- 1Centre for Research in Bioscience, College of Health, Science and Society, University of the West of England, Bristol, United Kingdom
- 2Centre for Ecosystems, Society and Biosecurity, Forest Research, Farnham, United Kingdom
- 3Harper Adams University, Newport, United Kingdom
Following a screening campaign of bleeding cankers of broadleaf hosts in Great Britain, numerous bacterial strains were isolated, identified by 16S rRNA and protein-coding gene sequencing and ultimately classified. During the course of the study, several Gram-negative, facultatively anaerobic strains were isolated from bleeding Platanus x acerifolia (London plane) and Tilia x europaea (common lime) cankers that could not be assigned to an existing species. Partial 16S rRNA gene sequencing placed these strains in the genus Erwinia, as a close phylogenetic relative of Erwinia toletana. In an effort to determine the taxonomic position of the strains, a polyphasic approach was followed including genotypic, genomic, phenotypic, and chemotaxonomic assays. Multilocus sequence analysis based on four protein-coding genes (gyrB, rpoB, infB, and atpD) confirmed the phylogenetic position of the strains as a novel taxon of subgroup 3 of the genus Erwinia, along with E. toletana and E. iniecta, and furthermore, provided support for their reclassification in a novel genus. Whole genome comparisons allowed the delimitation of the novel species and also supported the proposed transfer of subgroup 3 species to a novel genus in the Erwiniaeae. Phenotypically the novel species could be differentiated from E. toletana and E. iniecta, and the novel genus could be differentiated from the closely related genera Erwinia and Mixta. Therefore, we propose (1) the reclassification of E. toletana and E. iniecta in a novel genus, Winslowiella gen. nov., as Winslowiella toletana comb. nov. and Winslowiella iniecta comb. nov., with W. toletana comb. nov. as the type species (type strain A37T = CFBP 6631T = ATCC 700880T = CECT 5263T), and (2) classification of the novel strains as Winslowiella arboricola sp. nov. (type strain BAC 15a-03bT = LMG 32576T = NCPPB 4696T).
Introduction
The genus Erwinia has long been associated with plants and disease as either epiphytes, saprophytes, or pathogens (Hauben and Swings, 2015). Originally described in 1920 to unite the Gram-negative, fermentative, non-sporulating, peritrichously flagellated phytopathogenic bacteria, Erwinia is perhaps one of the oldest known plant-associated genera in the order Enterobacterales (Winslow et al., 1920). Indeed, numerous species in the genera Brenneria, Enterobacter, Lonsdalea, Pantoea, and Pectobacterium resided within Erwinia at some point in their taxonomic history. The genus currently comprises 21 species, including the important plant pathogens Erwinia amylovora, Erwinia piriflorinigrans, Erwinia psidii, Erwinia pyrifoliae, and Erwinia uzenensis as well the epiphytes Erwinia billingiae and Erwinia tasmaniensis (Parte et al., 2020). Erwinia toletana is considered an endophyte but this species plays an important role in olive knot formation through cooperation with the pathogen Pseudomonas savastanoi pv. savastanoi (Buonaurio et al., 2015).
Diseases typically caused by Erwinia species include fireblight of plants belonging to the family Rosaceae, particularly fruit trees (Vanneste, 2008); necrosis of pear blossom (López et al., 2011); dieback of Psidium guajava (Rodrigues Neto et al., 1987) and Carica papaya (Maktar et al., 2008); wilt and dieback of Eucalyptus species (Coutinho et al., 2011); and necrosis (Kim et al., 1996) and bacterial black shoot of pear trees (Matsuura et al., 2012). Apart from the diseases caused on fruit trees, there are few reports of Erwinia species isolated from woody hosts, specifically from broadleaf hosts.
As part of an ongoing survey of bleeding cankers and wetwood of broadleaf hosts in Great Britain,1 Tilia x europaea (common lime), Platanus x acerifolia (London plane), Fagus sylvatica (common beech), Betula spp. (birch), and Populus spp. (poplar) exhibiting symptoms were screened for the presence of bacterial pathogens. Following the isolation and identification of bacteria from bleeding cankers of these hosts, several strains from London plane and common lime could not be assigned to an existing species. Partial 16S rRNA gene sequencing of the strains demonstrated ∼99% similarity to E. toletana, but phylogenetically they appeared to belong to a novel taxon. A recent study examining the phylogenomic relationships between species of the family Erwiniaceae, identified three subgroups of species in the genus Erwinia (Soutar and Stavrinides, 2022). Subgroup 3 contained E. toletana and Erwinia iniecta and it was suggested that these species should be assigned to a novel genus. A second study demonstrated the close relationship between “Pantoea beijingensis,” E. toletana and E. iniecta and concluded that “P. beijingensis” should be renamed as “Erwinia beijingensis” (Xu et al., 2021). However, these suggestions were not formally proposed and implemented. A polyphasic approach was followed to determine the taxonomic position of the bleeding canker strains as a novel species within a novel genus, for which the name Winslowiella arboricola sp. nov. is proposed, as well as the reclassification of E. toletana and E. iniecta as Winslowiella toletana comb. nov. and Winslowiella iniecta comb. nov.
Materials and methods
Isolation and ecology
Bacterial strains were isolated from the bleeding cankers of Platanus x acerifolia (London plane) at St James Park, London and Tilia x europaea (common lime) at Tidworth Garrison, Wiltshire. Swab samples of the weeping exudate were collected from the London plane in May and October, 2020. A small bark panel (3 × 3 cm) was taken from the bleeding common lime in August 2019.
Swabs were rehydrated in sterile 1/4 Ringers, the swab spread on Luria-Bertani (LB) agar (Oxoid) and Eosin Methylene Blue (EMB) agar (Merck) which were initially incubated at 35°C under anaerobic conditions for 4 days to promote growth of facultatively anaerobic bacteria. Bark tissue was removed from the panel with a scalpel to expose the developing lesion. Wood chips were cut around the advancing lesion front, plated on LB agar and incubated at 25°C for 7 days. Single colonies were obtained through re-streaking on LB agar and incubation at 25°C.
The strains used in this study are listed in Supplementary Table 1.
Genotypic characterisation
Genomic DNA for genotypic characterisation was extracted using an alkali lysis method (Niemann et al., 1997) and stored at −20°C. Strains were initially screened and tentatively identified based on partial 16S rRNA gene sequences (using primer *pD), and the almost complete 16S rRNA gene sequence determined for the proposed type strain using the primers published by Coenye et al. (1999). Standard PCR conditions and cycles were used with an annealing temperature of 55°C. Multilocus sequence analysis (MLSA) based on partial sequences of four housekeeping genes (gyrB, rpoB, infB, and atpD) was performed on the strains tentatively identified as Erwinia sp. by partial 16S rRNA gene sequencing. MLSA PCR conditions, cycles and primers were applied as previously described (Brady et al., 2008), although with an updated version of the gyrB amplification primers: gyrB 01.2F (5′ TAARTT YGAYGAYAACTCBTAYAAACT 3′) and gyrB 02.2R (5′ CMCCYTCCACHARGTASAKTTC 3′). Consensus sequences for the 16S rRNA and housekeeping genes were generated in UGENE v38.1 (Okonechnikov et al., 2012) and aligned and trimmed in MEGA X (Kumar et al., 2018) to the following lengths: 16S rRNA—1346 bp, gyrB—742, rpoB—637, infB—615 bp, and atpD—642 bp. Maximum likelihood phylogenetic tree construction was performed on both datasets in PhyML 3.0 (Guindon et al., 2010) with 1,000 bootstrap replicates and automatic model selection by Smart Model Selection (Lefort et al., 2017).
To examine the genetic diversity between strains of the potential novel species, BOX PCR was performed using the primer and conditions previously published (Versalovic et al., 1994). Amplification products were separated on 1.5% agarose at 50 V for ∼3 h.
Genome features
The whole genomes of strains BAC 15a-03bT and Til 1, isolated from London plane and common lime respectively, were sequenced by MicrobesNG (Birmingham, UK). DNA was extracted by enzymatic cell lysis and purified with SPRI (Solid Phase Reversible Immobilisation) beads, before sequencing using the Illumina HiSeq platform. Adapters were trimmed from reads using Trimmomatic 0.30 with a sliding window quality cut-off of Q15 (Bolger et al., 2014), while de novo assembly was performed using SPAdes version 3.11.1 (Bankevich et al., 2012) and the resulting contigs annotated in the prokaryotic genome annotation pipeline (PGAP) (Tatusova et al., 2016). Average nucleotide identity (ANI) and average amino acid identity (AAI) were performed on the novel strains, along with validly published Erwinia species and “E. beijingensis,” using FastANI (Jain et al., 2018) and the AAI-matrix calculator from the Kostas Lab (Rodriguez-R and Konstantinidis, 2016), respectively. Additionally, pairwise percentage of conserved proteins (POCP) were calculated using the script pocp.rb (Hoelzer, 2020) which follows the approach by Qin et al. (2014).
Pairwise phylogenomic comparisons, between the novel species and current closest relatives from the genera Erwinia and Mixta, were carried out on the Type (Strain) Genome Server (TYGS) (Meier-Kolthoff and Göker, 2019) using Genome Blast Distance Phylogeny (GBDP). Accurate intergenomic distances were inferred under the algorithm “trimming” and distance formula d5 (Meier-Kolthoff et al., 2013), with 100 distance replicates each. The resulting intergenomic distances were used to infer a balanced minimum evolution tree with branch support via FASTME 2.1.6.1 including SPR postprocessing (Lefort et al., 2015) and 100 pseudo-bootstrap replicates. The tree was rooted at the midpoint (Farris, 1972).
Phenotypic and chemotaxonomic characterisation
Colony morphology was observed following growth of strains on LB agar incubated at 30°C for 48 h. The temperature range for growth was determined on LB agar incubated at 4, 10, 25, 30, 37, and 41°C. Tolerance of the novel species to salt was tested in saline-free nutrient broth with NaCl added in increments of 1% w/v from 1 to 10%, while pH tolerance was tested in LB broth with the pH adjusted from 4 to 10 in increments of 1 as previously described (Brady et al., 2022). Catalase and oxidase activity were determined by bubble production in 3% v/v H2O2 and by staining with Kovács reagent (1% tetra-methyl-p-phenylenediamine dihydrochloride), respectively. Cell size, morphology and motility were examined using a light microscope and the CellSens software v 1.11 (Olympus Life Science, Tokyo, Japan) following growth in LB broth at 25°C for ∼6 h. The flagella arrangement was visualised by transmission electron microscopy (FEI Tecnai 12 120kV BioTwin Spirit TEM) following negative staining as previously published (Brady et al., 2022).
Commercial phenotypic assays, including API 20E, API 50 CHB/E (bioMérieux), and GEN III GN/GP microplates (Biolog), were performed on strains BAC 15a-03bT, BAC 1-01-01, Til 1, and Til 5 according to the manufacturer’s instructions. The type strain of E. toletana LMG 24162T was included as a positive control. The API 20E galleries were scored after 24 h incubation at 37°C, while the API 50 CHB/E galleries and GEN III microplates were scored after 24 h incubation at 30°C, and again after 48 h. The fatty acid methyl ester (FAME) profile of the novel species was determined by Fera Science Ltd. (York, UK). Following the protocol based on the Sherlock Microbial Identification System Version 6.4 (MIDI Inc.), strains were cultivated on TSA at 28°C for 24 h prior to fatty acid extraction. The results obtained were compared against the library RTSBA6 6.21.
Virulence gene identification
The protein annotations generated through PGAP for the whole genome sequences of BAC 15a-03bT, Til 1, E. toletana DAPP-PG 735, E. iniecta B120T, and “E. beijingensis” JZB2120001T were queried through two databases to assess the pathogenic potential of their proteome. DIAMOND version v2.0.11.149 (Buchfink et al., 2021) was used to query genomes with the BlastP command against the Virulence Factor Database (VFDB) (Liu et al., 2022), accessed October 3, 2022. A query cut-off of 97% and a percentage identity equal or greater than 50 were used to ensure high sequence alignment identification by DIAMOND against the VFDB (Doonan et al., 2019). To assess the microbe-plant interaction potential of the four species, the annotations were queried using BlastP + HMMER Aligner/Mapper against the “plant bacterial only interaction factors” (PIFAR-Pred) database through the PLant-associated BActeria web resource (PLaBAse) (Martínez-García et al., 2016; Patz et al., 2021). For the identification of type III secretion system effectors (T3SS), open reading frames (ORFs) were first identified using orfipy and exported in fasta format (Singh and Wurtele, 2021). ORFs were then individually uploaded to the Effectidor web server for the prediction of T3SS effectors (Wagner et al., 2022).
Results and discussion
Genotypic characterisation
Pairwise 16S rRNA sequence similarity of BAC 15a-03bT, the proposed type strain of the novel species, in EzBioCloud (Yoon et al., 2019) showed greatest similarity to E. toletana (99.4%), Erwinia endophytica (98.2%), and E. billingiae (97.9%). These similarity values are reflected in the 16S rRNA gene phylogenetic tree (Supplementary Figure 1) where BAC 15a-03bT was situated on a separate branch between E. toletana and E. endophytica, albeit with no bootstrap support. The proposed novel species was confirmed as a new taxon in the concatenated MLSA phylogenetic tree (Figure 1), as all strains formed a single cluster without any reference strains of Erwinia species with validly published names, with 100% bootstrap support. This cluster was in a well-supported clade (88%) with E. toletana and E. iniecta, corresponding to Subgroup 3 from Soutar and Stavrinides (2022) providing support for their transfer to a novel genus. “Erwinia beijingensis” was situated on a separate branch on the border of this clade, but without bootstrap support, suggesting that its position could change with the addition of further novel species.
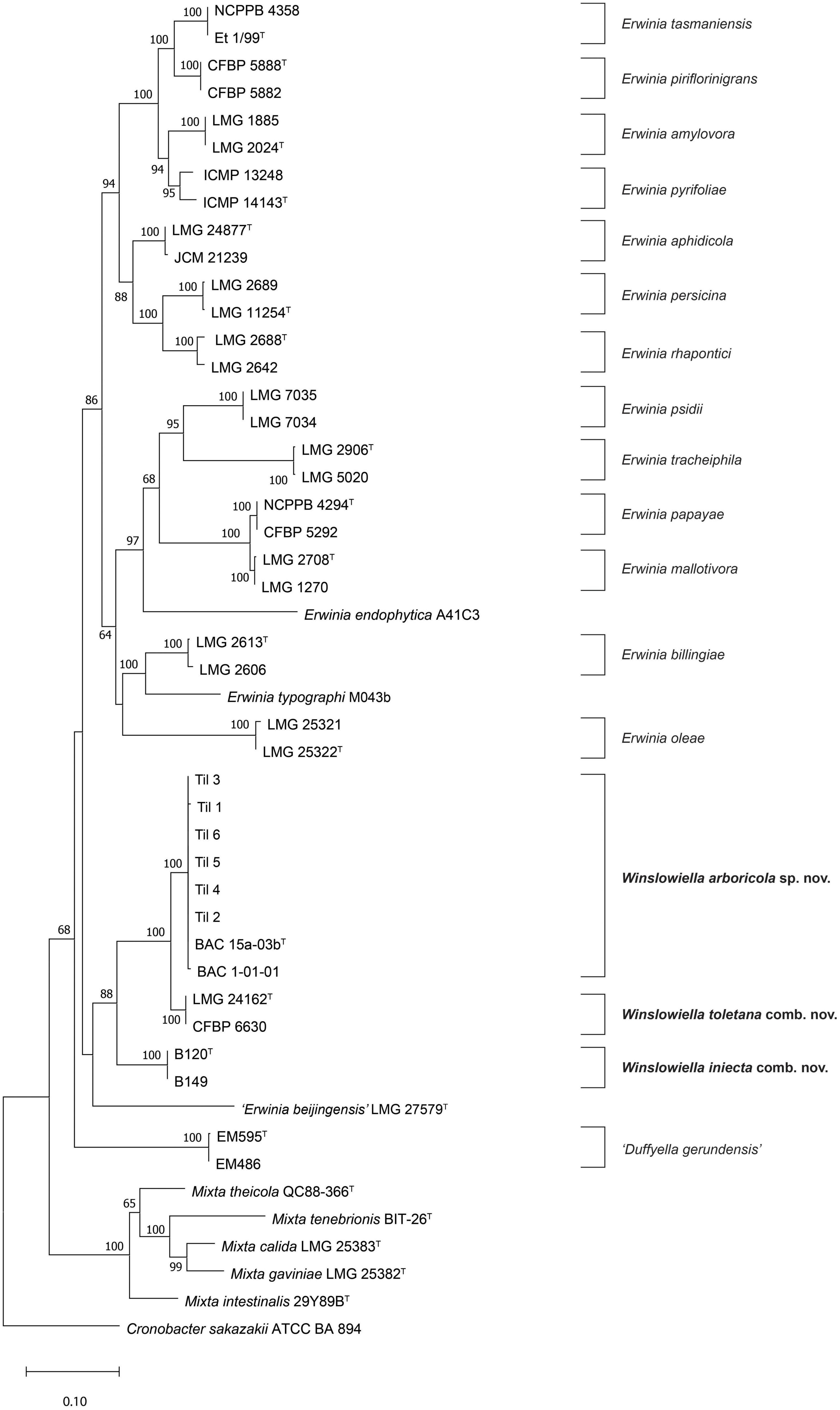
Figure 1. Maximum likelihood tree based on concatenated partial gyrB, rpoB, atpD, and infB gene sequences of Winslowiella gen. nov., Winslowiella arboricola sp. nov., and phylogenetic relatives. Bootstrap values after 1,000 replicates are expressed as percentages (values > 50% shown). Cronobacter sakazakii (ATCC-BAA 894) is included as an outgroup. The scale bar indicates the fraction of substitutions per site. T, type strain.
The BOX PCR profiles demonstrated that there were slight variations between strains isolated from the same tree host species, although identical clones isolated from common lime were also observed (Supplementary Figure 2). Several shared bands were observed between strains isolated from London plane and common lime. However, these are clearly different hosts situated in different counties, suggesting the strains do not originate from a single clone and that a genetically divergent population exists in Great Britain.
Genome features
Assembly of the sequences for BAC 15a-03bT and Til 1 resulted in genomes of 5.23–5.31 Mbp, with the DNA G + C content ranging from 53.5–53.6 mol%. Genome sequences were submitted to GenBank under the BioProject number PRJNA878947. Assembly accession numbers and genome features are listed in Supplementary Table 2. ANI values (Table 1) confirmed the status of the strains from bleeding cankers as a single novel taxon, with 100% similarity observed between the strains. The ANI data reflects the phylogenetic relationship observed between the novel species and E. iniecta and E. toletana, with similarity values ranging from 83.8 to 91.7% between the three species, although these are well below the suggested cut-off value of 95–96% for species delimitation (Goris et al., 2007). The remaining Erwinia species tested demonstrated lower values of 79.1–80.6% when compared to the novel species, E. iniecta and E. toletana. “E. beijingensis” exhibited ANI values of 80.4–80.5% to the three species under examination, which are in the same region as the values shared by E. aphidicola and the three species (80.5–80.6%). The conclusions inferred from the ANI analysis were confirmed by in silico DNA-DNA hybridisation (Meier-Kolthoff et al., 2013), the results for which are also presented in Table 1. The AAI data (Supplementary Table 3) were congruent with those from ANI, with higher values observed between the novel species, E. iniecta and E. toletana (86–95%) and lower values to the remaining Erwinia species (76–78%) providing justification for their transfer to a novel genus. The AAI values between the proposed novel genus and “E. beijingensis” are 81–82%, which are slightly higher than those exhibited by other Erwinia species but significantly less than the values observed within the novel genus. Genus delineation criteria, based on AAI, have been suggested with species exhibiting > 76% AAI to the type strain of the type species of the genus, and all type strains within the genus sharing > 74% AAI to each other (Nicholson et al., 2020). These cut-off values appear too relaxed for the Erwiniaceae, and Soutar and Stavrinides (2022) suggested that 79% may be an appropriate threshold for delineating genera within this family. However, several Erwinia species exhibited AAI values which can be considered “borderline.” The POCP values (Supplementary Table 3) demonstrated a similar pattern with higher values (75–87%) observed between species of the proposed novel genus, and lower values (64–73%) to “E. beijingensis” and Erwinia species. It is worth noting that E. aphidicola, E. oleae, and E. persicina all demonstrated higher POCP values to the proposed novel genus than “E. beijingensis.”
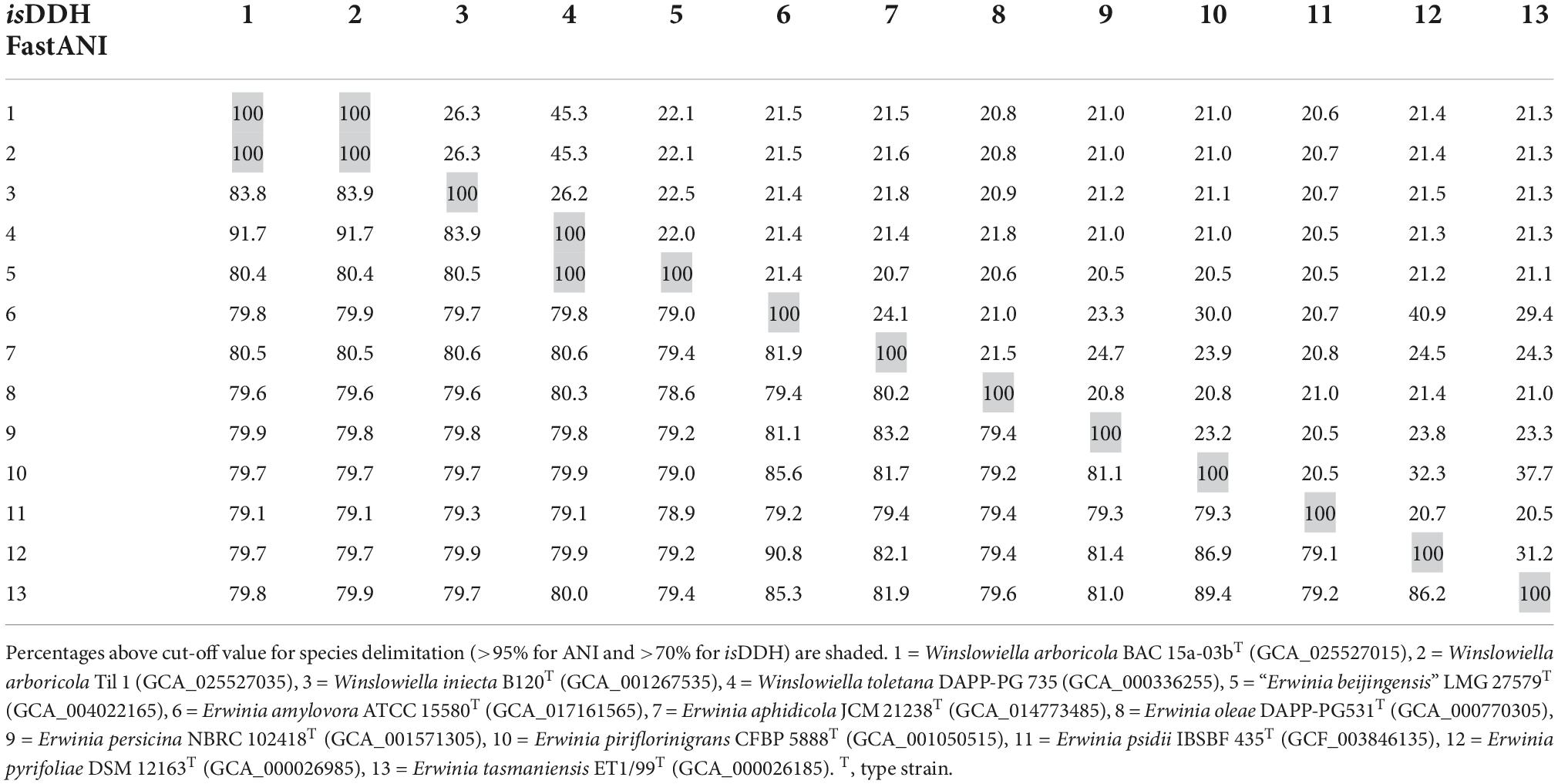
Table 1. DNA-DNA similarity values between Winslowiella arboricola sp. nov., Winslowiella iniecta comb. nov., Winslowiella toletana comb. nov., and existing species of the genus Erwinia based on average nucleotide identity (fastANI—lower left) and in silico DNA-DNA hybridisation (isDDH—upper right).
The phylogenomic tree generated in TYGS clustered the novel species, E. toletana and E. iniecta in a single clade on a separate lineage with 100% support (Figure 2). “E. beijingensis” was situated on a separate branch on the border of this clade, further removed from the proposed novel genus but with 100% support. The phylogeny of the whole genome tree demonstrates the divergence within the genus Erwinia with three clear subgroups of species, as defined previously (Soutar and Stavrinides, 2022) and confirms the proposal that E. iniecta and E. toletana should be reclassified within a novel genus along with the novel species.
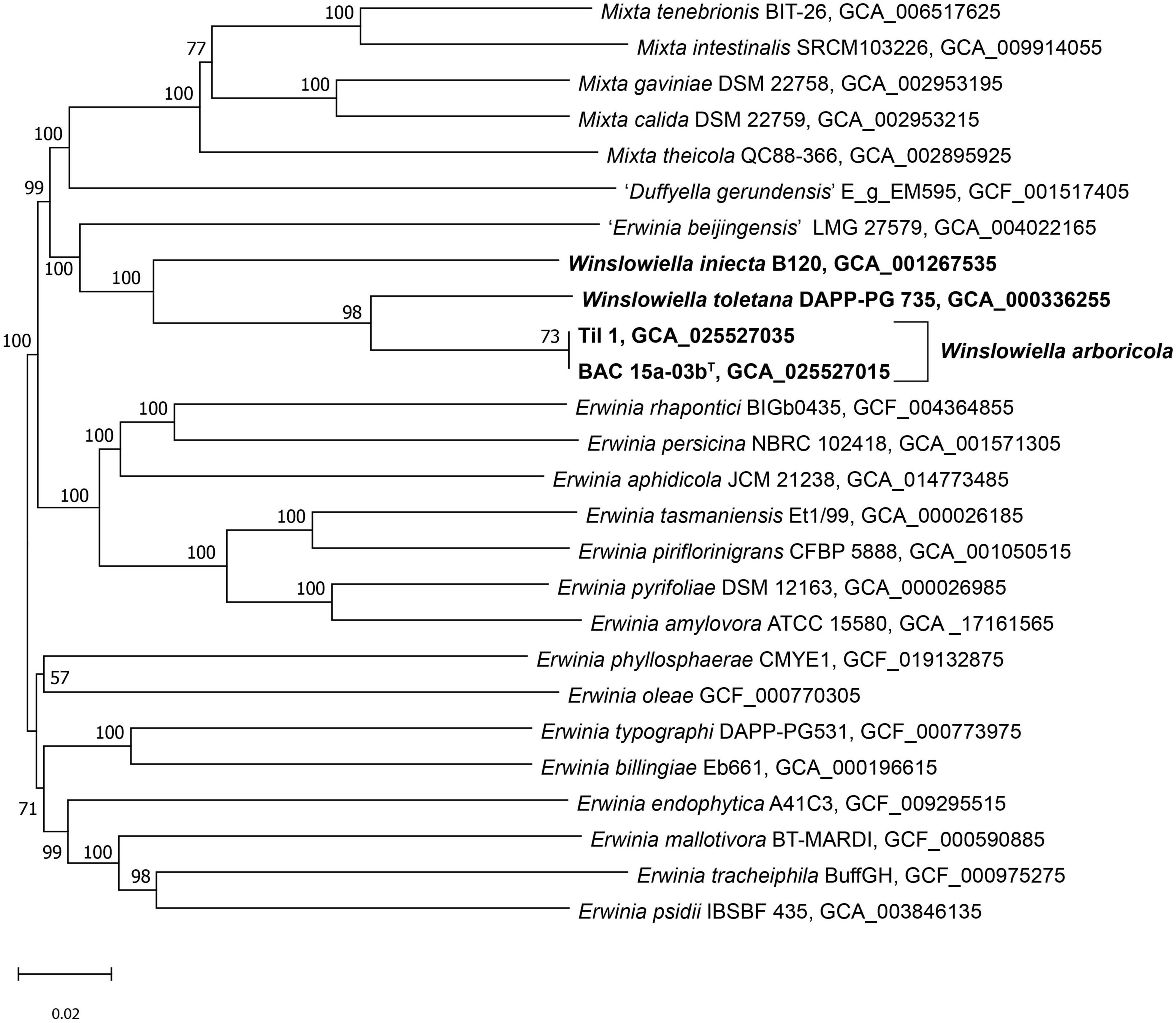
Figure 2. Phylogenomic tree of Winslowiella gen. nov., Winslowiella arboricola sp. nov., and phylogenetic relatives. GBDP pseudo-bootstrap support values > 60% shown at the nodes (from 100 replicates), with an average branch support of 85.4%. The branch lengths are scaled in terms of GBDP distance formula d5 and the tree is rooted at the midpoint. T, type strain.
Phenotypic and chemotaxonomic characterisation
After 48 h growth on LB agar, colonies were round, 2–3 mm in diameter, smooth with entire edges, slightly convex, translucent, and cream to pale yellow in colour. Strains grew readily at temperatures of 10–30°C after incubation for 3 days, with weak growth observed at 4 and 37°C. Salt was tolerated by strains in broth supplemented with up to 7% NaCl, while growth in a narrow pH range of 6–8 was observed, with weak growth at pH 9. The proposed novel species is catalase positive and oxidase negative. Cells are straight rods with rounded ends, typically 0.6–1.3 × 1.5–3.5 μm in size, can occur singly, and in pairs or chains and motile by peritrichous flagella (Supplementary Figure 3).
The novel species could be differentiated from its current closest phylogenetic relatives by several phenotypic characteristics including reactions to acetoin production, tryptophan deaminase activity, aspartic acid and pectin (differentiation from E. iniecta) and L-rhamnose, amygdalin, β-methyl-D-glucoside and D-serine (differentiation from E. toletana). Differentiating phenotypic characteristics are presented in Table 2, and positive phenotypic characteristics shared by all current members of the proposed novel genus are listed in Supplementary Table 4. The fatty acid profile of the novel species was most similar to its current closest phylogenetic relative, E. toletana, with C16:0, C18:1 ω7c, and summed features 2 and 3 composing the major fatty acids. The complete fatty acid profiles of the novel Erwinia species and current closest phylogenetic relatives are listed in Table 3. “E. beijingensis” exhibited a distinctive fatty acid profile when compared to species of the proposed novel genus, with a high percentage of the cyclopropane fatty acid C19:0 ω8c and only a minor amount of summed feature 3, which is a major fatty acid of the novel genus. Phenotypic data available from literature for the genera Erwinia and Mixta (Liu et al., 2013; Hauben and Swings, 2015; Ramírez-Bahena et al., 2016; Rezzonico et al., 2016; Mavima et al., 2022) were examined for characteristics to support the differentiation of the proposed novel genus. Although complete phenotypic information for species of Erwinia and Mixta is not widely published (Palmer et al., 2018), some useful phenotypic traits are listed in Table 4.
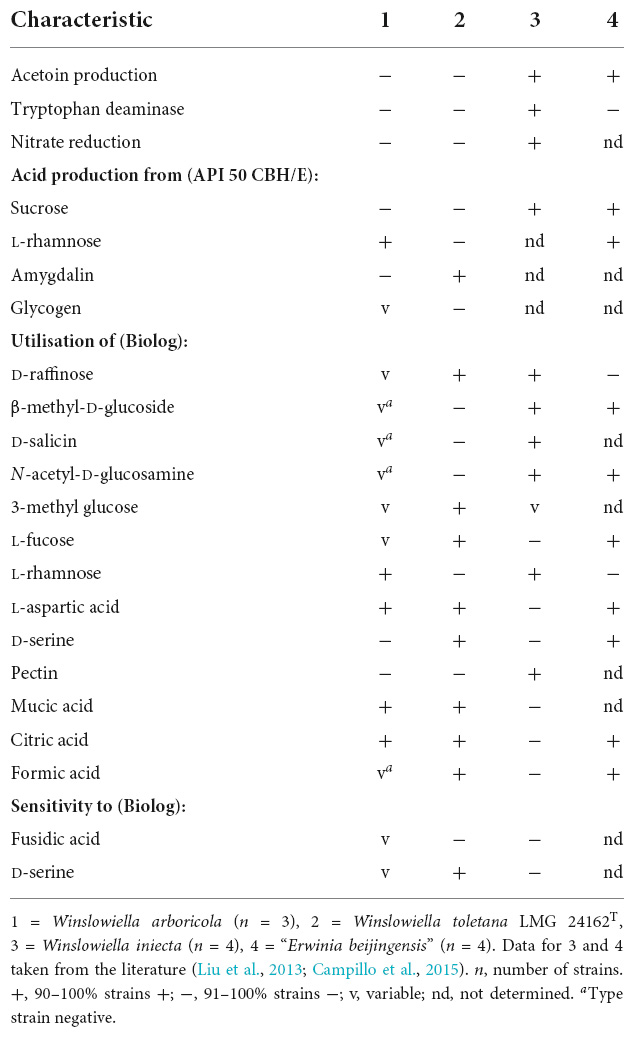
Table 2. Phenotypic characteristics that can distinguish Winslowiella arboricola sp. nov. from its current closest phylogenetic relatives.
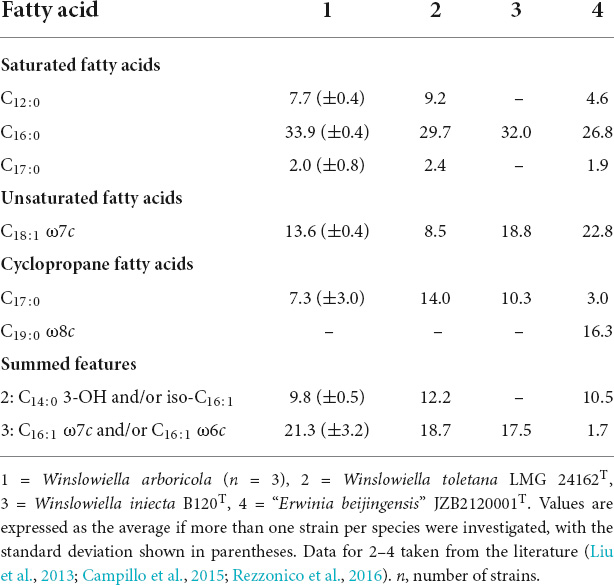
Table 3. Major fatty acid composition (percentage of peak areas) of Winslowiella arboricola sp. nov. and current closest phylogenetic relatives.
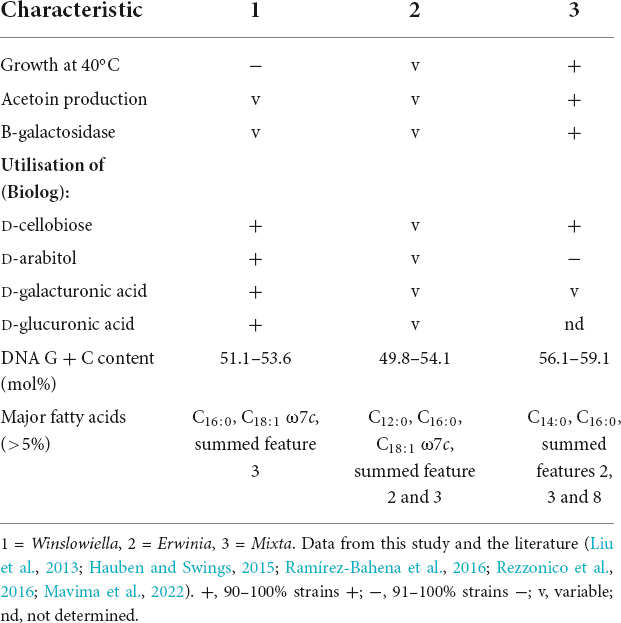
Table 4. Phenotypic characteristics for differentiation of the novel genus Winslowiella from the genera Erwinia and Mixta.
Virulence gene identification
The BlastP alignment results from the VFDB using the query parameters revealed the presence of 101 virulence genes for BAC 15a-03bT, Til 1 and E. toletana DAPP-PG 735 while 121 and 105 alignments were identified in E. iniecta B120T and “E. beijingensis” JZB2120001T, respectively. Within each comparison 11–23 type III secretion effector delivery system proteins such as SpaQ and Spa9 were identified, excluding “E. beijingensis.” This is most likely due to the stringent search parameters used, which when relaxed revealed a number of T3SS related genes which were previously identified (Xu et al., 2021). The other major protein roles identified were related to motility, immune modulation, invasion, and adherence. The presence of a T3SS has long been used to implicate bacteria as phytopathogens. Within the order Enterobacterales, it is usually an indicator of plant pathogens such as the soft rot pathogen Pectobacterium atrosepticum or the blight pathogens E. amylovora and E. pyrifoliae (Toth et al., 2006; Kube et al., 2010). Due to the importance of the secretion system, Effectidor was used to identify T3SS proteins and effectors from ORFs for each genome. Each species was found to contain 26 core TS33 proteins and related effectors, some of which appear to be novel.
The PIFAR-predictions also demonstrated similar implications for the novel species, with the identified “plant only interaction factors” being “genes that are related to plant interaction and virulence.” Nine different plant cell wall degrading enzymes (PCWDE) were identified in each genome. Many genes relating to detoxification and adhesion were identified amongst other plant interaction factors, such as hormone regulation and exopolysaccharides. Notable potential virulence genes are listed in Supplementary Table 5. The identified genes from both the VFDB and PIFAR comparisons indicate that all four species investigated contain genes used in invasion, adhesion and breakdown of plant tissue, indicating a strong pathogenic potential. Given the point of isolation of the novel species was from bleeding cankers of broadleaf hosts, further investigation of its role as novel pathogen is warranted.
Conclusion
The genus Erwinia has long been a repository for species causing necroses, wilts and soft rot of plant hosts. However, the Erwinia species with true phytopathogenic potential are outweighed by those seldom-isolated species or those reported as epiphytes, isolated from insects or opportunistic human pathogens. Currently, 21 species with validly published names are assigned to Erwinia (Parte et al., 2020) with more than half of these described in the last two decades. The relative ease and lowering costs of whole genome sequencing has resulted in a wealth of Erwinia genomes available for analysis, allowing construction of stable phylogenomic analyses. An increase in the classification of novel species has revealed the intra-genus divergence within Erwinia (Soutar and Stavrinides, 2022), and it is becoming clear that Erwinia will require further taxonomic re-evaluation as more novel species are described. The identification of a novel species in the present study, closely related to E. toletana and E. iniecta provides further evidence for their transfer to a novel genus. “E. beijingensis” appears to be a close relative of the novel genus, but borderline AAI and POCP values and a contrasting FAME profile exclude its transfer to the novel genus. Further work is still required to determine the true taxonomic position of “E. beijingensis.”
The data generated in this study provides support, based on phylogenetic, genomic and phenotypic analyses, for the classification of a novel species and the reclassification of E. toletana and E. iniecta in a novel genus closely related to the genus Erwinia. Therefore, we propose to transfer E. toletana and E. iniecta to the novel genus Winslowiella gen. nov. as Winslowiella toletana comb. nov. and Winslowiella iniecta comb. nov., and the description of Winslowiella arboricola sp. nov. for the strains isolated from bleeding cankers of broadleaf hosts in Great Britain (type strain = BAC 15a-03bT = LMG 32576T = NCPPB 4696T).
Description of Winslowiella gen. nov.
Winslowiella (Win.slow.i.el’la. N.L. fem. n. Winslowiella, named in honour of C.E. Winslow who proposed the genus name Erwinia).
The description is based on the data from Rojas et al. (2004); Campillo et al. (2015), and this study. Cells are Gram-negative, short, straight rods with rounded ends (0.5–1.5 × 1.5–3.5 μm) and can occur singly or in pairs and sometimes chains. Motile by peritrichous flagella. Catalase positive and oxidase negative, facultatively anaerobic. On LB agar colonies are slightly convex, circular with smooth edges, translucent, glistening, non-pigmented and cream to yellow in colour with a diameter of 1–3 mm. Strains can grow at 10–37°C, optimally at 28–30°C but not above 39°C, within the pH range of 6–8 and can tolerate supplemented salt conditions of up to 4%, with some species able to grow in 7% NaCl. Negative for arginine dihydrolase, lysine decarboxylase, ornithine decarboxylase, citrate utilisation, H2S production, urease, indole production, and gelatinase. β-galactosidase, tryptophan deaminase activity, acetoin production, and nitrate reduction are all variable.
Major fatty acids are C16:0, C18:1 ω7c, and summed feature 3 (C16:1 ω7c and/or C16:1 ω6c).
Winslowiella species are secondary invaders on diseased olive trees, and have been isolated from wheat aphids, bleeding cankers of broadleaf hosts and the wider environment.
The DNA G + C content ranges from 51.1 to 53.6 mol%. The type species is Winslowiella toletana.
Description of Winslowiella toletana comb. nov.
Winslowiella toletana (to.le.ta.na. N.L. fem. adj. toletana, from Toletum, the Roman name for Toledo, the location from which the organisms were isolated).
Basonym: Erwinia toletana (Rojas et al., 2004).
The description is as given above for the genus and E. toletana in Rojas et al. (2004).
The DNA G + C content is 53.6 mol%.
The type strain is A37T (=CFBP 6631T = ATCC 700880T = CECT 5263T) and was isolated from olive knots in association with Pseudomonas savastanoi pv. savastanoi as secondary invaders on diseased plants.
Description of Winslowiella iniecta comb. nov.
Winslowiella iniecta (in.iec’ta. L. fem. part. adj. iniecta, thrust in, injected, referring to the fact that the first strains isolated from artificial media were introduced or “thrust in” via the aphid stylet).
Basonym: Erwinia iniecta (Campillo et al., 2015).
The description is as given above for the genus and E. iniecta in Campillo et al. (2015).
The DNA G + C content ranges from 51.1 to 52.2 mol%.
The type strain is B120T (=CFBP 8182T = NCCB 100485T), and was isolated from artificial diets fed on by Diuraphis noxia, biotype 2.
Description of Winslowiella arboricola sp. nov.
Winslowiella arboricola (ar.bo.ri’cola. L. fem. n. arbor, a tree; L. masc./fem. n. suff. -cola, dweller; from L. masc./fem. n. incola, dweller; N.L. fem. n. arboricola, tree dweller).
The description is as given for the genus with the following additional characteristics. Strains can tolerate supplemented salt conditions of up to 7%. β-galactosidase activity is variable among strains, but tryptophan deaminase activity, acetoin production, and nitrate reduction are all negative. Acid is produced from L-arabinose, D-ribose, D-xylose, D-galactose, D-glucose, D-fructose, D-mannose, L-rhamnose, inositol, D-mannitol, N-acetyl glucosamine, arbutin, esculin ferric citrate, salicin, D-cellobiose, D-maltose, D-lactose, D-melibiose, D-trehalose, gentiobiose, and D-arabitol (API 50 CHB/E).
The following carbon sources are utilised in addition to those listed in Supplementary Table 4: β-methyl-D-glucoside, N-acetyl neuraminic acid, L-rhamnose, inosine, and mucic acid.
The DNA G + C content ranges from 53.5 to 53.6 mol%.
The type strain is BAC 15a-03bT = LMG 32576T = NCPPB 4696T, and was isolated from the bleeding lesion of a Platanus x hispanica (London plane) tree in London, Great Britain.
Data availability statement
The data presented in this study are deposited in GenBank/EMBL/DDBJ under the accession numbers: OP422451 (16S rRNA); OP414924-OP414931 (atpD); OP414932-OP414939 (gyrB); OP414940-OP414947 (infB); OP414950-OP414955 (rpoB); and JAODIL000000000-JAODIM000000000 (whole genome).
Author contributions
CB was involved in the conceptualisation, data curation, formal analysis, investigation, methodology, validation, visualization, and writing of the work. SK, BC, and DM were involved in the data curation, investigation of the work, and reviewing and editing of the manuscript. DA and SD were responsible for funding acquisition and reviewing and editing of the manuscript. All authors contributed to the article and approved the submitted version.
Funding
This research was supported by the UK Research and Innovation’s (UKRI) Strategic Priorities Fund (SPF) programme on Bacterial Plant Diseases (grant BB/T010886/1) funded by the Biotechnology and Biological Sciences Research Council (BBSRC), the Department for Environment, Food and Rural Affairs (Defra), the Natural Environment Research Council (NERC), and the Scottish Government. CB received additional funding from Woodland Heritage (Charity No. 1041611) and SD received funding from the Forestry Commission.
Acknowledgments
We thank Ana Perez for assistance with sampling and Martin Steele and Graham Sear for allowing us to sample at Tidworth Garrison. We gratefully acknowledge the Wolfson Bioimaging Facility, especially Lorna Hodgson, for assistance with the electron microscopy, and Aharon Oren for his advice on suitable names and for providing the etymology of these. We also thank Jan Leach and Emily Luna for discussions around E. iniecta. Genome sequencing was performed by MicrobesNG (http://www.microbesng.uk).
Conflict of interest
The authors declare that the research was conducted in the absence of any commercial or financial relationships that could be construed as a potential conflict of interest.
Publisher’s note
All claims expressed in this article are solely those of the authors and do not necessarily represent those of their affiliated organizations, or those of the publisher, the editors and the reviewers. Any product that may be evaluated in this article, or claim that may be made by its manufacturer, is not guaranteed or endorsed by the publisher.
Supplementary material
The Supplementary Material for this article can be found online at: https://www.frontiersin.org/articles/10.3389/fmicb.2022.1063107/full#supplementary-material
Footnotes
References
Bankevich, A., Nurk, S., Antipov, D., Gurevich, A. A., Dvorkin, M., Kulikov, A. S., et al. (2012). SPAdes: A new genome assembly algorithm and its applications to single-cell sequencing. J. Comput. Biol. 19, 455–477. doi: 10.1089/cmb.2012.0021
Bolger, A. M., Lohse, M., and Usadel, B. (2014). Trimmomatic: A flexible trimmer for Illumina sequence data. Bioinformatics 30, 2114–2120. doi: 10.1093/bioinformatics/btu170
Brady, C., Asselin, J. A., Beer, S., Brurberg, M. B., Crampton, B., Venter, S., et al. (2022). Rahnella perminowiae sp. nov., Rahnella bonaserana sp. nov., Rahnella rivi sp. nov. and Rahnella ecdela sp. nov., isolated from diverse environmental sources, and emended description of the genus Rahnella. Int. J. Syst. Evol. Microbiol. 72:005190. doi: 10.1099/ijsem.0.005190
Brady, C., Cleenwerck, I., Venter, S., Vancanneyt, M., Swings, J., and Coutinho, T. (2008). Phylogeny and identification of Pantoea species associated with plants, humans and the natural environment based on multilocus sequence analysis (MLSA). Syst. Appl. Microbiol. 31, 447–460. doi: 10.1016/j.syapm.2008.09.004
Buchfink, B., Reuter, K., and Drost, H. G. (2021). Sensitive protein alignments at tree-of-life scale using DIAMOND. Nat. Methods 18, 366–368. doi: 10.1038/s41592-021-01101-x
Buonaurio, R., Moretti, C., Da Silva, D. P., Cortese, C., Ramos, C., and Venturi, V. (2015). The olive knot disease as a model to study the role of interspecies bacterial communities in plant disease. Front. Plant Sci. 6:434. doi: 10.3389/fpls.2015.00434
Campillo, T., Luna, E., Portier, P., Saux, M. F., Lapitan, N., Tisserat, N. A., et al. (2015). Erwinia iniecta sp. nov., isolated from Russian wheat aphid (Diuraphis noxia). Int. J. Syst. Evol. Microbiol. 65, 3625–3633. doi: 10.1099/ijsem.0.000466
Coenye, T., Falsen, E., Vancanneyt, M., Hoste, B., Govan, J. R. W., Kersters, K., et al. (1999). Classification of Alcaligenes faecalis-like isolates from the environment and human clinical samples as Ralstonia gilardii sp. nov. Int. J. Syst. Bacteriol. 49, 405–413. doi: 10.1099/00207713-49-2-405
Coutinho, T. A., Brady, C. L., Vaart, M., Van Der Venter, S. N., Telechea, N., Rolfo, M., et al. (2011). A new shoot and stem disease of eucalyptus species caused by Erwinia psidii. Australas. Plant Pathol. 40, 55–60. doi: 10.1007/s13313-010-0013-y
Doonan, J., Denman, S., Pachebat, J. A., and McDonald, J. E. (2019). Genomic analysis of bacteria in the acute oak decline pathobiome. Microb. Genomics 5, 1–15. doi: 10.1099/mgen.0.000240
Goris, J., Konstantinidis, K. T., Klappenbach, J. A., Coenye, T., Vandamme, P., and Tiedje, J. M. (2007). DNA-DNA hybridization values and their relationship to whole-genome sequence similarities. Int. J. Syst. Evol. Microbiol. 57, 81–91. doi: 10.1099/ijs.0.64483-0
Guindon, S., Dufayard, J. F., Lefort, V., Anisimova, M., Hordijk, W., and Gascuel, O. (2010). New algorithms and methods to estimate maximum-likelihood phylogenies: Assessing the performance of PhyML 3.0. Syst. Biol. 59, 307–321. doi: 10.1093/sysbio/syq010
Hauben, L., and Swings, J. (2015). “Erwinia,” In Bergey’s manual of systematics of archaea and bacteria, eds M. E. Trujillo, S. Dedysh, P. DeVos, B. Hedlund, P. Kämpfer, F. A. Rainey, et al. doi: 10.1002/9781118960608.gbm01146
Hoelzer, M. (2020). Calculation of percentage of conserved proteins. Available online at: https://github.com/hoelzer/pocp (accessed October 20, 2022).
Jain, C., Rodriguez-R, L. M., Phillippy, A. M., Konstantinidis, K. T., and Aluru, S. (2018). High throughput ANI analysis of 90K prokaryotic genomes reveals clear species boundaries. Nat. Commun. 9:5114. doi: 10.1038/s41467-018-07641-9
Kim, W., Gardan, L., Rhim, S., and Geiderl, K. (1996). Erwinia pyrifoliae sp. nov., a novel pathogen that affects Asian pear trees (Pyrus pyrifolia Nakai). Int. J. Syst. Bacteriol. 49(Pt 2), 899–905.
Kube, M., Migdoll, A. M., Gehring, I., Heitmann, K., Mayer, Y., Kuhl, H., et al. (2010). Genome comparison of the epiphytic bacteria Erwinia billingiae and E. tasmaniensis with the pear pathogen E. pyrifoliae. BMC Genomics 11:393. doi: 10.1186/1471-2164-11-393
Kumar, S., Stecher, G., Li, M., Knyaz, C., and Tamura, K. (2018). MEGA X: Molecular evolutionary genetics analysis across computing platforms. Mol. Biol. Evol. 35, 1547–1549. doi: 10.1093/molbev/msy096
Lefort, V., Desper, R., and Gascuel, O. (2015). FastME 2.0: A comprehensive, accurate, and fast distance-based phylogeny inference program. Mol. Biol. Evol. 32, 2798–2800. doi: 10.1093/molbev/msv150
Lefort, V., Longueville, J.-E., and Gascuel, O. (2017). SMS: Smart model selection in PhyML. Mol. Biol. Evol. 34, 2422–2424. doi: 10.1093/molbev/msx149
Liu, B., Zheng, D., Zhou, S., Chen, L., and Yang, J. (2022). VFDB 2022: A general classification scheme for bacterial virulence factors. Nucleic Acids Res. 50, D912–D917. doi: 10.1093/nar/gkab1107
Liu, Y., Wang, S., Zhang, D., Wei, S., Zhao, S., Chen, S., et al. (2013). Pantoea beijingensis sp. nov., isolated from the fruiting body of Pleurotus eryngii. Antonie van Leeuwenhoek. Int. J. Gen. Mol. Microbiol. 104, 1039–1047. doi: 10.1007/s10482-013-0024-0
López, M. M., Roselló, M., Llop, P., Ferrer, S., Christen, R., and Gardan, L. (2011). Erwinia piriflorinigrans sp. nov., a novel pathogen that causes necrosis of pear blossoms. Int. J. Syst. Evol. Microbiol. 61, 561–567. doi: 10.1099/ijs.0.020479-0
Maktar, N. H., Kamis, S., Mohd Yusof, F. Z., and Hussain, N. H. (2008). Erwinia papayae causing papaya dieback in Malaysia. Plant Pathol. 57:774. doi: 10.1111/j.1365-3059.2008.01877.x
Martínez-García, P. M., López-Solanilla, E., Ramos, C., and Rodríguez-Palenzuela, P. (2016). Prediction of bacterial associations with plants using a supervised machine-learning approach. Environ Microbiol 18, 4847–4861. doi: 10.1111/1462-2920.13389
Matsuura, T., Mizuno, A., Tsukamoto, T., Shimizu, Y., Saito, N., Sato, S., et al. (2012). Erwinia uzenensis sp. nov., a novel pathogen that affects European pear trees (Pyrus communis L.). Int. J. Syst. Evol. Microbiol. 62, 1799–1803. doi: 10.1099/ijs.0.032011-0
Mavima, L., Steenkamp, E. T., Coetzee, M. P., and Palmer, M. (2022). “Mixta,” in Bergey’s manual of systematics of archaea and bacteria, eds M. E. Trujillo, S. Dedysh, P. DeVos, B. Hedlund, P. Kämpfer, F. A. Rainey, et al. doi: 10.1002/9781118960608.gbm02012
Meier-Kolthoff, J. P., and Göker, M. (2019). TYGS is an automated high-throughput platform for state-of-the-art genome-based taxonomy. Nat. Commun. 10:2182. doi: 10.1038/s41467-019-10210-3
Meier-Kolthoff, J. P., Auch, A. F., Klenk, H.-P., and Göker, M. (2013). Genome sequence-based species delimitation with confidence intervals and improved distance functions. BMC Bioinformatics 14:60. doi: 10.1186/1471-2105-14-60
Nicholson, A. C., Gulvik, C. A., Whitney, A. M., Humrighouse, B. W., Bell, M. E., Holmes, B., et al. (2020). Division of the genus Chryseobacterium: Observation of discontinuities in amino acid identity values, a possible consequence of major extinction events, guides transfer of nine species to the genus Epilithonimonas, eleven species to the genus Kaistella, and three species to the genus Halpernia gen. nov., with description of Kaistella daneshvariae sp. nov. and Epilithonimonas vandammei sp. nov. derived from clinical specimens. Int. J. Syst. Evol. Microbiol. 70, 4432–4450. doi: 10.1099/ijsem.0.003935
Niemann, S., Pühler, A., Tichy, H. V., Simon, R., and Selbitschka, W. (1997). Evaluation of the resolving power of three different DNA fingerprinting methods to discriminate among isolates of a natural Rhizobium meliloti population. J. Appl. Microbiol. 82, 477–484. doi: 10.1046/j.1365-2672.1997.00141.x
Okonechnikov, K., Golosova, O., and Fursov, M., and UGENE team (2012). Unipro UGENE: A unified bioinformatics toolkit. Bioinformatics 28, 1166–1167. doi: 10.1093/bioinformatics/bts091
Palmer, M., Steenkamp, E. T., Coetzee, M. P. A., Avontuur, J. R., Chan, W. Y., van Zyl, E., et al. (2018). Mixta gen. nov., a new genus in the Erwiniaceae. Int. J. Syst. Evol. Microbiol. 68, 1396–1407. doi: 10.1099/ijsem.0.002540
Parte, A. C., Sardà Carbasse, J., Meier-Kolthoff, J. P., Reimer, L. C., and Göker, M. (2020). List of prokaryotic names with standing in nomenclature (LPSN) moves to the DSMZ. Int. J. Syst. Evol. Microbiol. 70, 5607–5612. doi: 10.1099/ijsem.0.004332
Patz, S., Gautam, A., Becker, M., Ruppel, S., Rodríguez-Palenzuela, P., and Huson, D. (2021). PLaBAse: A comprehensive web resource for analyzing the plant growth-promoting potential of plant-associated bacteria. bioRxiv [Preprint]. bioRxiv 2021.12.13.472471.
Qin, Q. L., Xie, B., Zhang, X. Y., Chen, X. L., Zhou, B. C., Zhou, J., et al. (2014). A proposed genus boundary for the prokaryotes based on genomic insights. J. Bacteriol. 196, 2210–2215. doi: 10.1128/JB.01688-14
Ramírez-Bahena, M. H., Salazar, S., Cuesta, M. J., Tejedor, C., Igual, J. M., Fernández-Pascual, M., et al. (2016). Erwinia endophytica sp. nov., isolated from potato (Solanum tuberosum L.) stems. Int. J. Syst. Evol. Microbiol. 66, 975–981. doi: 10.1099/ijsem.0.000820
Rezzonico, F., Smits, T. H. M., Born, Y., Blom, J., Frey, J. E., Goesmann, A., et al. (2016). Erwinia gerundensis sp. nov., a cosmopolitan epiphyte originally isolated from pome fruit trees. Int. J. Syst. Evol. Microbiol. 66, 1583–1592. doi: 10.1099/ijsem.0.000920
Rodrigues Neto, J., Robbs, C. F., and Yamashiro, T. (1987). A bacterial disease of guava (Psidium guajava) caused by Erwinia psidii sp. nov. Fitopatol. Bras. 12, 345–350.
Rodriguez-R, L. M., and Konstantinidis, K. T. (2016). The enveomics collection: A toolbox for specialized analyses of microbial genomes and metagenomes. PeerJ 4:e1900v1. doi: 10.7287/peerj.preprints.1900v1
Rojas, A. M., García de los Rios, J. E., Fischer-Le Saux, M., Jimenez, P., Reche, P., Bonneau, S., et al. (2004). Erwinia toletana sp. nov., associated with Pseudomonas savastanoi-induced tree knots. Int. J. Syst. Evol. Microbiol. 54, 2217–2222. doi: 10.1099/ijs.0.02924-0
Singh, U., and Wurtele, E. S. (2021). orfipy: A fast and flexible tool for extracting ORFs. Bioinformatics 37, 3019–3020. doi: 10.1093/bioinformatics/btab090
Soutar, C. D., and Stavrinides, J. (2022). Phylogenomic analysis of the Erwiniaceae supports reclassification of Kalamiella piersonii to Pantoea piersonii comb. nov. and Erwinia gerundensis to the new genus Duffyella gen. nov. as Duffyella gerundensis comb. nov. Mol. Genet. Genomics 297, 213–225. doi: 10.1007/s00438-021-01829-3
Tatusova, T., DiCuccio, M., Badretdin, A., Chetvernin, V., Nawrocki, E. P., Zaslavsky, L., et al. (2016). NCBI prokaryotic genome annotation pipeline. Nucleic Acids Res. 44, 6614–6624. doi: 10.1093/nar/gkw569
Toth, I. K., Pritchard, L., and Birch, P. R. J. (2006). Comparative genomics reveals what makes an enterobacterial plant pathogen. Annu. Rev. Phytopathol. 44, 305–336. doi: 10.1146/annurev.phyto.44.070505.143444
Vanneste, J. (2008). Erwinia amylovora (fireblight). CABI datasheet, 21908. Available online at: https://www.cabi.org/isc/datasheet/21908 (accessed March 2, 2022).
Versalovic, J., Schneider, M., de Bruijn, F., and Lupski, J. R. (1994). Genomic fingerprinting of bacteria using repetitive sequence-based polymerase chain reaction. Methods Mol. Cell Biol. 5, 25–40.
Wagner, N., Avram, O., Gold-Binshtok, D., Zerah, B., Teper, D., and Pupko, T. (2022). Effectidor: An automated machine-learning-based web server for the prediction of type-III secretion system effectors. Bioinformatics 38, 2341–2343.
Winslow, C.-E. A., Broadhurst, J., Buchanan, R. E., Krumwiede, C., Rogers, L. A., and Smith, G. H. (1920). The families and genera of the bacteria final report of the committee of the society of American bacteriologists on characterization and classification of bacterial types. J. Bacteriol. 5, 191–229. doi: 10.1128/jb.5.3.191-229.1920
Xu, F., Yan, H., Liu, Y., Zhao, S., Song, S., Gu, T., et al. (2021). A re-evaluation of the taxonomy and classification of the type III Secretion system in a pathogenic bacterium causing soft rot disease of Pleurotus eryngii. Curr. Microbiol. 78, 179–189. doi: 10.1007/s00284-020-02253-3
Keywords: Erwinia, Erwiniaceae, Erwinia toletana, bleeding canker, Tilia, Platanus x acerifolia, Erwinia iniecta, Winslowiella
Citation: Brady C, Kaur S, Crampton B, Maddock D, Arnold D and Denman S (2022) Transfer of Erwinia toletana and Erwinia iniecta to a novel genus Winslowiella gen. nov. as Winslowiella toletana comb. nov. and Winslowiella iniecta comb. nov. and description of Winslowiella arboricola sp. nov., isolated from bleeding cankers on broadleaf hosts. Front. Microbiol. 13:1063107. doi: 10.3389/fmicb.2022.1063107
Received: 06 October 2022; Accepted: 31 October 2022;
Published: 17 November 2022.
Edited by:
Javier Pascual, Darwin Bioprospecting Excellence, SpainReviewed by:
Iain Sutcliffe, Northumbria University, United KingdomJesus L. Romalde, University of Santiago de Compostela, Spain
Copyright © 2022 Brady, Kaur, Crampton, Maddock, Arnold and Denman. This is an open-access article distributed under the terms of the Creative Commons Attribution License (CC BY). The use, distribution or reproduction in other forums is permitted, provided the original author(s) and the copyright owner(s) are credited and that the original publication in this journal is cited, in accordance with accepted academic practice. No use, distribution or reproduction is permitted which does not comply with these terms.
*Correspondence: Carrie Brady, carrie.brady@uwe.ac.uk