- 1Departamento de Microbiología, Facultad de Ciencias, Ceimar-Universidad de Málaga, Málaga, Spain
- 2Unidad de Bioinformática – SCBI, Universidad de Málaga, Málaga, Spain
- 3IFAPA Centro El Toruño, El Puerto de Santa María, Spain
- 4Departamento de Biología y Geología, Ceimar-Universidad de Almería, Almería, Spain
The inclusion of macroalgae in the diets of farmed fish offers the opportunity for an added-value dietary ingredient to the nutraceutical feed. The composition of algae varies greatly among species. Several Ulva species have been considered in aquafeed formulations for different farmed fish, and Ulva ohnoi is being applied recently. However, the effects of seaweed dietary inclusion on the host must be evaluated. Considering the important role of the host intestinal microbiota, the potential effects of U. ohnoi dietary inclusion need to be studied. In this study, the characterization of the intestinal microbiome of Solea senegalensis, a flatfish with high potential for aquaculture in South Europe, receiving U. ohnoi (5%)-supplemented diet for 90 days has been carried out. In addition, the functional profiles of bacterial communities have been determined by using PICRUSt, a computational approach to predict the functional composition of a metagenome by using marker gene data and a database of reference genomes. The results show that long-term dietary administration of U. ohnoi (5%)-supplemented feed modulates S. senegalensis intestinal microbiota, especially in the posterior intestinal section. Increased relative abundance of Vibrio jointly with decreased Stenotrophomonas genus has been detected in fish receiving Ulva diet compared to control-fed fish. The influence of the diet on the intestinal functionality of S. senegalensis has been studied for the first time. Changes in bacterial composition were accompanied by differences in predicted microbiota functionality. Increased abundance of predicted genes involved in xenobiotic biodegradation and metabolism were observed in the microbiota when U. ohnoi diet was used. On the contrary, predicted percentages of genes associated to penicillin and cephalosporin biosynthesis as well as beta-lactam resistance were reduced after feeding with Ulva diet.
Introduction
Macroalgae have been widely evaluated as a novel ingredient for aquafeed formulation in recent years (Guerreiro et al., 2019; Wang et al., 2019; Yeganeh and Adel, 2019; Naylor et al., 2021). Their nutritional composition include protein, lipids, and high levels of biologically active compounds such as polysaccharides, pigments, polyphenols, and vitamins that might exert beneficial effects on farmed fish (Yaakob et al., 2014; Wells et al., 2017; Moutinho et al., 2018). Firstly, Mustafa and Nakagawa (1995) summarized the role of macroalgae in fish nutrition and then numerous studies have evaluated their potential not only as dietary protein source, but also as functional ingredients in practical diets for a variety of fish species (Yildirim et al., 2009; Güroy et al., 2012; Peixoto et al., 2016, 2017; Zhu et al., 2016). However, the effects detected were dose-dependent and species-specific (Valente et al., 2006).
In this context, Ulva species are a good source of protein, minerals, and vitamins, especially vitamin C (Ortiz et al., 2006; García-Casal et al., 2007), and different studies aimed at assessing the dietary inclusion of Ulva in a wide range of farmed fish species have been carried out (Wassef et al., 2013; Silva et al., 2015; Valente et al., 2016; Vizcaíno et al., 2016; Magnoni et al., 2017; Kazemi et al., 2018) with positive results on growth performance, innate immune response, feed utilization and overall fish health status. Negative effects on growth have been reported with dietary levels of Ulva meal above 10% in aquafeed (Wassef et al., 2005; Diler et al., 2007; Azaza et al., 2008). On the contrary, when added to fish diet at low percentages, benefits such as improved growth, feed efficiency, nutrient utilization, modulation of immune response, and disease resistance have been described in several fish species (Mustafa and Nakagawa, 1995; Wassef et al., 2005; Valente et al., 2006; Ergün et al., 2009; Moutinho et al., 2018; Fumanal et al., 2020).
Although different Ulva species have been applied in several areas, the interest on the potential industrial use of Ulva ohnoi (Prabhu et al., 2019) and its applicability or its products in aquaculture is recent (Norambuena et al., 2015; Fernández-Díaz et al., 2017; Martínez-Antequera et al., 2021). U. ohnoi is widely distributed and easily grown, with fast growth rates even in recirculating fish farming systems (Oca et al., 2019; Kang et al., 2021). Several studies have described effects on the immune system after dietary inclusion of U. ohnoi (Fumanal et al., 2020; Kang et al., 2021; Martínez-Antequera et al., 2021). In addition, presence of nutraceutical components such as the polysaccharide ulvan has been identified in U. ohnoi extracts (Fernández-Díaz et al., 2017). Thus, this species was considered in the present study based on the possibility to easily obtain enough amounts of algal biomass and its potential use as source of nutraceutical components for Senegalese sole (Solea senegalensis).
Senegalese sole is a flatfish with great potential for marine aquaculture due to its high market value and consumer demand. Recent management and technical improvements in S. senegalensis culture are leading to important progress in productivity, but there are still unsolved questions regarding nutrition aspects under cultured conditions (Morais et al., 2016), and at present, inclusion of macroalgae in aquafeeds is being considered.
The gastrointestinal (GI) microbiota has a relevant role in animal nutrition, development, modulation of the immune system, and resistance against pathogens (Rawls et al., 2004; Salinas et al., 2006; Semova et al., 2012; Ingerslev et al., 2014a,b; Llewellyn et al., 2014; Wang et al., 2018) and its composition is shaped by different factors such as host species, trophic level, environment, and feeding habits (Romero et al., 2014; Eichmiller et al., 2016; Gonçalves and Gallardo-Escárate, 2017) variations in physiological parameters along the GI (Ye et al., 2014) and functional ingredients or nutraceuticals (Ringø et al., 2016). Composition of Ulva spp., as well as U. ohnoi, includes polysaccharides such as ulvan (Lahaye and Robic, 2007) and starch (Korzen et al., 2015; Farias et al., 2017). The hydrolysis of those compounds by bacteria has been documented (Michel and Czjzek, 2013; Reisky et al., 2019), so their metabolism by the intestinal microbiota of farmed fish deserve attention. In this context, recently, the administration during a short period of time of a low dietary level of U. ohnoi demonstrated to exert a modulation of the intestinal microbiota of farmed Senegalese sole specimens (Tapia-Paniagua et al., 2019). As far as we know, effects of long-term feeding of U. ohnoi on the GI microbiota and the potential implications on the functionality are not known. In order to achieve a better understanding of the role of gastrointestinal microbiota in fish health and digestion, further knowledge of the phylogenetic profile and functional capacities of the intestinal microbiota is necessary.
Furthermore, despite the fact that the microbial diversity of Senegalese sole has been described in a recent study (Tapia-Paniagua et al., 2019), there is no solid information about the functional capability of these microbial communities so far. In this sense, some bioinformatic tools can predict microbiome functionality, based on marker genes commonly used for diversity analysis, such as the 16S rRNA. In this work, the predictive software PICRUSt has been used for describing the effects of U. ohnoi 5% dietary supplementation on the main functional traits of S. senegalensis gut microbiome.
The aim of the present study was to investigate the effects of long-term feeding low dietary inclusion of U. ohnoi on the intestinal microbial composition of S. senegalensis. In addition, functional profiles of bacterial communities were evaluated by using phylogenetic investigation of communities by reconstruction of unobserved states (PICRUSt) (Langille et al., 2013), and the potential relationship between changes in microbial composition and functionality after U. ohnoi feeding was assessed.
Materials and Methods
Diet Composition and Preparation
U. ohnoi Hiraoka and Shimada, strain UOHN120810 was isolated from the outlet channel of IFAPA El Toruño (El Puerto de Santa María, Cádiz, Spain) fish facilities and vegetative clones maintained in culture. To obtain the U. ohnoi biomass needed for the feeding trial (1 kg m–3), U. ohnoi cultures were up-scaled to 1000-L tanks and grown in modified f/2 medium (Guillard, 1975) with 1.8 mM nitrate and 0.1 mM phosphate prepared with filtered (0.2 μm) natural seawater for 2 weeks under natural photoperiod light. Algae were harvested, rinsed with tap water, freeze-dried, and kept in a dry place until used as ingredient in the experimental diet.
Two isonitrogenous (55% on dry weight basis) and isolipidic (15% on dry weight) experimental diets were manufactured by LifeBioencapsulation SL (Spin-off, Universidad de Almeria, Spain). Ulva diet was formulated to include 5% (w/w) dry U. ohnoi biomass. An algae-free diet was used as control. The ingredient composition of experimental diets is shown in Table 1.
Feed ingredients were finely ground and mixed in a vertical helix ribbon mixer (Sammic BM-10, 10-L capacity, Sammic, Azpeitia, Spain) before fish oil and diluted choline chloride being added. All the ingredients were mixed together for 15 min, and, after, water (300 ml kg–1) was added to the mixture to obtain homogeneous dough. The dough was passed through a single screw laboratory extruder (Miltenz 51SP, JSConwell Ltd., New Zealand), to form 1–2 mm (diameter) and 2–3 mm (length) pellets. The extruder barrel consisted of four sections and the temperature profile in each section (from inlet to outlet) was 100, 95, 90, and 85°C, respectively. Finally, pellets were dried at room temperature for 24 h and kept in sealed plastic bags at −20°C until use.
Fish Maintenance
Juvenile Senegalese sole (S. senegalensis) (10.7 ± 2.9 g, mean initial body weight) were obtained from a commercial hatchery (Cupimar S.A., San Fernando, Cádiz, Spain) and transported to the research facilities of IFAPA El Toruño (El Puerto de Santa María, Cádiz, Spain). Fish were stocked at 1.5 kg m–2 in 6 tanks connected to a closed recirculation consisting of a mechanical filter, a skimmer, ultraviolet light, and a biofilter. Fish were fed daily at 2% fish biomass with an experimental diet considered as control diet for 10 days for acclimatizing the fish to the experimental conditions. After the acclimation period, experimental diets were randomly assigned to triplicate groups. Fish (12.3 ± 2.0 g mean body weight) of each set of three tanks were fed with two different experimental diets: control diet and diet containing U. ohnoi 5% (Ulva diet) for 90 days at a rate of 3% of their body weight. Different parameters were monitored during all the experimental period. The temperature, pH, salinity, and oxygen were maintained constant at 19.9 ± 0.7°C; 7.8 ± 0.2; 25.7 ± 1.5‰, and 7.0 ± 0.4 mg L–1, respectively. Nitrite and ammonia were checked once a week (values were below 0.1 mg L–1).
At the end of the feeding trial, fish were fasted for 12 h before sampling. Soles (n = 14) were carefully taken from their respective tanks and transferred to a new tank containing clove oil (200 ppm) to euthanize. Whole intestines of seven fish per treatment were aseptically removed, divided into two equal length sections (anterior and posterior), and stored separately in Trisure, −80°C, until further analysis.
Sampling Procedures and Sequence Analysis
Individual intestinal samples were collected from S. senegalensis specimens with 1 ml of PBS, pH 7.2, and 1 ml aliquot per sample (n = 28) was centrifuged (1,000 × g, 5 min). Total DNA was extracted from each sample according to manufacturer specifications using Trisure (Bioline, Spain). Afterward, 20 μl of total DNA was precipitated with 2 μl of sodium acetate 3 M and 46 μl isopropanol for purification. Then, DNA was centrifuged for 3 min at 12,000 × g, 4°C, supernatants were discarded, and the pellets were rinsed with cold 70% ethanol. After centrifugation for 5–15 min at 12,000 × g, 4°C, supernatants were discarded again and pellets were air dried. Finally, DNA was resuspended in water. DNA quality and integrity were visualized by gel electrophoresis. Concentration and purity were determined by using Qubit 2.0 fluorimeter (Thermo Scientific, Germany). Isolated DNA was stored at −20°C until further processing and 30 ng was used for subsequent analyses.
Libraries were constructed by the Ultrasequencing Service of the Bioinnovation Center (University of Malaga, Spain) using the Illumina® MiSeq Platform. Libraries were constructed by using the Illumina MiSeq Platform. Briefly, Illumina paired-end sequencing (2 × 300 bp) of each sample was carried out by using the primers 341F CCTACGGGNGGCWGCAG and 805R GACTACHVGGGTATCTAATCC, targeting V3–V4 regions of 16 S rRNA gene (Klindworth et al., 2013).
Illumina reads were analyzed with FastaQC software (Andrews et al., 2010) to assess sequence quality. Then, reads were processed using a pipeline based on the software package MOTHUR (version 1.39.5) (Schloss et al., 2009). Demultiplexed paired-end reads were merged and processed for primer sequence trimming according to amplicon size (400-600 bp). UCHIME version 4.2. (Effective Tags obtained)1 (Edgar et al., 2011) was used to detect and remove chimera; the remaining representative, non-chimeric sequences were aligned and clustered into operational taxonomic units (OTUs) in the Greengenes database (version 13.5) (McDonald et al., 2012) with 97% identity cutoff and the total count threshold was set at 0.005% (Bokulich et al., 2013).
After generating the taxonomic profile of microbiome samples, comparison of taxa present in the samples was carried out. The samples were normalized using the calculation of the rarefaction curves. All statistical analyses were performed using phyloseq and vegan libraries in R package (McMurdie and Holmes, 2013). To determine the level of sequencing depth, rarefaction curves were obtained by plotting the number of observed OTUs against the number of sequences and Good’s coverage coefficient was calculated. Alpha diversity was estimated based on Chao1, Shannon-Wiener, and Simpson indexes to determine taxonomic and phylogenetic structure diversity, respectively. The results are generally presented at phylum, class, family, and genus taxonomic levels.
Putative microbiota functions were predicted using PICRUSt (version 1.1.3), a tool designed to infer metagenomic information from 16S rRNA amplicon sequencing data using default values (Langille et al., 2013). The metagenomic data resulting from the clustering with the Greengenes database (version 13.5), were entered into the software. The metagenome prediction of the bacterial communities was carried out using the data set calculated after normalizing the number of copies of rRNA 16S to the size of the biome. Nearest Sequenced Taxon Index (NSTI) scores were obtained to assess the precision of predicted metagenomes and ranked at a 97% confidence value using the Kyoto Genome and Gene Encyclopedia Pathway Database (KEGG). All the functional categories were calculated (Kanehisa et al., 2014) and the bacterial functional profiles were compared up to level 3 of the KEGG modules.
Calculations and Statistical Analysis
Growth parameters and survival rates were calculated according to the following expressions:
Weight gain rate (WGR) (%) = 100 × (final body weight – initial body weight)/initial body weight; Feed conversion ratio (FCR) = Dry feed consumed (g)/wet weight gain (g) and Survival rate (%) = 100 × final fish number/initial fish number.
Normality (Shapiro–Wilk test) and homogeneity of variance (Levene’s test) were tested for all data before differences between the two experimental diets were determined and Student’s t-test comparison of means was used. All tests were performed with XLSTAT software and significance was set for p < 0.05.
Multivariate analysis of OTU data was performed via Principal Coordinate Analysis (PCoA) of OTU profiles using Bray–Curtis metric to depict differences between microbiota of each diet group. In addition, to test the hypothesis of no differences between the microbiota of the GI sections and diets assayed, dissimilarity matrices obtained with the Bray–Curtis index were analyzed by Permutation multivariate analysis of variance (PERMANOVA) with 999 permutations by using PAST software (Hammer et al., 2001) version 3.16.
Linear discriminant analysis (LDA) effect size (LEfSe) (Segata et al., 2011) was used to characterize microbial differences of biological relevance between the diets within the two different GI sections and between sections within the same diet. LEfSe analysis was performed using the Galaxy platform2. The taxa whose alpha value was less than 0.05 were selected as significant, first by the Kruskal–Wallis factorial rank-sum test and then by the Wilcoxon paired test, as well as a threshold of |2.0| for the LDA.
STAMP software (Statistical Analysis of Metagenomics Profiles) was used to analyze the differential abundances of predictive functions based on the microbiota of intestinal sections and diets researched, using ANOVA multiple-comparison test with post hoc Tukey–Kramer test (p corrected < 0.05).
Results
Fish Growth and Microbiota Sequencing Overview
Fish mortality during the experimental period was below 5%. Significant decreased final body weight and weight gain rate values were obtained for fish fed with U. ohnoi 5%-supplemented diet; however, no differences in FCR were detected based on the diet supplied (see Table 2 for details).
DNA was extracted from 28 fish intestinal samples, seven S. senegalensis specimens per diet and 2 intestinal regions per fish specimen. In total, 5,109,193 raw reads were obtained for both forward and reverse directions after sequencing. Non-specific amplicons not assigned amplicons to the target taxon, and chimeras were removed in the initial quality filtering, and a total of 1,453,999 reads were obtained, with 51,928.53 ± 17,165.22 (mean ± SD) sequences per sample that clustered in a total of 667 OTUs (97% similarity cutoff against the Greengenes database). Sequences were filtered by rarefaction curves to the minimum library size of 30,284 reads (Supplementary Figure 1) and singletons and doubletons were also removed. A total of 190 OTUs were obtained and used for subsequent analysis. Mean Good’s coverage estimator value was 99.94 ± 0.02 (mean ± SD) (ranging from 99.93 to 99.94%), indicating adequate sequencing depth.
Alpha diversity indices were calculated for microbiota data of fish fed both control and Ulva diets in section anterior and posterior. Statistical differences were not observed for microbiota species richness (Chao1) when Ulva diet was administered. Furthermore, no differences in Shannon and Simpson indices were observed between the microbiota of fish specimens fed control or Ulva diet regardless of the GI sections analyzed (see Tables 3A,B for details).
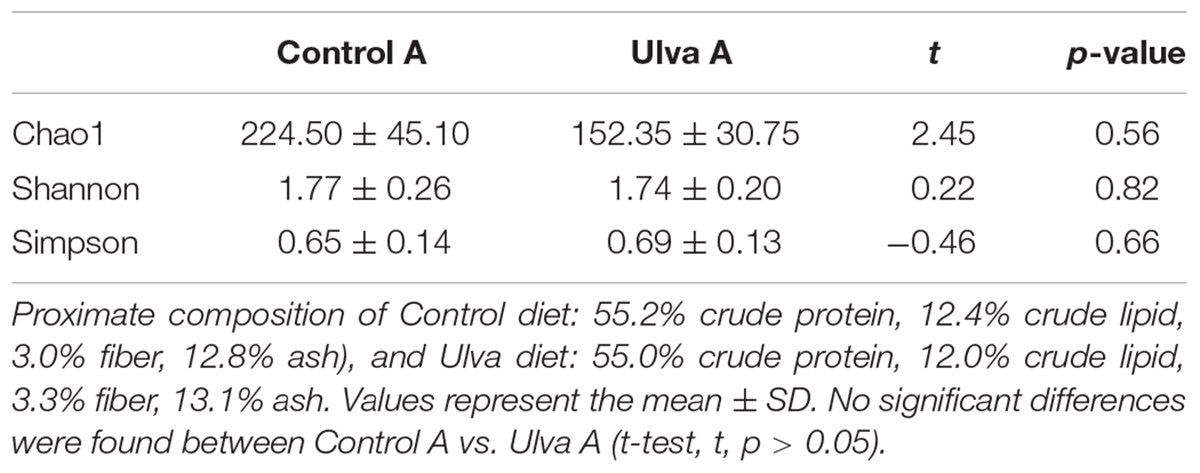
Table 3A. Alpha diversity of bacterial communities in anterior (A) intestinal tract sections of Solea senegalensis specimens.
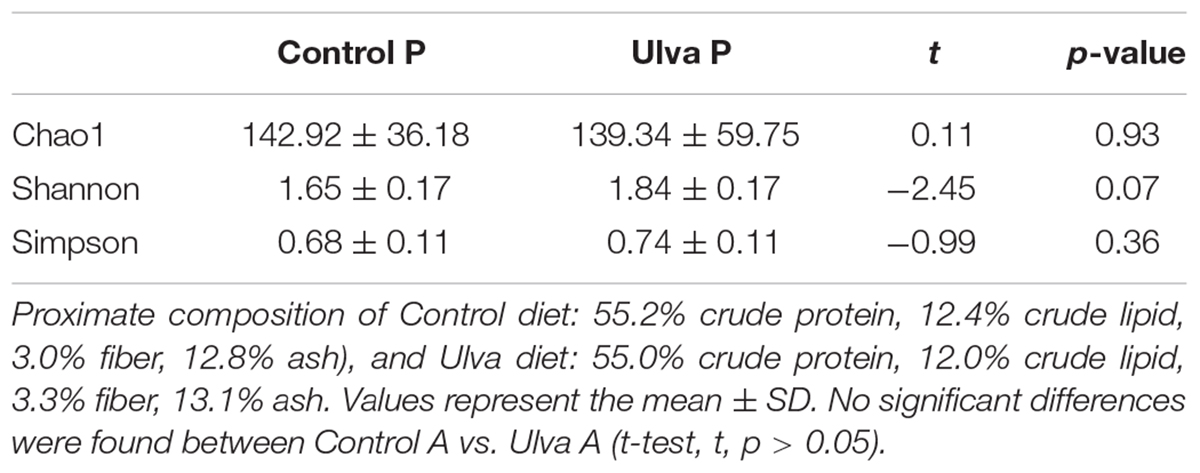
Table 3B. Alpha diversity of bacterial communities in posterior (P) intestinal tract sections of Solea senegalensis specimens.
Composition of Solea senegalensis Gastrointestinal Microbiota
Intestinal microbiota of S. senegalensis fed with control and Ulva-supplemented diets was dominated by Proteobacteria, Tenericutes, and Firmicutes in both GI sections (Figure 1). In all the cases, Proteobacteria was the most abundant phylum, ranging from 68.8 to 91.7% relative abundance. In addition, Spirochetes was also present, it being a predominant phylum in the posterior GI microbiota of fish fed with both diets (13.5–13.7%).
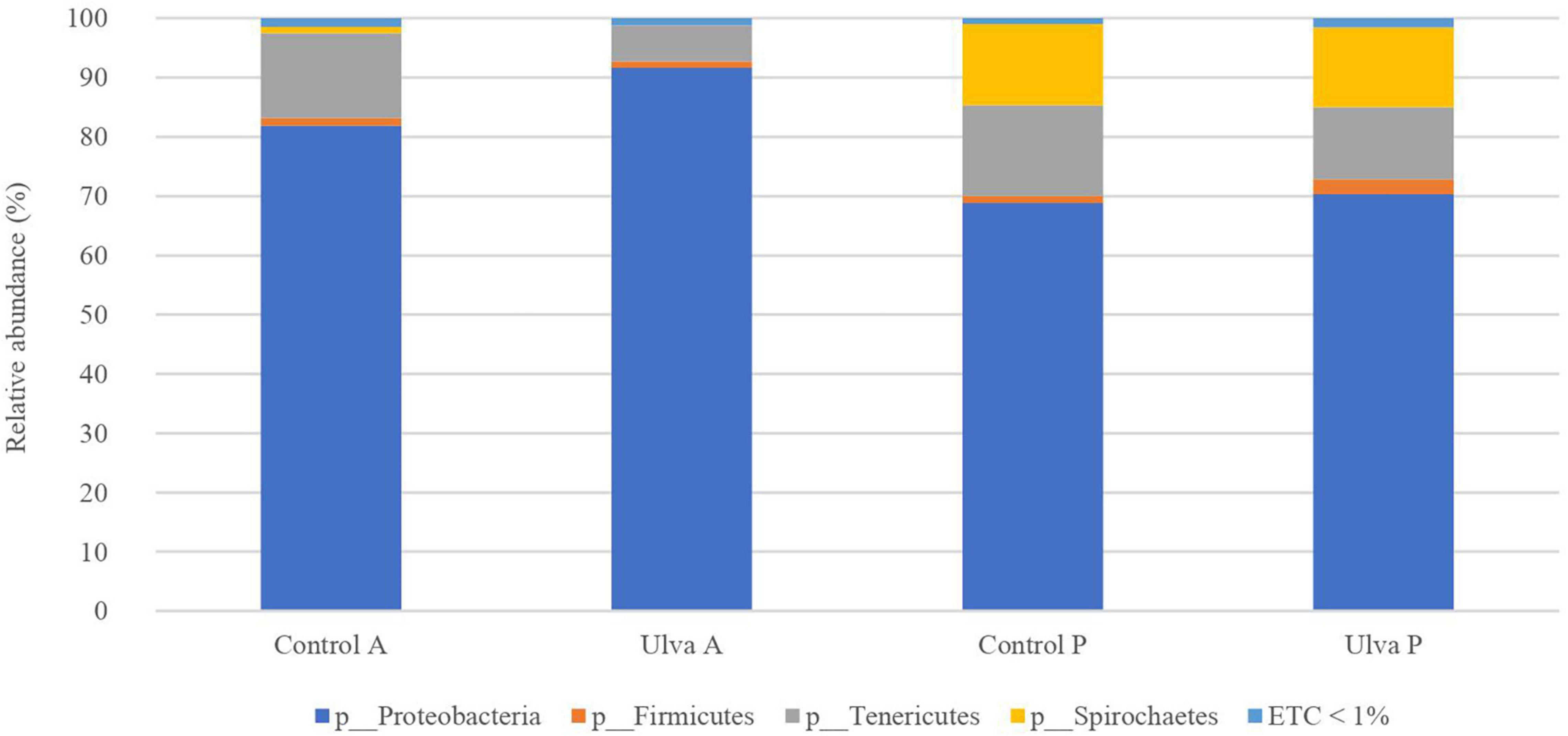
Figure 1. Average of the samples of gut microbiota (relative OTU composition) at phylum level of the gastrointestinal tract of S. senegalensis fed control (Control) and Ulva ohnoi supplemented (Ulva) diet for 90 days. A, anterior region; P, posterior region. ETC < 1% indicates relative abundance below 1%.
At the class level (Figure 2 and Supplementary Figure 2), Gammaproteobacteria was the most abundant class in both GI sections (ranging from 66.6 to 88.9%), followed by Mollicutes (from 6.0 to 15.3%). Interestingly, microbiota of S. senegalensis specimens fed with Ulva diet showed Mollicutes decreased relative abundance jointly with increased Gammaproteobacteria, it being more evident in anterior sections, although these changes were not reported as statistically significant (Wilcoxon test, U = 19, p = 0.15) (Figure 2).
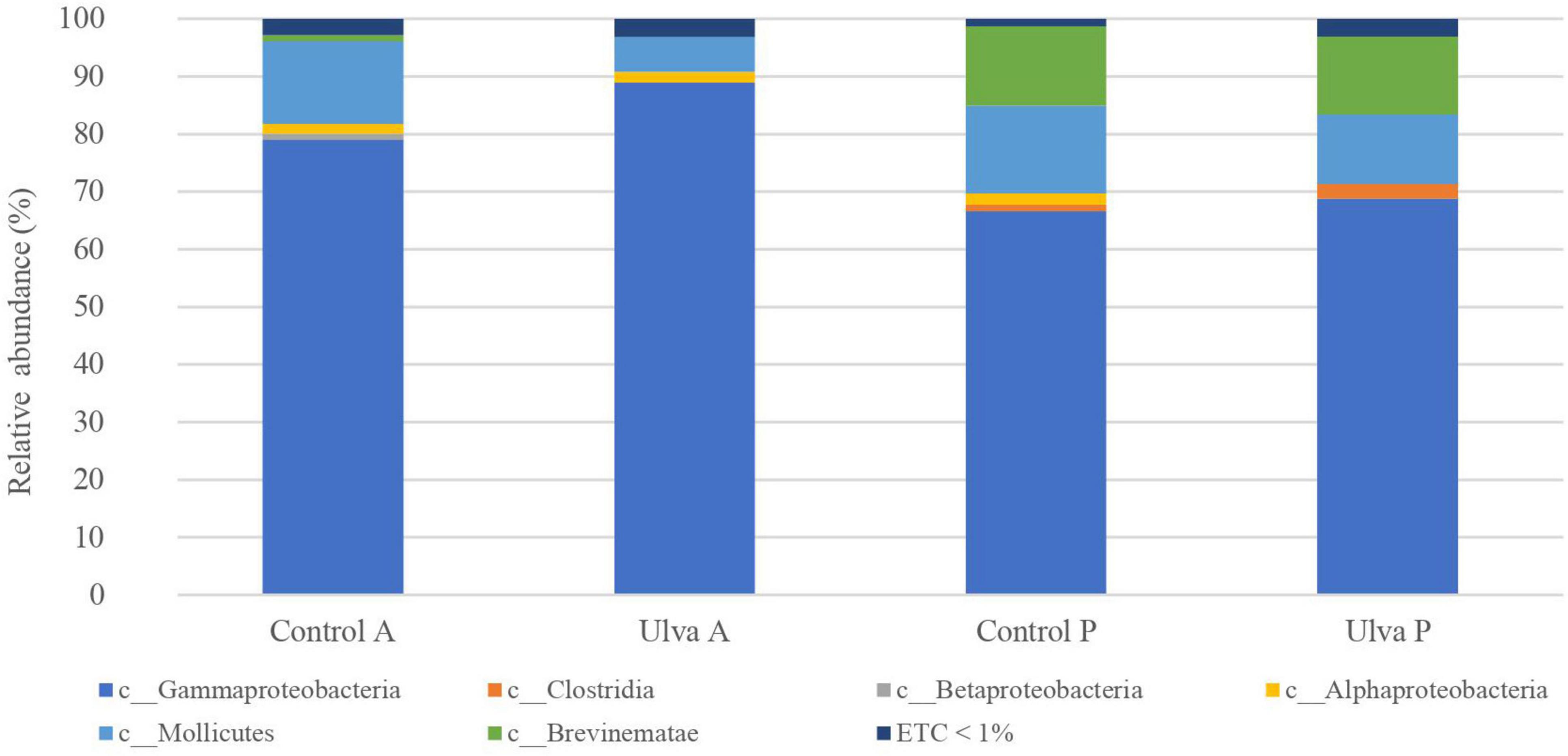
Figure 2. Average of the samples of gut microbiota (relative OTU composition) at class level of the gastrointestinal tract of S. senegalensis fed control (Control) and Ulva ohnoi supplemented (Ulva) diet for 90 days. A, anterior section; P, posterior section. ETC < 1% indicates relative abundance below 1%.
The taxonomic analysis at the family level showed higher relative abundance of Pseudomonadaceae members, it being predominant in both intestinal sections when S. senegalensis specimens were fed with control diet (Figure 3 and Supplementary Figure 3). However, abundance of Vibrionaceae increased in both intestinal sections of fish fed with Ulva diet compared to those fed with control diet. Mycoplasmataceae, Xanthomonadaceae, and Brevinemataceae were detected in lower abundance percentages in all the samples regardless of the section and diet considered, though relative abundance of the two first was higher in fish receiving control diet. Finally, the study at the genus level (Figure 4 and Supplementary Figure 4) showed Vibrio as the most abundant member in the microbiota of specimens fed with Ulva diet, while OTUs identified as “Pseudomonadaceae_unclassified” family were the most frequent in the GI microbiota when control diet was supplied, regardless of the section analyzed.
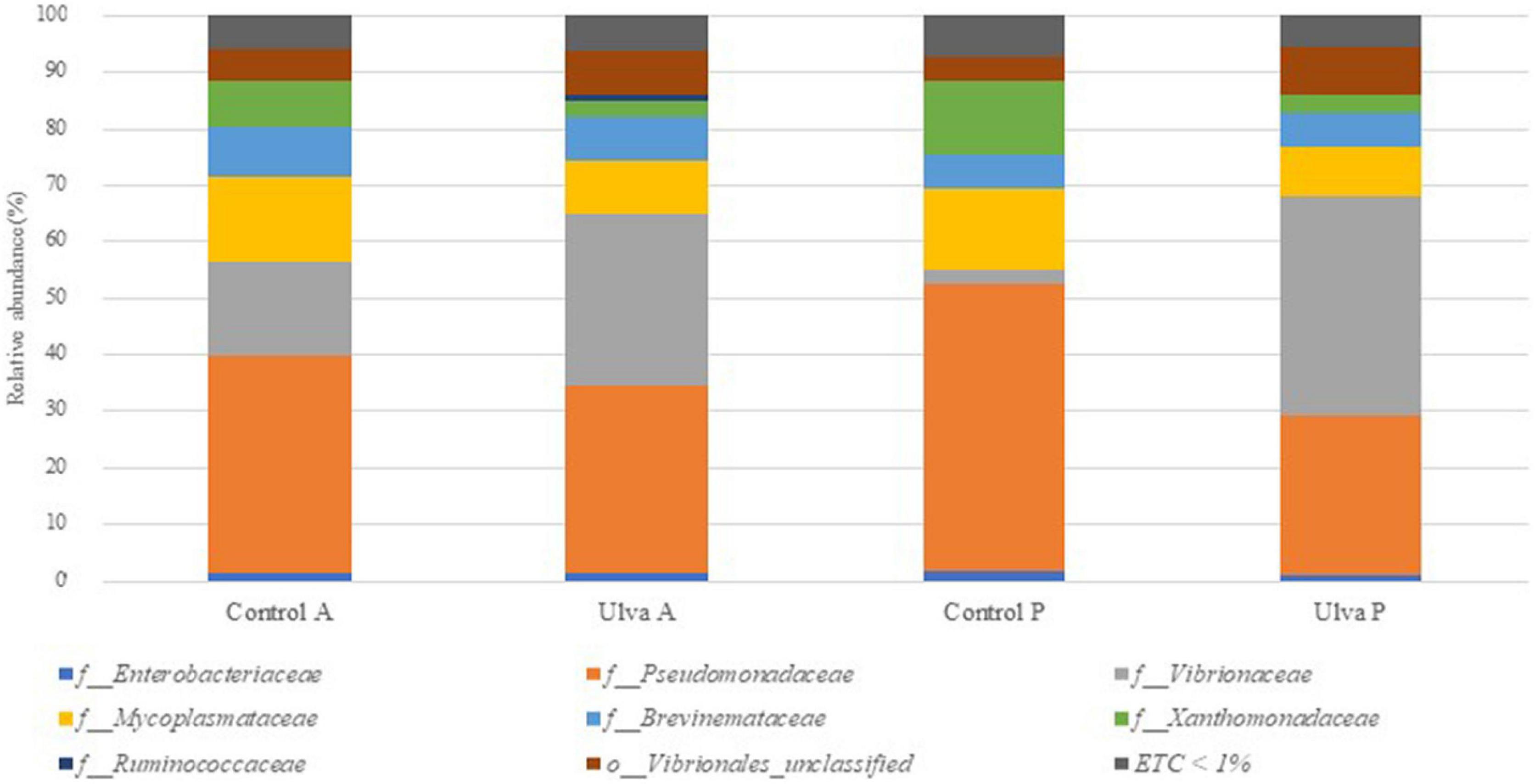
Figure 3. Average of the samples of gut microbiota (relative OTU composition) at family level of the gastrointestinal tract of S. senegalensis fed control (Control) and Ulva ohnoi supplemented (Ulva) diets for 90 days. A, anterior section; P, posterior section. ETC < 1% indicates relative abundance below 1%.
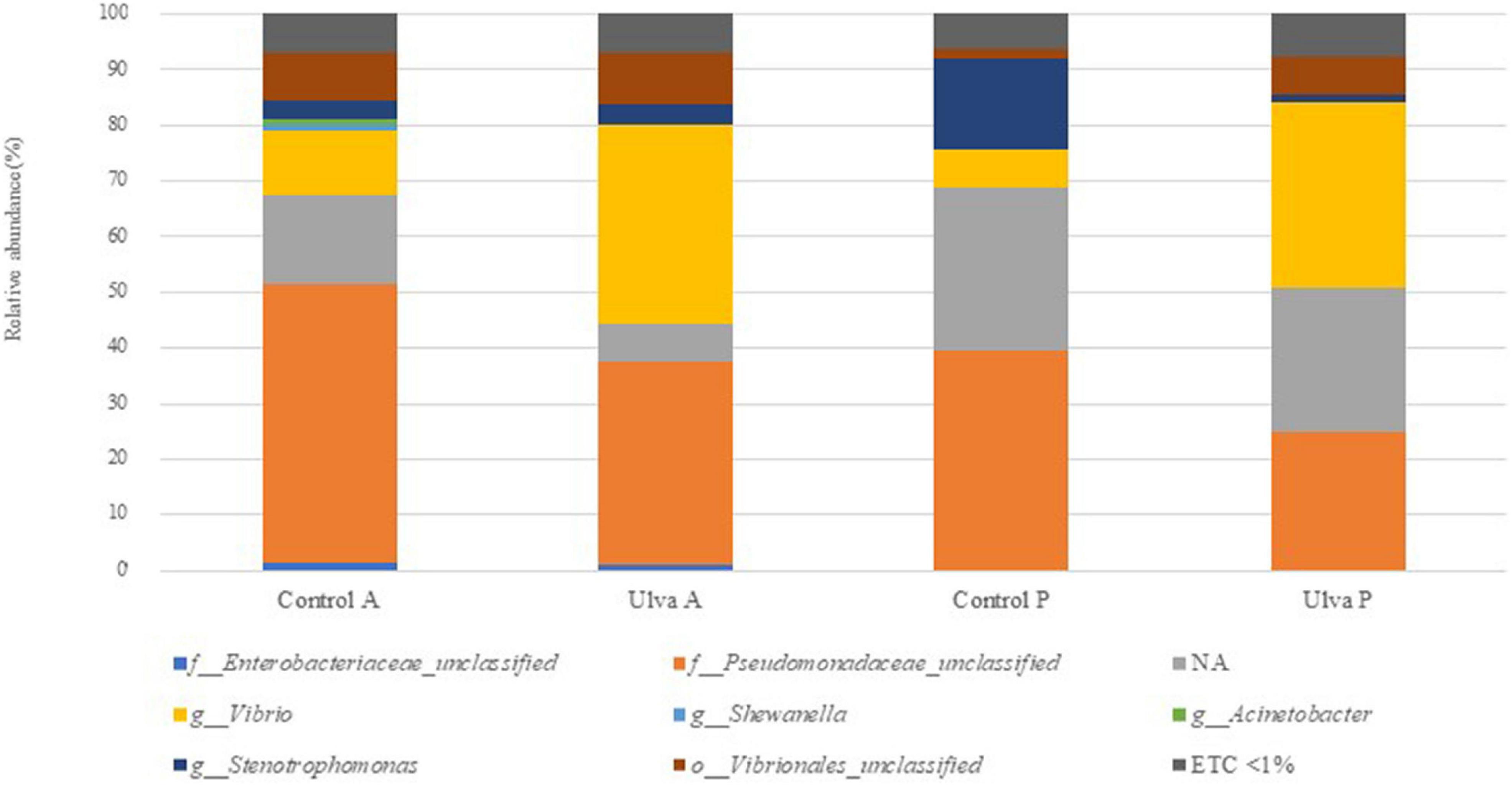
Figure 4. Average of the samples of gut microbiota (relative OTU composition) at genus level of the gastrointestinal tract of S. senegalensis fed control (Control) and Ulva ohnoi supplemented (Ulva) diet for 90 days. A, anterior section; P, posterior section. NA: OTUs not clustered with the database at the genus level. ETC < 1% indicates relative abundance below 1%.
Modulation of Intestinal Microbiota by Ulva ohnoi Diet
Beta diversity analysis was performed to explore differences in bacterial communities based on the diet received by the fish. Graphical representation of principal coordinate analysis (PCoA) based on Bray–Curtis distances among OTUs detected showed no separate clustering of anterior GI samples associated to the diet (Figure 5A). On the contrary, microbiota samples from posterior GI sections were clearly separated according to the diet received by S. senegalensis specimens (Figure 5B). Furthermore, PERMANOVA test confirmed the shift in posterior GI microbiota composition based on the diet, while no significant values were obtained for anterior GI sections used (PERMANOVA, F = 3.40, p = 0.005, and p = 0.49, respectively).
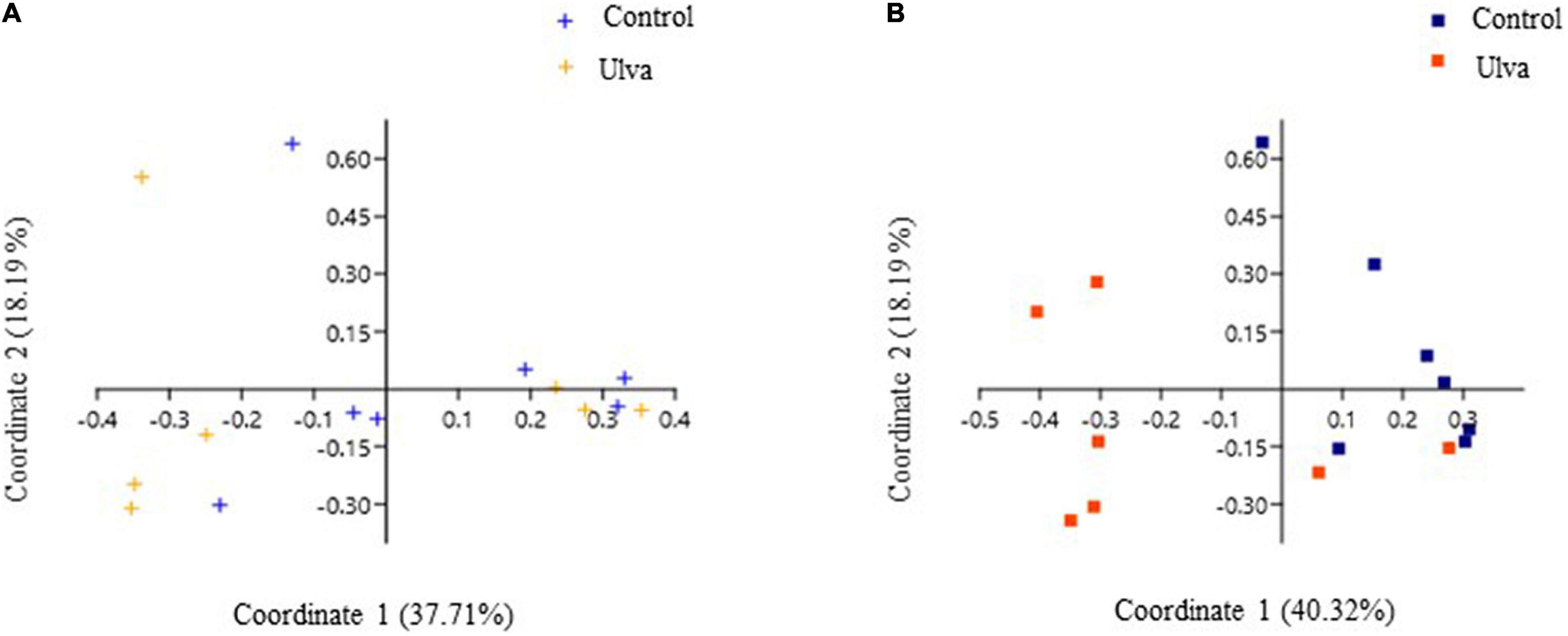
Figure 5. Principal coordinate analysis (PCoA) of bacterial community composition at OTU level based on Bray–Curtis distance matrix. The individual samples are color-coordinated according to the diet received by S. senegalensis specimens: control (Control) and Ulva ohnoi supplemented (Ulva) diets for 90 days. (A) Anterior GI sections, (B) Posterior GI sections.
In order to determine the OTUs most likely to explain differences between experimental diets and GI sections, LEfSe analysis (Kruskal–Wallis and Wilcoxon test p < 0.05 and LDA effect size > | 2.00|) was carried out. Histograms of the LDA scores computing for differentially abundant OTUs in the GI microbiota of S. senegalensis specimens fed with control and Ulva diets and anterior and posterior GI sections are shown in Figure 6.
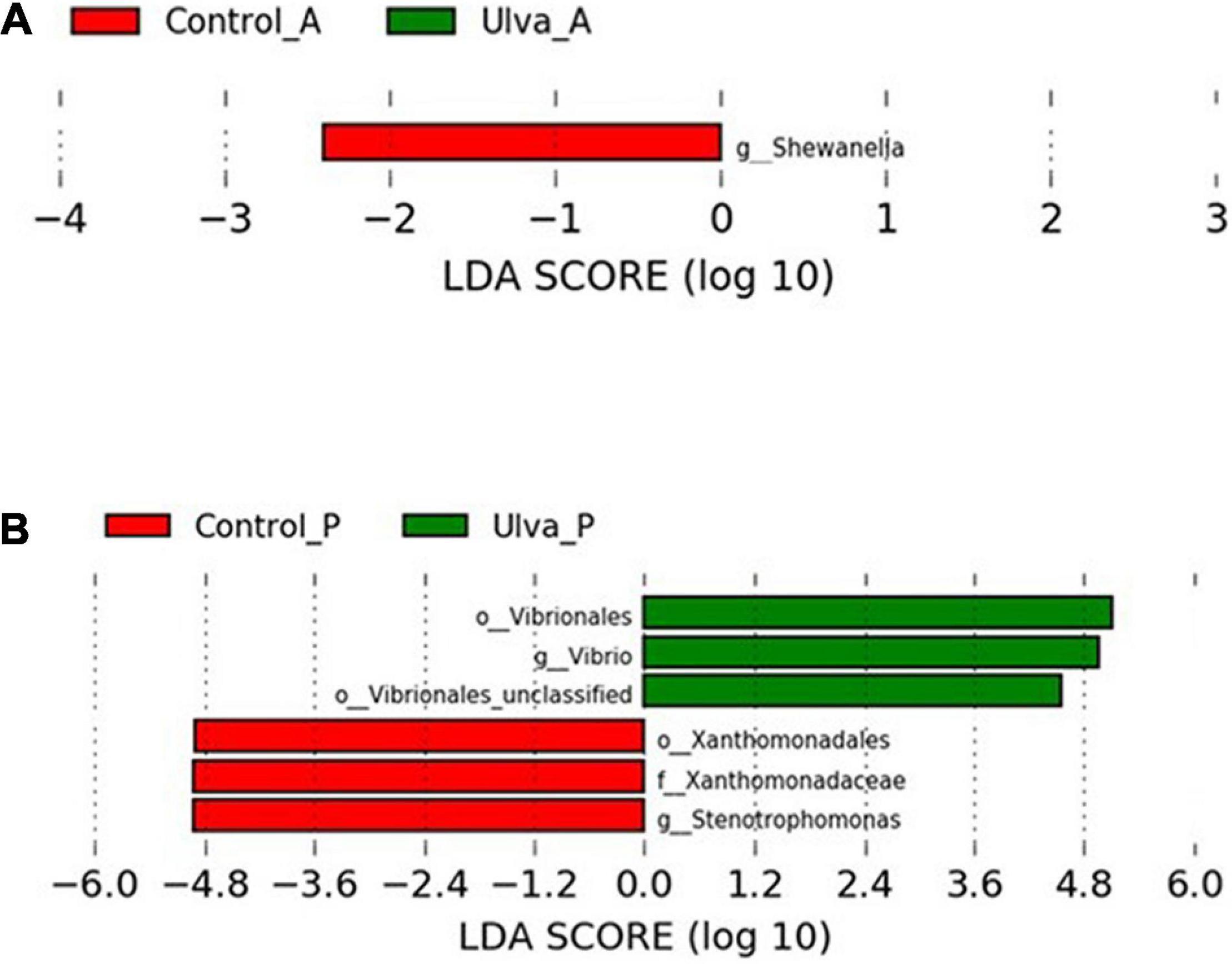
Figure 6. LDA scores for OTUs differentially abundant in the microbiota GI tract of S. senegalensis specimens fed control (Control) and Ulva ohnoi supplemented (Ulva) diets for 90 days (Kruskal–Wallis and Wilcoxon test, p < 0.05 and LDA effect size > | 2.00|). (A) anterior section; (B) posterior section.
When comparing the microbiota of anterior sections of fish fed with control and Ulva diets, only Shewanella genus were detected as significative (Wilcoxon test, U = 7, p = 0.02) (Figure 6A). On the contrary, microbiota of the posterior GI sections of fish fed control diet showed significantly increased abundance in Stenotrophomonas genus (Xanthomonadaceae family, Xanthomonadales order) whereas Vibrio genus (previously named taxa, Wilcoxon test, U = 0, p = 0.001), including Vibrionales order (Wilcoxon test, U = 2, p = 0.003), was differentially more abundant in the microbiota of fish fed with the algae (Figure 6B).
Predicted Gastrointestinal Microbiota Function
PICRUSt analysis was performed to predict functional capabilities of intestinal microbial communities detected in S. senegalensis specimens receiving the two diets assayed. The low NSTI value (0.068 ± 0.068) obtained in the PICRUSt analysis indicated good accuracy of prediction. A total of 328 KEGG functions corresponding to level 3 KO entries was identified.
Significant differences in the functional composition of microbial communities of fish fed with both diets were only detected in posterior GI sections. Thus, microbiota of fish fed with U. ohnoi-supplemented diet showed increased percentages of genes involved in cellular processes and signaling (electron transfer carriers), energy metabolism (methane metabolism), and xenobiotic biodegradation and metabolism (dioxin, xylene, and nitrotoluene degradation) (see Figure 7 for details). On the contrary, decreased abundance of genes involved in biosynthesis of other secondary metabolites (penicillin and cephalosporin biosynthesis), lipid metabolism (arachidonic acid metabolism), metabolism of other amino acids (beta-lactam resistance), replication and repairs (chromosome and non-homologous end-joining), and transcription (transcription machinery) was predicted for posterior GI microbiota of fish fed with Ulva diet compared to those receiving control diet (see Figure 7 for details).
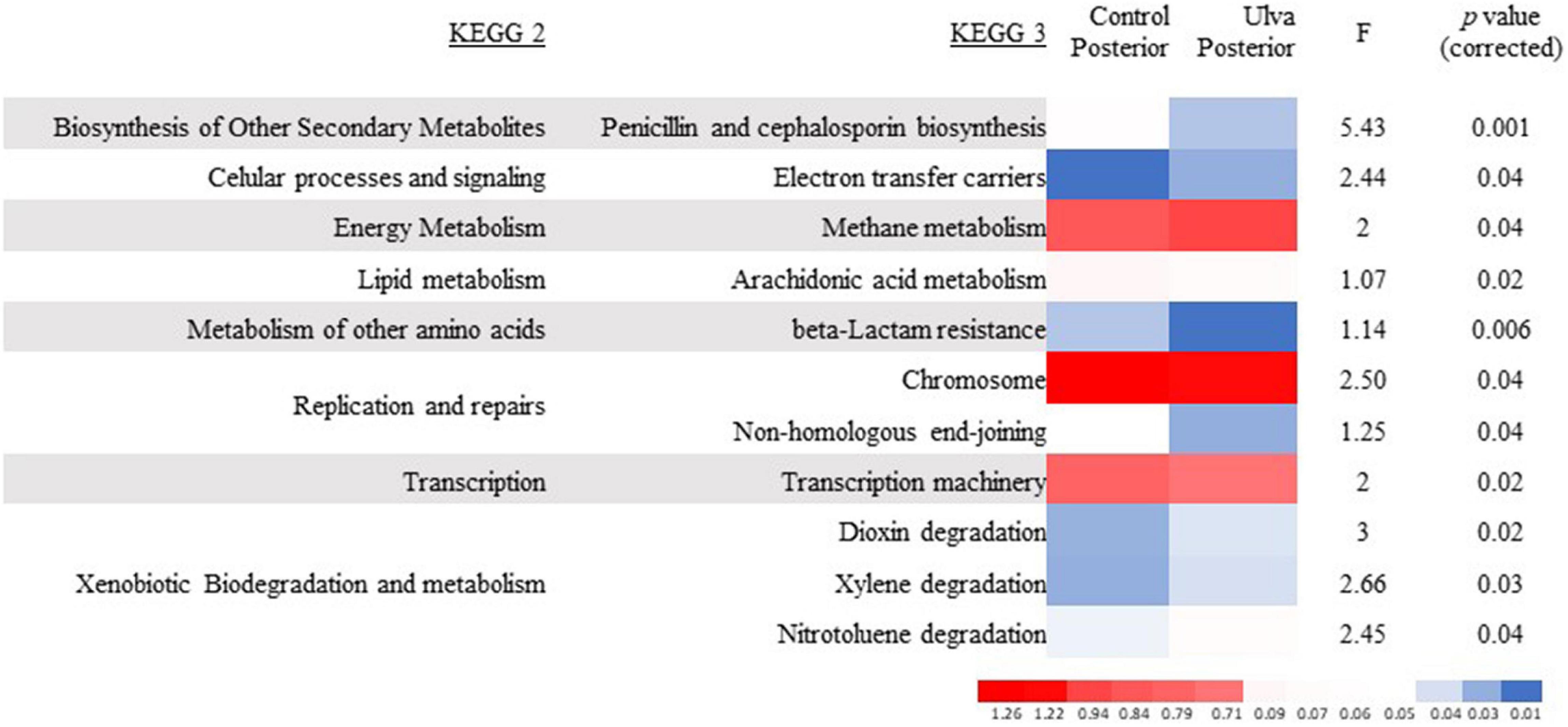
Figure 7. Heatmap showing significant differences (ANOVA, F, p < 0.05) in microbial functionality (PICRUSt) between the posterior intestinal microbiota of S. senegalensis fed control (Control) and U. ohnoi supplemented (Ulva) diet for 90 days. Relative abundance of sequences associated with KEGG functions of the samples.
Discussion
Marine macroalgae represent a promising source of sustainable protein and substances with nutraceutical values, with effects on fish physiology and immune system (Cerezuela et al., 2012; Packer et al., 2016; Fumanal et al., 2020; Ponce et al., 2020; Araújo et al., 2021).
In the present work, decreased growth and feed conversion rates observed in S. senegalensis receiving Ulva diet are in agreement with results obtained in previous studies carried out in farmed fish, such as rainbow trout (Yildirim et al., 2009) and S. senegalensis (Tapia-Paniagua et al., 2019). The reduced growth observed in fish fed the Ulva-supplemented diet has been attributed to the presence of antinutritive factors such as protease inhibitors (Sáez et al., 2013; Vizcaíno et al., 2016, 2019), which could have inhibited digestive proteases in the intestine and adversely affected nutrient uptake.
Microbial ecosystem function and stability are influenced by species and functional group richness (Bell et al., 2005), which, along with the biodiversity, are essential in protecting ecosystem functionality against perturbations (De et al., 2014; Gonçalves and Gallardo-Escárate, 2017). Different studies indicate that diet composition and changes are important factors in the modulation of the GI microbial communities in vertebrates (Askarian et al., 2012; Sullam et al., 2012; Ghanbari et al., 2015; Ringø et al., 2016; Gonçalves and Gallardo-Escárate, 2017). Our results show that the administration of U. ohnoi 5%-supplemented diet to S. senegalensis did not induce significant differences in the GI microbiota alpha-diversity indices. These results contrast with significantly increased Shannon diversity index values reported when a low U. ohnoi inclusion diet was administered to the same fish species for a shorter period (45 days) (Tapia-Paniagua et al., 2019). Shannon index reflects the diversity of the whole microbial community and differences based on the supplemented diet feeding period could be attributed to an adaptation period to the new diet in the microbial communities, it being possible to consider that the microbiota adjustment has been accomplished after 90 days. Thus, similar diversity index may be due to similar number of species and average or evenness of individual distribution among species, though species may be different. In this context, it has been reported that fish age had significant effects on the beta but not alpha diversity of gut microbiota in both freshwater and saltwater habitats (Zhao et al., 2020).
Overall composition detected in S. senegalensis GI microbiota in this study corroborate that members of Proteobacteria phylum dominate the microbiota of the GI tract in S. senegalensis specimens, whereas Tenericutes represents the second most abundant phylum in this environment (Tapia-Paniagua et al., 2019). Similarly, previous studies at class level have also identified γ-Proteobacteria and Mollicutes in S. senegalensis GI tract (Tapia-Paniagua et al., 2014, 2019). The taxonomic analysis at family level demonstrated that Pseudomonadaceae and Vibrionaceae were the most frequently Proteobacteria detected in the present work, like in the previous study carried out with younger Senegalese sole specimens by Tapia-Paniagua et al. (2019). These results demonstrate the presence of a long-term core microbiota in S. senegalensis GI tract.
Diet is considered one of the more relevant factors affecting intestinal microbiota (Webster et al., 2020). Though low-level dietary inclusion of U. ohnoi did not influence alpha diversity indices of S. senegalensis intestinal microbiota, results obtained indicate that changes in composition of microbial communities occur in posterior intestinal sections after long-term feeding with diet supplemented 5% of this macroalga. Thus, a shift in the presence of Stenotrophomonas (Xanthomonadaceae) members in favor of Vibrio genus was observed in the microbiota of fish fed with the macroalgae-supplemented diet.
Stenotrophomonas genus has been reported as a predominant member of the intestinal microbiota of farmed fish (Tapia-Paniagua et al., 2011; He et al., 2016; Puello et al., 2018), including S. senegalensis larvae, juvenile specimens (Tapia-Paniagua et al., 2014, 2019), and S. senegalensis specimens fed with U. ohnoi (Tapia-Paniagua et al., 2019). In addition, this genus has been identified as one of the main groups comprising microbiota of marine species such as rorquals (Toro et al., 2021). This genus includes species reported as fish opportunistic pathogens such as S. maltophilia (Geng et al., 2010; Abraham et al., 2016), while other strains isolated from microbiota of marine invertebrates (Reina et al., 2019) and fish (Torabi Delshad et al., 2018) have demonstrated the ability to degrade a broad range of N-acyl-homoserine signaling molecules essential for quorum sensing. This signaling system is involved in the control of the expression of genes related to virulence factors, and quenching signal molecules have been reported to result in attenuation of pathogen virulence (Reina et al., 2019). In this context, Stenotrophomonas strains have been reported as plant-probiotics showing abilities to degrade and reduce toxic compounds (Mukherjee and Roy, 2016; Zhang et al., 2017) and produce plant growth-promoting factors (Nevita et al., 2018). However, it would be interesting to check similar activities in strains of this genus isolated from fish. Since the libraries of our study were constructed using the V3V4 amplicon reconstruction with a size of 460 bp, the affinity of the taxa makes it very difficult to establish real phylogenetics.
LEfSe analysis showed differentially increased abundance of Vibrio in posterior intestinal sections of fish fed with Ulva diet. Vibrio genus members have been reported as abundant taxa in the intestinal microbiota of marine fish species (Nayak, 2010; Sullam et al., 2012; Tapia-Paniagua et al., 2019). Although sequences were not assigned to species level in our study, Tapia-Paniagua et al. (2019) observed that sequences related to Vibrio genus were mainly assigned to the chitinase-producing V. scophthalmi. Some Vibrio species are known as pathogenic for fish, but the presence of pathogens as part of the fish microbiome has been previously reported, though its presence did not imply any symptoms of disease (Roeselers et al., 2011; Xing et al., 2013; Wanka et al., 2018). Although it would be very interesting to know if the lineages found in this study are related to pathogenic strains or not, due to the reason explained above about the methodology, it is difficult to establish.
Analysis of the composition of microbial communities does not provide information on the metabolic functionality of the microbiota. PICRUSt analysis has been used to infer functional capabilities of the microbial communities in several studies carried out in fish (Eichmiller et al., 2016; Cornejo-Granados et al., 2017; Zhang et al., 2017; Huang et al., 2018; Kim et al., 2021). It has afforded to infer functional differences according to different intestinal sections (Zhang et al., 2017) and functional changes associated to the trophic level (Liu et al., 2016). As far as we know, this is the first work addressing the study of the functional potential of bacterial communities in the intestinal tract of S. senegalensis.
Predicted functions of microbiota did not show significant changes in anterior intestinal sections of fish fed control and U. ohnoi diets. However, along with differences in microbial composition, differences were predicted in microbiota functionality in the posterior intestinal tract. In these sections, predicted functionality of microbiota of fish receiving Ulva diet showed increased abundance of genes involved in xenobiotic biodegradation and metabolism (KEGG 2 level). Due to the limitation of the fishmeal to make aquafeeds, the dietary inclusion of higher levels of plant material is increasing. This substitution in the feed also introduces unwanted substances, some of them as xenobiotics (Sanden et al., 2018; Olsvik et al., 2019; Oliveira and Vasconcelos, 2020).
Gut microbiome has an important role not only on dietary nutrient digestion, but also on xenobiotics metabolism. Thus, activity of intestinal microbiota may result in the inactivation of xenobiotics or, on the contrary, lead to bioactivation of some compounds resulting in increased toxicity (Collins and Patterson, 2020). On the other hand, it has been reported that exposure to dioxin-type polychlorinated biphenyls (PCB) results in disrupted gut microbiota and host metabolism as well as intestinal inflammation (Petriello et al., 2018; Sun et al., 2019). The influence of xenobiotics on fish microbiota composition has already been documented. In fathead minnow (Pimephales promelas), the exposure to benzo[a]pyrene results in shifts in microbial composition and enrichment in hydrocarbon-degrading taxa (DeBofsky et al., 2020).
In the present study, the enrichment in microbial communities capable to degrade xenobiotics in S. senegalensis fed with U. ohnoi diet may be considered a positive trait. In this way, improved intestinal mucosa has been reported in S. senegalensis fed with this macroalgae (Vizcaíno et al., 2019). Similarly, Tarnecki et al. (2019) suggested that greater xenobiotic degradation ability of microbiota may contribute to increased Common snook (Centropomus undecimalis) survival in larvae treated with probiotics.
On the other hand, decreased abundance of genes related to beta-lactam resistance (KEGG 3 level) was predicted in the microbiota of fish fed with Ulva-supplemented diet compared to the control diet group. In a recent study, Kokou et al. (2020) have reported the existence of a core microbiota in European seabass (Dicentrarchus labrax) intestinal tract after treatment with antibiotics, though they did not elucidate if these populations contained increased numbers of antibiotic resistance genes. Increasing microbial resistance to antibiotics represents a global concern due to their potential transmission not only to animal but also to human pathogens. In addition, discharge of aquaculture waste containing antibiotic-resistant bacteria results in environmental impact, highlighting the importance of the presence of antibiotic resistance genes in the fish gut microbiome (Kokou et al., 2020). Lower abundance of antibiotic resistance genes predicted in the microbiota of S. senegalensis receiving dietary U. ohnoi could contribute to reduced antimicrobial resistance dispersion in aquaculture environments.
This finding was accompanied with higher abundance of genes related to penicillin and cephalosporin biosynthesis in fish fed with diet devoid of U. ohnoi. It may be considered that lower levels of antibiotics can be expected to be jointly found with lower resistance levels thanks to a selection pressure process.
On the other hand, presence of antibiotics in an ecosystem influences the populations comprising microbial communities. Whether differences observed in the microbiota composition of fish fed with control and Ulva diet may also reflect the presence of genes involved in antibiotic biosynthesis needs to be elucidated.
In summary, long-term dietary inclusion of U. ohnoi results in modulation of S. senegalensis intestinal microbiota, especially in posterior intestinal sections. In addition, influence of the diet on the intestinal functionality of this fish species has been studied for the first time. Increased abundance of predicted genes involved in xenobiotic biodegradation and metabolism has been observed in the microbiota when U. ohnoi diet was used. On the contrary, predicted percentages of genes associated to penicillin and cephalosporin biosynthesis as well as beta-lactam resistance were reduced after feeding Ulva diet. Results reported represent the starting point in the study of the effects of diets on potential changes in S. senegalensis intestinal tract microbial community functionality.
Data Availability Statement
The datasets presented in this study can be found in online repositories. The names of the repository/repositories and accession number(s) can be found below: https://www.ncbi.nlm.nih.gov/, PRJNA765168.
Ethics Statement
The animal study was reviewed and approved by European Union (2010/63/UE) and the Spanish legislation (RD 1201/2005 and RD 53/2013) for the use of laboratory animals. All procedures were authorized by the Bioethics and Animal Welfare Committee of the Institute of Agricultural and Fisheries Research and Training (IFAPA) and given the registration number 17/11/2016/171 according to the national authorities for regulation of animal care and experimentation.
Author Contributions
IC and RB performed the bioinformatic analysis and presented the results. ST-P and MF carried out the sampling and DNA extraction. CF-D and VA participated in sampling, cultivation of the algae, and fish maintenance. FA designed and prepared the aquafeeds. MM and MB performed the interpretation of results and wrote the manuscript. MB, MM, CF-D, and FA designed the work. All authors contributed to the discussion and final writing.
Funding
This work was supported by INIA, Ministerio de Economía y Competitividad and co-financed by FEDER funds (Grant RTA2014-00023 C02).
Conflict of Interest
The authors declare that the research was conducted in the absence of any commercial or financial relationships that could be construed as a potential conflict of interest.
Publisher’s Note
All claims expressed in this article are solely those of the authors and do not necessarily represent those of their affiliated organizations, or those of the publisher, the editors and the reviewers. Any product that may be evaluated in this article, or claim that may be made by its manufacturer, is not guaranteed or endorsed by the publisher.
Supplementary Material
The Supplementary Material for this article can be found online at: https://www.frontiersin.org/articles/10.3389/fmicb.2021.801744/full#supplementary-material
Footnotes
References
Abraham, T. J., Paul, P., Adikesavalu, H., Patra, A., and Banerjee, S. (2016). Stenotrophomonas maltophilia as an opportunistic pathogen in cultured African catfish Clarias gariepinus (Burchell, 1822). Aquaculture 450, 168–172. doi: 10.1016/j.aquaculture.2015.07.015
Andrews, S., Krueger, F., Segonds-Pichon, A., Biggins, L., Krueger, C., and Wingett, S. (2010). FastQC: a Quality Control Tool for High Throughput Sequence Data [Online]. Available online at: http://www.bioinformatics.babraham.ac.uk/projects/fastqc/
Araújo, R., Vázquez Calderón, F., Sánchez López, J., Azevedo, I. C., Bruhn, A., Fluch, S., et al. (2021). Current status of the algae production industry in Europe: an emerging sector of the blue bioeconomy. Front. Mar. Sci. 7:1247. doi: 10.3389/fmars.2020.626389
Askarian, F., Zhou, Z., Olsen, R. E., Sperstad, S., and Ringø, E. (2012). Culturable autochthonous gut bacteria in Atlantic salmon (Salmo salar L.) fed diets with or without chitin. Characterization by 16S rRNA gene sequencing, ability to produce enzymes and in vitro growth inhibition of four fish pathogens. Aquaculture 326–329, 1–8. doi: 10.1016/j.aquaculture.2011.10.016
Azaza, M. S., Mensi, F., Ksouri, J., Dhraief, M. N., Brini, B., Abdelmouleh, A., et al. (2008). Growth of Nile tilapia (Oreochromis niloticus L.) fed with diets containing graded levels of green algae ulva meal (Ulva rigida) reared in geothermal waters of southern Tunisia. J. Appl. Ichthyol. 24, 202–207. doi: 10.1111/j.1439-0426.2007.01017.x
Bell, T., Newman, J. A., Silverman, B. W., Turner, S. L., and Lilley, A. K. (2005). The contribution of species richness and composition to bacterial services. Nature 436, 1157–1160. doi: 10.1038/nature03891
Bokulich, N. A., Subramanian, S., Faith, J. J., Gevers, D., Gordon, J. I., Knight, R., et al. (2013). Quality-filtering vastly improves diversity estimates from Illumina amplicon sequencing. Nat. Methods 10, 57–59. doi: 10.1038/nmeth.2276
Cerezuela, R., Guardiola, F. A., Meseguer, J., and Esteban, M. Á (2012). Enrichment of gilthead seabream (Sparus aurata L.) diet with microalgae: effects on the immune system. Fish Physiol. Biochem. 38, 1729–1739. doi: 10.1007/s10695-012-9670-9
Collins, S. L., and Patterson, A. D. (2020). The gut microbiome: an orchestrator of xenobiotic metabolism. Acta Pharm. Sin. B 10, 19–32. doi: 10.1016/j.apsb.2019.12.001
Cornejo-Granados, F., Lopez-Zavala, A. A., Gallardo-Becerra, L., Mendoza-Vargas, A., Sánchez, F., Vichido, R., et al. (2017). Microbiome of Pacific Whiteleg shrimp reveals differential bacterial community composition between Wild, Aquacultured and AHPND/EMS outbreak conditions. Sci. Rep. 7:11783. doi: 10.1038/s41598-017-11805-w
De, B. C., Meena, D. K., Behera, B. K., Das, P., Das Mohapatra, P. K., and Sharma, A. P. (2014). Probiotics in fish and shellfish culture: immunomodulatory and ecophysiological responses. Fish Physiol. Biochem. 40, 921–971. doi: 10.1007/s10695-013-9897-0
DeBofsky, A., Xie, Y., Grimard, C., Alcaraz, A. J., Brinkmann, M., Hecker, M., et al. (2020). Differential responses of gut microbiota of male and female fathead minnow (Pimephales promelas) to a short-term environmentally-relevant, aqueous exposure to benzo[a]pyrene. Chemosphere 252:126461. doi: 10.1016/j.chemosphere.2020.126461
Diler, I., Tekinay, A., Guroy, D., Guroy, B., and Soyuturk, M. (2007). Effects of Ulva rigida on the growth, feed intake and body composition of common carp, Cyprinus carpio L. J. Biol. Sci. 7, 305–308.
Edgar, R. C., Haas, B. J., Clemente, J. C., Quince, C., and Knight, R. (2011). UCHIME improves sensitivity and speed of chimera detection. Bioinformatics 27, 2194–2200. doi: 10.1093/bioinformatics/btr381
Eichmiller, J. J., Hamilton, M. J., Staley, C., Sadowsky, M. J., and Sorensen, P. W. (2016). Environment shapes the fecal microbiome of invasive carp species. Microbiome 4:44. doi: 10.1186/s40168-016-0190-1
Ergün, S., Soyutürk, M., Güroy, B., Güroy, D., and Merrifield, D. (2009). Influence of Ulva meal on growth, feed utilization, and body composition of juvenile Nile tilapia (Oreochromis niloticus) at two levels of dietary lipid. Aquac. Int. 17, 355–361. doi: 10.1007/s10499-008-9207-5
Farias, D. R., Simioni, C., Poltronieri, E., Bouzon, Z. L., and Macleod, C. K. (2017). Fine-tuning transmission electron microscopy methods to evaluate the cellular architecture of Ulvacean seaweeds (Chlorophyta). Micron 96, 48–56. doi: 10.1016/j.micron.2017.02.003
Fernández-Díaz, C., Coste, O., and Malta, E. J. (2017). Polymer chitosan nanoparticles functionalized with Ulva ohnoi extracts boost in vitro ulvan immunostimulant effect in Solea senegalensis macrophages. Algal Res. 26, 135–142. doi: 10.1016/j.algal.2017.07.008
Fumanal, M., Di Zeo, D. E., Anguís, V., Fernández-Diaz, C., Alarcón, F. J., Piñera, R., et al. (2020). Inclusion of dietary Ulva ohnoi 5% modulates Solea senegalensis immune response during Photobacterium damselae subsp. piscicida infection. Fish Shellfish Immunol. 100, 186–197. doi: 10.1016/j.fsi.2020.03.007
García-Casal, M. N., Pereira, A. C., Leets, I., Ramírez, J., and Quiroga, M. F. (2007). High iron content and bioavailability in humans from four species of marine algae. J. Nutr. 137, 2691–2695. doi: 10.1093/jn/137.12.2691
Geng, Y., Wang, K., Chen, D., Huang, X., He, M., and Yin, Z. (2010). Stenotrophomonas maltophilia, an emerging opportunist pathogen for cultured channel catfish, Ictalurus punctatus, in China. Aquaculture 308, 132–135. doi: 10.1016/J.AQUACULTURE.2010.08.032
Ghanbari, M., Kneifel, W., and Domig, K. J. (2015). A new view of the fish gut microbiome: advances from next-generation sequencing. Aquaculture 448, 464–475. doi: 10.1016/j.aquaculture.2015.06.033
Gonçalves, A. T., and Gallardo-Escárate, C. (2017). Microbiome dynamic modulation through functional diets based on pre- and probiotics (mannan-oligosaccharides and Saccharomyces cerevisiae) in juvenile rainbow trout (Oncorhynchus mykiss). J. Appl. Microbiol. 122, 1333–1347. doi: 10.1111/jam.13437
Guerreiro, I., Magalhães, R., Coutinho, F., Couto, A., Sousa, S., Delerue-Matos, C., et al. (2019). Evaluation of the seaweeds Chondrus crispus and Ulva lactuca as functional ingredients in gilthead seabream (Sparus aurata). J. Appl. Phycol. 31, 2115–2124. doi: 10.1007/s10811-018-1708-7
Guillard, R. R. L. (1975). “Culture of phytoplankton for feeding marine invertebrates,” in Culture of Marine Invertebrate Animals, eds W. L. Smith and M. H. Chanley (Berlin: Springer), 29–60. doi: 10.1007/978-1-4615-8714-9_3
Güroy, B., Şahin, I., Mantoglu, S., and Kayali, S. (2012). Spirulina as a natural carotenoid source on growth, pigmentation and reproductive performance of yellow tail cichlid Pseudotropheus acei. Aquac. Int. 20, 869–878. doi: 10.1007/s10499-012-9512-x
Hammer, Ø, Harper, D. A. T., and Ryan, P. D. (2001). Past: paleontological statistics software package for education and data analysis. Palaeontol. Electron. 4:178.
He, S., Zhou, Z., Banerjee, G., Huang, L., Ray, A. K., and Ringø, E. (2016). Bacterial diversity in the digestive tracts of four indian air-breathing fish species investigated by PCR based denaturing gradient gel electrophoresis. Brazilian Arch. Biol. Technol. 59, 1–11. doi: 10.1590/1678-4324-2016160332
Huang, W., Cheng, Z., Lei, S., Liu, L., Lv, X., Chen, L., et al. (2018). Community composition, diversity, and metabolism of intestinal microbiota in cultivated European eel (Anguilla anguilla). Appl. Microbiol. Biotechnol. 102, 4143–4157. doi: 10.1007/s00253-018-8885-9
Ingerslev, H. C., Strube, M. L., Jørgensen, L. V. G., Dalsgaard, I., Boye, M., Madsen, L., et al. (2014a). Diet type dictates the gut microbiota and the immune response against Yersinia ruckeri in rainbow trout (Oncorhynchus mykiss). Fish Shellfish Immunol. 40, 624–633. doi: 10.1016/j.fsi.2014.08.021
Ingerslev, H. C., von Gersdorff Jørgensen, L., Lenz Strube, M., Larsen, N., Dalsgaard, I., Boye, M., et al. (2014b). The development of the gut microbiota in rainbow trout (Oncorhynchus mykiss) is affected by first feeding and diet type. Aquaculture 424–425, 24–34. doi: 10.1016/j.aquaculture.2013.12.032
Kanehisa, M., Goto, S., Sato, Y., Kawashima, M., Furumichi, M., and Tanabe, M. (2014). Data, information, knowledge and principle: back to metabolism in KEGG. Nucleic Acids Res. 42:D199-205. doi: 10.1093/nar/gkt1076
Kang, J., Park, J., Seo, J. K., Choi, W., Choi, S., Kim, J., et al. (2021). Intestinal anti-inflammatory activity of Ulva ohnoi oil in DSS-induced experimental mouse model. Sci. Rep. 11:15087. doi: 10.1038/s41598-021-94475-z
Kazemi, M., Abediankenari, A., and Rabieri, R. (2018). Effect of marine macroalgae on growth performance and immune response in rainbow trout fingerlings. J. Fish. Sci. Technol. 7, 9–16.
Kim, P. S., Shin, N.-R., Lee, J.-B., Kim, M.-S., Whon, T. W., Hyun, D.-W., et al. (2021). Host habitat is the major determinant of the gut microbiome of fish. Microbiome 9:166. doi: 10.1186/S40168-021-01113-X
Klindworth, A., Pruesse, E., Schweer, T., Peplies, J., Quast, C., Horn, M., et al. (2013). Evaluation of general 16S ribosomal RNA gene PCR primers for classical and next-generation sequencing-based diversity studies. Nucleic Acids Res. 41:e1. doi: 10.1093/nar/gks808
Kokou, F., Sasson, G., Mizrahi, I., and Cnaani, A. (2020). Antibiotic effect and microbiome persistence vary along the European seabass gut. Sci. Rep. 10:10003. doi: 10.1038/s41598-020-66622-5
Korzen, L., Pulidindi, I. N., Israel, A., Abelson, A., and Gedanken, A. (2015). Marine integrated culture of carbohydrate rich Ulva rigida for enhanced production of bioethanol. RSC Adv. 5, 59251–59256. doi: 10.1039/C5RA09037G
Lahaye, M., and Robic, A. (2007). Structure and functional properties of Ulvan, a Polysaccharide from Green Seaweeds. Biomacromolecules 8, 1765–1774. doi: 10.1021/bm061185q
Langille, M. G. I., Zaneveld, J., Caporaso, J. G., McDonald, D., Knights, D., Reyes, J. A., et al. (2013). Predictive functional profiling of microbial communities using 16S rRNA marker gene sequences. Nat. Biotechnol. 31, 814–821. doi: 10.1038/nbt.2676
Liu, H., Guo, X., Gooneratne, R., Lai, R., Zeng, C., Zhan, F., et al. (2016). The gut microbiome and degradation enzyme activity of wild freshwater fishes influenced by their trophic levels. Sci. Rep. 6:24340. doi: 10.1038/SREP24340
Llewellyn, M. S., Boutin, S., Hoseinifar, S. H., and Derome, N. (2014). Teleost microbiomes: the state of the art in their characterization, manipulation and importance in aquaculture and fisheries. Front. Microbiol. 5:207. doi: 10.3389/fmicb.2014.00207
Magnoni, L. J., Martos-Sitcha, J. A., Queiroz, A., Calduch-Giner, J. A., Gonçalves, J. F. M., Rocha, C. M. R., et al. (2017). Dietary supplementation of heat-treated Gracilaria and Ulva seaweeds enhanced acute hypoxia tolerance in gilthead seabream (Sparus aurata). Biol. Open 6, 897–908. doi: 10.1242/bio.024299
Martínez-Antequera, F. P., Martos-Sitcha, J. A., Reyna, J. M., and Moyano, F. J. (2021). Evaluation of the inclusion of the green seaweed Ulva ohnoi as an ingredient in feeds for gilthead sea bream (Sparus aurata) and european sea bass (Dicentrarchus labrax). Animals 11:1684. doi: 10.3390/ani11061684
McDonald, D., Price, M. N., Goodrich, J., Nawrocki, E. P., Desantis, T. Z., Probst, A., et al. (2012). An improved Greengenes taxonomy with explicit ranks for ecological and evolutionary analyses of bacteria and archaea. ISME J. 6, 610–618. doi: 10.1038/ismej.2011.139
McMurdie, P. J., and Holmes, S. (2013). Phyloseq: an R Package for reproducible interactive analysis and graphics of microbiome census data. PLoS One 8:e61217. doi: 10.1371/journal.pone.0061217
Michel, G., and Czjzek, M. (2013). “Polysaccharide-degrading enzymes from marine bacteria,” in Marine Enzymes for Biocatalysis: Sources, Biocatalytic Characteristics and Bioprocesses of Marine Enzymes, ed. Woodhead Publishing (Amsterdam: Elsevier), 429–464. doi: 10.1533/9781908818355.3.429
Morais, S., Aragão, C., Cabrita, E., Conceição, L. E. C., Constenla, M., Costas, B., et al. (2016). New developments and biological insights into the farming of Solea senegalensis reinforcing its aquaculture potential. Rev. Aquac. 8, 227–263. doi: 10.1111/RAQ.12091
Moutinho, S., Linares, F., Rodríguez, J. L., Sousa, V., and Valente, L. M. P. (2018). Inclusion of 10% seaweed meal in diets for juvenile and on-growing life stages of Senegalese sole (Solea senegalensis). J. Appl. Phycol. 30, 3589–3601. doi: 10.1007/s10811-018-1482-6
Mukherjee, P., and Roy, P. (2016). Genomic potential of Stenotrophomonas maltophilia in bioremediation with an assessment of its multifaceted role in our environment. Front. Microbiol. 7:967. doi: 10.3389/FMICB.2016.00967
Mustafa, M. G., and Nakagawa, H. (1995). A review - dietary benefits of algae as an additive in fish feed. Isr. J. Aquac. 47, 155–162. doi: 10.1186/1475-2859-11-96
Nayak, S. K. (2010). Role of gastrointestinal microbiota in fish. Aquac. Res. 41, 1553–1573. doi: 10.1111/J.1365-2109.2010.02546.X
Naylor, R. L., Hardy, R. W., Buschmann, A. H., Bush, S. R., Cao, L., Klinger, D. H., et al. (2021). A 20-year retrospective review of global aquaculture. Nature 591, 551–563. doi: 10.1038/s41586-021-03308-6
Nevita, T., Sharma, G. D., and Pandey, P. (2018). Composting of rice-residues using lignocellulolytic plant-probiotic Stenotrophomonas maltophilia, and its evaluation for growth enhancement of Oryza sativa L. Environ. Sustain. 1, 185–196. doi: 10.1007/s42398-018-0017-z
Norambuena, F., Hermon, K., Skrzypczyk, V., Emery, J. A., Sharon, Y., Beard, A., et al. (2015). Algae in fish feed: performances and fatty acid metabolism in juvenile atlantic salmon. PLoS One 10:e0124042. doi: 10.1371/JOURNAL.PONE.0124042
Oca, J., Cremades, J., Jiménez, P., Pintado, J., and Masaló, I. (2019). Culture of the seaweed Ulva ohnoi integrated in a Solea senegalensis recirculating system: influence of light and biomass stocking density on macroalgae productivity. J. Appl. Phycol. 31, 2461–2467. doi: 10.1007/s10811-019-01767-z
Oliveira, M., and Vasconcelos, V. (2020). Occurrence of mycotoxins in fish feed and its effects: a review. Toxins 12:160. doi: 10.3390/toxins12030160
Olsvik, P. A., Larsen, A. K., Berntssen, M. H. G., Goksøyr, A., Karlsen, O. A., Yadetie, F., et al. (2019). Effects of agricultural pesticides in aquafeeds on wild fish feeding on leftover pellets near fish farms. Front. Genet. 10:794. doi: 10.3389/fgene.2019.00794
Ortiz, J., Romero, N., Robert, P., Araya, J., Lopez-Hernández, J., Bozzo, C., et al. (2006). Dietary fiber, amino acid, fatty acid and tocopherol contents of the edible seaweeds Ulva lactuca and Durvillaea antarctica. Food Chem. 99, 98–104. doi: 10.1016/j.foodchem.2005.07.027
Packer, M. A., Harris, G. C., and Adams, S. L. (2016). “Food and feed applications of algae,” in Algae Biotechnology. Green Energy and Technology, eds F. Bux and Y. Chisti (Cham: Springer), 217–247. doi: 10.1007/978-3-319-12334-9_12
Peixoto, M. J., Salas-Leitón, E., Brito, F., Pereira, L. F., Svendsen, J. C., Baptista, T., et al. (2017). Effects of dietary Gracilaria sp. and Alaria sp. supplementation on growth performance, metabolic rates and health in meagre (Argyrosomus regius) subjected to pathogen infection. J. Appl. Phycol. 29, 433–447. doi: 10.1007/s10811-016-0917-1
Peixoto, M. J., Salas-Leitón, E., Pereira, L. F., Queiroz, A., Magalhães, F., Pereira, R., et al. (2016). Role of dietary seaweed supplementation on growth performance, digestive capacity and immune and stress responsiveness in European seabass (Dicentrarchus labrax). Aquac. Rep. 3, 189–197. doi: 10.1016/j.aqrep.2016.03.005
Petriello, M. C., Hoffman, J. B., Vsevolozhskaya, O., Morris, A. J., and Hennig, B. (2018). Dioxin-like PCB 126 increases intestinal inflammation and disrupts gut microbiota and metabolic homeostasis. Environ. Pollut. 242, 1022–1032. doi: 10.1016/j.envpol.2018.07.039
Ponce, M., Zuasti, E., Anguís, V., and Fernández-Díaz, C. (2020). Effects of the sulfated polysaccharide ulvan from Ulva ohnoi on the modulation of the immune response in Senegalese sole (Solea senegalensis). Fish Shellfish Immunol. 100, 27–40. doi: 10.1016/J.FSI.2020.02.054
Prabhu, M., Chemodanov, A., Gottlieb, R., Kazir, M., Nahor, O., Gozin, M., et al. (2019). Starch from the sea: the green macroalga Ulva ohnoi as a potential source for sustainable starch production in the marine biorefinery. Algal Res. 37, 215–227. doi: 10.1016/j.algal.2018.11.007
Puello, L., Montoya, O., Castañeda, V., and Moreno, L. (2018). Caracterización de la microbiota presente en el intestino de Piaractus brachypomus (Cachamablanca). Rev. Salud Anim. 40, 1–12.
Rawls, J. F., Samuel, B. S., and Gordon, J. I. (2004). From the cover: gnotobiotic zebrafish reveal evolutionarily conserved responses to the gut microbiota. Proc. Natl. Acad. Sci. U.S.A. 101, 4596–4601. doi: 10.1073/pnas.0400706101
Reina, J. C., Torres, M., and Llamas, I. (2019). Stenotrophomonas maltophilia AHL-degrading strains isolated from marine invertebrate microbiota attenuate the virulence of Pectobacterium carotovorum and Vibrio coralliilyticus. Mar. Biotechnol. 21, 276–290. doi: 10.1007/s10126-019-09879-w
Reisky, L., Prechoux, A., Zühlke, M.-K., Bäumgen, M., Robb, C., Gerlach, N., et al. (2019). A marine bacterial enzymatic cascade degrades the algal polysaccharide ulvan. Nat. Chem. Biol. 15, 803–4812. doi: 10.1038/s41589-019-0311-9
Ringø, E., Zhou, Z., Vecino, J. L. G., Wadsworth, S., Romero, J., Krogdahl, Å, et al. (2016). Effect of dietary components on the gut microbiota of aquatic animals. A never-ending story? Aquac. Nutr. 22, 219–282. doi: 10.1111/anu.12346
Roeselers, G., Mittge, E. K., Stephens, W. Z., Parichy, D. M., Cavanaugh, C. M., Guillemin, K., et al. (2011). Evidence for a core gut microbiota in the zebrafish. ISME J. 5, 1595–1608. doi: 10.1038/ismej.2011.38
Romero, J., Ringø, E., and Merrifield, D. L. (2014). “The gut microbiota of fish,” in Aquaculture Nutrition, eds D. Merrifield and E. Ringø (Chichester: John Wiley & Sons, Ltd), 75–100. doi: 10.1002/9781118897263.ch4.
Sáez, M. I., Martinez, T., and Alarcón, J. (2013). Effect of dietary inclusion of seaweeds on intestinal proteolytic activity of juvenile sea bream, Sparus aurata. Int. Aquafeed 3, 38–41.
Salinas, I., Díaz-Rosales, P., Cuesta, A., Meseguer, J., Chabrillón, M., Moriñigo, M. Á, et al. (2006). Effect of heat-inactivated fish and non-fish derived probiotics on the innate immune parameters of a teleost fish (Sparus aurata L.). Vet. Immunol. Immunopathol. 111, 279–286. doi: 10.1016/j.vetimm.2006.01.020
Sanden, M., Olsvik, P. A., Søfteland, L., Rasinger, J. D., Rosenlund, G., Garlito, B., et al. (2018). Dietary pesticide chlorpyrifos-methyl affects arachidonic acid metabolism including phospholipid remodeling in Atlantic salmon (Salmo salar L.). Aquaculture 484, 1–12. doi: 10.1016/j.aquaculture.2017.10.033
Schloss, P. D., Westcott, S. L., Ryabin, T., Hall, J. R., Hartmann, M., Hollister, E. B., et al. (2009). Introducing mothur: open-source, platform-independent, community-supported software for describing and comparing microbial communities. Appl. Environ. Microbiol. 75, 7537–7541. doi: 10.1128/AEM.01541-09
Segata, N., Izard, J., Waldron, L., Gevers, D., Miropolsky, L., Garrett, W. S., et al. (2011). Metagenomic biomarker discovery and explanation. Genome Biol. 12:R60. doi: 10.1186/GB-2011-12-6-R60
Semova, I., Carten, J. D., Stombaugh, J., Mackey, L. C., Knight, R., Farber, S. A., et al. (2012). Microbiota regulate intestinal absorption and metabolism of fatty acids in the Zebrafish. Cell Host Microbe 12, 277–288. doi: 10.1016/j.chom.2012.08.003
Silva, D. M., Valente, L. M. P., Sousa-Pinto, I., Pereira, R., Pires, M. A., Seixas, F., et al. (2015). Evaluation of IMTA-produced seaweeds (Gracilaria, Porphyra, and Ulva) as dietary ingredients in Nile tilapia, Oreochromis niloticus L., juveniles. Effects on growth performance and gut histology. J. Appl. Phycol. 27, 1671–1680. doi: 10.1007/s10811-014-0453-9
Sullam, K. E., Essinger, S. D., Lozupone, C. A., O’connor, M. P., Rosen, G. L., Knight, R., et al. (2012). Environmental and ecological factors that shape the gut bacterial communities of fish: a meta-analysis. Mol. Ecol. 21, 3363–3378. doi: 10.1111/j.1365-294X.2012.05552.x
Sun, Y., Tang, L., Liu, Y., Hu, C., Zhou, B., Lam, P. K. S., et al. (2019). Activation of aryl hydrocarbon receptor by dioxin directly shifts gut microbiota in zebrafish. Environ. Pollut. 255:113357. doi: 10.1016/J.ENVPOL.2019.113357
Tapia-Paniagua, S. T., Fumanal, M., Anguís, V., Fernández-DÍaz, C., Alarcón, F. J., Moriñigo, M. A., et al. (2019). Modulation of intestinal microbiota in Solea senegalensis fed low dietary level of Ulva ohnoi. Front. Microbiol. 10:171. doi: 10.3389/fmicb.2019.00171
Tapia-Paniagua, S. T., Reyes-Becerril, M., Ascencio-Valle, F., Esteban, M. Á, Clavijo, E., Balebona, M. C., et al. (2011). Modulation of the intestinal microbiota and immune system of farmed Sparus aurata by the administration of the yeast Debaryomyces hansenii L2 in conjunction with inulin. J. Aquac. Res. Dev. 1:12. doi: 10.4172/2155-9546.S1-012
Tapia-Paniagua, S., Lobo, C., Moreno-Ventas, X., de la Banda, I. G., Moriñigo, M. A., and Balebona, M. C. (2014). Probiotic supplementation influences the diversity of the intestinal microbiota during early stages of farmed senegalese sole (Solea senegalensis, Kaup 1858). Mar. Biotechnol. 16, 716–728. doi: 10.1007/s10126-014-9588-6
Tarnecki, A. M., Brennan, N. P., Schloesser, R. W., and Rhody, N. R. (2019). Shifts in the skin-associated microbiota of hatchery-reared common snook centropomus undecimalis during acclimation to the wild. Microb. Ecol. 77, 770–781. doi: 10.1007/s00248-018-1252-7
Torabi Delshad, S., Soltanian, S., Sharifiyazdi, H., Haghkhah, M., and Bossier, P. (2018). Identification of N -acyl homoserine lactone-degrading bacteria isolated from rainbow trout (Oncorhynchus mykiss). J. Appl. Microbiol. 125, 356–369. doi: 10.1111/jam.13891
Toro, F., Alarcón, J., Márquez, S., Capella, J., Bahamonde, P., Esperón, F., et al. (2021). Composition and structure of the skin microbiota of rorquals off the Eastern South Pacific. FEMS Microbiol. Ecol. 97:fiab050. doi: 10.1093/femsec/fiab050
Valente, L. M. P., Araújo, M., Batista, S., Peixoto, M. J., Sousa-Pinto, I., Brotas, V., et al. (2016). Carotenoid deposition, flesh quality and immunological response of Nile tilapia fed increasing levels of IMTA-cultivated Ulva spp. J. Appl. Phycol. 28, 691–701. doi: 10.1007/s10811-015-0590-9
Valente, L. M. P., Gouveia, A., Rema, P., Matos, J., Gomes, E. F., and Pinto, I. S. (2006). Evaluation of three seaweeds Gracilaria bursa-pastoris, Ulva rigida and Gracilaria cornea as dietary ingredients in European sea bass (Dicentrarchus labrax) juveniles. Aquaculture 252, 85–91. doi: 10.1016/j.aquaculture.2005.11.052
Vizcaíno, A. J., Fumanal, M., Sáez, M. I., Martínez, T. F., Moriñigo, M. A., Fernández-Díaz, C., et al. (2019). Evaluation of Ulva ohnoi as functional dietary ingredient in juvenile Senegalese sole (Solea senegalensis): effects on the structure and functionality of the intestinal mucosa. Algal Res. 42:101608. doi: 10.1016/J.ALGAL.2019.101608
Vizcaíno, A. J., Mendes, S. I., Varela, J. L., Ruiz-Jarabo, I., Rico, R., Figueroa, F. L., et al. (2016). Growth, tissue metabolites and digestive functionality in Sparus aurata juveniles fed different levels of macroalgae, Gracilaria cornea and Ulva rigida. Aquac. Res. 47, 3224–3238. doi: 10.1111/are.12774
Vizcaíno, A. J., Rodiles, A., López, G., Sáez, M. I., Herrera, M., Hachero, I., et al. (2018). Growth performance, body composition, and digestive functionality of Senegalese sole (Solea senegalensis Kaup, 1858) juveniles fed diets including microalgae freeze-dried biomass. Fish Physiol. Biochem. 442, 661–677. doi: 10.1007/S10695-018-0462-8
Wang, C., Liu, Y., Sun, G., Li, X., and Liu, Z. (2019). Growth, immune response, antioxidant capability, and disease resistance of juvenile Atlantic salmon (Salmo salar L.) fed Bacillus velezensis V4 and Rhodotorula mucilaginosa compound. Aquaculture 500, 65–74. doi: 10.1016/J.AQUACULTURE.2018.09.052
Wang, L., Zhao, X., Xu, H., Bao, X., Liu, X., Chang, Y., et al. (2018). Characterization of the bacterial community in different parts of the gut of sea cucumber (Apostichopus Japonicus) and its variation during gut regeneration. Aquac. Res. 49, 1987–1996. doi: 10.1111/are.13654
Wanka, K. M., Damerau, T., Costas, B., Krueger, A., Schulz, C., and Wuertz, S. (2018). Isolation and characterization of native probiotics for fish farming. BMC Microbiol. 18:119. doi: 10.1186/s12866-018-1260-2
Wassef, E. A., El-Sayed, A. F. M., Kandeel, K. M., and Sakr, E. M. (2005). Evaluation of Pterocla dia (Rhodophyta) and Ulva (Chlorophyta) meals as additives to gilthead seabream Sparus aurata diets. Egypt. J. Aquat. Res. 31, 321–332.
Wassef, E. A., El-Sayed, A.-F. M., and Sakr, E. M. (2013). Pterocladia (Rhodophyta) and Ulva (Chlorophyta) as feed supplements for European seabass, Dicentrarchus labrax L., fry. J. Appl. Phycol. 25, 1369–1376. doi: 10.1007/s10811-013-9995-5
Webster, T. M. U., Rodriguez-Barreto, D., Castaldo, G., Gough, P., Consuegra, S., and de Leaniz, C. G. (2020). Environmental plasticity and colonisation history in the Atlantic salmon microbiome: a translocation experiment. Mol. Ecol. 29, 886–898. doi: 10.1111/MEC.15369
Wells, M. L., Potin, P., Craigie, J. S., Raven, J. A., Merchant, S. S., Helliwell, K. E., et al. (2017). Algae as nutritional and functional food sources: revisiting our understanding. J. Appl. Phycol. 29, 949–982. doi: 10.1007/s10811-016-0974-5
Xing, M., Hou, Z., Yuan, J., Liu, Y., Qu, Y., and Liu, B. (2013). Taxonomic and functional metagenomic profiling of gastrointestinal tract microbiome of the farmed adult turbot (Scophthalmus maximus). FEMS Microbiol. Ecol. 86, 432–443. doi: 10.1111/1574-6941.12174
Yaakob, Z., Ali, E., Zainal, A., Mohamad, M., and Takriff, M. S. (2014). An overview: biomolecules from microalgae for animal feed and aquaculture. J. Biol. Res. 211, 1–10. doi: 10.1186/2241-5793-21-6
Ye, L., Amberg, J., Chapman, D., Gaikowski, M., and Liu, W. T. (2014). Fish gut microbiota analysis differentiates physiology and behavior of invasive Asian carp and indigenous American fish. ISME J. 8, 541–551. doi: 10.1038/ISMEJ.2013.181
Yeganeh, S., and Adel, M. (2019). Effects of dietary algae (Sargassum ilicifolium) as immunomodulator and growth promoter of juvenile great sturgeon (Huso huso Linnaeus, 1758). J. Appl. Phycol. 31, 2093–2102. doi: 10.1007/s10811-018-1673-1
Yildirim, Ö, Ergün, S., Yaman, S., and Türker, A. (2009). Rasyonlarda yem katki maddesi olarak iki deniz yosununun (Ulva lactuca, Enteremorpha linza) gökkuşaği alabaliğinin (Oncorhynchus mykiss) büyüme performansi, yem değerlendirme ve vücut kompozisyonu üzerine etkileri. Kafkas Univ. Vet. Fak. Derg. 15, 455–460. doi: 10.9775/kvfd.2009.042-a
Zhang, Q., Saleem, M., and Wang, C. (2017). Probiotic strain Stenotrophomonas acidaminiphila BJ1 degrades and reduces chlorothalonil toxicity to soil enzymes, microbial communities and plant roots. AMB Express 7:227. doi: 10.1186/S13568-017-0530-Y
Zhao, R., Symonds, J. E., Walker, S. P., Steiner, K., Carter, C. G., Bowman, J. P., et al. (2020). Salinity and fish age affect the gut microbiota of farmed Chinook salmon (Oncorhynchus tshawytscha). Aquaculture 528:735539.
Keywords: microbiome, Ulva, algae, Solea senegalensis, aquafeed
Citation: Cerezo IM, Fumanal M, Tapia-Paniagua ST, Bautista R, Anguís V, Fernández-Díaz C, Alarcón FJ, Moriñigo MA and Balebona MC (2022) Solea senegalensis Bacterial Intestinal Microbiota Is Affected by Low Dietary Inclusion of Ulva ohnoi. Front. Microbiol. 12:801744. doi: 10.3389/fmicb.2021.801744
Received: 25 October 2021; Accepted: 20 December 2021;
Published: 08 February 2022.
Edited by:
Franck Carbonero, Washington State University Health Sciences Spokane, United StatesReviewed by:
Raquel Xavier, Centro de Investigacao em Biodiversidade e Recursos Geneticos (CIBIO-InBIO), PortugalMario Tello, University of Santiago, Chile
Copyright © 2022 Cerezo, Fumanal, Tapia-Paniagua, Bautista, Anguís, Fernández-Díaz, Alarcón, Moriñigo and Balebona. This is an open-access article distributed under the terms of the Creative Commons Attribution License (CC BY). The use, distribution or reproduction in other forums is permitted, provided the original author(s) and the copyright owner(s) are credited and that the original publication in this journal is cited, in accordance with accepted academic practice. No use, distribution or reproduction is permitted which does not comply with these terms.
*Correspondence: Miguel A. Moriñigo, bW9yaW5pZ29AdW1hLmVz