- 1Departamento de Ecología y Biodiversidad, Facultad de Ciencias de la Vida, Universidad Andrés Bello, Santiago, Chile
- 2Facultad de Ciencias de la Vida, Centro de Investigación para la Sustentabilidad, Universidad Andrés Bello, Santiago, Chile
- 3Millennium Initiative for Collaborative Research on Bacterial Resistance (MICROB-R), Santiago, Chile
- 4Escuela de Medicina Veterinaria, Facultad de Agronomía e Ingeniería Forestal, Facultad de Ciencias Biológicas y Facultad de Medicina, Pontificia Universidad Católica de Chile, Santiago, Chile
- 5Department of Animal Production and Preventive Veterinary Medicine, School of Veterinary Medicine and Animal Science, São Paulo State University (UNESP), Botucatu, Brazil
- 6Microbiology and Antibiotic Resistance Team (MicroART), Department of Veterinary Sciences, University of Trás-os-Montes and Alto Douro (UTAD), Vila Real, Portugal
- 7Associated Laboratory for Green Chemistry (LAQV-REQUIMTE), University NOVA of Lisbon, Lisbon, Portugal
- 8Veterinary and Animal Research Centre, Associate Laboratory for Animal and Veterinary Science (AL4AnimalS), University of Trás-os-Montes and Alto Douro (UTAD), Vila Real, Portugal
- 9Área Bioquímica y Biología Molecular, Universidad de La Rioja, Logroño, Spain
The role of wildlife with long-range dispersal such as gulls in the global dissemination of antimicrobial resistance (AMR) across natural and anthropogenic aquatic environments remains poorly understood. Antibiotic-resistant bacteria have been detected in resident and migratory gulls worldwide for more than a decade, suggesting gulls as either sentinels of AMR pollution from anthropogenic sources or independent reservoirs that could maintain and disperse AMR across aquatic environments. However, confirming either of these roles remains challenging and incomplete. In this review, we present current knowledge on the geographic regions where AMR has been detected in gulls, the molecular characterization of resistance genes, and the evidence supporting the capacity of gulls to disperse AMR across regions or countries. We identify several limitations of current research to assess the role of gulls in the spread of AMR including most studies not identifying the source of AMR, few studies comparing bacteria isolated in gulls with other wild or domestic species, and almost no study performing longitudinal sampling over a large period of time to assess the maintenance and dispersion of AMR by gulls within and across regions. We suggest future research required to confirm the role of gulls in the global dispersion of AMR including the standardization of sampling protocols, longitudinal sampling using advanced satellite tracking, and whole-genome sequencing typing. Finally, we discuss the public health implications of the spread of AMR by gulls and potential solutions to limit its spread in aquatic environments.
Introduction
Antimicrobial resistance (AMR) is a major global health challenge affecting human, animal, and environmental health (FAO and WHO, 2019; WHO, 2019). Thus, a One Health approach is required to understand the dynamics of AMR between humans and animals (Salgado-Caxito et al., 2021). Many studies have reported the presence of antibiotic-resistant bacteria (ARB) in wild animals, highlighting their potential role in the spread of clinically important bacteria to humans and domestic animals (Wang et al., 2017; Benavides et al., 2018; Dolejska and Literak, 2019). Wildlife such as wild birds, particularly the ones living in proximity to human settings or agriculture fields, can acquire AMR from anthropogenic sources when feeding on landfills and wastewater (Nelson et al., 2008; Wang et al., 2017). Despite several reports of wild birds carrying ARB (Wang et al., 2017), their impact on the dissemination of ARB in aquatic environments remains still poorly understood.
Gulls can impact the spread of ARB of public health concern by acting either as (i) receivers of ARB or antibiotic-resistant genes (ARGs) and acting as sentinels of human environmental pollution to natural ecosystems (Guenther et al., 2011) or as (ii) reservoirs of ARBs and ARGs, capable of dispersing ARB or ARGs to different geographic locations and to other species including humans and domestic animals. In particular, the migratory capacity of several gull species such as the Franklin’s gull (Leucophaeus pipixcan), migrating across America from Canada to Chile, could result in the dissemination of ARB and ARGs over extensive geographic areas, dispersing AMR from regions with high levels of AMR to less affected areas (Báez et al., 2015; Dolejska and Literak, 2019). Gulls are also present in most urban and rural environments, and their feces are extensively dispersed in the environment (Bonnedahl and Järhult, 2014). Several studies have detected ARGs in gulls (Oravcova et al., 2017; Ahlstrom et al., 2019b; Haenni et al., 2020). In particular, AMR has been detected in several species of seagulls, which have large breeding distributions in urban areas and feed on human waste (Bonnedahl et al., 2015; Stedt et al., 2015; Ahlstrom et al., 2019a). Thus, gulls have been suggested as potential reservoirs of ARB and ARGs, although evidence proving their role as reservoirs has not been provided (Radhouani et al., 2010; Aberkane et al., 2015; Merkeviciene et al., 2018).
In this scoping review, we summarized the current knowledge regarding the global dissemination of ARB and ARGs among gulls and assess whether there is evidence supporting the assumption that gulls can act as reservoirs of AMR. In particular, we aim to provide a comprehensive overview of the geographic location where ARB and ARGs have been found in gulls, the gull and bacteria species involved, as well as the antibiotic families and genes detected. To discuss the public health implications of gulls, we summarized whether bacteria of critical importance according to WHO have been detected in gulls. We also assessed the number of publications that had either identified the origin of AMR found in gulls or tested and concluded that gulls can disperse AMR across the landscape or to other species. Based on this current evidence, we discussed several recommendations aiming to improve our understanding of the role of gulls in the dissemination of AMR.
Materials and Methods
We performed a scoping review following the Preferred Reporting Items for Systematic Reviews and Meta-Analyses extension for Scoping Reviews (PRISMA-ScR) checklist (Tricco et al., 2018; Supplementary Table 1). All authors defined research questions, objectives, search strategy, and inclusion/exclusion criteria through previous discussions.
Search Strategy
The search was performed in PubMed, Scopus, and Web of Science databases using three general queries: (antibiotic resist∗ OR antimicrobial resist∗), (Escherichia OR Klebsiella OR Staphylococcus OR Enterococcus OR Enterobacter∗ OR Salmonella OR Pseudomonas), and (bacteria). Each of them was merged with (marine bird∗ OR aquatic bird∗ OR gull∗ OR Larus). Details of the search strategy are available as an additional file (Supplementary Table 2). Visualization, duplicate removal, and storing collected data were performed in Microsoft Excel.
Eligibility Criteria
We aimed to identify peer-reviewed studies on AMR in different wild species of gulls (i.e., seagulls) showing the presence and/or potential transmission of ARB and ARGs. Thus, we included only studies providing at least one of the following information: (i) wild gull species where ARB was recovered, (ii) phenotypic resistance to specific antibiotics in bacteria isolated from gulls, and/or (iii) ARGs identified in bacteria isolated from gulls. There were no restrictions related to the year of publication or geographical location. Any type of reviews or studies including in vivo experiments, samples of gulls from rehabilitation centers, or containing previously published data were excluded. Details of all inclusion and exclusion criteria are provided in Supplementary Table 3.
Identification and Screening of Articles
After the removal of duplicates, we identified a total of 3,475 articles published from 1964 to January 2021, including 3 additional references that were identified from reading these papers. Pre-selection by title and abstract reduced to 227 articles for full-text analysis, and 90 fulfilled the preestablished criteria and were included in the final analysis (Figure 1). The remaining 140 articles did not fit our inclusion criteria as they did not include gull samples, did not present AMR information/data, data from gulls were previously published, the study included experimental infection, the study was performed on captive gulls or in rehabilitation centers, the study sampling was conducted postmortem, or the full text of the article was not available.
Data Extraction
Extracted data were independently performed by three authors (DZG, MSC, and ZRS) and verified by other authors. Disagreements were resolved through discussion. The obtained data were entered into a Microsoft Excel template adapted from a previous study (Supplementary Table 4; Salgado-Caxito et al., 2021). This file included the title of the article, authorship, year of publication, gull species included in the study and whether the species was migratory or not, the number of sampled individuals, the bacteria species studied, the number of recovered isolates, the antimicrobial susceptibility tests performed, the name and family of the antibiotics tested, and the molecular typing used (i.e., PCR, sequencing, and whole-genome sequencing) when available. To assess the current knowledge on the role of gulls as reservoirs of ARB or ARGs, we also specifically extracted from studies (i) whether the study compared gulls to other animals in the area; (ii) whether the study identified the origin (e.g., anthropogenic source) of the ARB or ARGs found; (iii) if gulls were sampled more than once, particularly in both areas of migration (origin and destination); (iv) if molecular typing of ARB was performed; and (iv) if an individual follow-up and sampling of gulls were performed, along with the method used.
Statistical Analysis
We estimated the proportion of studies filling a given criteria (e.g., studies identifying the presence of ARGs or the origin of ARB) using R. 3.1.6 (R Development Core Team).
Results
Geographic Locations and Gull and Bacteria Species Studied
Our scoping review identified 90 articles published between 1981 and 2020, although only 22% of these studies were published before 2010. The number of studies published on gulls increased from 1 in 1981 to 10 per year in 2020 and peaked in 2017 with 12 articles (Figure 2B). Studies were conducted in gulls from all five continents, but the majority of publications were made in Europe (58%) followed by North America (19%) (Figure 2A). Studies were conducted in a total of 31 countries, with high-income countries such as the United States (17%), Portugal (12%) and Spain (10%) conducting the highest number of studies (Figure 2C). In contrast, in middle- and low-income countries, few publications were conducted (Morocco, 1%; South Africa, 1%; Bangladesh, 1%).
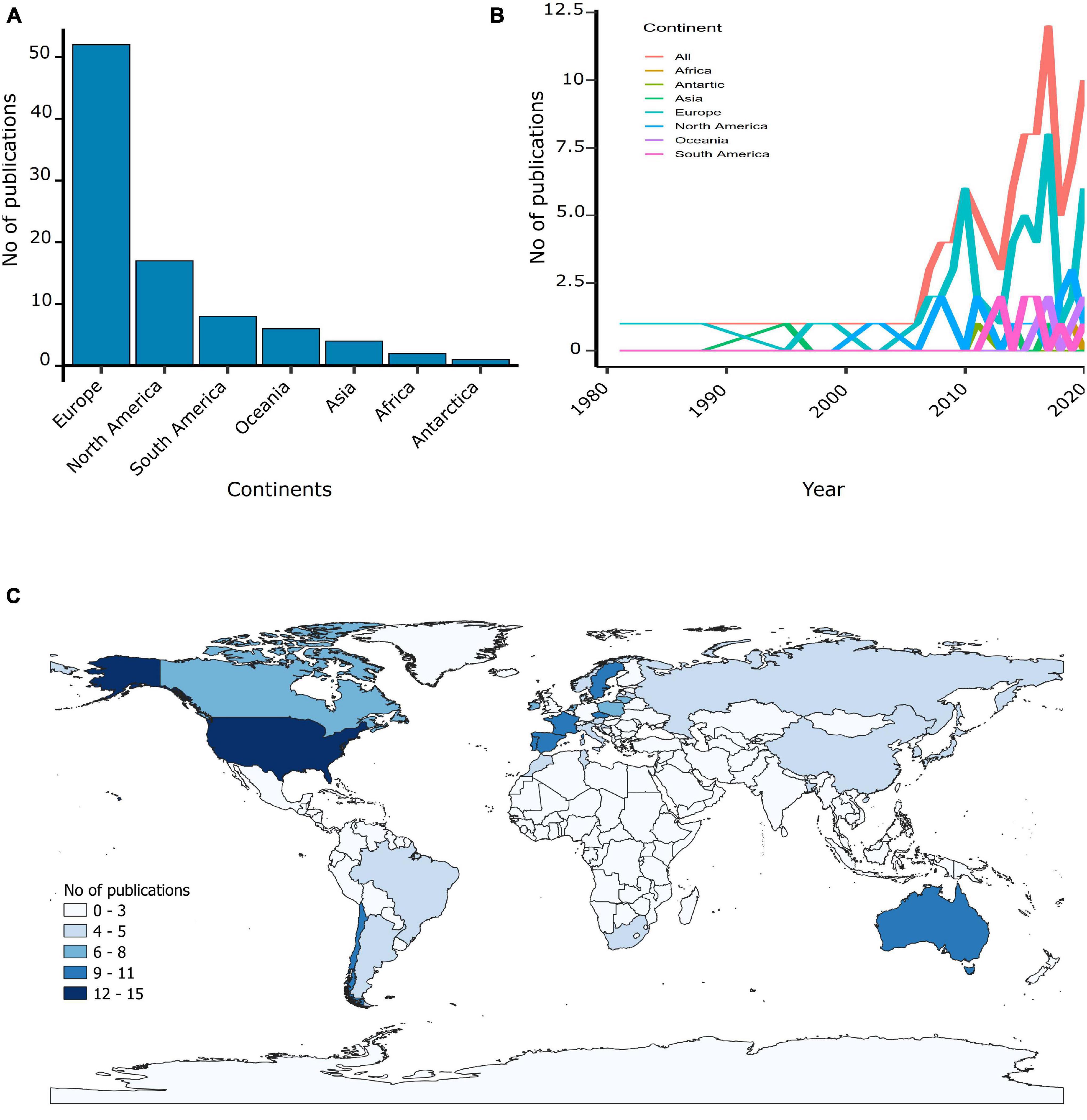
Figure 2. Geographical locations of the AMR studies found in gulls. (A) Number of publications per continents. (B) Number of publications per continent over the 1980–2020 period. (C) Number of publications of AMR in gulls per country in gradient.
From 100 species of gulls known (IUCN, 2021), ARB or ARGs were recovered from 23 species. Most gulls studied (74%) were migratory species. The number of studies per gull species was highly heterogeneous (Figure 3B). The majority of studies focused on the herring gull (Larus argentatus, 26%), followed by the laughing gull (Chroicocephalus ridibundus, 23%) and the yellow-legged gull (Larus michahellis, 19%) (Figure 3A). These three species are widely distributed in the northern hemisphere.
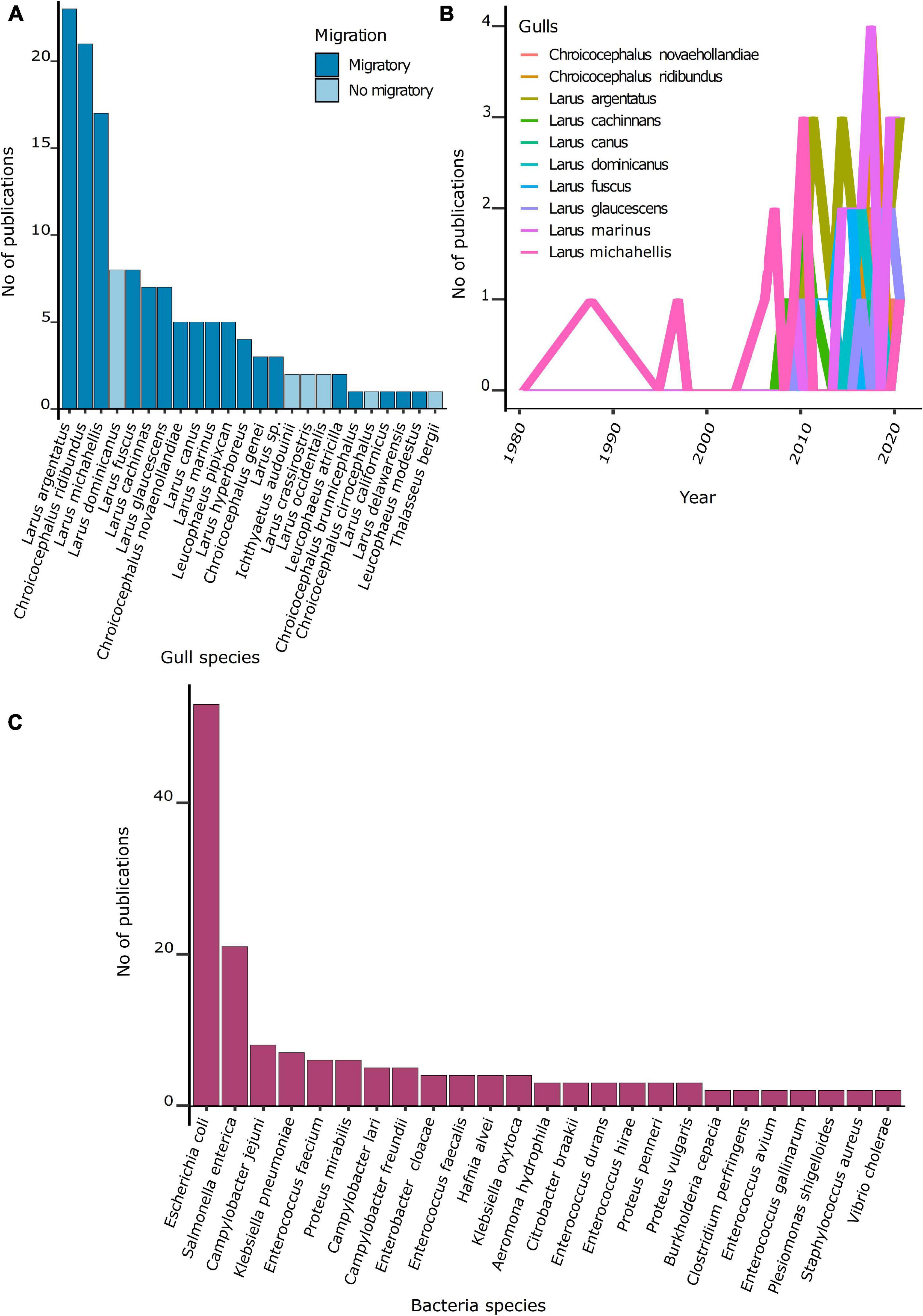
Figure 3. Number of publications per gull and bacteria species. (A) Number of publications per gull species. (B) Number of publications per gull species over the 1980–2020 period. (C) Number of publications per bacteria species.
Among the 90 studies, 49 ARB species were recovered from gulls. Most studies focused on Escherichia coli (59%), Salmonella enterica (23%), Campylobacter jejuni (8%), and Klebsiella pneumoniae (8%) (Figure 3C). The temporal trend of publications showed that after 2008, most studies have focused on E. coli.
Antibiotic Susceptibility in Bacteria From Gulls
Screening of ARB using selective media supplemented with antibiotics before antimicrobial susceptibility tests was performed in 43% of studies. Seventeen percent of studies performed antibiotic susceptibility tests after isolation in non-supplemented media. The remaining 40% of the studies did not present the methodology for recovering isolates. Forty-one publications had information about the number of positive individuals, and 68% of those studies were conducted in Europe. The highest proportion of animals harboring bacteria resistant to at least one antibiotic (referred as positive animals) was estimated in one study in Africa (70%) that included less than 50 individuals. The highest proportion of positive gulls was observed among Larus dominicanus (100%), while Proteus mirabilis showed the highest proportion of positive individuals (27.9%) (Table 1). Given the high heterogeneity in susceptibility methods and antibiotics tested, a comparison of ARB prevalence across studies, defined as the number of positive individuals over the total of sampled animals, could not be performed. Regarding the methodology used to test susceptibility, 68% of studies confirmed phenotypic resistance using the disk diffusion method (CLSI, 2018). Overall, resistance to 79 antibiotic agents from 21 families was tested (Supplementary Table 5), including antibiotics used in human medicine such as beta-lactams (i.e., penicillin, cephalosporins, and carbapenems), tetracyclines, fluoroquinolones, sulfonamides, aminoglycosides, nitrofurans, macrolides, monobactam, polypeptides, glycopeptides, and lincosamides. More than 50% of studies reported at least one bacterial isolate resistant to tetracycline (58%) and ampicillin (52%), followed by chloramphenicol (47%), streptomycin (44%), trimethoprim–sulfamethoxazole (38%), gentamicin (36%), nalidixic acid (35%), and ciprofloxacin (32%) (Figure 4A). In particular, broad-spectrum beta-lactams used in human medicine such as amoxicillin with clavulanic acid and ceftazidime were reported in 20% of publications.
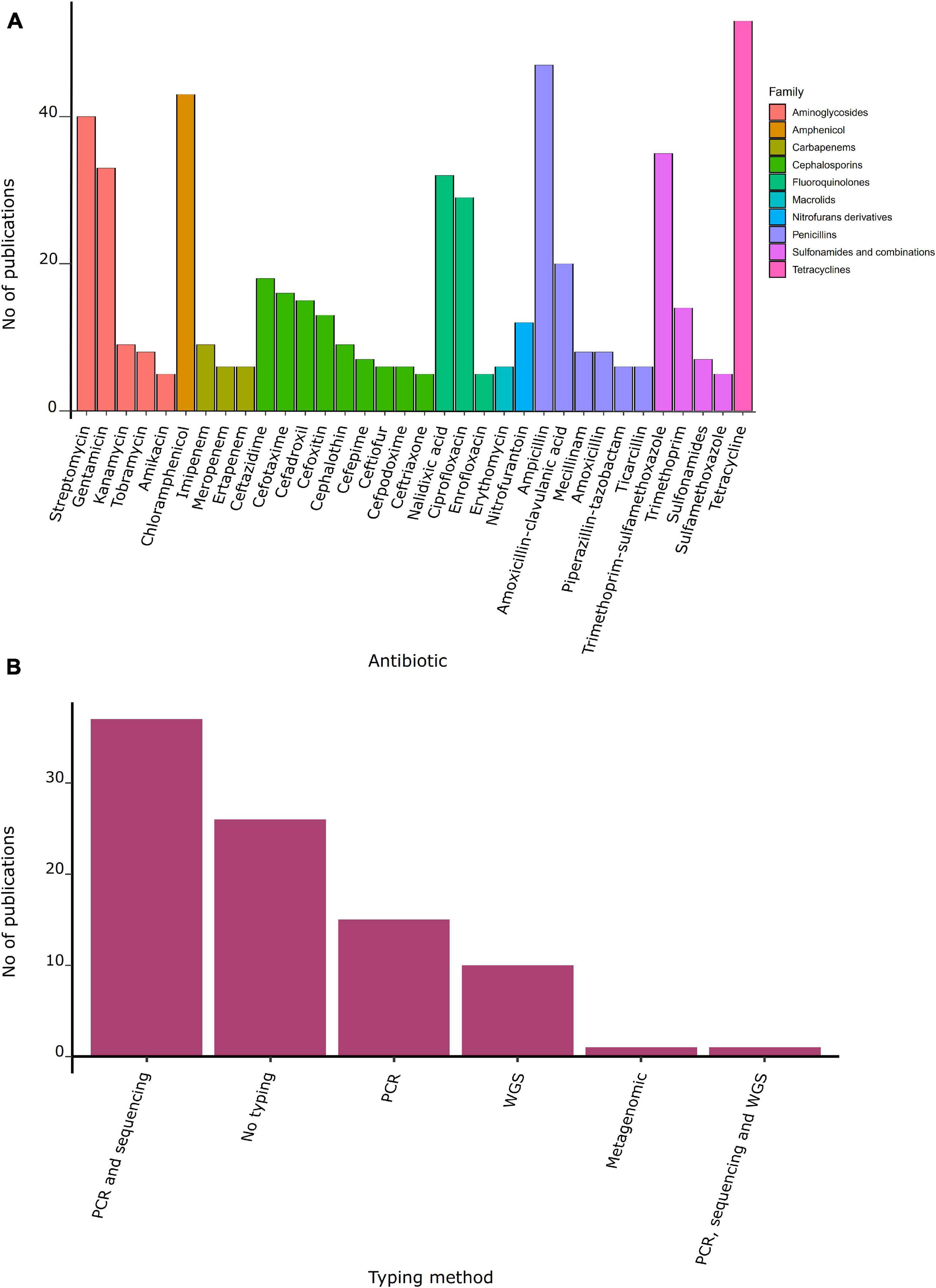
Figure 4. Antibiotic resistance and molecular method used. (A) Number of publications by family of antibiotic. (B) Number of publications by typing method.
Among the four antimicrobial-resistant pathogens considered as a “critical priority” by the WHO (WHO, 2017), all were tested at least once in the reviewed studies. Third-generation cephalosporin-resistant Enterobacterales from gulls were the most reported (41%), followed by carbapenem-resistant Enterobacterales (10%) (Table 2). Among “high-priority” pathogens, Campylobacter spp. (6%) and Salmonella spp. (8%) both resistant to fluoroquinolones were the most identified. No “medium-priority” pathogen has been recovered from gulls.
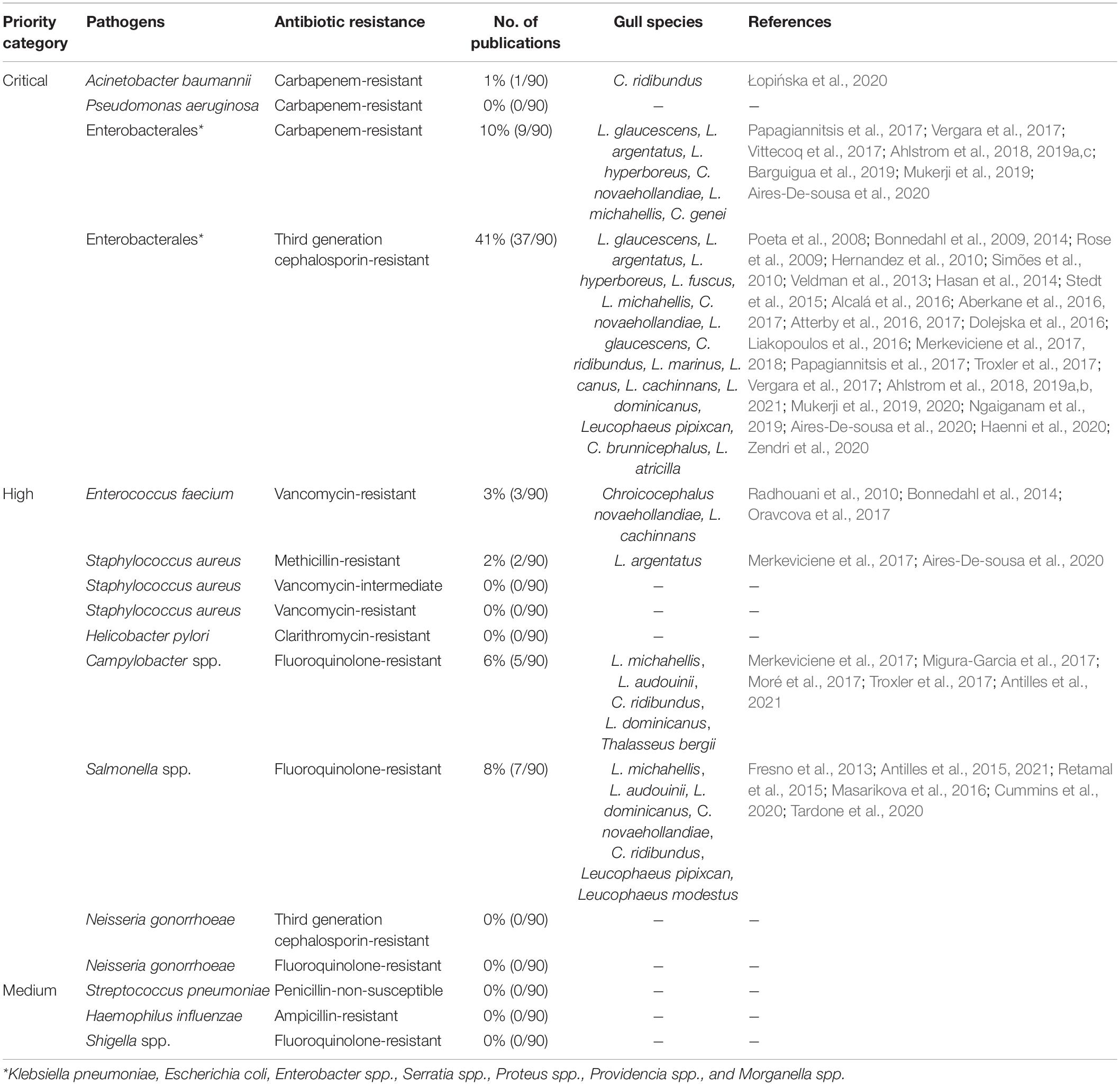
Table 2. ARB of the “Global Priority Pathogens” list of the World Health Organization isolated from gulls reported between 1981 and 2020.
Molecular Characterization of ARGs in Gulls
ARGs were reported in 70% of studies conducted in gulls (Tables 3, 4). Mobile genetic elements (MGE) were identified in 43% of studies, with 35 studies confirming that ARGs were inserted on an MGE. Sixteen percent of studies detected ARGs using PCR alone, or in combination with sequencing (43%). Only 8% of studies characterized bacteria by whole-genome sequencing, and one study used a metagenomic approach (Figure 4B).
Most studies detecting ARGs focused on beta-lactamase genes including extended-spectrum beta-lactamases (ESBL), AmpC-type beta-lactamases, and carbapenemases, which were identified in all continents but Antarctica (Table 3). Among these beta-lactamases, ESBL were the most identified genes, evenly distributed across continents, particularly the genotype blaCTX–M. Studies detected blaCTX–M–14 and blaCTX–M–15 in Asia, Europe, North and South America, and Oceania. Likewise, blaCTX–M–55 was reported in all these continents with the exception of South America. Beta-lactamases blaCMY–2 (AmpC) were reported in Europe, North America, and Oceania, and blaOXA–48 (carbapenemase) were reported in Africa, Europe, North America, and Oceania (Table 3). Of the 15 studies that found AmpC-type beta-lactamases, 7 identified that they were inserted on an MGE, 2 identified them on the bacterial core genome, 2 detected both chromosomal and acquired AmpC, and 4 studies did not identify the location of the AmpC gene. Genes conferring resistance to other antibiotics such as fluoroquinolones, aminoglycosides, sulfonamides along with trimethoprim, polypeptides, tetracyclines, chloramphenicol, macrolides, streptogramins, glycopeptides, fosfomycin, and rifamycin were reported in 59 studies (Table 4). Asia only reported beta-lactam resistance genes.
Origin of AMR in Gulls
Only 19% of studies suggested a potential origin for the ARB or ARGs detected among gulls. Landfill (41%), places close to gulls nesting, and/or resting areas with high human density (29%), sewage effluents (29%), and contaminated water (6%) were suspected. Suspicions were based on potential contamination sources around the sampling area. However, only one study (Masarikova et al., 2016) carried out sampling to verify whether the gulls acquired the bacteria from a specific contamination source, comparing bacteria from gulls to bacteria isolated from sewage water near their nesting sites. The same AMR phenotypic profiles were obtained in both sample types, and pulsed-field gel electrophoresis (PFGE) detected the same AMR profiles in bacterial clones from wastewater and gulls.
Evidence of Gulls Acting as Reservoirs of ARB or ARGs
Two studies (2%) tagged gulls in both the origin and final movement areas to identify whether they were capable of spreading AMR across the landscape (Palmgren et al., 2006; Ahlstrom et al., 2019a). Ahlstrom et al. (2019a) sampled individual gulls at different periods of time obtaining fecal samples at a landfill and in places where humans and seagulls gathered. Satellite telemetry was used to monitor individuals for up to 3 months, and whole-genome sequencing of bacteria was used to compare E. coli isolates between different locations. Their results showed that the prevalence and genetic typing of AMR isolates were highly similar between gulls and a landfill. Palmgren et al. (2006) ringed gulls and sampled 1,047 individuals for up to 3 years. This study failed to detect long-term carriage of antibiotic-resistant Salmonella since all positive individuals were negative during the sampling 2 months later.
Discussion
AMR has been detected in resident and migratory gulls worldwide for more than a decade (Fenlon, 1981; Tsubokura et al., 1995; Smith et al., 2002). However, the role of gulls as reservoirs (i.e., having the capacity to disperse and transmit AMR to other species) remains unknown. Our review identified 90 studies on AMR in wild gulls. AMR has been widely detected across all continents including in 23 of 100 species of gulls (IUCN, 2021), 49 bacteria species with 9 of 13 ARB classified as critical priority for human health (WHO, 2017), ARGs from 13 classes, and 47 antibiotic types. Our results show that, with the exception of China, studies in middle- and low-income countries are rare. Similarly, most studies have focused on a few species of gulls from Europe (e.g., L. argentatus and C. ridibundus) and most on E. coli and Salmonella spp. Despite ARB and ARGs being widely detected in gulls, our analyses showed that the origin of these AMR remains unknown in 81% of studies, and only two studies followed gulls across time (for up to 3 years), but none has been able to prove that gulls were reservoirs (Palmgren et al., 2006; Ahlstrom et al., 2019a). Therefore, our review highlights the need to increase surveillance of AMR in gulls and design innovative studies aiming to assess their role as reservoirs, which can have major implications for public and conservation measures to limit the global spread of AMR in aquatic systems.
The detection of AMR in gulls across all continents, including critically important antibiotic-resistant pathogens such as ESBL and carbapenemase-producing E. coli and S. enterica, illustrates the potential of gulls to participate in the alarming global spread of AMR (Dolejska et al., 2016; Ahlstrom et al., 2019c; Aires-De-sousa et al., 2020; Cummins et al., 2020). The migratory capacity of gulls makes them an ideal host to spread ARB and ARGs across landscapes and ecosystems. For example, Ahlstrom et al. (2021) reported that gulls of the Larus genus, including L. argentatus, can migrate 3,000 km over a week, Larus fuscus can migrate from Europe to Africa (Kilpi and Saurola, 1984), while Leucophaeus pipixcan migrates from North to South America (Hernandez et al., 2013; Barbieri et al., 2016). In contrast, other gull species such as L. dominicanus are resident but also carry ESBL-resistant E. coli with ARGs genes such as blaCTX–M and blaSHV and aminoglycoside-resistant Salmonella enteritidis with str genes (Liakopoulos et al., 2016; Toro et al., 2016). Although these species might not necessarily contribute to the long-range dispersal of AMR, they could participate in local transmission to other species and humans (Vigo et al., 2011; Retamal et al., 2015; Toro et al., 2016). Overall, this review highlights that gulls are at least sentinels of ARB and ARGs spreading in the environment, calling for future research in species and countries where AMR has not yet been studied. In particular, environmental and animal health national and international authorities should consider gulls in the surveillance of AMR within the environment.
Although AMR is widely spread among gulls, there are almost no data on the origin of the observed ARB and ARGs. In fact, less than 20% of studies included in this review mentioned potential sources of AMR contamination. Given that AMR has exponentially increased with antibiotic use in humans and livestock and several gull species feed on human and agricultural waste, most studies suspect a human origin including landfills, places close to gulls nesting, and/or resting areas that have a high human density, sewage effluents, and contaminated water (Bonnedahl et al., 2014; Atterby et al., 2016; Mukerji et al., 2019; Ahlstrom et al., 2021). This is consistent with the overall assumption that wildlife becomes contaminated with AMR from anthropogenic sources in studies suggesting transmission in areas where wildlife lives and feeds (Dolejska and Literak, 2019). However, no study has fully proven the origin of AMR in gulls, and other environmental factors such as co-selection with heavy metals and microplastics can also generate AMR (Gullberg et al., 2014; Dong et al., 2021). In our review, only one study sampled a potential contamination source to identify the origin of AMR find in gulls, showing that isolates from wastewater and gulls had the same macrorestriction profiles (Masarikova et al., 2016). One possible explanation for the small number of studies trying to identify the origin of AMR in gulls could be that no standard sampling protocol or specific criteria are available to fully determine the origin. Alternatively, logistical challenges such as collecting both wildlife, domestic animals, and human environments at the same time could limit the realization of these studies. Future research could follow methodologies used by studies performed on bacteria susceptible to antibiotics and other wildlife. For example, Nelson et al. (2008) characterized E. coli from gulls, garbage, and sewage by ribotyping, finding isolates with > 90% similarity in the band patterns between gulls and sewage. However, this study was not included in this review because it did not test for ARB or ARGs. Similarly, other studies have simultaneously sampled domestic animals and wildlife where contact between species can be frequent (e.g., small-scale farms) to assess potential cross-species transmission of ESBL-E. coli (Benavides et al., 2021). Although challenging, identifying the origin of AMR in gulls is essential when planning preventive strategies to limit the spread of AMR in natural ecosystems. Seagulls are characterized by being ubiquitous in most urban and rural environments, and many of them are migratory, so it is assumed that gulls may disperse ARB and ARGs between countries or even continents. Despite this assumption, only two studies included in this review performed longitudinal samplings to test the long-term carriage of ARB or ARGs in gulls (Palmgren et al., 2006; Ahlstrom et al., 2019a), requiring further research to identify their implication as reservoirs of AMR.
The detected ARB and ARGs found in gulls have major implications for both animal and human health. E. coli was the most common bacterial species reported followed by Salmonella, similarly to other wildlife species (Vittecoq et al., 2016). Both bacterial species are important for public health and are considered a critical priority for human and animal health (Vittecoq et al., 2016; WHO, 2017). E. coli and Salmonella spp. can also be found at equilibrium as commensal bacteria, so the impact of these bacteria of gull’s health is unknown. Other reported pathogens found in gulls, such as Campylobacter spp. and Klebsiella pneumoniae, are considered as zoonotic pathogens and could represent a threat to human health. Global priority antibiotic-resistant pathogens for human and animal health were increasingly reported among gulls after 2008, particularly those considered as “critical” (WHO, 2017). Broad-spectrum antimicrobial therapies are commonly used to treat bacterial infections in both humans and animals (Bush and Jacoby, 2010). The widespread detection of third-generation cephalosporin-resistant Enterobacterales in addition to resistance to other important antimicrobials, such as carbapenems and fluoroquinolones, could compromise the effective treatment rates representing an important threat to public and veterinary health.
The higher detection of antibiotic-resistant enterobacteria could be explained by the relatively easier collection of fecal samples compared to capturing and sampling gulls to detect other pathogens (e.g., blood bacteria). Thus, the absence of other global-priority ARB in current studies such as Pseudomonas aeruginosa carbapenem-resistant (critical priority), Staphylococcus aureus vancomycin-intermediate or -resistant (high priority), and Shigella spp. fluoroquinolone-resistant (medium priority) could reflect a lack of research and not necessarily that these bacteria are not circulating among gulls. In fact, one study using a metagenomic approach found 31 previously undescribed ARGs, while another detected more than 70 bacterial species and 24 ARGs (Martiny et al., 2011; Merkeviciene et al., 2017). This review identified a high diversity of ARGs including those implicated in bacterial infections of humans and animals such as ESBL and carbapenemases (Bevan et al., 2017; Li et al., 2019). The presence of ARGs inserted in MGE could facilitate the spread of these resistance genes within gulls and between humans and other animals (Loayza et al., 2020). Our review also shows a wide diversity of bacterial clones and ARGs found in gulls. Whole-genome sequencing for bacterial typing was used in 13% of studies since 2011. Thus, the more widespread use of this technique in the following years could increase the detection of ARB clones and ARGs in gulls.
Our review showed that North America and Europe had the most diverse molecular diversity among ARB, which could be associated with more available molecular typing techniques compared to low-income countries. For example, ESBL- E. coli ST131, previously associated with nosocomial infections in humans, has been identified in gulls mainly from the United States (Bonnedahl et al., 2014; Ahlstrom et al., 2018, 2019a, 2021) and Portugal (Simões et al., 2010; Vredenburg et al., 2014; Varela et al., 2015). Despite fewer information available, ST131 has also been reported in gulls from low- and middle-income countries (LMICs) such as Bangladesh (Hasan et al., 2014). Future research should also evaluate the pathogenic potential of the detected ARB using whole-genome sequencing to detect virulence factors and other pathogenic genetic material (e.g., biofilms).
Conclusion and Future Directions
Our review identified an increasing interest in ARB and ARGs among gulls in the last decade, although there is a considerable lack of information in LMICs, particularly regarding migratory species. Despite the widespread detection and high diversity of ARB and ARGs worldwide, there is no evidence that gulls act as reservoirs of ARB and ARGs. Furthermore, most of the studies could not demonstrate whether ARB and ARGs in gulls came from anthropogenic sources. Finally, we could not compare ARB or ARGs prevalence across studies due to their heterogeneity in the results and methodologies to assess AMR.
Knowledge gaps identified in this review can be overcome by future research. First, the use of whole-genome sequencing combined with sampling across different species could help assessing cross-species transmission between gulls and humans, domestic animals, or other wild species that do not usually interact with humans but share nesting sites with gulls (e.g., penguins). Secondly, future research should identify if environmental factors such as plastic and heavy metal pollution are also selecting ARB and ARGs in gulls independently of contact with humans. Thirdly, the clinical relevance and conservation implications of the detected ARB for gull’s health require further investigation. In particular, there is no evidence that the observed bacteria cause any pathogenicity to the studied gulls nor complicate treatment of gulls with antibiotics in rehabilitation centers. Finally, innovative techniques such as satellite tracking and collaborations across research teams in different countries where gulls migrate (e.g., Canada to Chile for the Franklin’s gulls) could help elucidate whether gulls are spreading ARB and/or ARGs across countries and continents during their migration.
Author Contributions
JB and DZ-G: conceptualization. DZ-G: data curation. JB, DZ-G, ZR-S, and MS-C: formal analysis, investigation, and methodology. JB: funding acquisition, project administration, resources, software, supervision, validation, and visualization. DZ-G, JB, ZR-S, MS-C, CT, and PP: writing—original draft and writing—review and editing. All authors have read and agreed to the published version of the manuscript.
Funding
This work was funded by the National Agency for Research and Development (ANID) FONDECYT Iniciación 11181017, awarded to JB, the National Agency for Research and Development (ANID) Beca Doctorado Nacional No. 21201076, awarded by ZR-S, the Concurso de Apoyo a la Realización de Actividades de Investigación en Etapa de Pregrado (APP 2019-2) UNAB, awarded to DZ-G, and the ANID Millennium Science Initiative/Millennium Initiative for Collaborative Research on Bacterial Resistance, MICROB-R, NCN17_081.
Conflict of Interest
The authors declare that the research was conducted in the absence of any commercial or financial relationships that could be construed as a potential conflict of interest.
Publisher’s Note
All claims expressed in this article are solely those of the authors and do not necessarily represent those of their affiliated organizations, or those of the publisher, the editors and the reviewers. Any product that may be evaluated in this article, or claim that may be made by its manufacturer, is not guaranteed or endorsed by the publisher.
Acknowledgments
We thank members of The Monkey Lab (@themonkey_lab) for the useful comments.
Supplementary Material
The Supplementary Material for this article can be found online at: https://www.frontiersin.org/articles/10.3389/fmicb.2021.703886/full#supplementary-material
References
Aberkane, S., Compain, F., Barraud, O., Ouédraogo, A. S., Bouzinbi, N., Vittecoq, M., et al. (2015). Non-O1/non-O139 Vibrio cholerae avian isolate from France cocarrying the blaVIM-1 and blaVIM-4 genes. Antimicrob. Agents Chemother. 59, 6594–6596. doi: 10.1128/AAC.00400-15
Aberkane, S., Compain, F., Decré, D., Dupont, C., Laurens, C., Vittecoq, M., et al. (2016). High prevalence of SXT/R391-related integrative and conjugative elements carrying blaCMY-2 in Proteus mirabilis isolates from gulls in the South of France. Antimicrob. Agents Chemother. 60, 1148–1152. doi: 10.1128/AAC.01654-15
Aberkane, S., Compain, F., Decré, D., Pantel, A., Vittecoq, M., Solassol, J., et al. (2017). Persistence of bla CMY-2 -producing Proteus mirabilis in two gull colonies at a 1-year interval in Southern France. J. Glob. Antimicrob. Resist. 9, 138–140. doi: 10.1016/j.jgar.2017.05.005
Ahlstrom, C. A., Bonnedahl, J., Woksepp, H., Hernandez, J., Olsen, B., and Ramey, A. M. (2018). Acquisition and dissemination of cephalosporin-resistant E. coli in migratory birds sampled at an Alaska landfill as inferred through genomic analysis. Sci. Rep. 8:7361. doi: 10.1038/s41598-018-25474-w
Ahlstrom, C. A., Bonnedahl, J., Woksepp, H., Hernandez, J., Reed, J. A., Tibbitts, L., et al. (2019a). Satellite tracking of gulls and genomic characterization of faecal bacteria reveals environmentally mediated acquisition and dispersal of antimicrobial-resistant Escherichia coli on the Kenai Peninsula, Alaska. Mol. Ecol. 28, 2531–2545. doi: 10.1111/mec.15101
Ahlstrom, C. A., Ramey, A. M., Woksepp, H., and Bonnedahl, J. (2019b). Early emergence of mcr- 1-positive Enterobacteriaceae in gulls from Spain and Portugal. Environ. Microbiol. Rep. 11, 669–671. doi: 10.1111/1758-2229.12779
Ahlstrom, C. A., Ramey, A. M., Woksepp, H., and Bonnedahl, J. (2019c). Repeated detection of carbapenemase-producing Escherichia coli in gulls inhabiting Alaska. Antimicrob. Agents Chemother. 63, e758–e719. doi: 10.1128/AAC.00758-19
Ahlstrom, C. A., van Toor, M. L., Woksepp, H., Chandler, J. C., Reed, J. A., Reeves, A. B., et al. (2021). Evidence for continental-scale dispersal of antimicrobial resistant bacteria by landfill-foraging gulls. Sci. Total Environ. 764:144551. doi: 10.1016/j.scitotenv.2020.144551
Aires-De-sousa, M., Fournier, C., Lopes, E., de Lencastre, H., Nordmann, P., and Poirel, L. (2020). High colonization rate and heterogeneity of ESBL-and carbapenemase-producing enterobacteriaceae isolated from gull feces in Lisbon, Portugal. Microorganisms 8, 1–12. doi: 10.3390/microorganisms8101487
Alcalá, L., Alonso, C. A., Simón, C., González-Esteban, C., Orós, J., Rezusta, A., et al. (2016). Wild birds, frequent carriers of extended-spectrum β-lactamase (ESBL) producing Escherichia coli of CTX-M and SHV-12 types. Microb. Ecol. 72, 861–869. doi: 10.1007/s00248-015-0718-0
Alroy, K., and Ellis, J. C. (2011). Pilot study of antimicrobial-resistant Escherichia coli in herring gulls (Larus argentatus) and wastewater in the Northeastern United States. J. Zoo Wildl. Med. 42, 160–163. doi: 10.1638/2010-0130.1
Antilles, N., García-Bocanegra, I., Alba-Casals, A., López-Soria, S., Pérez-Méndez, N., Saco, M., et al. (2021). Occurrence and antimicrobial resistance of zoonotic enteropathogens in gulls from southern Europe. Sci. Total Environ. 763:143018. doi: 10.1016/j.scitotenv.2020.143018
Antilles, N., Garcia-Migura, L., Joensen, K. G., Leekitcharoenphon, P., Aarestrup, F. M., Cerdà-Cúellar, M., et al. (2015). Audouins gull, a potential vehicle of an extended spectrum ß-lactamase producing Salmonella Agona. FEMS Microbiol. Lett. 362, 1–4. doi: 10.1093/femsle/fnu039
Atterby, C., Börjesson, S., Ny, S., Järhult, J. D., Byfors, S., and Bonnedahl, J. (2017). ESBL-producing Escherichia coli in Swedish gulls—a case of environmental pollution from humans? PLoS One 12:e0190380. doi: 10.1371/journal.pone.0190380
Atterby, C., Ramey, A. M., Hall, G. G., Järhult, J., Börjesson, S., and Bonnedahl, J. (2016). Increased prevalence of antibiotic-resistant E. coli in gulls sampled in Southcentral Alaska is associated with urban environments. Infect. Ecol. Epidemiol. 6:32334. doi: 10.3402/IEE.V6.32334
Báez, J., Hernández-García, M., Guamparito, C., Díaz, S., Olave, A., Guerrero, K., et al. (2015). Molecular characterization and genetic diversity of ESBL-producing escherichia coli colonizing the migratory Franklin’s Gulls (Leucophaeus pipixcan) in Antofagasta, North of Chile. Microb. Drug Resist. 21, 111–116. doi: 10.1089/mdr.2014.0158
Barbieri, E., Alberto Goncalves, C., and Francisco Silveira, L. D. A. (2016). First occurrence of Franklin’s Gull (Leucophaeus pipixcan, Wagler 1831) in Ilha Comprida beach, Southern coast of São Paulo state, Brazil. Pan Am. J. Aquat. Sci. 11:159.
Barguigua, A., Rguibi Idrissi, H., Nayme, K., and Timinouni, M. (2019). Virulence and antibiotic resistance patterns in E. coli, Morocco. Ecohealth 16, 570–575. doi: 10.1007/s10393-019-01404-8
Benavides, J. A., Salgado-Caxito, M., Opazo-Capurro, A., González, M. P., Piñeiro, A., Otto, M. M., et al. (2021). ESBL-producing Escherichia coli carrying CTX-M genes circulating among livestock, dogs, and wild mammals in small-scale farms of central Chile. Antibiotics 10:510. doi: 10.3390/antibiotics10050510
Benavides, J. A., Shiva, C., Virhuez, M., Tello, C., Appelgren, A., Vendrell, J., et al. (2018). Extended-spectrum beta-lactamase-producing Escherichia coli in common vampire bats Desmodus rotundus and livestock in Peru. Zoonoses Public Health 65, 454–458. doi: 10.1111/zph.12456
Bevan, E. R., Jones, A. M., and Hawkey, P. M. (2017). Global epidemiology of CTX-M β-lactamases: temporal and geographical shifts in genotype. J. Antimicrob. Chemother. 72, 2145–2155. doi: 10.1093/jac/dkx146
Bonnedahl, J., and Järhult, J. D. (2014). Antibiotic resistance in wild birds. Ups. J. Med. Sci. 119, 113–116. doi: 10.3109/03009734.2014.905663
Bonnedahl, J., Drobni, M., Gauthier-Clerc, M., Hernandez, J., Granholm, S., Kayser, Y., et al. (2009). Dissemination of Escherichia coli with CTX-M type ESBL between humans and yellow-legged gulls in the South of France. PLoS One 4:e5958. doi: 10.1371/journal.pone.0005958
Bonnedahl, J., Drobni, P., Johansson, A., Hernandez, J., Melhus, Å, Stedt, J., et al. (2010). Characterization, and comparison of human clinical and black-headed gull (Larus ridibundus) extended-spectrum β-lactamase-producing bacterial isolates from Kalmar, on the southeast coast of Sweden. J. Antimicrob. Chemother. 65, 1939–1944. doi: 10.1093/jac/dkq222
Bonnedahl, J., Hernandez, J., Stedt, J., Waldenström, J., Olsen, B., and Drobni, M. (2014). Extended- spectrum β-lactamases in escherichia coli and Klebsiella pneumoniae in Gulls, Alaska, USA. Emerg. Infect. Dis. 20, 897–899. doi: 10.3201/eid2005.130325
Bonnedahl, J., Stedt, J., Waldenström, J., Svensson, L., Drobni, M., and Olsen, B. (2015). Comparison of extended-spectrum β-lactamase (ESBL) CTX-M genotypes in franklin gulls from Canada and Chile. PLoS One 10:e0141315. doi: 10.1371/journal.pone.0141315
Bush, K., and Jacoby, G. A. (2010). Updated functional classification of β-lactamases. Antimicrob. Agents Chemother. 54, 969–976. doi: 10.1128/AAC.01009-09
Carroll, D., Wang, J., Fanning, S., and Mcmahon, B. J. (2015). Antimicrobial resistance in wildlife: implications for public health. Zoonoses Public Health 62, 534–542. doi: 10.1111/zph.12182
Čížek, A., Dolejská, M., Karpíšková, R., Dědičová, D., and Literák, I. (2007). Wild black-headed gulls (Larus ridibundus) as an environmental reservoir of Salmonella strains resistant to antimicrobial drugs. Eur. J. Wildl. Res. 53, 55–60. doi: 10.1007/s10344-006-0054-2
CLSI (2018). Performance Standards for Antimicrobial Susceptibility Testing, 28th Edn. Wayne, PA: Clinical and Laboratory Standards Institute.
Cummins, M. L., Sanderson-Smith, M., Newton, P., Carlile, N., Phalen, D. N., Maute, K., et al. (2020). Whole-genome sequence analysis of an extensively drug-resistant Salmonella enterica Serovar Agona isolate from an Australian silver gull (Chroicocephalus novaehollandiae) reveals the acquisition of multidrug resistance plasmids. mSphere 5, 1–14. doi: 10.1128/msphere.00743-20
Dolejska, M., and Literak, I. (2019). Wildlife is overlooked in the epidemiology of medically important antibiotic-resistant bacteria. Antimicrob. Agents Chemother. 63, 1–5. doi: 10.1128/AAC.01167-19
Dolejská, M., Bierošová, B., Kohoutová, L., Literák, I., and Čížek, A. (2009). Antibiotic-resistant Salmonella and Escherichia coli isolates with integrons and extended-spectrum beta-lactamases in surface water and sympatric black-headed gulls. J. Appl. Microbiol. 106, 1941–1950. doi: 10.1111/j.1365-2672.2009.04155.x
Dolejska, M., Cizek, A., and Literak, I. (2007). High prevalence of antimicrobial-resistant genes and integrons in Escherichia coli isolates from Black-headed Gulls in the Czech Republic. J. Appl. Microbiol. 103, 11–19. doi: 10.1111/j.1365-2672.2006.03241.x
Dolejska, M., Masarikova, M., Dobiasova, H., Jamborova, I., Karpiskova, R., Havlicek, M., et al. (2016). High prevalence of Salmonella and IMP-4-producing Enterobacteriaceae in the silver gull on Five Islands, Australia. J. Antimicrob. Chemother. 71, 63–70. doi: 10.1093/jac/dkv306
Dong, H., Chen, Y., Wang, J., Zhang, Y., Zhang, P., Li, X., et al. (2021). Interactions of microplastics and antibiotic resistance genes and their effects on the aquaculture environments. J. Hazard. Mater. 403:123961. doi: 10.1016/j.jhazmat.2020.123961
FAO and WHO (2019). Joint FAO/WHO Expert Meeting in Collaboration with OIE on Foodborne Antimicrobial Resistance: Role of the Environment, Crops and Biocides: Meeting Report. Rome: FAO.
Fenlon, D. R. (1981). Seagulls (Larus spp.) as vectors of salmonellae: an investigation into the range of serotypes and numbers of salmonellae in gull faeces. J. Hyg. 86, 195–202. doi: 10.1017/S0022172400068911
Fresno, M., Barrera, V., Gornall, V., Lillo, P., Paredes, N., Abalos, P., et al. (2013). Identification of diverse Salmonella serotypes, virulotypes, and antimicrobial resistance phenotypes in Waterfowl from Chile. Vector Borne Zoonotic Dis. 13, 884–887. doi: 10.1089/vbz.2013.1408
Gionechetti, F., Zucca, P., Gombac, F., Monti-Bragadin, C., Lagatolla, C., Tonin, E., et al. (2008). Characterization of antimicrobial resistance and class 1 integrons in enterobacteriaceae isolated from mediterranean herring gulls (Larus cachinnans). Microb. Drug Resist. 14, 93–99. doi: 10.1089/mdr.2008.0803
Gomez-Alvarez, V., Ashbolt, N. J., Griffith, J. F., Santo Domingo, J., and Lu, J. (2019). Whole-genome sequencing of four campylobacter strains isolated from gull excreta collected from Hobie Beach (Oxnard, CA, USA). Microbiol. Resour. Announc. 8:e00560-19. doi: 10.1128/mra.00560-19
Guenther, S., Ewers, C., and Wieler, L. H. (2011). Extended-spectrum beta-lactamases producing E. coli in wildlife, yet another form of environmental pollution? Front. Microbiol. 2:246. doi: 10.3389/fmicb.2011.00246
Gullberg, E., Albrecht, L. M., Karlsson, C., Sandegren, L., and Andersson, D. I. (2014). Selection of a multidrug resistance plasmid by sublethal levels of antibiotics and heavy metals. MBio 5:e01918-14. doi: 10.1128/mBio.01918-14
Haenni, M., Métayer, V., Jarry, R., Drapeau, A., Puech, M. P., Madec, J. Y., et al. (2020). Wide spread of blaCTX–M–9/mcr-9 IncHI2/ST1 plasmids and CTX-M-9-producing Escherichia coli and Enterobacter cloacae in rescued wild animals. Front. Microbiol. 11:601317. doi: 10.3389/fmicb.2020.601317
Hasan, B., Melhus, Å, Sandegren, L., Alam, M., and Olsen, B. (2014). The gull (Chroicocephalus brunnicephalus) as an environmental bioindicator and reservoir for antibiotic resistance on the coastlines of the Bay of Bengal. Microb. Drug Resist. 20, 466–471. doi: 10.1089/mdr.2013.0233
Hernandez, J., Bonnedahl, J., Eliasson, I., Wallensten, A., Comstedt, P., Johansson, A., et al. (2010). Globally disseminated human pathogenic Escherichia coli of O25b-ST131 clone, harbouring blaCTX-M-15, found in Glaucous-winged gull at remote Commander Islands, Russia. Environ. Microbiol. Rep. 2, 329–332. doi: 10.1111/j.1758-2229.2010.00142.x
Hernandez, J., Johansson, A., Stedt, J., Bengtsson, S., Porczak, A., Granholm, S., et al. (2013). Characterization and comparison of extended-spectrum β-lactamase (ESBL) resistance genotypes and population structure of Escherichia coli isolated from Franklin’s gulls (Leucophaeus pipixcan) and humans in Chile. PLoS One 8:e76150. doi: 10.1371/journal.pone.0076150
IUCN (2021). Laridae Family. Available online at: https://www.iucnredlist.org/search/list?taxonomies=22673045&searchType=species (accessed April 12, 2021)
Kilpi, M., and Saurola, P. (1984). Migration and wintering strategies of juvenile and adult Larus marinus, L. argentatus and L. fuscus from Finland. Orn. Fennica 61, 1–8.
Li, J., Bi, Z., Ma, S., Chen, B., Cai, C., He, J., et al. (2019). Inter-host transmission of carbapenemase-producing Escherichia coli among humans and backyard animals. Environ. Health Perspect. 127:107009. doi: 10.1289/EHP5251
Liakopoulos, A., Olsen, B., Geurts, Y., Artursson, K., Berg, C., Mevius, D. J., et al. (2016). Molecular characterization of extended-spectrum-cephalosporin-resistant Enterobacteriaceae from wild kelp gulls in South America. Antimicrob. Agents Chemother. 60, 6924–6927. doi: 10.1128/AAC.01120-16
Literak, I., Dolejska, M., Janoszowska, D., Hrusakova, J., Meissner, W., Rzyska, H., et al. (2010). Antibiotic-resistant escherichia coli bacteria, including strains with genes encoding the extended-spectrum beta-lactamase and QnrS, in waterbirds on the Baltic Sea coast of Poland. Appl. Environ. Microbiol. 76, 8126–8134. doi: 10.1128/AEM.01446-10
Literak, I., Manga, I., Wojczulanis-Jakubas, K., Chroma, M., Jamborova, I., Dobiasova, H., et al. (2014). Enterobacter cloacae with a novel variant of ACT AmpC beta-lactamase originating from glaucous gull (Larus hyperboreus) in Svalbard. Vet. Microbiol. 171, 432–435. doi: 10.1016/j.vetmic.2014.02.015
Loayza, F., Graham, J. P., and Trueba, G. (2020). Factors obscuring the role of E. coli from domestic animals in the global antimicrobial resistance crisis: an evidence-based review. Int. J. Environ. Res. Public Health 17:3061. doi: 10.3390/ijerph17093061
Łopińska, A., Indykiewicz, P., Skiebe, E., Pfeifer, Y., Trček, J., Jerzak, L., et al. (2020). Low occurrence of acinetobacter baumannii in gulls and songbirds. Polish J. Microbiol. 69, 85–90. doi: 10.33073/pjm-2020-011
Martiny, A. C., Martiny, J. B. H., Weihe, C., Field, A., and Ellis, J. C. (2011). Functional metagenomics reveals previously unrecognized diversity of antibiotic resistance genes in gulls. Front. Microbiol. 2:238. doi: 10.3389/fmicb.2011.00238
Masarikova, M., Manga, I., Cizek, A., Dolejska, M., Oravcova, V., Myskova, P., et al. (2016). Salmonella enterica resistant to antimicrobials in wastewater effluents and black-headed gulls in the Czech Republic, 2012. Sci. Total Environ. 542, 102–107. doi: 10.1016/j.scitotenv.2015.10.069
Merkeviciene, L., Klimiene, I., Siugzdiniene, R., Virgailis, M., Mockeliunas, R., and Ruzauskas, M. (2018). Prevalence and molecular characteristics of multi-resistant Escherichia coli in wild birds. Acta Vet. Brno 87, 9–18. doi: 10.2754/avb201887010009
Merkeviciene, L., Ruzauskaite, N., Klimiene, I., Siugzdiniene, R., Dailidaviciene, J., Virgailis, M., et al. (2017). Microbiome and antimicrobial resistance genes in microbiota of cloacal samples from European herring gulls (Larus argentatus). J. Vet. Res. 61, 27–35. doi: 10.1515/jvetres-2017-0004
Migura-Garcia, L., Ramos, R., and Cerdà-Cuéllar, M. (2017). Antimicrobial resistance of Salmonella serovars and Campylobacter spp. Isolated from an opportunistic gull species, yellow-legged gull (Larus michahellis). J. Wildl. Dis. 53, 148–152. doi: 10.7589/2016-03-051
Moré, E., Ayats, T., Ryan, P. G., Naicker, P. R., Keddy, K. H., Gaglio, D., et al. (2017). Seabirds (Laridae) as a source of Campylobacter spp., Salmonella spp. and antimicrobial resistance in South Africa. Environ. Microbiol. 18, 3896–3909. doi: 10.1111/1462-2920.13874
Mukerji, S., Gunasekera, S., Dunlop, J. N., Stegger, M., Jordan, D., Laird, T., et al. (2020). Implications of foraging and interspecies interactions of birds for carriage of Escherichia coli strains resistant to critically important antimicrobials. Appl. Environ. Microbiol. 86, 1–13. doi: 10.1128/AEM.01610-20
Mukerji, S., Stegger, M., Truswell, A. V., Laird, T., Jordan, D., Abraham, R. J., et al. (2019). Resistance to critically important antimicrobials in Australian silver gulls (Chroicocephalus novaehollandiae) and evidence of anthropogenic origins. J. Antimicrob. Chemother. 74, 2566–2574. doi: 10.1093/jac/dkz242
Nelson, M., Jones, S. H., Edwards, C., and Ellis, J. C. (2008). Characterization of Escherichia coli populations from gulls, landfill trash, and wastewater using ribotyping. Dis. Aquat. Organ. 81, 53–63. doi: 10.3354/dao01937
Ngaiganam, E. P., Pagnier, I., Chaalal, W., Leangapichart, T., Chabou, S., Rolain, J. M., et al. (2019). Investigation of urban birds as source of β-lactamase-producing Gram-negative bacteria in Marseille city, France. Acta Vet. Scand. 61:51. doi: 10.1186/s13028-019-0486-9
Oravcova, V., Svec, P., and Literak, I. (2017). Vancomycin-resistant enterococci with vanA and vanB genes in Australian gulls. Environ. Microbiol. Rep. 9, 316–318. doi: 10.1111/1758-2229.12542
Palmgren, H., Aspán, A., Broman, T., Bengtsson, K., Blomquist, L., Bergström, S., et al. (2006). Salmonella in Black-headed gulls (Larus ridibundus); prevalence, genotypes and influence on Salmonella epidemiology. Epidemiol. Infect. 134, 635–644. doi: 10.1017/S0950268805005261
Papagiannitsis, C. C., Kutilova, I., Medvecky, M., Hrabak, J., and Dolejska, M. (2017). Characterization of the complete nucleotide sequences of IncA/C2 plasmids carrying in809-like integrons from enterobacteriaceae isolates of wildlife origin. Antimicrob. Agents Chemother. 61:e01093-17. doi: 10.1128/AAC.01093-17
Poeta, P., Radhouani, H., Igrejas, G., Gonçalves, A., Carvalho, C., Rodrigues, J., et al. (2008). Seagulls of the Berlengas natural reserve of Portugal as carriers of fecal Escherichia coli harboring CTX-M and TEM extended-spectrum beta-lactamases. Appl. Environ. Microbiol. 74, 7439–7441. doi: 10.1128/AEM.00949-08
Radhouani, H., Igrejas, G., Pinto, L., Gonalves, A., Coelho, C., Rodrigues, J., et al. (2011). Molecular characterization of antibiotic resistance in enterococci recovered from seagulls (Larus cachinnans) representing an environmental health problem. J. Environ. Monit. 13, 2227–2233. doi: 10.1039/c0em00682c
Radhouani, H., Poeta, P., Igrejas, G., Gonçalves, A., Vinué, L., and Torres, C. (2009). Antimicrobial resistance and phylogenetic groups in isolates of Escherichia coli from seagulls at the berlengas nature reserve. Vet. Rec. 165, 138–142. doi: 10.1136/vr.165.5.138
Radhouani, H., Poeta, P., Pinto, L., Miranda, J., Coelho, C., Carvalho, C., et al. (2010). Proteomic characterization of vanA-containing Enterococcus recovered from Seagulls at the Berlengas natural reserve, W Portugal. Proteome Sci. 8, 1–12. doi: 10.1186/1477-5956-8-48
Retamal, P., Fresno, M., Dougnac, C., Gutierrez, S., Gornall, V., Vidal, R., et al. (2015). Genetic and phenotypic evidence of the Salmonella enterica serotype Enteritidis human-animal interface in Chile. Front. Microbiol. 6:464. doi: 10.3389/fmicb.2015.00464
Rose, J. M., Gast, R. J., Bogomolni, A., Ellis, J. C., Lentell, B. J., Touhey, K., et al. (2009). Occurrence and patterns of antibiotic resistance in vertebrates off the Northeastern United States coast. FEMS Microbiol. Ecol. 67, 421–431. doi: 10.1111/j.1574-6941.2009.00648.x
Ruzauskas, M., and Vaskeviciute, L. (2016). Detection of the mcr-1 gene in Escherichia coli prevalent in the migratory bird species Larus argentatus. J. Antimicrob. Chemother. 71, 2333–2334. doi: 10.1093/jac/dkw245
Salgado-Caxito, M., Benavides, J. A., Adell, A. D., Paes, A. C., and Moreno-Switt, A. I. (2021). Global prevalence and molecular characterization of extended-spectrum β-lactamase producing- in dogs and cats – a scoping review and meta-analysis. One Heal. 12:100236. doi: 10.1016/j.onehlt.2021.100236
Simões, R. R., Poirel, L., Da Costa, P. M., and Nordmann, P. (2010). Seagulls and beaches as reservoirs for multidrug-resistant Escherichia coli. Emerg. Infect. Dis. 16, 110–112. doi: 10.3201/eid1601.090896
Smith, W. A., Mazet, J. A. K., and Hirsh, D. C. (2002). Salmonella in California wildlife species: prevalence in rehabilitation centers and characterization of isolates. J. Zoo Wildl. Med. 33, 228–235.
Stedt, J., Bonnedahl, J., Hernandez, J., Waldenström, J., McMahon, B. J., Tolf, C., et al. (2015). Carriage of CTX-M type extended spectrum β-lactamases (ESBLs) in gulls across Europe. Acta Vet. Scand. 57, 1–8. doi: 10.1186/s13028-015-0166-3
Tardone, R., Rivera, D., Dueñas, F., Sallaberry-Pincheira, N., Hamilton-West, C., Adell, A. D., et al. (2020). Salmonella in raptors and aquatic wild birds in Chile. J. Wildl. Dis. 56, 707–712. doi: 10.7589/2019-08-198
Toro, M., Retamal, P., Ayers, S., Barreto, M., Allard, M., Brown, E. W., et al. (2016). Whole-genome sequencing analysis of Salmonella enterica serovar enteritidis isolates in chile provides insights into possible transmission between gulls, poultry, and humans. Appl. Environ. Microbiol. 82, 6223–6232. doi: 10.1128/AEM.01760-16
Tricco, A. C., Lillie, E., Zarin, W., O’Brien, K. K., Colquhoun, H., Levac, D., et al. (2018). PRISMA extension for scoping reviews (PRISMA-ScR): checklist and explanation. Ann. Intern. Med. 169, 467–473. doi: 10.7326/M18-0850
Troxler, S., Hess, C., Konicek, C., Knotek, Z., Barták, P., and Hess, M. (2017). Microdilution testing reveals considerable and diverse antimicrobial resistance of Escherichia coli, thermophilic Campylobacter spp. and Salmonella spp. isolated from wild birds present in urban areas. Eur. J. Wildl. Res. 63, 1–11. doi: 10.1007/s10344-017-1125-2
Tsubokura, M., Matsumoto, A., Otsuki, K., Animas, S. B., and Sanekata, T. (1995). Drug resistance and conjugative R plasmids in Escherichia coli strains isolated from migratory waterfowl. J. Wildl. Dis. 31, 352–357. doi: 10.7589/0090-3558-31.3.352
Varela, A. R., Manageiro, V., Ferreira, E., Guimarães, M. A., Da Costa, P. M., Caniça, M., et al. (2015). Molecular evidence of the close relatedness of clinical, gull and wastewater isolates of quinolone-resistant Escherichia coli. J. Glob. Antimicrob. Resist. 3, 286–289. doi: 10.1016/j.jgar.2015.07.008
Veldman, K., van Tulden, P., Kant, A., Testerink, J., and Mevius, D. (2013). Characteristics of cefotaxime-resistant Escherichia coli from wild birds in The Netherlands. Appl. Environ. Microbiol. 79, 7556–7561. doi: 10.1128/AEM.01880-13
Vergara, A., Pitart, C., Montalvo, T., Roca, I., Sabaté, S., Hurtado, J. C., et al. (2017). Prevalence of extended-spectrum-β-lactamase- and/or carbapenemase-producing Escherichia coli isolated from yellow-legged gulls from Barcelona, Spain. Antimicrob. Agents Chemother. 61:e02071-16. doi: 10.1128/AAC.02071-16
Vigo, G. B., Leotta, G. A., Caffer, M. I., Salve, A., Binsztein, N., and Pichel, M. (2011). Isolation and characterization of Salmonella enterica from Antarctic wildlife. Polar Biol. 34, 675–681. doi: 10.1007/s00300-010-0923-8
Vittecoq, M., Godreuil, S., Prugnolle, F., Durand, P., Brazier, L., Renaud, N., et al. (2016). REVIEW: antimicrobial resistance in wildlife. J. Appl. Ecol. 53, 519–529. doi: 10.1111/1365-2664.12596
Vittecoq, M., Laurens, C., Brazier, L., Durand, P., Elguero, E., Arnal, A., et al. (2017). VIM-1 carbapenemase-producing Escherichia coli in gulls from southern France. Ecol. Evol. 7, 1224–1232. doi: 10.1002/ece3.2707
Vredenburg, J., Varela, A. R., Hasan, B., Bertilsson, S., Olsen, B., Narciso-da-Rocha, C., et al. (2014). Quinolone-resistant Escherichia coli isolated from birds of prey in Portugal are genetically distinct from those isolated from water environments and gulls in Portugal, Spain and Sweden. Environ. Microbiol. 16, 995–1004. doi: 10.1111/1462-2920.12231
Wallensten, A., Hernandez, J., Ardiles, K., González-Acuña, D., Drobni, M., and Olsen, B. (2011). Extended spectrum beta-lactamases detected in Escherichia coli from gulls in Stockholm, Sweden. Infect. Ecol. Epidemiol. 1:7030. doi: 10.3402/iee.v1i0.7030
Wang, J., Ma, Z. B., Zeng, Z. L., Yang, X. W., Huang, Y., and Liu, J. H. (2017). The role of wildlife (wild birds) in the global transmission of antimicrobial resistance genes. Zool. Res. 38, 55–80. doi: 10.24272/j.issn.2095-8137.2017.003
WHO (2017). Global Priority List of Antibiotic-Resistant Bacteria to Guide Research, Discovery, and Development of New Antibiotics. Geneva: WHO.
WHO (2019). 2019 Antibacterial Agents in Clinical Development: An Analysis of the Antibacterial Clinical Development Pipeline. Geneva: WHO.
Keywords: marine birds, One Health, seagulls, wildlife, bacteria, antimicrobial resistance, AMR, ESBL
Citation: Zeballos-Gross D, Rojas-Sereno Z, Salgado-Caxito M, Poeta P, Torres C and Benavides JA (2021) The Role of Gulls as Reservoirs of Antibiotic Resistance in Aquatic Environments: A Scoping Review. Front. Microbiol. 12:703886. doi: 10.3389/fmicb.2021.703886
Received: 30 April 2021; Accepted: 21 June 2021;
Published: 23 July 2021.
Edited by:
Jorge Andres Olivares Pacheco, Pontificia Universidad Católica de Valparaíso, ChileReviewed by:
Takehiko Kenzaka, Osaka Ohtani University, JapanAndres Felipe Opazo-Capurro, University of Concepcion, Chile
Copyright © 2021 Zeballos-Gross, Rojas-Sereno, Salgado-Caxito, Poeta, Torres and Benavides. This is an open-access article distributed under the terms of the Creative Commons Attribution License (CC BY). The use, distribution or reproduction in other forums is permitted, provided the original author(s) and the copyright owner(s) are credited and that the original publication in this journal is cited, in accordance with accepted academic practice. No use, distribution or reproduction is permitted which does not comply with these terms.
*Correspondence: Julio A. Benavides, YmVuYXZpZGVzanVsaW9AeWFob28uZnI=