- 1Department of Biology, Geology, Physics, and Inorganic Chemistry, Universidad Rey Juan Carlos, Madrid, Spain
- 2Unidad de Bioinformática, Instituto de Salud Carlos III, Madrid, Spain
- 3Área de Electromagnetismo, Universidad Rey Juan Carlos, Madrid, Spain
- 4Department of Plant Biology, Rutgers University, New Brunswick, NJ, United States
Seed microbiota is becoming an emergent area of research. Host plant microbial diversity is increasingly well described, yet relatively little is known about the stressors driving plant endomicrobiota at the metaorganism level. The present work examines the role of horizontal and vertical transmission of bacterial microbiota in response to abiotic stress generated by arsenic. Horizontal transmission is achieved by bioaugmentation with the endophyte Rhodococcus rhodochrous, while vertical transmission comes via maternal inheritance from seeds. To achieve this goal, all experiments were conducted with two Jasione species. J. montana is tolerant to arsenic (As), whereas J. sessiliflora, being phylogenetically close to J. montana, was not previously described as As tolerant. The Jasione core bacterial endophytes are composed of genera Pseudomonas, Ralstonia, Undibacterium, Cutibacterium, and Kocuria and family Comamanadaceae across different environmental conditions. All these operational taxonomic units (OTUs) coexisted from seeds to the development of the seedling, independently of As stress, or bioaugmentation treatment and Jasione species. R. rhodochrous colonized efficiently both species, driving the endomicrobiota structure of Jasione with a stronger effect than As stress. Despite the fact that most of the OTUs identified inside Jasione seeds and seedlings belonged to rare microbiota, they represent a large bacterial reservoir offering important physiological and ecological traits to the host. Jasione traits co-regulated with R. rhodochrous, and the associated microbiota improved the host response to As stress. NGS-Illumina tools provided further knowledge about the ecological and functional roles of plant endophytes.
Introduction
Microorganisms with 3 billion years of evolution have acquired a wide range of metabolic activities, being able to colonize almost all ecological niches including plants and animals. Plant microbiomes can modify the genomic and metabolic functions of their hosts, improving functions and stress tolerance (Cordovez et al., 2019). In this sense, plants are constituted by the genome of the plant and its associated microbiota, which establish an inter- and intracellular association (Thijs et al., 2016; Cordovez et al., 2019). Plants have a set of microorganisms necessary for their survival considered as core or obligate microbiota (Hardoim et al., 2008; Vandenkoornhuyse et al., 2015; Trivedi et al., 2020), generally obtained by a vertical maternal route (Truyens et al., 2015; Klaedtke et al., 2016; Moreira et al., 2021). Another set of microorganisms is considered accessory or facultative, providing mutualistic or antagonistic interactions to the plant under certain environmental conditions, generally obtained by horizontal transmission (Clay, 1998; Hardoim et al., 2015). Among microbiota associated with host plants, endophytes are “all living organisms inside plant tissues” (De Barry, 1866), but since its origin, this term has evolved over time to become more complex. Some authors have considered this term to refer to the location of the microorganisms (Schulz and Boyle, 2005; Hardoim et al., 2015), but others have included the nature of the interaction with the plant (Perotti, 1926; Wennstrom, 1994; Verma and White, 2019). The lack of consensus on this term may lead to controversy.
The same plant species may recruit different endophyte assemblages depending on the environment. The soil reservoir of endophytes will offer a wide range of physiological functions to the host plant providing growth promotion (Compant et al., 2021), more protection against pathogens (Matsumoto et al., 2021) and herbivory (Verma et al., 2017), and a better adaptation to environmental conditions and abiotic stress (Rodríguez et al., 2008).
Arsenic is considered one of the major stresses to plants (Kumar and Verma, 2018) and is a highly toxic metalloid from anthropogenic and natural sources. Plant exposure to As, even at low concentrations, could trigger negative consequences in morphology, physiology, and biochemistry (Moreno-Jiménez et al., 2008; Abbas et al., 2018). However, some plants are able to complete their life cycles in environments highly contaminated with As (García-Salgado et al., 2011; Guterres et al., 2019; Calabrese and Agathokleous, 2021).
Many studies examine host plant microbial diversity, but studies on the mechanisms of how stressors drive plant endomicrobiota at the metaorganism level are lacking. We hypothesized that endophytes play an important role on host traits against environmental stress. To test this hypothesis, the present work addresses the role of horizontal and vertical transmission of bacteria in two species of Jasione against As stress. The choice of this host plant was inclined toward Jasione montana L. because it is tolerant to arsenic (Gutiérrez-Ginés et al., 2015; Molina et al., 2021) and Jasione sessiliflora Boiss. & Reut., which is phylogenetically close (sister group) to J. montana (Pérez-Espona et al., 2005) and has not been previously described as As tolerant. For bioaugmentation experiments, we used Rhodococcus rhodochrous, an As-resistant and plant growth-promoting bacteria endophyte from J. montana collected from highly As-contaminated soils (Molina et al., 2021).
Materials and Methods
Jasione montana and Jasione sessiliflora
Seeds of J. montana were collected by Pauthier and Lang, 2010 in Saint-Georges (France). The area was located on a road edge in acid rocky ground at 787 m altitude in the Garabit viaduct (N 44° 58′ 21.9″, E 3° 10′ 27.8″). Seeds of J. sessiliflora were collected by Pauthier, 1996 in siliceous soils at 650 m altitude in Villefort, Lozère (N 44° 29′ 7″, E 3° 55’25″). Seeds from both species were given to us by the germplasm bank of the French National Museum of Natural History. Seeds were stored in airtight containers at low temperatures (−18°C) after desiccation in desiccation chambers (15% relative humidity).
Bacteria for Bioaugmentation
Rhodococcus rhodochrous (Nocardiaceae) is an endophyte isolated from an adult plant of J. montana collected in a highly polluted area in the Mónica mine, Bustarviejo (19 × 103 mg kg–1). R. rhodococcus has been previously identified (Molina et al., 2021) and shows resistance up to 450 mM of arsenate a, produces indole acetic acid (IAA), and inhibits 40% of the growth of Alternaria sp., which is a potential pathogenic ascomycete fungus (Soares et al., 2016) also isolated from Jasione seeds (Molina et al., 2021). Due to these characteristics, this bacterium has been used for the study the horizontal transmission of bacteria.
Experimental Design
The Role of Endomicrobiota for Plant Development
Seeds were subjected to two procedures (Figure 1). Sterilization (S) and No sterilization (no S). For No S, seeds were washed to remove only bacterial and fungal epiphytes with 2% (v/v) sodium hypochlorite during 5 min with slow rotation and rinsed three times with milliQ water (Molina et al., 2021). For the S procedure, seeds were washed with streptomycin sulfate (100 μg/ml, Sigma-Aldrich, MO, United States) during 24 h in continuous agitation (Molina et al., 2021) to obtain a gnotobiotic organism.
Each treatment for each plant was replicated eight times; each replicate consisted of 25–30 seeds per Petri dish in a basal germination medium (0.7% agarose, Sigma-Aldrich). Dishes were incubated in a cultivation chamber (Selecta HOTCOLD-GL, Refrigerated Precision Cabinet) for 30 days at 24°C and 12-h photoperiod cycles with continuous illumination. Dishes were periodically changed to eliminate any fungal contamination. Germination was indicated when seeds showed hypocotyl and/or radicle. Complete development of healthy seedlings was indicated when seedlings had, at least, hypocotyl, main root or radicle, and cotyledons.
The Role of Vertical and Horizontal Transmission of Microbiota in Response to as Stress
After 30 days of incubation, we applied four treatments to each of the seeds from C treatment (Figure 1). As treatment (addition of 125μM As[V]; As), bioaugmentation treatment (addition of R. rhodochrous; BA), combination treatment (As + BAs), and control treatment (no As + no BA). All experiments were carried out under sterile conditions avoiding any contamination by bacteria except for the controlled colonization by R. rhodochrous. All seedlings were planted in autoclaved individual tubes with Murashige & Skoog nutrient medium (Sigma Aldrich). Tubes were cultivated in the same cultivation chamber to have the same temperature and light conditions during germination experiments. We rotated tubes every 2 to 3 days to avoid position effects during the 45 days of incubation. There were a total of 25 seedling per treatment (Figure 1).
The number of leaves and roots and the length of stem and main root were analyzed with the software ImageJ1. The main root was measured from the first lateral branch to the root cap. The shoot was measured from the first branch of the root to the apical shoot tip. A plant was considered dead when, visibly, it did not have photosynthetic pigments.
Identification of Endophytic Bacteria From Seeds and Seedlings of J. montana and J. sessiliflora
Seeds (50 seeds from each plant species) and seedlings (four from each plant species) were washed with hypochlorite 2% (v/v) to remove epiphytic microorganisms as we mentioned above. We used DNEASY Plant Kit (Qiagen, Hilden, Germany), and for the seeds, we used DNA Isolation Kit (MO BIO Technologies, CA, United States). Quant-IT PicoGreen reagent (Thermo Fisher Scientific, MA, United States) was used to measure the DNA concentration of each sample. DNA samples were used to amplify the V5–V6 region of the 16S rRNA gene using the protocol in Molina et al. (2021). Also, blocking primers were used in order not to amplify DNA from mitochondria and chloroplast (Arenz et al., 2015). PCR products had a length of approximately 421 bp. PCR products with the extension tails (PE1-CS1 and PE2-BC-CS2) allowed the addition of 10 nucleotide barcodes and a specific Illumina sequence in the second low-cycle number PCR, using a Fluidigm protocol:
V5-F: ACACTGACGACATGGTTCTACAGGATTAGATA CCCTGGTA
V6-R: TACGGTAGCAGAGACTTGGTCTCGACRRCCAT GCANCACCT
PE1-CS1: 5′-AATGATACGGCGACCACCGAGATCTACA CTGACGACATGGTTCTACA-3′
PE2-BC-CS2: 5′-CAAGCAGAAGACGGCATACGAGAT-[10 nucleotides barcode]-TACGGTAGCAGAGACTT GGTCT-3′.
DNA samples were sequenced with Illumina MiSeq (2 × 301). Pass filter reads were prepared using standard procedures (MiSeq Real Time Analysis, Illumina). Sequences were de-noised, merged, and clustered into operational taxonomic units (OTUs) using DADA2 (Callahan et al., 2016) in Qiime 2 version 2020.6 (Boylen et al., 2019). OTUs were aligned and classified with a Qiime2 q2-feature-classifier with a pre-trained Naïve Bayes classifier on the Silva 99% OTUs full-length sequences (version 138)2. The number of sequences per sample was rarefied to ensure even sampling (Suplementary Figure 1). Taxonomic assignments were made by comparison with their homolog in GenBank using BLAST (Altschul et al., 1997).
Diversity indices were then calculated on relative abundance OTU tables. Seeds and plant taxonomic richness were expressed as the number of OTUs. The diversity was measured by Simpson’s diversity index (1-Ds), while evenness was measured by Pielou’s evenness index (J′).
Statistical Analysis
All data were analyzed with R v.3.6.0 (R Core Team, 2019). We compared germination and plant development among treatments by generalized linear mixed models (GLMMs), including species and treatment as a fixed factor and dish and seed as random factors. Random factors were not significant; therefore, we analyzed germination, plant development, and number of leaves and roots with generalized linear models (GLMs). Data presented heteroscedasticity; therefore, GLMs were estimated with a Poisson distribution for germination, plant development, and number of leaves and roots, but the length of stem and main root was estimated with Gaussian distribution. The best model selection was based on the Akaike’s information criterion (AIC) corrected for small sample size (AICc). Akaike weights (Burnham and Anderson, 2002) were calculated for the final model, including all significant variables, and then for models in which the variables were sequentially excluded.
The similarity among whole samples was estimated with the index similarity coefficient using the Bray–Curtis distance with the function vegdist in the R package Vegan (Oksanen, 2016). The dendrogram was generated using the unweighted pair group with arithmetic means (UPGMA) using the function hclust of the stats package in R (version 1.2.5033). The overlapping areas of the Venn diagrams are used to represent shared OTUs between all treatments (BA, no BA, As, and no As) and species (J. montana and J. sessiliflora).
Results
The Role of Jasione Endomicrobiota in Modulating Plant Development
Jasione montana (Figure 2A and Table 1) had a significant higher percentage of germination (80.1% ± 2.5%) and healthy plant development (44.7% ± 9.4%) than J. sessiliflora (60.9% ± 2.3% and 34.7% ± 8.0%, respectively). The role of Jasione endomicrobiota was found to be important for plant development with significantly higher scores (Figure 2B and Table 1) observed in the holobiotic (plant with bacteria endomicrobiota; 71.4% ± 3.0%) than in the gnotobiotic model (6.2% ± 1.5%).
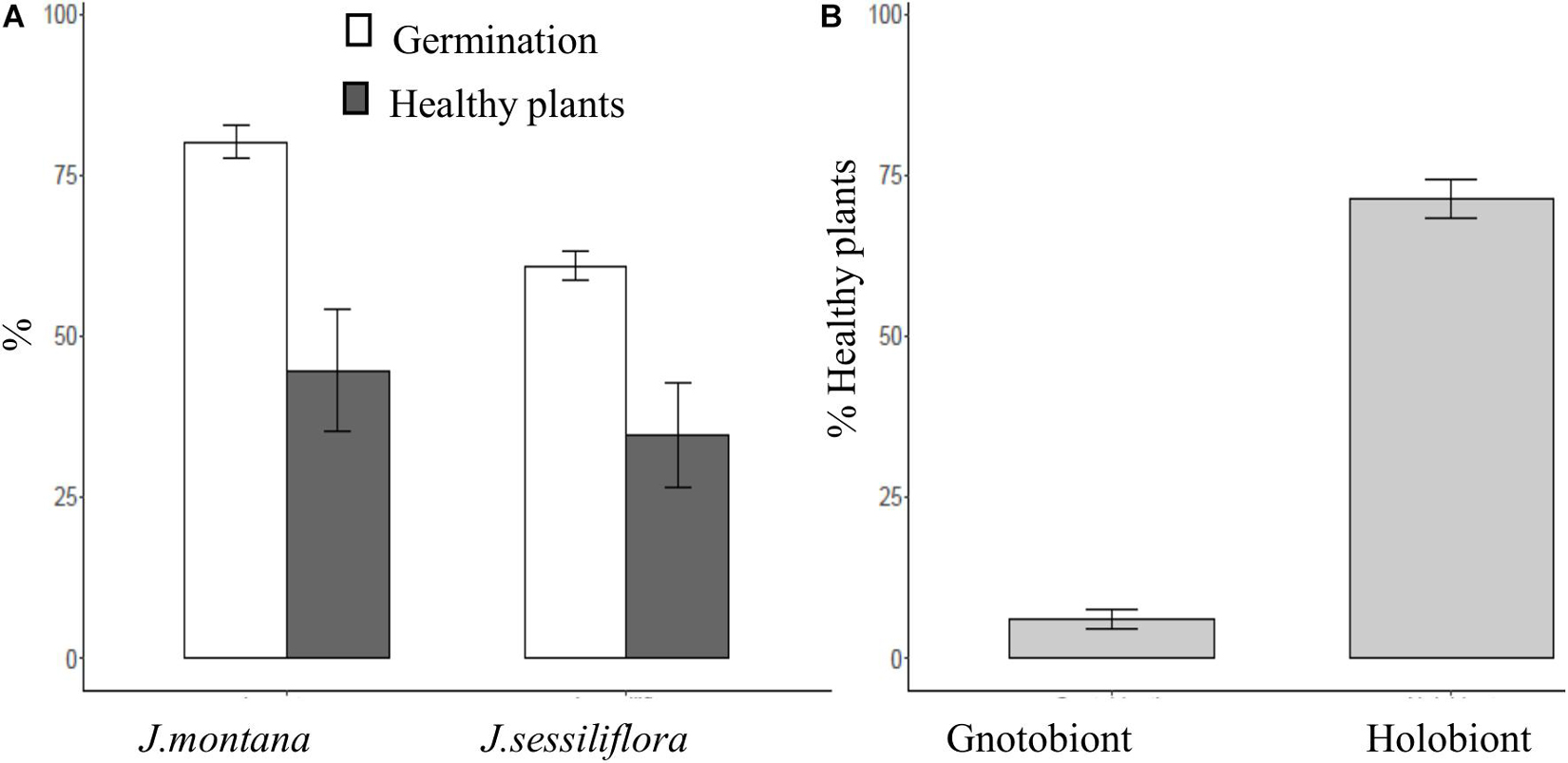
Figure 2. (A) Percentage of germination and healthy plants of J. montana and J. sessiliflora (white and gray, respectively). (B) Percentage of healthy plants in gnotobiotic (seeds without endophytes) and holobiotic (seeds with endophytes) models. All statistical parameters are shown in Table 2. The error bars are standard error.
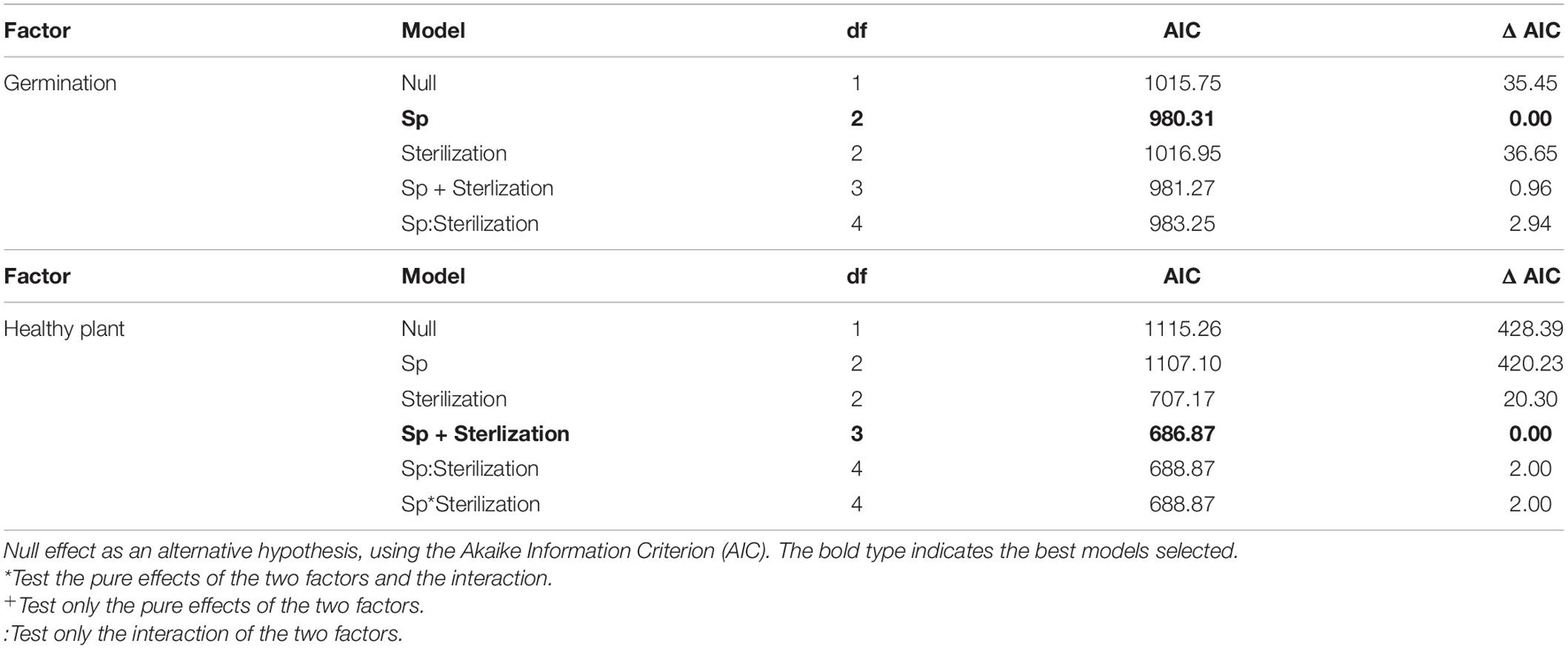
Table 1. Generalized linear model analyses to test the effect of species (spp.; J. montana and J. sessiliflora) and sterilization treatment (no S = holobiotic vs. S = gnotobiotic) and interactions of both (sp and sterilization) on the germination and plant development of healthy plants.
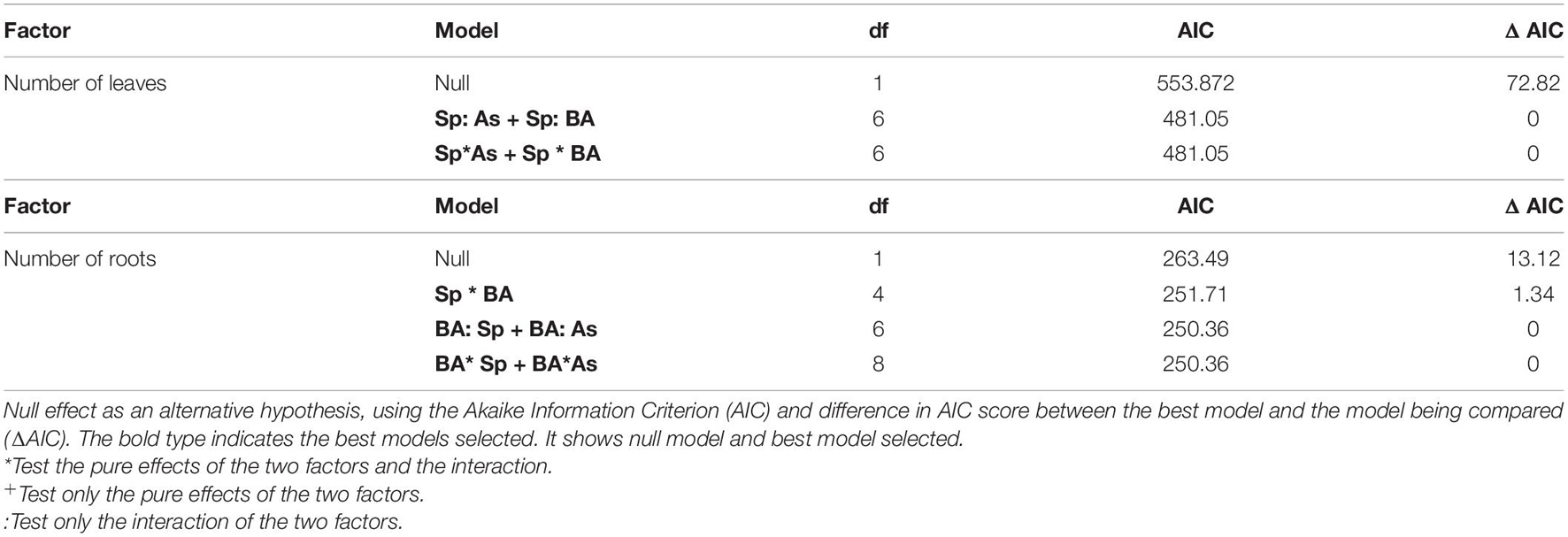
Table 2. Generalized linear model analyses to test the effect of species (J. montana and J. sessiliflora), As, and BA (R. rhodochrous) on the number of plant leaves and lateral roots.
Effects of Arsenic and BA on Jasione Develpment
After 45 days of incubation, the treatment BA (addition of R. rhodochrous) did not show any effect on the number of leaves in J. sessiliflora (7.6 ± 1.2 and 6.5 ± 1.4 leaves without BA and with BA, respectively) whereas it had a positive effect (4.6 ± 1.0 and 8.4 ± 0.8 leaves without BA and with BA, respectively) in J. montana (Figure 3A and Table 2). The opposite pattern was observed on the number of lateral roots (LR). The addition of R. rhodochrous (treatment BA) did not show any effect in J. montana (1.2 ± 0.5 and 1.6 ± 0.4 LR without BA and with BA, respectively) whereas J. sessiliflora (0.9 ± 0.3 and 0.3 ± 0.2 LR without BA and with BA, respectively) showed a significantly reduced number of leaves (Figure 3B and Table 2). Accordingly, As did not show any significant effect on the number of leaves (7.1 ± 1.0 and 6.6 ± 0.9 leaves without As and with As, respectively) of J. montana (Figure 4 and Table 2) whereas J. sessiliflora showed a significantly reduced number of leaves under As stress (9.3 ± 1.2 and 3.1 ± 0.6 leaves without As and with As, respectively). However, the interaction of As and BA treatments was significant (Table 2). The addition of R. rhodochrous (BA treatment) increased the number of LR under As stress (0.7 ± 0.3 and 1.6 ± 0.6 LR without BA and with BA, respectively) whereas it did not show any effect (1.1 ± 0.3 and 0.7 ± 0.2 LR without BA and with BA, respectively) under conditions without stress (Figure 5 and Table 2). The lengths of the roots and shoots were not significantly changed in any treatment.
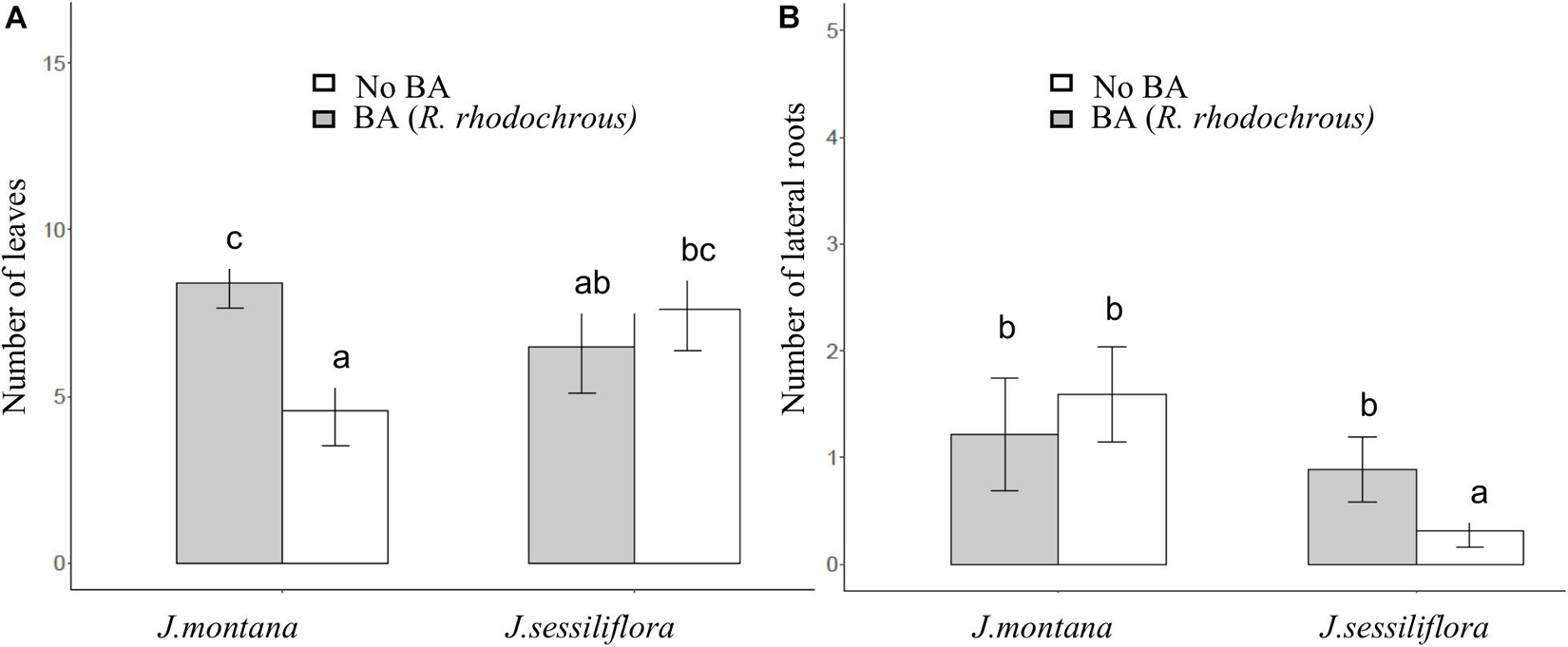
Figure 3. The effect of BA treatment and species of Jasione (J. montana and J. sessiliflora) on the number of leaves (A) and the number of lateral roots (B). All statistical parameters are shown in Table 2. The error bars are standard error. Letters indicate the different group of means in SNK tests at p < 0.05.
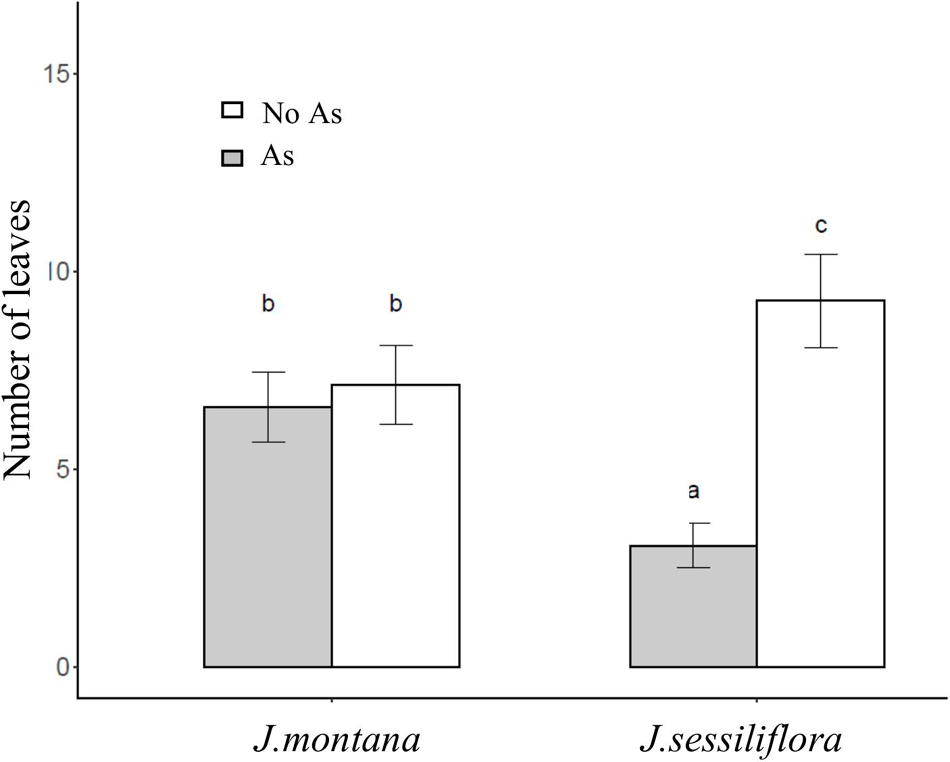
Figure 4. The effect of the As treatment and Jasione sp. on the number of leaves. All statistical parameters are shown in Table 2. The error bars are standard error. Letters indicate the different group of means in SNK tests at p < 0.05.
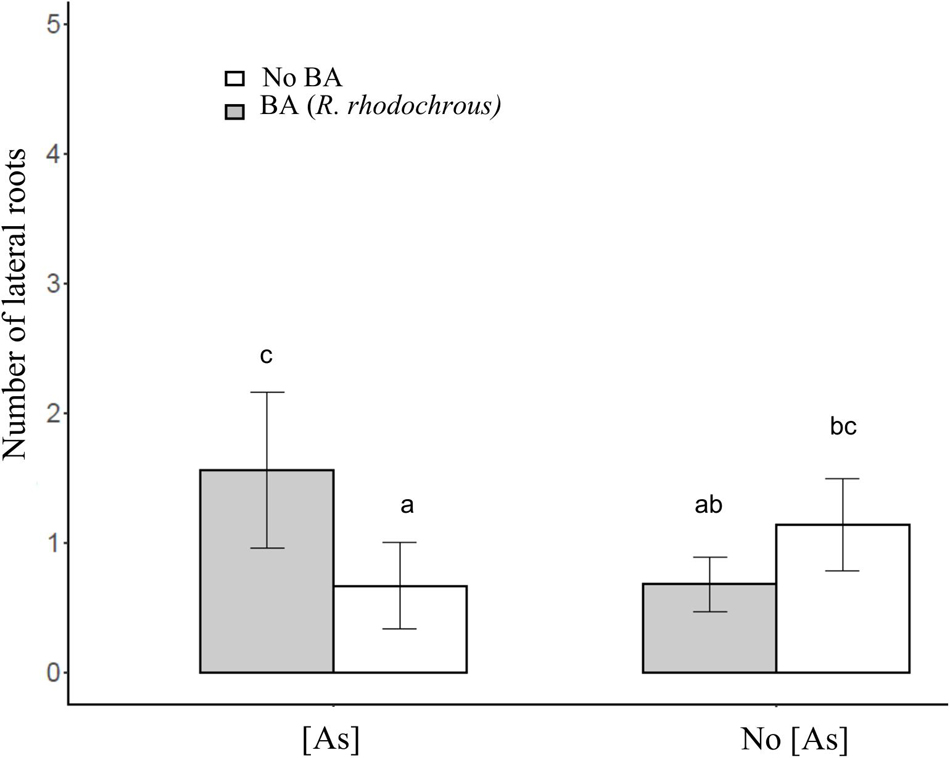
Figure 5. The effect of the BA and As treatment on the number of lateral roots. All statistical parameters are shown in Table 2. The error bars are standard error. Letters indicate the different group of means in SNK tests at p < 0.05.
Molecular Identification and Characterization of Jasione Endomicrobiota
Rhodococcus rhodochrous was the dominant OTU when it was inoculated and was present in all adult plants under BA treatments (Figures 6, 7). Cluster dendrogram analysis (UPGMA) based on the relative abundance of all OTUs revealed three major distinct clusters (Figure 6): (1) seeds group, (2) BA group, and (3) NoBA group. Among OTUs identified through high-throughput sequencing, the most common OTUs observed inside Jasione samples were genera Pseudomonas spp., Ralstonia spp., Undibacterium spp., Cutibacterium spp., Kocuria spp., and Comamonadaceae family (Figure 7A). Even though Jasione plants were grown under sterile conditions to avoid contamination, the number of OTUs within seeds was lower than those present in adult plants (Table 3). OTUs exclusively associated with plants growing under As stress were genus Brevundimonas spp. and family Oxalobacteraceae; shared with BA treatments were genera Nocardioides spp. and Sphingomonas spp.; and shared with NoBA were genera Methylobacterium spp., Enhydrobacter spp., Staphylococcus spp., and Stenotrophomonas spp. (Figure 7A). OTUs exclusively associated with plants in the BA treatment included species R. rhodochrous (which was inoculated), Massilia spp., and Asinibacterium spp. OTUs exclusively present in the NoBA treatment were families Lachnospiraceae, Erwiniaceae, and Micrococcaceae and genera Bacillus spp., Lawsonella spp., Chryseobacterium spp., Paenibacillus spp., and Rickettsiella grylli. No OTUs were exclusively in No As treatment (Figure 7A). The OTU exclusively associated with J. montana was Rhodococcus spp. and those associated with J. sessiliflora were the families Hymenobacteraceae and Caulobacteraceae (Figure 7B). A dynamic rank-abundance distribution curve for the endomicrobiota of Jasione is shown in Figure 7C. A small number of OTUs were dominant, while most of the OTUs were considered rare because of their low abundances.
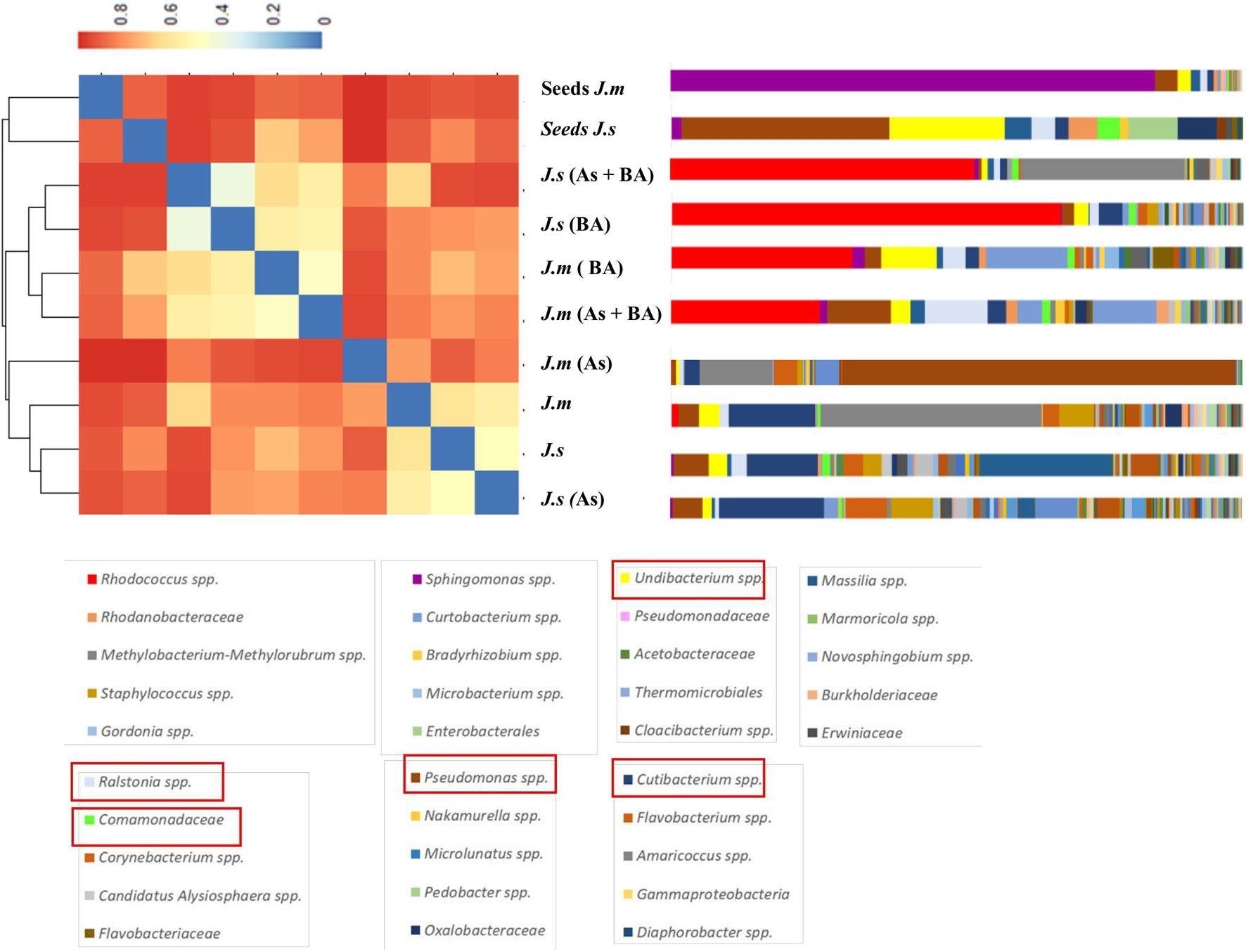
Figure 6. Hierarchical Clustering Heatmap Plot of dendrograms using Unweighted Pair Group Method with Arithmetic Mean (UPGMA) method. The heatmap represents a grid of colored points where each color represents a gradient of dissimilarity. Seeds of J. montana (J. m seeds), seeds of J. sessiliflora (J. s seeds), seedlings of J. montana with BA (R. rhodochrous) and As (J. m + BA + As), seedlings of J. sessiliflora with BA and As (J. s + BA + As), seedlings of J. montana with BA (J. m + BA), seedlings of J. sessiliflora with BA (J. s + BA), seedlings of J. montana (J. m), seedlings of J. sessiliflora (J. s), and seedlings of J. montana with As (J. m + As).
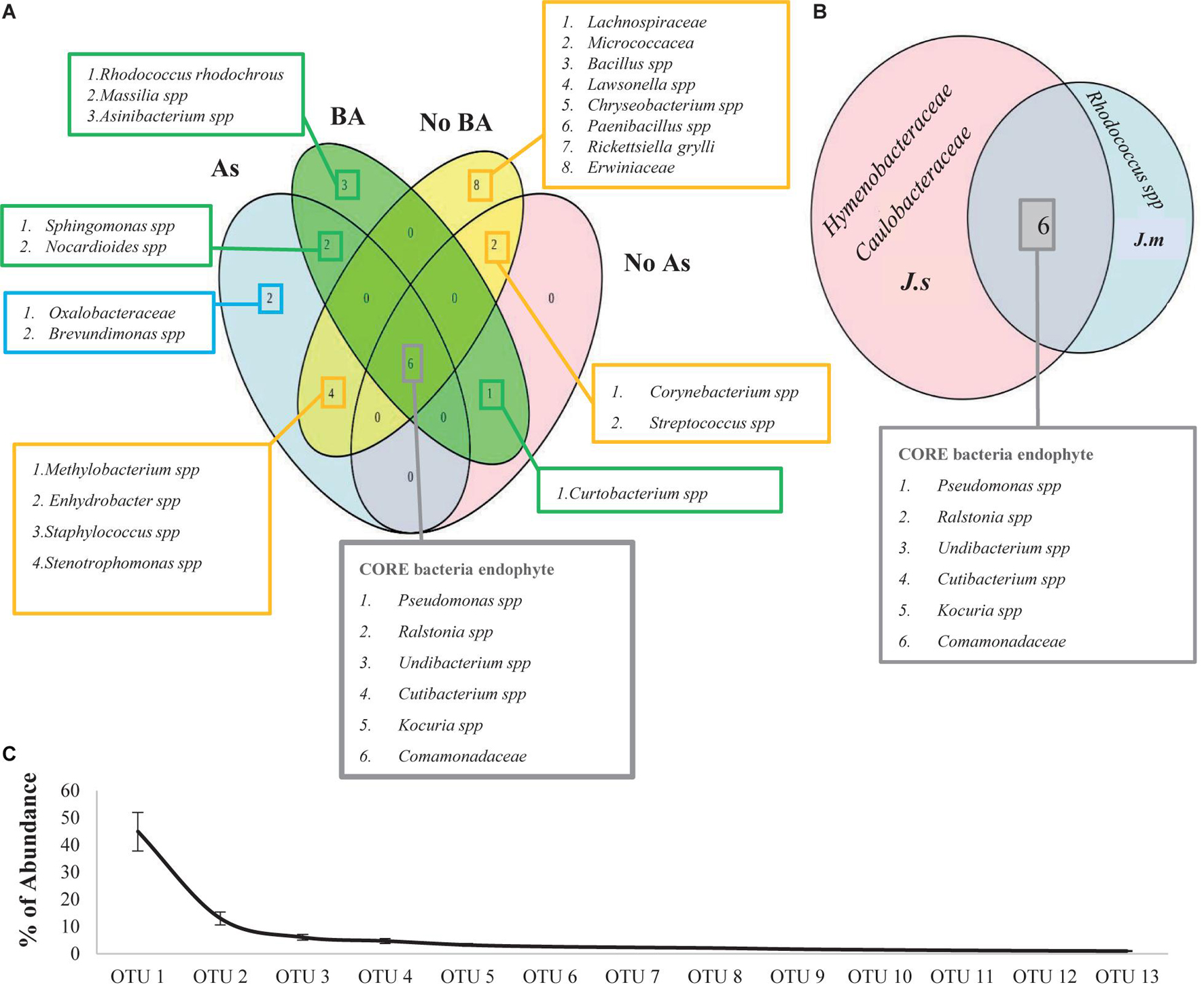
Figure 7. (A) The contribution of the OTUs in each group of samples, resulting from Illumina 16S rRNA gene analysis. Seeds of J. montana (J. m seeds), seeds of J. sessiliflora (J. s seeds), seedlings of J. montana with BA (R. rhodochrous) and As (J. m + BA + As), seedlings of J. sessiliflora with BA and As (J. s + BA + As), seedlings of J. montana with BA (J. m + BA), seedlings of J. sessiliflora with BA (J. s + BA), seedlings of J. montana (J. m), seedlings of J. sessiliflora (J. s), and seedlings of J. montana with As (J. m + As). The OTUs in red squares belong to the core microbiota of Jasione. (B) Venn diagram shows all OTUs presented at all Jasione samples (Jasione core bacteria endophytes; gray box), OTUs present at all samples BA (green boxes), OTUs present at all samples no BA (yellow boxes), OTUs present at all samples grown under As conditions (blue boxes), and OTUs present at all samples grown without As (red boxes). Venn diagram shows Jasione core bacteria endophytes (gray box) and the exclusive core bacteria endophytes to J. montana (blue box) and J. sessiliflora (red box). (C) Average (SE) dynamic rank abundance curve for endophytes within seeds and seedlings of Jasione under different treatments SI. Rarefaction curves generated by QIIME reflects the sequencing depth of each sample under a similarity of 0.97. Rarefaction curves were saturated indicating that the sampling depths were sufficient to capture the overall microbial diversity of all samples.
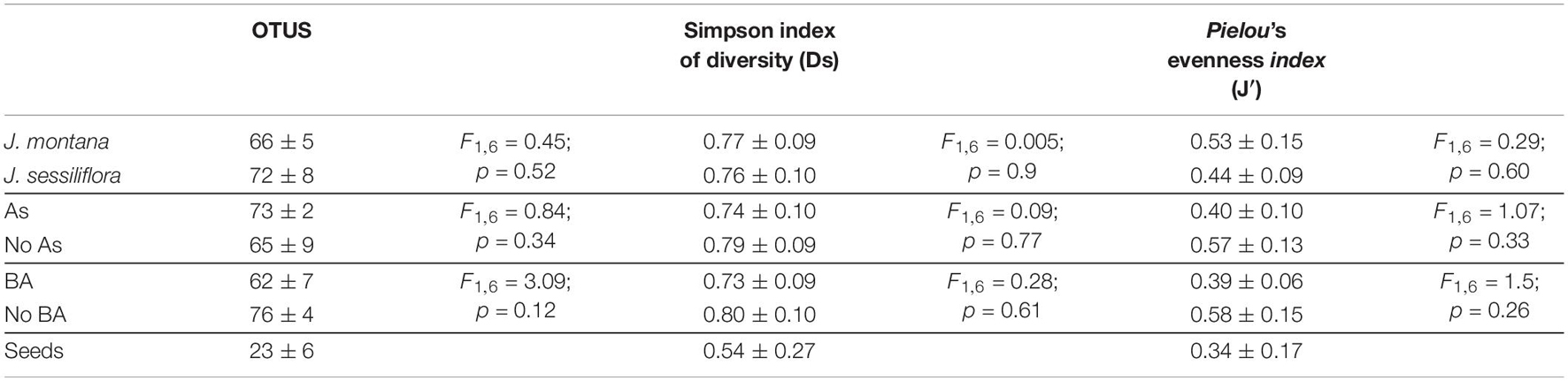
Table 3. Average (± SE) and ANOVA for the effects of species of Jasione, As, and BA treatment on total number of OTUs (S), Simpson index of diversity (Ds), and Pielou’s evenness index (J′).
Discussion
The study of seed endomicrobiota is an emergent area of research (Nelson, 2018; Verma and White, 2019; Franco-Franklin et al., 2021; Xu et al., 2021). Diversity of seed endomicrobiota is highly underestimated since most studies have used culturable techniques and not culture-independent approaches (Hardoim, 2019). Very little is known regarding how the seed endobiota modulates plant development.
The Role of Endomicrobiota in Jasione Development
Jasione seeds contained an average of 23 ± 6 OTUs. During Jasione seed imbibition and germination, seed endomicrobiota become activated (including germinating endospores) to create part of the spermosphere and play a role in development of the seedling and the adult plant (Chang et al., 2021; Molina et al., 2021). OTUs found in adult plants (70 ± 6) likely came from seeds (Barret et al., 2015). Seeds are in a dormant state, and it is probable that bacteria in seeds are also in a state of dormancy (Truyens et al., 2015). Since seed endomicrobiota are exposed to high osmotic pressures within seeds, endospore formation may be a feature to withstand this environment (Compant et al., 2011; Truyens et al., 2015). However, standard DNA purification procedures are not suitable to disrupt endospores. Some authors (e.g., Esteban et al., 2020) have proposed, as an alternative approach, a microwave treatment before the standard method to extract DNA from endospore and resistant structures. The lower number of OTUs identified inside the seeds is probably due to the presence of endospores where DNA was not extracted and amplified. Our findings suggest that combined OTU identifications from sterilized seeds and plants developed under axenic conditions show a more complete seed endomicrobiota diversity.
When endomicrobiota were removed from seeds with antibiotics, the gnotobiotic plants show a lower percentage of germination and plant development than holobiotic plants (plants with endomicrobiota associated). Previous studies have suggested that antibiotic treatment might cause irreparable damage to seed organelles, such as mitochondria (Robert and Hevor, 2007). However, the addition of endophytes to gnotobiotic organisms frequently improves seedling growth and plant development (Herrera et al., 2016; Verma and White, 2017; Verma et al., 2017; Molina et al., 2021), suggesting that organelles are not damaged with the sterilization treatment. We agree with previous conclusions (Truyens et al., 2015; Herrera et al., 2016; Verma and White, 2017; Verma et al., 2017; Chang et al., 2021) that bacterial endomicrobiota of seeds are essential to seed germination and plant development.
Core Bacterial Endophytes of Jasione
Most studies of plant microbiota are focused on the rhizosphere microbiome (Thijs et al., 2016; Mohanram and Kumar, 2019) because it is a reservoir of potential microorganisms with benefits for plants and therefore may be used as biofertilizers for sustainable agriculture. However, not all microorganisms from the rhizosphere are able to colonize efficiently into plant tissues; only 2–5% are considered plant growth-promoting bacteria (PGPB) (Antoun and Kloepper, 2001). Therefore, a better starting point to obtain plant microbes would be to focus on the study of the seed endomicrobiota (Matsumoto et al., 2021; Moreira et al., 2021); this especially since they are selected generation to generation for the ability to enter the host plant (Hardoim et al., 2008). Nevertheless, seeds have already shown to be a microbiota reservoir providing beneficial traits to the host plant (Ewald, 1987; Rudgers et al., 2009; Hardoim, 2019; Wang et al., 2021). Jasione persistent OTUs were genera Pseudomonas spp., Ralstonia spp., Undibacterium spp., Cutibacterium spp., and Kocuria spp. and family Comamonadaceae. These OTUs were inside all seedling plants of J. montana and J. sessiliflora and detected across all treatments. These OTUs have been previously identified as plant microbiota. Members of genus Pseudomonas have shown various PGP traits such as IAA and siderophore production and phosphate solubilization (Kumar et al., 2020). In addition, Pseudomonas spp. are common plant endophytes (Compant et al., 2011; Pacifico et al., 2019; Wang et al., 2021), acting also as biocontrol agents (Munakata et al., 2020) and members of the core microbiome of many plant species, including canola (Brassica napus) (Moreira et al., 2021). Recently, Pseudomonas has been found in rice plants with the ability to decrease arsenic translocation from roots into grains (Dolphen and Thiravetyan, 2019; Wang et al., 2021), and in lettuce roots as rare microbiota that protect against plant pathogens (Cardinale et al., 2015). Accordingly, other PGPB species, including Cutibacterium spp., identified in wheat (Triticum) seeds (Kuźniar et al., 2020; Abdullaeva et al., 2020); Undibacteriumin spp. identified in maize (Zea mays) (Liu et al., 2013); and Kocuria spp. identified in alfalfa (Medicago sativa) (López et al., 2018). Species of Comamonadaceae family were found to dominate barley (Hordeum vulgare) roots and rhizospheres (Bulgarelli et al., 2015). Species of genus Ralstonia can also colonize plants as endophytes (van Overbeek et al., 2004) and can be a diazotrophic endophyte with a capacity to increase N availability to plants (Patel and Archana, 2017). Together with species of genus Pseudomonas, Ralstonia species are part of the core microbiota of some grapevines (Pacifico et al., 2019). More recently, Ralstonia eutropha was found to alleviate As toxicity to wheat plants by increasing the efficiency of root energy metabolism and cell wall biosynthesis (Wang et al., 2018). Therefore, these OTUs can be considered as core bacterial endophytes since they are inside tissues of J. montana and J. sessiliflora and provide benefits to the host.
Rare Endomicrobiota of Seeds
The long tail of the rank abundance curve (Figure 7) represents the rare microbiota of Jasione. Most of the OTUs were below 1% of the contribution to total diversity. Only a few OTUs such as R. rhodochrous (inoculated), Sphingomonas spp., and Paenibacillus spp. represent more than 50% of the total microbiota. For instance, the members of the genera associated with BA treatment such as Asinibacterium and Nocardioides are considered rare since they only represent less than 1% of the total diversity of the Jasione seed endomicrobiota. However, species of genus Asinibacterium have shown tolerance to uranium (Brzoska et al., 2019) and species of genus Nocardioides have shown halotolerance and methylotrophic strains with the capacity to alleviate salinity stress of crop plants (Meena et al., 2020). Species of genus Brevundimonas, associated with As treatment, only represented 0.4% but some strains showed versatility to degrade recalcitrant aromatic compounds (Parthasarathy et al., 2017). Thus, most of the OTUs from Jasione seeds were rare microbiota. However, despite their low contribution, they represent a large bacterial reservoir with rapid responses to environmental changes (Du et al., 2020; Ji et al., 2020), playing important physiological and ecological roles in improving host traits (Sogin et al., 2006; Elshahed et al., 2008; Lynch and Neufeld, 2015).
Biotic and Abiotic Factors Controlling Endomicrobiota Structure
The degree of similarity between seed endomicrobiota of J. montana and J. sessiliflora is high. Endomicrobiota structure is a consequence of ecological and evolutionary forces driving microbial diversity and structure (West et al., 2019; Özkurt et al., 2020). The plant microbiome is thought to be controlled by various processes such as selection and speciation (which are deterministic events), drift, and dispersal represented by stochastic events (Vellend, 2016). Both niche (deterministic processes) and neutral (stochastic processes) theories have been advanced to explain microbial community assembly (Zhou and Ning, 2017; Wilber et al., 2020). Previous works have shown (Wilber et al., 2020) that abiotic (i.e., As) and biotic (i.e., addition of R. rhodochrous) factors affect bacterial selection from the host microbial assemblage after accounting for the effects of dispersal and drift.
The high similarity between the seed endomicrobiota of both species and the fact that J. montana and J. sessiliflora are phylogenetically very close could be a starting point to investigate coevolution between these two organisms. Jasione microbial assemblage are modulated by abiotic (i.e., As) or biotic (i.e., addition of R. rhodochrous) factors with important consequences at a functional and genetic level. From a functional point of view, these closely related species (plant genome plus its associated microbiota) showed different responses against stresses. The negative consequences of the As stress were only observed within J. sessiliflora but not in J. montana. These results would suggest that only J. montana have physiological traits against As stress. The exclusive J. montana core bacterial endophyte was composed by genus Rhodococcus. Members of this genus (R. aetherivorans, R. erythropolis, R. equi, and R. rhodochrous) have also been described as PGPB or endophytes resistant to As (Gu et al., 2018; Molina et al., 2021; Navazas et al., 2021). From a functional point of view, R. rhodochrous has been shown to colonize efficiently Jasione species, modifying the response to As stress. According to Thijs et al. (2016), plant microbiota assembly under environmental stress depends on the recruitment of key species. The bioaugmentation with R. rhodochrous provides different physiological traits depending on the Jasione species. For instance, R. rhodochrous provided synergistic mechanisms to J. montana, increasing the number of leaves. The same endophyte was antagonistic to J. sessiliflora, decreasing the number of LR. It is interesting that under As stress, R. rhodochrous provided benefits to both species, increasing the number of LR over the control conditions under equivalent stress. Previous investigations have already shown that continuous exposure of plants to stress conditions decreases plant endogenous IAA levels. However, the inoculation with endophytes and rhizobacteria increases IAA synthesis (Sheng et al., 2008; Rajkumar et al., 2009; Khaksar et al., 2017). Therefore, R. rhodochrous may affect Jasione traits in two ways as follows. R. rhodochrous is a PGPB that is resistant to As and produces IAA (Molina et al., 2021). This phytohormone increases LR (Lavenus et al., 2013) but also alleviates ROS accumulation produced by As stress (Khaksar et al., 2017). In addition, R. rhodochrous is capable of metabolizing As (V) to organic forms, possibly less toxic (Molina et al., 2021), which is an obvious advantage for both species but especially for J. sessiliflora, which does not have this microorganism among the components of its microbiota. Therefore, R. rhodochrous may modulate Jasione root plasticity, which is a key adaptative trait to cope As stress (Lavenus et al., 2013). Our results provide empirical evidence for the role of a key species (R. rhodochrous) transmitted horizontally, driving the structure and final functionality of host endomicrobiota against As stress. Our findings suggest the Jasione traits are influenced by R. rhodochrous and associated microbiota (Ravanbakhsh et al., 2019) and improve host response to As stress.
Conclusion
In this study, we have shown that Jasione traits are influenced by both R. rhodochrous and seed-associated endomicrobiota and improve host response against As stress. Our experimental evidence shows that R. rhodochrous efficiently colonizes Jasione plants, influencing the structure and final functionality of the endomicrobiota. Furthermore, in this study, next-generation sequencing technologies (NGS-Illumina) helped to identify Jasione core bacterial endophytes composed of genera Pseudomonas spp., Ralstonia spp., Undibacterium spp., Cutibacterium spp., and Kocuria spp. and family Comamanadaceae. This technology also provided empirical evidence of the rare endomicrobiota inside Jasione seeds. These rare members represent most of the total diversity, and despite their low contribution, they offer important physiological and ecological traits to host. Therefore, plant seed endomicrobiota or endophytes should be considered to be a resource for exploitation; they have potential applications and utility to environmental remediation, sustainable agriculture, and biotechnology.
Data Availability Statement
The datasets presented in this study can be found in online repositories. The names of the repository/repositories and accession number(s) can be found below: https://www.ncbi.nlm.nih.gov/, SUB9031392. Sequence data were deposited in the NCBI Sequence REacd Archive, Bioproject ID PRJNA699582, accessions SAMN17799125 to SAMN17799134.
Author Contributions
MM and NG-B: conceptualization and methodology. IM-R, IC, and MA: the expertise on the software. NG-B, IM-R, and MA: formal analysis. NG-B and IM-R: writing the original draft. JW: showing the techniques to manipulate and isolate endophytes. MM, MA, IC, and JW: review and editing. All authors contributed extensively to the work, and read and agreed to the published version of the manuscript.
Funding
This work has been supported by the Research grant from Universidad Rey Juan Carlos (AYUDA PUENTE 2019-DRIADES Project). IM-R gratefully acknowledges PEJD-2019-PRE/AMB-16306 from the Madrid Community program. Funding support was also provided by USDA-NIFA Multistate Project W4147 and the New Jersey Agricultural Experiment Station.
Conflict of Interest
The authors declare that the research was conducted in the absence of any commercial or financial relationships that could be construed as a potential conflict of interest.
Publisher’s Note
All claims expressed in this article are solely those of the authors and do not necessarily represent those of their affiliated organizations, or those of the publisher, the editors and the reviewers. Any product that may be evaluated in this article, or claim that may be made by its manufacturer, is not guaranteed or endorsed by the publisher.
Acknowledgments
The authors would like to thank Aida Vaquero (URJC) during the experimental phase of the work and Marcos Méndez (URJC) for their support and assistance during the initial stages and development and Pauthier and Lang for providing the seeds from the collection of the French National Museum of Natural History.
Supplementary Material
The Supplementary Material for this article can be found online at: https://www.frontiersin.org/articles/10.3389/fmicb.2021.664271/full#supplementary-material
Footnotes
- ^ https://imagej.nih.gov/ij/
- ^ https://data.qiime2.org/2020.6/common/silva-138-99-515-806-nb-classifier.qza
References
Abbas, G., Murtaza, B., Bibi, I., Shahid, M., Niazi, N. K., Khan, M. I., et al. (2018). Arsenic uptake, toxicity, detoxification, and speciation in plants: physiological, biochemical, and molecular aspects. Int. J. Environ. Res. Public Health 15, 59–104. doi: 10.3390/ijerph15010059
Abdullaeva, Y., Manirajan, B. A., Honermeier, B., Schnell, S., and Cardinale, M. (2020). Domestication affects the composition, diversity, and co-occurrence of the cereal seed microbiota. J. Adv. Res. 31, 75–86. doi: 10.1016/j.jare.2020.12.008
Altschul, S. F., Madden, T. L., Schäffer, A. A., Zhang, J., Zhang, Z., Miller, W., et al. (1997). Gapped BLAST and PSI-BLAST: a new generation of protein database search programs. Nucleic Acids Res. 25, 3389–3402. doi: 10.1093/nar/25.17.3389
Antoun, H., and Kloepper, J. W. (2001). “Plant growth promoting rhizobacteria,” in Encyclopedia of Genetics, 2nd Edn. eds S. Brenner and J. H. Miller (Amsterdam: Elsevier), 1477–1480.
Arenz, B. E., Schlatter, D. C., Bradeen, J. M., and Kinkel, L. L. (2015). Blocking primers reduce co-amplification of plant DNA when studying bacterial endophyte communities. J. Microbiol. Methods 117, 1–3. doi: 10.1016/j.mimet.2015.07.003
Barret, M., Briand, M., Bonneau, S., Préveaux, A., Valière, S., Bouchez, O., et al. (2015). Emergence shapes the structure of the seed microbiota. Appl. Environ. Microbiol. 81, 1257–1266. doi: 10.1128/AEM.03722-14
Boylen, E., Rideout, J. R., Dillon, M. R., Bokulich, M. R., Abnet, C. C., and Caporaso, J. G. (2019). Reproducible, interactive, scalable and extensible microbiome data science using QIIME 2. Nat. Biotechnol. 37, 852–857. doi: 10.1038/s41587-019-0209-9
Brzoska, R. M., Huntemann, M., Clum, A., Chen, A., Kyrpides, N., Palaniappan, K., et al. (2019). Complete genome sequence for Asinibacterium sp. Strain OR53 and draft genome sequence for Asinibacterium sp. Strain OR43, two bacteria tolerant to uranium. Microbiol. Resour. Announc. 8:e01701-18. doi: 10.1128/MRA.01701-18
Bulgarelli, D., Garrido-Oter, R., Munch, P. C., Weiman, A., Droge, J., Pan, Y., et al. (2015). Structure and function of the bacterial root microbiota in wild and domesticated barley. Cell Host Microbe. 17, 392–403. doi: 10.1016/j.chom.2015.01.011
Burnham, K., and Anderson, D. (2002). Model Selection and Multimodel Inference:A Practical Information-Theoretic Approach, 2nd Edn. New York, NY: Springer.
Calabrese, E. J., and Agathokleous, E. (2021). Accumulator plants and hormesis. Environ. Pollut. 274:116526. doi: 10.1016/j.envpol.2021.116526
Callahan, B. J., McMurdie, P. J., Rosen, M. J., Han, A. W., Johnson, A. J. A., and Holmes, S. P. (2016). DADA2: high-resolution sample inference from Illumina amplicon data. Nat. Methods 13, 581–583.
Cardinale, M., Grube, M., Erlacher, A., Quehenberger, J., and Berg, G. (2015). Bacterial networks and co-occurrence relationships in the lettuce root microbiota. Environ. Microbiol. 17, 239–252. doi: 10.1111/1462-2920.12686
Chang, X., Kingsley, K. L., and White, J. F. (2021). Chemical interactions at the interface of plant root hair cells and intracellular bacteria. Microorganisms 9:1041. doi: 10.3390/microorganisms9051041
Clay, K. (1998). Fungal endophytes of grasses: a defensive mutualism between plants and fungi. Ecology 69, 10–16.
Compant, S., Cambon, M. C., Vacher, C., Mitter, B., Samad, A., and Sessitsch, A. (2021). The plant endosphere world–bacterial life within plants. Environ. Microbiol. 23, 1812–1829. doi: 10.1111/1462-2920.15240
Compant, S., Mitter, B., Colli-Mull, J. G., Gangl, H., and Sessitsch, A. (2011). Endophytes of grapevine flowers, berries, and seeds: identification of cultivable bacteria, comparison with other plant parts, and visualization of niches of colonization. Microb. Ecol. 62, 188–197. doi: 10.1007/s00248-011-9883-y
Cordovez, V., Dini-Andreote, F., Carrión, V. J., and Raaijmakers, J. M. (2019). Ecology and evolution of plant microbiomes. Annu. Rev. Microbiol. 73, 69–88.
De Barry, A. (1866). Morphologie und Physiologie der Pilze, Flechten, und Myxomyceten. Hofmeister’s Hand- Book of Physiological Botany, Vol. II., Leipzig: W. Engelmann.
Dolphen, R., and Thiravetyan, P. (2019). Reducing arsenic in rice grains by leonardite and arsenic–resistant endophytic bacteria. Chemosphere 223, 448–454. doi: 10.1016/j.chemosphere.2019.02.054
Du, S., Dini-Andreote, F., Zhang, N., Liang, C., Yao, Z., Zhang, H., et al. (2020). Divergent co-occurrence patterns and assembly processes structure the abundant and rare bacterial communities in a salt marsh ecosystem. Appl. Environ. Microbiol. 86:e00322-20. doi: 10.1128/AEM.00322-20
Elshahed, M. S., Youssef, N. H., Spain, A. M., Sheik, C., Najar, F. Z., and Krumholz, L. R. (2008). Novelty and uniqueness patterns of rare members of the soil biosphere. Appl. Environ. Microbiol. 74, 5422–5428. doi: 10.1128/AEM.00410-08
Esteban, M., Marcos, P., Horna, C., Galan-Malo, P., Mata, L., and Sánchez, L. (2020). Evaluation of methods for DNA extraction from Clostridium tyrobutyricum spores and its detection by qPCR. J. Microbiol. Methods 169:105818. doi: 10.1016/j.mimet.2019.105818
Ewald, P. W. (1987). Transmission modes and evolution of the parasitism-mutualism continuum. Ann. N. Y. Acad. Sci. 503, 295–306. doi: 10.1111/j.1749-6632.1987.tb40616.x
Franco-Franklin, V., Moreno-Riascos, S., and Ghneim-Herrera, T. (2021). Are endophytic bacteria an option for increasing heavy metal tolerance of plants? A meta-analysis of the effect size. Front. Environ. Sci. 8:603668. doi: 10.3389/fenvs.2020.603668
García-Salgado, S., García-Casillas, D., Quijano-Nieto, M., and Bonilla-Simón, M. (2011). Arsenic and heavy metal uptake and accumulation in native plant species from soils polluted by mining activities. Water Air Soil Pollut. 223, 559–572. doi: 10.1007/s11270-011-0882-x
Gu, Y., Wang, Y., Sun, Y., Zhao, K., Xiang, Q., and Chen, Q. (2018). Genetic diversity and characterization of arsenic-resistant endophytic bacteria isolated from Pteris vittata, an arsenic hyperaccumulator. BMC Microbiol. 18:42. doi: 10.1186/s12866-018-1184-x
Guterres, J., Rossato, L., Doley, D., Pudmenzky, A., Bee, C., and Cobena, V. (2019). Assessing germination characteristics of Australian native plant species in metal/metalloid solution. J. Hazard. Mater. 364, 173–181. doi: 10.1016/j.jhazmat.2018.10.019
Gutiérrez-Ginés, M. J., Pastor, J., and Hernández, A. J. (2015). “Heavy metals in native mediterranean grassland species growing at abandoned mine sites: ecotoxicological assessment and phytoremediation of polluted soils,” in Heavy Metal Contamination of Soils, eds I. Sherameti and A. Varma (Cham: Springer), 159–178.
Hardoim, P. (2019). “The ecology of seed microbiota,” in Seed Endophytes, eds S. Verma and J. White (Cham: Springer), 103–125.
Hardoim, P. R., van Overbeek, L. S., Berg, G., Pirttilä, A. M., Company, S., and Sessitsch, A. (2015). The Hidden World within plants: ecological and evolutionary considerations for defining functioning of microbial endophytes. Microbiol. Mol. Biol. Rev. 79, 293–320. doi: 10.1128/MMBR.00050-14
Hardoim, P. R., van Overbeek, L. S., and van Elsas, J. D. (2008). Properties of bacterial endophytes and their proposed role in plant growth. Trends Microbiol. 16, 463–471. doi: 10.1016/j.tim.2008.07.008
Herrera, S. D., Grossi, C., Zawoznik, M., and Groppa, M. D. (2016). Wheat seeds harbour bacterial endophytes with potential as plant growth promoters and biocontrol agents of Fusarium graminearum. Microbiol. Res. 186–187, 37–43. doi: 10.1016/j.micres.2016.03.002
Ji, M., Kong, W., Stegen, J., Yue, L., Wang, F., and Ferrari, B. C. (2020). Distinct assembly mechanisms underlie similar biogeographical patterns of rare and abundant bacteria in Tibetan Plateau grassland soils. Environ. Microbiol. 22, 2261–2272. doi: 10.1111/1462-2920.14993
Khaksar, G., Treesubsuntorn, C., and Thiravetyan, P. (2017). Euphorbia milii-native bacteria interactions under airborne formaldehyde stress: effect of epiphyte and endophyte inoculation in relation to IAA, ethylene and ROS levels. Plant Physiol. Biochem. 111, 284–294. doi: 10.1016/j.plaphy.2016.12.011
Klaedtke, S., Jacques, M. A., Raggi, L., Préveaux, A., Bonneau, S., and Barret, M. (2016). Terroir is a key driver of seed-associated microbial assemblages. Environ. Microbiol. 18, 1792–1804. doi: 10.1111/1462-2920.12977
Kumar, A., and Verma, J. P. (2018). Does plant—microbe interaction confer stress tolerance in plants: a review? Microbiol. Res. 207, 41–52. doi: 10.1016/j.micres.2017.11.004
Kumar, A., Voropaeva, O., Maleva, M., Panikovskaya, K., Borisova, G., and Bruno, L. B. (2020). Bioaugmentation with copper tolerant endophyte Pseudomonas lurida strain EOO26 for improved plant growth and copper phytoremediation by Helianthus annuus. Chemosphere 266:128983. doi: 10.1016/j.chemosphere.2020.128983
Kuźniar, A., Włodarczyk, K., Grza̧dziel, J., Woźniak, M., Furtak, K., and Wolińska, A. (2020). New insight into the composition of wheat seed microbiota. Int. J. Mol. Sci. 21:4634. doi: 10.3390/ijms21134634
Lavenus, J., Goh, T., Roberts, I., Guyomarc’h, S., Lucas, M., and Laplaze, L. (2013). Lateral root development in Arabidopsis: fifty shades of auxin. Trends Plant Sci. 18, 450–458. doi: 10.1016/j.tplants.2013.04.006
Liu, Y., Zuo, S., Zou, Y., Wang, J., and Song, W. (2013). Investigation on diversity and population succession dynamics of endophytic bacteria from seeds of maize (Zea mays L., Nongda108) at different growth stages. Ann. Microbiol. 63, 71–79. doi: 10.1007/s13213-012-0446-3
López, J. L., Alvarez, F., Príncipe, A., Salas, M. E., Lozano, M. J., and Lagares, A. (2018). Isolation, taxonomic analysis, and phenotypic characterization of bacterial endophytes present in alfalfa (Medicago sativa) seeds. J. Biotechnol. 267, 55–62. doi: 10.1016/j.jbiotec.2017.12.020
Lynch, M. D., and Neufeld, J. D. (2015). Ecology and exploration of the rare biosphere. Nat. Rev. Microbiol. 13, 217–229. doi: 10.1038/nrmicro3400
Matsumoto, H., Fan, X., Wang, Y., Kusstatscher, P., Duan, J., and Wang, M. (2021). Bacterial seed endophyte shapes disease resistance in rice. Nat. Plants 7, 60–72. doi: 10.1038/s41477-020-00826-5
Meena, K. K., Bitla, U. M., Sorty, A. M., Singh, D. P., Gupta, V. K., and Kumar, S. (2020). Mitigation of salinity stress in wheat seedlings due to the application of phytohormone-rich culture filtrate extract of methylotrophic actinobacterium Nocardioides sp. NIMMe6. Front. Microbiol. 11:2091. doi: 10.3389/fmicb.2020.02091
Mohanram, S., and Kumar, P. (2019). Rhizosphere microbiome: revisiting the synergy of plant-microbe interactions. Ann. Microbiol. 69, 307–320. doi: 10.1007/s13213-019-01448-9
Molina, M. D. C., White, J. F., García-Salgado, S., Quijano, M., and González-Benítez, N. (2021). A gnotobiotic model to examine plant and microbiome contributions to survival under arsenic stress. Microorganisms 9:45. doi: 10.3390/microorganisms9010045
Moreira, M. Z., Helgason, B., and Germida, J. (2021). Environment has a stronger effect than host plant genotype in shaping spring Brassica napus seed microbiomes. Phytobiomes J. 1–11. doi: 10.1094/PBIOMES-08-20-0059-R
Moreno-Jiménez, E., Peñalosa, J. M., Carpena-Ruiz, R. O., and Esteban, E. (2008). Comparison of arsenic resistance in Mediterranean woody shrubs used in restoration activities. Chemosphere 71, 466–473. doi: 10.1016/j.chemosphere.2007.10.030
Munakata, Y., Gavira, C., Genestier, J., Bourgaud, F., Hehn, A., and Slezack-Deschaumes, S. (2020). Composition and functional comparison of vetiver root endophytic microbiota originating from different geographic locations that show antagonistic activity towards Fusarium graminearum. Microbiol. Res. 243:126650. doi: 10.1016/j.micres.2020.126650
Navazas, A., Thijs, S., Feito, I., Vangronsveld, J., Peláez, A. I., and González, A. (2021). Arsenate-reducing bacteria affect as accumulation and tolerance in Salix atrocinerea. Sci. Total Environ. 769:144648. doi: 10.1016/j.scitotenv.2020.144648
Nelson, E. B. (2018). The seed microbiome: origins, interactions, and impacts. Plant Soil 422, 7–34. doi: 10.1007/s11104-017-3289-7
Oksanen, J. (2016). Design Decisions and Implementation Details in Vegan, in Vignette of the Package Vegan. R Package Version, Vol. 2016, 2–4.
Özkurt, E., Hassani, M. A., Sesiz, U., Künzel, S., Dagan, T., Özkan, H., et al. (2020). Seed-derived microbial colonization of wild emmer and domesticated bread wheat (Triticum dicoccoides and T. aestivum) seedlings shows pronounced differences in overall diversity and composition. mBio 11:e02637-20. doi: 10.1128/mBio.02637-20
Pacifico, D., Squartini, A., Crucitti, D., Barizza, E., Lo Schiavo, F., Muresu, R., et al. (2019). The role of the endophytic microbiome in the grapevine response to environmental triggers. Front. Plant Sci. 10:1256. doi: 10.3389/fpls.2019.01256
Parthasarathy, S., Azam, S., Lakshman Sagar, A., Narasimha Rao, V., and Siddavattam, D. (2017). Genome-guided insights reveal organophosphate-degrading Brevundimonas diminuta as Sphingopyxis wildii and define its versatile metabolic capabilities and environmental adaptations. Genome Biol. Evol. 9, 77–81. doi: 10.1093/gbe/evw275
Patel, J. K., and Archana, G. (2017). Diverse culturable diazotrophic endophytic bacteria from Poaceae plants show cross-colonization and plant growth promotion in wheat. Plant Soil 417, 99–116. doi: 10.1007/s11104-017-3244-7
Pérez-Espona, S., Sales, F., Hedge, I. C., and Moller, M. (2005). Phylogeny and species relationships in Jasiones (Campanulaceae) with emphasis on the ‘montana-complex’. Edinb. J. Bot. 62, 29–51. doi: 10.1017/S0960428606000047
Perotti, R. (1926). On the limits of biological inquiry on soil science. Proc. Int. Soc. Soil Sci. 2, 146–161.
R Core Team (2019). R: A Language and Environment for Statistical Computing. Vienna: R Foundation for Statistical Computing.
Rajkumar, M., Ae, N., and Freitas, H. (2009). Endophytic bacteria and their potential to enhance heavy metal phytoextraction. Chemosphere 77, 153–160. doi: 10.1016/j.chemosphere.2009.06.047
Ravanbakhsh, M., Kowalchuk, G. A., and Jousset, A. (2019). Root-associated microorganisms reprogram plant life history along the growth–stress resistance trade-off. ISME J. 13, 3093–3101. doi: 10.1038/s41396-019-0501-1
Robert, F., and Hevor, T. K. (2007). Abnormal organelles in cultured astrocytes are largely enhanced by streptomycin and intensively by gentamicin. Neuroscience 144, 191–197. doi: 10.1016/j.neuroscience.2006.08.059
Rodríguez, R. J., Henson, J., van Volkenburgh, E., Hoy, M., Wright, L., and Redman, R. S. (2008). Stress tolerance in plants via habitat-adapted symbiosis. ISME J. 2, 404–416. doi: 10.1038/ismej.2007.106
Rudgers, J. A., Afkhami, M. E., Rúa, M. A., Davitt, A. J., Hammer, S., and Huguet, V. M. (2009). A fungus among us: broad patterns of endophyte distribution in the grasses. Ecology 90, 1531–1539. doi: 10.1890/08-0116.1
Schulz, B., and Boyle, C. (2005). The endophytic continuum. Mycol. Res. 109, 661–686. doi: 10.1017/S095375620500273
Sheng, X. F., Xia, J. J., Jiang, C. Y., He, L. Y., and Qian, M. (2008). Characterization of heavy metal-resistant endophytic bacteria from rape (Brassica napus) roots and their potential in promoting the growth and lead accumulation of rape. Environ. Pollut. 156, 1164–1170. doi: 10.1016/j.envpol.2008.04.007
Soares, M. A., Li, H. Y., Bergen, M., da Silva, J. M., Kowalski, K. P., and White, J. F. (2016). Functional role of an endophytic Bacillus amyloliquefaciens in enhancing growth and disease protection of invasive English ivy (Hedera helix L.). Plant Soil 405, 107–123. doi: 10.1007/s11104-015-2638-7
Sogin, M. L., Morrison, H. G., and Huber, J. A. (2006). Microbial diversity in the deep sea and the underexplored “rare biosphere.”. Proc. Natl. Acad. Sci. U.S.A. 103, 12115–12120. doi: 10.1073/pnas.0605127103
Thijs, S., Sillen, W., Rineau, F., Weyens, N., and Vangronsveld, J. (2016). Towards an enhanced understanding of plant–microbiome interactions to improve phytoremediation: engineering the metaorganism. Front. Microbiol. 7:341. doi: 10.3389/fmicb.2016.00341
Trivedi, P., Leach, J. E., Tringe, S. G., Sa, T., and Singh, B. K. (2020). Plant–microbiome interactions: from community assembly to plant health. Nat. Rev. Microbiol. 18, 607–621. doi: 10.1038/s41579-020-0412-1
Truyens, S., Weyens, N., Cuypers, A., and Vangronsveld, J. (2015). Bacterial seed endophytes: genera, vertical transmission, and interaction with plants. Environ. Microbiol. Rep. 7, 40–50.
van Overbeek, L. S., Bergervoet, J. H. W., Jacobs, F. H. H., and van Elsas, J. D. (2004). The low-temperature-induced viable-but-nonculturable state affects the virulence of Ralstonia solanacearum Biovar 2. Phytopathology 94, 463–469. doi: 10.1094/PHYTO.2004.94.5.463
Vandenkoornhuyse, P., Quaiser, A., Duhamel, M., Le Van, A., and Dufresne, A. (2015). The importance of the microbiome of the plant holobiont. New Phytol. 206, 1196–1206. doi: 10.1111/nph.13312
Vellend, M. (2016). The Theory of Ecological Communities. Princeton, NJ: Princeton University Press.
Verma, S. K., Kingsley, K., Irizarry, I., Bergen, M., Kharwar, R. N., and White, J. F. (2017). Seed vectored endophytic microbes involved in modulation of development in rice seedlings. J. Appl. Microbiol. 122, 1680–1691. doi: 10.1111/jam.13463
Verma, S. K., and White, J. F. (2017). Indigenous endophytic seed bacteria promote seedling development and defend against fungal disease in browntop miller (Urochloa ramose L.). J. Appl. Microbiol. 124, 764–778. doi: 10.1111/jam.13673
Wang, X. H., Wang, Q., Nie, Z. W., He, L. Y., and Sheng, X. F. (2018). Ralstonia eutropha Q2-8 reduces wheat plant above-ground tissue cadmium and arsenic uptake and increases the expression of the plant root cell wall organization and biosynthesis-related proteins. Environ. Pollut. 242, 1488–1499.
Wang, Z., Zhu, Y., Jing, R., Wu, X., Li, N., Liu, H., et al. (2021). High-throughput sequencing-based analysis of the composition and diversity of endophytic bacterial community in seeds of upland rice. Arch. Microbiol. 203, 609–620. doi: 10.1007/s00203-020-02058-9
West, A. G., Waite, D. W., Deines, P., Bourne, D. G., Digby, A., Mckenzie, V. J., et al. (2019). The microbiome in threatened species conservation. Biol. Conserv. 229, 85–98. doi: 10.1016/j.biocon.2018.11.016
Wilber, M. Q., Jani, A. J., Mihaljevic, J. R., and Briggs, C. J. (2020). Fungal infection alters the selection, dispersal and drift processes structuring the amphibian skin microbiome. Ecol. Lett. 23, 88–98. doi: 10.1111/ele.13414
Xu, L., Pierroz, G., Wipf, H. M. L., Gao, C., Taylor, J. W., Lemaux, P. G., et al. (2021). Holo-omics for deciphering plant-microbiome interactions. Microbiome 9:69. doi: 10.1186/s40168-021-01014-z
Keywords: arsenic, gnotobiont, Jasione sessiliflora, vertical transmission, horizontal transmission, bacterial endophyte core, Rhodococcus rhodochrous, Jasione montana
Citation: González-Benítez N, Martín-Rodríguez I, Cuesta I, Arrayás M, White JF and Molina MC (2021) Endophytic Microbes Are Tools to Increase Tolerance in Jasione Plants Against Arsenic Stress. Front. Microbiol. 12:664271. doi: 10.3389/fmicb.2021.664271
Received: 05 February 2021; Accepted: 08 June 2021;
Published: 06 October 2021.
Edited by:
Milko Alberto Jorquera, University of La Frontera, ChileReviewed by:
Massimiliano Cardinale, University of Salento, ItalyAradhana Mishra, National Botanical Research Institute (CSIR), India
Copyright © 2021 González-Benítez, Martín-Rodríguez, Cuesta, Arrayás, White and Molina. This is an open-access article distributed under the terms of the Creative Commons Attribution License (CC BY). The use, distribution or reproduction in other forums is permitted, provided the original author(s) and the copyright owner(s) are credited and that the original publication in this journal is cited, in accordance with accepted academic practice. No use, distribution or reproduction is permitted which does not comply with these terms.
*Correspondence: Natalia González-Benítez, bmF0YWxpYS5nb256YWxlekB1cmpjLmVz