- 1Key Laboratory of Low Carbon Culture and Safety Production in Cattle in Sichuan, Animal Nutrition Institute, Sichuan Agricultural University, Chengdu, China
- 2Department of Bioengineering, Sichuan Water Conservancy Vocational College, Chengdu, China
- 3College of Animal Science and Technology, Sichuan Agricultural University, Chengdu, China
- 4Yunnan Animal Science and Veterinary Institute, Kunming, China
The suitable supplement pattern affects the digestion and absorption of trace minerals by ruminants. This study aimed to compare the effects of coated and uncoated trace elements on growth performance, apparent digestibility, intestinal development and microbial diversity in growing sheep. Thirty 4-month-old male Yunnan semi-fine wool sheep were randomly assigned to three treatments (n = 10) and fed with following diets: basal diet without adding exogenous trace elements (CON), basal diet plus 400 mg/kg coated trace elements (CTE, the rumen passage rate was 65.87%) and basal diet plus an equal amount of trace elements in uncoated form (UTE). Compared with the CON group, the average daily weight gain and apparent digestibility of crude protein were higher (P < 0.05) in the CTE and UTE groups, while there was no difference between the CTE and UTE groups. The serum levels of selenium, iodine and cobalt were higher (P < 0.05) in the CTE and UTE groups than those in the CON group, the serum levels of selenium and cobalt were higher (P < 0.05) in the CTE group than those in the UTE group. Compared with the CON and UTE groups, the villus height and the ratio of villus height to crypt depth in duodenum and ileum were higher (P < 0.05) in the CTE groups. The addition of trace minerals in diet upregulated most of the relative gene expression of Ocludin, Claudin-1, Claudin-2, ZO-1, and ZO-2 in the duodenum and jejunum and metal ion transporters (FPN1 and ZNT4) in small intestine. The relative abundance of the genera Christensenellaceae R-7 group, Ruminococcus 1, Lachnospiraceae NK3A20 group, and Ruminococcaceae in ileum, and Ruminococcaceae UCG-014 and Lactobacillus in colon was higher in the CTE group that in the CON group. These results indicated that dietary trace mineral addition improved the growth performance and intestinal development, and altered the structure of intestinal bacteria in growing sheep. Compared to uncoated form, offering trace mineral elements to sheep in coated form had a higher absorption efficiency, however, had little effect on improving growth performance of growing sheep.
1 Introduction
Trace elements such as iron (Fe), copper (Cu), manganese (Mn), zinc (Zn), selenium (Se), iodine (I), and cobalt (Co) are an essential class of substances for animals provided primarily by food sources. Although the mass proportion in animal body is less than 0.01%, trace elements play an indispensable role in maintaining their normal physiological activities, such as hemopoiesis, immune response, energy metabolism, enzyme activity and reproductive function (Rabiee et al., 2010; Overton and Yasui, 2014). A deficiency of any of these trace minerals could lead to a negative impact on the health and productivity of livestock (Spears and Weiss, 2008). Commonly, inorganic salts of trace elements, such as sulfate, oxide, carbonate and chloride are supplemented in diets to meet the requirement of livestock for trace elements. However, for ruminants, the ionic bonds in inorganic salts usually dissociate as they flow through the digestive tract, allowing undesirable interactions with other molecules and preventing absorption, thus reducing the bioavailability and efficiency of trace elements (Spears, 1996, 2003; Samarin et al., 2022). For example, sulfates are first reduced to sulfides by rumen microbes, which react with molybdate to form thiomolybdate and then react with cupric ion to generate un-digestible compounds, thereby limiting the absorption of dietary Cu (Dick et al., 1975). The efficiency of absorption of Cu and Se by ruminants is much lower than that of non-ruminants (Spears, 2003). Furthermore, in some scenarios, rumen fermentation was affected by feeding conventional mineral salts, as was ruminal digestibility of nutrients (Genther and Hansen, 2015; Faulkner and Weiss, 2017; Arce-Cordero et al., 2020). To improve the availability of trace elements, the addition of coated trace elements (slow release in the rumen) to the diet was a practicable strategy (Abdelrahman et al., 2017; Arce-Cordero et al., 2020; Potier et al., 2022).
There were a series of reports on the effective application of coated trace elements in ruminants. Previous studies reported that supplementation of coated slow-release trace elements at late gestation increased the content of Zn and Se in plasma and milk of ewes, and enhanced the birth and weaning weight of lambs (Abdelrahman et al., 2017; Aliarabi et al., 2019). Supplementation of coated sustained-release trace minerals also improved the milk performance and altered rumen microbial structure of lactating yaks (Zhao et al., 2022). Compared with the addition of copper sulfate in diets, coated copper sulfate addition improved lactation performance and apparent nutrient digestibility in dairy cows (Wang C. et al., 2021), increased the average daily gain and stimulated rumen enzyme activity in Holstein bulls (Wu et al., 2021). The addition of an equal amount of coated sodium selenite contributed to improve the milk production of dairy cows (Zhang et al., 2020), improved the ruminal fermentation and nutrient digestibility of dairy bulls (Liu et al., 2020), compared to uncoated sodium selenite. However, to our knowledge, there is very little information comparing uncoated and coated compound trace elements in ruminants.
The improved bioavailability of trace elements is beneficial to exert their biological functions. More than 50% of known enzymes have at least one metal as a cofactor, of which zinc is the most common, followed by iron and manganese (Andreini et al., 2008). Most trace minerals are also involved in redox reactions, as free radicals are able to interact with metal-binding superoxide dismutase and break down into less or less toxic substances, indicating that trace minerals play an important role in alleviating oxidative stress (Pajarillo et al., 2021). Dietary zinc oxide supplementation enhanced intestinal morphological and functional development by increasing antioxidant capacity and maintaining mucosal barrier integrity in weaned piglets (Zhu et al., 2017, 2017). Besides, trace elements such as Fe, Mn, Zn, Se and Cu have been revealed to regulate the gut microbiota in different animals or in vitro (Dostal et al., 2015; Zhang et al., 2019; Foong et al., 2020; Kociova et al., 2020; Wang et al., 2020). The regulation of the gut barrier and immune response by selenium intake has been attributed to modulation of the gut microbiota (Kasaikina et al., 2011). We suspected that the coated trace elements had better biological activity on growth performance and intestinal development in sheep compared to uncoated trace elements. Thus, the ultimate objective of this study was to compare the effects of coated and uncoated trace elements on growth performance, apparent digestibility, intestinal development, and microbial diversity in ileum and colon of growing sheep.
2 Materials and methods
2.1 Approval by the ethics committee
All animals in this study were treated according to the Chinese Guidelines for Animal Welfare and the protocol was approved by the Experimental Animal Committee of Animal Nutrition Institute, Sichuan Agricultural University (Approval number: #SCAUAC2019-36).
2.2 Experimental design, diets, and animals
Exp. 1. The experiment was performed at the farm of Kunming Yixingheng Livestock Technology Co., Ltd. (Yunnan, China; 25.07°N, 102.55°E, and 2,200 m elevation) in August 2019. Three 15-month-old male Yunnan semi-fine wool sheep (55 ± 2.3 kg of body weight) with permanent rumen cannulas were used as experimental animals. All experimental sheep were housed in individual pens and fed twice per day (0700 and 1700 h) and had free access to water and diets. The basal diet was formulated according to the recommendation of National Research Council (2007) for growing sheep except for trace elements, and the composition and nutrient levels are shown in Table 1. The coated trace elements (contained 120 mg Fe, 16 mg Cu, 50 mg Mn, 52 mg Zn, 0.8 mg Se, 1.0 mg I and 0.8 mg Co per gram) were commercially produced and provided by Fujian Syno Biotech Co., Ltd. (Fujian, China). The final product of coated trace elements is a molten granulate produced by proportioning an inorganic trace element premix and basic coating materials (carboxymethyl cellulose, resistant starch and maltodextrin) in the solid phase. Approximately 5.0 g of coated trace elements was added to a heat-sealed nylon bag (4 × 6 cm in bag size; 50 μm in pore size). Each sheep was considered a replicate, each sample was repeated three times, and each sample had two parallel replicates per sheep for a total of six replicates. A maximum of four bags with samples were placed in the liquid phase of the rumen contents through a polyethylene hose at the same time. The coated trace elements were sequentially incubated in different batches for 0, 2, 4, 8, 16, 24, 36, and 48 h.
Exp. 2. The experiment was performed at the same farm as Exp. 1 from August to September 2021. A total of thirty 4-month-old male Yunnan semi-fine wool sheep (29.7 ± 0.3 kg of body weight) were divided into three treatments in a completely randomized design as follows: CON treatment, fed with a basal diet without adding exogenous trace elements; CTE treatment, fed with a basal diet plus 400 mg/kg coated trace elements in Exp. 1; UTE treatment, fed with a basal diet plus an equal amount of trace elements with uncoated form. The basal diet was kept the same as that in Exp. 1. The addition of 400 mg/kg coated trace elements had the best growth performance of Yunnan semi-fine wool sheep, according to our previous study (unpublished data). The uncoated trace elements are constituted by ferrous sulfate heptahydrate, copper sulfate pentahydrate, manganese sulfate monohydrate, zinc sulfate heptahydrate, sodium selenite pentahydrate, potassium iodide, and cobalt chloride hexahydrate. All experimental sheep were housed in separate pens and fed twice daily at 0700 and 1700 h (allowed 5% refusal). The trial lasted for 40 days, of which 10 days were the adaptation period and 30 days were the formal trial period.
2.3 Sample collection
All mobile nylon bags were incubated at the same time (0630 h) prior to morning feeding and retrieved according to the expected incubation time in Exp. 1. After removal from the rumen, the nylon bags were repeatedly rinsed under cold running water to remove adhesions and subsequently placed in cold water for soaking while the nylon bags were repeatedly kneaded by hand gently until the filtered water was clear (Wang E. et al., 2021). The cleaned nylon bags were dried to constant weight at 65°C for 48 hours, and the weight after drying was recorded and the rumen disappearance rate of the coated trace elements was calculated by the difference from the initial weight.
In Exp. 2, the body weight of experimental sheep was determined before the morning feeding at the beginning (day 0) and end (day 30) of the experiment to calculate the average daily gain (ADG). The orts were collected and weighed every day, the dry matter intake (DMI) per sheep was obtained from the ration supplied and refused, and the feed conversion ratio (FCR) was calculated as dividing DMI by ADG. Four sheep were randomly selected from each group and housed in individual metabolic cages for 7 days (from day 10 to 16, the first two days were the adaptation period), and all feces were collected, weighed and recorded. A 5% aliquot of each fecal sample was removed and immediately stored at −20°C until further analysis. Jugular vein blood samples were collected on day 30 before feeding in the morning and centrifuged at 1,600 × g for 10 minutes to obtain serum, which were then aliquoted and stored at −20°C until the next analysis. On day 30, four sheep of close to group average weight in each treatment were slaughtered, and tissue samples of duodenum, jejunum and ileum and chyme samples of ileum and colon were collected. For the tissue samples, the 2- and 6-cm segments were cut at mid-duodenum, mid-jejunum and mid-ileum, respectively (Hou et al., 2010). The 2-cm intestinal segments were flushed gently with pre-cooled 0.9% sodium chloride solution (w/v, physiological saline, Sichuan Kelun Pharmaceutical Co., Ltd., Chengdu, China) and then placed in 4% paraformaldehyde fix solution (Beyotime, Shanghai, China) for morphological analysis. The 6-cm intestinal segments were opened longitudinally and the contents were flushed with pre-cooled physiological saline and immediately frozen in liquid nitrogen for total RNA extraction. Chyme from the mid-section of the ileum and colon was collected in 2 mL sterile cryopreserved tubes (Wuxi NEST Biotechnology Co., Ltd., Wuxi, China) for bacterial DNA extraction. All sampling procedure were finished within 30 minutes after the sheep being slaughtered.
2.4 Total tract apparent nutrient digestibility
Fecal samples from each sheep were thawed and mixed together, then dried in a forced air oven at 55°C for 48 hours and ground to pass through a 40-mesh screen before analysis, as were the samples of diet and orts. The content of dry matter (DM), crude protein (CP), acid detergent fiber (ADF), calcium (Ca) and phosphorus (P) was analyzed according to AOAC (AOAC International, 2007), and the content of neutral detergent fiber (NDF) were determined according to van Soest et al. (1991). Consequently, the apparent digestibility of nutrients can be calculated as follow: apparent nutrient digestibility = [(feed intake × nutrient content in the diet) − (fecal output × nutrient content in the feces)]/(feed intake × nutrient content in the diet × 100% (Stein and Bohlke, 2007).
2.5 Intestinal morphological observation
The 2-cm intestinal segments for morphological analysis were dehydrated and embedded in paraffin, cut into three sections with 5 μm thick and stained with Hematoxylin and Eosin (H&E). Morphological observation was carried out with the Nikon ECLIPSE 80i microscope (Nikon Co., Ltd., Tokyo, Japan). Villus height (distance from villus tip to crypt ostia) and width (distance from widest villus), crypt depth (distance from crypt ostia to base) were measured with the Image-Pro Plus 6.0 software (Media Cybernetics Inc., Bethesda, MD, United States). The ratio of villus height and crypt depth (V/C) was calculated as dividing villus height by crypt depth. Only the villi heights and crypt depths from vertically oriented ten adjacent villi were measured and averaged (Hou et al., 2010).
2.6 Serum sample analysis
The serum concentrations of Fe, Cu, Mn, Zn, Se, I and Co were determined in an induced coupled plasma mass-spectrometry (iCAP™ RQ ICP-MS, Thermo Fisher Scientific, Waltham, MA, USA) as described previously with minor modifications (Liu et al., 2014). In brief, 0.1 mL of reconstituted serum samples were diluted to 2 mL with a 1: 20 (v/v) sample diluent solution [constructed from 0.01% (w/v) Triton X-100, 0.005% (w/v) L-cysteine, 0.5% (v/v) nitric acid and deionized water]. The standard solutions containing the required seven single elements were purchased from the TMRM QC standards material center (Changzhou, China), and then diluted with sample diluent solution to appropriate concentrations according to the predicted concentrations of trace elements in serum. The major ICP-MS operating parameters were set as follows: Analyzer Pressure Readback, 1E-06 mbar; Spray Chamber Temperature, 2°C; Cool Flow, 14 L/min; Auxilliary Flow, 0.79 L/min; Nebulizer Flow, 1.06 L/min; Plasma Power, 1550 W; Q Cell gas, He 4.0 mL/min; injection method, automatic injection.
2.7 Real-time quantitative PCR (RT-PCR) analysis
Intestinal tissue samples were ground into powder in liquid nitrogen. Total RNA was extracted from tissue samples with the RNA extraction and purification kit (Tiangen Biotech Co. Ltd., Beijing, China) according to the manufacturer’s instructions. The concentration and purity of total RNA were determined with the NanoDrop 2000 spectrophotometer (Thermo Fisher Scientific, Wilmington, DE, USA). The integrity of total RNA was assessed by the 1% denatured agarose gel electrophoresis. Subsequently, the qualified total RNA was reverse transcribed to cDNA by a reverse transcription Kit (HiScript® III RT SuperMix for qPCR (+gDNA wiper), Vazyme Biotech Co., Ltd., Nanjing, China). The real-time quantitative PCR (RT-PCR) reaction was performed with a real-time quantitative PCR Kit (ChamQ SYBR Color qPCR Master Mix (low ROX premixed), Vazyme Biotech Co., Ltd., Nanjing, China) in a QuantStudio™ 6 Real-Time PCR system (Applied Biosystems, Foster City, CA, USA) following the manufacturer’s instruction. Relative expression of genes was calculated by using the comparative cycle threshold (2–ΔΔCt) method. The mean value of gene expression levels in the CON treatment was set to 1.00, and GAPDH was used as internal control. The sequence and product size of genes used in this study are shown in Table 2.
2.8 DNA Extraction, amplification, and sequencing
The microbial diversity in ileal and colonic chyme was detected by 16s rDNA sequencing. The total genome DNA (gDNA) from the chyle samples was extracted and purified with the ZymoBIOMICS™ DNA Microprep Kit (Zymo Research, Orange, CA, USA). The NanoDrop 2000 spectrophotometer (Thermo Fisher Scientific) and 0.8% agarose gel electrophoresis were used to detect the concentration and integrity of DNA, respectively. The universal bacterial primer (515F: 5′-GTGYCAGCMGCCGCGGTAA-3′ and 806R: 5′-GGACTACHVGGGTWTCTAAT-3′) with sequencing adapter and barcode were used to amplified the V3-V4 regions of the 16S rRNA gene (Caporaso et al., 2011). The PCR reaction was conducted in the GeneAmp PCR system 9700 thermocycler (Applied Biosystem, Foster city, CA, USA) with the high-fidelity polymerase KOD-401B FX Neo (Toyobo, Osaka, Japan) and the program was as follows: 94°C for 1 min, followed by 30 cycles at 94°C for 20 s, 54°C for 30 s and 72°C for 30 s, and a final extension at 72°C for 5 min. The resulting PCR products were extracted from a 2% agarose gel and further purified with the Zymoclean Gel Recovery Kit (Zymo Research) and quantified with the Qubit 2.0 Fluorometer (Thermo Fisher Scientific, Waltham, MA, USA). Sequencing by synthesis was performed with the HiSeq Rapid SBS kit v2 (200 cycles, Illumina, Inc., San Diego, CA, USA) on the Illumina HiSeq2500 platform at Chengdu Rhonin Biosciences Co., Ltd. (Chengdu, China).
2.9 Sequencing data analyses
The processing of sequencing data was performed as previously described (Zhou et al., 2022). Briefly, the Fast Length Adjustment of SHort reads (FLASH v1.2.11)1 were used to splice the paired-end sequencing reads obtained from the Illumina platform, which then assigned to samples based on the unique barcode 37. The raw data was generated by truncating barcode sequences, followed by trimming using Trimmomatic v0.36,2 and using the Uchime algorithm3 to identify and remove chimera sequences based on the “Gold” database4 to obtain clean reads (Edgar et al., 2011; Bolger et al., 2014). The qualified reads were clustered into operational taxonomic units (OTUs) by using the Usearch (a free unique sequence analysis tool)5 based on a 97% similarity threshold (Edgar, 2013). The analysis of α-diversity (including Shannon, Simpson, Chao1, ACE, the Good’s coverage, and PD indexes) and β-diversity as well as principal coordinate analysis (PCoA) of the Bray-Curtis distance were performed using the R package (Kembel et al., 2010; Chen et al., 2012). The potential Kyoto Encyclopedia of Genes and Genomes (KEGG) functional profiles of microbial communities were predicted with Tax4Fun6 (a free software package that predicts the functional capabilities of microbial communities based on 16S rRNA datasets) based on the SILVA ribosomal RNA database,7 and the secondary metabolic pathways were clustered (Aßhauer et al., 2015).
2.10 Calculations and statistical analysis
The degradation kinetic parameters of coated trace elements over time were calculated with the following exponential equation (Ørskov and McDonald, 1979): DR = a + b (1– e –c×t), where “DR” is the disappearance rate of coated trace elements at time “t” (%), “a” is the rapidly degradable fraction (%), “b” is the potentially degradable fraction (%) and “c” is the rate at which b is degraded per hour (rate constant). The values of “a,” “b,” and “c” were calculated with the non-linear procedure of SPSS v23.0 (SPSS Inc., Chicago, IL, USA). The effective degradability (ED, %) was calculated according to (Ørskov and McDonald, 1979): ED = a + [b × c/(c + k)], where “a,” “b,” and “c” are the same parameters as above, “k” is the rumen particle passage rate (%/h). When fed ad libitum, the value of “k” was 0.06, according to (Ørskov and McDonald, 1979).
The experimental data in Exp. 2 were statistically analyzed with one-way analysis of variance (ANOVA) followed Duncan’s post-hoc test or Kruskal–Wallis-test with SPSS version 23.0. Each sheep was regarded as a statistical unit. P ≤ 0.05 was considered statistically significant, 0.05 < P < 0.10 was considered as trends. The results were shown as mean and standard error (SEM).
3 Results
3.1 Rumen degradation rate
The ruminal disappearance rates of coated trace elements at different times are shown in Figure 1. The non-linear model resulted in the degradation kinetic parameters as follow: a = 6.292, b = 81.728, c = 0.031 and R2 = 0.974. Thus, the rumen effective degradability of coated trace elements can be calculated as 34.13%, the rumen passage rate was 65.87%.
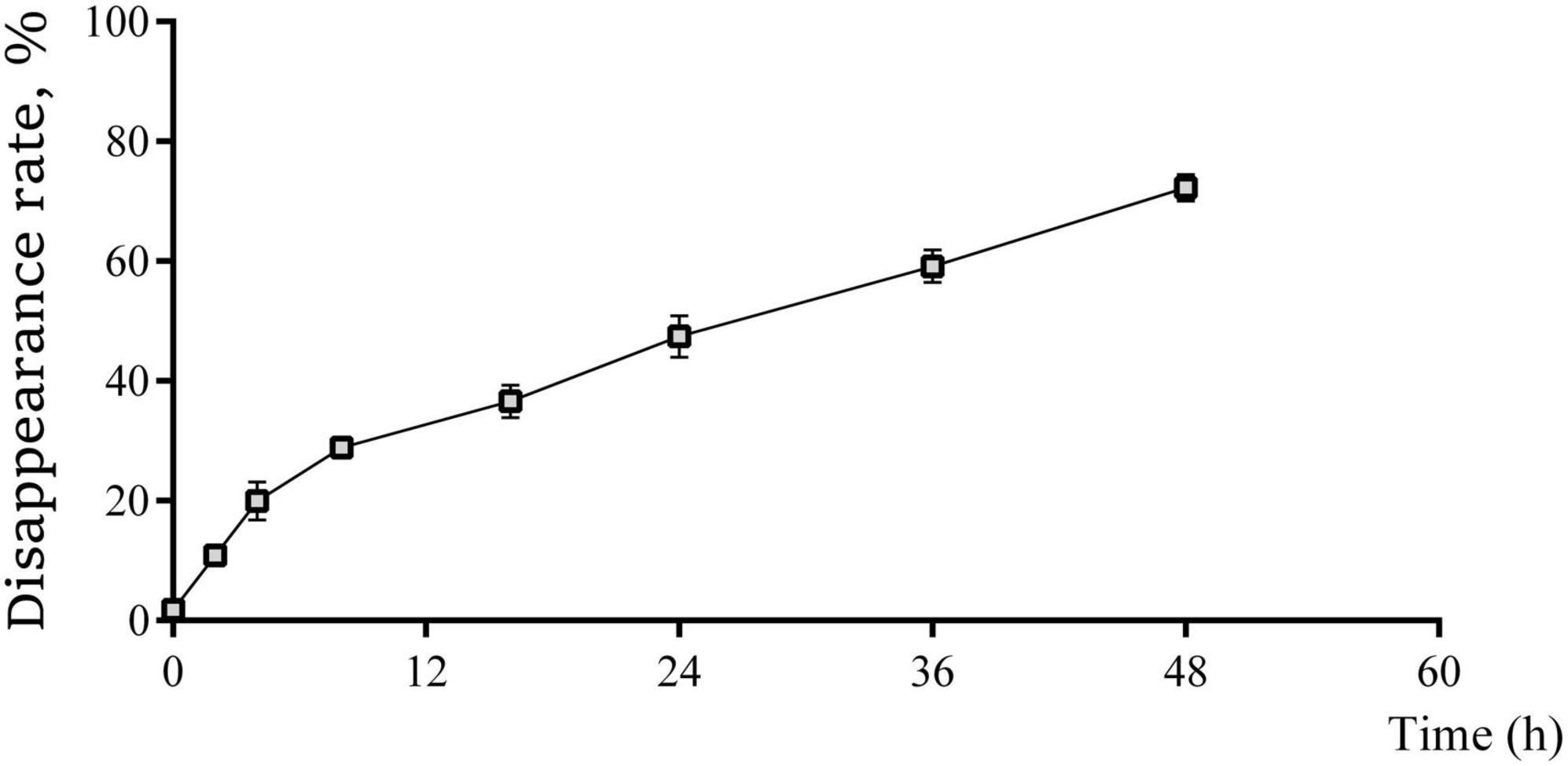
Figure 1. The real-time rumen disappearance rate of coated trace elements within 48 h of culture in nylon bag. Data are represented as mean and standard deviation, n = 3.
3.2 Growth performance
Compared with the CON treatment, the final body weight and ADG were increased (P < 0.05) in the CTE and UTE treatments, the FCR was improved (P < 0.05) in the CTE and UTE treatments, whereas there was no difference between the CTE and UTE treatments (Table 3).
3.3 Total tract apparent nutrient digestibility
The apparent digestibility of CP was higher (P < 0.05) in the CTE and UTE treatments than that in the CON treatment, and that of other nutrients was not affected by the addition of trace elements (Table 4).
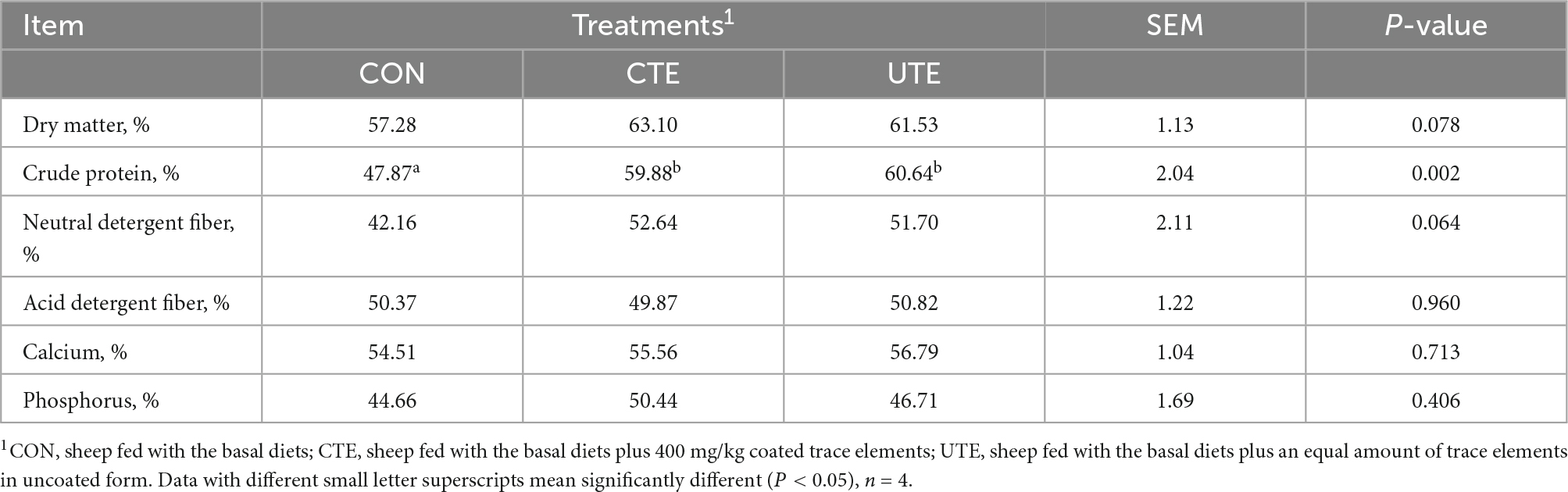
Table 4. Effects of coated and uncoated trace elements on total tract apparent digestibility of nutrients.
3.4 Serum trace elements
Compared with the CTE treatment, the serum levels of Se and Co were lower (P < 0.05) in both the CON and UTE treatments, the serum level of I was lower (P < 0.05) in the CON treatment (Table 5).
3.5 Intestinal morphology
Dietary coated trace elements increased (P < 0.05) the villus height in the duodenum, jejunum and ileum, increased (P < 0.05) the crypt depth in the jejunum and the V/C in the ileum. No difference in intestinal morphology was observed between UTE and CON treatments (Table 6).
3.6 Relative gene expression of tight junction protein
As shown in Figure 2, compared with the CON treatment, both supplementation of coated and uncoated trace elements upregulated (P < 0.05) the relative mRNA abundance of Claudin-1, Claudin-2, and Occludin in the duodenum and jejunum. Dietary coated trace elements upregulated (P < 0.05) the mRNA abundance of ZO-1 and ZO-2 in the duodenum and jejunum, dietary uncoated trace elements upregulated (P < 0.05) the mRNA abundance of ZO-2 in the duodenum.
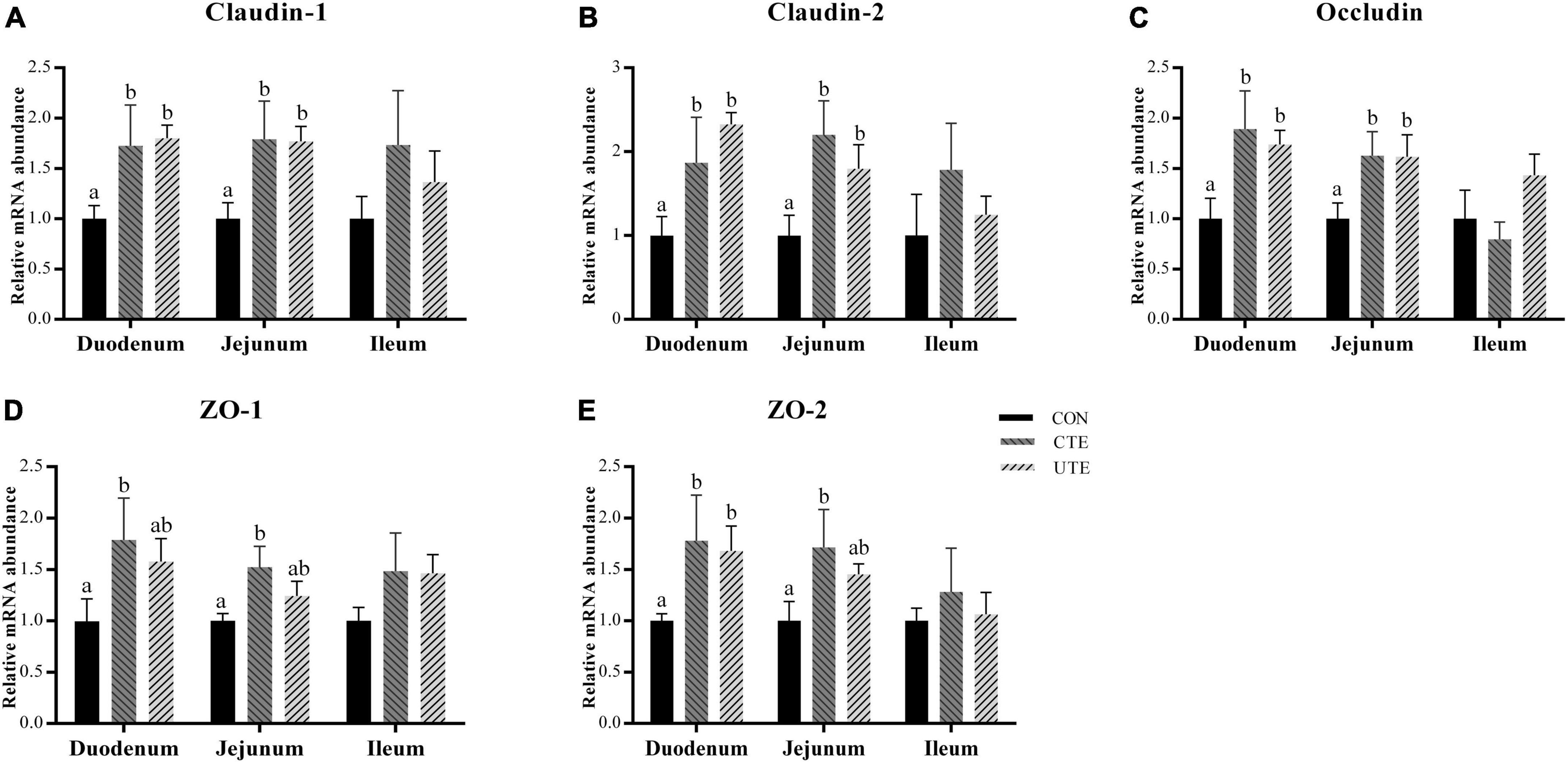
Figure 2. Effects of coated and uncoated trace elements on the relative gene expression of Claudin-1 (A), Claudin-2 (B), Occludin (C), ZO-1 (D), and ZO-2 (E) in small intestine. CON, sheep fed with the basal diets; CTE, sheep fed with the basal diets plus 400 mg/kg coated trace elements; UTE, sheep fed with the basal diets plus an equal amount of trace elements in uncoated form. Data are represented as mean and standard error and with different small letter superscripts mean significantly different (P < 0.05), n = 4.
3.7 Relative gene expression of metal ion transporters
The mRNA abundance of FPN1 and ZNT4 in the duodenum, jejunum and ileum was higher (P < 0.05) in the CTE treatment than that in the CON treatment, the mRNA abundance of FPN1 and ZNT4 in the duodenum and FPN1 in the jejunum was higher (P < 0.05) in the UTE treatment than that in the CON treatment (Figure 3).
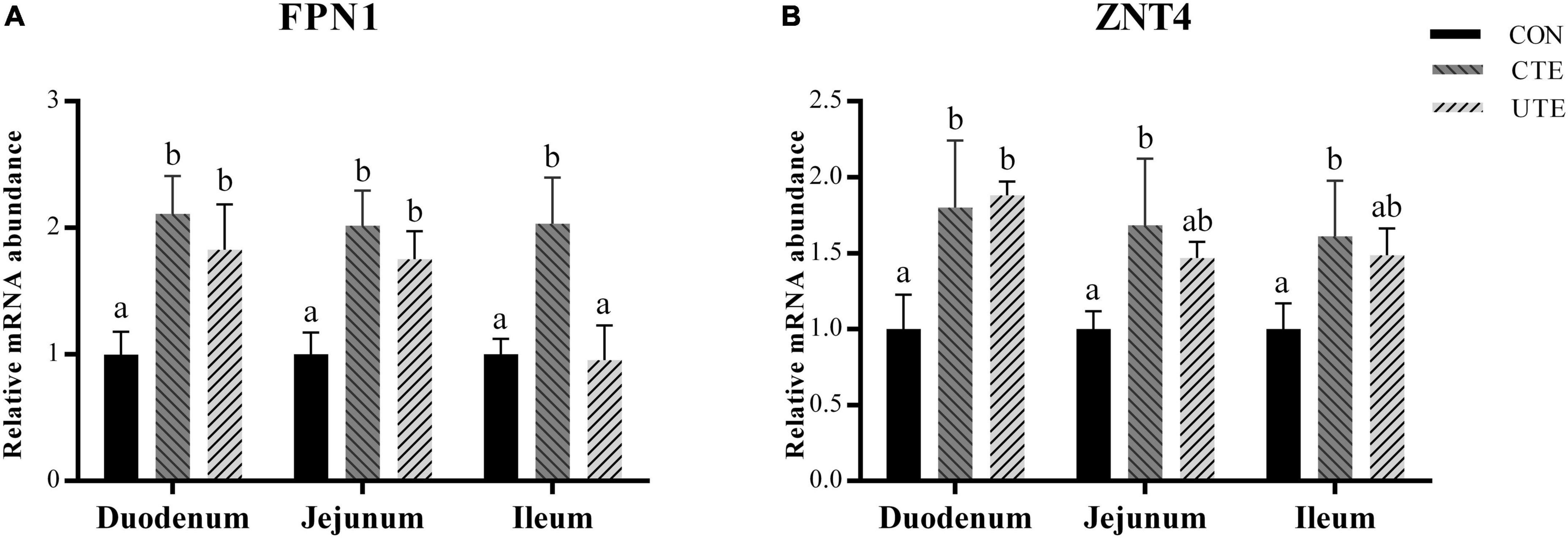
Figure 3. Effects of coated and uncoated trace elements on the relative gene expression of ferroportin 1 (FPN1, A) and zinc transporter 4 (ZNT4, B) in small intestine. CON, sheep fed with the basal diets; CTE, sheep fed with the basal diets plus 400 mg/kg coated trace elements; UTE, sheep fed with the basal diets plus an equal amount of trace elements in uncoated form. Data are represented as mean and standard error and with different small letter superscripts mean significantly different (P < 0.05), n = 4.
3.8 Sequencing data, α-diversity, and β-diversity analysis
A total of 409,756 valid sequences in ileal chyme samples and 403,426 valid sequences in colon chyme samples which met quality control were obtained from the 16S rRNA high-throughput sequencing analysis. Based on 97% sequence similarity from valid sequences, these sequences were completely clustered into 9,936 OTUs in ileal chyme samples and 13,868 OTUs in colon chyme samples. The sequencing data information of bacteria in intestinal samples was shown in Supplementary Table 1. A total of 504 OTUs in ileum chyme samples were shared across the three treatments, and the number of sequences in shared OTUs accounted for 44.41% of the total effective sequences (Figure 4A). A total of 663 OTUs in colon chyme samples were shared across the three treatments, and the number of sequences in shared OTUs accounted for 44.41% of the total effective sequences (Figure 4B). The effect of coated and uncoated trace elements on α-diversity index of bacteria was shown in Supplementary Table 2. The Good’s coverage index was greater than 0.990, indicating that the sampling had sufficient sequence coverage to detect the majority of rumen bacteria. The indices of Shannon, Simpson, Chao1, ACE, PD in the ileal chyme samples were higher (P < 0.05) in the CTE treatment than those in the CON treatment, indicating that dietary coated trace elements increased bacterial diversity in the ileum. There was no difference in the α-diversity index of bacteria in the colon chyme among the three groups, indicating that dietary coating or uncoated micronutrients had no effect on bacterial diversity in colon chyme. The percent variation in the ileum chyme samples was represented by PCo1 (20.9%) and PCo2 (17.3%), the percent variation in the colon chyme samples was represented by PCo1 (21.7%) and PCo2 (15.4%); the closer the distance in the figure, the more similar the bacterial community composition of the samples (Figure 5).
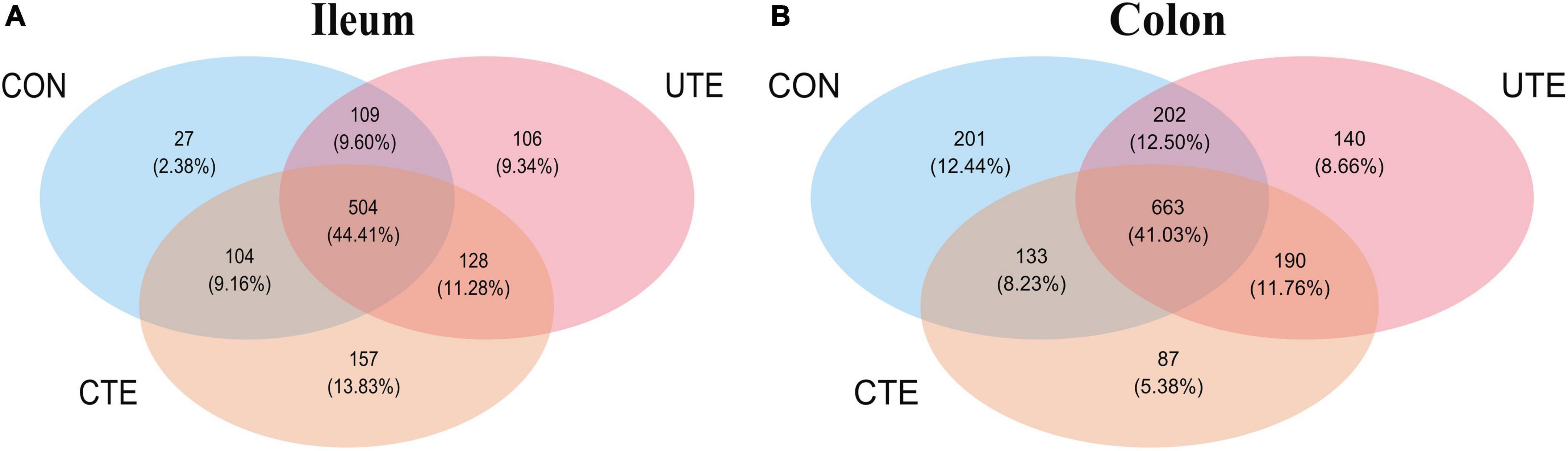
Figure 4. Venn diagram of the OTUs among the three treatments of bacteria in the ileum (A) and colon (B). CON, sheep fed with the basal diets; CTE, sheep fed with the basal diets plus 400 mg/kg coated trace elements; UTE, sheep fed with the basal diets plus an equal amount of trace elements in uncoated form. n = 4.
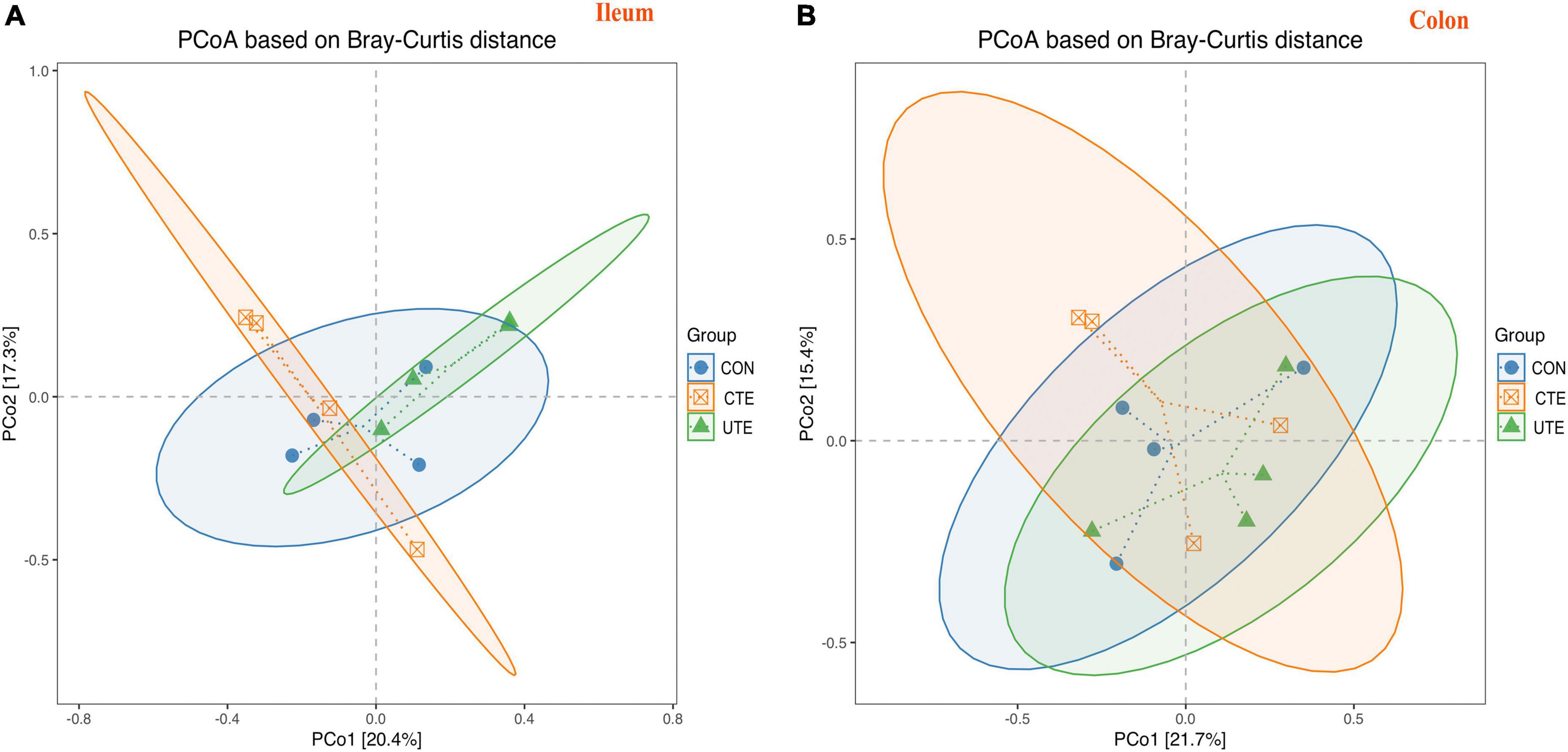
Figure 5. Bray–Curtis distance matrix PCoA of bacterial samples in the ileum (A) and colon (B). CON, sheep fed with the basal diets; CTE, sheep fed with the basal diets plus 400 mg/kg coated trace elements; UTE, sheep fed with the basal diets plus an equal amount of trace elements in uncoated form. n = 4.
3.9 Bacteria abundance at phylum and genus levels
There were 20 and 16 phyla taxonomically classified in the ileum and colon chyme samples, respectively. The dominant phyla of ileum bacteria were Firmicutes (57.28–77.24%) and Euryarchaeota (6.50–13.81%) (Figure 6A), and the dominant phyla of colon bacteria were Firmicutes (43.64–51.87%) and Bacteroidetes (33.39–42.06%) (Figure 6B). The relative abundance of phylum Firmicutes in the ileum was higher (P < 0.05) in the CTE treatment than that in the UTE treatment (Figure 6C). The relative abundance of phylum Euryarchaeota in the ileum was higher (P < 0.05) in the UTE treatment than that in the CON and CTE treatments (Figure 6D). The relative abundance of phylum Proteobacteria in the ileum was lower (P < 0.05) in the CTE treatment than that in the UTE treatment (Figure 6E). The relative abundance of phylum Kiritimatiellaeota in the ileum was higher (P < 0.05) in the CON treatment than that in the CTE and UTE treatments (Figure 6F).
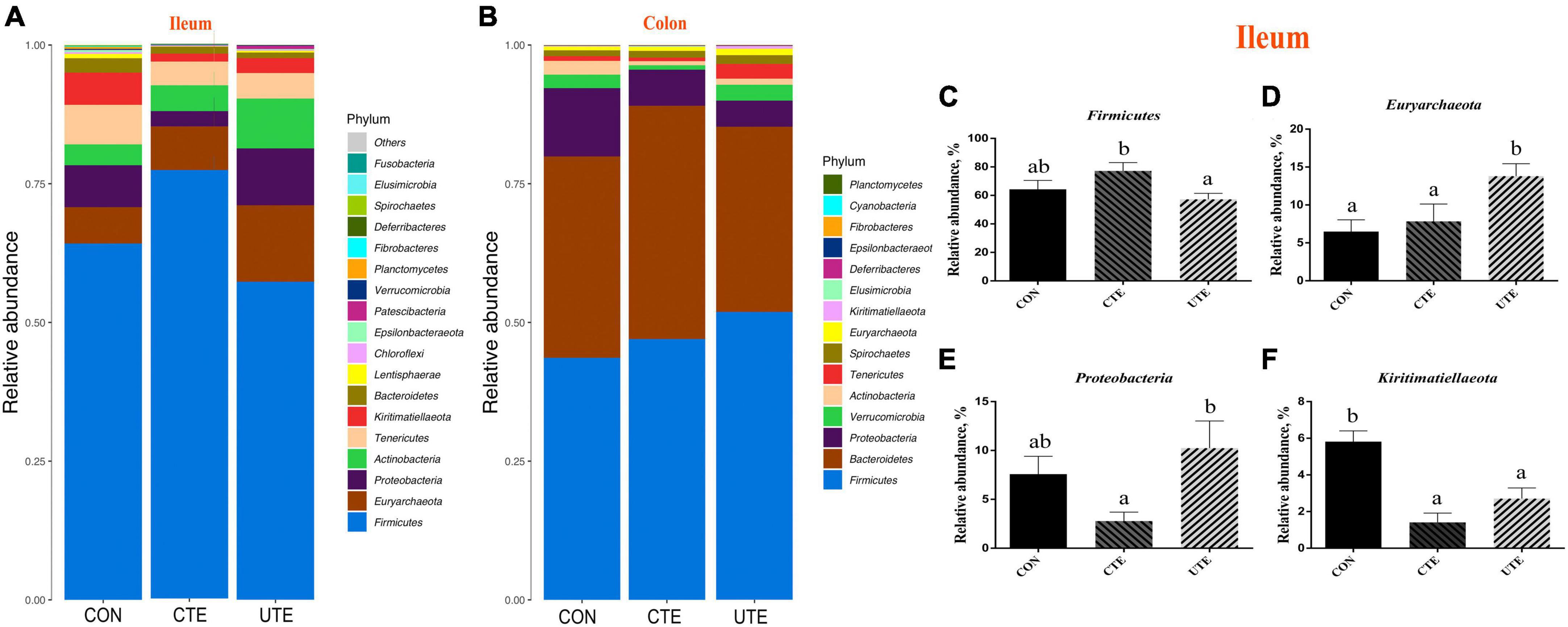
Figure 6. Effects of coated and uncoated trace elements on the relative abundance of bacteria at phylum level. (A) The bacterial composition at phylum level in the ileum; (B) the bacterial composition at phylum level in the colon; (C–F) bacteria with differences in abundance in the ileum at the phylum level. CON, sheep fed with the basal diets; CTE, sheep fed with the basal diets plus 400 mg/kg coated trace elements; UTE, sheep fed with the basal diets plus an equal amount of trace elements in uncoated form. Data are represented as mean and standard error and with different small letter superscripts mean significantly different (P < 0.05), n = 4.
There were 239 and 188 genera taxonomically classified in the ileum and colon chyme samples, respectively. The top three dominant genera of ileum bacteria were Ruminococcus 2 (5.53–37.14%), Eubacterium (6.22–15.42%) and Methanobrevibacter (5.60–13.59%), as shown in Figure 7A, and the top three dominant genera of colon bacteria were Rikenellaceae RC9 gut group (6.72–15.97%), Alistipes (6.64–12.56%) and Succinivibrio (3.41–11.25%), as shown in Figure 7B. The relative abundance of genus Methanobrevibacter in the ileum was higher (P < 0.05) in the UTE treatment than that in the CON treatment (Figure 7C). The relative abundance of genera Christensenellaceae R-7group, Ruminococcus 1 and f__Ruminococcaceae/g_ in the ileum was higher (P < 0.05) in the CTE treatment than that in the CON and UTE treatments (Figures 6D, E, G). The relative abundance of genus Lachnospiraceae NK3A20 group in the ileum was higher (P < 0.05) in the CON treatment than that in the CTE and UTE treatments (Figure 6F). The relative abundance of genus Ruminococcaceae UCG-014 and Lactobacillus in the colon was higher (P < 0.05) in the CTE treatment than that in the CON and UTE treatments (Figures 6H, I).
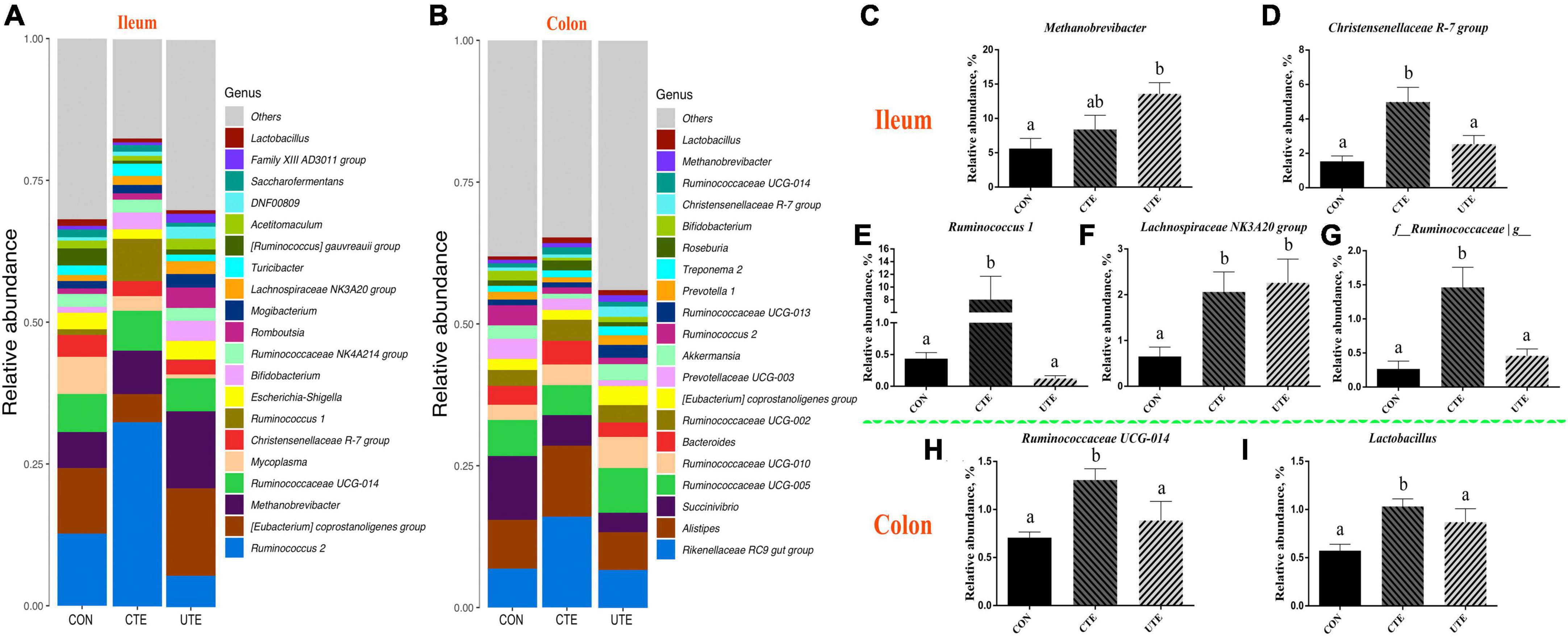
Figure 7. Effects of coated and uncoated trace elements on the relative abundance of bacteria at genus level. (A) The bacterial composition at genus level in the ileum; (B) the bacterial composition at genus level in the colon; (C–G) bacteria with differences in abundance in the ileum at the phylum level; (H–I) bacteria with differences in abundance in the colon at the phylum level. CON, sheep fed with the basal diets; CTE, sheep fed with the basal diets plus 400 mg/kg coated trace elements; UTE, sheep fed with the basal diets plus an equal amount of trace elements in uncoated form. Data are represented as mean and standard error and with different small letter superscripts mean significantly different (P < 0.05), n = 4.
3.10 Prediction of bacterial function
The effect of coated and uncoated trace elements on the prediction of function of bacteria in the ileum and colon were shown in Supplementary Tables 3, 4. The main gene functions of bacteria in the ileum associated with translation (∼5.7%), replication and repair (∼5.5%) and drug resistance: antineoplastic (∼5.0%), as shown in Supplementary Table 3. The main gene functions of bacteria in the colon are associated with immune system (∼5.2%), replication and repair (∼5.0%) and drug resistance: antineoplastic (∼5.0%), as shown in Supplementary Table 4. As shown in Figure 8, the relative abundance of transcription and cellular community of bacteria in the ileum was higher (P < 0.05) in the UTE treatment than that in the CON treatment, the relative abundance of transport and catabolism of bacteria in the ileum was higher (P < 0.05) in the UTE treatment than that in the CTE treatment. Compared with the CON and UTE treatments, the relative abundance of aging and substance dependence of bacteria in the ileum was lower (P < 0.05) in the UTE treatment, and the relative abundance of immune system and nervous system of bacteria in the ileum was higher (P < 0.05) in the UTE treatment. For the gene functions of bacteria in the colon, the relative abundance of development and regeneration was lower (P < 0.05) in the CTE treatment than that in the CON and UTE treatments, the relative abundance of infectious disease: parasitic was lower (P < 0.05) in the CTE treatment than that in the UTE treatment.
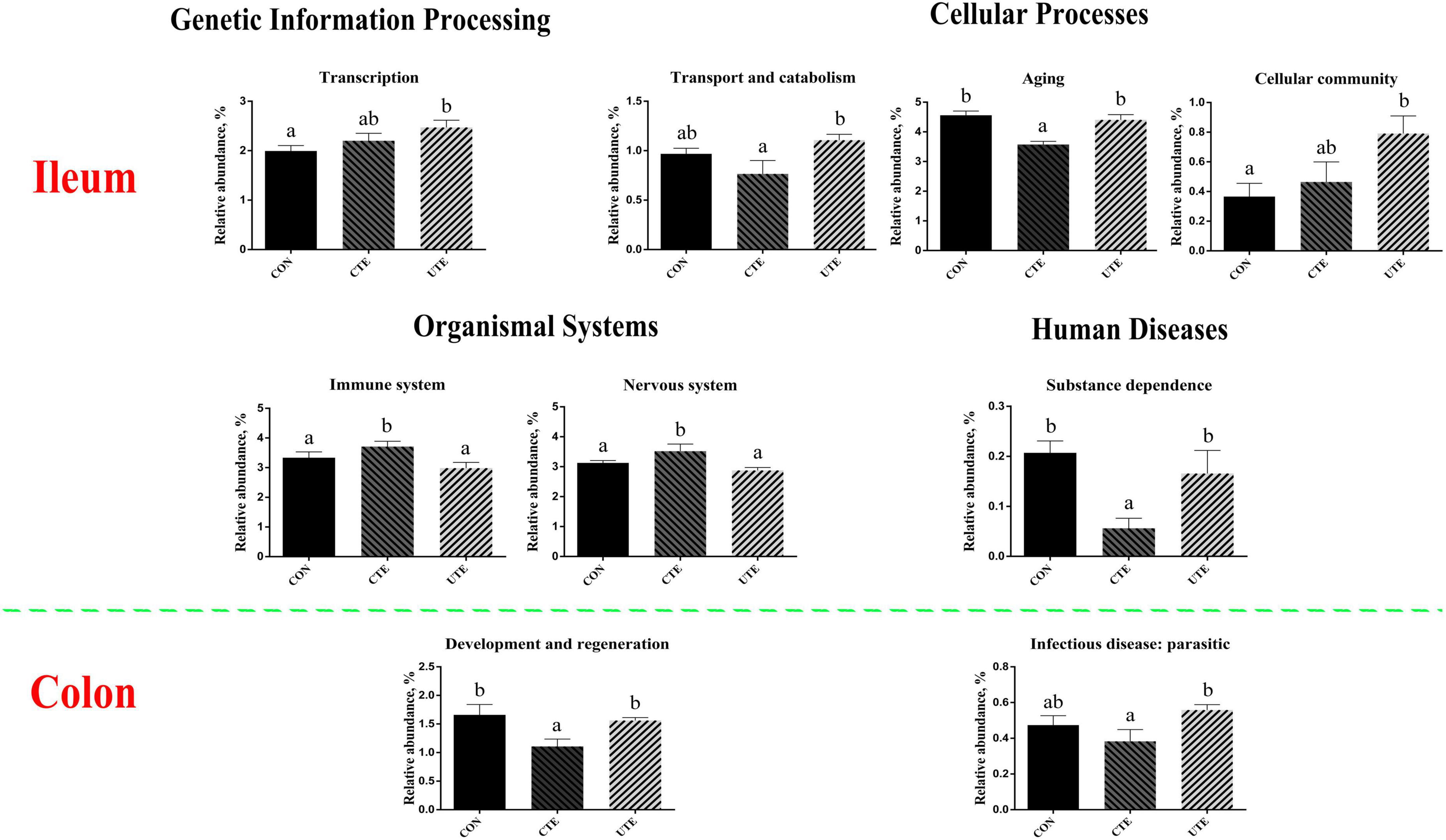
Figure 8. The prediction of bacterial function based on Kyoto Encyclopedia of Genes and Genomes (KEGG) pathway at level 2. CON, sheep fed with the basal diets; CTE, sheep fed with the basal diets plus 400 mg/kg coated trace elements; UTE, sheep fed with the basal diets plus an equal amount of trace elements in uncoated form. Data are represented as mean and standard error and with different small letter superscripts mean significantly different (P < 0.05), n = 4.
4 Discussion
Due to its easier absorption, it is generally accepted that organic trace elements are used more efficiently than inorganic trace elements for ruminants (Byrne and Murphy, 2022). Besides organic trace elements, coating technique may be an effective measure to improve the bioavailability of inorganic trace elements. In the current study, the effective degradability of the coated trace elements was determined to be 34.13% in Exp. 1, indicating that most of them (65.87%) could escape from the rumen and enter the subsequent gastrointestinal tract. In Exp. 2, the ADG of sheep fed the basal diet without the addition of exogenous trace elements was significantly lower than that of sheep fed the basal diet with coated or uncoated trace elements. This result may be caused by insufficient trace elements in the basal diet (mainly composed of corn, soybean meal, heat bran, corn silage and wheat straw) to meet the requirements of the growing Yunnan semi-fine wool sheep. There is well-established evidence that the deficiency of trace elements can negatively affect the growth of animals (Prasad, 1995; Eder et al., 1996; Kegley et al., 2016; Huang et al., 2017), as trace elements are involved in maintaining normal intestinal absorption and microbial homeostasis (Pajarillo et al., 2021; Skalny et al., 2021). The measurement of serum mineral concentrations is a popular and potentially valuable method for evaluating the trace mineral nutritional status and the mineral bioavailability of animals (Herdt and Hoff, 2011; Burrow et al., 2020). Thus, we measured the levels of trace elements in serum and found that the levels of Se, Co and I in the serum of sheep supplemented with coated trace elements were increased compared to CON and UTE treatments. The rumen binding of abundant fiber components and minerals in ruminant feeds may affect the subsequent absorption of some trace elements in the gut (Whitehead et al., 1985). Under the present experimental conditions, increasing the rumen passage rate appears to be an effective strategy to improve the bioavailability of trace elements. Compared with the addition of coated trace elements, the growth performance of sheep fed with uncoated trace elements did not show an advantage. This may be due to the fact that trace elements added as salt also provide sufficient amounts of minerals to growing sheep, after all, they remain the main mineral providers in livestock feed, albeit with relatively low bioavailability.
Some researchers believe that in ruminants, trace elements in the form of salts are more soluble in the rumen, chemical forms of trace mineral elements can be altered, and adverse reactions between rumen contents can affect rumen fermentation patterns, potentially affecting digestibility of nutrients (Galbraith et al., 2016; Arce-Cordero et al., 2020; Miller et al., 2020). In recent years, attempts have been made to replace mineral salts with trace elements in their hydroxyl or coating form. Addition of hydroxy trace minerals improved the digestibility of nutrients in dairy cows (Miller et al., 2020; Guimaraes et al., 2022) and steers (Caldera et al., 2019; Guimaraes et al., 2021) compared to supplementation of sulfate-sourced trace elements. However, Miller et al. (2020) reported that the source of trace mineral (sulfate or hydroxy source) had no effect on rumen turnover, and particle passage rates in Holstein cows. Arce-Cordero et al. (2020) also reported that dietary rumen-protected copper sulfate did not affect the ruminal digestibility of nutrients compared to unprotected copper sulfate. In this study, we found that the addition of coated or uncoated trace elements had no effect on apparent nutrient digestibility, with the exception of CP digestibility. The apparent digestibility of CP was higher in the CTE and UTE treatments than that in the CON treatment, which was consistent with the result of growth performance. In accordance with our findings, previous studies have shown higher digestibility of CP in dairy cows (El Ashry et al., 2012), goats (Salama et al., 2003) and growing lambs (Samarin et al., 2022) supplemented with a mix of trace minerals from different sources. Trace elements have been proven to play a critical role in regulating intestinal health and the microbiome (Shannon and Hill, 2019; Barra et al., 2021), which may be factors affecting nutrient digestibility.
The morphology of the intestinal mucosa is an important indicator of intestinal absorption function in ruminant livestock. Most absorption of trace minerals occurs in the small intestine, mainly in the duodenum, although absorption can occur anywhere in the gastrointestinal tract (Smith, 2004; Goff, 2018). The sheep in the CTE treatment had a greater villus height in the duodenum, jejunum and ileum than those sheep in the CON and UTE treatments, indicating that dietary coated trace elements were beneficial to improve the absorption function of small intestinal. Previous studies reported that a single supplement of dietary trace minerals had a positive effect on the gut health of animals, such as Fe (Zhou et al., 2020), Cu (Yin et al., 2021), Zn (Wang et al., 2018) and Se (Samo et al., 2020). Replacing inorganic trace elements with complex organic trace minerals in the diet could increase the villus height in the ileum of weaned piglets (Wang et al., 2022). The addition of coated organic trace elements could also significantly improve the villus height and villus/crypt ratio in late-phase laying hens (Chen et al., 2022). In addition to intestinal morphology, we also detected the gene expression of tight junction protein in small intestine and found that dietary coated trace elements upregulated the relative mRNA abundance of tight junction proteins (Claudin-1, Claudin-2, Occludin, ZO-1, and ZO-2) in duodenum and jejunum compared to dietary uncoated trace elements. Altering the supply form and amount of Zn could upregulate the gene expression of Occludin and ZO-1 in ileal mucosa, but did not affect intestinal mucosal morphology (Wang et al., 2018). The deficiency or excess of trace elements can regulate the microenvironment of animals’ intestines, including the microbiome, nutrient availability, stress and mucosal immunity (Pajarillo et al., 2021). This could be the reason that the addition of trace elements improved the apparent digestibility of the crude protein. In this study, the supply of equal amounts of uncoated trace elements limited the biological effects in the small intestine due to low bioavailability. The absorption of metal ions by intestinal epithelial cells depends on divalent metal transporters, which are then transported to the blood by transmembrane proteins such as ferroportin and zinc transporters (Hundal and Taylor, 2009; Espinoza et al., 2012). The addition of trace elements had a positive effect on the relative mRNA abundance of iron transporter and zinc transporter (FPN1 and ZNT4), especially coated trace elements. This may be related to the amount of trace elements reaching the small intestine.
Some trace minerals ingested by animals are utilized by gut microbiota to maintain basic physiological activity, although hosts have evolved mechanisms that prevent bacteria from taking advantage of them through chelation (Becker and Skaar, 2014). Meanwhile, trace minerals also regulate the composition and diversity of intestinal microorganisms. Previous studies have reported that Fe could regulate the ability of microorganisms to obtain energy from nutrients ingested by hosts (Dostal et al., 2015), Mn could regulate the detoxification involved in reactive oxygen species, carbohydrates, lipids, and protein metabolism, and DNA replication (Kehres and Maguire, 2003; Zaharik and Brett Finlay, 2004), Zn could regulate host defenses, antioxidant systems, gene expression, virulence factors (Velasco et al., 2018; Eijkelkamp et al., 2019), and Cu could reduce the relative abundance of potential pathogens (Villagómez-Estrada et al., 2020). These are all involved in the survival and adaptation of the microbiome. In this study, the most dominant bacterial phylum in ileum and colon was Firmicutes, which is consistent with previous studies in sheep (Wang et al., 2017; Ma et al., 2022). Compared with the CON treatment, the addition of uncoated trace elements increased the proportion of the phylum Euryarchaeota, and the change of the abundance of Kiritimatiellaeota was the most obvious with the addition of trace elements. Euryarchaeota is the phylum of Archaea, including some methane-producing bacteria commonly found in the gut, known for metabolizing nutrients and metabolites of other bacteria, leading to elevated levels of short-chain fatty acids, such as acetate (Horz and Conrads, 2010; Primec et al., 2019). Dietary zinc oxide supplementation significantly increased the abundance of Euryarchaeota in the colon, while other information about the association between Euryarchaeota and trace minerals has been rarely reported. Kiritimatiellaeota mediated pyruvate to generate acetyl-CoA and then enter the tricarboxylic acid (TCA) cycle and involve arginine biosynthesis, and also regulated fatty acid synthesis. Kiritimatiellaeota mediates the breakdown of pyruvate into acetyl-CoA, which then enters the tricarboxylic acid (TCA) cycle and involves arginine biosynthesis to utilize dietary N and produce energy (Guo et al., 2021). However, the cause for the addition of trace elements to reduce the proportion of Kiritimatiellaeota in the ileum need to be further studied. Compared to uncoated trace elements, the addition of coated trace elements increased the proportion of the phylum Firmicutes while decreased the proportion of the phylum Proteobacteria. The proportion of dominant bacterial phyla in the ileum was significantly affected by whether or not it is coated, probably caused by the coating technique changing the amount of trace elements reaching the ileum. At the genus level, the proportion of Christensenellaceae R-7 group, f_ Ruminococcaceae/g_ and Ruminococcus 1 in the ileum was positively affected by the addition of coated trace elements. Christensenellaceae R-7 group play a pivotal role in the degradation of carbohydrates to acetate (Morotomi et al., 2012), and the increase in their abundance was positively correlated with the improvement of growth performance and meat quality in Hu lambs (Xiong et al., 2021). Both f_ Ruminococcaceae/g_ and Ruminococcus 1 belong to family Ruminococcaceae, which have great ability in the complex carbohydrates digestion to generate short chain fatty acids (SCFAs) (Xie et al., 2022). It has been reported that SCFAs can improve intestinal health by maintaining the integrity of intestinal barrier, secreting mucus and preventing inflammation (Peng et al., 2009; Lewis et al., 2010; Primec et al., 2017). The similar result appeared in the colon that the addition of coated trace elements increased the proportion of Ruminococcaceae UCG-014.
To further explore the role of microbiota in gut, the prediction of bacterial function based on KEGG pathway was performed. Findings of the present study revealed significant differences in bacterial function between sheep supplemented with coated trace elements or not. In the ileal microbiota of sheep fed with coated trace elements, there were more genes associated with the organism systems (immune system and nervous system), which critically influence the homeostasis of normal physiological functions of the host (Xing et al., 2019). Homeostasis of the gut microbiota play an important role in host health and aging (Han et al., 2017). The genes associated with aging were fewer in the CTE treatment than that in the UTE treatment, indicating that feeding coated trace elements is more beneficial for gut health in sheep. In the colon, the genes related to development and regeneration, infectious disease: parasitic were also fewer in the CTE treatment than that in the UTE treatment. Both substance dependence and infectious disease: parasitic belong to the biological pathway of human disease and have obvious associations with host health (Li and Agarwal, 2009). The decreased occurrence of these genes mirrors the decrease in sequences affiliated with intestinal injury, supporting the importance of these bacteria within the gut health. The limitation of this study is that metagenomic shotgun sequencing of gut digesta was not performed, which will provide more accurate and direct evidence for the function of gut microbiota in future studies.
5 Conclusion
In summary, most of the coated trace elements (65.87%) used in this experiment were able to escaped from the rumen. Under the dietary conditions of the current study, the addition of trace elements improved the growth performance and the apparent digestibility of CP of growing sheep. The addition of dietary coated trace elements had a more positive effect on serum trace element levels and intestinal development, although there was no difference in growth performance, compared to uncoated trace elements. Generally, this study partly revealed the advantages of coated trace elements over traditional trace elements for intestinal development in growing sheep, providing a theoretical basis for their further utilization on ruminants.
Data availability statement
The datasets presented in this study can be found in online repositories. The names of the repository/repositories and accession number(s) can be found at: https://www.ncbi.nlm.nih.gov/, PRJNA894949.
Ethics statement
This animal study was reviewed and approved by the Experimental Animal Committee of Animal Nutrition Institute, Sichuan Agricultural University.
Author contributions
JZ and YR wrote the original draft, performed data statistical analysis, and produced figures and tables. YR and XW performed the experiments. SY, RH, QP, and YJ provided great help in data analysis. QH, JZ, and BX performed the trial investigation and design. ZW, BX, LW, HZ, and YJ reviewed and edited the draft. All authors contributed to the article and approved the submitted version.
Funding
This research was funded by the China Agriculture Research System of MOF and MARA (CARS-39), the company Fujian Syno Biotech Co., Ltd., and the company Shanghai Menon Animal Nutrition Technology Co., Ltd. The funder was not involved in the study design, collection, analysis, interpretation of data, the writing of this article, or the decision to submit it for publication.
Acknowledgments
We gratefully acknowledge the laboratory members for their help throughout the experimental process.
Conflict of interest
The authors declare that the research was conducted in the absence of any commercial or financial relationships that could be construed as a potential conflict of interest.
Publisher’s note
All claims expressed in this article are solely those of the authors and do not necessarily represent those of their affiliated organizations, or those of the publisher, the editors and the reviewers. Any product that may be evaluated in this article, or claim that may be made by its manufacturer, is not guaranteed or endorsed by the publisher.
Supplementary material
The Supplementary Material for this article can be found online at: https://www.frontiersin.org/articles/10.3389/fmicb.2022.1080182/full#supplementary-material
Footnotes
- ^ http://www.geneious.com/plugins/flash/
- ^ http://www.usadellab.org/cms/?page=trimmomatic
- ^ http://drive5.com/usearch/manual/uchime_algo.html
- ^ http://drive5.com/uchime/uchime_download.html
- ^ http://drive5.com/uparse/
- ^ http://tax4fun.gobics.de/
- ^ http://www.arb-silva.de/
References
Abdelrahman, M. M., Aljumaah, R. S., and Khan, R. U. (2017). Effects of prepartum sustained-release trace elements ruminal bolus on performance, colustrum composition and blood metabolites in Najdi ewes. Environ. Sci. Pollut. Res. 24, 9675–9680. doi: 10.1007/s11356-017-8625-1
Aliarabi, H., Fadayifar, A., Alimohamady, R., and Dezfoulian, A. H. (2019). The effect of maternal supplementation of zinc, selenium, and cobalt as slow-release ruminal bolus in late pregnancy on some blood metabolites and performance of ewes and their lambs. Biol. Trace Elem. Res. 187, 403–410. doi: 10.1007/s12011-018-1409-8
Andreini, C., Bertini, I., Cavallaro, G., Holliday, G. L., and Thornton, J. M. (2008). Metal ions in biological catalysis: From enzyme databases to general principles. JBIC J. Biol. Inorg. Chem. 13, 1205–1218. doi: 10.1007/s00775-008-0404-5
AOAC International (2007). Official methods of analysis, 18th Edn, eds W. Hortwitz and G. W. Latimer Jr. (Rockville, MD: AOAC Int).
Arce-Cordero, J. A., Monteiro, H. F., Lelis, A. L., Lima, L. R., Restelatto, R., Brandao, V. L. N., et al. (2020). Copper sulfate and sodium selenite lipid-microencapsulation modifies ruminal microbial fermentation in a dual-flow continuous-culture system. J. Dairy Sci. 103, 7068–7080. doi: 10.3168/jds.2019-17913
Aßhauer, K. P., Wemheuer, B., Daniel, R., and Meinicke, P. (2015). Tax4Fun: Predicting functional profiles from metagenomic 16S rRNA data. Bioinformatics 31, 2882–2884. doi: 10.1093/bioinformatics/btv287
Barra, N. G., Anhê, F. F., Cavallari, J. F., Singh, A. M., Chan, D. Y., and Schertzer, J. D. (2021). Micronutrients impact the gut microbiota and blood glucose. J. Endocrinol. 250, R1–R21. doi: 10.1530/JOE-21-0081
Becker, K. W., and Skaar, E. P. (2014). Metal limitation and toxicity at the interface between host and pathogen. FEMS Microbiol. Rev. 38, 1235–1249. doi: 10.1111/1574-6976.12087
Bolger, A. M., Lohse, M., and Usadel, B. (2014). Trimmomatic: A flexible trimmer for Illumina sequence data. Bioinformatics 30, 2114–2120. doi: 10.1093/bioinformatics/btu170
Burrow, K., Young, W., McConnell, M., Carne, A., Barr, D., Reid, M., et al. (2020). The effect of sheep and cow milk supplementation of a low calcium diet on the distribution of macro and trace minerals in the organs of weanling rats. Nutrients 12:594. doi: 10.3390/nu12030594
Byrne, L., and Murphy, R. A. (2022). Relative bioavailability of trace minerals in production animal nutrition: A review. Animals (Basel) 12:1981. doi: 10.3390/ani12151981
Caldera, E., Weigel, B., Kucharczyk, V. N., Sellins, K. S., Archibeque, S. L., Wagner, J. J., et al. (2019). Trace mineral source influences ruminal distribution of copper and zinc and their binding strength to ruminal digesta1,2,3. Journal Anim. Sci. 97, 1852–1864. doi: 10.1093/jas/skz072
Caporaso, J. G., Lauber, C. L., Walters, W. A., Berg-Lyons, D., Lozupone, C. A., Turnbaugh, P. J., et al. (2011). Global patterns of 16S rRNA diversity at a depth of millions of sequences per sample. Proc. Natl. Acad. Sci. U.S.A 108, 4516–4522. doi: 10.1073/pnas.1000080107
Chen, J., Bittinger, K., Charlson, E. S., Hoffmann, C., Lewis, J., Wu, G. D., et al. (2012). Associating microbiome composition with environmental covariates using generalized UniFrac distances. Bioinformatics 28, 2106–2113. doi: 10.1093/bioinformatics/bts342
Chen, X., Ma, X.-M., Yang, C.-W., Jiang, S.-Z., Huang, L.-B., Li, Y., et al. (2022). Low level of dietary organic trace elements improve the eggshell strength. trace element utilization, and intestinal function in late-phase laying hens. Front. Vet. Sci. 9:903615. doi: 10.3389/fvets.2022.903615
Dick, A. T., Dewey, D. W., and Gawthorne, J. M. (1975). Thiomolybdates and the copperÔÇômolybdenumÔÇôsulphur interaction in ruminant nutrition. J. Agric. Sci. 85, 567–568. doi: 10.1017/S0021859600062468
Dostal, A., Lacroix, C., Bircher, L., van Pham, T., Follador, R., Zimmermann, M. B., et al. (2015). Iron modulates butyrate production by a child gut microbiota in vitro. mBio 6:e01453. doi: 10.1128/mBio.01453-15
Eder, K., Kralik, A., and Kirchgessner, M. (1996). The effect of manganese supply on thyroid hormone metabolism in the offspring of manganese-depleted dams. Biol. Trace Elem. Res. 55:137. doi: 10.1007/BF02784175
Edgar, R. C. (2013). UPARSE: Highly accurate OTU sequences from microbial amplicon reads. Nat. Methods 10, 996–998. doi: 10.1038/nmeth.2604
Edgar, R. C., Haas, B. J., Clemente, J. C., Quince, C., and Knight, R. (2011). UCHIME improves sensitivity and speed of chimera detection. Bioinformatics 27, 2194–2200. doi: 10.1093/bioinformatics/btr381
Eijkelkamp, B. A., Morey, J. R., Neville, S. L., Tan, A., Pederick, V. G., Cole, N., et al. (2019). Dietary zinc and the control of Streptococcus pneumoniae infection. PLoS Pathog. 15:e1007957. doi: 10.1371/journal.ppat.1007957
El Ashry, G. M., Hassan, A. A. M., and Soliman, S. M. (2012). Effect of feeding a combination of zinc, manganese and copper methionine chelates of early lactation high producing dairy cow. Food Nutr. Sci. 3, 193–203. doi: 10.1016/S0377-8401(01)00279-6
Espinoza, A., Le Blanc, S., Olivares, M., Pizarro, F., Ruz, M., and Arredondo, M. (2012). Iron, copper, and zinc transport: Inhibition of divalent metal transporter 1 (DMT1) and human copper transporter 1 (hCTR1) by shRNA. Biol. Trace Elem. Res. 146, 281–286. doi: 10.1007/s12011-011-9243-2
Faulkner, M. J., and Weiss, W. P. (2017). Effect of source of trace minerals in either forage-or by-product–based diets fed to dairy cows: 1. Production and macronutrient digestibility. J. Dairy Sci. 100, 5358–5367. doi: 10.3168/jds.2016-12095
Foong, J. P. P., Hung, L. Y., Poon, S., Savidge, T. C., and Bornstein, J. C. (2020). Early life interaction between the microbiota and the enteric nervous system. Am. J. Physiol. Gastrointest. Liver Physiol. 319, G541–G548. doi: 10.1152/ajpgi.00288.2020
Galbraith, M. L., Vorachek, W. R., Estill, C. T., Whanger, P. D., Bobe, G., Davis, T. Z., et al. (2016). Rumen microorganisms decrease bioavailability of inorganic selenium supplements. Biol. Trace Elem. Res. 171, 338–343. doi: 10.1007/s12011-015-0560-8
Genther, O. N., and Hansen, S. L. (2015). The effect of trace mineral source and concentration on ruminal digestion and mineral solubility. J. Dairy Sci. 98, 566–573. doi: 10.3168/jds.2014-8624
Goff, J. P. (2018). Invited review: Mineral absorption mechanisms, mineral interactions that affect acid–base and antioxidant status, and diet considerations to improve mineral status. J. Dairy Sci. 101, 2763–2813. doi: 10.3168/jds.2017-13112
Guimaraes, O., Jalali, S., Wagner, J. J., Spears, J. W., and Engle, T. E. (2021). Trace mineral source impacts rumen trace mineral metabolism and fiber digestion in steers fed a medium-quality grass hay diet. J. Anim. Sci. 99, 1–7. doi: 10.1093/jas/skab220
Guimaraes, O., Wagner, J. J., Spears, J. W., Brandao, V. L. N., and Engle, T. E. (2022). Trace mineral source influences digestion, ruminal fermentation, and ruminal copper, zinc, and manganese distribution in steers fed a diet suitable for lactating dairy cows. Animal 16:100500. doi: 10.1016/j.animal.2022.100500
Guo, N., Wu, Q., Shi, F., Niu, J., Zhang, T., Degen, A. A., et al. (2021). Seasonal dynamics of diet-gut microbiota interaction in adaptation of yaks to life at high altitude. NPJ Biofilms Microbiomes 7:38. doi: 10.1038/s41522-021-00207-6
Han, B., Sivaramakrishnan, P., Lin, C.-C. J., Neve, I. A. A., He, J., Tay, L. W. R., et al. (2017). Microbial genetic composition tunes host longevity. Cell 169, 1249–1262.e13. doi: 10.1016/j.cell.2017.05.036
Herdt, T. H., and Hoff, B. (2011). The use of blood analysis to evaluate trace mineral status in ruminant livestock. Vet. Clin. North Am. Food Anim. Pract. 27, 255–283. doi: 10.1016/j.cvfa.2011.02.004
Horz, H.-P., and Conrads, G. (2010). The discussion goes on: What is the role of Euryarchaeota in humans? Archaea 2010:967271. doi: 10.1155/2010/967271
Hou, Y., Wang, L., Ding, B., Liu, Y., Zhu, H., Liu, J., et al. (2010). Dietary α-ketoglutarate supplementation ameliorates intestinal injury in lipopolysaccharide-challenged piglets. Amino Acids 39, 555–564. doi: 10.1007/s00726-010-0473-y
Huang, X., Sun, B., Zhang, J., Gao, Y., Li, G., and Chang, Y. (2017). Selenium deficiency induced injury in chicken muscular stomach by downregulating selenoproteins. Biol. Trace Elem. Res. 179, 277–283. doi: 10.1007/s12011-017-0946-x
Hundal, H. S., and Taylor, P. M. (2009). Amino acid transceptors: Gate keepers of nutrient exchange and regulators of nutrient signaling. Am. J. Physiol. Endocrinol. Metab. 296, E603–E613. doi: 10.1152/ajpendo.91002.2008
Kasaikina, M. V., Kravtsova, M. A., Lee, B. C., Seravalli, J., Peterson, D. A., Walter, J., et al. (2011). Dietary selenium affects host selenoproteome expression by influencing the gut microbiota. FASEB J. 25, 2492–2499. doi: 10.1096/fj.11-181990
Kegley, E. B., Ball, J. J., and Beck, P. A. (2016). BILL E. KUNKLE INTERDISCIPLINARY BEEF SYMPOSIUM: Impact of mineral and vitamin status on beef cattle immune function and health1,2. J. Anim. Sci. 94, 5401–5413. doi: 10.2527/jas.2016-0720
Kehres, D. G., and Maguire, M. E. (2003). Emerging themes in manganese transport, biochemistry and pathogenesis in bacteria. FEMS Microbiol. Rev. 27, 263–290. doi: 10.1016/S0168-6445(03)00052-4
Kembel, S. W., Cowan, P. D., Helmus, M. R., Cornwell, W. K., Morlon, H., Ackerly, D. D., et al. (2010). Picante: R tools for integrating phylogenies and ecology. Bioinformatics 26, 1463–1464. doi: 10.1093/bioinformatics/btq166
Kociova, S., Dolezelikova, K., Horky, P., Skalickova, S., Baholet, D., Bozdechova, L., et al. (2020). Zinc phosphate-based nanoparticles as alternatives to zinc oxide in diet of weaned piglets. J. Anim. Sci. Biotechnol. 11:59. doi: 10.1186/s40104-020-00458-x
Lewis, K., Lutgendorff, F., Phan, V., Söderholm, J., Sherman, P., and McKay, D. (2010). Enhanced translocation of bacteria across metabolically stressed epithelia is reduced by butyrate. Inflamm. Bowel Dis. 16, 1138–1148. doi: 10.1002/ibd.21177
Li, Y., and Agarwal, P. (2009). A pathway-based view of human diseases and disease relationships. PLoS One. 4:e4346. doi: 10.1371/journal.pone.0004346
Liu, X., Piao, J., Huang, Z., Zhang, S.-Q., Li, W., Tian, Y., et al. (2014). Determination of 16 selected trace elements in children plasma from china economical developed rural areas using high resolution magnetic sector inductively coupled mass spectrometry. J. Anal. Methods Chem. 2014:975820. doi: 10.1155/2014/975820
Liu, Y. J., Zhang, Z. D., Dai, S. H., Wang, Y., Tian, X. F., Zhao, J. H., et al. (2020). Effects of sodium selenite and coated sodium selenite addition on performance, ruminal fermentation, nutrient digestibility and hepatic gene expression related to lipid metabolism in dairy bulls. Livest. Sci. 237:104062. doi: 10.1016/j.livsci.2020.104062
Ma, Y., Deng, X., Yang, X., Wang, J., Li, T., Hua, G., et al. (2022). Characteristics of bacterial microbiota in different intestinal segments of aohan fine-wool sheep. Front. Microbiol. 13:874536. doi: 10.3389/fmicb.2022.874536
Miller, M. D., Lanier, J. S., Kvidera, S. K., Dann, H. M., Ballard, C. S., and Grant, R. J. (2020). Evaluation of source of corn silage and trace minerals on rumen characteristics and passage rate of Holstein cows. J. Dair. Sci. 103, 8864–8879. doi: 10.3168/jds.2020-18490
Morotomi, M., Nagai, F., and Watanabe, Y. (2012). Description of Christensenella minuta gen. nov., sp. nov., isolated from human faeces, which forms a distinct branch in the order Clostridiales, and proposal of Christensenellaceae fam. nov. Int. J. Syst. Evol. Microbiol. 62, 144–149. doi: 10.1099/ijs.0.026989-0
National Research Council (2007). Nutrient requirements of small ruminants: Sheep, goats, cervids, and new world camelids. Washington, DC: The National Academies Press.
Ørskov, E. R., and McDonald, I. (1979). The estimation of protein degradability in the rumen from incubation measurements weighted according to rate of passage. J. Agric. Sci. 92, 499–503. doi: 10.1017/S0021859600063048
Overton, T. R., and Yasui, T. (2014). Practical applications of trace minerals for dairy cattle. J. Anim. Sci. 92, 416–426. doi: 10.2527/jas.2013-7145
Pajarillo, E. A. B., Lee, E., and Kang, D.-K. (2021). Trace metals and animal health: Interplay of the gut microbiota with iron, manganese, zinc, and copper. Anim. Nutr. 7, 750–761. doi: 10.1016/j.aninu.2021.03.005
Peng, L., Li, Z.-R., Green, R. S., Holzman, I. R., and Lin, J. (2009). Butyrate enhances the intestinal barrier by facilitating tight junction assembly via activation of AMP-activated protein kinase in Caco-2 cell monolayers. J. Nutr. 139, 1619–1625. doi: 10.3945/jn.109.104638
Potier, R., Blanc, B., Braud, C., Freret, S., and Cesbron, N. (2022). Effect of sustained-release trace element ruminal bolus on plasma trace mineral profiles in captive blesbok antelopes (Damaliscus pygargus phillipsi). J. Zoo Wildl. Med. 53, 259–265. doi: 10.1638/2021-0120
Primec, M., Klemenak, M., Di Gioia, D., Aloisio, I., Bozzi Cionci, N., Quagliariello, A., et al. (2019). Clinical intervention using Bifidobacterium strains in celiac disease children reveals novel microbial modulators of TNF-α and short-chain fatty acids. Clin. Nutr. 38, 1373–1381. doi: 10.1016/j.clnu.2018.06.931
Primec, M., Mičetić-Turk, D., and Langerholc, T. (2017). Analysis of short-chain fatty acids in human feces: A scoping review. Anal. Biochem. 526, 9–21. doi: 10.1016/j.ab.2017.03.007
Rabiee, A. R., Lean, I. J., Stevenson, M. A., and Socha, M. T. (2010). Effects of feeding organic trace minerals on milk production and reproductive performance in lactating dairy cows: A meta-analysis. J. Dairy Sci. 93, 4239–4251. doi: 10.3168/jds.2010-3058
Salama, A. A. K., Caja, G., Albanell, E., Such, X., Casals, R., and Plaixats, J. (2003). Effects of dietary supplements of zinc-methionine on milk production, udder health and zinc metabolism in dairy goats. J. Dairy Res. 70, 9–17. doi: 10.1017/s0022029902005708
Samarin, A. A., Norouzian, M. A., and Afzalzadeh, A. (2022). Effect of trace mineral source on biochemical and hematological parameters, digestibility, and performance in growing lambs. Trop. Anim. Health Prod. 54:40. doi: 10.1007/s11250-021-03042-1
Samo, S. P., Malhi, M., Kachiwal, A. B., Gadahi, J. A., Parveen, F., Kalhoro, N. H., et al. (2020). Supranutritional selenium level minimizes high concentrate diet-induced epithelial injury by alleviating oxidative stress and apoptosis in colon of goat. BMC Vet. Res. 16:462. doi: 10.1186/s12917-020-02653-4
Shannon, M. C., and Hill, G. M. (2019). Trace mineral supplementation for the intestinal health of young monogastric animals. Front. Vet. Sci. 6:73. doi: 10.3389/fvets.2019.00073
Skalny, A. V., Aschner, M., Lei, X. G., Gritsenko, V. A., Santamaria, A., Alekseenko, S. I., et al. (2021). Gut microbiota as a mediator of essential and toxic effects of zinc in the intestines and other tissues. Int. J. Mol. Sci. 22:13074. doi: 10.3390/ijms222313074
Smith, D. (2004). Minerals in animal and human nutrition (2003), 2nd edn. Trop. Anim. Health Prod. 36:774. doi: 10.1023/B:TROP.0000046014.56139.49
Spears, J. W. (1996). Organic trace minerals in ruminant nutrition. Anim. Feed Sci. Technol. 58, 151–163. doi: 10.1016/0377-8401(95)00881-0
Spears, J. W. (2003). Trace mineral bioavailability in ruminants. J. Nutr. 133, 1506S–1509S. doi: 10.1093/jn/133.5.1506S
Spears, J. W., and Weiss, W. P. (2008). Role of antioxidants and trace elements in health and immunity of transition dairy cows. Vet. J. 176, 70–76. doi: 10.1016/j.tvjl.2007.12.015
Stein, H. H., and Bohlke, R. A. (2007). The effects of thermal treatment of field peas (Pisum sativum L.) on nutrient and energy digestibility by growing pigs. J. Anim. Sci. 85, 1424–1431. doi: 10.2527/jas.2006-712
van Soest, P. J., Robertson, J. B., and Lewis, B. A. (1991). Methods for dietary fiber, neutral detergent fiber, and nonstarch polysaccharides in relation to animal nutrition. J. Dairy Sci. 74, 3583–3597. doi: 10.3168/jds.S0022-0302(91)78551-2
Velasco, E., Wang, S., Sanet, M., Fernández-Vázquez, J., Jové, D., Glaría, E., et al. (2018). A new role for Zinc limitation in bacterial pathogenicity: Modulation of α-hemolysin from uropathogenic Escherichia coli. Sci. Rep. 8:6535. doi: 10.1038/s41598-018-24964-1
Villagómez-Estrada, S., Pérez, J. F., Darwich, L., Vidal, A., van Kuijk, S., Melo-Durán, D., et al. (2020). Effects of copper and zinc sources and inclusion levels of copper on weanling pig performance and intestinal microbiota. J. Anim. Sci. 98:skaa117. doi: 10.1093/jas/skaa117
Wang, C., Han, L., Zhang, G. W., Du, H. S., Wu, Z. Z., Liu, Q., et al. (2021). Effects of copper sulphate and coated copper sulphate addition on lactation performance, nutrient digestibility, ruminal fermentation and blood metabolites in dairy cows. Br. J. Nutr. 125, 251–259. doi: 10.1017/S0007114520002986
Wang, E., Wang, J., Lv, J., Sun, X., Kong, F., Wang, S., et al. (2021). Comparison of ruminal degradability, indigestible neutral detergent fiber, and total-tract digestibility of three main crop straws with alfalfa hay and corn silage. Animals (Basel) 11:3218. doi: 10.3390/ani11113218
Wang, C., Zhang, L., Ying, Z., He, J., Zhou, L., Zhang, L., et al. (2018). Effects of dietary zinc oxide nanoparticles on growth, diarrhea, mineral deposition, intestinal morphology, and barrier of weaned piglets. Biol. Trace Elem. Res. 185, 364–374. doi: 10.1007/s12011-018-1266-5
Wang, H., Yang, F., Xin, R., Cui, D., He, J., Zhang, S., et al. (2020). The gut microbiota attenuate neuroinflammation in manganese exposure by inhibiting cerebral NLRP3 inflammasome. Biomed. Pharm. 129:110449. doi: 10.1016/j.biopha.2020.110449
Wang, J., Fan, H., Han, Y., Zhao, J., and Zhou, Z. (2017). Characterization of the microbial communities along the gastrointestinal tract of sheep by 454 pyrosequencing analysis. Asian Australas. J. Anim. Sci. 30, 100–110. doi: 10.5713/ajas.16.0166
Wang, S., Wu, S., Zhang, Y., Chen, J., and Zhou, X. (2022). Effects of different levels of organic trace minerals on oxidative status and intestinal function in weanling piglets. Biol. Trace Elem. Res. doi: 10.1007/s12011-022-03174-x
Whitehead, D. C., Goulden, K. M., and Hartley, R. D. (1985). The distribution of nutrient elements in cell wall and other fractions of the herbage of some grasses and legumes. J. Sci. Food Agric. 36, 311–318. doi: 10.1002/jsfa.2740360502
Wu, Q., La, S. K., Wang, C., Zhang, J., Liu, Q., Guo, G., et al. (2021). Effects of coated copper sulphate and coated folic acid supplementation on growth, rumen fermentation and urinary excretion of purine derivatives in Holstein bulls. Anim. Feed Sci. Technol. 276:114921. doi: 10.1016/j.anifeedsci.2021.114921
Xie, J., Li, L.-f, Dai, T.-Y., Qi, X., Wang, Y., and Zheng, T.-z, et al. (2022). Short-chain fatty acids produced by ruminococcaceae mediate α-linolenic acid promote intestinal stem cells proliferation. Mol. Nutr. Food Res. 66:e2100408. doi: 10.1002/mnfr.202100408
Xing, H., Chen, J., Peng, M., Wang, Z., Liu, F., Li, S., et al. (2019). Identification of signal pathways for immunotoxicity in the spleen of common carp exposed to chlorpyrifos. Ecotoxicol. Environ. Safety 182:109464. doi: 10.1016/j.ecoenv.2019.109464
Xiong, Y., Guo, C., Wang, L., Chen, F., Dong, X., Li, X., et al. (2021). Effects of paper mulberry silage on the growth performance, rumen microbiota and muscle fatty acid composition in Hu Lambs. Fermentation 7:286. doi: 10.3390/fermentation7040286
Yin, L., Yang, Q., Zhang, Y., Wan, D., Yin, Y., Wang, Q., et al. (2021). Dietary copper improves intestinal morphology via modulating intestinal stem cell activity in Pigs. Animals (Basel) 11:2513. doi: 10.3390/ani11092513
Zaharik, M. L., and Brett Finlay, B. (2004). Mn2+ and bacterial pathogenesis. FBL 9, 1035–1042. doi: 10.2741/1317
Zhang, R., Gu, J., Wang, X., Li, Y., Liu, J., Lu, C., et al. (2019). Response of antibiotic resistance genes abundance by graphene oxide during the anaerobic digestion of swine manure with copper pollution. Sci. Total Environ. 654, 292–299. doi: 10.1016/j.scitotenv.2018.11.094
Zhang, Z. D., Wang, C., Du, H. S., Liu, Q., Guo, G., Huo, W. J., et al. (2020). Effects of sodium selenite and coated sodium selenite on lactation performance, total tract nutrient digestion and rumen fermentation in Holstein dairy cows. Animal 14, 2091–2099. doi: 10.1017/S1751731120000804
Zhao, Z. W., Ma, Z. Y., Wang, H. C., and Zhang, C. F. (2022). Effects of trace minerals supply from rumen sustained release boluses on milk yields and components, rumen fermentation and the rumen bacteria in lactating yaks (Bos grunniens). Anim. Feed Sci. Technol. 283:115184. doi: 10.1016/j.anifeedsci.2021.115184
Zhou, J., Ding, Z., Pu, Q., Xue, B., Yue, S., Guan, S., et al. (2022). Rumen fermentation and microbiome responses to enzymatic hydrolysate of cottonseed protein supplementation in continuous in vitro culture. Animals (Basel) 12:2113. doi: 10.3390/ani12162113
Zhou, J., Dong, Z., Wan, D., Wang, Q., Haung, J., Huang, P., et al. (2020). Effects of iron on intestinal development and epithelial maturation of suckling piglets. J. Anim. Sci. 98:skaa213. doi: 10.1093/jas/skaa213
Keywords: trace elements, sheep, growth performance, intestinal development, gut microbiota
Citation: Zhou J, Ren Y, Wen X, Yue S, Wang Z, Wang L, Peng Q, Hu R, Zou H, Jiang Y, Hong Q and Xue B (2022) Comparison of coated and uncoated trace elements on growth performance, apparent digestibility, intestinal development and microbial diversity in growing sheep. Front. Microbiol. 13:1080182. doi: 10.3389/fmicb.2022.1080182
Received: 26 October 2022; Accepted: 01 December 2022;
Published: 20 December 2022.
Edited by:
Junhua Liu, Nanjing Agricultural University, ChinaCopyright © 2022 Zhou, Ren, Wen, Yue, Wang, Wang, Peng, Hu, Zou, Jiang, Hong and Xue. This is an open-access article distributed under the terms of the Creative Commons Attribution License (CC BY). The use, distribution or reproduction in other forums is permitted, provided the original author(s) and the copyright owner(s) are credited and that the original publication in this journal is cited, in accordance with accepted academic practice. No use, distribution or reproduction is permitted which does not comply with these terms.
*Correspondence: Bai Xue, ✉ MTM1OTJAc2ljYXUuZWR1LmNu
†These authors have contributed equally to this work and share first authorship