the study are included in the article/supplementary material, further inquiries can be directed to the corresponding authors.
Author Contributions
MA, GSJ, and JG are supervisors and advisors of the Ph.D. thesis of MB, respectively, and actively contributed to the design of the study. MB and PB performed the material preparation, data collection, and analysis. MB, PB, and MA analyzed and interpreted the soil properties, and enzyme activity data. MA contributed to identifying and classifying the vegetation cover. MB and JG analyzed the data. MB, PB, JG, and GSJ wrote the manuscript. All the authors contributed to the study conception and design, and read and approved the final manuscript.
Funding
This study was supported by the Tarbiat Modares University (Ph.D. thesis), the Iran National Science Foundation (No. 97024680, 2018), Tehran, Iran, and the Czech Science Foundation (18-26191S).
Conflict of Interest
The authors declare that the research was conducted in the absence of any commercial or financial relationships that could be construed as a potential conflict of interest.
Publisher’s Note
All claims expressed in this article are solely those of the authors and do not necessarily represent those of their affiliated organizations, or those of the publisher, the editors and the reviewers. Any product that may be evaluated in this article, or claim that may be made by its manufacturer, is not guaranteed or endorsed by the publisher.
Acknowledgments
We are grateful to E. Sharifi Moghadam, J. Eslamdoust, A. Khodadust, M. Mohammadi, A. Daryaei, and M.R. Soleimani for their tireless assistance during field work, and M. Štursová, H. Noraie, and M. Haghdoust for assistance with laboratory analyses. We would like to thank the members of the Laboratory of Environmental Microbiology of the Institute of Microbiology in Prague for their assistance in enzyme activity measurement.
References
Alef, K. (1995). “Estimating of soil respiration,” in Methods In Soil Microbiology and Biochemistry, eds K. Alef and P. Nannipieri (New York, NY: Academic), 464–470.
Allison, L. E. (1975). “Organic carbon,” in Part 2: Methods of Soil Analysis, ed. C. A. Black (Madison, WI: American Society of Agronomy), 1367–1378.
Anderson, T.-H., and Domsch, K. (1990). Application of eco-physiological quotients (qCO2 and qD) on microbial biomasses from soils of different cropping histories. Soil Biol. Biochem. 22, 251–255. doi: 10.1016/0038-0717(90)90094-g
Baldrian, P. (2009). Microbial enzyme-catalyzed processes in soils and their analysis. Plant Soil Environ. 55, 370–378. doi: 10.17221/134/2009-pse
Baldrian, P., Merhautová, V., Petránková, M., Cajthaml, T., and Šnajdr, J. (2010). Distribution of microbial biomass and activity of extracellular enzymes in a hardwood forest soil reflect soil moisture content. Appl. Soil Ecol. 46, 177–182. doi: 10.1016/j.apsoil.2010.08.013
Baldrian, P., Šnajdr, J., Merhautová, V., Dobiášová, P., Cajthaml, T., and Valášková, V. (2013). Responses of the extracellular enzyme activities in hardwood forest to soil temperature and seasonality and the potential effects of climate change. Soil Biol. Biochem. 56, 60–68. doi: 10.1016/j.soilbio.2012.01.020
Baldrian, P., and Štursová, M. (2011). “Enzymes in forest soils,” in Soil Enzymology, eds G. Shukla and A. Varma (Berlin: Springer-Verlag), 61–73. doi: 10.1007/978-3-642-14225-3_4
Banday, M., Bhardwaj, D. R., and Pala, N. A. (2019). Influence of forest type, altitude and NDVI on soil properties in forests of North Western Himalaya, India. Acta Ecol. Sin. 39, 50–55. doi: 10.1016/j.chnaes.2018.06.001
Bayranvand, M., Akbarinia, M., Salehi Jouzani, G., Gharechahi, J., and Alberti, G. (2021a). Dynamics of humus forms and soil characteristics along a forest altitudinal gradient in Hyrcanian forest. iForest-Bioge. Fore 14, 26–33. doi: 10.3832/ifor3444-013
Bayranvand, M., Akbarinia, M., Salehi Jouzani, G., Gharechahi, J., Kooch, Y., and Baldrian, P. (2021b). Composition of soil bacterial and fungal communities in relation to vegetation composition and soil characteristics along an altitudinal gradient. FEMS Microbiol. Ecol. 97:fiaa201.
Bayranvand, M., Kooch, Y., Hosseini, S. M., and Alberti, G. (2017a). Humus forms in relation to altitude and forest type in the Northern mountainous regions of Iran. Forest Ecol. Manag. 385, 78–86. doi: 10.1016/j.foreco.2016.11.035
Bayranvand, M., Kooch, Y., and Rey, A. (2017b). Earthworm population and microbial activity temporal dynamics in a Caspian Hyrcanian mixed forest. Eur. J. For. Res. 136, 447–456. doi: 10.1007/s10342-017-1044-5
Bello, C., Galetti, M., Pizo, M. A., Magnago, L. F. S., Rocha, M. F., Lima, R. A., et al. (2015). Defaunation affects carbon storage in tropical forests. Sci. Adv. 1:e1501105. doi: 10.1126/sciadv.1501105
Bremner, J. M., Mulvaney, C. S., and Madison, W. I. (1982). “Nitrogen,” in Methods of Soil Analysis, Second Edn, eds A. L. Page, R. H. Miller, and R. R. Keeney (Madison, WI: American Society of Agronomy), 595–624.
Brookes, P., Landman, A., Pruden, G., and Jenkinson, D. (1985). Chloroform fumigation and the release of soil nitrogen: a rapid direct extraction method to measure microbial biomass nitrogen in soil. Soil Biol. Biochem. 17, 837–842. doi: 10.1016/0038-0717(85)90144-0
Cardelli, V., De Feudis, M., Fornasier, F., Massaccesi, L., Cocco, S., Agnelli, A., et al. (2019). Changes of topsoil under Fagus sylvatica along a small latitudinal-altitudinal gradient. Geoderma 344, 164–178. doi: 10.1016/j.geoderma.2019.01.043
Cheng, W., Zhang, Q., Coleman, D. C., Carroll, C. R., and Hoffman, C. A. (1996). Is available carbon limiting microbial respiration in the rhizosphere? Soil Biol. Biochem. 28, 1283–1288. doi: 10.1016/s0038-0717(96)00138-1
Cui, Y., Bing, H., Fang, L., Jiang, M., Shen, G., Yu, J., et al. (2019). Extracellular enzyme stoichiometry reveals the carbon and phosphorus limitations of microbial metabolisms in the rhizosphere and bulk soils in alpine ecosystems. Plant Soil 458, 7–20. doi: 10.1007/s11104-019-04159-x
D’Alò, F., Odriozola, I., Baldrian, P., Zucconi, L., Ripa, C., Cannone, N., et al. (2021). Microbial activity in alpine soils under climate change. Sci. Total Environ. 783:147012. doi: 10.1016/j.scitotenv.2021.147012
Delgado-Baquerizo, M., Eldridge, D. J., Maestre, F. T., Karunaratne, S. B., Trivedi, P., Reich, P. B., et al. (2017). Climate legacies drive global soil carbon stocks in terrestrial ecosystems. Sci. Adv. 3:e1602008. doi: 10.1126/sciadv.1602008
Devi, S. B., and Sherpa, S. S. S. S. (2019). Soil carbon and nitrogen stocks along the altitudinal gradient of the Darjeeling Himalayas, India. Environ. Monit. Assess. 191:361.
Feng, C., Ma, Y., Jin, X., Wang, Z., Ma, Y., Fu, S., et al. (2019). Soil enzyme activities increase following restoration of degraded subtropical forests. Geoderma 351, 180–187. doi: 10.1016/j.geoderma.2019.05.006
Frouz, J. (2018). Effects of soil macro-and mesofauna on litter decomposition and soil organic matter stabilization. Geoderma 332, 161–172. doi: 10.1016/j.geoderma.2017.08.039
García-Palacios, P., Maestre, F. T., Kattge, J., and Wall, D. H. (2013). Climate and litter quality differently modulate the effects of soil fauna on litter decomposition across biomes. Ecol. Lett. 16, 1045–1053. doi: 10.1111/ele.12137
Gebrewahid, Y., Gebre-Egziabhier, T.-B., Teka, K., and Birhane, E. (2018). Carbon stock potential of scattered trees on farmland along an altitudinal gradient in Tigray, Northern Ethiopia. Environ. Process. 7:40.
Kang, H., Kang, S., and Lee, D. (2009). Variations of soil enzyme activities in a temperate forest soil. Ecol. Res. 24, 1137–1143. doi: 10.1007/s11284-009-0594-5
Karger, D. N., Conrad, O., Böhner, J., Kawohl, T., Kreft, H., Soria-Auza, R. W., et al. (2017). Climatologist at high resolution for the earth’s land surface areas. Sci. Data 4:170122.
Khaleghi, P., Abasi, H., Hosani, S., Frohar, M., and Ghelichnian, H. (1997). Caspian Forests Profile, Waz Research Forest. Tehran: Research Institute for Forests and Rangelands, Ministry of Jihad-e-Production Department of Education and Research.
Kooch, Y., and Bayranvand, M. (2017). Composition of tree species can mediate spatial variability of C and N cycles in mixed beech forests. Forest Ecol. Manag. 401, 55–64. doi: 10.1016/j.foreco.2017.07.001
Kooch, Y., and Bayranvand, M. (2019). Labile soil organic matter changes related to forest floor quality of tree species mixtures in Oriental beech forests. Ecol. Indic. 107:105598. doi: 10.1016/j.ecolind.2019.105598
Kotas, P., Šantrůčková, H., Elster, J., and Kaštovská, E. (2018). Soil microbial biomass, activity and community composition along altitudinal gradients in the High Arctic (Billefjorden, Svalbard). Biogeosciences 15:1879. doi: 10.5194/bg-15-1879-2018
Krashevska, V., Sandmann, D., Marian, F., Maraun, M., and Scheu, S. (2017). Leaf litter chemistry drives the structure and composition of soil testate amoeba communities in a tropical montane rainforest of the Ecuadorian Andes. Microb. Ecol. 74, 681–690. doi: 10.1007/s00248-017-0980-4
Liang, W. J., Lou, Y. L., Li, Q., Zhong, S., and Zhang, X. K. (2009). Nematode faunal response to longterm application of nitrogen fertilizer and organic manure in Northeast China. Soil Biol. Biochem. 41, 883–890. doi: 10.1016/j.soilbio.2008.06.018
Lladó, S., López-Mondéjar, R., and Baldrian, P. (2017). Forest soil bacteria: diversity, involvement in ecosystem processes, and response to global change. Microbiol. Mol. Biol. Rev. 81:e00063-16.
Marian, F., Brown, L., Sandmann, D., Maraun, M., and Scheu, S. (2019). Roots, mycorrhizal fungi and altitude as determinants of litter decomposition and soil animal communities in tropical montane rainforests. Plant Soil 438, 1–18. doi: 10.1007/s11104-019-03999-x
Massaccesi, L., Feudis, M. D., Leccese, A., and Agnelli, A. (2020). Altitude and vegetation affect soil organic carbon, basal respiration and microbial biomass in apennine forest soils. Forests 11:710. doi: 10.3390/f11060710
Mayzlish, E., and Steinberger, Y. (2004). Effects of chemical inhibitors on soil protozoan dynamics in a desert ecosystem. Biol. Fert. Soils 39, 415–421. doi: 10.1007/s00374-004-0723-9
Meng, C., Tian, D., Zeng, H., Li, Z., Chen, H. Y., and Niu, S. (2020). Global meta-analysis on the responses of soil extracellular enzyme activities to warming. Sci. Total Environ. 705:135992. doi: 10.1016/j.scitotenv.2019.135992
Ndossi, E. M., Becker, J. N., Hemp, A., Dippold, M. A., Kuzyakov, Y., and Razavi, B. S. (2020). Effects of land use and elevation on the functional characteristics of soil enzymes at Mt. Kilimanjaro. Eur. J. Soil Biol. 97:103167. doi: 10.1016/j.ejsobi.2020.103167
Neatrour, M. A., Jones, R. H., and Golladay, S. W. (2005). Correlations between soil nutrient availability and fine-root biomass at two spatial scales in forested wetlands with contrasting hydrological regimes. Can. J. For. Res. 35, 2934–2941. doi: 10.1139/x05-217
Nottingham, A. T., Meir, P., Velasquez, E., and Turner, B. L. (2020). Soil carbon loss by experimental warming in a tropical forest. Nature 584, 234–237. doi: 10.1038/s41586-020-2566-4
Nottingham, A. T., Whitaker, J., Ostle, N. J., Bardgett, R. D., McNamara, N. P., Fierer, N., et al. (2019). Microbial responses to warming enhance soil carbon loss following translocation across a tropical forest elevation gradient. Ecol. Lett. 22, 1889–1899. doi: 10.1111/ele.13379
Quan, Q., Tian, D., Luo, Y., Zhang, F., Crowther, T. W., Zhu, K., et al. (2019). Water scaling of ecosystem carbon cycle feedback to climate warming. Sci. Adv. 5:eaav1131. doi: 10.1126/sciadv.aav1131
R Core Team (2016). R: A Language and Environment for Statistical Computing. Vienna: R Foundation for Statistical Computing.
Razanamalala, K., Razafimbelo, T., Maron, P.-A., Ranjard, L., Chemidlin, N., Lelièvre, M., et al. (2018). Soil microbial diversity drives the priming effect along climate gradients: a case study in Madagascar. ISME J. 12, 451–462. doi: 10.1038/ismej.2017.178
Sierra, J., and Causeret, F. (2018). Changes in soil carbon inputs and outputs along a tropical altitudinal gradient of volcanic soils under intensive agriculture. Geoderma 320, 95–104. doi: 10.1016/j.geoderma.2018.01.025
Šnajdr, J., Dobiášová, P., Urbanová, M., Petránková, M., Cajthaml, T., Frouz, J., et al. (2013). Dominant trees affect microbial community composition and activity in post-mining afforested soils. Soil Biol. Biochem. 56, 105–115. doi: 10.1016/j.soilbio.2012.05.004
Šnajdr, J., Valášková, V., Merhautová, V., Cajthaml, T., and Baldrian, P. (2008). Activity and spatial distribution of lignocellulose-degrading enzymes during forest soil colonization by saprotrophic basidiomycetes. Enzyme Microb. Technol. 43, 186–192. doi: 10.1016/j.enzmictec.2007.11.008
Štursová, M., and Baldrian, P. (2011). Effects of soil properties and management on the activity of soil organic matter transforming enzymes and the quantification of soil-bound and free activity. Plant Soil 338, 99–110. doi: 10.1007/s11104-010-0296-3
Talebi, K. S., Sajedi, T., and Pourhashemi, M. (2013). Forests of Iran: A Treasure From the Past, a Hope for the Future. Berlin: Springer Science & Business Media.
Urbanová, M., Šnajdr, J., and Baldrian, P. (2015). Composition of fungal and bacterial communities in forest litter and soil is largely determined by dominant trees. Soil Biol. Biochem. 84, 53–64. doi: 10.1016/j.soilbio.2015.02.011
Valášková, V., Šnajdr, J., Bittner, B., Cajthaml, T., Merhautová, V., Hofrichter, M., et al. (2007). Production of lignocellulose-degrading enzymes and degradation of leaf litter by saprotrophic basidiomycetes isolated from a Quercus petraea forest. Soil Biol. Biochem. 39, 2651–2660. doi: 10.1016/j.soilbio.2007.05.023
Walker, T. W., Kaiser, C., Strasser, F., Herbold, C. W., Leblans, N. I., Woebken, D., et al. (2018). Microbial temperature sensitivity and biomass change explain soil carbon loss with warming. Nat. Clim. Change 8, 885–889. doi: 10.1038/s41558-018-0259-x
Xiao, L., Li, P., Shi, P., and Liu, Y. (2019). Soil nutrient stoichiometries and enzymatic activities along an elevational gradient in the dry-hot valley region of southwestern China. Arch. Agron. Soil Sci. 65, 322–333. doi: 10.1080/03650340.2018.1502882
Keywords: forest soils, litter quality, enzyme activity, microbial entropy, N stock
Citation: Bayranvand M, Akbarinia M, Salehi Jouzani G, Gharechahi J and Baldrian P (2021) Distribution of Soil Extracellular Enzymatic, Microbial, and Biological Functions in the C and N-Cycle Pathways Along a Forest Altitudinal Gradient. Front. Microbiol. 12:660603. doi: 10.3389/fmicb.2021.660603
Received: 29 January 2021; Accepted: 23 July 2021;
Published: 03 September 2021.
Edited by:
Siu Mui Tsai, University of São Paulo, BrazilReviewed by:
Stefanie Schulz, Helmholtz-Gemeinschaft Deutscher Forschungszentren (HZ), GermanyCaio Augusto Yoshiura, University of São Paulo, Brazil
Copyright © 2021 Bayranvand, Akbarinia, Salehi Jouzani, Gharechahi and Baldrian. This is an open-access article distributed under the terms of the Creative Commons Attribution License (CC BY). The use, distribution or reproduction in other forums is permitted, provided the original author(s) and the copyright owner(s) are credited and that the original publication in this journal is cited, in accordance with accepted academic practice. No use, distribution or reproduction is permitted which does not comply with these terms.
*Correspondence: Mohammad Bayranvand, bS5iYXlyYW52YW5kQGdtYWlsLmNvbQ==; Petr Baldrian, YmFsZHJpYW5AYmlvbWVkLmNhcy5jeg==