- 1State Veterinary and Food Institute (SVFI), Dolny Kubin, Slovakia
- 2Maisons-Alfort Laboratory for Food Safety, Salmonella and Listeria Unit, University of Paris-Est, French Agency for Food, Environmental and Occupational Health & Safety (ANSES), Maisons-Alfort, France
Over the past 11 years, the Slovak National Reference Laboratory has collected a panel of 988 Listeria monocytogenes isolates in Slovakia, which were isolated from various food sectors (61%), food-processing environments (13.7%), animals with listeriosis symptoms (21.2%), and human cases (4.1%). We serotyped these isolates by agglutination method, which revealed the highest prevalence (61.1%) of serotype 1/2a and the lowest (4.7%) of serotype 1/2c, although these represented the majority of isolates from the meat sector. The distribution of CCs analyzed on 176 isolates demonstrated that CC11-ST451 (15.3%) was the most prevalent CC, particularly in food (14.8%) and animal isolates (17.5%). CC11-ST451, followed by CC7, CC14, and CC37, were the most prevalent CCs in the milk sector, and CC9 and CC8 in the meat sector. CC11-ST451 is probably widely distributed in Slovakia, mainly in the milk and dairy product sectors, posing a possible threat to public health. Potential persistence indication of CC9 was observed in one meat facility between 2014 and 2018, highlighting its general meat-related distribution and potential for persistence worldwide.
Introduction
Listeria monocytogenes is a Gram-positive intracellular bacterium responsible for a serious food-borne zoonosis called listeriosis. This pathogen is transmissible to humans through the consumption of contaminated food. It can lead to mild gastroenteritis and also to serious infections of the bloodstream or the central nervous system as well as abortions. The organism is able to pass from cell to cell, allowing it to cross blood–brain and placental barriers (Janakiraman, 2008). Immuno-compromised persons, pregnant women, infants, and the elderly are the most vulnerable to listeriosis (Vazquez-Boland et al., 2001). In 2015–2019, the listeriosis trend in Europe remained stable after a long period of increase but had the highest case-fatality rate (8.9%) among the outbreak-related illness (EFSA and ECDC, 2021). In Slovakia, 18 human listeriosis cases and four deaths were reported in the year 2019, which is 6% above the 5-year average (Slovak Focal Point for the Scientific, and Technical Matters of the Efsa., 2020).
Some L. monocytogenes strains can persist for a long period of time in various types of food-processing environment (FPE), including chilled processing plants (Lundén et al., 2004; Schmitz-Esser et al., 2015). Listeria monocytogenes contamination may occur as a result of poor hygiene during food processing or packaging (Carpentier and Cerf, 2011).
Animal listeriosis is generally subclinical, but more serious forms have also been observed (OIE, 2018), with symptoms including septicaemia, encephalitis, meningitis, meningoencephalitis, rhombencephalitis, abortion, stillbirth, perinatal infections, and/or gastroenteritis (Okada et al., 2011; OIE, 2018).
Listeria monocytogenes is a genetically heterogeneous species divided into four phylogenetic lineages, of which lineages I and II are the most frequently encountered. The strains from lineage I (serotypes 1/2b, 4b) are in general found in higher frequency in human outbreaks than lineage II strains (serotypes 1/2a, 1/2c), which are commonly isolated from food, natural and farm environments, and animal and sporadic human listeriosis cases (Orsi et al., 2011).
Several methods have been developed to investigate the genetic diversity of L. monocytogenes strains. Agglutination serotyping is considered to be the first level of discrimination between isolates and can differentiate 13 serotypes. For many years, pulsed-field gel electrophoresis (PFGE) had been the gold standard for L. monocytogenes subtyping (Graves and Swaminathan, 2001). Another key typing approach has been multi-locus sequence typing (MLST) based on the standardized nomenclature derived from the sequences of seven housekeeping genes (Ragon et al., 2008). Unique combinations of alleles from MLST analysis determine strain sequence types (STs) and clonal complexes (CCs), which are now systematically used to describe the population structure of L. monocytogenes (Ragon et al., 2008; Cantinelli et al., 2013; Maury et al., 2016). Certain CCs account for the majority of outbreaks and sporadic cases in humans (Maury et al., 2016) and animals (Dreyer et al., 2016). More recently, typing based on whole-genome sequencing (WGS) of L. monocytogenes has become a very powerful tool and more and more studies are employing this method for national surveillance, outbreak detection, or tracking of the sources of listeriosis (Jackson et al., 2016; Moura et al., 2017; Pietzka et al., 2019).
The State Veterinary and Food Institute (SVFI) has been the designated Slovak National Reference Laboratory (NRL) for L. monocytogenes since 2007. This national-level laboratory is engaged in the surveillance and typing of L. monocytogenes isolates from food items and animals. It participates in the European surveillance network for L. monocytogenes, along with 34 other NRLs coordinated by the European Reference Laboratory (EURL).1 The PFGE method is routinely performed in the Slovak NRL. The isolates are assigned to an MLST CC using a mapping method detailed in Félix et al. (2018). Whole-genome sequencing of the isolates is currently being introduced.
Only a few studies are available on the genomic or genetic diversity of L. monocytogenes in Slovakia, and they focus only on selected food manufacturers, either in the dairy sector (Véghová et al., 2015) or in the meat sector (Véghová et al., 2016). To date, no data are available on a large and diverse panel of food isolates for Slovakia. The purpose of this study was thus to provide an overview of the population structure of the L. monocytogenes isolates over the past 11 years in Slovakia. This survey should lead to better management and understanding of food-related health risks.
This study had two objectives. The first was to analyse the genetic diversity of all L. monocytogenes isolates from food, FPEs, and animals available in the NRL collection. The second was to compare this diversity to that of isolates from human outbreaks during the same time period. The genetic diversity of 988 isolates was assessed using conventional agglutination serotyping. In a subset of 176 isolates, CCs were deduced either from PFGE profiles (127 isolates) or from WGS analysis (49 isolates).
Materials and Methods
Isolate Panel
A panel of 988 isolates collected during the 2010–2020 period was selected for this study (Supplementary Table 1). The isolates were from four compartments: food, FPEs, animals, and humans.
The vast majority of the isolates (947) were from the NRL microbial collection: 603 (61%) isolates were from food, 135 (13.7%) from FPEs, and 209 (21.2%) from animals (40.7% of animal isolates were isolated from sheep; 29.2% from cattle) with clinical manifestations of listeriosis. Detailed epidemiological information (sampling stage, context, sources, food matrix, and food product) were compiled in the NRL molecular database (BioNumerics, vers. 7.6.3, Applied Maths, Sint-Martens-Latem, Belgium).
The number of food isolates per sampling period (2010–2020) was generally evenly distributed across years, with an average of 54.8 isolates per year (Figure 1). The food isolates included 328 (54.5%) isolates from milk and dairy products, 135 (22.4%) from meat and meat products, and 140 (23.1%) from other food products (ready-to-eat delicatessen products predominated, representing 85.6% of isolates in this category). Another 135 (13.7%) isolates were from FPEs, mainly from dairy facilities (Figure 1). The 603 food isolates were from official Slovak controls and from the producer’s own internal testing procedures. The 209 (21.2%) animal isolates were collected by private veterinarians.
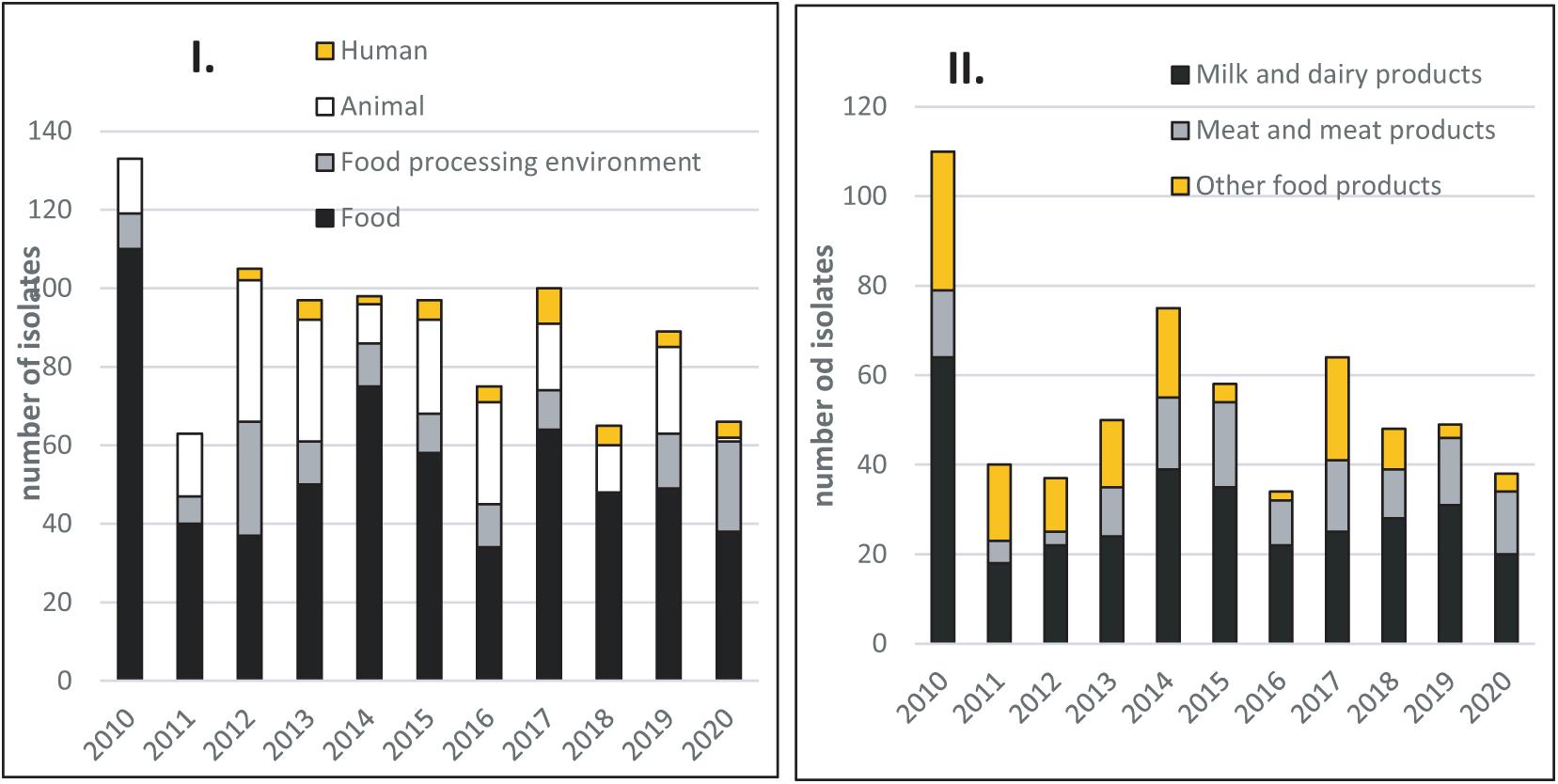
Figure 1. (I) Distribution of Listeria monocytogenes isolates collected yearly from food, food-processing environments, animals, and human cases of listeriosis between 2010 and 2020 in Slovakia. (II) Distribution of Listeria monocytogenes isolates according to food origin during the 2010–2020 period.
These food, FPE, and animal isolates were supplemented with 41 isolates (4.1%) from human listeriosis cases (Figure 1). These human case isolates were collected between 2012 and 2020 by the Slovak Public Health Authority (PHA), and sent to the NRL.
A subset of 176 isolates was then selected from the available typing data collection. The majority of isolates were chosen from 2014 (n = 51; 29%), 2015 (n = 41; 23.3%), and 2018 (n = 29; 16.5%) years in which intensive typing had been performed in Slovakia during national surveillance programs. These 121 isolates were then supplemented with 55 isolates (31.2%) from the 2010–2020 period, with PFGE or WGS typing data available. Whereas origin of the isolates is very constant throughout time, we considered this selection to be representative extraction of the whole panel. This subset (n = 176) included isolates from four compartments in particular: 115 food sector isolates (65.3%), with 68 dairy isolates (59.2%), 28 meat isolates (24.3%), and 19 isolates (16.5%) from other foods. Of the 61 remaining isolates, 21.3% were from FPEs, 65.6% from animals, and 13.1% from human cases (Supplementary Table 1).
Listeria monocytogenes Isolates Recovery
The isolates were recovered from a long-term storage at –80°C using tryptone soya yeast extract agar (TSYEA, Biokar Diagnostics, Allonne, France).
Agglutination Serotyping (988 Isolates)
Serotypes can be identified based on the somatic (O) and flagellar (H) antigens. Antisera against eight somatic (OI/II, OI, OIV, OV/VI, OVI, OVII. OVIII, OIX) and four flagellar (HA, HAB, HC, HD) antigens were used as recommended by the manufacturer of a commercially distributed kit (Denka Seiken, Tokyo, Japan).
Determination of Clonal Complexes and Sequences Types (176 Isolates)
Molecular Typing Using Pulsed-Field Gel Electrophoresis (127 Isolates)
Pulsed-field gel electrophoresis (PFGE) was performed according to the protocol described in Roussel et al. (2014) in the years of isolate collection. Electrophoresis was performed on 1% agarose gel (SeaKem Gold Agarose, Lonza, Rockland, ME, United States) with a CHEF Mapper® XA system (Bio-Rad, Hercules, CA, United States). Salmonella serovar Braenderup H9812 DNA digested by XbaI enzyme (Thermo Scientific, Vilnius, Lithuania) was used as a reference standard.
BioNumerics software (vers. 7.6.3) was used to create a database and compare the PFGE profiles of 164 L. monocytogenes isolates. Epidemiological duplicates were excluded. They were defined as isolates sharing indistinguishable PFGE profiles, isolated the same year and provided by the same food business operator or the same diagnostic food laboratory, as described in Félix et al. (2018). A total of 164 PFGE profiles were interpreted according to the standard operating procedure (SOP) detailed in Michelon et al. (2015). One hundred thirty-four isolates were grouped to clusters according to the 85% similarity rule. Of these, five L. monocytogenes isolates were selected for WGS, through the H2020 European Joint Program (EJP) ListAdapt (Félix et al., 2020), and two human ST451 isolates (deduced from PFGE) were sequenced at the Slovak NRL, resulting in 127 PFGE profiles (Supplementary Figure 1 dendrogram) for mapping to MLST data. For these 127 isolates, the CCs were deduced from the PFGE profiles, using the mapping protocol detailed in Félix et al. (2018).
Whole-Genome Sequencing and Multi-Locus Sequence Typing (49 Isolates)
A total of 49 isolates were whole-genome sequenced: 45 isolates were sequenced by external partners as part of European research projects, such as the H2020 European Joint Program (EJP) ListAdapt (Félix et al., 2020) and the EFSA-LISEQ project (Nielsen et al., 2017; Painset et al., 2019); 1 isolate was sequenced by the Austrian NRL; and 3 isolates were sequenced by the Slovak NRL in an iSeq 100 sequencing machine (Illumina). The 49 WGS datasets were assembled using SPADES and analyzed to determine their MLST (7 loci, Salcedo et al., 2003; Ragon et al., 2008), core-genome MLST (cgMLST) (1,748 loci, Moura et al., 2016), and whole-genome MLST (wgMLST) with 4,804 loci in BioNumerics software version 7.6.3 with connection to the EURL for the Listeria monocytogenes calculation engine. Raw sequencing reads were quality-checked according to the following criteria: PHRED score of raw reads greater than 30 and average depth coverage greater than 30 × (Neuman et al., 2013). The target length of the de novo assembly was between 2.8 and 3.1 Mb, and identification of 95%–100% of cgMLST loci was necessary for assignment to a CC-ST profile (Moura et al., 2016).
Comparison of the Genomes of Seven ST451 Isolates With Publicly Available Genomes
Out of the 49 isolates for which the whole genome was sequenced, seven CC11-ST451 isolates were collected in Slovakia (four animal, one milk, and two human isolates). These seven genomes were compared with the 144 genomes of CC11-ST451 isolates available in public databases to see the possible relatedness between them as the CC11-ST451 was the most abundant in Slovak isolate collection and not typically present in Europe. One hundred six genomes were from NCBI-ENA databases, 8 genomes from the EFSA project LISEQ (Nielsen et al., 2017), 29 genomes from Lüth et al. (2021), and 1 genome from Kuch et al. (2018) (Supplementary Table 2). The genomes were quality-checked and compared using cgMLST (1748 loci, Moura et al., 2016) of all the ST451 isolates, as described in Section “Whole-Genome Sequencing and Multi-Locus Sequence Typing (49 Isolates).”
Results
Serotype Distribution According to the Compartment and Food Sector
Out of the 988 isolates studied, the majority (61.1%) belonged to serogroup 1/2a, 17.8% to 1/2b, 12.7% to 4b, and 4.7% to 1/2c. The remaining isolates (3.7%) were assigned to other serotypes. Serotype 1/2a represented 72.0% of the isolates from the milk and dairy product sector, 64.4% from meat and meat product sector, 38.6% of other food sectors, 57.0% from the FPE sector, 63.6% from animal samples, and finally 41.5% from human cases. In the food sectors, serotypes 1/2b and 4b were mainly detected in food matrices other than dairy and meat (35.7% and 22.1%, respectively) (Table 1). The distribution of serotypes throughout the years 2010-2020 was mostly uniform with the average 62.5% representing 1/2a serotype per year (46.7%–75.3%), 16.9% of 1/2b serotype per year (6.2%–32.3%), 4.5% of 1/2c serotype per year (1.0%–9.2%), and 12.4% of 4b serotype per year (3.1%–32.4%).
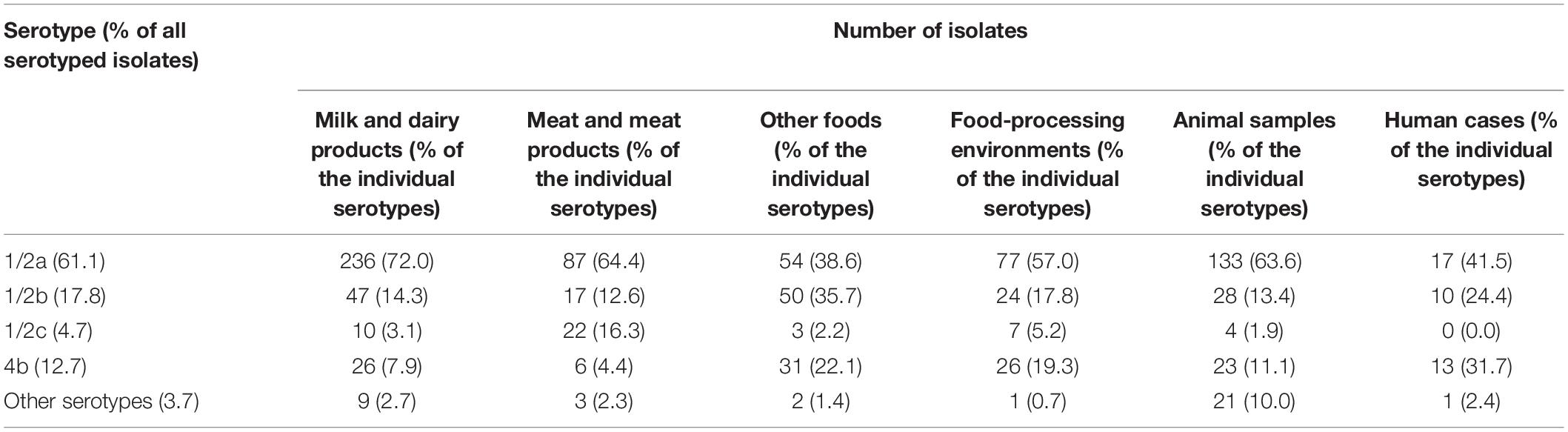
Table 1. Distribution of Listeria monocytogenes serotypes according to isolate compartment and food sector.
Clonal Complexes Distribution According to the Compartment and Food Sector
CC11-ST451 (serotype 1/2a) was the most prevalent CC in the panel of 176 isolates (15.3%, n = 27) (Figure 2 and Table 2). The origin of these CC11-ST451 isolates is summarized in Supplementary Table 1. In particular, the highest frequency of CC11-ST451 (22.1%, n = 15) was observed in dairy products (Figure 2 and Table 2).
The CC11-ST451 isolates analyzed here were isolated from very diverse milk and dairy products collected in a geographic area of roughly 200 km in diameter (north-western central Slovakia) throughout the 2014–2020 period. Many CC11-ST451 animal isolates (from clinical sheep cases, sheep’s milk) were also from the same region and collected during this same time period. These results revealed a wide distribution of CC11-ST451 in the north-western area of central Slovakia.
The other prevalent CCs among dairy products and animals were CC7 and CC37 (Figure 2 and Table 2). Meat-related isolates of L. monocytogenes mainly grouped into CC9, followed by CC8. Human isolates belonged to CC11-ST451 (Lineage II), CC4, CC6, and CC1 (Lineage I) (Figure 2 and Table 2). The minimum spanning tree (Figure 3) shows the phylogenetic relatedness between the CCs.
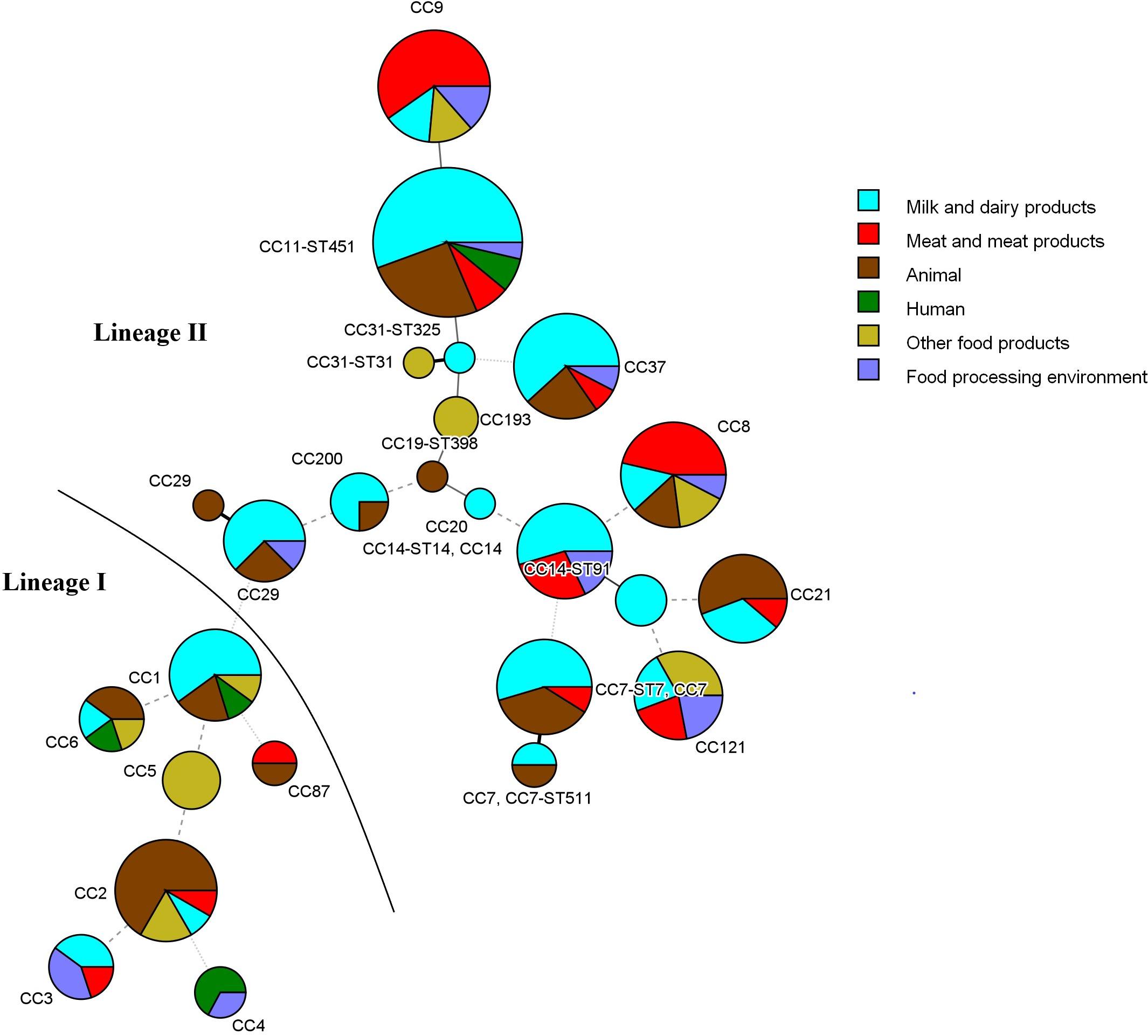
Figure 3. Minimum spanning tree of MLST data for the 173 L. monocytogenes isolates analyzed (3 isolates with unassigned CC were excluded). Each CC is represented by a circle whose size corresponds to the number of isolates in the clonal group. STs are known only for isolates that were whole-genome sequenced. Lines connecting the circles indicate phylogenetic relatedness and the number of allelic differences (thick solid line—1; thin solid line—2; dashed line—3; dotted line—4 and above).
Indication of Potential Persistence in One Meat Product Facility
The same PFGE profile was observed for three isolates collected between 2014 and 2018 in one meat product facility (Figure 4). This profile mapped to CC9 (serotype 1/2c).

Figure 4. PFGE profiles obtained from L. monocytogenes isolates from one meat associated facility between 2014 and 2018.
Comparison of the Genomes of Seven CC11-ST451 Isolates With Publicly Available Genomes
Out of the 27 CC11-ST451 isolates, 7 isolates were selected for WGS and compared using cgMLST, which showed at least 18 allele differences (AD) between each other, with the exception of human isolates that differed by only one AD. Subsequently, the seven isolates were compared with the CC11-ST451 isolates from public databases and external collaboration (Section “Comparison of the Genomes of Seven ST451 Isolates With Publicly Available Genomes”). None of the seven Slovak isolates showed fewer than seven AD with any other isolate (Figure 5). Moreover, the results of our work undertaken in collaboration with Austrian colleagues [Dr. Adriana Cabal, Austrian Agency for Health and Food Safety (AGES)] showed that the seven Slovak isolate genomes did not match with fewer than seven AD across the approximately 600 CC11-ST451 isolates present in the Austrian national database. The sequences of human isolates did not match any other isolate genomes but clustered together with only one AD in the cgMLST network.
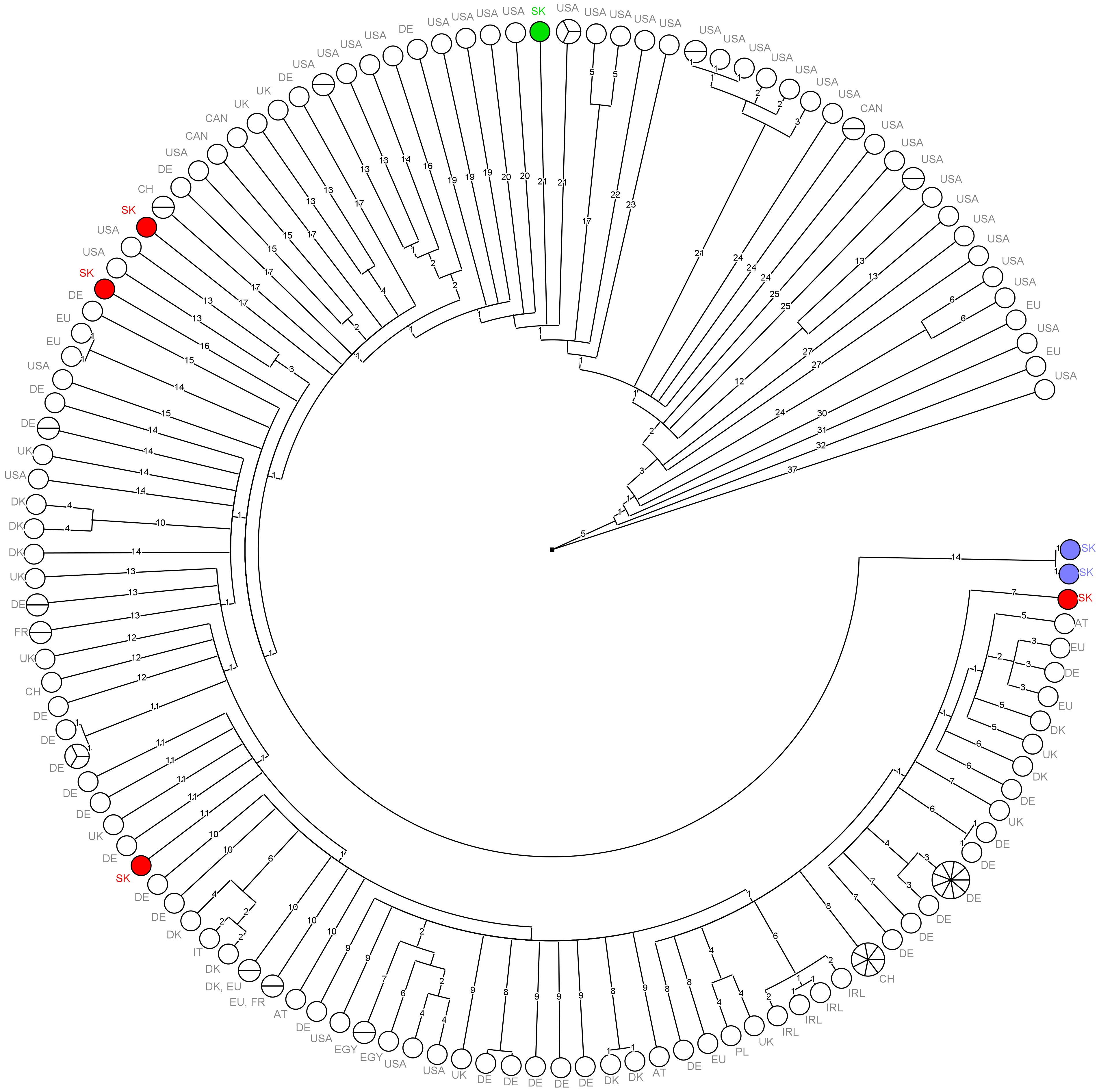
Figure 5. Minimum spanning tree of cgMLST data for 151 L. monocytogenes CC11-ST451 isolates. Branch numbers indicate the number of allelic differences between the isolates. Colored circles represent the seven Slovak ST451 isolates.
Discussion
Listeriosis is a serious public health and food safety problem in European countries, including Slovakia. Little data are available on the population genetic structure of food isolates in this country. Considering that food products are the main sources of human infections, it is very important to study the overall distribution of isolates throughout the country to improve understanding of the circulation of this disease.
The 988 L. monocytogenes isolates analyzed in this study by Denka Seiken kit which is often considered the “gold standard” for its serotyping (Burall et al., 2011) were isolated throughout the 2010–2020 period, providing a representative coverage of isolates circulating in Slovakia. Out of the 988 isolates tested using conventional serotyping, more than half (61.1%) were serotype 1/2a. This prevalence is consistent with many previous studies performed on isolates from various types of foods, mostly milk and dairy and meat products (Kramarenko et al., 2013; Martín et al., 2014; Ebner et al., 2015). The distribution of serotypes throughout the years suggested an even distribution of serotypes 1/2a, 1/2c, and 4b. The descending trend in distribution of 1/2b was observed in the 2010–2020 period.
In this study, CC1, CC2, CC4, and CC6 made up 66.7% of lineage I isolates and were isolated from food, FPEs, and animals, as well as from human cases. This result is similar to that reported in Lachtara et al. (2021) on the prevalence of CC1, CC2, and CC6 in food and FPE isolates in Poland. These CCs have previously been described as hypervirulent (Maury et al., 2016). CC1 and CC2 were concluded as being predominant in all world regions with an exception of northern Africa for CC1. Interestingly, CC6 had been observed mainly in Europe in a study of Chenal-Francisque et al. (2011).
Regarding the food isolates, serotypes 1/2b and 4b were detected mainly among the isolates from milk and dairy products and other food products, this latter category being predominated by ready-to-eat delicatessen products (mainly ready-to-eat salads). These isolates were collected mainly from production plants. Considering that the typing data in this category were only available for a limited number of isolates mainly from 2014, more extensive evaluation of the CC’s diversity should be performed in future to clarify its importance in public health protection. The decreasing trend of the 1/2b serotype in the period of 2010–2020 might be explained by the reduced popularity of rather unhealthy delicatessen products and increased healthier eating habits of Slovak population (Holotová et al., 2021). Prevalence of serotypes 1/2b and 4b has been reported in several studies on milk, meat, and ready-to eat products (Beak et al., 2000; Braga et al., 2017).
Serotype 1/2c was prevalent in isolates from meat, corroborating results from other studies conducted on the meat sector in Europe (Gianfranceschi et al., 2009; Ortiz et al., 2010; Prencipe et al., 2012; Meloni et al., 2014; Ebner et al., 2015; Morganti et al., 2015; Félix et al., 2018).
An indication of potential persistence of a CC9 L. monocytogenes isolate was observed at one meat production site between 2014 and 2018. This result substantiates previous studies demonstrating persistence of CC9 over many years in meat-processing facilities in Switzerland (Stoller et al., 2019) and in meat products and in pig slaughterhouse environments worldwide (Martín et al., 2014; Wang et al., 2015; Véghová et al., 2016; Félix et al., 2018; Maury et al., 2019; Demaître et al., 2020; Fagerlund et al., 2020).
In this study, two CCs, CC1 and CC37, were frequently observed among the isolates sourced from milk and dairy products and from sheep. This result is in accordance with a 2019 study (Papić et al., 2019) in Slovenia that showed that CC1 and CC37 were responsible for the majority of the clinical cases in animals, mainly small ruminants and cattle. CC37 is also the most prevalent isolate found on pig and ruminant farms in France (Félix et al., 2018) and frequently reported from ruminant farms in Switzerland (Dreyer et al., 2016), in the natural environment throughout Europe (Haase et al., 2014) and in the natural environment in Austria (Linke et al., 2014). Similar to our findings, CC7 and CC37 were the most abundant in the study focusing on milk and milk-related environment in the United States (Kim et al., 2018).
Interestingly, CC121 isolates, which are overrepresented in all food sectors in Europe and worldwide (Chenal-Francisque et al., 2011; Henri et al., 2016; Félix et al., 2018) and which can persist in FPEs (Holch et al., 2013; Rychli et al., 2017; Stoller et al., 2019), were of minor occurrence in our study, with only a few CC121 isolates from food sectors being observed. Also, CC121 belongs to the most prevalent clones worldwide (Chenal-Francisque et al., 2011). No CC121 isolates were isolated from animals, similar to previous reports (Linke et al., 2014; Dreyer et al., 2016; Félix et al., 2018).
In this study, CC11-ST451 was prevalent in the milk and dairy products sector. This result is concordant with an Austrian study that revealed that (i) dairy products are three times more likely to be contaminated with CC11-ST451 than the products from other food categories and (ii) a large part of CC11-ST451 food isolates could be traced back to a specific cheese-producing facility (Cabal et al., 2019). However, of the three ST451 human cases reported in Austria in 2017, none were related to the cheese-producing facility and all three were distinguishable, thus most likely arising from different contamination sources. CC11-ST451 isolates have been also reported in dairy products in the Czech Republic (Tomaštíková et al., 2019).
These findings, together with the frequent detection of CC7 in dairy products in Austria (Cabal et al., 2019) and the location of Austria and Czech Republic in Central Europe, are in accordance with our observation of CC11-ST451 and CC7 in the isolates from the dairy sector and the hypothesis of a multiple sources of introduction of these clones that likely circulate intensively.
CC11-ST451 isolates were also detected in the meat production sector, particularly in one rabbit meat-processing plant in Czech Republic. Persistence of these isolates in FPEs was first suggested by Gelbíčová et al. (2019). Interestingly, only one CC11-ST451 isolate was detected among the FPE isolates in our study.
Human cases of listeriosis caused by CC11-ST451 have been reported in Germany (Halbedel et al., 2018), France (Moura et al., 2017), Austria (Cabal et al., 2019), and Poland (Kuch et al., 2018). A nosocomial outbreak linked to ST451 was described lately in Italy (Russini et al., 2021). Furthermore, this clone is considered hypervirulent based on its prevalence in human cases and in food contamination in France (Fritsch et al., 2018).
Conversely, a very low occurrence of ST451 (fewer than 10 isolates) was reported in a wide-scale study (Painset et al., 2019) on 1,142 European isolates (isolated in 22 EU Member States and 1 Non-Member State). The frequent occurrence of CC11-ST451 in Slovakia and in neighboring countries suggests that this ST is specific to Central Europe.
The isolate distribution in Central Europe over a period of 11 years highlights a possible distribution of the CC11-ST451 clone in the milk and meat sectors in Slovakia. The seven genomes of Slovak CC11-ST451 isolates were dispersed among the available known genomes of ST451 isolates collected worldwide. The source of the CC11-ST451 isolates in Slovakia can potentially be determined with the systematic sequencing of the ST451 isolate genomes, now being collected by the Slovak NRL.
The importance of subtyping of L. monocytogenes isolates in Slovakia and in Europe in general is an important tool for food safety management. With the increasing production of food, globalization of the market, and consumers’ focus on traditional farm products with the characteristic small production and less trained staff in the field of the food safety, there is a higher risk of contamination of FPE and final food products. Isolate typing allows the identification of such threats and control measures implementation.
Conclusion
This study is the first comprehensive insight into L. monocytogenes isolate diversity in Slovakia, covering isolates from the different food sectors available in the NRL collection, as well as isolates from clinical animal cases and several human outbreaks. The distribution of 988 serotypes was similar to that generally observed in Europe. The CC11-ST451 type, frequently reported in Central Europe, predominated in the Slovakia dataset, being detected mainly in isolates from dairy products.
Data Availability Statement
The datasets presented in this study can be found in online repositories. The names of the repository/repositories and accession number(s) can be found below: http://www.ebi.ac.uk/ena/, PRJEB38828, PRJNA475189, and PRJEB45859.
Author Contributions
LC was in charge of serotyping. ZK was in charge of PFGE data production, analysis, and interpretation. ZK and BF performed mapping of PFGE profiles to MLST CCs and WGS analysis. ZK, SR, and BF wrote the manuscript. All authors read, commented, and approved the final version.
Funding
A part of this work was supported by the One Health European Joint Programme, European Union’s Horizon 2020 Research and Innovation Programme (Grant Agreement No 773830).
Conflict of Interest
The authors declare that the research was conducted in the absence of any commercial or financial relationships that could be construed as a potential conflict of interest.
Publisher’s Note
All claims expressed in this article are solely those of the authors and do not necessarily represent those of their affiliated organizations, or those of the publisher, the editors and the reviewers. Any product that may be evaluated in this article, or claim that may be made by its manufacturer, is not guaranteed or endorsed by the publisher.
Acknowledgments
We thank Katleen Vranckx from Applied Maths for screening NCBI SRA for ST451 isolates and Adriana Cabal from AGES for comparing Slovak isolates with the Austrian collection. We also acknowledge the financial contribution of Mikuláš Trstenský.
Supplementary Material
The Supplementary Material for this article can be found online at: https://www.frontiersin.org/articles/10.3389/fmicb.2021.729050/full#supplementary-material
Supplementary Figure 1 | Contains PFGE profiles for 127 strains which were mapped to MLST CC.
Footnotes
References
Beak, S. Y., Lim, S. Y., Lee, D. H., Min, K. H., and Kim, C. M. (2000). Incidence and characterization of Listeria monocytogenes from domestic and imported foods in Korea. J. Food Prot. 63, 186–189. doi: 10.4315/0362-028x-63.2.186
Braga, V., Vazquez, S., Vico, V., Pastorino, V., Ines Mota, M., Legnani, M., et al. (2017). Prevalence and serotype distribution of Listeria monocytogenes isolated from foods in Montevideo-Urugway. Braz. J. Microbiol. 48, 689–694. doi: 10.1016/j.bjm.2017.01.010
Burall, L. S., Simpson, A. C., and Datta, A. R. (2011). Evaluation of a serotyping scheme using a combination of an antibody-based serogrouping method and multiplex PCR assay for identifying the major serotypes of Listeria monocytogenes. J. Food Prot. 74, 403–409. doi: 10.4315/0362-028X.JFP-10-355
Cabal, A., Pietzka, A., Huhulescu, S., Allerberger, F., Ruppitsch, W., and Schmid, D. (2019). Isolate based surveillance of Listeria monocytogenes by whole genome sequencing in Austria. Front. Microbiol. 10:2282. doi: 10.3389/fmicb.2019.02282
Cantinelli, T., Chenal-Francisque, V., Diancourt, L., Frezal, L., Leclercq, A., Wirth, T., et al. (2013). “Epidemic clones” of Listeria monocytogenes are widespread and ancient clonal groups. J. Clin. Microbiol. 51, 3770–3779. doi: 10.1128/JCM.01874-13
Carpentier, B., and Cerf, O. (2011). Review – persistence of Listeria monocytogenes in food industry equipment and premises. Int. J. Food Microbiol. 145, 1–8. doi: 10.1016/j.ijfoodmicro.2011.01.005
Chenal-Francisque, V., Lopez, J., Cantinelli, T., Caro, V., Tran, C., Leclercq, A., et al. (2011). Worldwide distribution of major clones of Listeria monocytogenes. Emerg. Infect. Dis. 17, 1110–1112. doi: 10.3201/eid1706.101778
Demaître, N., Van Damme, I., De Zutter, L., Geeraerd, A. H., Rasschaert, G., and De Reu, K. (2020). Occurrence, distribution and diversity of Listeria monocytogenes contamination on beef and pig carcasses after slaughter. Meat Sci. 169:108177. doi: 10.1016/j.meatsci.2020.108177
Dreyer, M., Aguilar-Bultet, L., Rupp, S., Guldimann, C., Stephan, R., Schock, A., et al. (2016). Listeria monocytogenes sequence type 1 is predominant in ruminant rhombencephalitis. Sci. Rep. 6:36419. doi: 10.1038/srep36419
Ebner, R., Stephan, R., Althaus, D., Brisse, S., Maury, M., and Tasara, T. (2015). Phenotypic and genotypic characteristics of Listeria monocytogenes strains isolated during 2011-2014 from different food matrices in Switzerland. Food Control 57, 321–326. doi: 10.1016/j.foodcont.2015.04.030
EFSA and ECDC (2021). The European Union One Health 2019 zoonoses report. EFSA J. 19:e06406. doi: 10.2903/j.efsa.2021.6406
Fagerlund, A., Langsrud, S., and Møretrø, T. (2020). In-depth longitudinal study of Listeria monocytogenes ST9 isolates from the meat processing industry: resolving diversity and transmission patterns using whole-genome sequencing. Appl. Environ. Microbiol. 86:e00579–20. doi: 10.1128/AEM.00579-20
Félix, B., Feurer, C., Maillet, A., Guillier, L., Boscher, E., Kerouanton, A., et al. (2018). Population genetic structure of listeria monocytogenes strains isolated from the pig and pork production chain in France. Front. Microbiol. 9:684. doi: 10.3389/fmicb.2018.00684
Félix, B., Sevellec, Y., Palma, F., Felten, A., Radomski, N., Mallet, L., et al. (2020). A European-Wide Dataset to Decipher Adaptation Mechanisms of Listeria monocytogenes to Diverse Ecological Niches (unpublished, in revision). In Scientific Data. London: Nature Publishing Group.
Fritsch, L., Guillier, L., and Augustin, J.-C. H. (2018). Next generation quantitative microbiological risk assessment: refinement of the cold smoked salmon-related listeriosis risk model by integrating genomic data. Microb. Risk Anal. 10, 20–27. doi: 10.1016/j.mran.2018.06.003
Gelbíčová, T., Florianová, M., Tomaštíková, Z., Pospíšilová, L., Koláčková, I., and Karpíšková, R. (2019). Prediction of persistence of Listeria monocytogenes ST451 in a Rabbit meat processing plant in the czech republic. J. Food Prot. 82, 1350–1356.
Gianfranceschi, M. V., D’Ottavio, M. C., Gattuso, A., Bella, A., and Aureli, P. (2009). Distribution of serotypes and pulsotypes of Listeria monocytogenes from human, food and environmental isolates (Italy 2002-2005). Food Microbiol. 26, 520–526. doi: 10.1016/j.fm.2009.03.003
Graves, L. M., and Swaminathan, B. (2001). PulseNet standardized protocol for subtyping Listeria monocytogenes by macrorestriction and pulsed-field gel electrophoresis. Int. J. Food Microbiol. 65, 55–62. doi: 10.1016/s0168-1605(00)00501-8
Haase, J. K., Didelot, X., Lecuit, M., and Korkeala, H. L., monocytogenes Mlst Study Group and Achtman, M. (2014). The ubiquitous nature of Listeria monocytogenes clones: a large-scale multilocus sequence typing study. Environ. Microbiol. 16, 405–416. doi: 10.1111/1462-2920.12342
Halbedel, S., Prager, R., Fuchs, S., Trost, E., Werner, G., and Flieger, A. (2018). Whole-genome sequencing of recent Listeria monocytogenes isolates from Germany reveals population structure and disease clusters. J. Clin. Microbiol. 56:e00119-18. doi: 10.1128/JCM.00119-18
Henri, C., Felix, B., Guillier, L., Leekitcharoenphon, P., Michelon, D., Mariet, J. F., et al. (2016). Population genetic structure of Listeria monocytogenes strains as determined by pulsed-field gel electrophoresis and multilocus sequence typing. Appl. Environ. Microbiol. 82, 5720–5728. doi: 10.1128/AEM.00583-16
Holch, A., Webb, K., Lukjancenko, O., Ussery, D., Rosenthal, B. M., and Gram, L. (2013). Genome sequencing identifies two nearly unchanged strains of persistent Listeria monocytogenes isolated at two different fish processing plants sampled 6 years apart. Appl. Environ. Microbiol. 79, 2944–2951. doi: 10.1128/AEM.03715-12
Holotová, M., Horská, E., and Nagyová, Ĺ (2021). Changing patterns of sustainable food consumption regarding environmental and social impact-insights from Slovakia. Front. Sustain. Food Syst. 5:703827. doi: 10.3389/fsufs.2021.703827
Jackson, B. R., Tarr, C., Strain, E., Jackson, K. A., Conrad, A., Carleton, H., et al. (2016). Implementation of nationwide real-time whole-genome sequencing to enhance listeriosis outbreak detection and investigation. Clin. Infect. Dis. 63, 380–386. doi: 10.1093/cid/ciw242
Janakiraman, V. (2008). Listeriosis in pregnancy: diagnosis, treatment, and prevention. Rev. Obstet. Gynecol. 1, 179–185.
Kim, S. W., Haendiges, J., Keller, E. N., Myers, R., Kim, A., Lombard, J. E., et al. (2018). Genetic diversity and virulence profiles of Listeria monocytogenes recovered from bulk tank milk, milk filters, and milking equipment from dairies in the United States (2002 to 2014). PLoS One 13:e0197053. doi: 10.1371/journal.pone.0197053
Kramarenko, T., Roasto, M., Meremäe, K., Kuningas, M., Põltsama, P., and Elias, T. (2013). Listeria monocytogenes prevalence and serotype diversity in various foods. Food Control 30, 24–29. doi: 10.1016/j.foodcont.2012.06.047
Kuch, A., Goc, A., Belkiewicz, K., Filipello, V., Ronkiewicz, P., Gołȩbiewska, A., et al. (2018). Molecular diversity and antimicrobial susceptibility of Listeria monocytogenes isolates from invasive infections in Poland (1997–2013). Sci. Rep. 8:14562. doi: 10.1038/s41598-018-32574-0
Lachtara, B., Osek, J., and Wieczorek, K. (2021). Molecular typing of Listeria monocytogenes IVb serogroup isolated from food and food production environments in Poland. Pathogens 10:482. doi: 10.3390/pathogens10040482
Linke, K., Rückerl, I., Brugger, K., Karpiskova, R., Walland, J., Muri-Klinger, S., et al. (2014). Reservoirs of Listeria species in three environmental ecosystems. Appl. Environ. Microbiol. 80, 5583–5592. doi: 10.1128/AEM.01018-14
Lundén, J., Tolvanen, R., and Korkeala, H. (2004). Human Listeriosis outbreaks linked to dairy products in Europe. J. Dairy Sci. 87(E. Suppl.), E6–E11.
Lüth, S., Deneke, C., Kleta, S., and Al Dahouk, S. (2021). Translatability of WGS typing results can simplify data exchange for surveillance and control of Listeria monocytogenes. Microbial Genomics 7:000491. doi: 10.1099/mgen.0.000491
Martín, B., Perich, A., Gomez, D., Yanguela, J., Rodríguez, A., Garriga, M., et al. (2014). Diversity and distribution of Listeria monocytogenes in meat processing plants. Food Microbiol. 44, 119–127. doi: 10.1016/j.fm.2014.05.014
Maury, M. M., Bracq-Dieye, H., Huang, L., Vales, G., Lavina, M., Thouvenot, P., et al. (2019). Hypervirulent Listeria monocytogenes clones’ adaption to mammalian gut accounts for their association with dairy products. Nat. Commun. 10, 1–13. doi: 10.1038/s41467-019-10380-0
Maury, M. M., Tsai, Y. H., Charlier, C., Touchon, M., Chenal-Francisque, V., Leclercq, A., et al. (2016). Uncovering Listeria monocytogenes hypervirulence by harnessing its biodiversity. Nat. Genet. 48, 308–313. doi: 10.1038/ng.3501
Meloni, D., Consolati, S. G., Mazza, R., Mureddu, A., Fois, F., Piras, F., et al. (2014). Presence and molecular characterisation of the major serovars of Listeria monocytogenes in ten Sardinian fermented sausage processing plants. Meat Sci. 97, 443–450. doi: 10.1016/j.meatsci.2014.02.012
Michelon, D., Félix, B., Vingadassalon, N., Mariet, J. F., Larsson, J. T., Møller-Nielsen, E., et al. (2015). PFGE Standard operating procedures for Listeria monocytogenes: harmonizing the typing of food and clinical strains in Europe. Foodborne Pathog. Dis. 12, 244–252. doi: 10.1089/fpd.2014.1877
Morganti, M., Scaltriti, E., Cozzolino, P., Bolzoni, L., Casadei, G., Pierantoni, M., et al. (2015). Processing-dependent and clonal contamination patterns of Listeria monocytogenes in the cured ham food chain revealed by genetic analysis. Appl. Environ. Microbiol. 82, 822–831. doi: 10.1128/AEM.03103-15
Moura, A., Criscuolo, A., Pouseele, H., Maury, M. M., Leclercq, A., Tarr, C., et al. (2016). Whole genome-based population biology and epidemiological surveillance of Listeria monocytogenes. Nat. Microbiol. 2:16185. doi: 10.1038/nmicrobiol.2016.185
Moura, A., Tourdjman, M., Leclercq, A., Hamelin, E., Laurent, E., Fredriksen, N., et al. (2017). Real-time whole-genome sequencing for surveillance of Listeria monocytogenes, France. Emerg. Infect. Dis. 23, 1462–1470. doi: 10.3201/eid2309.170336
Neuman, J. A., Isakov, O., and Shomron, N. (2013). Analysis of insertion-deletion from deep-sequencing data: software evaluation for optimal detection. Brief. Bioinform. 14, 46–55. doi: 10.1093/bib/bbs013
Nielsen, E. M., Björkman, J. T., Kiil, K., Grant, K., Dallman, T., Painset, A., et al. (2017). Closing gaps for performing a risk assessment on Listeria monocytogenes in ready-to-eat (RTE) foods: activity 3, the comparison of isolates from different compartments along the food chain, and from humans using whole genome sequencing (WGS) analysis. EFSA Support. Publ. 14:1151E.
OIE (2018). Chapter 3.9.6. Listeria monocytogenes. Manual of Diagnostic Tests and Vaccines for Terrestrial Animals. Available online at: https://www.oie.int/fileadmin/Home/eng/Health_standards/tahm/3.09.06_LISTERIA_MONO.pdf. (accessed May 07, 2021)
Okada, Y., Okutani, A., Suzuki, H., Asakura, H., Monden, S., Nakama, A., et al. (2011). Antimicrobial susceptibilities of Listeria monocytogenes isolated in Japan. J. Vet. Med. Sci. 73, 1681–1684. doi: 10.1292/jvms.11-0051
Orsi, R. H., den Bakker, H. C., and Wiedmann, M. (2011). Listeria monocytogenes lineages: genomics, evolution, ecology, and phenotypic characteristics. Int. J. Med. Microbiol. 301, 79–96. doi: 10.1016/j.ijmm.2010.05.002
Ortiz, S., López, V., Villatoro, D., López, P., and Carlos Dávila, J. (2010). A 3-year surveillance of the diversity and persistence of Listeria monocytogenes in an Iberian pig slaughterhouse and processing plant. Foodborne Pathog. Dis. 7, 1177–1184. doi: 10.1089/fpd.2010.0535
Painset, A., Björkman, J. T., Kiil, K., Guillier, L., Mariet, J. F., Félix, B., et al. (2019). LiSEQ – whole-genome sequencing of a cross-sectional survey od Listeria monocytogenes in ready-to-eat foods and human clinical cases in Europe. Microb. Genom. 5:e000257. doi: 10.1099/mgen.0.000257
Papić, B., Pate, M., Félix, B., and Kušar, D. (2019). Genetic diversity of Listeria monocytogenes strains in ruminant abortion and rhombencephalitis cases in comparison with the natural environment. BMC Microbiol. 19:299. doi: 10.1186/s12866-019-1676-3
Pietzka, A., Allerberger, F., Murer, A., Lennkh, A., Stöger, A., Cabal Rosel, A., et al. (2019). Whole genome sequencing based surveillance of L. monocytogenes for early detection and investigations of Listeriosis outbreaks. Front. Public Health 7:139. doi: 10.3389/fpubh.2019.00139
Prencipe, V. A., Rizzi, V., Acciari, V., Iannetti, L., Giovannini, A., Serraino, A., et al. (2012). Listeria monocytogenes prevalence, contamination levels and strains characterization throughout the Parma ham processing chain. Food Control 25, 150–158. doi: 10.1016/j.foodcont.2011.10.018
Ragon, M., Wirth, T., Hollandt, F., Lavenir, R., Lecuit, M., Le Monnier, A., et al. (2008). A new perspective on Listeria monocytogenes evolution. PLoS Pathog. 4:e1000146. doi: 10.1371/journal.ppat.1000146
Roussel, S., Michelon, D., Lombard, B., and Lailler, R. (2014). Molecular typing of Listeria monocytogenes strains isolated from food, feed and animals: state of play and standard operating procedures for pulsed-field gel electrophoresis (PFGE) typing, profile interpretation and curation. EFSA Support. Publ. 11:81. doi: 10.2903/sp.efsa.2014.EN-702
Russini, V., Spaziante, M., Zottola, T., Fermani, A. G., Di Giampietro, G., Blanco, G., et al. (2021). A nosocomial outbreak of invasive listeriosis in an italian hospital: epidemiological and genomic features. Pathogens 10:591.
Rychli, K., Wagner, E. M., Ciolacu, L., Zaiser, A., Tasara, T., Wagner, M., et al. (2017). Comparative genomics of human and non-human Listeria monocytogenes sequence type 121 strains. PLoS One 12:e0176857. doi: 10.1371/journal.pone.0176857
Salcedo, C., Arreaza, L., Alcala, B., De La Fuente, L., and Vazquez, J. (2003). Development of a multilocus sequence typing method for analysis of Listeria monocytogenes clones. J. Clin. Microbiol. 41, 757–762. doi: 10.1128/jcm.41.2.757-762.2003
Schmitz-Esser, S., Muller, A., Stessl, B., and Wagner, M. (2015). Genomes of sequence type 121 Listeria monocytogenes strains harbor highly conserved plasmids and prophages. Front. Microbiol. 6:380. doi: 10.3389/fmicb.2015.00380
Slovak Focal Point for the Scientific and Technical Matters of the Efsa. (2020). Summary Report of Zoonosis, Alimentary and Water-Borne Infections in the Slovak Republic in 2019, First Edn. Ministry of Agriculture and Rural Development of the Slovak Republic. Available online at: https://zenodo.org/record/4388999#.YJaWwrUzZPY (accessed May 07, 2021).
Stoller, A., Stevens, M. J. A., Stephan, R., and Guldimann, C. (2019). Characteristics of Listeria monocytogenes strains persisting in a meat processing facility over a 4-year period. Pathogens 8:32. doi: 10.3390/pathogens8010032
Tomaštíková, Z., Gelbíčová, T., and Karpíšková, R. (2019). Population structure of Listeria monocytogenes isolated from human listeriosis cases and from ready-to-eat foods in the Czech Republic. J. Food Nutr. Res. 58, 99–106.
Vazquez-Boland, J. A., Kuhn, M., Berche, P., Chakraborty, T., Dominguez-Bernal, G., Goebel, W., et al. (2001). Listeria pathogenesis and molecular virulence determinants. Clin. Microbiol. Rev. 14, 584–640. doi: 10.1128/CMR.14.3.584-640.2001
Véghová, A., Koreňová, J., Minarovičová, J., Drahovská, H., Siekel, P., and Kaclíková, E. (2015). Isolation and characterization of Listeria monocytogenes from the environment of the three ewe’s milk processing factories in Slovakia. J. Food Nutr. Res. 54, 252–259.
Véghová, A., Minarovičová, J., Koreňová, J., Drahovská, H., and Kaclíková, E. (2016). Prevalence and tracing of persistent Listeria monocytogenes strains in meat processing facility production chain. J. Food Saf. 1–10. doi: 10.1111/jfs.12315
Keywords: Listeria monocytogenes, serotyping, PFGE, WGS, genomic diversity
Citation: Kubicová Z, Roussel S, Félix B and Cabanová L (2021) Genomic Diversity of Listeria monocytogenes Isolates From Slovakia (2010 to 2020). Front. Microbiol. 12:729050. doi: 10.3389/fmicb.2021.729050
Received: 22 June 2021; Accepted: 01 October 2021;
Published: 02 November 2021.
Edited by:
Mostafa Ghanem, University of Maryland, College Park, United StatesReviewed by:
Gonçalo Nieto Almeida, Instituto Nacional Investigaciao Agraria e Veterinaria (INIAV), PortugalSVS Malik, Indian Veterinary Research Institute (IVRI), India
Laurel S. Burall, United States Food and Drug Administration, United States
Vânia Borges Ferreira, Escola Superior de Biotecnologia – Universidade Católica Portuguesa, Portugal
Copyright © 2021 Kubicová, Roussel, Félix and Cabanová. This is an open-access article distributed under the terms of the Creative Commons Attribution License (CC BY). The use, distribution or reproduction in other forums is permitted, provided the original author(s) and the copyright owner(s) are credited and that the original publication in this journal is cited, in accordance with accepted academic practice. No use, distribution or reproduction is permitted which does not comply with these terms.
*Correspondence: Zuzana Kubicová, enV6YW5hLmt1Ymljb3ZhQHN2cHUuc2s=