- 1Key Laboratory of Bio-Resource and Eco-Environment of Ministry of Education, College of Life Sciences, Sichuan University, Chengdu, Sichuan, China
- 2Animal Disease Prevention and Food Safety Key Laboratory of Sichuan Province, College of Life Sciences, Sichuan University, Chengdu, Sichuan, China
Antibiotic growth promoters (AGPs) have been used as feed additives to improve feed efficiency in food animals for more than six decades. However, the wide use of AGPs has led to the emergence of antibiotic-resistant pathogens of animal origin, posing a significant threat to food safety and public health. China prohibited the addition of AGPs to animal feed from July 2020. The impacts caused by the withdrawal of AGPs on the prevalence and antibiotic resistance of foodborne pathogens have not been illustrated. Here, a total of 471 strains of Campylobacter were isolated from pigs from three pig farms and two slaughterhouses in Sichuan Province for 4 consecutive years (2018–2021), including 2 years before and 2 years after the ban on AGPs in China. The isolation rate of Campylobacter had a slight increase after prohibiting the addition of AGPs to the feed. Contrary to what we expected, the antibiotic susceptibility test and WGS data showed that the antibiotic resistance to gentamicin and florfenicol and the abundance of virulence genes increased significantly after the ban of AGPs. Comparison of the isolates of swine origin with isolates of human origin indicated the potential of antibiotic-resistant Campylobacter transmission from pigs to humans. These data suggested that phasing out AGPs may lead to increased use of therapeutic antimicrobials, promoting the prevalence and transmission of both antibiotic resistance and virulence genes.
Introduction
In the initial stage of antibiotic use, most of them were shared by humans and animals for the purpose of treatment (Richou, 1952). In 1950, the United States first added antibiotics to animal feed as livestock and poultry antibiotic growth promoters (AGPs), and then this strategy was widely used in other countries, including China (Dibner and Richards, 2005). Industries and farmers have benefited from AGPs due to increased productivity. However, with the increasing research on the association of the antibiotic resistance of bacteria and the AGPs used in animals (Elliott and Barnes, 1959; Bush et al., 2011; Wang et al., 2017), recommendations to ban AGPs in animal feed have been discussed by scientists and politicians. The first nation to eliminate the use of antibiotics for growth promotion was Sweden in 1986 (Dibner and Richards, 2005), and since then, many countries have prohibited the addition of AGPs to animal feed (Maron et al., 2013; Salim et al., 2018). China also adopted this legislation, and the regulations came into force on July 2020, banning 11 antibiotics, including bacitracin zinc premix, flavomycin premix, virginiamycin premix, natriuretic peptide premix, avilamycin premix, kitasamycin premix, hygromycin calcium premix, chlortetracycline premix, enramycin premix, bacitracin methylene salicylate premix, and quinolone premix, as feed additives.
After the introduction of a ban on the use of AGPs for livestock, the selection pressure of antibiotics on bacteria was relieved, and the antibiotic resistance profile of intestinal bacteria changed rapidly (Wang et al., 2020). However, there are still many antibiotics used for the treatment of bacterial infections. According to this, we hypothesized that the prevalence and the antibiotic resistance profile may be affected by this prohibition and investigated an important member of the swine gut microbiota, Campylobacter spp., with a whole-genome sequencing approach. Campylobacter species are among the most frequently identified bacterial causes of human gastroenteritis (Kaakoush et al., 2015). As a foodborne pathogen, Campylobacter is constantly exposed to antimicrobial agents. To counteract selection pressure, Campylobacter has developed various mechanisms, including mutations in target genes, such as 23S rRNA mutations to macrolides and gyrA mutation to fluoroquinolones (Tang et al., 2017), multidrug efflux pump CmeABC or RE-CmeABC (Lin et al., 2002; Yao et al., 2016), and horizontally acquired antibiotic resistance genes, such as rRNA methylase Erm(B) (Wang et al., 2014). Some of the mechanisms confer resistance to a specific class of antimicrobials, while others may confer multidrug resistance. In recent years, we have also identified the cross-resistance genes optrA and cfr(C), conferring resistance to structurally unrelated antibiotics (Tang et al., 2020). Of particular concern is resistance to the oxazolidinone class, which is used as the last resort for treating MDR Gram-positive bacterial infections in humans.
The mechanism by which Campylobacter infects the host and induces disease is complex and unique. Unlike classical virulence factors such as T3SS and lipopolysaccharide (LPS) observed in other enteropathogens such as enterotoxigenic E. coli and Salmonella, Campylobacter lacks or does not extensively rely on many classical virulence factors (Gaytán et al., 2016). Campylobacter has a series of special virulence genes.
The classification of major virulence factors in Campylobacter includes motility, invasion, toxin, adhesion, effect delivery system, and immune modulation (van Vliet et al., 1999). The mobility of Campylobacter is encoded by coding genes, but the flagella not only acts as a motor organ but also acts as a secretion device, which can transfer invasion-related virulence factors such as the Campylobacter invasion antigen (Cia) protein, which is delivered into the cytoplasm of the host (Rivera-Amill et al., 2001; Guerry, 2007). Effector delivery systems include not only flagella but also the type IV secretion system, which is used to secrete effectors such as CDT (cytolethal distending toxin) (Kienesberger et al., 2011). The immunomodulatory system of Campylobacter mainly includes capsule and lipooligosaccharide (LOS), which play an important role in bacterial survival and persistence in the environment and in evasion of host immune responses. The presence of heptose residues in the capsule may be important for virulence (Karlyshev et al., 2005). LOS diversity is significant for the ability to colonize diverse hosts and gut niches (Guerry et al., 2000). One should pay attention to the virulence factors of Campylobacter because the presence and abundance of Campylobacter virulence genes can assess the potential threat of the strain to the host.
To analyze the evolutionary changes in Campylobacter after the ban of AGPs, we carried out an extensive and consecutive sampling regime in Sichuan Province for 4 years, including 2 years before the ban of AGPs and 2 years after that.
Materials and methods
Sample collection, Campylobacter isolation, and identification
Campylobacter strains were isolated under two conditions: (1) AGPs could be used as feed additives for livestock (in 2018 and 2019) and (2) after the ban on the addition of AGPs to the feed (after July 2020 and 2021). A total of 1,090 swine fecal samples and cecal contents were collected from three pig farms and two pig slaughterhouses in Sichuan Province, which is one of the largest pig raising provinces in China, for four consecutive years, including 2018 (n = 334), 2019 (n = 376), 2020 (n = 110), and 2021 (n = 120). Fresh swine fecal samples or cecal contents were collected and streaked onto Skirrow agar plates containing Campylobacter selective supplements and incubated at 42°C for 48 h under microaerobic conditions (5% O2, 10% CO2, and 85% N2). Two sets of previously published PCR primers were used to identify and differentiate C. jejuni and C. coli (Linton et al., 1996, 1997).
Antimicrobial susceptibility testing
In total, 200 C. coli (50 from each year) isolates were randomly chosen and assayed in the antimicrobial susceptibility testing. The broth microdilution method as recommended by CLSI was used to determine the susceptibility of Campylobacter isolates to eight antibiotics (florfenicol, linezolid, gentamicin, tetracycline, lincomycin, erythromycin, ciprofloxacin, and ampicillin), and the antimicrobial resistance breakpoints were chosen according to the interpretive standards established by the CLSI (Clinical and Laboratory Standards Institute, 2010; Watts et al., 2013). C. coli 33559 was used as a quality control strain.
Whole-genome sequencing
Genomic DNA of 120 C. coli isolates (30 from each year) was extracted using the Wizard Genomic DNA purification kit (Promega). The libraries were prepared using a TruSeq DNA PCR-free library preparation kit, and whole-genome sequencing was performed on an Illumina MiSeq platform (Shanghai Personal Biotechnology Co., Ltd., China) using a 350-bp paired-end library with ∼200-fold average coverage. The paired-end reads were assembled de novo using SOAPdenovo v2.04 and Gapcloser v1.12.
Antimicrobial resistance genes, virulence genes, and multilocus sequence typing analysis
All contigs were searched for antimicrobial resistance genes (ARGs) by ResFinder 3.21 (Florensa et al., 2022). Virulence genes (VGs) of Campylobacter isolates were obtained by comparing the assembled draft genome with the online website of the VFDB database2 (Chen et al., 2005). All the VGs were grouped according to the information from the VFDB. Heatmaps showing ARG co-occurrence and exclusivity and virulence genes were generated using the R package (ggplot2, default parameters). Multilocus sequence typing (MLST) was used to search for the sequences of seven selected Campylobacter housekeeping genes, aspA (aspartase A), glnA (glutamine synthase), gltA (citrate synthase), glyA (serine hydroxymethyltransferase), pgm (phosphoglucomutase), tkt (transketolase), and uncA (ATP synthase alpha subunit), using the online website MLST 2.03 (Larsen et al., 2012).
Phylogenetic analysis and pan-genomic analysis
A total of 225 genomes, including 120 of this study and 105 C. coli isolates of patient origin downloaded from the National Center for Biotechnology Information (NCBI) database, were used to generate a core-genome single nucleotide polymorphism (SNP) alignment and construct a phylogenetic tree using CSI Phylogeny 1.44 (Kaas et al., 2014). The phylogenetic tree was annotated in iTOL5 (Letunic and Bork, 2021). The fasta sequences of 120 strains of swine-derived Campylobacter coli were annotated by RAST6 to obtain genbank files (Aziz et al., 2008). Pan-genomic analysis of these genbank files was performed by GView Server7 (Petkau et al., 2010) using the default parameters: percent identity cutoff (90), alignment length cutoff (100), and e-values (<1e−10).
Statistical analysis
Statistical analysis was performed on the data using the software SPSS, two-sided test, and the data with P < 0.05 were considered significantly different.
Data availability
All data analyzed in this study are publicly available. The whole-genome sequencing data downloaded from NCBI are listed in Supplementary Table 1. The genomes of the 120 newly sequenced C. coli isolates were deposited in the NCBI database under accession number PRJNA817390.
Results
Prevalence of Campylobacter before and after antibiotic bans in fodder
A total of 471 Campylobacter isolates were collected during 2018–2021 from swine fecal samples or cecal contents from Sichuan Province. The overall prevalence rate of Campylobacter was 43.2% (471/1,090), and the prevalence rate varied among the samples derived from different years, with 43.1% (n = 144/334) in 2018, 39.6% (n = 149/376) in 2019, 44% (n = 88/200) in 2020, and 50% (n = 90/180) in 2021 (Figure 1A). Before the antibiotic prohibition, the overall prevalence rate of Campylobacter in swine feces was 41.3%, and after that, the overall prevalence rate of Campylobacter in swine feces had a slight increase and reached 46.8%. Of the Campylobacter isolates, 95.5% (450/471) were identified as C. coli, and 3.1% (15/471) were determined to be C. jejuni by PCR. The remaining six isolates were of different Campylobacter spp. than C. jejuni and C. coli and were not characterized further to the species level.
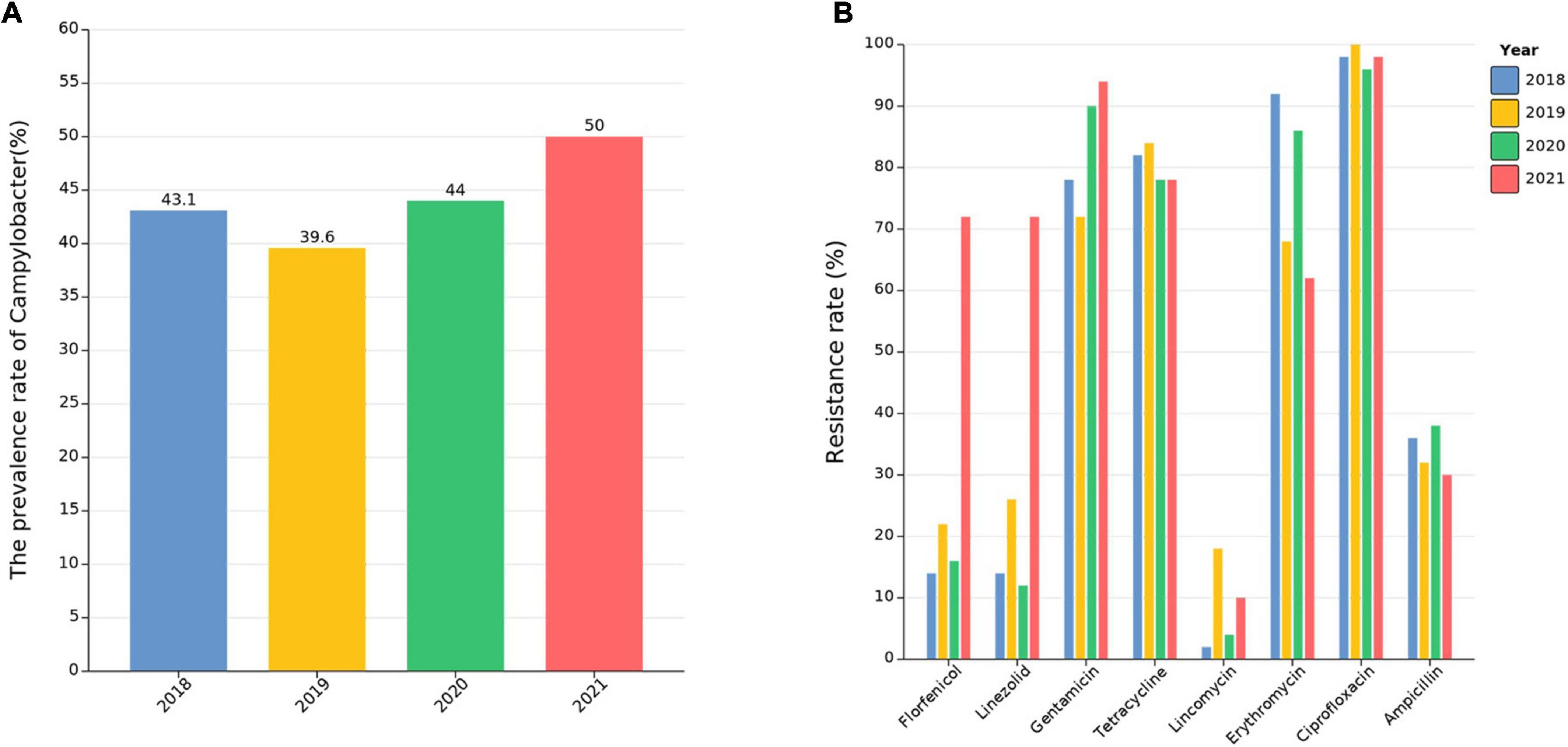
Figure 1. The prevalence and antibiotic resistance rate in Campylobacter isolates. (A) The prevalence rate of Campylobacter over 4 years. (B) Antibiotic resistance rates of isolated Campylobacter spp. as determined by antibiotic susceptibility testing.
Antimicrobial resistance patterns among Campylobacter isolates
Overall, Campylobacter showed high rates of susceptibility to meropenem and tigecycline (Supplementary Table 2), and erythromycin resistance levels decreased from 92% in 2018 to 62% in 2021. However, the levels of resistance to gentamicin and florfenicol increased significantly between 2018 and 2021; gentamicin resistance increased from 78% in 2018 and 72% in 2019 to 90% in 2020 and 94% in 2021, and the florfenicol resistance rate fluctuated between 14 and 22% from 2018 to 2020 but sharply increased to 72% in 2021. Tetracycline resistance rates remained at a high level and fluctuated between 78 and 84% throughout the study period, while ampicillin resistance remained fairly stable (between 30 and 38%). Both SAPs (Strains isolated after AGP prohibition) and SBPs (Strains isolated before AGP prohibition) showed high rates of resistance to ciprofloxacin (between 96 and 100%) (Figure 1B and Supplementary Table 2).
Antibiotic resistance genes of Campylobacter isolates
Based on the genomic analysis, we examined ARGs to tetracycline, aminoglycoside, β-lactam, phenicols, and macrolide, which are important antimicrobial agents in human and/or veterinary medicine. The identified tetracycline resistance genes in Campylobacter isolates included tet(O), tet(M), and tet(O/32/O). The prevalence of the tet(O) gene remained fairly stable from 2018 to 2021 (∼73.3%), but the average prevalence of the tet(O/32/O) gene decreased from 25% in 2018–2019 to 5% in 2020–2021, and the tet(M) increased from 0 to 13.3%; 86.7% of the isolates (n = 104) harbored at least one tet gene. A number of ARGs to aminoglycoside were identified, including aadE-Cc, aac(6′)-aph(2″), ant(6)-la, aph(3″)-lll, and aph(2″)-lf. Most notably, the prevalence of the aadE-Cc gene, which mediates resistance to gentamicin, rose rapidly since the ban of AGPs in feed, from 63.3% in 2018 to 90% in 2021. Although chloramphenicol was banned in food-producing animals in China, florfenicol, a fluorinated thiamphenicol derivative, is still in use. The phenicol resistance genes identified in the sequenced Campylobacter isolates included cat, fexA, cfr(C) and optrA. Notably, the prevalence of the optrA gene, which confers cross-resistance to florfenicol and linezolid, sharply increased from 3.3% in 2018 to 60% in 2021. The prevalence of the cfr(C) gene, mediating resistance to five structurally unrelated drugs, fluctuated between 3.3 and 16.7% during the study period. The prevalence of lnu(C) has increased over time, from 0% in 2018 and 13.3% in 2019 to 6.7% in 2020 and 23.3% in 2021. The identified β-lactam resistance genes included blaOXA–193, blaOXA–489, and blaOXA–61. The blaOXA–193 gene emerged in Campylobacter in 2021, and its percentage was 16.7%. Eighty percent of the isolates (n = 96) harbored at least one of these β-lactam resistance genes. Additionally, macrolide and fluoroquinolone resistance were mainly mediated by point mutations in 23S rRNA and gyrA, respectively. The prevalence of the 2075A > G mutation remained relatively high (60–86.7%), and all 120 sequenced strains contained the gyrA (T86I) mutation (Figure 2A and Supplementary Table 3). These data highly matched the results of antibiotic susceptibility testing.
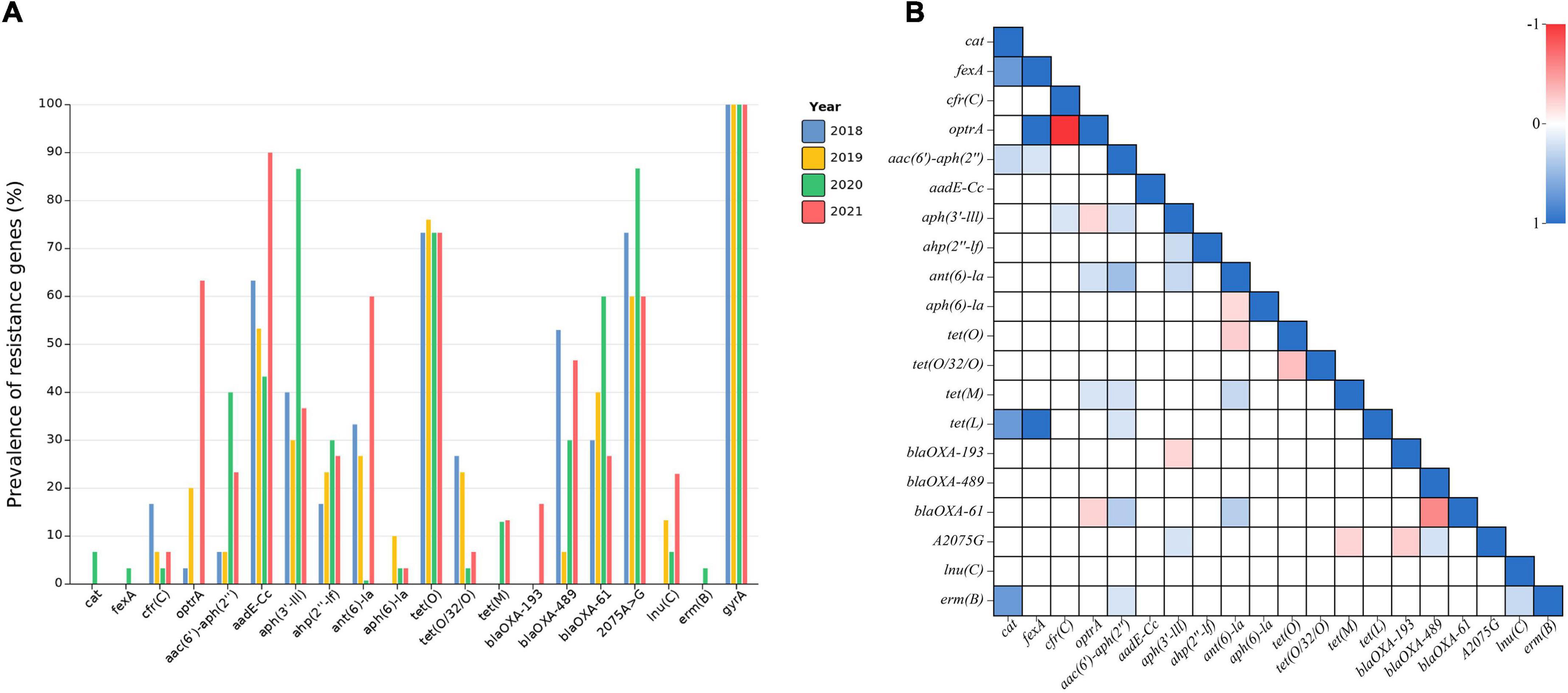
Figure 2. Antibiotic resistance genes in Campylobacter isolates. (A) The distribution of resistance genes in Campylobacter isolates. (B) Co-occurrence of ARGs in Campylobacter. Significant pairwise values from 1 (blue) to −1 (red) (that is, co-occurrence and mutual exclusivity) are shown.
In addition, several AGRs coexist frequently (Figure 2B), such as cat with tet(L) and erm(B), tet(L) with fexA, aac(6′)-aph(2″) with fexA, and cfr(C) with aph(3′-III). Some ARGs coexisted less in the same strain, such as optrA with aph(3′-III), tet(O) with aph(6)-la and tet(O/32/O), blaOXA–61 with blaOXA–489, and blaOXA–193 with aph(3′-III) (Figure 2B and Supplementary Table 4).
Multilocus sequence typing and phylogenetic analysis of Campylobacter coli isolates of swine and patient origin
Multilocus sequence typing assigned the 120 isolates to 34 distinct sequence types (STs), except for six isolates with novel STs (Supplementary Table 5). The most prevalent STs were ST854, ST828, ST1058, and ST902, with 25 (20.8%), 16 (13.3%), 15 (12.5%), and 10 (8.3%) isolates, respectively. The prevalence of ST1508 and ST828 increased since the ban of AGPs in 2020. Conversely, ST854 and ST1112 were more prevalent before the ban (Figure 3A). Although most isolates showed host specificity and were unique to the isolates’ origin, both MLST and core-genome single nucleotide polymorphism (SNP)-based phylogenetic analysis showed commonality between strains from swine and human, typified by genotypes ST828, ST854, ST825, ST1113, ST1464, ST1595, ST872, and ST1145 (Figure 3B; Litrup et al., 2007).
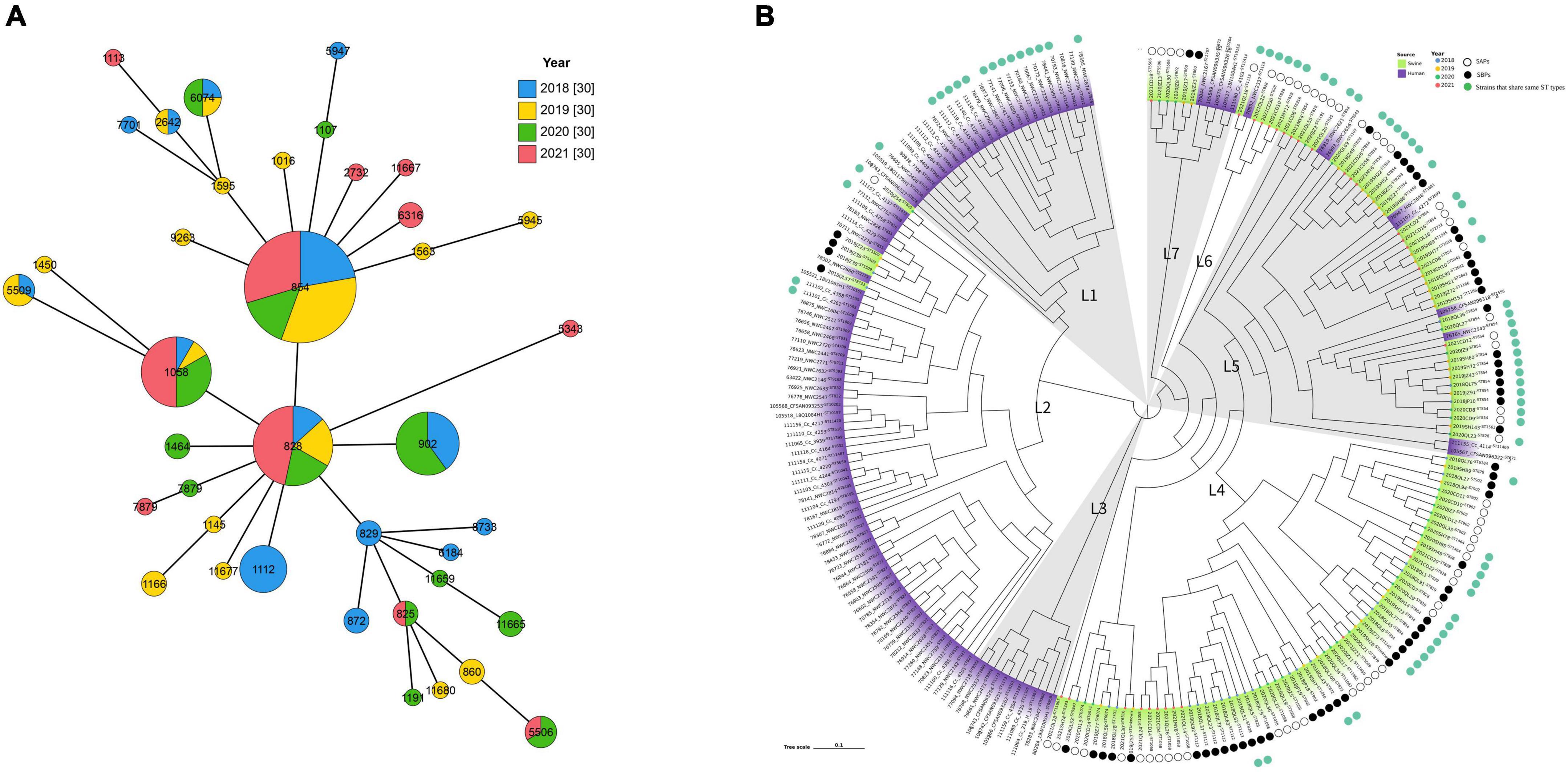
Figure 3. Phylogenetic analysis of Campylobacter isolates. (A) ST typing of 120 Campylobacter isolates over 4 years. Blue: 2018 (n = 30), Yellow: 2019 (n = 30), Green: 2020 (n = 30), and Pink: 2021 (n = 30). Numbers in circles represent ST-types. (B) SNP phylogenetic tree of 120 swine-derived Campylobacter isolates and 105 human-derived Campylobacter isolates from a public database. The superscript means ST type; Purple: human-derived; Bright green: swine-derived; Black solid dots: SBPs; white hollow dots: SAPs; blue solid dots: same MLST types shared by swine- and human-derived Campylobacter isolates.
Virulence gene profiles of Campylobacter isolates
Campylobacter virulence genes are of great significance for the colonization and adaptation of the strain to the host. A total of 100 virulence genes were clustered into 8 classes and 27 groups. No obvious trends or changes in virulence genes responsible for adherence, glycosylation system, motility and export apparatus, secretion system, or toxin were observed. However, the average number of virulence genes responsible for capsule biosynthesis and transport has increased over time (from 17 in 2018 to 26 in 2021). Additionally, LOS-related genes responsible for serum resistance and immune evasion increased significantly in Campylobacter isolates after banning the use of antibiotics as growth promoters (Figure 4A and Supplementary Table 6). These genes mediate the anti-phagocytosis of Campylobacter to the host and play an important role in intercellular adhesion and biofilm formation. Further analysis found that SAPs had a higher prevalence than SBPs in genes responsible for immune evasion and secretion systems (T4SS and other related genes). However, O-linked flagellar glycosylation-related genes and flagella-related genes, which are important genes that modify the glycosylation of flagellum and synthesize components of flagella (Song et al., 2015), were absent in some SAPs (Figure 4B).
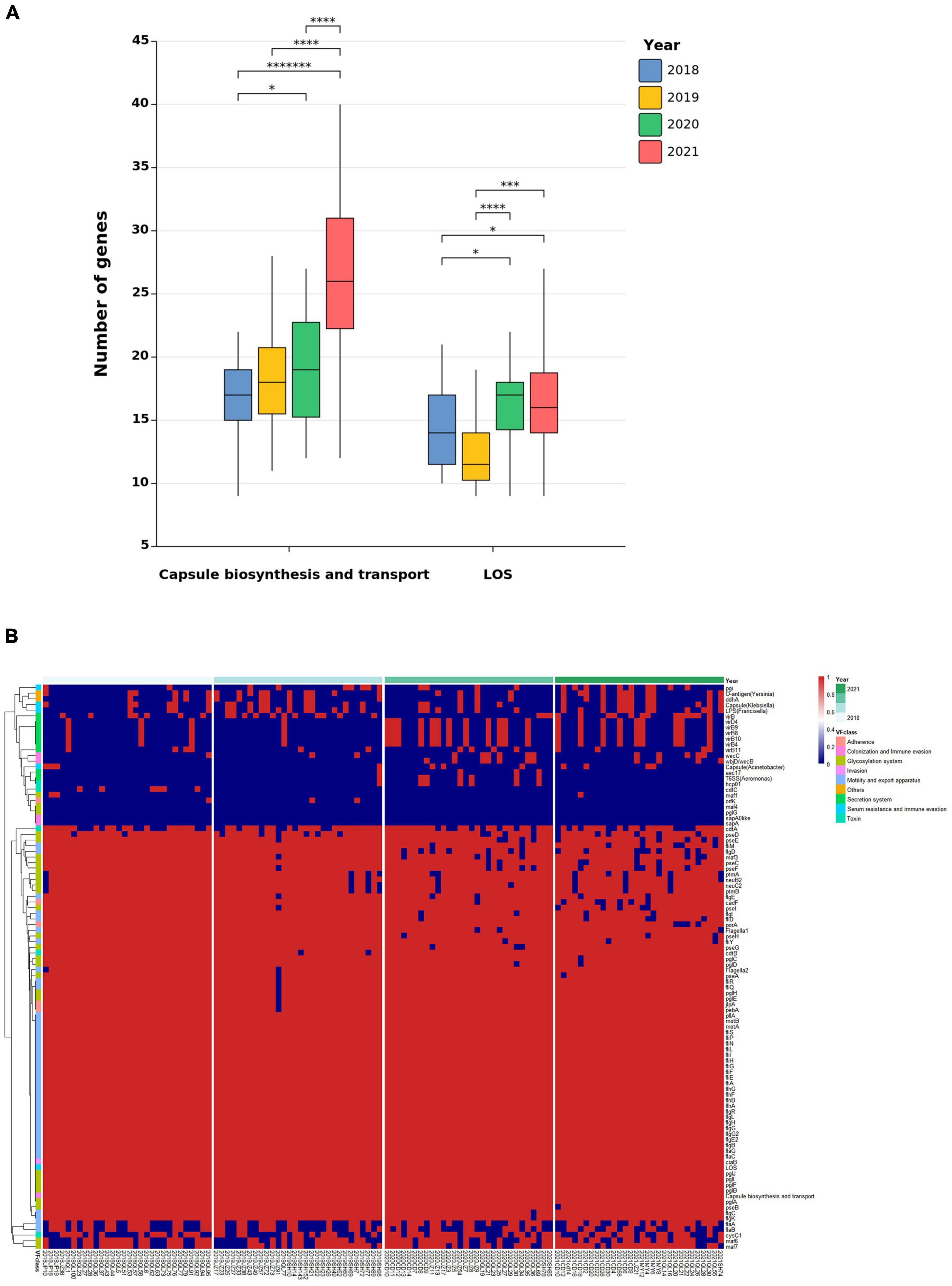
Figure 4. The distribution of virulence genes in Campylobacter isolates. (A) Predicted numbers of capsule- and LOS-related virulence genes in Campylobacter isolates (Blue: 2018, Yellow: 2019, Green: 2020, and Pink: 2021; the median number of genes is denoted as the horizontal line inside the boxplot. *p < 0.05; ***p < 0.0001; ****p < 0.00001, *******p < 0.0000001). (B) Heatmap of Campylobacter virulence genes isolated over 4 years (hierarchical clustering of Y-axis virulence genes, blue: absence, and red: presence).
Pan-genomic analysis
The results of the pan-genomic analysis are shown in Figure 5. A clear observation can be made from the figure that, the genomes of SAPs have more accessory genes than SBPs.
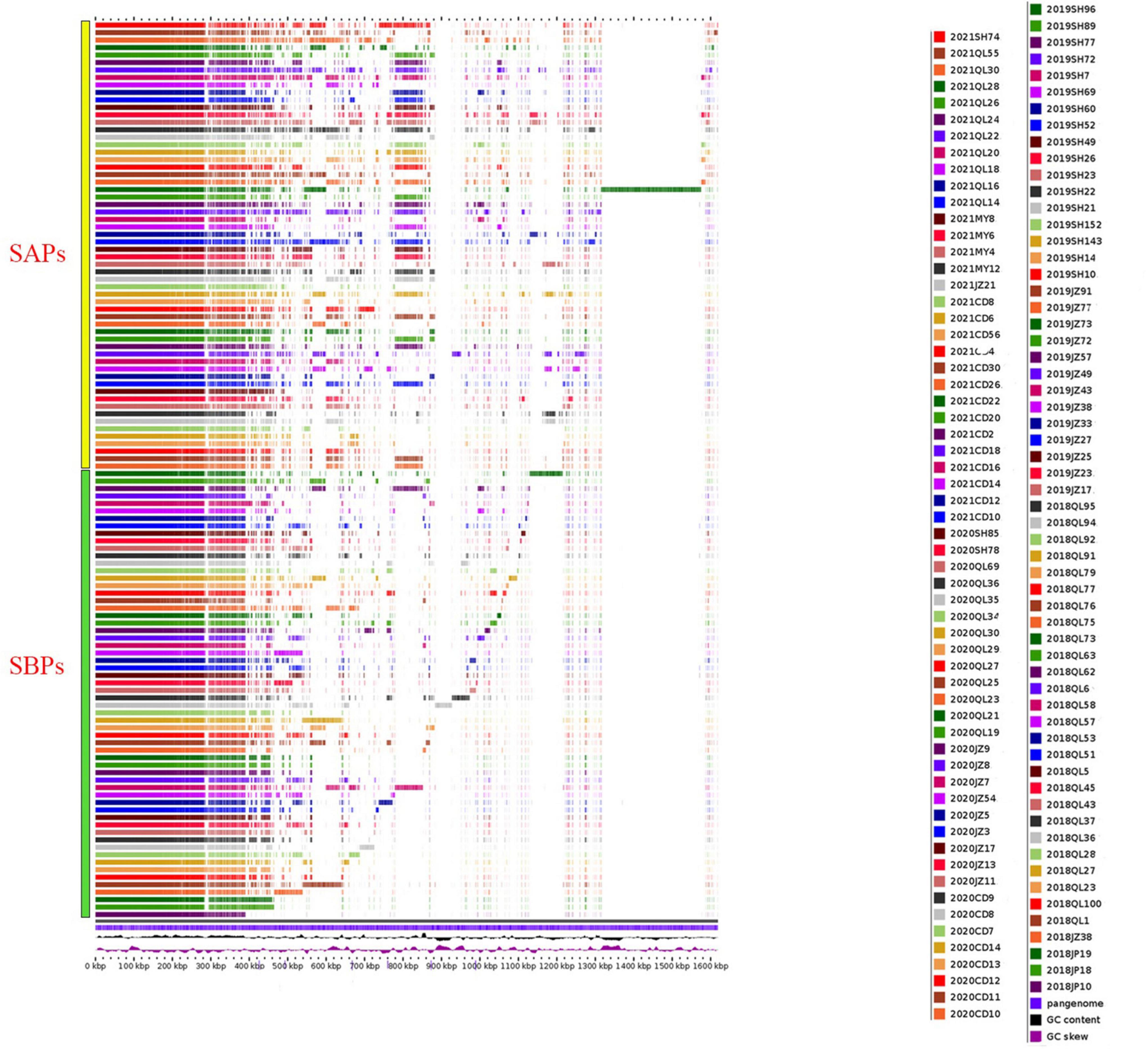
Figure 5. Pan-genomic analysis of 120 Campylobacter strains of porcine origin. Each row represents the sequence of one strain. Yellow: SAPs, green: SBPs. Pan-genome, GC content, and GC skew are indicated by blue-purple, black, and purple at the bottom.
Discussion
Our study explored the influence of withdrawing AGRs from swine diets on the prevalence and antibiotic resistance profile of Campylobacter in pigs. After the AGP ban, there was relatively little increase in the isolation rate of Campylobacter. However, the prevalence rate of ARGs mediating resistance to gentamycin and florfenicol, as well as the virulence genes responsible for capsule biosynthesis and transport, increased significantly. When comparing the MLST and genomic sequence, commonality exists between Campylobacter isolates of swine origin and patients’ origin, indicating potential transmission between swine and humans.
Antibiotic growth promotion in agricultural animal production has been practiced for approximately 50 years. The use of AGPs in feed not only significantly reduces the diversity of the animal’s gut microbiome (Antonopoulos et al., 2009) but also promotes bacterial genome evolution to combat antibiotic pressure (Davies and Davies, 2010). Banning AGPs frees gut microbes from antibiotic stress, and Campylobacter adapts to changes in its environment. Although the addition of antibiotics to feed has been eliminated, antibiotics in veterinary medicine, especially in the treatment of infectious diseases, are still in use. At present, the antibiotics commonly used for treatment in Chinese pig farms include: gentamicin, flupenthixol, tilmicosin, doxycycline, enrofloxacin, amoxicillin, amikacin, virginiamycin, etc. The observation that the dissemination of florfenicol resistance in Campylobacter dramatically increased after the withdrawal of AGPs might be attributable to the following: (I) although chloramphenicol has been banned, other antimicrobial agents within the same class, such as florfenicol, were widely used as therapeutic drugs in livestock, and provided selection pressure; (II) coselection by other antimicrobial agents might have contributed to the transmission of phenicol-related genes; for example, based on our previous study (Tang et al., 2020), the cfr(C) gene was located in a plasmid together with aph(3′-III) and tet(O). These genes can be coselected when exposed to any one of the corresponding antimicrobial agents; (III) the overuse of disinfectants can also promote the transmission of ARGs when they colocalize with disinfectant resistance genes. In recent years, due to outbreaks of infectious diseases, such as African swine fever, disinfectants have been widely used in swine farms and have placed selection pressure. The prevalence rate of antibiotic resistance genes such as tetracyclines, quinolones and nalidixic acid has always been maintained at a high level. However, it is noteworthy that we observed a decline in the resistance rate of the tested Campylobacter to erythromycin, and since macrolide antibiotics are clinically important for the treatment of Campylobacter infections, the decline in their resistance rate is a positive feedback for the prohibition of AGPs. It is an interesting phenomenon regarding the presence of tet(M) in Campylobacter. In the current reports, the resistance of Campylobacter to tetracycline is mainly mediated by the tet(O), tet(O/32/O), and CmeABC genes, and there are few reports in the literature on the presence of tet(M) genes in Campylobacter and mediating drug resistance (Taylor and Courvalin, 1988; Gibreel et al., 2007). Therefore, further experimental studies are needed to determine whether the tet(M) gene found in the Campylobacter tested mediates drug resistance. Quinolone resistance caused by mutation of the gryA gene exists in all sequenced isolates, indicating that quinolones are no longer against infections caused by Campylobacter in this fraction (Hormeño et al., 2016). Both cfr(C) and optrA mediate cross-resistance to florfenicol and linezolid. However, the phenomenon that the prevalence rate of cfr(C) shows a decreasing trend over time, while the prevalence of optrA sharply increases in 2021, needs further investigation. Overall, no significant decrease in the antibiotic resistance (except for erythromycin) in Campylobacter was observed after the prohibition of antibiotics as growth promoters. Conversely, some antibiotic resistance rates are rising.
Although diverse MLSTs were observed in Campylobacter isolates derived from both before and after the AGP ban, the prevalence of some ST types increased or decreased over time, probably because these strains acquire or lose resistance genes or virulence genes that make them better or no longer able to adapt to environmental stress. Both MLST and genomic data showed that some swine- and human-derived strains share high genetic similarity, suggesting that swine-derived Campylobacter may be transmitted to humans through pork products, which is in line with previous research (Gorkiewicz et al., 2002; Sheppard et al., 2009).
The capsule biosynthesis and transport system is responsible for bacterial survival and persistence in the environment and evasion of the host immune response (Bacon et al., 2001). LOS diversity is important for the ability to colonize a wide variety of hosts and intestinal niches (Guerry et al., 2002). Their average numbers in SAPs increased significantly, which may indicate that SAPs are stronger than SBPs in terms of persistence in the environment and invasion of organisms. Another obvious difference in the virulence genes before and after the ban of AGPs was that the abundance of secretion system (virB\D)-related genes in the SAP strains increased significantly. The isolates in 2018 and 2019 had fewer virB\D genes, but the genomes of most SAPs contain T4SS-related genes. The basic secretion system function in Campylobacter is achieved by flagella (Guerry, 2007); however, some Campylobacter acquires type IV (T4SS) or type VI (T6SS) secretion systems. Type IV secretion systems are membrane-associated transporter complexes used by Gram-negative and Gram-positive bacteria to deliver substrate molecules to various target cells in a contact-dependent manner (Alvarez-Martinez and Christie, 2009). T4SS contributes to pathogenesis in multiple ways, including increased genomic plasticity, spread of antibiotic resistance, enhanced surface colonization and biofilm formation, and specific injection of virulence proteins (Kienesberger et al., 2011). The increase in the abundance of the type IV secretion system after the banning of APGs indicated that the virulence of SAPs may be stronger than that of SBPs. The type IV secretion system also mediates the horizontal transfer of bacterial genes, and strains with T4SS have more possibilities for gene export and acquisition (Cascales and Christie, 2003). This is important for the acquisition and dissemination of genes detrimental to humans by the Campylobacter genome. Interestingly, we also observed that more O-linked flagellar glycosylation-related genes (pse) and Flagella-related genes were absent in SAPs than in SBPs. O-linked flagellar glycosylation and Flagella-related genes play important roles in the function of flagella (Song et al., 2015). Therefore, its absence in turn leads to impaired flagellar function.
Similar to the results of virulence gene prediction, the results of pan-genomic analysis showed that Campylobacter strains possess more accessory genes and their genomes are more diverse after the prohibition of AGPs. Combining the trends in virulence genes with the results of the pangenome analysis, we suggest that the possible reason for this is that the prohibition of AGPs produces drastic changes in the environment in which Campylobacter lives. The use of AGPs inhibits competition between bacteria by suppressing the growth and reproduction of intestinal bacteria (Lee et al., 2017; Tang et al., 2017). This inhibitory effect is long-term and persistent because AGPs are mixed with the feed. Once AGPs are banned, this inhibitory effect disappears and competition between bacteria for ecological niches increases. The use of therapeutic antibiotics also inhibits the growth of colonies, but its effect is short-term because farms do not generally use large amounts of therapeutic antibiotics continuously. After the ban of AGPs, the fierce competition among colonies will force Campylobacter to evolve to adapt to the new survival environment. These evolutions can be characterized by changes in the genome, as in this study with the increase of virulence genes as well as other accessory genes.
Conclusion
This study represents a systematic investigation of the impact of AGP withdrawal on the foodborne pathogen Campylobacter. Samples were collected for four consecutive years, including 2 years before the AGP ban and 2 years after that. We found that the isolation rate of Campylobacter slightly increased after the AGP ban, but the florfenicol- and gentamicin-related ARGs increased significantly. Additionally, the number of virulence genes responsible for capsule biosynthesis and the transport system, serum resistance and immune evasion also significantly increased. And the accessory genome was also more enriched. Genomic alignment showed that swine- and human-derived isolates share the same ST types. These data suggested that after antibiotic growth promoters are banned, pathogenic infections may increase, leading to increased use of therapeutic antibiotics on farms and thus to increased rates of antibiotic resistance in Campylobacter.
Data availability statement
The datasets presented in this study can be found in online repositories. The names of the repository/repositories and accession number(s) can be found below: https://www.ncbi.nlm.nih.gov/, PRJNA817390.
Author contributions
RW: conceptualization, methodology, software, formal analysis, and writing—original draft preparation. CL: methodology and writing—original draft preparation. MZ: data curation. HW and YT: conceptualization, methodology, and funding acquisition. All authors contributed to the article and approved the submitted version.
Funding
This work was supported by the Sichuan Science and Technology Programs (2021ZDZX0010), the National Natural Science Foundation of China (U21A20257), and the Fundamental Research Funds for the Central Universities (2020SCUNL206).
Conflict of interest
The authors declare that the research was conducted in the absence of any commercial or financial relationships that could be construed as a potential conflict of interest.
Publisher’s note
All claims expressed in this article are solely those of the authors and do not necessarily represent those of their affiliated organizations, or those of the publisher, the editors and the reviewers. Any product that may be evaluated in this article, or claim that may be made by its manufacturer, is not guaranteed or endorsed by the publisher.
Supplementary material
The Supplementary Material for this article can be found online at: https://www.frontiersin.org/articles/10.3389/fmicb.2022.1004725/full#supplementary-material
Footnotes
- ^ http://cge.cbs.dtu.dk/services/ResFinder/
- ^ http://www.mgc.ac.cn/VFs
- ^ http://cge.cbs.dtu.dk/services/MLST/
- ^ http://cge.cbs.dtu.dk/services/CSIPhylogeny/
- ^ https://itol.embl.de/
- ^ https://rast.nmpdr.org
- ^ https://server.gview.ca
References
Alvarez-Martinez, C. E., and Christie, P. J. (2009). Biological diversity of prokaryotic type IV secretion systems. Microbiol. Mole. Biol. Rev. 73, 775–808.
Antonopoulos, D. A., Huse, S. M., Morrison, H. G., Schmidt, T. M., Sogin, M. L., and Young, V. B. (2009). Reproducible community dynamics of the gastrointestinal microbiota following antibiotic perturbation. Infect. Immun. 77, 2367–2375. doi: 10.1128/IAI.01520-08
Aziz, R. K., Bartels, D., Best, A. A., DeJongh, M., Disz, T., Edwards, R. A., et al. (2008). The RAST Server: rapid annotations using subsystems technology. BMC Genom. 9:75. doi: 10.1186/1471-2164-9-75
Bacon, D. J., Szymanski, C. M., Burr, D. H., Silver, R. P., Alm, R. A., and Guerry, P. (2001). A phase-variable capsule is involved in virulence of Campylobacter jejuni 81-176. Mole. Microbiol. 40, 769–777.
Bush, K., Courvalin, P., Dantas, G., Davies, J., Eisenstein, B., Huovinen, P., et al. (2011). Tackling antibiotic resistance. Nat. Rev. Microbiol. 9, 894–896.
Cascales, E., and Christie, P. J. (2003). The versatile bacterial type IV secretion systems. Nat. Rev. Microbiol. 1, 137–149.
Chen, L., Yang, J., Yu, J., Yao, Z., Sun, L., Shen, Y., et al. (2005). VFDB: a reference database for bacterial virulence factors. Nucl. Acids Res. 33, D325–D328.
Clinical and Laboratory Standards Institute (2010). Performance standards for antimicrobial susceptibility testing. Wayne, PA: CLSI.
Davies, J., and Davies, D. (2010). Origins and evolution of antibiotic resistance. MMBR 74, 417–433.
Dibner, J. J., and Richards, J. D. (2005). Antibiotic growth promoters in agriculture: history and mode of action. Poult. Sci. 84, 634–643.
Elliott, S. D., and Barnes, E. M. (1959). Changes in serological type and antibiotic resistance of Lancefield group D streptococci in chickens receiving dietary chlortetracycline. J. Gen. Microbiol. 20, 426–433. doi: 10.1099/00221287-20-2-426
Florensa, A. F., Kaas, R. S., Clausen, P. T. L. C., Aytan-Aktug, D., and Aarestrup, F. M. (2022). ResFinder - an open online resource for identification of antimicrobial resistance genes in next-generation sequencing data and prediction of phenotypes from genotypes. Microbial. Genom. 8:000748. doi: 10.1099/mgen.0.000748
Gaytán, M. O., Martínez-Santos, V. I., Soto, E., and González-Pedrajo, B. (2016). Type Three Secretion System in Attaching and Effacing Pathogens. Front. Cell. Infect. Microbiol. 6:129. doi: 10.3389/fcimb.2016.00129
Gibreel, A., Wetsch, N. M., and Taylor, D. E. (2007). Contribution of the CmeABC efflux pump to macrolide and tetracycline resistance in Campylobacter jejuni. Antimicrob. Agents Chemother. 51, 3212–3216. doi: 10.1128/AAC.01592-06
Gorkiewicz, G., Feierl, G., Zechner, R., and Zechner, E. L. (2002). Transmission of Campylobacter hyointestinalis from a pig to a human. J. Clin. Microbiol. 40, 2601–2605.
Guerry, P. (2007). Campylobacter flagella: not just for motility. Trends Microbiol. 15, 456–461. doi: 10.1016/j.tim.2007.09.006
Guerry, P., Ewing, C. P., Hickey, T. E., Prendergast, M. M., and Moran, A. P. (2000). Sialylation of lipooligosaccharide cores affects immunogenicity and serum resistance of Campylobacter jejuni. Infect. Immun. 68, 6656–6662. doi: 10.1128/IAI.68.12.6656-6662.2000
Guerry, P., Szymanski, C. M., Prendergast, M. M., Hickey, T. E., Ewing, C. P., Pattarini, D. L., et al. (2002). Phase variation of Campylobacter jejuni 81-176 lipooligosaccharide affects ganglioside mimicry and invasiveness in vitro. Infect. Immun. 70, 787–793.
Hormeño, L., Palomo, G., Ugarte-Ruiz, M., Porrero, M. C., Borge, C., Vadillo, S., et al. (2016). Identification of the main quinolone resistance determinant in Campylobacter jejuni and Campylobacter coli by MAMA-DEG PCR. Diagn. Microbiol. Infect. Dis. 84, 236–239. doi: 10.1016/j.diagmicrobio.2015.11.002
Kaakoush, N. O., Castano-Rodriguez, N., Mitchell, H. M., and Man, S. M. (2015). Global Epidemiology of Campylobacter Infection. Clin. Microbiol. Rev. 28, 687–720.
Kaas, R. S., Leekitcharoenphon, P., Aarestrup, F. M., and Lund, O. (2014). Solving the problem of comparing whole bacterial genomes across different sequencing platforms. PLoS One 9:e104984. doi: 10.1371/journal.pone.0104984
Karlyshev, A. V., Ketley, J. M., and Wren, B. W. (2005). The Campylobacter jejuni glycome. FEMS Microbiol. Rev. 29, 377–390.
Kienesberger, S., Schober Trummler, C., Fauster, A., Lang, S., Sprenger, H., Gorkiewicz, G., et al. (2011). Interbacterial macromolecular transfer by the Campylobacter fetus subsp. venerealis type IV secretion system. J. Bacteriol. 193, 744–758. doi: 10.1128/JB.00798-10
Larsen, M. V., Cosentino, S., Rasmussen, S., Friis, C., Hasman, H., Marvig, R. L., et al. (2012). Multilocus sequence typing of total-genome-sequenced bacteria. J. Clin. Microbiol. 50, 1355–1361. doi: 10.1128/JCM.06094-11
Lee, J.-Y., Han, G. G., Lee, H.-B., Lee, S.-M., Kang, S.-K., Jin, G.-D., et al. (2017). Prohibition of antibiotic growth promoters has affected the genomic profiles of Lactobacillus salivarius inhabiting the swine intestine. PLoS one 12:e0186671. doi: 10.1371/journal.pone.0186671
Letunic, I., and Bork, P. (2021). Interactive Tree Of Life (iTOL) v5: an online tool for phylogenetic tree display and annotation. Nucleic Acids Res. 49, W293–W296. doi: 10.1093/nar/gkab301
Lin, J., Michel, L. O., and Zhang, Q. (2002). CmeABC functions as a multidrug efflux system in Campylobacter jejuni. Antimicrob. Agents Chemother. 46, 2124–2131. doi: 10.1128/AAC.46.7.2124-2131.2002
Linton, D., Lawson, A. J., Owen, R. J., and Stanley, J. (1997). PCR detection, identification to species level, and fingerprinting of Campylobacter jejuni and Campylobacter coli direct from diarrheic samples. J. Clin. Microbiol. 35, 2568–2572. doi: 10.1128/jcm.35.10.2568-2572.1997
Linton, D., Owen, R. J., and Stanley, J. (1996). Rapid identification by PCR of the genus Campylobacter and of five Campylobacter species enteropathogenic for man and animals. Res. Microbiol. 147, 707–718. doi: 10.1016/s0923-2508(97)85118-2
Litrup, E., Torpdahl, M., and Nielsen, E. M. (2007). Multilocus sequence typing performed on Campylobacter coli isolates from humans, broilers, pigs and cattle originating in Denmark. J. Appl. Microbiol. 103, 210–218. doi: 10.1111/j.1365-2672.2006.03214.x
Maron, D. F., Smith, T. J., and Nachman, K. E. (2013). Restrictions on antimicrobial use in food animal production: an international regulatory and economic survey. Glob. Health 9:48. doi: 10.1186/1744-8603-9-48
Petkau, A., Stuart-Edwards, M., Stothard, P., and Van Domselaar, G. (2010). Interactive microbial genome visualization with GView. Bioinformatics 26, 3125–3126. doi: 10.1093/bioinformatics/btq588
Richou, R. (1952). [The use of antibiotics in veterinary medicine especially in the treatment of infectious diseases]. Rev. Pathol. Comp. 52, 309–311.
Rivera-Amill, V., Kim, B. J., Seshu, J., and Konkel, M. E. (2001). Secretion of the virulence-associated Campylobacter invasion antigens from Campylobacter jejuni requires a stimulatory signal. J. Infect. Dis. 183, 1607–1616. doi: 10.1086/320704
Salim, H. M., Huque, K. S., Kamaruddin, K. M., and Beg, M. (2018). Global restriction of using antibiotic growth promoters and alternative strategies in poultry production. Sci. Prog. 101, 52–75.
Sheppard, S. K., Dallas, J. F., Strachan, N. J. C., MacRae, M., and McCarthy, N. D. (2009). Campylobacter genotyping to determine the source of human infection. Clin. Infect. Dis. 48, 1072–1078. doi: 10.1086/597402
Song, W. S., Nam, M. S., Namgung, B., and Yoon, S.-I. (2015). Structural analysis of PseH, the Campylobacter jejuni N-acetyltransferase involved in bacterial O-linked glycosylation. Biochem. Biophys. Res. Commun. 458, 843–848. doi: 10.1016/j.bbrc.2015.02.041
Tang, Y., Fang, L., Xu, C., and Zhang, Q. (2017). Antibiotic resistance trends and mechanisms in the foodborne pathogen, Campylobacter. Anim. Health Res. Rev. 18, 87–98. doi: 10.1017/S1466252317000135
Tang, Y., Lai, Y., Wang, X., Lei, C., Li, C., Kong, L., et al. (2020). Novel insertion sequence ISChh1-like mediating acquisition of optrA gene in foodborne pathogen Campylobacter coli of swine origin. Vet. Microbiol. 252:108934. doi: 10.1016/j.vetmic.2020.108934
Taylor, D. E., and Courvalin, P. (1988). Mechanisms of antibiotic resistance in Campylobacter species. Antimicrob. Agents Chemother. 32, 1107–1112.
van Vliet, A. H., Baillon, M. L., Penn, C. W., and Ketley, J. M. (1999). Campylobacter jejuni contains two fur homologs: characterization of iron-responsive regulation of peroxide stress defense genes by the PerR repressor. J. Bacteriol. 181, 6371–6376. doi: 10.1128/JB.181.20.6371-6376.1999
Wang, Y., Xu, C., Zhang, R., Chen, Y., Shen, Y., Hu, F., et al. (2020). Changes in colistin resistance and mcr-1 abundance in Escherichia coli of animal and human origins following the ban of colistin-positive additives in China: an epidemiological comparative study. Lancet Infect. Dis. 20, 1161–1171. doi: 10.1016/S1473-3099(20)30149-3
Wang, Y., Zhang, M., Deng, F., Shen, Z., Wu, C., Zhang, J., et al. (2014). Emergence of multidrug-resistant Campylobacter species isolates with a horizontally acquired rRNA methylase. Antimicrob. Agents Chemother. 58, 5405–5412. doi: 10.1128/AAC.03039-14
Wang, Y., Zhang, R., Li, J., Wu, Z., Yin, W., Schwarz, S., et al. (2017). Comprehensive resistome analysis reveals the prevalence of NDM and MCR-1 in Chinese poultry production. Nat. Microbiol. 2:16260. doi: 10.1038/nmicrobiol.2016.260
Watts, J. L., Shryock, T. R., Apley, M., Bade, D. L., Brown, S. D., Gray, J. T., et al. (2013). Performance Standards for Antimicrobial disk and dilution susceptibility test for bacteria isolated from animals; Approved standard. Wayne, PA: CLSI.
Keywords: Campylobacter, antibiotic growth promoters, swine, antibiotic resistance, virulence factors (VFS)
Citation: Wen R, Li C, Zhao M, Wang H and Tang Y (2022) Withdrawal of antibiotic growth promoters in China and its impact on the foodborne pathogen Campylobacter coli of swine origin. Front. Microbiol. 13:1004725. doi: 10.3389/fmicb.2022.1004725
Received: 27 July 2022; Accepted: 22 August 2022;
Published: 08 September 2022.
Edited by:
Shaolin Wang, China Agricultural University, ChinaReviewed by:
Shangshang Qin, Zhengzhou University, ChinaXiaoming Wang, Nanjing Agricultural University, China
Copyright © 2022 Wen, Li, Zhao, Wang and Tang. This is an open-access article distributed under the terms of the Creative Commons Attribution License (CC BY). The use, distribution or reproduction in other forums is permitted, provided the original author(s) and the copyright owner(s) are credited and that the original publication in this journal is cited, in accordance with accepted academic practice. No use, distribution or reproduction is permitted which does not comply with these terms.
*Correspondence: Hongning Wang, d2hvbmduaW5nQDE2My5jb20=; Yizhi Tang, dGFuZ3lpemhpMjNAMTYzLmNvbQ==