- 1Key Laboratory of Forage Cultivation, Processing and High Efficient Utilization, Ministry of Agriculture, Key Laboratory of Grassland Resources, Ministry of Education, College of Grassland, Resources and Environment, Inner Mongolia Agricultural University, Hohhot, China
- 2National Engineering Laboratory of Biological Feed Safety and Pollution Prevention and Control, Key Laboratory of Molecular Nutrition, Ministry of Education, Key Laboratory of Animal Nutrition and Feed, Ministry of Agriculture and Rural Affairs, Key Laboratory of Animal Nutrition and Feed Science of Zhejiang Province, Institute of Feed Science, Zhejiang University, Hangzhou, China
This study aimed to isolate and identify lactic acid bacteria (LAB) from the native grass and naturally fermented silage from the Mongolian Plateau. The effect of selected strains on bacterial community and quality of native grass silage was also studied. Strains XM2, 265, and 842 could grow normally at 15°C–30°C, pH 4.0–8.0, and NaCl 3 and 6.5%; they were identified as Lactiplantibacillus plantarum subsp. plantarum, Pediococcus acidilactici, and Latilactobacillus graminis, by sequencing 16S rRNA, respectively. The three strains (XM2, 265, and 842) and one commercial additive (L) were used as inoculants and singularly added to the native grass. Compared to the control, the dry matter content was significantly (p < 0.05) lower in L and XM2 groups. The water-soluble carbohydrate content was significantly (p < 0.05) higher in control than in other groups. Compared with the control, the crude protein and ammonia nitrogen contents were significantly (p < 0.05) higher and lower in the LAB-treated groups, and the acid and detergent fiber contents were significantly (p < 0.05) reduced in the L and XM2 groups than those in other groups. There was a significant (p < 0.05) difference in the pH value, lactic acid content, and lactic acid-to-acetic acid ratio in L and XM2 groups than in other groups. Compared with the control, the number of LAB was significantly (p < 0.05) higher in LAB-treated silages, whereas no significant (p > 0.05) differences were observed in yeast and aerobic bacteria in all groups. Compared to the control, the Shannon index was significantly (p < 0.05) reduced. Simpson and Chao1 were significantly (p < 0.05) increased. Principal coordinate analysis based on the unweighted UniFrac distance showed clear separation of the bacterial community in fresh materials and LAB-treated silages. Besides, compared to the control, the principal coordinate analysis of LAB-treated silages was also separate. After 30 days of fermentation, the relative abundance of Firmicutes increased and was the primary phylum in all silages. Compared with the control, the abundance of Firmicutes and Proteobacteria was significantly (p < 0.05) higher and lower in L and XM2 groups. In contrast, no significant differences were observed among control, 265, and 842 groups. At the genus level, the relative abundance of Lactobacillus, Enterobacter, Pediococcus, and Weissella was increased and dominated the native grass fermentation. Compared with the control, the abundance of Lactobacillus was significantly (p < 0.05) higher in L, XM2, and 842 groups, while no significant (p > 0.05) differences were observed between the control and 265 groups. The abundance of Pediococcus was higher than that in other groups. Consequently, the results demonstrated that LAB significantly influenced silage fermentation by reconstructing microbiota, and Lactobacillus was the dominant genus in the native grass silages. Furthermore, the results showed that strain XM2 could effectively improve the silage quality, and it is considered a potential starter for the native grass silage.
Introduction
Native grasslands are widely distributed in the north and west of China, including the Mongolian Plateau, Qinghai, and the Tibet Plateau, are an essential resource in animal production, and grow well in fall and autumn, providing sufficient nutrition and biomass for animals (Du et al., 2019). Hay is a traditional method for maintaining forage, while the disadvantages of native grass hay, including hay quality and palatability, make it hard to shake off the seasonal and yearly imbalance of available forage (Yan et al., 2019). Ensiling is a traditional way for preservation of animal feed and green forage crops because it can supply forages for animals year-round, effectively reduce the nutrition loss of forages, and prolong storage time (Pahlow et al., 2003). However, the moisture and water-soluble carbohydrate (WSC) content and the number of lactic acid bacteria (LAB) were lower than the requirement for a well-preserved silage (You et al., 2021). Therefore, it is difficult to produce high-quality silage of native grass using natural fermentation.
Generally, LAB additives are a practical method for improving fermentation quality, which is widely used for silage preparation. The LAB group has been selected from various forages, including alfalfa (Ogunade et al., 2018), Elymus nutans (Xu et al., 2018), oat (Romero et al., 2017), King grass (Shah et al., 2018), Moringa oleifera (Wang Y. et al., 2018), native grass (You et al., 2021), and Teff (Tilahun et al., 2018). Ensiling involves complex microbial interactions, and the microbial ecology associated with silages was conducted using classical microbial techniques (Keshri et al., 2019). Studies on the microbial composition during silage fermentation have shown that Lactobacillus often plays an important role during the later stages of ensiling and manufactures the amount of lactic acid to improve silage fermentation (Ding et al., 2019). Previous studies also found that Lactobacillus, Weissella, Pseudomonas, and Leuconostoc spp. in silage samples were the dominant genera (Guan et al., 2018; Keshri et al., 2019; Yang et al., 2019; Bai et al., 2020). Although the isolation, selection, and application of LAB are critical for silage fermentation and bacterial communities, the LAB was isolated from various forages and grasses with different effects on silage fermentation by various environments (Yang et al., 2010; Zhang et al., 2015; Yan et al., 2019), but few LAB have been isolated and applied on native grass in the Mongolian Plateau. Some LAB strains were isolated from the native grass and naturally fermented silage in the Mongolian Plateau in our research. These strains grew under low-pH conditions in an anaerobic environment, producing more lactic acid, and widely used carbohydrates. Still, the effects of these strains on silage fermentation of native grass remain unclear.
Consequently, this study aimed to select and identify LAB strains using physiological and morphological tests and molecular methods from native grass, and its effects on the bacterial community and the silage quality of native grass were also studied.
Materials and Methods
Lactic Acid Bacteria Strains
A total of 23 LAB strains were isolated from 35 native grass and naturally fermented silage samples. The grass was harvested at the milk stage from typical and meadow steppe in Inner Mongolia Plateau, China, in August 2018 and 2019. The grassland contained typical and meadow steppe flora of HulunBuir, Inner Mongolia, with the Giant Feathergrass (Stipa gigantea Link.) and Chinese Leymus (Leymus chinensis [Trin.] Tzvel.), and the Baical Needlegrass (Stipa baicalensis Roshev.) and Chinese Leymus (Leymus chinensis [Trin.] Tzvel.) being the dominant species, respectively. The 10-g silage samples from each silage sample were homogenized with 90 ml of distilled water; serial dilutions were used for potential LAB culturing and purification by streaking on de Man, Rogosa, Sharpe (MRS) agar (Difco Laboratories, Detroit, MI, United States) four times at 30°C for 48 h and stored at −80°C in MRS broth with 20% glycerol.
Physiological and Morphological Tests
Gram staining, catalase activity, and gas production from glucose were determined as previously reported (Duan et al., 2008; You et al., 2021). Growth at different temperatures and pH environments (HCl or NaOH were used for adjusting pH) was based on the method of Cai et al. (1999c). Salt tolerance was determined using the method of Wang S. et al. (2018). The growth was measured and evaluated using optical density (OD) value (You et al., 2021). API 50 CH (BioMérieux, Marcy l’ Etoile, Lyons, France) was used to determine the carbohydrate assimilation (Cai et al., 1999a).
Lactic Acid Bacteria Identification by 16S rRNA Sequencing
To extract the DNA of the screened strains, Bacterial DNA kit was used (Tiangen Biotech Co., Ltd., Beijing, China). The primers 27F (5′-AGAGTTTGATCCTGGCTCAG-3′) and 1492R (5′-TACGGCTACCTTGTTACGACT-3′) were used in the polymerase chain reaction (PCR) (Cai et al., 1999b). The species identification was conducted as previously reported (Zhang et al., 2015). The 16S rRNA sequences were identified using BLAST analysis on GenBank (Ennahar et al., 2003).
Preparation of Silage
Native grass was harvested at the milk stage on August 20, 2020, in Chifeng, China. The native grassland was meadow steppe flora of Baarin Left Banner, Inner Mongolian Plateau, including Giant Feather Grass (S. gigantea Link.), Chinese Leymus [L. chinensis (Trin.) Tzvel.], and Dahurian Bushclover [Lespedeza davurica (Laxm.) Schindl] as the dominant species. Strains XM2, 265, and 842, and commercial LAB additive (defined as L, CH, Lactobacillus plantarum, Snow Brand Seed Co., Ltd, Sapporo, Japan) were used as inoculants. All LAB strains were added singularly at 105 cfu/g fresh materials (FM), and the control was added to the same volume of distilled water (Wang Y. et al., 2018; You et al., 2021). The FM was chopped using a manual forage chopper (Xianglong Co., Ltd., Linyi, China) with 2- to 3-cm lengths and was immediately moved to the laboratory. About 500 g of FM in a plastic polyethylene bottle (1 L capacity) and air were eliminated. Each treatment was prepared with three replicates and stored at ambient temperature for 30 days.
Chemical and Fermentation Characteristics Analysis
Dry matter content of raw material and 30 days of fermentation of native grass silage samples was determined by drying samples in a forced-air oven at 65°C for 72 h. However, based on the Association of Official Analytical Chemists, crude protein (CP) content was determined (Association of Official Analytical Chemistry, 1990). Fiber fractions were determined according to the method of Van Soest et al. (1991). The WSC content was determined as previously reported (Murphy, 1958).
The 10-g sample was added to 90 ml of distilled water and remained for 24 h at 4°C in a refrigerator, and the extract was filtered through four layers of cheesecloth. The pH value and organic acid contents were determined using the method of You et al. (2021). The ammonia nitrogen (NH3-N) content was assessed as described by Kleinschmit et al. (2005).
Microbial Composition Analysis
The microbial compositions of FM and silages were analyzed using the plate count method. Under anaerobic conditions on MRS agar incubated at 30°C, the counts of LAB were quantified for 48 h (You et al., 2021); coliform bacteria, aerobic bacteria, mold, and yeast were calculated using the previously reported method (You et al., 2021) and expressed on colony-forming units (cfu)/g of FM.
DNA Extraction, Polymerase Chain Reaction Amplification, and Sequencing
Bacterial community genomic DNA was extracted from native grass and silage samples using the E.Z.N.A.® sample DNA kit (Omega Bio-tek, Norcross, GA, United States). The NanoDrop 2000 UV-vis Spectrophotometer (Thermo Scientific, Wilmington, United States) was used in determining the concentration and purity of the extracted DNA and 1% agarose gel electrophoresis was used to analyze the quality of extracted DNA (Bai et al., 2020). All extracted DNA samples were frozen at −20°C for analysis.
Majorbio Bio-Pharm Technology Co., Ltd. (Shanghai, China) performed PCR amplification and bioinformatic analysis. The hypervariable region V3–V4 of the bacterial 16S rRNA gene was amplified using primer 338F (5′-ACTCCTACGGGAGG CAGCAG-3′) and 806R (5′-GGACTACHVGGGTWTCTAAT-3′). The 16S amplification was conducted according to the description of Tian et al. (2017). Raw sequence data were uploaded to the NCBI’s Sequence Read Archive under study accession number PRJNA758799.
Statistical Analyses
Paired-end reads were assigned to samples based on their unique barcode, truncated by cutting off the barcode and primer sequence and merged using FLASH (v1.2.8) (Mago and Salzberg, 2011). Under specific filtering conditions, quality filtering on raw tags was performed to obtain high-quality clean tags according to fqtrim (v0.94) (Li et al., 2021). The chimeric sequences were filtered using Vsearch software (v2.3.4) (Rognes et al., 2016), and the high-quality sequences with ≥ 97% similarity were assigned to the same operational taxonomic units (OTUs) (Li et al., 2021). Taxonomic summaries were conducted by calculating the relative abundance across samples and normalizing to 100%. Alpha diversity and beta diversity were computed by normalizing to the same sequences randomly using QIIME2. OmicStudio tools1 were used to perform most of the graphics drawing. The chemical composition, silage quality, and microorganism population of silages were performed using a one-way analysis of variance with three replicates. Duncan’s tests separated significant differences and were considered statistically significant at the 5% probability level (SAS Inc, 2007).
Results
The Selection of Isolated Lactic Acid Bacteria Based on Morphological and Physiological Tests
The selection of isolated LAB based on morphological and physiological tests is indicated in Table 1. All isolated strains were Gram-positive and catalase-negative, the XM2 strain was rod-shaped, and strains 265 and 842 were cocci-shaped. All strains normally grew at 15 and 30°C, pH 4.0–8.0, while strain 265 normally grew at 50°C, and strains XM2 and 842 grew normally at 10°C. Under NaCl concentrations of 3.0 and 6.5%, all isolated strains grew normally. According to the results of gas for glucose, the three strains possessed similar fermentation patterns and were homofermentative, while different ferment carbohydrates are indicated in Table 2. The three isolated strains that could ferment carbohydrates in API are listed in Table 2. In this study, the GenBank data library was used to analyze the similarity through BLAST2, strain XM2 possessed a higher similarity with L. plantarum, strain 265 possessed a higher similarity with Pediococcus acidilactici, and strain 842 possessed a higher similarity with Lactobacillus graminis, with 99.87, 99.58, and 99.63% similarities in their 16S rRNA gene sequence, respectively (Table 3 and Supplementary Figure 1). The strains’ nucleotide sequences were also transferred to GenBank with accession numbers MT358326, MT358327, and MT358333 for XM2, 265, and 842, respectively. Based on the new classification of Lactobacillus genus, strains XM2, 265, and 842 were identified as Lactiplantibacillus plantarum subsp. plantarum, P. acidilactici, and Latilactobacillus graminis, respectively.
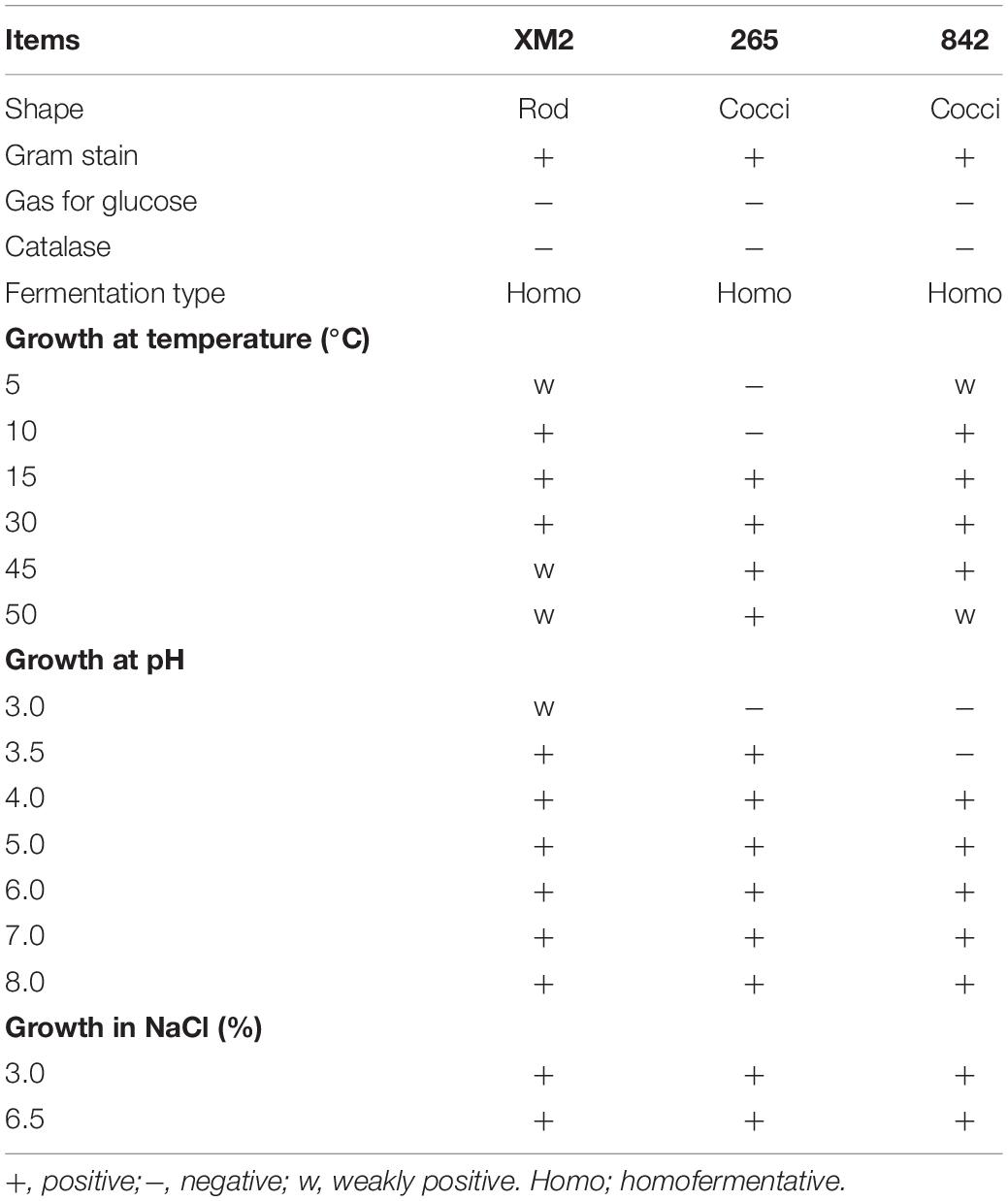
Table 1. The selection of isolated lactic acid bacteria on the base of the morphological and physiological tests.
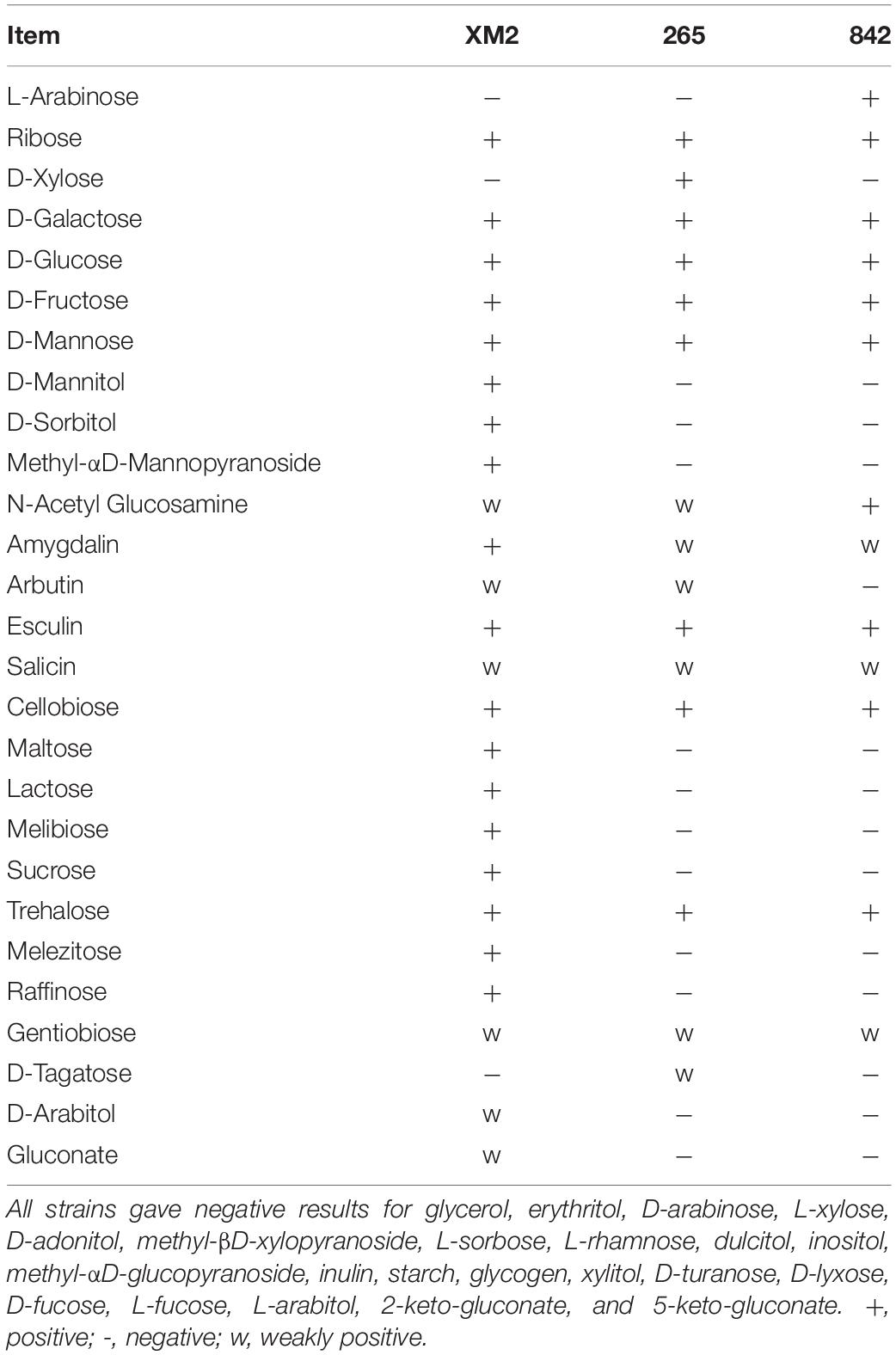
Table 2. The characteristics of isolated lactic acid bacteria on the base of carbohydrate fermentation.
The Chemical Compositions and Microbial Population of the Fresh Materials Before Ensiling
The chemical compositions and microbial population of the substrates prior to ensiling are shown in Table 4. The moisture content of native grass was 40.66%, and WSC, CP, NDF, and ADF were 4.36, 11.74, 58.15, and 30.07% on a DM basis, respectively. Microbial populations in the native grass for LAB, coliform bacteria, aerobic bacteria, and yeasts were 4.01, 6.93, 7.68, and 7.41 log10cfu/g FM, respectively. Mold was not detected in native grass.
Chemical Compositions, Silage Quality, and Microbial Populations of Ensiling
The chemical compositions, silage quality, and microbial populations on 30 days of native grass ensiling are indicated in Table 5. There were significant (p < 0.05) differences in DM contents in the L and XM2 groups than in the control, while no significant (p > 0.05) differences were observed among control, 265, and 842 groups. The WSC content was significantly (p < 0.05) greater in control than in other groups. Compared to control, the NH3-N and CP contents were significantly (p < 0.05) greater and lower than in the L and XM2 groups, respectively. The NDF and ADF contents also significantly decreased in L and XM2 groups compared to that in the control. After 30 days of fermentation, compared to control, the pH in inoculated silages was significantly (p < 0.05) lower, and lactic acid (LA) content and lactic acid-to-acetic acid ratio were significantly (p < 0.05) increased. The number of LAB was significantly (p < 0.05) greater in inoculated silages than in control. No significant (p > 0.05) differences were observed in yeast and aerobic bacteria in all groups, and coliform bacteria and mold were lower than detectable in all groups.
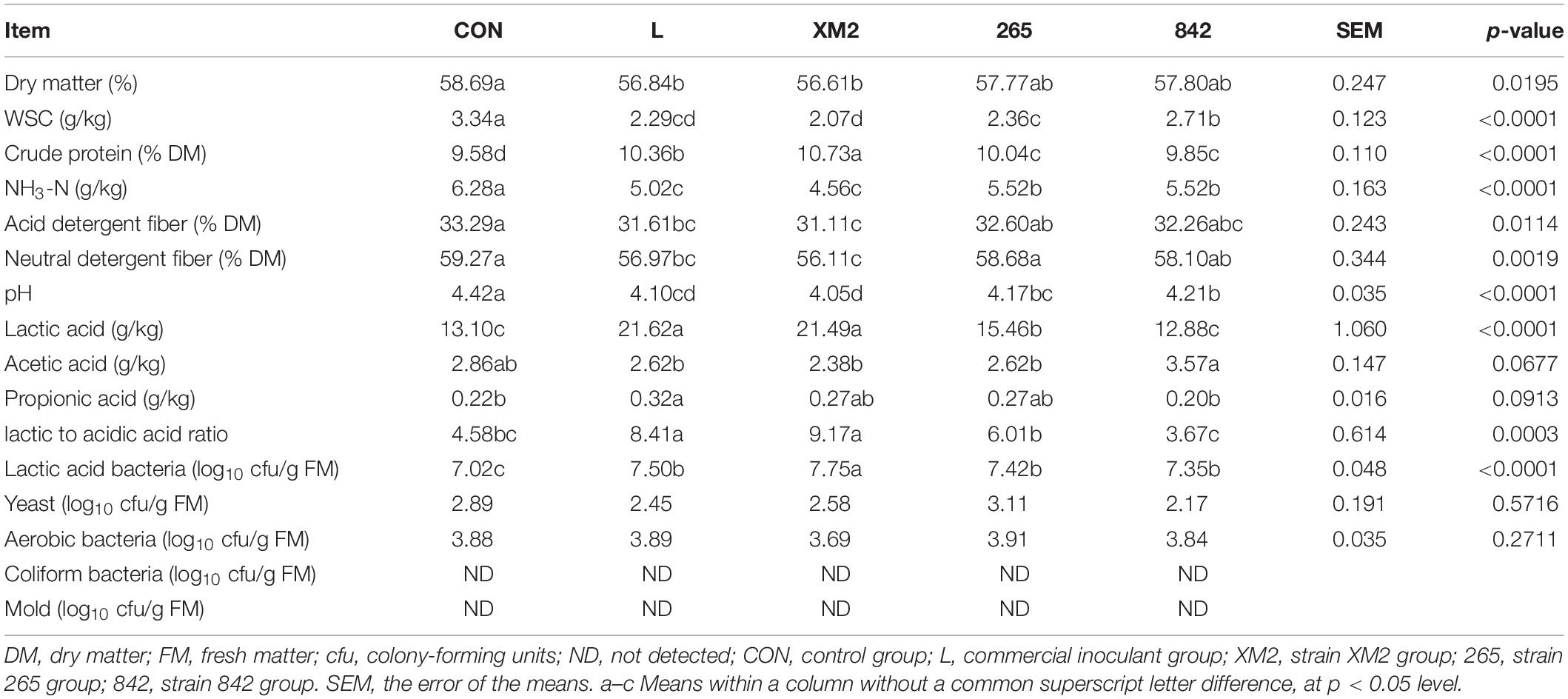
Table 5. Chemical compositions, fermentation characteristics, and microbial populations on 30 days of ensiling.
Microbial Diversity of Fresh Materials and Native Grass After Ensiling
This study also analyzed the bacterial microbiota in silages and identified the species-discriminatory taxa. The sequencing information and bacterial diversity analysis are indicated in Supplementary Table 1. Compared to FM, the OTUs, Shannon, and Chao1 decreased significantly (p < 0.05). The Good’s coverage of all groups was more than 99%. Compared to the control, the Shannon index was significantly (p < 0.05) reduced, and Simpson and Chao1 were significantly (p < 0.05) increased.
Next, principal coordinate analysis (PCoA) was conducted based on the unweighted UniFrac distance to determine whether the microbial community structure changed in FM and silages (Figure 1). The PCoA plot exhibited a clear separation of bacterial community in FM and silages; besides, L, XM2, and other LAB-treated groups were also separate.
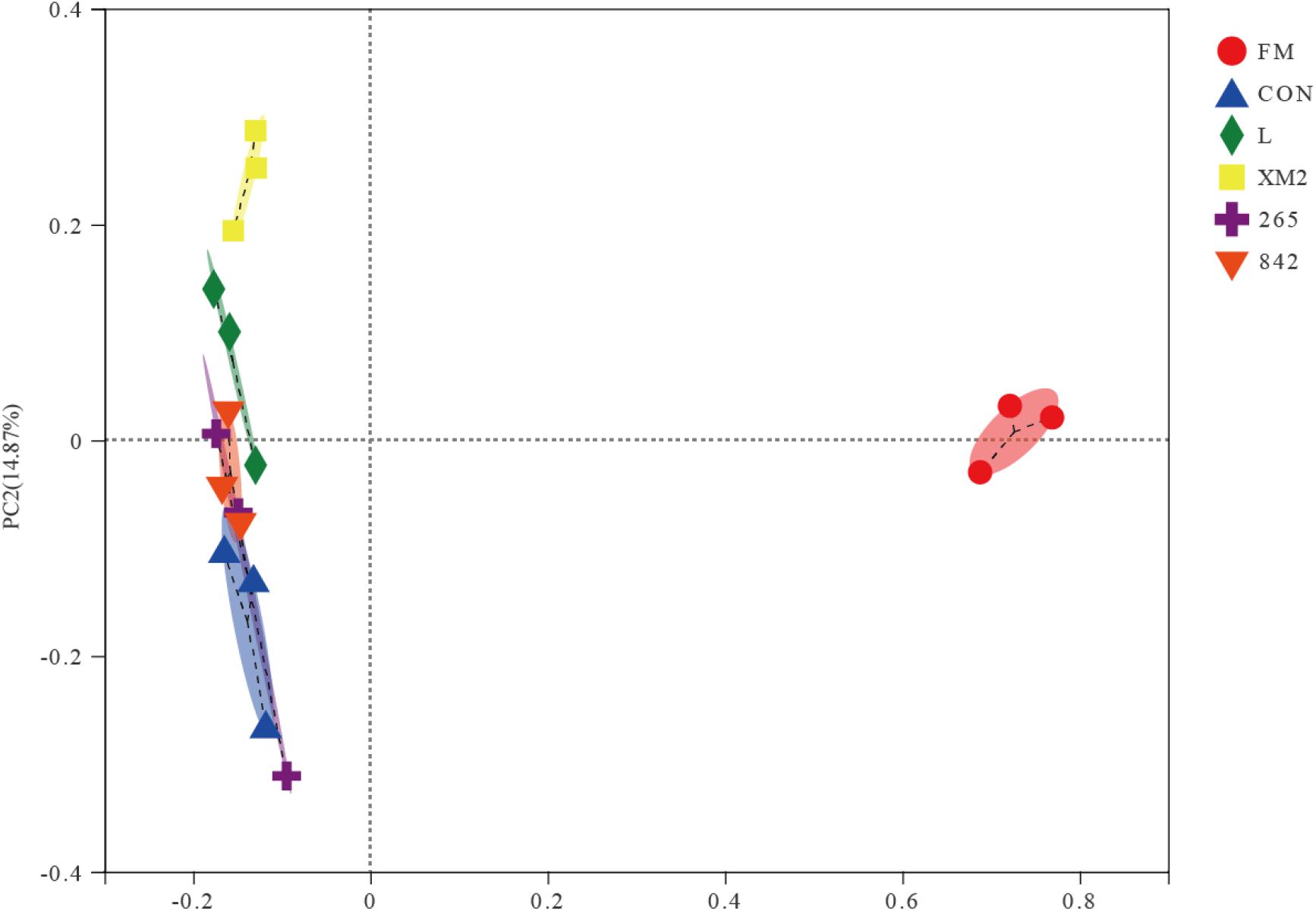
Figure 1. Principal coordinate analysis (PCoA) of the bacterial community of FM and native grass on 30 days of ensiling. FM, fresh native grass; CON, control group; L, commercial inoculant group; XM2, strain XM2 group; 265, strain 265 group; 842, strain 842 group.
The relative abundance of bacteria in FM and silages of 30 days of ensiling are indicated in Figure 2. In FM, the dominant phyla of native grass were Proteobacteria and Actinobacteriota (Figure 2A). After 30 days of ensiling, the relative abundance of Firmicutes increased and was the primary phylum in all silages (80%, Figure 2B), and significant (p < 0.05) differences were observed among FM and silages in Firmicutes, Proteobacteria, and Actinobacteriota at the phylum level (Figure 2B). Compared to control, the abundance of Firmicutes and Proteobacteria was significantly (p < 0.05) higher and lower in L and XM2 groups, whereas no significant differences were observed among control, 265, and 842 groups. In FM, the dominant phyla of native grass were Pantoea, whose abundance was more than 50% (Figure 2C). After 30 days of fermentation, the relative abundance of Lactobacillus, Enterobacter, Pediococcus, and Weissella was increased and dominated the native grass fermentation among silages (80%, Figure 2B), and significant (p < 0.05) differences were observed among FM and silages in Lactobacillus, Enterobacter, Pediococcus, and Weissella (Figure 2D). Compared to control, the abundance of Lactobacillus was significantly (p < 0.05) higher in L, XM2, and 842 groups, while no significant (p > 0.05) difference was observed between control and 265 groups. The abundance of Pediococcus was the (p < 0.05) highest than in other groups.
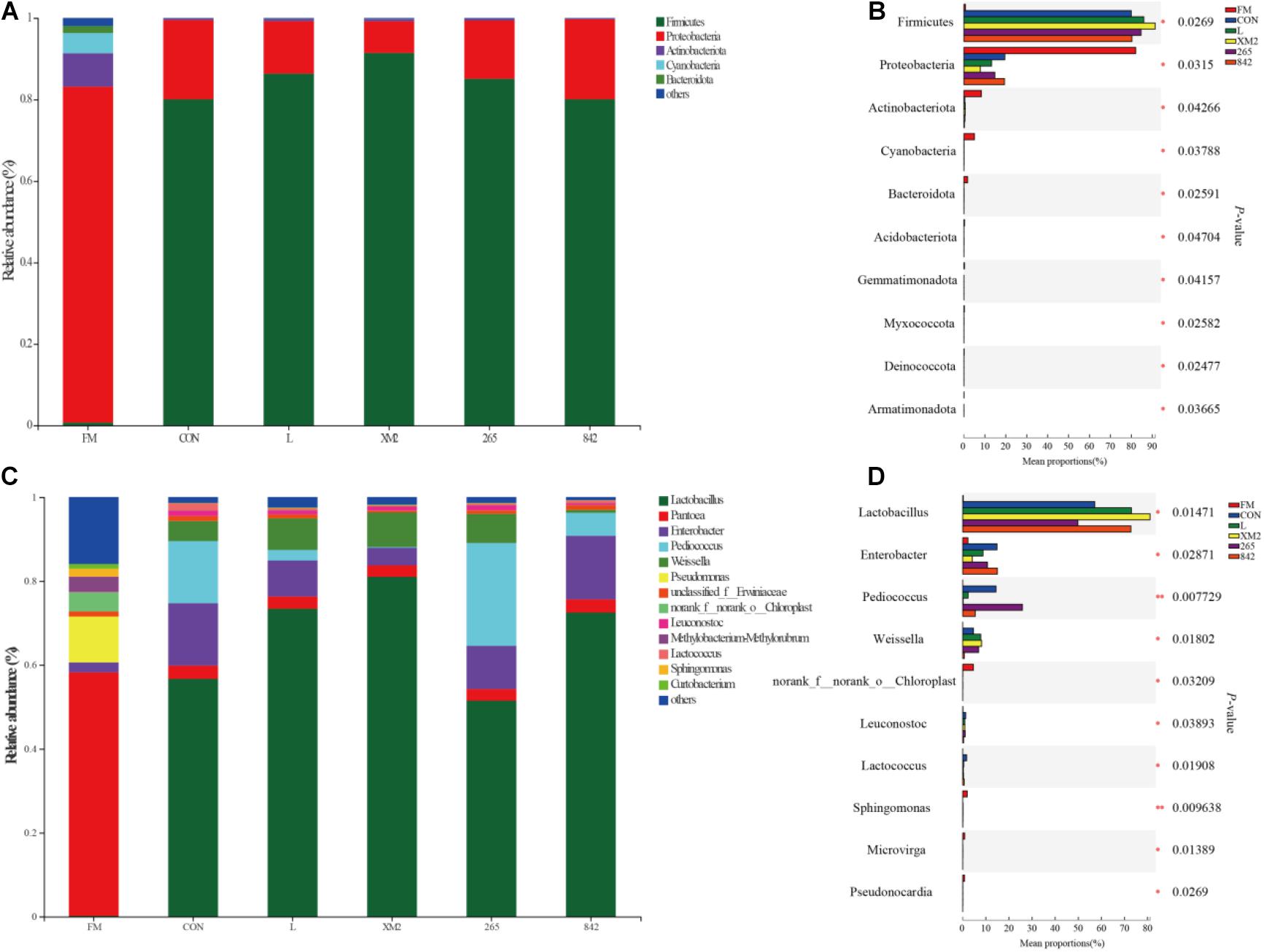
Figure 2. The bacterial community of FM and native grass on 30 days of ensiling. (A) The bacterial community was shown at the phylum level. (B) The extended error bar plot displaying the significant differences among groups at the phylum level (C). The bacterial community was shown at the genus level. (D) The extended error bar plot displaying significant differences among groups at the genus level. *Shows that the significant difference was at p < 0.05 level. FM, fresh native grass; CON, control group; L, commercial inoculant group; XM2, strain XM2 group; 265, strain 265 group; 842, strain 842 group.
The linear discriminant analysis effect size (LefSe) was applied to explore the relative richness (p < 0.05, LDA > 3.0) of silages (Figure 3). The Lactobacillus was enriched in the XM2 group, and Pediococcus was enriched in the 265 group.
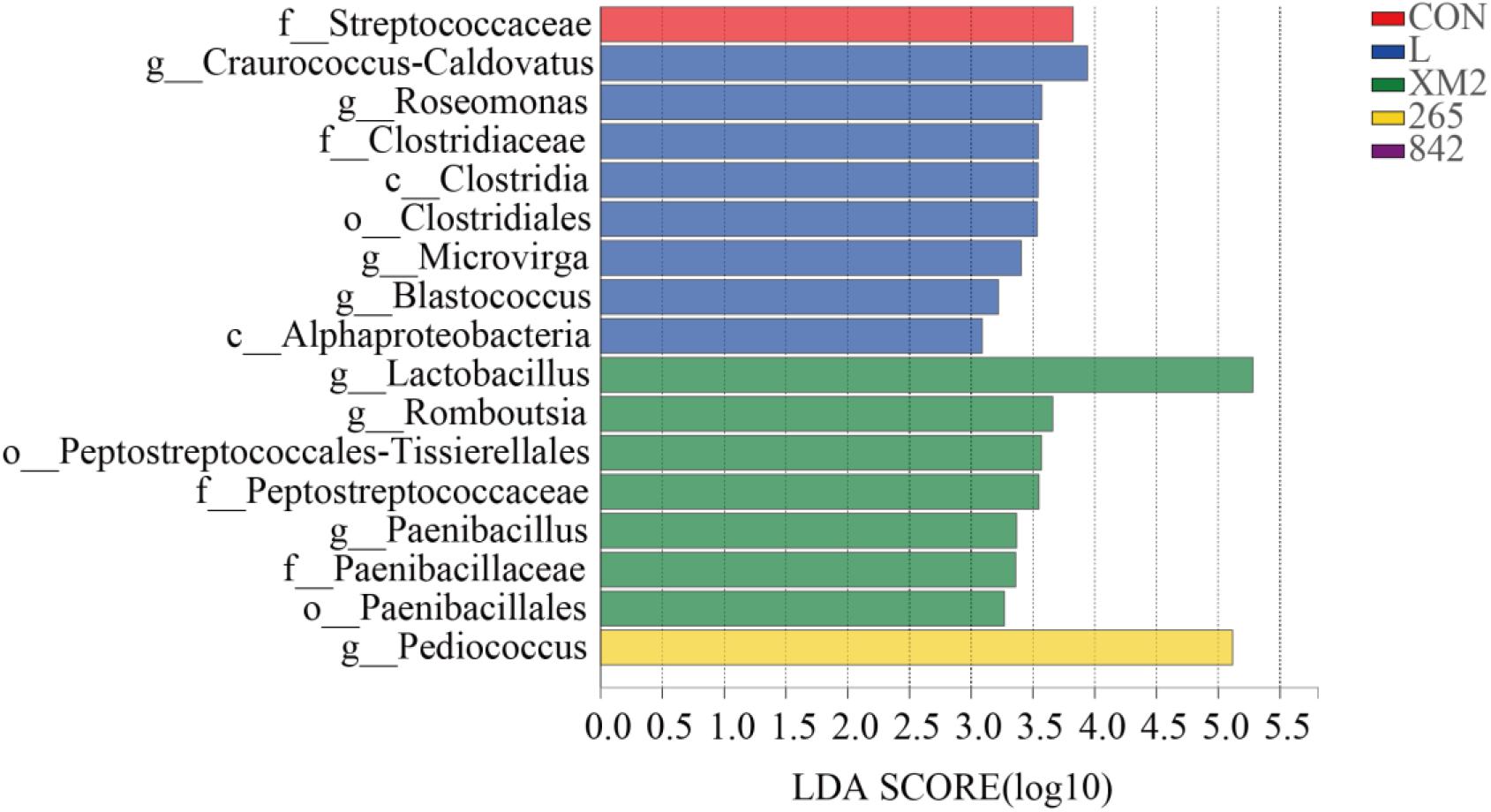
Figure 3. The linear discrimination analysis (LDA) coupled on the bacterial community of native grass on 30 days of ensiling with effect size (LEfSe) analysis. The significant difference in species was estimated by an LDA score greater at default score = 3. The length of the histogram shows the LDA score of differences in these groups. CON, control group; L, commercial inoculant group; XM2, strain XM2 group; 265, strain 265 group; 842, strain 842 group.
Discussion
Forage crops, silages, and dairy products have been found in various LAB species, and many isolates have been identified as the Lactobacillus group (You et al., 2021). Previously published studies also showed that Lactobacillus is the primary microorganism on forages and silages (Cai et al., 1999c). However, it is challenging to identify the species among differentiated species by morphological, physiological, and biochemical tests (Chen et al., 2013). The 16S rDNA sequence analysis identifies organisms by genus and species (Ennahar et al., 2003).
Similarly, strains XM2, 265, and 842 at pH 4.0 could grow normally and strain XM2 weakly grew at pH 3.0. These results showed that strain XM2 had a high tolerance to acidity and the ability to grow and thrive in low-pH environments, which agree with the findings that L. plantarum displayed high resistance to a low-pH environment (Wang S. et al., 2018). Additionally, these isolated strains grew normally from 5°C to 30°C. While significant differences were observed among these strains, strains XM2 and 842 grew normally at 10°C, and strain 867 grew well at 50°C, which could be attributed to the unique environment, long periods of natural selection, and evolution on the Inner Mongolian Plateau (You et al., 2021). Therefore, the unique traits of these isolated LAB strains could have applications as additives on silage fermentation. What is more, strain XM2 could ferment more substrates than the other strains, which is consistent with the previous report that shows that the L. plantarum group could ferment a wide variety of carbohydrates (McDonald et al., 1991).
The moisture content of the grass is one of the essential factors that can directly influence the silage fermentation quality. In this study, the moisture content of native grass agrees with the previous report that shows the range of 44.93–47.51% in meadow steppe on the Mongolian Plateau (Hou et al., 2017). The plant diversity and environment may contribute to the difference. The WSC content was lower than an adequate WSC concentration that good silage needs WSC content higher than 5% on DM for LAB fermentation (Amer et al., 2012). This result follows the previous study on the low WSC content in native grass (Hou et al., 2017). The CP, ADF, and NDF contents followed those of Du et al. (2019), who discovered the native grass with a lower CP content and higher NDF and ADF contents.
Generally, the silage fermentation process and fermentation quality were determined by the counts and species of epiphytic LAB (Yan et al., 2019). A previously published study showed that the well-preserved silages need the numbers of Lactobacilli to be at least 105 cfu/g FM of ensiling (Cai et al., 1999c). However, in this study, the numbers of LAB and undesirable microorganisms were lower (105 cfu/g) and higher (104–108 cfu/g) in FM than the required values, which could lead to poor fermentation quality. Additionally, LAB groups also included other species that may have few effects on fermentation. Consequently, the use of LAB additives is necessary for native grass silage; not only the low moisture, WSC content, and LAB counts, but also the growth of undesirable microorganisms was inhibited during the early stages of fermentation.
The DM content was lower in the inoculated groups than in the control in this study. Besides, the WSC content was significantly reduced in inoculated silages compared to the control silage, which agrees with Kleinschmit and Kung (2006), who found that grass silages with inoculant had a lower WSC content than that in the control. The WSC contents were fermented by LAB and transformed into organic acids, ethanol, and carbon dioxide by microorganisms during silage fermentation (Muck et al., 2017; Wang S. et al., 2018). The acid hydrolysis during ensiling also reduced the DM content (Zhao et al., 2018). Therefore, the DM, WSC, NDF, ADF, and pH decreased in inoculated silages. The CP and NH3-N contents in control were lower and greater than those in the inoculated silages, reflecting the growth of undesirable microorganisms and the accumulation of NH3-N during ensiling (Dong et al., 2020). The higher CP and lower NH3-N contents in inoculated silages may be attributed to the lower pH, which inhibits the growth and activities of undesirable microorganisms (Arriola et al., 2011; Heinritz et al., 2012).
The pH value in inoculated silages was lower than that in the control, while the lactic acid in the control was lower than that in L, XM2, and 265 groups. These results may be attributed to a high amount of LA with reducing pH values in the anaerobic environment (Fiyla, 2003). A previous study indicated that a pH of less than 4.20 could inhibit the growth of harmful bacteria and ensure fermentation quality (Wang et al., 2019). The pH value of the control was significantly higher than that of inoculated silages, and the pH value was 4.42. The lower number of LAB and higher numbers of yeasts and aerobic bacteria may be the main reason because the LA and other nutrients used by yeasts, molds, and other aerobic microorganisms produce large quantities of metabolism, resulting in pH increase (Woolford, 1990). In this study, the coliform bacteria and mold were undetectable after 30 days of fermentation, which is in agreement with the report that coliform bacteria and mold were not detected after ensiling for 30 days (Dong et al., 2020). The growth of coliform bacteria and mold inhibited by lower pH was the main reason (Lima et al., 2010; Reich and Kung, 2010).
Amplicon sequencing of bacteria in fresh native grass and silage samples was performed. Good’s coverage values in fresh matter and silages were higher than 99%, suggesting that sequencing could adequately reflect the dynamic change in the bacterial community (Yang et al., 2019). Compared to FM, the diversity and richness were reduced in inoculated silages, especially in inoculated silages, which follow Ogunade et al. (2018), who found that LAB additives could reduce the bacterial diversity due to the increase in the abundance of the predominant genus. The antibacterial activity that affects the composition of different bacteria and the lower pH inhibiting the growth of the desirable bacteria in this study may be the main reason (Bai et al., 2020). Compared to the control, the diversity and species richness of the microbial communities in the LAB-treated silages decreased, which agrees with previous studies that show that the addition of LAB could reduce diversity and richness (Dong et al., 2019).
The PCoA plot showed a clear separation of bacteria in FM and silages, which indicated that ensiling reconstructs the microbial community. These results followed the work of Zeng et al. (2020) that showed that the bacterial communities were distinguished in FM and silages. Besides, compared to the control, the PCoA of LAB-treated silages was also separate, which showed that the additives significantly influenced the microbial community.
This study showed that Proteobacteria was the most abundant phylum in FM, comprising more than 80% of the microflora, which agrees with reports that Proteobacteria was the dominant phylum in fresh forage (Dong et al., 2019). After 30 days of ensiling, the abundance of Firmicutes increased and dominated the fermentation in the native grass silages (80%), and the abundance of Proteobacteria decreased. These results were similar to the reports of Yang et al. (2019) and Bai et al. (2020). Compared to the control, the abundance of Firmicutes and Proteobacteria was significantly increased and lower in L and XM2 groups, whereas no significant differences were observed among control, 265, and 842 groups, which the higher microbial populations of LAB could explain.
This study showed that Pantoea was the major facultative aerobic genera in FM. Pantoea has been discovered in fresh alfalfa (Ogunade et al., 2018) and soybean (Ni et al., 2017). After 30 days of fermentation, drops in Pantoea abundances could be attributed to their high sensitivity to pH decline (Ogunade et al., 2018). Lactobacillus, Pedicoccus, Weissella, and Leuconostoc are considered as the four most predominant LAB genera responsible for driving lactic fermentation during ensiling (Pang et al., 2012; Ni et al., 2017; Guan et al., 2018; Liu et al., 2019). During ensiling, a significant shift in the bacterial community from Proteobacteria to Firmicutes could be explained by increased abundance of genera Lactobacillus, Weissella, and Pediococcus, which flourished in the environmental conditions developed during ensiling (Keshri et al., 2019). Therefore, the abundance of Lactobacillus, Enterobacter, Pediococcus, and Weissella increased and dominated the silage fermentation among silages (80%). In this study, the LAB-treated silages exhibited an increase in Lactobacillus than in the control, which agrees with the findings that LAB-treated silages could increase the abundance of Lactobacillus (Liu et al., 2019; Yan et al., 2019; Bai et al., 2020). Additionally, the highest abundance of Lactobacillus was found in the XM2 group; strain XM2 isolated from native grass and the higher microbial populations in the XM2 group may be the main reason. Weissella was the other predominant microbe in all silages throughout the fermentation and was the early colonizer (Graf et al., 2016; Guan et al., 2018); Pediococcus contributed to an initial decline in silage pH, resulting in an anaerobic environment suitable for developing Lactobacillus (Yang et al., 2019). The previously published studies showed that Weissella was heterofermentative. These two LAB genera could not thrive at a pH environment lower than 4.5 and were thus active only during the early stages of ensiling. The follow-up lactic acid production mainly depends on Lactobacillus, which becomes active and thrives as pH decreases (Cai et al., 1998). However, the abundance of Weissella was significantly reduced in the 842 group, which could be explained by adding L. graminis, which could inhibit the growth of Weissella. The abundance of Pediococcus significantly increased in the 265 group, which could contribute to the addition of P. acidilactici. Lactobacillus was enriched in the XM2 group, and Pediococcus was enriched in the 265 group, which is in agreement with the addition of LAB in the XM2 and 265 groups.
Conclusion
The bacterial community of fresh native grass was discovered to be dominated by Proteobacteria, Actinobacteriota, and Cyanobacteria. This study demonstrated that the addition of LAB could influence silage fermentation by reconstructing microbiota. Lactobacillus was the dominant genus in the native grass silages, followed by Enterobacter and Pediococcus. Strain XM2 exhibited the potential possibility to respond to improving silage fermentation in native grass. Further results indicated that strain XM2 could effectively improve the silage quality, and it was proposed to be a potential starter culture for native grass silage.
Data Availability Statement
The original contributions presented in the study are included in the article/Supplementary Material, further inquiries can be directed to the corresponding author/s.
Author Contributions
SY: investigation, methodology, visualization, validation, data curation, writing—original draft, and conceptualization. SD: investigation, software, formal analysis, and writing—review and editing. GG: conceptualization, funding acquisition, supervision, and writing—review and editing. TW: project administration and supervision. YJ: conceptualization, funding acquisition, project administration, and supervision. All authors contributed intellectual input and assisted with this study and manuscript.
Funding
This work was supported by the National Natural Science Fund (31460638), the Science and Technology Major Project of Inner Mongolia (2019ZD997), and Technology Project of Inner Mongolia (2020GG0032), China.
Conflict of Interest
The authors declare that the research was conducted in the absence of any commercial or financial relationships that could be construed as a potential conflict of interest.
Publisher’s Note
All claims expressed in this article are solely those of the authors and do not necessarily represent those of their affiliated organizations, or those of the publisher, the editors and the reviewers. Any product that may be evaluated in this article, or claim that may be made by its manufacturer, is not guaranteed or endorsed by the publisher.
Supplementary Material
The Supplementary Material for this article can be found online at: https://www.frontiersin.org/articles/10.3389/fmicb.2021.731770/full#supplementary-material
Footnotes
References
Amer, S., Hassanat, F., Berthiaume, R., Seguin, P., and Mustafa, A. F. (2012). Effects of water soluble carbohydrate content on ensiling characteristics, chemical composition and in vitro gas production of forage millet and forage sorghum silages. Anim. Feed Sci. Tech. 177, 23–29. doi: 10.1016/j.anifeedsci.2012.07.024
Arriola, K. G., Kim, S. C., and Adesogan, A. T. (2011). Effect of applying inoculants with heterolactic or homolactic and heterolactic bacteria on the fermentation and quality of corn silage. J. Dairy Sci. 94, 1511–1516. doi: 10.3168/jds.2010-3807
Association of Official Analytical Chemistry (1990). Offcial Methods of Analysis, 15th Edn. Arlington, TX: AOAC International.
Bai, J., Xu, D., Xie, D., Wang, M., Li, Z., and Guo, X. (2020). Effects of antibacterial peptide-producing Bacillus subtilis and Lactobacillus buchneri on fermentation, aerobic stability, and microbial community of alfalfa silage. Bioresour. Technol. 315:123881. doi: 10.1016/j.biortech.2020.123881
Cai, Y., Benno, Y., Ogawa, M., and Kumai, S. (1999a). Effect of applying lactic acid bacteria isolated from forage crops on fermentation characteristics and aerobic deterioration of silage. J. Dairy Sci. 82, 520–526. doi: 10.3168/jds.S0022-0302(99)75263-X
Cai, Y., Kumai, S., Ogawa, M., Benno, Y., and Nakase, T. (1999b). Characterization and identification of Pediococcus species isolated from forage crops and their application for silage preparation. Appl. Environ. Microb. 65, 2901–2906.
Cai, Y., Suyanandana, P., Saman, P., and Benno, Y. (1999c). Classification and characterization of lactic acid bacteria isolated from the intestines of common carp and fresh water prawns. J. Gen. Appl. Microbiol. 45, 177–184. doi: 10.2323/jgam.45.177
Cai, Y., Benno, Y., Ogawa, M., Ohmomo, S., Kumai, S., and Nakase, T. (1998). Influence of Lactobacillus spp. from an inoculant and of Weissella and Leuconostoc spp. from forage crops on silage fermentation. Appl. Environ. Microbiol. 64, 2982–2987. doi: 10.1089/oli.1.1998.8.351
Chen, M. M., Liu, Q. H., Xin, G. R., and Zhang, J. G. (2013). Characteritics of lactic acid bacteria isolates and their inoculating effects on the silage fermentation at a high temperature. Lett. Appl. Microbiol. 56, 71–78. doi: 10.1111/lam.12018
Ding, Z. T., Xu, D. M., Bai, J., Li, F. H., Adesogan, A. T., Zhang, P., et al. (2019). Characterization and identification of ferulic acid esterase-producing Lactobacillus species isolated from Elymus nutans silage and their application in ensiled alfalfa. J. Appl. Microbiol. 127, 985–995. doi: 10.1111/jam.14374
Dong, L., Zhang, H., Gao, Y., and Diao, Q. (2020). Dynamic profiles of fermentation characteristics and bacterial community composition of Broussonetia papyrifera ensiled with perennial ryegrass. Bioresour. Technol. 310:123396. doi: 10.1016/j.biortech.2020.123396
Dong, Z. H., Li, J. F., Chen, L., Wang, S. R., and Shao, T. (2019). Effects of freeze–thaw event on microbial community dynamics during red clover ensiling. Front. Microbiol. 10:1559. doi: 10.3389/fmicb.2019.01559
Du, S., You, S., Bao, J., Ge, G., Jia, Y., and Cai, Y. (2019). Evaluation of the growth performance and meat quality of Mongolian lamb fed grass, hay or pellets of Inner Mongolian native grass. Small Rumin. Res. 181, 34–38. doi: 10.1016/j.smallrumres.2019.10.008
Duan, Y., Tan, Z., Wang, Y., Li, Z., Li, Z., Qin, G., et al. (2008). Identification and characterization of lactic acid bacteria isolated from Tibetan Qula cheese. J. Gen. Appl. Microbiol. 54, 51–60.
Ennahar, S., Cai, Y., and Fujita, Y. (2003). Phylogenetic diversity of lactic acid bacteria associated with paddy rice silage as determined by 16S ribosomal DNA analysis. Appl. Environ. Microb. 69, 444–451. doi: 10.1128/AEM.69.1.444-451.2003
Fiyla, I. (2003). The effect of Lactobacillus buchneri and Lactobacillus plantarum on the fermentation, aerobic stability, and ruminal degradability of low dry matter corn and sorghum silages. J. Dairy Sci. 86, 3575–3581. doi: 10.3168/jds.S0022-0302(03)73963-0
Graf, K., Ulrich, A., Ilder, C., and Klocken, M. (2016). Bacterial community dynamics during ensiling of perennial ryegrass at two compaction levels monitored by terminal restriction fragment length polymorphism. J. Appl. Microbiol. 120, 1479–1491. doi: 10.1111/jam.13114
Guan, H., Yan, Y., Li, X., Li, X., Shuai, Y., Feng, G., et al. (2018). Microbial communities and natural fermentation of corn silages prepared with farm bunker-silo in Southwest China. Bioresour. Technol. 265, 282–290. doi: 10.1016/j.biortech.2018.06.018
Heinritz, S. N., Martens, S. D., Avila, P., and Hoedtke, S. (2012). The effect of inoculant and sucrose addition on the silage quality of tropical forage legumes with varying ensilability. Anim. Feed Sci. Tech. 174, 201–210. doi: 10.1016/j.anifeedsci.2012.03.017
Hou, M., Ge, G., Liu, T., Jia, Y., and Cai, Y. (2017). Silage preparation and fermentation quality of natural grasses treated with lactic acid bacteria and cellulase in meadow steppe and typical steppe. Asian Australas. J. Anim. 30, 788–796. doi: 10.5713/ajas.16.0578
Keshri, J., Chen, Y., Pinto, R., Kroupitski, Y., Weinberg, Z. G., and Sela Saldinger, S. (2019). Bacterial dynamics of wheat silage. Front. Microbiol. 10:1532. doi: 10.3389/fmicb.2019.01532
Kleinschmit, D. H., and Kung, L. Jr. (2006). A meta-analysis of the effects of Lactobacillus buchneri on the fermentation and aerobic stability of corn and grass and small-grain silages. J. Dairy Sci. 89, 4005–4013.
Kleinschmit, D. H., Schmidt, R. J., and Kung, L. (2005). The effects of various antifungal additives on the fermentation and aerobic stability of corn silage. J. Dairy Sci. 88, 2130–2139. doi: 10.3168/jds.S0022-0302(05)72889-7
Li, S., Dong, L., Lian, W. H., Lin, Z. L., Lu, C. Y., Xu, L., et al. (2021). Exploring untapped potential of Streptomyces spp. in Gurbantunggut Desert by use of highly selective culture strategy. Sci. Total. Environ. 790:148235. doi: 10.1016/j.scitotenv.2021.148235
Lima, R., Lourenco, M., Diaz, R., Castro, A., and Fievez, V. (2010). Effect of combined ensiling of sorghum and soybean with or without molasses and Lactobacilli on silage quality and in vitro rumen fermentation. Anim. Feed Sci. Technol. 155, 122–131. doi: 10.1016/j.anifeedsci.2009.10.008
Liu, B., Huan, H., Gu, H., Xu, N., Shen, Q., and Ding, C. (2019). Dynamics of a microbial community during ensiling and upon aerobic exposure in lactic acid bacteria in-oculation-treated and untreated barley silages. Bioresour. Technol. 273, 212–219. doi: 10.1016/j.biortech.2018.10.041
Mago, T., and Salzberg, S. L. (2011). FLASH: fast length adjustment of short reads to improve genome assemblies. Bioinformatics 27, 2957–2963. doi: 10.1093/bioinformatics/btr507
McDonald, P., Henderson, A. R., and Heron, S. J. E. (1991). Biochemistry of silage, 2nd Edn. Chacombe: Marlow.
Muck, R. E., Nadeau, E. M. G., McAllister, T. A., Contreras-Govea, F. E., Santos, M. C., and Kung, L. (2017). Silage review: recent advances and future uses of silage additives. J. Dairy Sci. 101, 3980–4000. doi: 10.3168/jds.2017-13839
Murphy, R. P. (1958). A method for the extraction of plant samples and the determination of total soluble carbohydrates. J. Sci. Food Agric. 9, 714–717.
Ni, K. K., Minh, T. T., Tu, T. T., Tsuruta, T., Pang, H., and Nishino, N. (2017). Comparative microbiota assessment of wilted Italian ryegrass, whole crop corn, and wilted alfalfa silage using denaturing gradient gel electrophoresis and next-generation sequencing. Appl. Microbiol. Biotechnol. 101, 1385–1394. doi: 10.1007/s00253-016-7900-2
Ogunade, I. M., Jiang, Y., Pech Cervantes, A. A., Kim, D. H., Oliveira, A. S., and Vyas, D. (2018). Bacterial diversity and composition of alfalfa silage as analysed by Illumina Miseq sequencing: effects of Escherichia coli O157:H7 and silage additives. J. Dairy Sci. 101, 2048–2059. doi: 10.3168/jds.2017-12876
Pahlow, G., Muck, R. E., Driehuis, F., Oude Elferink, S. J. W. H., and Spoelstra, S. F. (2003). Microbiology of Ensiling. Silage Science and Technology. Hoboken, NJ: John Wiley and Sons, Ltd.
Pang, H., Tan, Z., Qin, G., Wang, Y., Li, Z., Jin, Q., et al. (2012). Phenotypic and phylogenetic analysis of lactic acid bacteria isolated from forage crops and grasses in the Tibetan Plateau. J. Microbio. 1, 63–71. doi: 10.1007/s12275-012-1284-5
Reich, L. J., and Kung, L. (2010). Effects of combining Lactobacillus buchneri 40788 with various lactic acid bacteria on the fermentation and aerobic stability of corn silage. Anim. Feed Sci. Technol. 159, 105–109. doi: 10.1016/j.anifeedsci.2010.06.002
Rognes, T., Flouri, T., Nichols, B., Quince, C., and Mahé, F. (2016). Vsearch: a versatile open source tool for metagenomics. PeerJ 4:e2584. doi: 10.7717/peerj.2584
Romero, J. J., Zhao, Y., Balseca-Paredes, M. A., Tiezzi, F., Gutierrez-Rodriguez, E., and Castillo, M. S. (2017). Laboratory silo type and inoculation effects on nutritional composition, fermentation, and bacterial and fungal communities of oat silage. J. Dairy Sci. 100, 1812–1828. doi: 10.3168/jds.2016-11642
Shah, A. A., Yuan, X. J., Zhihao, D., Li, J. F., and Shao, T. (2018). Isolation and molecular identification of lactic acid bacteria from King grass and their application to improve the fermentation quality of sweet Sorghum. World J. Microb. Biotechnol. 34:4. doi: 10.1007/s11274-017-2387-2
Tian, J., He, N., Hale, L., Niu, S., Yu, G., Liu, Y., et al. (2017). Soil organic matter availability and climate drive latitudinal patterns in bacterial diversity from tropical to cold-temperate forests. Funct. Ecol. 32, 61–70. doi: 10.1111/1365-2435.12952
Tilahun, B., Tesfaye, A., Muleta, D., Bahiru, A., Terefework, Z., and Wessel, G. (2018). Isolation and molecular identification of lactic acid bacteria using 16s rRNA genes from fermented teff (Eragrostis tef (Zucc.)) Dough. Int. J. Food Sci. 2018:8510620. doi: 10.1155/2018/8510620
Van Soest, J. P., Robertson, J. B., and Lewis, B. A. (1991). Methods for dietary fiber, neutral detergent fiber and non-starch polysaccharides in relation to animal nutrition. J. Dairy Sci. 74, 3583–3597. doi: 10.3168/jds.S0022-0302(91)78551-2
Wang, S., Li, J., Dong, Z., Chen, L., Yuan, X., and Shao, T. (2018). The effects of lactic acid bacteria strains isolated from various substrates on the fermentation quality of common vetch (Vicia sativa L.) in Tibet. Grass Forage Sci. 73, 639–647. doi: 10.1111/gfs.12363
Wang, Y., He, L., Xing, Y., Zhou, W., Pian, R., Yang, F., et al. (2019). Bacterial diversity and fermentation quality of Moringa oleifera leaves silage prepared with lactic acid bacteria inoculants and stored at different temperatures. Bioresour. Technol. 284, 349–358. doi: 10.1016/j.biortech.2019.03.139
Wang, Y., Wang, C., Zhou, W., Yang, F. Y., Chen, X. Y., and Zhang, Q. (2018). Effects of wilting and Lactobacillus plantarum addition on the fermentation quality and microbial community of Moringa oleifera leaf silage. Front. Microbiol. 9:1817. doi: 10.3389/fmicb.2018.01817
Woolford, M. (1990). The detrimental effects of air on silages. J. Appl. Bacteriol. 68, 101–116. doi: 10.1111/j.1365-2672.1990.tb02554.x
Xu, D. M., Ke, W. C., Zhang, P., Li, F. H., and Guo, X. S. (2018). Characteristics of Pediococcus pentosaceus Q6 isolated from Elymus nutans growing on the Tibetan Plateau and its application for silage preparation at low temperature. J. Appl. Microbiol. 126, 40–48. doi: 10.1111/jam.14110
Yan, Y., Li, X., Guan, H., Huang, L., Ma, X., Peng, Y., et al. (2019). Microbial community and fermentation characteristics of Italian ryegrass silage prepared with corn stover and lactic acid bacteria. Bioresour. Technolol. 279, 177–183. doi: 10.1016/j.biortech.2019.01.107
Yang, J., Cao, Y., Cai, Y., and Tereda, F. (2010). Natural populations of lactic acid bacteria isolated from vegetable residues and silages fermentation. J. Dairy Sci. 93, 3136–3145. doi: 10.3168/jds.2009-2898
Yang, L. L., Yuan, X. J., Li, J. F., Dong, Z. H., and Shao, T. (2019). Dynamics of microbial community and fermentation quality during ensiling of sterile and nonsterile alfalfa with or without Lactobacillus plantarum inoculant. Bioresour. Technol. 275, 280–287. doi: 10.1016/j.biortech.2018.12.067
You, S., Du, S., Ge, G., Wan, T., and Jia, Y. (2021). Selection of lactic acid bacteria from native grass silage and its effects as inoculant on silage fermentation. Agron. J. 113, 3169–3177.
Zeng, T., Li, X., Guan, H., Yang, W., Liu, W., Liu, J., et al. (2020). Dynamic microbial diversity and fermentation quality of the mixed silage of corn and soybean grow in strip intercropping system. Bioresour. Technol. 313:123655. doi: 10.1016/j.biortech.2020.123655
Zhang, Q., Yu, Z., and Wang, X. (2015). Isolating and evaluating lactic acid bacteria strains with or without sucrose for effectiveness of silage fermentation. Grassl. Sci. 61, 167–176. doi: 10.1111/grs.12097
Zhao, J., Dong, Z. H., Li, J. F., Chen, L., Bai, Y. F., Jia, Y. S., et al. (2018). Ensiling as pretreatment of rice straw: the effect of hemicellulase and Lactobacillus plantarum on hemicellulose degradation and cellulose conversion. Bioresour. Technolol. 266, 158–165. doi: 10.1016/j.biortech.2018.06.058
Keywords: isolation, lactic acid bacteria, bacterial community, native grass, fermentation quality
Citation: You S, Du S, Ge G, Wan T and Jia Y (2021) Microbial Community and Fermentation Characteristics of Native Grass Prepared Without or With Isolated Lactic Acid Bacteria on the Mongolian Plateau. Front. Microbiol. 12:731770. doi: 10.3389/fmicb.2021.731770
Received: 28 June 2021; Accepted: 06 September 2021;
Published: 01 October 2021.
Edited by:
Yimin Cai, Japan International Research Center for Agricultural Sciences (JIRCAS), JapanReviewed by:
Anna Reale, Institute of Food Sciences, National Research Council (CNR), ItalyWei Li, Nanjing Agricultural University, China
Mao Li, Tropical Crops Genetic Resources Institute, Chinese Academy of Tropical Agricultural Sciences, China
Copyright © 2021 You, Du, Ge, Wan and Jia. This is an open-access article distributed under the terms of the Creative Commons Attribution License (CC BY). The use, distribution or reproduction in other forums is permitted, provided the original author(s) and the copyright owner(s) are credited and that the original publication in this journal is cited, in accordance with accepted academic practice. No use, distribution or reproduction is permitted which does not comply with these terms.
*Correspondence: Gentu Ge, Z2VnZW50dUAxNjMuY29t; Tao Wan, d2FudGFvNDI1QHNvaHUuY29t; Yushan Jia, anlzX25tQHNpbmEuY29t
†These authors have contributed equally to this work and share first authorship