- 1Institute of Evolution and Marine Biodiversity, Ocean University of China, Qingdao, China
- 2Laboratory of Protozoological Biodiversity and Evolution in Wetland, College of Life Sciences, Shaanxi Normal University, Xi’an, China
- 3Division of Invertebrate Zoology, American Museum of Natural History, New York, NY, United States
- 4Sackler Institute for Comparative Genomics, American Museum of Natural History, New York, NY, United States
Forming cysts is a common and important bionomic strategy for microorganisms to persist in harsh environments. In ciliated protists, many species have been reported to form cysts when facing unfavorable conditions. Despite traditional studies on the morphological features of cysts and the chemical composition of cyst wall, recent research has focused more on the molecular mechanisms of encystment. The present work reviews studies on developmental features and molecular information of resting cysts in ciliates, and pays more attention to the following questions: what are the inducing factors of encystment and excystment? How does the cell change morphologically during these dynamic processes? And what molecular mechanisms underlie those changes? We also present and summarize the characteristics of cysts from diverse ciliate lineages in a phylogenetic framework, aiming to provide new perspectives for studies on adaptive evolution of unicellular eukaryotes.
Introduction
Cyst formation, which is common in microbial organisms, is considered as an adaptive strategy against adverse environmental conditions (Corliss and Esser, 1974). The process of encystment and excystment (i.e., the E-E cycle) involves dramatic structural changes, including cell volume decrease, cyst wall (CW) formation, nuclear fusion (of some species) and ciliature resorption and regeneration (Gutiérrez et al., 1990). Additionally, the E-E cycle can differ from species to species in many aspects, for example, by the morphology of mature cysts and by the manner of escaping from the CW during excystment.
Ciliates are a hyper-diverse group of unicellular organisms and an essential component of microbial food webs (Bai et al., 2020; Ma et al., 2020; Wu et al., 2020; Zhang et al., 2020; Zhao et al., 2020, 2021; Chi et al., 2021; Wang C. et al., 2021). They are characterized by possessing two distinct types of nuclei, the somatic macronucleus and the germline micronucleus, within one cell (Prescott, 1994; Sheng et al., 2020). Despite the diverse life history, ciliates mainly reproduce sexually (i.e., conjugation) and asexually (i.e., binary fission) (Prescott, 1994; Chi et al., 2020; Gao et al., 2020). Ciliates are extremely widespread across various habitats and environmental conditions (Wang J. et al., 2021), including ephemeral vernal pools and hot springs (Kahan, 1972; Reid and John, 1983). They are capable of turning into cryptobiotic forms when facing unfavorable conditions (Beers, 1927; Repak, 1968; Gutiérrez et al., 1990; Foissner et al., 2005), among which cyst formation is a common way to engage into resting and resistant stages and to support cell dispersion (Farmer, 1980).
Research on ciliate cysts started in the mid-19th century, Claparède and Lachmann (1858) reported the first description of cysts from a shelled choreotrich ciliate Amphorides amphora and other tintinnids. Hereafter, the structural changes, and more recently, molecular mechanisms of the E-E cycle in ciliates have been investigated through light and electron microscopy, various staining methods, molecular techniques and ’omic analyses (e.g., Pan et al., 2019). These studies suggest that ciliates can be coaxed into cysts by various factors and the morphology of cysts vary among species (van Wagtendonk, 1955; Corliss and Esser, 1974). In addition, the expression level of related proteins might be up-regulated or down-regulated, promoting cells to form cysts or to detach from the CW during excystment as they return to suitable environments (Chen et al., 2014; Gao et al., 2015).
Given the long history of studying microorganisms in dormant stages, these stages have been reported under numerous names, such as spores, sporulation, stomatocysts, or cysts (Benítez and Gutiérrez, 1997; Foissner, 2006). The definitions given to these terms vary among studies, which means that the same word might refer to different types of cysts in different investigations. For example, forming reproductive cysts is a necessary stage in some ciliates’ life cycle (Xu et al., 2007), which is different from resting cysts. In addition, for those species undergoing asexual reproduction solely through reproductive cysts, they usually form cysts under sufficient nutrition, which is distinct from resting cyst formation under harsh environment (e.g., lack of nutrition) (Benčat’ová et al., 2020).
In the present work, we largely focus on resting cysts and choose “cyst” as the general term, which consists of resting cysts (including temporary cysts), reproductive cysts, digestive cysts, etc. We aim to summarize the basic structure, inducing factors, structural changes and responding molecular mechanisms in resting cysts.
The Ability to Encyst
Extreme caution needs to be taken in determining whether or not a species is capable of encysting and excysting. There could be several reasons why a species has yet been reported to form cysts: (1) the species may be poorly studied, (2) the E-E cycle may rarely occur in this species. Therefore, the ability and opportunity to identify the onset of the E-E cycle are slim, especially if cyst formation is not being the species’ primary way of surviving harsh environment, (3) lastly, it is possible that this species cannot form cysts.
With the above being said, we can still review the literature and gain some hints on which ciliate groups or species are more likely to form cyst and why. For example, a number of soil-living ciliates, represented by colpodids, can transform into resting cysts promptly against desiccation, temperature fluctuations and acid (Matsuoka et al., 2017). Some ciliates achieve survival in tide pools by alternating between cysts and free-swimming forms synchronously with tides (Jonsson, 1994; Montagnes et al., 2002). Forming cysts is also essential for lineages that live in vernal pools or other environments where the pH, temperature and salinity fluctuates significantly.
Structure of Cysts
To date, cysts from about 40 ciliate species have been well documented, though most studies focused predominantly on cyst morphology (Verni and Rosati, 2011; Figure 1).
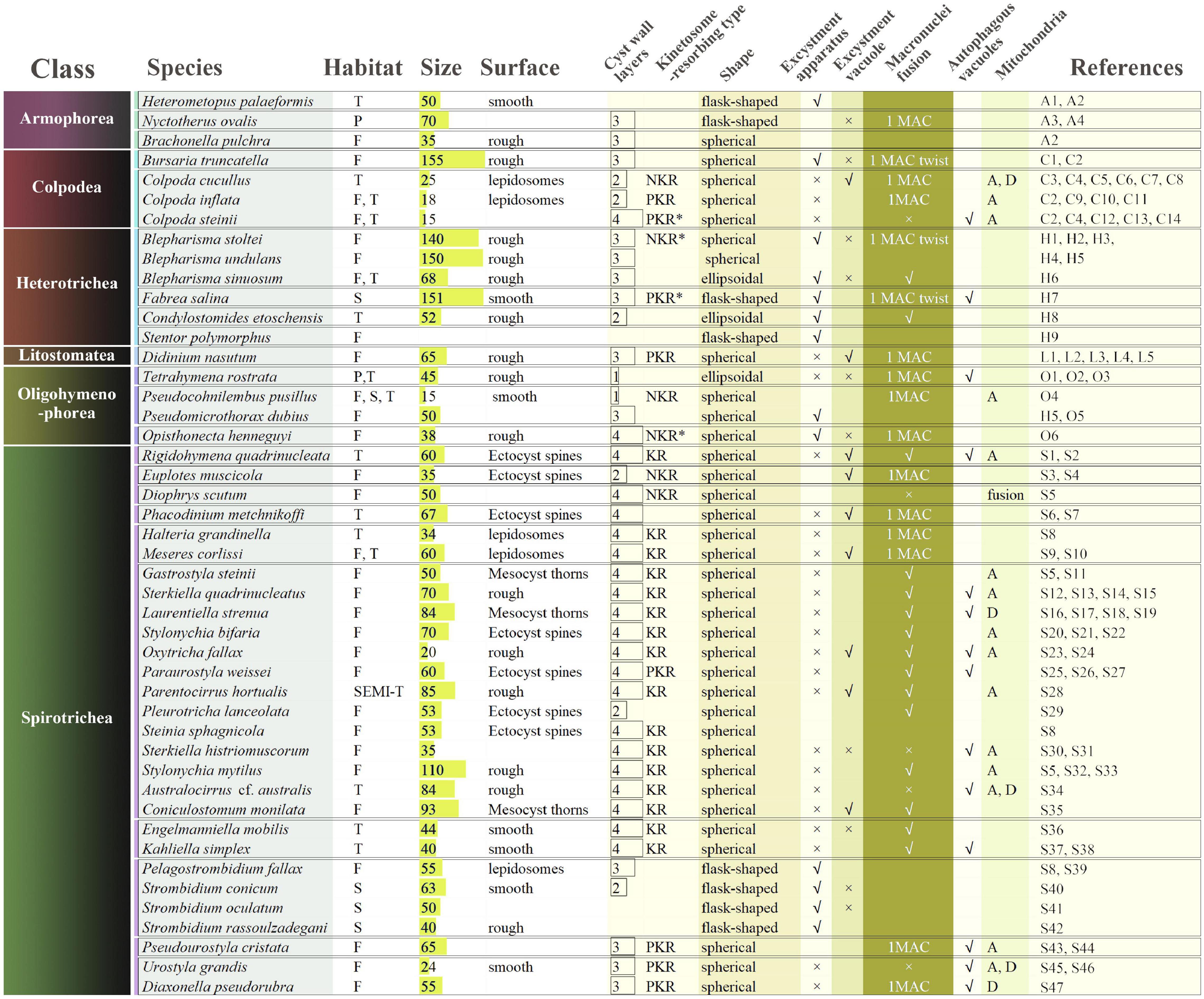
Figure 1. Comparison of morphological characteristics of resting cysts of 46 ciliate species representing 6 classes. T, terrestrial; P, parasitic; F, fresh water; S, sea water; SEMI-T, semi- terrestrial; A, aggregation; D, degeneration; ∗, classified by the present work. The detailed information of the references is shown in Supplementary Material.
Most cysts are spherical (Mulisch and Hausmann, 1989; Figures 2, 3) or ellipsoidal (Foissner et al., 2002), although flask-shape (Kim and Taniguchi, 1995) or disk-shaped (Gurdebeke et al., 2018) cysts have also been observed in several species. The size of cysts ranges from 15 μm in Pseudocohnilembus pusillus (Olendzenski, 1999) to 225 μm in Blepharisma japonicum (Giese, 1973). In most cases, the volume of cysts is much smaller than vegetative cells (Foissner et al., 2006) with the exception of Strombidium oculatum (Jonsson, 1994). Despite a few species that form colored cysts resulting from food or pigment granules, cysts of most species are colorless (Repak, 1968; Foissner et al., 2007; Benčat’ová et al., 2016; Benčat’ová and Tirjaková, 2017; Cavaleiro et al., 2018).
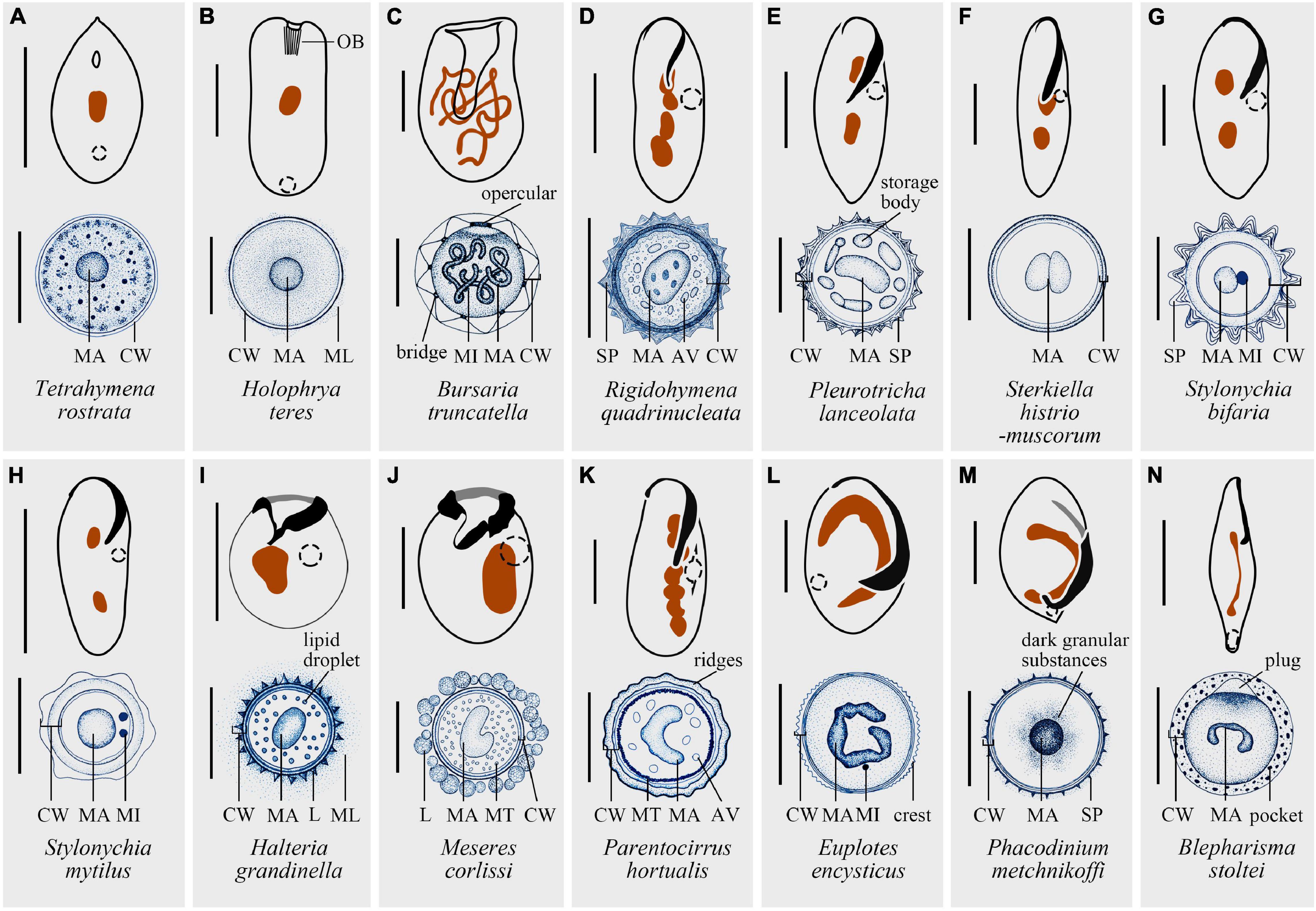
Figure 2. Structure diagrams of resting cysts and vegetative cells of 14 representative species. (A) Tetrahymena rostrata, modified after Stout (1954) and Zebrun et al. (1967). (B) Holophrya teres, modified after Benčat’ová et al. (2020) and Foissner (2021). (C) Bursaria truncatella: bridge, between the inner and outer cyst walls; opercular, upon emergence pore; modified after Beers (1948) and Krause and Braucker (2009). (D) Rigidohymena quadrinucleata, modified after Benčat’ová and Tirjaková (2017) and Wang J. et al. (2017). (E) Pleurotricha lanceolata: storage bodies, discontinuous ring of hyaline “lakes” in protoplast; modified after Manwell (1928), Jeffries (1956), Jeffries and Mellott (1968), and Dragesco (1972). (F) Sterkiella histriomuscorum, modified after Adl and Berger (1997), Grisvard et al. (2008) and Jiang et al. (2013). (G) Stylonychia bifaria, modified after Ricci et al. (1985), Wirnsberger et al. (1985) and Verni and Rosati (2011). (H) Stylonychia mytilus, modified after Jones (1974), Walker et al. (1975) and Zhang and Pang (1981). (I) Halteria grandinella, modified after Song (1993) and Foissner et al. (2007). (J) Meseres corlissi, modified after Petz and Foissner (1992) and Foissner et al. (2005). (K) Parentocirrus hortualis: ridges, generated by the whole cyst wall; modified after Voß (1997) and Benčat’ová et al. (2016). (L) Euplotes encysticus, modified after Rawlinson and Gates (1985), Gu and Zhang (1992), Gu and Xu (1995) and Fan et al. (2010). (M) Phacodinium metchnikoffi: dark granular substances, assumed to be the reserve products; modified after Fernandez-Galiano and Calvo (1992), de Pablo (2010) and Benčat’ová and Tirjaková (2018). (N) Blepharisma stoltei: pockets, intrinsic part of the outer wall and appearing as disk-like structures; modified after Repak and Pfister (1967), Repak (1968) and Chi et al. (2021). MA, macronucleus; CW, cyst wall; OB, oral basket; ML, mucous layer; SP, spines; AV, autophagic vacuoles; L, lepidosomes; MT, mitochondrium. Scale bars: 30 μm (A,F,G,I,J,L), 50 μm (B,D,E,K,M), 100 μm (C,H,N).
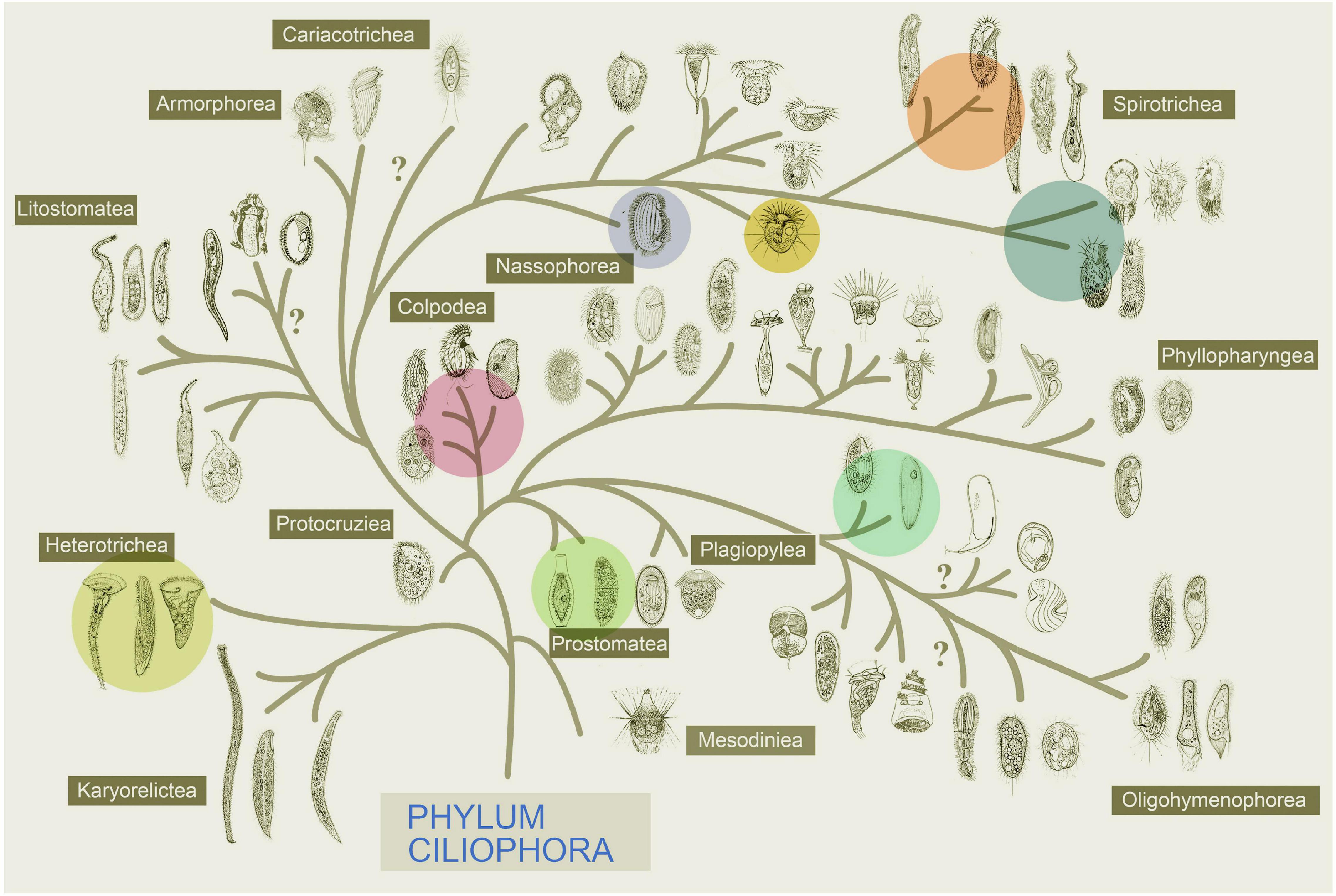
Figure 3. Systematic positions of the 14 species described in Figure 2, of which 5 classes within the phylum Ciliophora are represented (marked in color, modified after Gao et al., 2016).
One of the crucial structures of cysts is the CW. CW has almost always been described as multiple layers (e.g., reviewed in Li et al., 2017), for example, two layers in Euplotes muscicola (Rawlinson and Gates, 1985), three layers in Urostyla grandis (Liu et al., 2009) and four layers in Parentocirrus hortualis (Benčat’ová et al., 2016). Here we follow Foissner’s (2005) extensive description of CW on Meseres corlissi and depict CW as five distinct layers, naming metacyst, endocyst, mesocyst, ectocyst, and pericyst, from touching the cell body to the outmost layer. Metacyst is located closest to the cell body and composed of fibrous material. Endocyst does not bear a clear structure and is the thickest layer of CW. Both mesocyst and ectocyst are built by fine fibers with different arrangement, while the scale-like lepidosomes is the main component of pericyst (Foissner, 2005). Pericyst can be adhesive, trapping bacteria as future food source or sticking to substrates and adjacent cysts (Repak and Pfister, 1967). Additionally, specialized structures like plugs (Repak and Pfister, 1967) and ornamentations were also reported (Foissner et al., 2007).
The internal structure may also differ from vegetative forms. Macronuclear fusion occurs in some multinucleated species (Rosati et al., 1983), while in other species the macronuclei twist together to squeeze into a much smaller space. Changes not only take place in the macronuclei, but also in the micronuclei, which may fuse (Zhang and Pang, 1981), degrade (Grimes, 1973a), or remain unchanged (Olendzenski, 1999).
Although there are fewer observations on the impact of encystment on other intracellular organelles, for some taxa their mitochondria may cluster or remain scattered in the mature cyst (Walker and Maugel, 1980; Verni et al., 1984). For some species, contractile vacuoles disappear, whereas autophagic vacuoles are found intact in resting cysts of many ciliates (Benčat’ová and Tirjaková, 2017; Figures 2, 4).
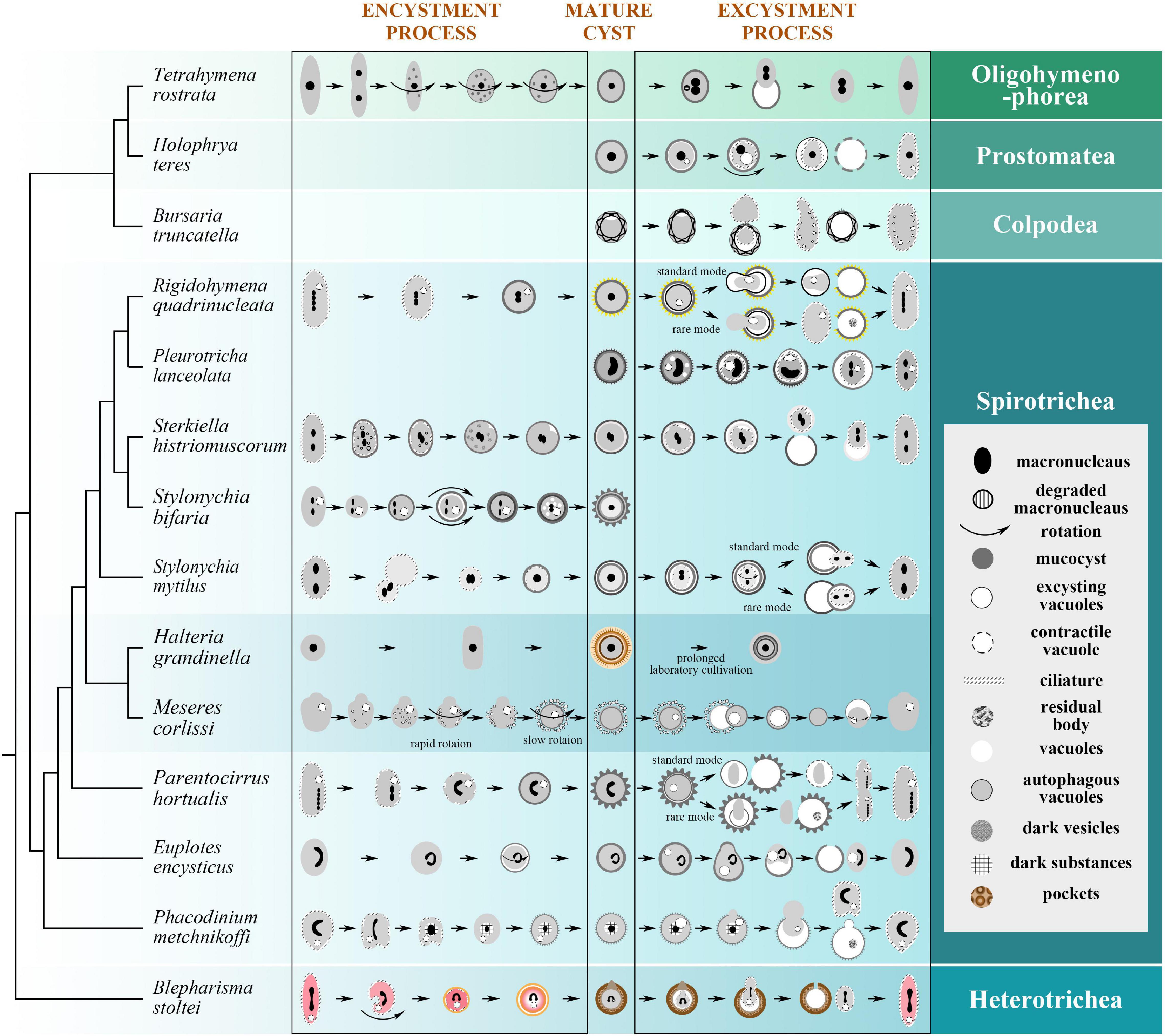
Figure 4. Encystment and/or excystment process of 14 representative species in Figure 2, with their phylogenetic relationships (Lynn, 2008; Berger, 2011; Gao et al., 2016; Wang J. et al., 2017; Wang et al., 2019). During the encystment process, the vegetative cells reduce in size, transform to spherical shape, absorb the ciliary structure, and secrete the CW; during the excystment process, the mature cysts restore some if not all vegetative structures within the CW prior to rupturing the CW and exiting. For the legend: macronuclei are not drawn in all stages for certain species; in Tetrahymena rostrata, the old macronucleus is degraded, while the new macronuclei are produced by the micronuclei; curved arrows indicate rotation and degree; mucocyst is secreted to form the cyst wall; degrading or regenerating ciliature is drawn as broken lines; vacuoles in Parentocirrus hortualis empty into the so-called contractile vacuole; pockets are disk-like structures consisting of unknown material. Tetrahymena rostrata (McArdle et al., 1980; Segade et al., 2016); Holophrya teres (Benčat’ová et al., 2020); Bursaria truncatella (Beers, 1948); Rigidohymena quadrinucleata (Benčat’ová and Tirjaková, 2017); Pleurotricha lanceolata (Jeffries, 1956); Sterkiella histriomuscorum (Adl and Berger, 1997); Stylonychia bifaria (Verni and Rosati, 2011); Stylonychia mytilus (Zhang and Pang, 1981); Halteria grandinella (Foissner et al., 2007); Meseres corlissi (Foissner et al., 2006; Müller, 2007); Parentocirrus hortualis (Benčat’ová et al., 2016); Euplotes encysticus (Rawlinson and Gates, 1985; Gu and Zhang, 1992; Wang B. et al., 2017); Phacodinium metchnikoffi (Benčat’ová and Tirjaková, 2018); Blepharisma stoltei (Repak, 1968).
Factors Required for Encystment and Excystment
Factors Inducing Encystment
Resting cysts can not only be spontaneously generated in nature (Ricci et al., 1985; Olendzenski, 1999), but also be induced to form under laboratory conditions (Arroyo-Begovich and Cárabez-Trejo, 1982). It has been suggested that no single inducer is effective to all ciliates (Corliss and Esser, 1974), and one species may require multiple factors to form cysts (with exceptions, see below; Barker and Taylor, 1931; Johnson and Evans, 1941; van Wagtendonk, 1955).
Nutritional deficiency is a dominant factor in inducing cyst formation. This has been evident by the intracristal inclusions of mitochondria (Vickerman, 1960; Bowers and Korn, 1969), nucleolar fusion (Raikov, 1982; Frenkel, 1992), and decrease of RNA synthesis (Gutiérrez et al., 1990), found in both cysted and starved vegetive cells, indicating the connection between encystment and starvation. It is noteworthy that not only general food insufficiency can induce cyst formation, lack of specific food source, such as vitamins, can also lead to encystment (Garnjobst, 1947; van Wagtendonk, 1955; Corliss and Esser, 1974). Interstingly, in Pelagostrombidiurn fallax, excess food (Rhodomonas sp.) besides starvation can lead to cyst formation (Müller, 1996).
Other conditions, including unfavorable changes in temperature (Müller and Wünsch, 1999; Kim et al., 2002), freezing (Uspenskaya and Lozina-Lozinsky, 1979), humidity (Gutiérrez et al., 2001), salinity (Li et al., 2017), ultraviolet irradiation (Uspenskaya and Lozina-Lozinsky, 1979; Matsuoka et al., 2017), dehydration (Corliss and Esser, 1974; Gutiérrez et al., 2001), and population density (Corliss and Esser, 1974; Matsuoka, 2013; Chen et al., 2018; Sogame et al., 2019) have also been reported to be involved in inducing encystment. Additionally, change in pH (Sogame et al., 2011), concentration of Ca2+ or K+ (Yamaoka et al., 2004; Matsuoka, 2013) and oxygen (Corliss and Esser, 1974) may induce cyst formation in some species as well.
Factors Inducing Excystment
Generally speaking, cells tend to excyst when external environment conditions become more favorable (Zhao et al., 2009; Verni and Rosati, 2011). It has been suggested that sufficient food, optimal temperature and high oxygen concentration would prompt the cells to excyst (van Wagtendonk, 1955; Müller, 2002). The optimal temperature of different species ranges widely from 19.5°C (Gastrostyla stein) to 35°C (Woodrufia metabolica) (Jeffries, 1956). Moreover, cells exposed to low light condition tend not to excyst compared to those under sufficient light (Kamiyama et al., 1995).
On one hand, certain conditions might only work for some species but not others. For instance, increased pH extends the time required for excystment in Stylonychia pustulata and Pleurotricha lanceolate, while it does not influence the process in species such as Colpoda duodenaria and Didinium nasutum (Jeffries, 1956). Whereas, in most cases, exctsyment could be induced by re-feeding (Rawlinson and Gates, 1985; Benčat’ová et al., 2020). However, the concentration and source of food is important. For example, the addition of a moderate concentration of phytoplankton facilitates excystment in tintinnid ciliates (Kamiyama, 1994), while excessive concentrations of phytoplankton inhibit excystment (Kamiyama, 1997). As for the impact of food source, Jeffries (1956) suggested that dilute plant infusions are often the best for excystment.
Structural Changes During Encystment and Excystment
Changes During Encystment
The process of encystment involves changes not only in shape and the cortex (e.g., CW secretion and ciliature resorption) but also inside the cell (e.g., macronuclear aggregation, organelles clustering) among other characteristic cell recycling and remodeling processes (Walker and Maugel, 1980; Verni et al., 1984; Figures 4, 5).
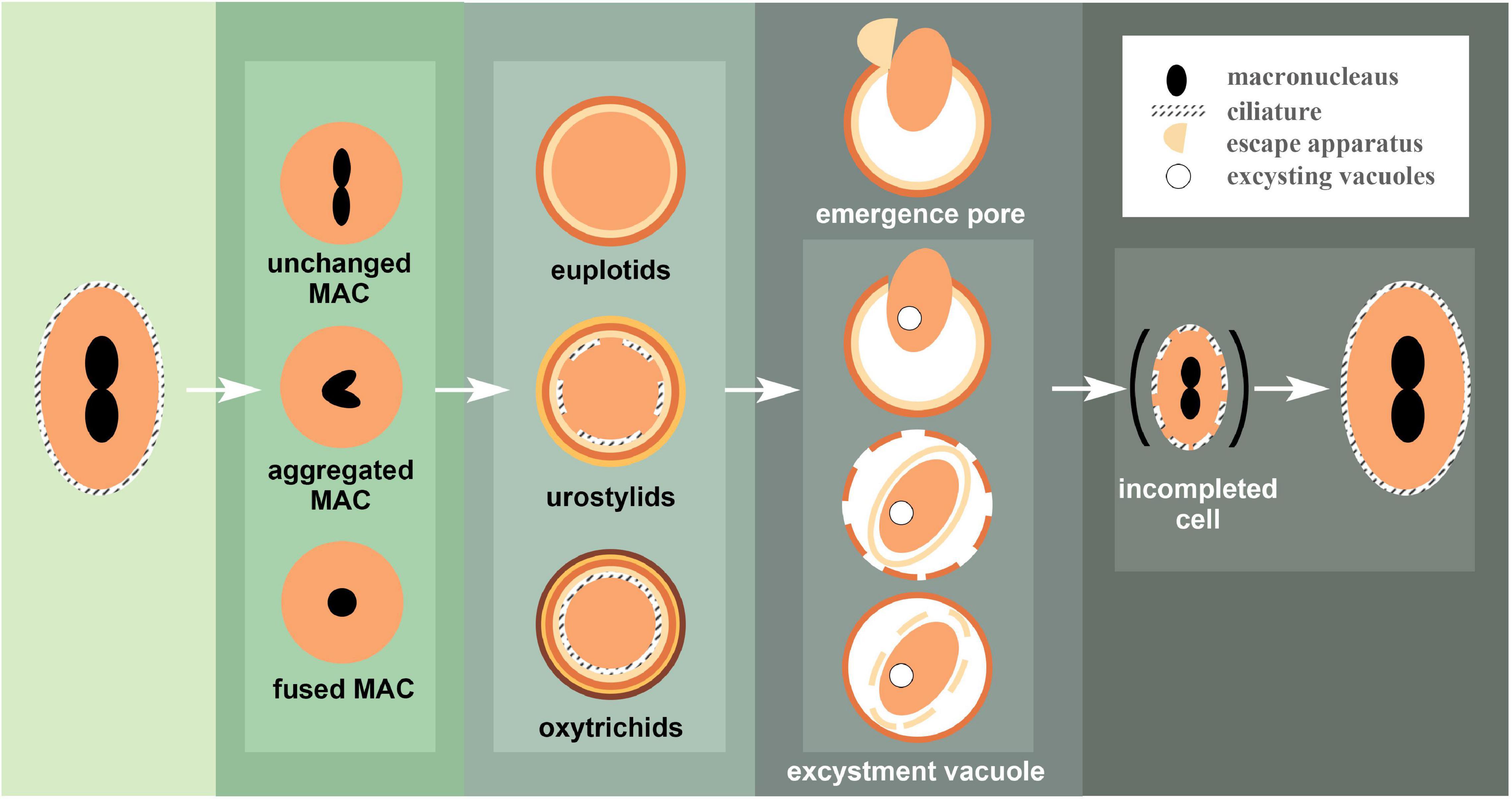
Figure 5. Exemplar patterns of encystment and excystment observed in ciliates. When vegetative cells encyst, the changes in macronuclear morphology are represented by three cases. Mature cysts are similarly divided into three categories based on the degree of kinetosomes resorption, which corresponds to the number of cyst wall layers. During excystment, the (in) completed vegetative cell escapes the cyst wall through an escape apparatus or under the pressure of an excystation vacuole.
Encystment generally begins with decreasing cell volume as well as transforming into spherical body shape, either due to cytoplasmic dehydration (Gutiérrez et al., 2001, 2003), or direct excretion of cytoplasmic contents (Zhang and Pang, 1981). This process leads to vast cell surface area reduction, organelle clustering (Martín-González et al., 2001), strong autophagic activity and decrease in the metabolic rate (Gutiérrez et al., 2001). In addition, Ricci et al. (1985) suggested that smaller volume requires less and thinner CW, which may be beneficial for fast CW formation and rapid response to the changing environment.
CW generation is key to the encystment process, which usually initiates after the body shape change (Figure 4). The two most intriguing questions are: (1) which part of the cell generates the CW? and (2) how is this achieved? A series of delicate works by Foissner and co-authors provided a very detailed and systematic description of CW formation in the planktonic ciliate, Meseres corlissi (Foissner, 2005; Foissner et al., 2005, 2006; Foissner and Pichler, 2006). The main components of the CW are the various protein complexes and carbohydrates, which usually exist in the form of chitin (Xu et al., 2007). CWs not only derive from various sources among species (e.g., precursors generated de novo; organelles present in the vegetative cell; combination of precursors and organelles present in the vegetative cell; without recognizable, membrane-bound precursors) (Foissner et al., 2006), but also show different origins for distinct CW layers. That’s to say, for cysts of some species, formation of the multi-layer barrier might depend on more than one secretory organelle, such as stacks of disks for ectocyst, long fibrous bodies for mesocyst, small dense bodies for the granular layers in Oxytricha fallax (Gutiérrez et al., 1983).
Dedifferentiation is another main feature of encystment, which is represented by resorption of the ciliature (Corliss and Esser, 1974). It is widely accepted that ciliate resting cysts are divided into three types based on the extent of ciliature resorption (Li et al., 2017): (a) non-kinetosome-resorbing cysts (NKR), with ciliary shafts (i.e., ciliary structure above kinetosomes) partially dedifferentiated (Chen et al., 2014), which appears in euplotids, nassophorean (Rawlinson and Gates, 1985), and colpodean ciliates (Tibbs, 1968); (b) partial-kinetosome-resorbing (PKR) cysts, found in urostylids and Dileptus visscheri whose oral and somatic ciliature disappear, while some single kinetosomes remain intact (Calvo et al., 2003); (c) kinetosome-resorbing (KR) that appears in the cysts of oxytrichids, with the absence of cilia and even of basal bodies (Grimes, 1973b). Interestingly, there is no apparent connection with ciliates’ phylogenetic position and type/extent of ciliature resorption. Among closely related taxa, ciliature resorption can be particularly different. As seen in Australocirrus cf. australis, disassembled ventral cirri were observed first, followed by the dorsal bristles (Li et al., 2017), while in Sterkiella histriomuscorum, dorsal kineties were found resorbed before its ventral cirri (Adl and Berger, 1997).
In addition to the changes on or above the cortex, various rearrangements have been reported to take place in the macro- and micronuclei. Besides macronuclear fusion, which is described in several species (Kamra and Sapra, 1991; Benčat’ová and Tirjaková, 2017), changes in the macronuclei include the condensation, fusion (Gutiérrez et al., 1998; Popenko et al., 1998; Martín-González et al., 2001) and extrusion (Jeffries, 1956) of chromatin, as well as the segregation, extrusion and fusion of nucleoli (Popenko et al., 1998; Martín-González et al., 2001).
Compared with macronuclei, detailed investigations of micronuclei are challenging, as micronuclei are usually too small to be clearly observed and illustrated during encystment, although Gutiérrez et al. (1998) have described that degradation and chromatin condensation occurs in micronuclei of several species. Furthermore, the numerous envelopes encapsulating micronuclei in the mature cysts may play a role in protecting the micronuclei from autophagy. It has been suggested that the extensive changes in the nuclei serve to reinforce gene-silencing and genome preservation (Gutiérrez et al., 1998).
Expendable organellar materials, such as mitochondria (Funatani et al., 2010) and ribosomes (Grimes, 1973a), can be recycled through autophagy, and new secretory organelles emerged (McArdle et al., 1980), facilitating encystment related functions. It’s suggested that the organelles and other structures in autophagic vacuoles are digested into smaller elements, so as to provide energy and materials for biosynthetic during the E-E cycle.
Changes During Excystment
Contrary to encystment, the process of excystment aims to bring encysted cells back to their vegetative forms when facing favorable environment (Figures 4, 5).
Ciliary structures degenerate during encystment reappear when excysting. For those species with complicated and specialized ciliature (e.g., hypotrichs), the order of ciliary recovery in different cell area varies among species (Grimes, 1973a; Adl and Berger, 1997). Additionally, in some species, the ciliature is fully restored prior to the very first division after escaping from the CW [e.g., Sterkiella histriomuscorum (Adl and Berger, 1997)], while it can take several divisions to return to the original vegetative pattern in other species [e.g., Parentocirrus hortualis (Benčat’ová et al., 2016)].
Similar process occurs inside the cell. Macronuclei that have twisted or merged during encystment restore their original organization, which includes recovering the shape of the nuclei, [e.g., Euplotes encysticus (Rawlinson and Gates, 1985)], and the number of nuclei [e.g., Histriculus similis (Calvo et al., 1988) and Stylonychia mytilus (Zhang and Pang, 1981)]. In addition, chromatin extrusion has been observed in Colpoda inflata, suggesting potential changes in the macronuclear genome after excystment as well (Chessa et al., 2001). The number and distribution of mitochondria and other organelles also return to the vegetative form. For example, in Oxytricha fallax, the mitochondria will swell and disperse subsequently (Grimes, 1973a).
Along with the dynamic changes within the cell, the cell body eventually escapes from the CW. The escape is assisted either by a preformed apparatus (i.e., emergence pore with a removable “plug” or “operculum”) and/or a rupture generated by the pressure of cell movement inside the CW and excystation vacuole, which has been described as the contractile vacuole in Pleurotricha lanceolata (Jeffries, 1956). This process is typically initiated by the active rotation of the cell within the cyst, followed by lifting the plug or operculum, or generation of a rupture of a small pore (at uncertain position) on the CW. Subsequently, the cell body squeezes out through the opening and eventually regains the vegetative form after a period of free swimming.
The fate of the CW after excystment varies greatly among species: (1) the CW as a whole is left behind in the environment; (2) the encysted cell is still enclosed by the inner CW layer when breaking through the outer CW layer, afterward the inner CW layer will be ruptured and/or resorbed later, like in Pleurotricha lanceolate (Jeffries, 1956), Sterkiella histriomuscorum (Adl and Berger, 1997), Coniculostomum monilata (Kamra and Sapra, 1991), and Histriculus similis (Calvo et al., 1988); 3) all CW layers are left behind with well as the material of ruptured inner membrane (Phacodinium metchnikoffi, Benčat’ová and Tirjaková, 2018) in the empty resting cyst (Figure 4). The fate of the CW is not restricted to a single mode in one species. For example, both first and second types exist in Rigidohymena quadrinucleata (Benčat’ová and Tirjaková, 2017) and Stylonychia mytilus cysts (Zhang and Pang, 1981), and both second and third types have been found in Parentocirrus hortualis (Benčat’ová et al., 2016).
Mechanism Involved in Encystment and Excystment
With the development of molecular biology and high throughput sequencing techniques, studies on ciliate cysts have been extended beyond morphological analyses to the exploration of the underlying molecular mechanisms. No evidence of DNA synthesis (i.e., replication) has been revealed during encystment, accompanied by extremely low levels of transcription and translation (Gutiérrez et al., 1990). However, continued protein synthesis and low-level energy metabolism led to the hypothesis that the dormant cysts are not simply “sleeping” (Chen et al., 2014). Here we summarize some important pathways, focusing on signal transduction and metabolism during the E-E cycle.
Mechanisms Involved in Encystment
Signal Transduction
The first question intrigued the researchers is how the cell “senses” the environmental signal and initiates encystment. Several studies presented that the Ca2+/calmodulin pathway may play an important role in signal transduction during encystment (e.g., Pan et al., 2019; Matsuoka, 2021). This signaling pathway has been extensively investigated in two ciliate systems, Pseudourostyla cristata and Colpoda cucullus, which could be induced to encyst with Ca2+ in the culture medium (Matsuoka et al., 2009; Pan et al., 2019). Results from both systems hint that the pathway might be triggered by the increase of intracellular Ca2+, which could result from either an inflow from extracellular environment or a release from intracellular vesicles that store Ca2+ (Matsuoka et al., 2009). Shimada et al. (2021) proposed that, when Colpoda cucullus is induced to encyst by rapidly increase temperature, its transient receptor potential (TRP) channels may sense this stimulation, and inositol trisphosphate (IP3) will induce the release of Ca2+ into the cytoplasm from the endoplasmic reticulum, which is supported by the identification of IP3 receptor calcium ion channel protein in cyst wall proteins (Wang B. et al., 2017).
Subsequently, Ca2+ was suggested to cause an elevation of cyclic adenosine monophosphate (cAMP) concentration by activating adenylate cyclase (Matsuoka, 2021). Through transcriptome analysis, three pathways downstream of cAMP were illustrated: protein kinase A (PKA) related to protein phosphorylation, adenosine monophosphate activated protein kinase (AMPK) pathway related to autophagy, and PI3K/AKT pathway related to metabolism (Jiang et al., 2019).
It is known that PKA can elevate the phosphorylation level in certain proteins, thereby affecting the expression of those proteins. For example, when introducing cAMP into Colpoda cucullus, the phosphorylation level was increase in some proteins, including actin, ribosomal P0 proteins and histone H4 hyperacetylated form (Sogame et al., 2012a,2014). Actin is suggested crucial for the dynamic structural changes during encystment (Matsuoka, 2021). The phosphorylation of actin can lead to polymerization or depolymerization of actin filaments, eventually reflecting in the spherical shape of the mature cysts (Matsuoka, 2021). At the same time, the expression of actin and actin-binding protein were found upregulated in studied lineages during the process (Bouyer et al., 2009; Pan et al., 2019). In addition, the elevation and reorganization of F-actin promote the cell shrinkage and CW generation, respectively (Pan et al., 2019; Matsuoka, 2021).
The AMPK pathway, which could be activated by starvation, further regulates the autophagy through the mammalian target of rapamycin (mTOR) (Jiang et al., 2019) or forkhead box O (FOXO) signaling pathway (Pan et al., 2019). The FOXO signaling pathway involves in the regulation of genes related to stress response processes (e.g., autophagy and antioxidant) during cyst formation in Pseudourostyla cristata (Pan et al., 2019). However, it is noteworthy that AMPK seems to be differentially regulated, i.e., upregulated (Jiang et al., 2019) or downregulated (Pan et al., 2019), in different species during encystment.
Energy Metabolism
Like food vacuoles in vegetative cells, there are autophagy vacuoles in resting cysts, which could digest substances and create energy for cysts (Wu et al., 2004). The respiratory rate of Colpoda steinii decreases to a relatively low level during encystment (Tibbs and Marshall, 1969), and nearly no metabolic activity has been found in the mitochondrial membrane of resting cysts (Funatani et al., 2010; Sogame et al., 2014), which is consistent with the mitochondria aggregation (Figure 1) or decrease (Li et al., 2017).
Corliss and Esser (1974) summarized the reduction of respiration and decrease of enzyme activity in resting cysts of different ciliates. The electrophoresis experiment showed that, some important metabolic-related enzymes in resting cysts, such as ATPase, malic dehydrogenase and glutamic acid dehydrogenase, may own same composition as in vegetative cells, while their activity are drastically reduced (Chen et al., 2005).
The down-regulation of the related material biosynthesis might directly lead to the reduction of energy metabolism. For instance, the reduction of the synthesis of dihydrolipoyl dehydrogenase and isocitrate dehydrogenase involved in the TCA cycle, ATP synthase subunit beta involved in oxidative phosphorylation (Chen et al., 2018), and proteins related to glycolysis/gluconeogenesis (Jiang et al., 2019; Pan et al., 2019), indicates that metabolism of carbohydrate, lipid and amino acid are decreased. On the other hand, several energy metabolism related proteins were detected up-regulated or specifically expressed, such as lysozyme, which are thought to store energy for the cysts, and ε-trimethyllysine hydroxylase, inferred to influence the energy metabolism process (Chen et al., 2014).
Mechanisms Involved in Excystment
Breakdown of CW is an important step during excystment process. Ubiquitin, ubiquitin carboxyl terminal hydrolase family protein and cullin family protein are speculated to participate in the degradation process (Wang B. et al., 2017). Besides, cysteine protease with different functions may also involve in the CW breakdown process or signal transduction pathway that is sensitive to ambient pH (Villalobo et al., 2003).
The homologs of dead box RNA helicases, which regulate transcription and mRNA turnover, were detected 10 min after the induction of excystment in Colpoda (Sogame et al., 2013), indicating the recover of transcription. Comparative transcriptome analyses of Colpoda aspera showed that the synthesis of palmitic acid and its synthetic substrate hexadecanoyl-CoA are both up-regulated when forming resting cysts. Palmitic acid acts as precursor to synthesize other longer fatty acids during vegetative periods, and was speculated to accumulate as fat storage to be used during excystment (Jiang et al., 2019).
Elongation factor 1α (EF-1α) is upregulated or downregulated during encystment process, and downregulated in the excystment process (Sogame et al., 2012b,2013; Chen et al., 2018). As a multifunctional protein, EF-1α may be involved in protein synthesis, proteasomal degradation, nuclear export, as well as actin or microtubule bundling activity (Sogame et al., 2013; Chen et al., 2018), which can partially explain the contradictory results and imply the relationship between EF-1α and the structural changes in the excystment process.
Speculation
Forming cysts is thought to be beneficial for ciliates in several ways, including facilitating survival under adverse environment, promoting dispersion to new conditions and retarding the aging of the population. Dynamic changes presented in morphological and molecular levels are regulated by complex pathways and numerous genes are involved in the regulatory network that we are starting to understand. Intriguing questions like how the different pathways interplay and how the ciliature degenerates and regenerates are waiting to be solved. Further elucidation of the underlying mechanisms of the E-E cycle will provide more insights on reversible cell differentiation in eukaryotes.
Author Contributions
YY contributed to conception and design of the study. YL organized the resources needed for the study. YL wrote the first draft of the manuscript. YL, YW, SZ, XM-A, and YY contributed to manuscript revision and approved the submitted version. All authors contributed to the article and approved the final version.
Funding
This work was supported by the National Natural Science Foundation of China (Project No. 32030015) and the Fundamental Research Funds for the Central Universities to YY (202141007).
Conflict of Interest
The authors declare that the research was conducted in the absence of any commercial or financial relationships that could be construed as a potential conflict of interest.
Publisher’s Note
All claims expressed in this article are solely those of the authors and do not necessarily represent those of their affiliated organizations, or those of the publisher, the editors and the reviewers. Any product that may be evaluated in this article, or claim that may be made by its manufacturer, is not guaranteed or endorsed by the publisher.
Acknowledgments
We would like to thank Prof. Weibo Song Ocean University of China (OUC) for his help with improving our manuscript, and for Mr. Qingxiang Yuan’s help with Figure 2.
Supplementary Material
The Supplementary Material for this article can be found online at: https://www.frontiersin.org/articles/10.3389/fmicb.2022.785502/full#supplementary-material
References
Adl, S. M., and Berger, J. D. (1997). Timing of life cycle morphogenesis in synchronous samples of Sterkiella histriomuscorum. 1. The vegetative cell cycle. Eur. J. Protistol. 33, 99–109. doi: 10.1016/S0932-4739(97)80025-9
Arroyo-Begovich, A., and Cárabez-Trejo, A. (1982). Location on chitin in the cyst wall of Entamoeba invadens with colloidal gold tracers. J. Parasitol. 68, 253–258. doi: 10.2307/3281183
Bai, Y., Wang, R., Song, W., Suzuki, T., and Hu, X. (2020). Redescription of five tintinnine ciliates (Alveolata: Ciliophora: Oligotrichea) from coastal waters of Qingdao, China. Mar. Life Sci. Technol. 2, 209–221.
Barker, H. A., and Taylor, C. V. (1931). A study of the conditions of encystment of Colpoda cucullus. Physiol. Zool. 4, 620–634. doi: 10.1086/physzool.4.4.30151165
Beers, C. D. (1927). Factors involved in encystment in the ciliate Didinium nasutum. J. Morphol. 43, 499–520. doi: 10.1002/jmor.1050430208
Benčat’ová, S., Križanová, F., and Tirjaková, E. (2020). Current knowledge of the starvation and reproduction stages of the limnic species Holophrya teres (Ciliophora: Prostomatea). Biologia 75, 413–422. doi: 10.2478/s11756-019-00311-1
Benčat’ová, S., and Tirjaková, E. (2017). A study on resting cysts of an oxytrichid soil ciliate, Rigidohymena quadrinucleata (Dragesco & Njine, 1971) Berger, 2011 (Ciliophora, Hypotrichia), including notes on its encystation and excystation process. Acta Protozool. 56, 77–91. doi: 10.4467/16890027AP.17.007.7482
Benčat’ová, S., and Tirjaková, E. (2018). Light microscopy observations on the encystation and excystation processes of the ciliate Phacodinium metchnikoffi (Ciliophora, Phacodiniidae), including additional information on its resting cysts structure. Biologia 73, 467–476. doi: 10.2478/s11756-018-0059-9
Benčat’ová, S., Tirjaková, E., and Vïaènı, P. (2016). Resting cysts of Parentocirrus hortualis Voss, 1997 (Ciliophora, Hypotrichia), with preliminary notes on encystation and various types of excystation. Eur. J. Protistol. 53, 45–60. doi: 10.1016/j.ejop.2015.12.003
Benítez, L., and Gutiérrez, J. C. (1997). Encystment-specific mRNA is accumulated in the resting cysts of the ciliate Colpoda inflata. Biochem. Mol. Biol. Int. 41, 1137–1141. doi: 10.1080/15216549700202221
Berger, H. (2011). Monograph of the Gonostomatidae and Kahliellidae (Ciliophora, Hypotricha). Berlin: Springer. doi: 10.1007/978-94-007-0455-8
Bouyer, S., Rodier, M. H., Guillot, A., and Hechard, Y. (2009). Acanthamoeba castellanii: proteins involved in actin dynamics, glycolysis, and proteolysis are regulated during encystation. Exp. Parasitol. 123, 90–94. doi: 10.1016/j.exppara.2009.06.006
Bowers, B., and Korn, E. D. (1969). The fine structure of Acanthamoeba castellanii (Neff strain). II. Encystment. J. Cell Biol. 41, 786–805. doi: 10.1083/jcb.41.3.786
Calvo, P., Fernandez-Aliseda, M. C., Garrido, J., and Torres, A. (2003). Ultrastructure, encystment and cyst wall composition of the resting cyst of the peritrich ciliate Opisthonecta henneguyi. J. Eukaryot. Microbiol. 50, 49–56. doi: 10.1111/j.1550-7408.2003.tb00105.x
Calvo, P., Martin, J., Delgado, P., and Torres, A. (1988). Cortical morphogenesis during excystment in Histriculus similis (Hypotrichida: Oxytrichidae). J. Protozool. 35, 177–181. doi: 10.1111/j.1550-7408.1988.tb04320.x
Cavaleiro, J., Fernandes, N. M., da Silva-Neto, I. D., and Soares, C. A. G. (2018). Resting cysts of the pigmented ciliate Blepharisma sinuosum Sawaya, 1940 (Ciliophora: Heterotrichea). J. Eukaryot. Microbiol. 65, 422–426. doi: 10.1111/jeu.12483
Chen, F., Xue, Y., Pan, N., Bhatti, M. Z., Niu, T., and Chen, J. (2018). New contribution to the morphology and molecular mechanism of Euplotes encysticus encystment. Sci. Rep. 8:12795. doi: 10.1038/s41598-018-31160-8
Chen, J., Gao, X., Wang, B., Chen, F., Wu, N., and Zhang, Y. (2014). Proteomic approach to reveal the proteins associated with encystment of the ciliate Euplotes encysticus. PLoS One 9:e97362. doi: 10.1371/journal.pone.0097362
Chen, J., Li, Y., Zeng, H., and Gu, F. (2005). A comparison of components and their activities of isozymes between resting cyst and vegetative cell of the ciliate Tachysoma pellionella (Protozoa, Ciliophora) (in Cinese). Chin. J. Cell Biol. 27, 590–592.
Chessa, M. G., Gallus, L., Tiano, L., Trielli, F., and Corrado, M. U. D. (2001). Variations in macronuclear chromatin structure and chromatin extrusion in excystment from resting cysts of Colpoda inflata. Eur. J. Protistol. 37, 281–290. doi: 10.1078/0932-4739-00825
Chi, Y., Chen, X., Li, Y., Wang, C., Zhang, T., Ayoub, A. L., et al. (2021). New contributions to the phylogeny of the ciliate class Heterotrichea (Protista, Ciliophora): analyses at family-genus level and new evolutionary hypotheses. Sci. China Life Sci. 64, 606–620. doi: 10.1007/s11427-020-1817-5
Chi, Y., Duan, L. L., Luo, X. T., Cheng, T., Warren, A., Huang, J., et al. (2020). A new contribution to the taxonomy and molecular phylogeny of three freshwater, well-known species of the ciliate genus Spirostomum (Protozoa: Ciliophora: Heterotrichea). Zool. J. Linn. Soc. 189, 158–177.
Claparède, E., and Lachmann, J. (1858). Études sur les infusoires et les rhizopodes. Genève: Mémoires de l’Institut Genèvois.
Corliss, J. O., and Esser, S. C. (1974). Comments on the role of the cyst in the life cycle and survival of free-living protozoa. Trans. Am. Micros. Soc. 93, 578–593. doi: 10.2307/3225158
de Pablo, P. C. (2010). Caracterización Filogenética de Phacodinium Metchnikoffi: Análisis Comparativo de Datos Morfológicos, Morfogenéticos y Moleculares. Madrid: Universidad Comlutense de Madrid.
Fan, X., Huang, J., Lin, X., Li, J., Al-Rasheid, K. A. S., and Hu, X. (2010). Morphological and molecular characterization of Euplotes encysticus (Protozoa: Ciliophora: Euplotida). J. Mar. Biol. Assoc. U.K. 90, 1411–1416. doi: 10.1017/S002531541000038X
Farmer, J. N. (1980). “The protozoa,” in Introduction to Protozoology, ed. J. N. Farmer (London: The C.V. Mosby Company), 265–273.
Fernandez-Galiano, D., and Calvo, P. (1992). Redescription of Phacodinium metchnikoffi (Ciliophora, Hypotrichida): general morphology and taxonomic position. J. Protozool. 39, 443–448. doi: 10.1111/j.1550-7408.1992.tb04829.x
Foissner, W. (2005). The unusual, lepidosome-coated resting cyst of Meseres corlissi (Ciliophora: Oligotrichea): transmission electron microscopy and phylogeny. Acta Protozool. 44, 217–230.
Foissner, W. (2006). Biogeography and dispersal of micro-organisms: a review emphasizing protists. Acta Protozool. 45, 111–136. doi: 10.1021/jp805737a
Foissner, W. (2021). A detailed description of a Brazilian Holophrya teres (Ehrenberg, 1834) and nomenclatural revision of the genus Holophrya (Ciliophora, Prostomatida). Eur. J. Protistol. 80:125662. doi: 10.1016/j.ejop.2019.125662
Foissner, W., Agatha, S., and Berger, H. (2002). Soil ciliates (Protozoa, Ciliophora) From Namibia (Southwest Africa), With Emphasis On Two Contrasting Environments, The Etosha Region And The Namib Desert. Linz: Oberösterreichisches Landesmuseum.
Foissner, W., Müller, H., and Agatha, S. (2007). A comparative fine structural and phylogenetic analysis of resting cysts in oligotrich and hypotrich Spirotrichea (Ciliophora). Eur. J. Protistol. 43, 295–314. doi: 10.1016/j.ejop.2007.06.001
Foissner, W., Müller, H., and Weisse, T. (2005). The unusual, lepidosome-coated resting cyst of Meseres corlissi (Ciliophora: Oligotrichea): light and scanning electron microscopy, cytochemistry. Acta Protozool. 44, 201–215.
Foissner, W., and Pichler, M. (2006). The unusual, lepidosome-coated resting cyst of Meseres corlissi (Ciliophora: Oligotrichea): genesis of four complex types of wall precursors and assemblage of the cyst wall. Acta Protozool. 45, 339–366.
Foissner, W., Pichler, M., Al-Rasheid, K. A. S., and Weisse, T. (2006). The unusual, lepidosome-coated resting cyst of Meseres corlissi (Ciliophora: Oligotrichea): encystment and genesis and release of the lepidosomes. Acta Protozool. 45, 323–338. doi: 10.1186/1475-2859-5-32
Frenkel, M. A. (1992). Fine structure of the macronucleus in the resting cysts of the Ciliate Tillina magna. Arch. Protistendk. 141, 27–40. doi: 10.1016/S0003-9365(11)80046-1
Funatani, R., Kida, A., Watoh, T., and Matsuoka, T. (2010). Morphological events during resting cyst formation in the ciliate Colpoda cucullus. Protistology 6, 204–217.
Gao, F., Warren, A., Zhang, Q., Gong, J., Miao, M., Sun, P., et al. (2016). The all-data-based evolutionary hypothesis of ciliated protists with a revised classification of the phylum Ciliophora (Eukaryota, Alveolata). Sci. Rep. 6:24874. doi: 10.1038/srep24874
Gao, X., Chen, F., Niu, T., Qu, R., and Chen, J. (2015). Large-scale identification of encystment-related proteins and genes in Pseudourostyla cristata. Sci. Rep. 5:11360. doi: 10.1038/srep11360
Gao, Y. Y., Gong, R. T., Jiang, Y. H., Pan, B., Li, Y., Warren, A., et al. (2020). Morphogenetic characters of the model ciliate Euplotes vannus (Ciliophora, Spirotrichea): notes on cortical pattern formation during conjugational and postconjugational reorganization. Eur. J. Protistol. 73:125675.
Garnjobst, L. (1947). The effect of certain deficient media on resting cyst formation in Colpoda duodenaria. Physiol. Zool. 20, 5–14. doi: 10.1086/physzool.20.1.30151935
Giese, A. C. (1973). Blepharisma: The Biology of a Light Sensitive Protozoa. Stanford: Stanford University Press.
Grimes, G. W. (1973a). Differentiation during encystment and excystment in Oxytricha fallax. J. Protozool. 20, 92–104. doi: 10.1111/j.1550-7408.1973.tb06009.x
Grimes, G. W. (1973b). Morphological discontinuity of kinetosomes during the life cycle of Oxytricha fallax. J. Cell Biol. 57, 229–232. doi: 10.1083/jcb.57.1.229
Grisvard, J., Lemullois, M., Morin, L., and Baroin-Tourancheau, A. (2008). Differentially expressed genes during the encystment–excystment cycle of the ciliate Sterkiella histriomuscorum. Eur. J. Protistol. 44, 278–286. doi: 10.1016/j.ejop.2008.02.003
Gu, F., and Xu, J. (1995). A TEM study on pre-excystment cellular structures of Euplotes encysticus. Cell Res. 5, 125–133. doi: 10.1038/cr.1995.12
Gu, F., and Zhang, Z. (1992). Studies on the macronucleus and micronucleus during encystment and excystment in Euplotes Encystius (in Chinese). Acta Zool. Sin. 38, 208–213.
Gurdebeke, P. R., Mertens, K. N., Takano, Y., Yamaguchi, A., Bogus, K., Dunthorn, M., et al. (2018). The affiliation of Hexasterias problematica and Halodinium verrucatum sp. nov. to ciliate cysts based on molecular phylogeny and cyst wall composition. Eur. J. Protistol. 66, 115–135. doi: 10.1016/j.ejop.2018.09.002
Gutiérrez, J. C., Callejas, S., Borniquel, S., Benítez, L., and Martín-González, A. (2001). Ciliate cryptobiosis: a microbial strategy against environmental starvation. Int. Microbiol. 4, 151–157. doi: 10.1007/s10123-001-0030-3
Gutiérrez, J. C., Diaz, S., Ortega, R., and Martín-González, A. (2003). Ciliate resting cyst walls: a comparative review. Recent Res. Dev. Microbiol. 7, 361–379.
Gutiérrez, J. C., Martín-González, A., and Callejas, S. (1998). Nuclear changes, macronuclear chromatin reorganization and DNA modifications during ciliate encystment. Eur. J. Protistol. 34, 97–103. doi: 10.1016/S0932-4739(98)80018-7
Gutiérrez, J. C., Martín-González, A., and Matsusaka, T. (1990). Towards a generalized model of encystment (cryptobiosis) in ciliates: a review and a hypothesis. Biosystems 24, 17–24. doi: 10.1016/0303-2647(90)90025-v
Gutiérrez, J. C., Torres, A., and Pérez-Silva, J. (1983). Structure of cyst wall precursors and kinetics of their appearance during the encystment of Laurentiella acuminata (Hypotrichida, Oxytrichidae). J. Protozool. 30, 226–233. doi: 10.1111/j.1550-7408.1983.tb02908.x
Jeffries, W. B. (1956). Studies on excystment in the hypotrichous ciliate Pleurotricha lanceolata. J. Protozool. 3, 136–144. doi: 10.1111/j.1550-7408.1956.tb02448.x
Jeffries, W. B., and Mellott, J. L. (1968). New observations on the anatomy of Pleurotricha lanceolata. J. Protozool. 15, 741–747. doi: 10.1111/j.1550-7408.1968.tb02204.x
Jiang, C., Wei, W., Yan, G., Shi, T., and Miao, W. (2019). Transcriptome analysis reveals the molecular mechanism of resting cyst formation in Colpoda aspera. J. Eukaryot. Microbiol. 66, 212–220. doi: 10.1111/jeu.12643
Jiang, J., Ma, H., and Shao, C. (2013). Morphology and morphogenesis of Sterkiella histriomuscorum (Ciliophora, Hypotricha) (in Chinese). Acta Hydrobiol. Sin. 37, 227–234. doi: 10.7541/2013.9
Johnson, W. H., and Evans, F. R. (1941). A further study of environmental factors affecting cystment in Woodruffia metabolica. Physiol. Zool. 14, 227–237. doi: 10.1086/physzool.14.2.30161741
Jones, E. E. (1974). The Protozoa of Mobile Bay, Alabama. Mobile: University of South Alabama Monographs.
Jonsson, P. R. (1994). Tidal rhythm of cyst formation in the rock pool ciliate Strombidium oculatum Gruber (Ciliophora, Oligotrichida): a description of the functional biology and an analysis of the tidal synchronization of encystment. J. Exp. Mar. Biol. Ecol. 175, 77–103. doi: 10.1016/0022-0981(94)90177-5
Kahan, D. (1972). Cyclidium citrullus Cohn, a ciliate from the hot springs of Tiberias (Israel). J. Protozool. 19, 593–597. doi: 10.1111/j.1550-7408.1972.tb03536.x
Kamiyama, T. (1994). Effects of extracellular products from phytoplankton on the excystment of tintinnids from marine sediments. Mar. Ecol. Prog. Ser. 105, 199–201. doi: 10.3354/meps105199
Kamiyama, T. (1997). Effects of phytoplankton abundance on excystment of tintinnid ciliates from marine sediments. J. Oceanogr. 53, 299–302.
Kamiyama, T., Itakura, S., and Nagasaki, K. (1995). Effects of irradiance on excystment of tintinnids from marine sediments. J. Oceanogr. 51, 615–618. doi: 10.1007/BF02270527
Kamra, K., and Sapra, G. R. (1991). Formation of a transient corticotype during excystment in Coniculostomum monilata (Oxytrichidae, Hypotrichida) and its modification by reorganizations. Eur. J. Protistol. 27, 331–342. doi: 10.1016/S0932-4739(11)80249-X
Kim, Y. O., Suzuki, T., and Taniguchi, A. (2002). A new species in the genus Cyrtostrombidium (Ciliophora, Oligotrichia, Oligotrichida): its morphology, seasonal cycle and resting stage. J. Eukaryot. Microbiol. 49, 338–343. doi: 10.1111/j.1550-7408.2002.tb00380.x
Kim, Y. O., and Taniguchi, A. (1995). Excystment of the oligotrich ciliate Strombidium conicum. Aquat. Microb. Ecol. 9, 149–156. doi: 10.3354/ame009149
Krause, M., and Braucker, R. (2009). Gravitaxis of Bursaria truncatella: electrophysiological and behavioural analyses of a large ciliate cell. Eur. J. Protistol. 45, 98–111. doi: 10.1016/j.ejop.2008.09.001
Li, Q., Sun, Q., Fan, X., Wu, N., Ni, B., and Gu, F. (2017). The differentiation of cellular structure during encystment in the soil hypotrichous ciliate Australocirrus cf. australis (Protista, Ciliophora). Anim. Cells Syst. 21, 45–52. doi: 10.1080/19768354.2016.1262896
Liu, Z., Li, Y., Li, J., and Gu, F. (2009). Some ultrastructural observations of the vegetative, resting and excysting ciliate, Urostyla grandis (urostylidae, hypotrichida). Biol. Res. 42, 395–401. doi: 10.4067/S0716-97602009000400001
Lynn, D. H. (2008). The Ciliated Protozoa: Characterization, Classification, and Guide to the Literature. Berlin: Springer.
Ma, M., Xu, Y., Yan, Y., Li, Y., Warren, A., and Song, W. (2020). Taxonomy and molecular information of four karyorelictids in genera Apotrachelocerca and Tracheloraphis (Protozoa, Ciliophora), with notes on their systematic positions and description of two new species. Zool. J. Linn. Soc. 192, 690–709. doi: 10.1093/zoolinnean/zlaa109
Manwell, R. D. (1928). Conjugation, division, and encystment in Pleurotricha lanceolata. Biol. Bull. 54, 417–463.
Martín-González, A., Palacios, G., and Gutiérrez, J. C. (2001). Macronuclear chromatin changes during encystment in the ciliate Colpoda inflata: formation of crystal-like structures in the resting cyst chromatin and nucleolar condensation. Eur. J. Protistol. 37, 121–136. doi: 10.1078/0932-4739-00796
Matsuoka, K., Funadani, R., and Matsuoka, T. (2017). Tolerance of Colpoda cucullus resting cysts to ultraviolet irradiation. J. Protozool. Res. 27, 1–7.
Matsuoka, T. (2013). Evaluation of intracellular Ca2+ concentration by fura 2 ratiometry in encystment-induced Colpoda cucullus. Acta Protozool. 52, 51–54. doi: 10.4467/16890027AP.13.005.0833
Matsuoka, T. (2021). Early signaling pathways mediating dormant cyst formation in terrestrial unicellular eukaryote Colpoda. FEMS Microbiol. Lett. 368:fnab019. doi: 10.1093/femsle/fnab019
Matsuoka, T., Kondoh, A., Sabashi, K., Nagano, N., Akematsu, T., Kida, A., et al. (2009). Role of Ca2+ and cAMP in a cell signaling pathway for resting cyst formation of ciliated protozoan Colpoda cucullus. Protistology 6, 103–110.
McArdle, E. W., Bergquist, B. L., and Ehret, C. F. (1980). Structural changes in Tetrahymena rostrata during induced encystment. J. Protozool. 27, 388–397. doi: 10.1111/j.1550-7408.1980.tb05382.x
Montagnes, D. J. S., Lowe, C., Poulton, A., and Jonsson, P. R. (2002). Redescription of Strombidium oculatum Gruber 1884 (Ciliophora, Oligotrichia). J. Eukaryot. Microbiol. 49, 329–337. doi: 10.1111/j.1550-7408.2002.tb00379.x
Mulisch, M., and Hausmann, K. (1989). Localization of chitin on ultrathin sections of cysts of two ciliated protozoa, Blepharisma undulans and Pseudomicrothorax dubius, using colloidal gold conjugated wheat germ agglutinin. Protoplasma 152, 77–86. doi: 10.1007/BF01323065
Müller, H. (1996). Encystment of the freshwater ciliate Pelagostrombidium fallax (Ciliophora, Oligotrichida) in laboratory culture. Aquat. Microb. Ecol. 11, 289–295. doi: 10.3354/ame011289
Müller, H. (2002). Laboratory study of the life cycle of a freshwater strombidiid ciliate. Aquat. Microb. Ecol. 29, 189–197. doi: 10.3354/ame029189
Müller, H. (2007). Live observation of excystment in the spirotrich ciliate Meseres corlissi. Eur. J. Protistol. 43, 95–100. doi: 10.1016/j.ejop.2006.11.003
Müller, H., and Wünsch, C. (1999). Seasonal dynamics of cyst formation of pelagic strombidiid ciliates in a deep prealpine lake. Aquat. Microb. Ecol. 17, 37–47. doi: 10.3354/ame017037
Olendzenski, L. C. (1999). Growth, fine structure and cyst formation of a microbial mat ciliate: Pseudocohnilembus pusillus (Ciliophora, scuticociliatida). J. Eukaryot. Microbiol. 46, 132–141. doi: 10.1111/j.1550-7408.1999.tb04596.x
Pan, N., Niu, T., Bhatti, M. Z., Zhang, H., Fan, X., Ni, B., et al. (2019). Novel insights into molecular mechanisms of Pseudourostyla cristata encystment using comparative transcriptomics. Sci. Rep. 9:19109. doi: 10.1038/S41598-019-55608-7
Petz, W., and Foissner, W. (1992). Morphology and morphogenesis of Strobilidium caudatum (Fromentel), Meseres corlissi n. sp., Halteria grandinella (Müller), and Strombidium rehwaldi n. sp., and a proposed phylogenetic system for oligotrich ciliates (Protozoa, Ciliophora). J. Protozool. 39, 159–176. doi: 10.1111/j.1550-7408.1992.tb01296.x
Popenko, V. I., Cherny, N. E., Ivanova, J. L., and Yakovleva, M. G. (1998). Ultrastructure of the macronucleus in the resting cysts of the ciliate Bursaria truncatella. Eur. J. Protistol. 34, 18–28. doi: 10.1016/S0932-4739(98)80036-9
Prescott, D. M. (1994). The DNA of ciliated protozoa. Microbiol. Rev. 58, 233–267. doi: 10.1128/Mmbr.58.2.233-267.1994
Rawlinson, N. G., and Gates, M. A. (1985). The excystment process in the ciliate Euplotes muscicola: an integrated light and scanning electron microscopic study. J. Protozool. 32, 729–735. doi: 10.1111/j.1550-7408.1985.tb03109.x
Reid, P. C., and John, A. W. G. (1983). Resting cysts in the ciliate class Polyhymenophorea: phylogenetic implications. J. Protozool. 30, 710–713. doi: 10.1111/j.1550-7408.1983.tb05348.x
Repak, A. J. (1968). Encystment and excystment of the heterotrichous ciliate Blepharisma stoltei Isquith. J. Protozool. 15, 407–412. doi: 10.1111/j.1550-7408.1968.tb02148.x
Repak, A. J., and Pfister, R. M. (1967). Electron microscopical observations on the extracellular structures of the resting cyst of Blepharisma stoltei. Trans. Amer. Micros. Soc. 86, 417–421. doi: 10.2307/3224264
Ricci, N., Verni, F., and Rosati, G. (1985). The cyst of Oxytricha bifaria (Ciliata: Hypotrichida). I. Morphology and significance. Trans. Am. Micros. Soc. 104, 70–78. doi: 10.2307/3226358
Rosati, G., Verni, F., and Nieri, L. (1983). Investigation of the cyst wall of the hypotrich ciliate Gastrostyla steini Engelmann. Monitore Zool. Ital. 17, 19–26. doi: 10.1080/00269786.1983.10736413
Segade, P., Garcia, N., Garcia Estevez, J. M., Arias, C., and Iglesias, R. (2016). Encystment/excystment response and serotypic variation in the gastropod parasite Tetrahymena rostrata (Ciliophora, Tetrahymenidae). Parasitol. Res. 115, 771–777. doi: 10.1007/s00436-015-4802-7
Sheng, Y., Duan, L., Cheng, T., Qiao, Y., Stover, N. A., and Gao, S. (2020). The completed macronuclear genome of a model ciliate Tetrahymena thermophila and its application in genome scrambling and copy number analyses. Sci. China Life Sci. 63, 1534–1542. doi: 10.1007/s11427-020-1689-4
Shimada, Y., Hasegawa, Y., Harada, Y., Nakamura, R., Matsuoka, T., and Arikawa, M. (2021). Signaling in temperature-induced resting cyst formation in the ciliated protozoan Colpoda cucullus. Eur. J. Protistol. 79:125800. doi: 10.1016/j.ejop.2021.125800
Sogame, Y., Kida, A., and Matsuoka, T. (2011). Possible involvement of endocyst in tolerance of the resting cyst of Colpoda cucullus against HCl. Afr. J. Microbiol. Res. 5, 4316–4320. doi: 10.5897/AJMR11.190
Sogame, Y., Kojima, K., Takeshita, T., Fujiwara, S., Miyata, S., Kinoshita, E., et al. (2012a). Protein phosphorylation in encystment-induced Colpoda cucullus: localization and identification of phosphoproteins. FEMS Microbiol. Lett. 331, 128–135. doi: 10.1111/j.1574-6968.2012.02560.x
Sogame, Y., Kojima, K., Takeshita, T., Kinoshita, E., Funadani, R., and Matsuoka, T. (2013). Excystment-dependent alteration of protein expression in terrestrial ciliate Colpoda cucullus. Microbes Environ. 28, 388–390. doi: 10.1264/jsme2.me12200
Sogame, Y., Kojima, K., Takeshita, T., Kinoshita, E., and Matsuoka, T. (2012b). EF-1α and mitochondrial ATP synthase β chain: alteration of their expression in encystment-induced Colpoda cucullus. J. Eukaryot. Microbiol. 59, 401–406. doi: 10.1111/j.1550-7408.2012.00628.x
Sogame, Y., Kojima, K., Takeshita, T., Kinoshita, E., and Matsuoka, T. (2014). Identification of cAMP-dependent phosphorytlated proteins involved in the formation of environment-resistant resting cysts by the terrestrial ciliate Colpoda cucullus. Inv. Surv. J. 11, 213–218.
Sogame, Y., Saito, R., Koizumi, R., Shimizu, T., and Ono, T. (2019). Evidence of stress recovery in free-living ciliate Colpoda cucullus: the repair capability of resting cysts to damage caused by gamma irradiation. Acta Protozool. 58, 25–29. doi: 10.4467/16890027AP.19.006.10837
Song, W. (1993). Studies on the cortical morphogenesis during cell division in Halteria grandinella (Müller, 1773)(Ciliophora, Oligotrichida). Chin. J. Oceanol. Limnol. 11, 122–129. doi: 10.1007/BF02850818
Stout, J. D. (1954). The ecology, life history and parasitism of Tetrahymena [Paraglaucoma] rostrata (Kahl) Corliss. J. Protozool. 1, 211–215. doi: 10.1111/j.1550-7408.1954.tb00819.x
Tibbs, J. (1968). Fine structure of Colpoda steinii during encystment and excystment. J. Protozool. 15, 725–732. doi: 10.1111/j.1550-7408.1968.tb02202.x
Tibbs, J., and Marshall, B. J. (1969). Electron transport and phospholipase activity in encysting cells of the ciliate Colpoda steinii. Biochim. Biophys. Acta 172, 382–388. doi: 10.1016/0005-2728(69)90134-0
Uspenskaya, Z. I., and Lozina-Lozinsky, L. K. (1979). Antigen rearrangements in Colpoda maupasi cells after freezing at -196 °C, and after shortwave ultraviolet irradiation. Cryobiology 16, 542–549. doi: 10.1016/0011-2240(79)90072-5
van Wagtendonk, W. J. (1955). “Encystment and excystment of protozoa,” in Biochemistry and Physiology of Protozoa 2, eds S. H. Hutner and A. Lwoff (New York: Academic Press), 85–90.
Verni, F., and Rosati, G. (2011). Resting cysts: a survival strategy in Protozoa Ciliophora. Ital. J. Zool. 78, 134–145. doi: 10.1080/11250003.2011.560579
Verni, F., Rosati, G., and Ricci, N. (1984). The cyst of Oxytricha bifaria (Ciliata Hypotrichida). II: the ultrastructure. Protistologica 20, 87–95.
Vickerman, K. (1960). Structural changes in mitochondria of Acanthamoeba at encystation. Nature 188, 248–249. doi: 10.1038/188248a0
Villalobo, E., Moch, C., Fryd-Versavel, G., Fleury-Aubusson, A., and Morin, L. (2003). Cysteine proteases and cell differentiation: excystment of the ciliated protist Sterkiella histriomuscorum. Eukaryot. Cell 2, 1234–1245. doi: 10.1128/EC.2.6.1234-1245.2003
Voß, H.-J. (1997). Morphology and morphogenesis of Parentocirrus hortualis nov. gen., nov. spec: a new genus within the redefined family Kahliellidae sensu Eigner 1995 (Ciliophora, Hypotrichida). Eur. J. Protistol. 33, 30–47. doi: 10.1016/S0932-4739(97)80019-3
Walker, G. K., and Maugel, T. K. (1980). Encystment and excystment in hypotrich ciliates II. Diophrys scutum and remarks on comparative features. Protistologica 16, 525–531.
Walker, G. K., Maugel, T. K., and Goode, D. (1975). Some ultrastructural observations on encystment in Stylonychia mytilus (Ciliophora: Hypotrichida). Trans. Am. Micros. Soc. 94, 147–154. doi: 10.2307/3225545
Wang, B., Niu, T., Bhatti, M. Z., Chen, F., Wu, L., and Chen, J. (2017). Identification of cyst wall proteins of the hypotrich ciliate Euplotes encysticus using a proteomics approach. J. Microbiol. 55, 545–553. doi: 10.1007/s12275-017-6422-7
Wang, C., Gao, Y., Lu, B., Chi, Y., Zhang, T., El-Serehy, H. A., et al. (2021). Large-scale phylogenomic analysis provides new insights into the phylogeny of the class Oligohymenophorea (Protista, Ciliophora) with establishment of a new subclass Urocentria nov. subcl. Mol. Phylogenet. Evol. 159:107112. doi: 10.1016/J.Ympev.2021.107112
Wang, C., Yan, Y., Chen, X., Al-Farraj, S. A., El-Serehy, H. A., and Gao, F. (2019). Further analyses on the evolutionary “key-protist” Halteria (Protista, Ciliophora) based on transcriptomic data. Zool. Scr. 48, 813–825. doi: 10.1111/zsc.12380
Wang, J., Li, L., Warren, A., and Shao, C. (2017). Morphogenesis and molecular phylogeny of the soil ciliate Rigidohymena quadrinucleata (Dragesco and Njine, 1971) Berger, 2011 (Ciliophora, Hypotricha, Oxytrichidae). Eur. J. Protistol. 60, 1–12. doi: 10.1016/j.ejop.2017.04.006
Wang, J., Zhang, T., Li, F., Warren, A., Li, Y., and Shao, C. (2021). A new hypotrich ciliate, Oxytricha xianica sp. nov., with notes on the morphology and phylogeny of a Chinese population of Oxytricha auripunctata Blatterer & Foissner, 1988 (Ciliophora, Oxytrichidae). Mar. Life Sci. Technol. 3, 303–312. doi: 10.1007/s42995-020-00089-1
Wirnsberger, E., Foissner, W., and Adam, H. (1985). Morphological, biometric, and morphogenetic comparison of two closely related species, Stylonychia vorax and S. pustulata (Ciliophora: Oxytrichidae). J. Protozool. 32, 261–268. doi: 10.1111/j.1550-7408.1985.tb03048.x
Wu, T., Li, Y., Lu, B., Shen, Z., Song, W., and Warren, A. (2020). Morphology, taxonomy and molecular phylogeny of three marine peritrich ciliates, including two new species: Zoothamnium apoarbuscula n. sp. and Z. apohentscheli n. sp.(Protozoa, Ciliophora, Peritrichia). Mar. Life Sci. Technol. 2, 334–348. doi: 10.1007/s42995-020-00046-y
Wu, Y., Ji, L., and Gu, F. (2004). Advances in the study on resting cells in Protozoa (in Chinese). Chin. J. Zool. 39, 91–95.
Xu, R., Li, J., and Bai, Q. (2007). Advances in the studies on cysts of protozoans (in Chinese). Acta Sci. Nat. Univ. Sunyatseni 46, 64–69.
Yamaoka, M., Watoh, T., and Matsuoka, T. (2004). Effects of salt concentration and bacteria on encystment induction in ciliated protozoan Colpoda sp. Acta Protozool. 43, 93–98.
Zebrun, W., Corliss, J. O., and Lom, J. (1967). Electron microscopical observations on the mucocysts of the ciliate Tetrahymena rostrata. Trans. Am. Micros. Soc. 86, 28–36. doi: 10.2307/3224421
Zhang, T., Dong, J., Cheng, T., Duan, L., and Shao, C. (2020). Reconsideration of the taxonomy of the marine ciliate Neobakuella aenigmatica Moon et al., 2019 (Protozoa, Ciliophora, Hypotrichia). Mar. Life Sci. Technol. 2, 97–108. doi: 10.1007/s42995-020-00032-4
Zhang, Z., and Pang, Y. (1981). A study of the process of encystment and excystment of Stylonychia mytilus (in Chinese). J. East China Norm. Univ. 2, 113–119.
Zhao, L., Gao, F., Gao, S., Liang, Y., Long, H., Lv, Z., et al. (2021). Biodiversity-based development and evolution: the emerging research systems in model and non-model organisms. Sci. China Life Sci. 64, 1236–1280. doi: 10.1007/s11427-020-1915-y
Zhao, L., Li, Y., Li, J., and Gu, F. (2009). Some ultrastructural observations of the vegetative, resting and excysting ciliate, Urostyla grandis (Urostylidae, Hypotrichida). Biol. Res. 42, 395–401. doi: 10.4067/S0716-97602009000400001
Keywords: resting cyst, bionomic strategy, structure, factors, molecular mechanism
Citation: Li Y, Wang Y, Zhang S, Maurer-Alcalá XX and Yan Y (2022) How Ciliated Protists Survive by Cysts: Some Key Points During Encystment and Excystment. Front. Microbiol. 13:785502. doi: 10.3389/fmicb.2022.785502
Received: 29 September 2021; Accepted: 11 January 2022;
Published: 17 February 2022.
Edited by:
Weiwei Liu, South China Sea Institute of Oceanology (SCSIO), Chinese Academy of Sciences (CAS), ChinaCopyright © 2022 Li, Wang, Zhang, Maurer-Alcalá and Yan. This is an open-access article distributed under the terms of the Creative Commons Attribution License (CC BY). The use, distribution or reproduction in other forums is permitted, provided the original author(s) and the copyright owner(s) are credited and that the original publication in this journal is cited, in accordance with accepted academic practice. No use, distribution or reproduction is permitted which does not comply with these terms.
*Correspondence: Ying Yan, eWFueWluZ0BvdWMuZWR1LmNu